- 1Research Center for Optoelectronic Materials and Devices, School of Physical Science Technology, Guangxi University, Nanning, China
- 2College of Mathematics and Physics, Beijing University of Chemical Technology, Beijing, China
- 3School of Electronics and Information, Nantong University, Nantong, China
- 4State Key Laboratory of Chemical Resource Engineering, Beijing University of Chemical Technology, Beijing, China
- 5Guangxi Key Laboratory of Processing for Non-ferrous Metal and Featured Materials, Guangxi University, Nanning, China
The high-quality semi-polar (11-22) AlN thin films were grown on m-plane sapphire substrates by hydride vapor phase epitaxy (HVPE). The surface morphology and crystalline quality of the AlN film were greatly influenced by the growth temperature and the substrate miscut angle. As the temperature increased, the grain size on the surface increased and the grain density decreased. In addition, the higher growth temperature also resulted in smaller values of the full width at half maximum (FWHM) of X-ray rocking curves (XRC) when temperature was more than 1,460 °C. At high temperature of 1,530 °C, the introduction of 1° -off miscut angle to the substrate resulted in smooth surface, low density of stacking faults and low FWHM of XRC. The misfit dislocation density was calculated from the tilt angle of epilayer measured by X-ray reciprocal space mappings along [−1−123] AlN. The misfit dislocation density of the sample grown on 1° -off substrates was 6.7 × 105 cm−2. The improvement of crystal quality is believed to be due to the enhancement of adatom mobility at higher temperatures and also the appropriate miscut variation.
Introduction
Recently, research on semi-polar III-nitrides materials and devices, mainly GaN and AlN, has attracted much attention due to the greatly weakened Quantum Confined Stark Effect (QCSE) of optoelectronic devices based on the semi-polar nitrides [1–4]. High-quality semi-polar GaN and AlN are typically grown on homoepitaxial substrates cut from thick bulk crystals, but this approach is limited by small size and high cost [5–7]. To resolve this issue, semi-polar GaN is directly grown on a foreign substrate, such as m-plane sapphire or trenches in c-plane sapphire with trenches [6, 8]. In contrast, it is difficult to obtain semi-polar AlN on foreign substrates with high crystal quality and flat surface because of the large lattice mismatch, thermal mismatch, and anisotropy [9, 10]. The flat surface morphology is extremely important for the application of AlN since high-quality optoelectronic devices must be fabricated on atomic scale AlN substrate [11–13]. In general, controlling the strain of epilayer during growth is an effective way to obtain high-quality epitaxial films with flat surface. The strain relaxation mechanism in AlN films is related to the specific slip systems of the wurtzite structure [14]. The driving force for the tilt formation and glide of misfit dislocation (MD) is caused by an active <11-20>-slip system [7]. For c-plane AlN films, it is well known that the misorientation caused by miscut sapphire substrates strongly affects the crystallization quality and surface morphology of the epitaxial layers [11]. However, there are few studies on the effect of growth temperature and substrate misorientation of semi-polar (11-22) on the quality of AlN, in which the threading dislocations (TDs) present on the (0001) slip plane bend and slip to release the mismatch stress [15].
In this paper, semi-polar (11-22) AlN films were grown on m-plane (10-10) sapphire by hydride vapor phase epitaxy (HVPE). We investigated the influence of growth temperature and substrate misorientation on the stress release of semi-polar (11-22) AlN. The epitaxial layer stress relaxation was found to be associated with the tilt of the (11-22) AlN films along the [-1-123] AlN. At high temperature of 1,530°C, the introduction of 1 off miscut angle to the substrate resulted in smooth surface, low density of stacking faults and low FWHM of XRC. Combining temperature and appropriate miscut variation is an effective method to rapidly obtain high-quality (11-22) AlN thick film.
Materials and methods
The semi-polar (11-22) AlN was grown on home-made horizontal HVPE. Two kinds of m-plane sapphire were used as substrate. The miscut angle of the m-plane sapphire substrate tilts towards the (10-12) plane along the [0001]. HCl and ammonia were used as input active gases. Before AlN deposition, HCl flowed over Al source to form gaseous aluminum chlorides at 550°C. The pressure was kept at 10 Torr during the growth. At first, m-plane sapphire substrate is heated to nitridation temperature in the carrier gas and kept for 10 min in H2 ambient to remove the surface oxide and achieve thermal stability. Next, sapphire nitridation is performed between 1,200°C and 1,500°C for 10 min under the ambient of NH3 (0.5 SLM), followed by the growth of 80 nm buffer layer at the nitridation temperature. Then the sapphire substrate is heated to 1,500°C and a thickness of 2.9 µm AlN film is grown with a V/III ratio of 30. A summary of growth conditions for four samples are listed in Table 1.
High-resolution X-ray diffraction (HRXRD) were performed on a PANalytical X'Pert3 MRD diffractometer system, using four-crystal Ge (220) as the monochromator. The X-ray source was Cukα1 with a wavelength of 0.1540598 nm. HRXRD was used to measure the degree of tilt by (11-22) AlN mapping and the tilt of the epilayer lattice for heteroepitaxial (11-22) AlN films on [(10-10) sapphire substrates. The anisotropy of the tilt was observed by X-ray mapping paralleled to the orthogonal in-plane directions. The scanning electron microscope (SEM) was used to observe the surface morphology of AlN films.
Results and discussion
Figure 1 shows the symmetric XRD 2θ-ω scans of all AlN samples, where the diffraction peaks at 71.25° and 68.2° correspond to AlN (11-22) and sapphire (30-30), respectively. All samples are verified to be (11-22) single-phase since only one AlN diffraction peak is observed. Besides, the heteroepitaxial relationship between AlN and sapphire is determined as (11-22) AlN//(30-30) sapphire. Moreover, the in-plane crystal orientation relationship between AlN and sapphire is [1-100] AlN//[11-20] sapphire and [-1-123] AlN//[0001] sapphire, which is in consistent with the previously reported (11-22) AlN epilayers grown by plasma-assisted molecular-beam epitaxy and metal organic vapor phase deposition [16, 17]. From samples A, B and C, it is found that the FWHM of AlN (11-22) diffraction peak decreases and the peak intensity increases with the increasing growth temperature, which indicates the high growth temperature helps to improve the crystal quality of the (11-22) AlN film.
In addition to the crystal quality, the surface morphology of these semi polar AlN films is also obviously different. As shown in Figures 2, 3D islands are prevalent on the surface of samples A, B and C, resulting in a pronounced rough surface of the (11-22) AlN film. Interestingly, with the temperature increasing from 1,380 C to 1,530 C, the surface of the (11-22) AlN film could not merge, while the grain size gradually increases. This phenomenon can be explained that the migration capability of Al atom is sensitive to the temperature. It is well known that the bond energy of Al–N bond (2.88 eV) is much larger comparing to Ga-N (2.2 eV) and In-N (1.93 eV) bonds, leading to a smaller surface mobility and shorter diffusion length of Al atom [18]. As the growth temperature increases from 1,380 C to 1,530 C, the enhanced Al atom mobility promotes the growth of grains, so the grain size increases and the grain density decreases obviously as shown in Figures 2A–C and Table 1. However, increasing the growth temperature does not make (11-22) AlN step flow growth. Even at the high temperature of 1,530°C (11-22), AlN surface still does not merge. To promote AlN surface merging, a 1° -off m-plane sapphire substrate is introduced to solve this problem. As illustrated in Figure 2D, the surface of the semi-polar (11-22) AlN films becomes smooth when using high growth temperature of 1,530°C and 1° -off miscut substrate simultaneously. Under these conditions, the FWHM of (11-22) AlN XRC is further reduced, indicating that the crystal quality is further improved, as shown in Figure 3.
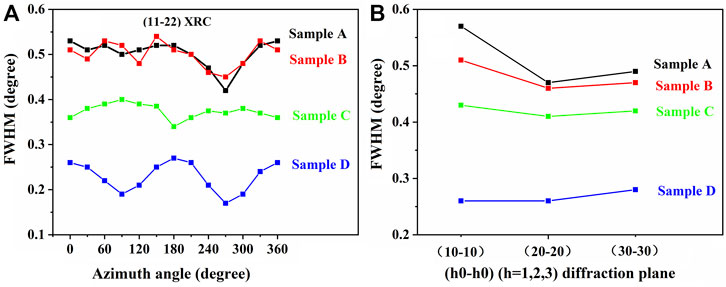
FIGURE 3. (A) FWHM of AlN [1–12] rocking curves as a function of azimuth angle, and (B) the FWHM of AlN [13], [10] and (30-30) rocking curves.
X-ray rocking curve (XRC) measurements are performed on all samples to characterize the crystal quality of semi-polar (11-22) AlN films. HRXRD is used to measure the FWHM of symmetric and skew symmetric crystallographic plane caused by dislocations, which can be used to calculate the density of dislocations (11-22), and we utilized the modified-Williamson-Hall plot to linearly fit the (h0-h0) XRD data, from the intercept, the stacking fault could be calculated [23]. Figure 3A shows the FWHM of symmetric scan AlN (11-22) XRD as a function of azimuth angle. When the growth temperature below 1,460 C for samples grown on substrate without miscut angle, the FWHM values almost unchanged along all the azimuth angles. However, when the temperature increases to be 1,530°C, the crystal quality is improved significantly. Sample D has the smallest values at all azimuth angles, which indicates the substrate with a miscut angle of 1 is indeed beneficial to improve the crystal quality of epilayers. The skew symmetric scans of AlN (10-10), (20-20), and (30-30) XRD are conducted to evaluate the basal stacking-faults in semi-polar (11-22) AlN as shown in Figure 3B. Obviously, as the growth temperature increases, the FWHM values decrease for all three diffractions as the growth temperature increase, which indicates that the increase of growth temperature can decrease the density of stacking faults. Sample D has the smallest FWHM values for all three skew symmetric scans, which indicates the miscut angle of the substrate benefits for the decrease of basal stacking faults.
Superficially, the growth temperature and substrate off-angle improve (11-22) AlN crystal quality and surface morphology. Essentially, these changes are due to the modulation of the stress release of the AlN epitaxial layer by the growth temperature and substrate off-angle. In order to explore the mechanism of stress release, we measured the HRXRD reciprocal space maps (RSMs) using the triple-axis diffractometer. The RSMs are obtained by taking a series of ω–2θ scans at successive ω values and presenting the results in the 2D plane. The intensity shown in RSMs is normally a projection of the ω scan spots intensity onto a 2D plane. Figure 4 shows the symmetric (11-22) AlN RSMs along the [1-123] AlN direction. The specific direction of RSMs could directly test the interplanar tilt between the epitaxial layer and substrate in the same direction displaying by the tilt angle. The tilt angle is shown in Figure 4D, which is the angle between (30-30) sapphire to Origin of reciprocal space (0, 0) and (11-22) AlN to Origin of reciprocal space (0, 0). In wurtzite nitrides, there is a relationship between epitaxial tilt and dislocation density. Therefore, it is necessary to regulate stress during early growth. Through Figure 4, both growth temperature and substrate miscut are found to influence the epitaxial tilt angle, the results shown in Figure 5.
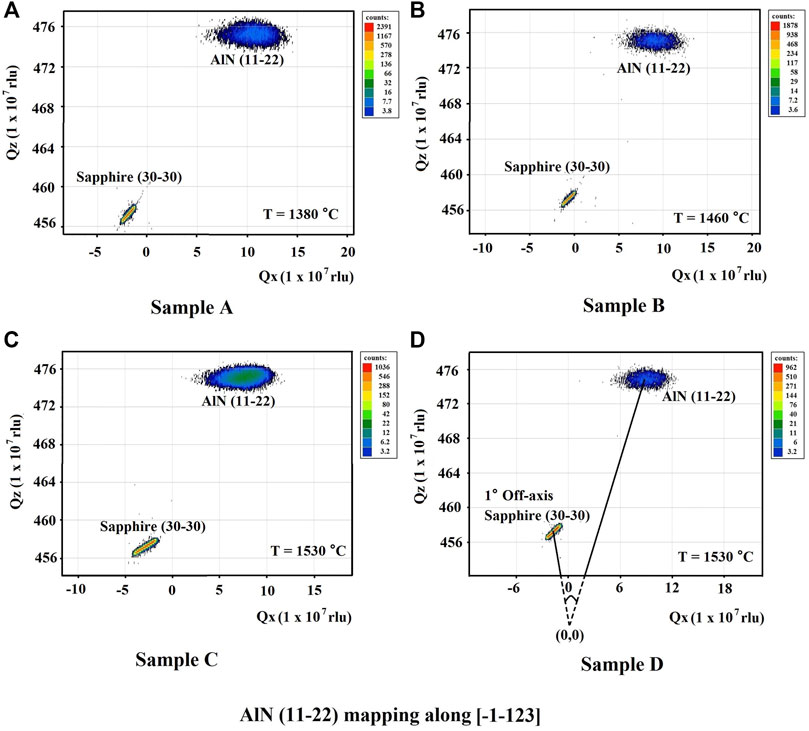
FIGURE 4. X-ray reciprocal space mappings along [−1−123] AlN for (A) Sample A, (B) Sample B, (C) Sample C, and (D) Sample D.
Figure 6 shows the schematic of MD configuration in semi-polar (11-22) AlN films. In wurtzite nitrides, the basal {0001, <11-20>} is the main slip system [7, 14], thereby the MD in semi-polar nitrides are formed by the glide of threading dislocations in semi-polar nitrides. In semi-polar (11-22) AlN grown on m-plane sapphire, the lattice mismatch along the in-plane [1-100] AlN is 13.28%, while it is -9.61% along the [-1-123] [24]. As a result (11-22), AlN film are in compressive strain along the in-plane [1-100] and in tensile strain along [-1-123]. Therefore, a large number of MD are formed due to the large lattice mismatch between the (11-22) AlN film and the m-plane sapphire substrate. Comparing to the c-plane of wurtzite nitrides, the shear stress exhibits an in-plane anisotropic distribution on the semi-polar plane. Specifically, along the [-1-123] AlN direction and the [1-100] AlN direction, the shear stress are maximum and 0, respectively. Therefore, epitaxial tilt occurs along the in-plane [-1-123] AlN direction, and the Burgers vector b of MD is a/3 < 11-20>. According to the formula of tilt [7]:
where α is tilt angle,
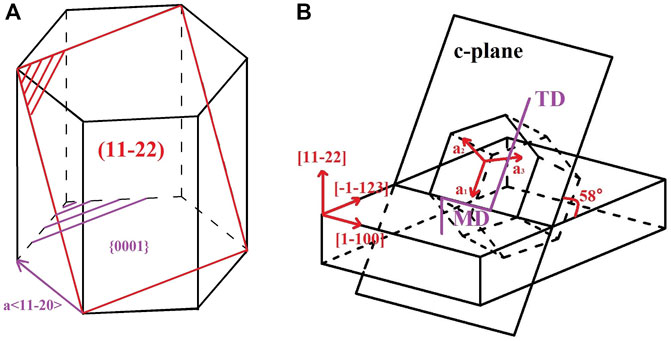
FIGURE 6. Schematic of misfit dislocation configuration in semi-polar (11-22) AlN films. (A) is cubic chart; (B) is cross-section drawn.
Conclusion
In summary, single-phase high-quality semi-polar (11-22) AlN films were grown on m-plane sapphire substrates with different miscut angles by HVPE. The semi-polar (11-22) AlN films have the best crystal quality, corresponding to a temperature of 1530 °C and 1° -off m-plane sapphire substrate. The surface morphology and dislocation density of semi-polar (11-22) AlN films are significantly influenced by the vicinal angles of m-plane sapphire substrates. We found that a proper MD density (6.5 × 105 cm-2) contributes to coalescence of (11-22) AlN grains. Combining temperature and appropriate miscut variation is an effective method to rapidly obtain high-quality (11-22) AlN thick film.
Data availability statement
The original contributions presented in the study are included in the article/supplementary material, further inquiries can be directed to the corresponding authors.
Author contributions
SM carried out the experiments and wrote the manuscript. LT revised the manuscript and design part of the experiments. LY, TS, and LX contributed to the discussion. ZJ and SW contributed to the concept, design of the experiments. All authors contributed to manuscript revision, read and approved the submitted version.
Funding
This work was partly supported by the Key-area research and development program of Guangdong Province (2020B010172001), the National Natural Science Foundation of China (61874007, 12074028, 52102152, 62074087), the Shandong Provincial Major Scientific and Technological Innovation Project (2019JZZY010209), the funding (No.T3120099202, T3120097921, and C31200992004) for the talent of Guangxi province, Guangxi Science and Technology Base and Talented Special project (No.AD20238088) and talent model base (No. AE31200065), China.
Conflict of interest
The authors declare that the research was conducted in the absence of any commercial or financial relationships that could be construed as a potential conflict of interest.
Publisher’s note
All claims expressed in this article are solely those of the authors and do not necessarily represent those of their affiliated organizations, or those of the publisher, the editors and the reviewers. Any product that may be evaluated in this article, or claim that may be made by its manufacturer, is not guaranteed or endorsed by the publisher.
References
1. Sun W. H, Yang J. W, Chen C. Q, Zhang J. P, Gaevski M. E, Kuokstis E, et al. Gan/Algan multiple quantum wells on a-plane Gan pillars for stripe-geometry nonpolar ultraviolet light-emitting devices. Appl Phys Lett (2003) 83(13):2599–601. doi:10.1063/1.1614835
2. Khan A, Balakrishnan K, Katona T. Ultraviolet light-emitting diodes based on group three nitrides. Nat Photon (2008) 2:77–84. doi:10.1038/nphoton.2007.293
3. Balakrishnan K, Adivarahan V, Fareed Q, Lachab M, Zhang B, Khan A. First demonstration of semipolar deep ultraviolet light emitting diode on M-plane sapphire with algan multiple quantum wells. Jpn J Appl Phys (2010) 49(4):040206. doi:10.1143/jjap.49.040206
4. Lu S, Zhang Y, Qiu Y, Liu X, Zhang M, Luo D. Efficiency boosting by thermal harvesting in ingan/Gan light-emitting diodes. Front Phys (2021) 9:9. doi:10.3389/fphy.2021.752476
5. Liu N, Jiang Y, Xiao J, Liang Z, Wang Q, Zhang G. Fabrication of 2-inch free-standing Gan substrate on sapphire with a combined buffer layer by hvpe. Front Chem (2021) 9:671720. . doi:10.3389/fchem.2021.671720
6. Ichikawa S, Funato M, Kawakami Y. Metalorganic vapor phase epitaxy of pit-free aln homoepitaxial films on various semipolar substrates. J Cryst Growth (2019) 522:68–77. doi:10.1016/j.jcrysgro.2019.06.010
7. Xing K, Tseng C, Wang L, Chi P, Wang J, Chen P, et al. Semi-polar (11-22) Gan epitaxial films with significantly reduced defect densities grown on M-plane sapphire using a sequence of two in situ sinx interlayers. Appl Phys Lett (2019) 114(13):131105. doi:10.1063/1.5085012
8. Tyagi A, Wu F, Young E. C, Chakraborty A, Ohta H, Bhat R, et al. Partial strain relaxation via misfit dislocation generation at heterointerfaces in (Al, In)GaN epitaxial layers grown on semipolar (112¯2) GaN free standing substrates. Appl Phys Lett (2009) 95(25):251905. Artn 251905. doi:10.1063/1.3275717
9. Monavarian M, Rashidi A, Feezell D. A decade of nonpolar and semipolar iii-nitrides: A Review of successes and challenges. Phys Status Solidi A (2019)(1) 1800628. doi:10.1002/pssa.201800628
11. Mao Song S, Li J. F., Zhang J. C., Sun W. H. The fabrication of aln by hydride vapor phase epitaxy. J Semicond (2019) 40(12):121803. doi:10.1088/1674-4926/40/12/121803
12. Sun M. S., Zhang J. C., Huang J., Li X. W., Wang L. J., Liu X. H., et al. Influence of thickness on strain state and surface morphology of aln grown by hvpe. J Semicond (2016) 37(12):123001. . doi:10.1088/1674-4926/37/12/123001
13. Zhang J. C, Zhu Y. H, Egawa T, Sumiya S, Miyoshi M, Tanaka M. Quantum-well and localized state emissions in alingan deep ultraviolet light-emitting diodes. Appl Phys Lett (2007) 91(22):221906. . doi:10.1063/1.2817947
14. Srinivasan S, Geng L, Liu R, Ponce FA, Narukawa Y, Tanaka S. Slip systems and misfit dislocations in ingan epilayers. Appl Phys Lett (2003) 83(25):5187–9. doi:10.1063/1.1633029
15. Hsu P. S, Young EC, Romanov A. E, Fujito K, DenBaars S. P, Nakamura S, et al. Misfit dislocation formation via pre-existing threading dislocation glide in (112¯2) semipolar heteroepitaxy. Appl Phys Lett (2011) 99(8):081912. . doi:10.1063/1.3628459
16. Stellmach J, Frentrup M, Mehnke F, Pristovsek M, Wernicke T, Kneissl M. MOVPE growth of semipolar AlN on m-plane sapphire. J Cryst Growth (2012) 355(1):59–62. doi:10.1016/j.jcrysgro.2012.06.047
17. Lahourcade L, Bellet-Amalric E, Monroy E, Abouzaid M, Ruterana P. Plasma-assisted molecular-beam epitaxy of aln(112¯2) on M sapphire. Appl Phys Lett (2007) 90(13):131909. doi:10.1063/1.2716375
18. Keller S, DenBaars S. P. Metalorganic chemical vapor deposition of group iii nitrides - a discussion of critical issues. J Cryst Growth (2003) 248:479–86. doi:10.1016/s0022-0248(02)01867-5
19. Metzger T, Hopler R, Born E, Ambacher O, Stutzmann M, Stommer R, et al. Defect structure of epitaxial Gan films determined by transmission electron microscopy and triple-Axis X-ray diffractometry. Philosophical Mag A (1998) 77(4):1013–25. doi:10.1080/01418619808221225
20. Chierchia R, Bottcher T, Heinke H, Einfeldt S, Figge S, Hommel D. Microstructure of heteroepitaxial Gan revealed by X-ray diffraction. J Appl Phys (2003) 93(11):8918–25. doi:10.1063/1.1571217
21. Sun X, Li D, Chen Y, Song H, Jiang H, Li Z, et al. In situ observation of two-step growth of aln on sapphire using high-temperature metal–organic chemical vapour deposition. Cryst Eng Comm (2013) 15(30):6066. doi:10.1039/c3ce40755a
22. Ben J, Sun X, Jia Y, Jiang K, Shi Z, Liu H, et al. Defect evolution in aln templates on pvd-aln/sapphire substrates by thermal annealing. Cryst Eng Comm (2018) 20(32):4623–9. doi:10.1039/c8ce00770e
10. Paskova T. Development and prospects of nitride materials and devices with nonpolar surfaces. Phys Stat Sol (2008) 245(b):1011–25. doi:10.1002/pssb.200743274
23. McLaurin M. B, Hirai A, Young E, Wu F, Speck J. S. Basal plane stacking-fault related anisotropy in X-ray rocking curve widths of M-plane Gan. Jpn J Appl Phys (2008) 47(7):5429–31. doi:10.1143/Jjap.47.5429
Keywords: AlN, Semi-Polar, HVPE, temperature, miscut angle
Citation: Maosong S, Ting L, Yong L, Shuxin T, Xu L, Jicai Z and Wenhong S (2022) Influence of growth temperature and miscut angle of m-plane sapphire substrate on the semi-polar (11–22) AlN film grown by HVPE. Front. Phys. 10:1076895. doi: 10.3389/fphy.2022.1076895
Received: 22 October 2022; Accepted: 03 November 2022;
Published: 23 November 2022.
Edited by:
Chenguang He, Guangdong Academy of Sciences, ChinaCopyright © 2022 Maosong, Ting, Yong, Shuxin, Xu, Jicai and Wenhong. This is an open-access article distributed under the terms of the Creative Commons Attribution License (CC BY). The use, distribution or reproduction in other forums is permitted, provided the original author(s) and the copyright owner(s) are credited and that the original publication in this journal is cited, in accordance with accepted academic practice. No use, distribution or reproduction is permitted which does not comply with these terms.
*Correspondence: Liu Ting, bGl1dGluZzIwMjFAYnVjdC5lZHUuY24=; Zhang Jicai, amN6aGFuZ0BtYWlsLmJ1Y3QuZWR1LmNu; Sun Wenhong, MjAxODAwMDFAZ3h1LmVkdS5jbg==