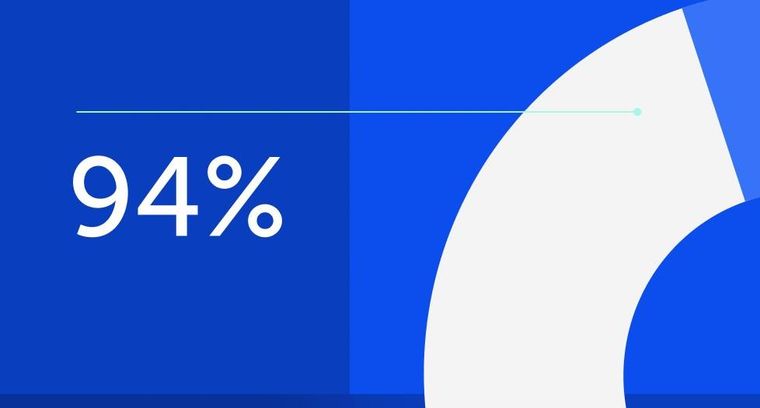
94% of researchers rate our articles as excellent or good
Learn more about the work of our research integrity team to safeguard the quality of each article we publish.
Find out more
EDITORIAL article
Front. Phys., 13 December 2022
Sec. Soft Matter Physics
Volume 10 - 2022 | https://doi.org/10.3389/fphy.2022.1111099
This article is part of the Research TopicExploring the Mechanical Properties of Soft Materials at Multiple Length ScalesView all 5 articles
Editorial on the Research Topic
Exploring the mechanical properties of soft materials at multiple length scales
Colloidal assemblies, biopolymer networks, membranes, and many other soft materials present interactions and structural arrangements developed over a broad range of length scales from the nano/microscale for basic constituents to the macroscopic scale for bulk material. This complexity is reflected in their mechanical response at different times and frequencies, resulting in different behaviors at multiple length scales. Measuring and understanding materials’ multi-scale mechanical response is of pivotal importance to design and control their final applications, which span the fields of biotechnology, medicine, building materials, food science, 3D printing, and many others. However, this remains a difficult task, and the development of advanced methodologies using experimental, theoretical, and/or computational approaches is required as illustrated in the articles composing this Research Topic.
For biopolymer networks, Gurmessa et al. show that a combination of microfluidics, microrheology using optical tweezers, and structural investigation through confocal microscopy, is needed to reveal and understand the viscoelastic properties of actin networks. In particular, the evolution of the viscoelasticity of networks that are crosslinked with myosin II minifilaments is studied as a function of the salinity of a buffer containing Mg2+ and K+. The use of a microfluidic perfusion chamber allows the simultaneous cycling of Mg2+ and K+ concentrations in the buffer while measuring the time-dependent viscoelasticity by means of microrheology with optical tweezers. By cycling from low to high salinity conditions, the authors show an interesting, non-monotonic dependence of the solutions’ viscoelastic response. This could be linked to actin bundling, assisted by Mg2+, during the initial increase of salt content, as well as successive network disruption promoted by slower depolymerization triggered by K+. These results and future work exploring different types of salts and their concentrations, as well as different combinations of actin and myosin, will contribute to a better understanding of the complex mechanics of the cell cytoskeleton, of which, semiflexible actin filament networks are key components.
Computational approaches are also fundamental to explore and predict the viscoelastic response of such filamentous biomaterials, complementing experimental investigations. The mini review by Rizzi presents an overview of the state-of-the-art of these approaches and highlights future challenges and perspectives. Since polymer solutions and networks present structures and dynamics that span several length scales, a combination of different simulation techniques and theoretical approaches is needed to fully predict their mechanical response. These include atomistic simulations and coarse-grained methods, with the latter used in most cases, even in the description of self-assembly of filaments. Different degrees of coarse graining allow simulations of the response of dilute, concentrated, and entangled solutions, as well as cross-linked networks, providing interesting information on the effects of various parameters such as flexibility, concentration, crosslink density, and connectivity (the last two concerning networks). One of the main limitations of these approaches is the need for large-scale simulations and the necessity to use an optimum level of coarse graining in the system.
For determining the mechanical response of soft materials at different length scales, it is fundamental to understand the length-scale–dependent microscopic dynamics of the material’s constituents. Differential dynamic microscopy (DDM) is a technique that can provide such information through the measurement of the wave vector–dependent intermediate scattering function. This technique is advantageous because it can be implemented in a conventional microscopy setup, exploiting the many contrast techniques available. Brizioli et al. apply this technique to investigate the Brownian yet non-gaussian diffusion (BNG) of tracer particles in a glassy amorphous matrix; an experimental condition that is characteristic of microrheological measurements. It must be noted that, due to the small size of the tracers, conventional particle tracking analyses cannot be performed in this case. The use, for the first time, of a reciprocal space approach to probe BNG allows testing and validation of the recent predictions of diffusing diffusivity theories, which associate the temporal variation of particle diffusivity to motion in heterogenous environments that exhibit different physical properties. In the studied system, such variations occur on a time scale that corresponds to the time needed to explore a number of voids within the glassy matrix. This DDM approach could be extended to the investigation of tracer dynamics in sheared materials or filamentous networks, such as those considered in the previously discussed works.
In biological systems, the mechanical properties of objects or assemblies at the mesoscale, such as cells, model membranes, and tissues, are of special interest. Specialized microscale techniques have been developed to measure these properties, including atomic force microscopy (AFM) micromechanics, micropipettes, and microfluidic constrictions. In the work by Layachi et al., a microfluidic approach (enabling micropipette aspiration with simultaneous imaging) is applied to measure the rheological response of vesicle prototissues. Such biomimetic models, thanks to their reduced complexity with respect to cellular tissues, are powerful systems to enable understanding of intricate biological processes. A combination of time-resolved fluorescence microscopy and particle image velocimetry is used to obtain information, both at the global scale of the entire prototissue, or at the local scale of several vesicles. The prototissues present a viscoelastic behavior that can be described using a generalized Kelvin-Voigt model, with parameters that do not significantly vary between different levels of vesicle adhesion. Local analysis using particle image velocimetry reveals that increasing pressure leads to compaction and a decrease of the permeability of the prototissues. These could be associated, determined by additional image analysis, to reorganization events between several vesicles. The proposed methodology therefore allows a detailed and complete description of the mechanical response of vesicle prototissues, and it is envisaged that such a methodology could be applied in the future to other multicellular structures, thus providing further insights into their complex mechanical response.
The works collected in this Research Topic provide excellent examples of the wealth of information that can be acquired on the links between the structure, dynamics, and mechanical response of soft materials when investigating systems at multiple length scales utilizing a combination of advanced experimental, theoretical, and computational techniques.
ML wrote the first draft of the manuscript. All authors contributed to revision, read, and approved the submitted version.
The authors declare that the research was conducted in the absence of any commercial or financial relationships that could be construed as a potential conflict of interest.
All claims expressed in this article are solely those of the authors and do not necessarily represent those of their affiliated organizations, or those of the publisher, the editors and the reviewers. Any product that may be evaluated in this article, or claim that may be made by its manufacturer, is not guaranteed or endorsed by the publisher.
Keywords: mechanics, soft materials, colloidal assemblies, biopolymer networks, vesicles
Citation: Laurati M, Tassieri M, Espinosa G, Oliveira MSN and Joseph P (2022) Editorial: Exploring the mechanical properties of soft materials at multiple length scales. Front. Phys. 10:1111099. doi: 10.3389/fphy.2022.1111099
Received: 29 November 2022; Accepted: 30 November 2022;
Published: 13 December 2022.
Edited and reviewed by:
Jasper Van Der Gucht, Wageningen University and Research, NetherlandsCopyright © 2022 Laurati, Tassieri, Espinosa, Oliveira and Joseph. This is an open-access article distributed under the terms of the Creative Commons Attribution License (CC BY). The use, distribution or reproduction in other forums is permitted, provided the original author(s) and the copyright owner(s) are credited and that the original publication in this journal is cited, in accordance with accepted academic practice. No use, distribution or reproduction is permitted which does not comply with these terms.
*Correspondence: Marco Laurati, bWFyY28ubGF1cmF0aUB1bmlmaS5pdA==
Disclaimer: All claims expressed in this article are solely those of the authors and do not necessarily represent those of their affiliated organizations, or those of the publisher, the editors and the reviewers. Any product that may be evaluated in this article or claim that may be made by its manufacturer is not guaranteed or endorsed by the publisher.
Research integrity at Frontiers
Learn more about the work of our research integrity team to safeguard the quality of each article we publish.