- 1School of Optical and Electronic Information and Wuhan National Laboratory for Optoelectronics, Huazhong University of Science and Technology, Wuhan, China
- 2Max-Planck Institute of Quantum Optics, Garching, Germany
- 3School of Optics and Photonics, Beijing Institute of Technology, Beijing, China
- 4GBA Branch of Aerospace Information Research Institute, Chinese Academy of Sciences, Guangzhou, China
High-repetition-rate ultrafast laser oscillators with high average power and short pulse duration provide excellent sources for generating optical frequency combs. Here we report a Kerr-lens mode locked Yb:YAG thin-disk oscillator delivering 203-MHz pulses at an average power of 9.4 W. A single additional nonlinear plate was inserted inside the cavity to enhance the Kerr lens effect, which leads to a substantial broadening of the mode-locked spectrum. The resultant pulse duration is 55 fs. The demonstrated oscillator combines a high repetition rate, a high average power and short pulse duration within one resonator, offering an ideal prerequisite for the optical-frequency metrology and frequency-comb spectroscopy with high signal-to-noise ratio.
Introduction
Ultrafast laser oscillators with high average power and short pulse duration are always pursued due to their numerous applications in many fields, such as spectroscopy [1, 2], cell applications [3], trace gas analysis [4], biological imaging [5]. Furthermore, such oscillators with high repetition rate are excellent laser sources for generating optical frequency combs. The increased power per mode under the condition of high repetition rate can improve the signal-to-noise ratio in optical-frequency metrology and frequency-comb spectroscopy [6]. Until now numerous works have been done to increase the performance of the laser oscillators towards higher average power, shorter pulse duration and higher repetition rate. Based on fiber oscillators, the repetition rate of the generated pulses can reach up to GHz, and the pulse duration can be shortened down to ∼50 fs. Based on fiber oscillators, the repetition rate of the generated pulses can reach up to multi-GHz [7, 8], and the pulse duration can be shortened down to ∼30 fs [9]. However, it is difficult to achieve sub 50-fs laser pulses with a high repetition rate at the same time. Moreover, the average power of the traditional ultrafast fiber oscillators is limited to milliwatts level due to the strong nonlinear effect in the fibers. Although the average power could be boosted based on large-pitch fiber oscillators, the repetition rate was restricted (<100 MHz) by the fiber length [10]. For ultrafast bulk-crystal laser oscillators, substantial progress has been made in promoting one or two of those three aspects [11–16], However, it is rather difficult to combine all these desired properties within one oscillator, limited by several reasons such as thermal effects of the bulk crystals, the narrow bandwidth of the gain medium and the mode locking condition and so on.
Compared to the fiber and bulk-crystal mode-locked oscillators, thin-disk technology has shown great advantage in scaling the average power and pulse energy since its invention in 1994 [17]. Pulses with average powers of several hundreds of watts and pulse energies of several tens of microjoules have been achieved directly from the Yb:YAG thin-disk oscillators with either Kerr lens mode locking (KLM) or semiconductor saturable absorber mirrors (SESAM) [18–21]. Based on the scheme of strong self-phase modulation, the pulses delivered from Yb:YAG thin-disk oscillators can be shortened with pulse durations down to ∼50 fs while maintaining a high average power level [22, 23]. To obtain short-duration pulses from a thin-disk oscillator, new materials with broader spectrum bandwidth than Yb:YAG were also used as thin-disk gain media such as Yb:Lu2O3, Yb:LuScO3 and Yb:CAlGO [24–26], resulting in the generation of sub 100-fs pulses with average powers up to tens of watts. In addition, with Ho:YAG thin disks, passive mode locking at the wavelength of 2.1 μm was realized first by KLM and then followed by SESAM mode locking, which delivered femtosecond pulses with average powers of about several tens of watts [27–29].
In terms of repetition rate, most of the thin-disk oscillators operated at a repetition rate below 100 MHz due to the conflicting requirements between the short cavity length and the large laser beam size. One solution is to generate mode-locked pulses inside a ring cavity, however, it still confronts the same problem mentioned above when further increase of the repetition rate up to more than 200 MHz is required. Another solution utilized asymmetric cavity configuration through two concave mirrors with different radii of curvature (ROC) in a standing-wave cavity, which reconciled the inconvenience of arrangement due to the short cavity length and the large beam size on the thin disk. This scheme has resulted in pulses generation with pulse duration around 250 fs and average powers of 75 W at a repetition rate up to 200 and 260 MHz from Yb:YAG thin-disk oscillators [30], showing great potential in generating high-repetition-rate, high-power frequency combs. Based on these results, a new scheme named distributed kerr-lens mode locking (DKLM) was invented to enhance the Kerr-lens effect, which greatly broadened the output spectrum of the Yb:YAG thin-disk oscillators and shortened the pulse duration down to sub 50 fs [31]. However, the average power was limited to around 4 W, partly because of the high reflection loss from the surfaces of multiple nonlinear Kerr plates. In this work, we optimize the DKLM scheme by replacing the separate Kerr plates with a single plate possessing high nonlinear coefficient. This significantly decreases the cavity loss and results in a combination of a high repetition rate (>200 MHz), high average power (∼10 W) and short pulse duration (∼50 fs) within one oscillator.
Experimental setup
The experimental setup is shown in Figure 1. The Yb:YAG thin disk is ∼100 μm thick and placed inside a 36-pass pump module used as one of the folding mirrors in a Z-shaped cavity. It is pumped by a fiber-coupled diode laser at 940 nm with a pump beam size on the disk of 2.5 mm in diameter. We designed and built the cavity with a telescope section consisting of two concave mirrors with different radii of curvature (150 and 50 mm). This asymmetric configuration enables a larger mode size in the cavity arm containing the Yb:YAG disk (Arm 2). The dispersive mirrors are also placed in this arm in order to avoid damage caused by the high intensities during the mode locking start-up. In contrast, the other arm (Arm 1) has a much smaller beam size with a diameter on the OC of 160 μm. The intracavity mode distribution is illustrated in Figure 2. It can be found that the beam is well collimated in Arm 2, indicating an almost same beam size (2.2 mm) on the end HR mirror and the disk. The whole cavity length was around 740 mm, corresponding to a repetition rate of 203 MHz. A 2-mm thick sapphire plate is placed in the focus of the telescope section as the Kerr medium, which provides the necessary self-focusing effect and self-amplitude modulation assisted with a hard aperture for the initiation of the Kerr lens mode locking. To enhance the Kerr-lens effect, a piece of TiO2 crystal is inserted in the Arm 1 at the Brewster angle close to the OC. A pair of high-dispersion mirrors were used to provide a negative round-trip group-delay-dispersion of −2000 fs2.
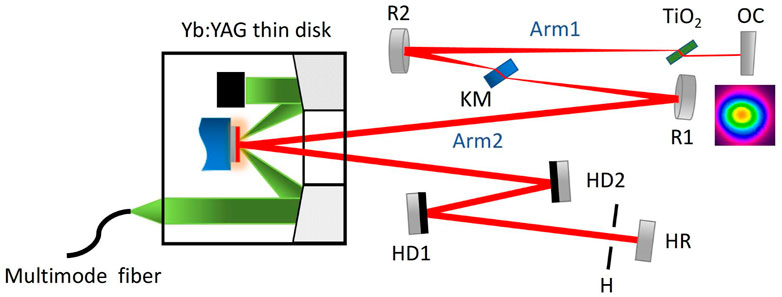
FIGURE 1. Schematic of the Yb:YAG thin-disk oscillator. The Yb:YAG thin disk is wedged with a ROC of −20 m. HR, high-reflection mirror; HD, high-dispersion mirrors; OC, output coupler; KM, Kerr medium (sapphire plate); H, hard aperture; R1 and R2, concave mirrors with ROC of −150 and −50 mm, respectively.
Results and discussion
The experiment was carried out based on the DKLM concept, similar to that reported in our previous work [31]. In that work, we increased the overall modulation depth for the passive mode locker and enhanced the Kerr lens effect by distributing various Kerr lenses at proper locations inside the oscillator cavity. As a result, the output pulse durations from an Yb:YAG thin-disk oscillator were well below what the emission bandwidth limit (FWHM) of the gain medium can support. However, the decreased optical-to-optical efficiencies and average powers with more crystals inserted are related to the significant losses induced by the multiple Kerr plates. Since there were six uncoated plates inserted in the beam path (excluding the KM), the losses resulted from absorption and surface reflection cannot be ignored. The most direct way of decreasing these losses is to use single crystal instead of a group of them, and the total nonlinearity provided by single crystal and by a set of crystals should be equal. Therefore in this work, we optimized the DKLM scheme with this idea and chose a 0.5-mm thick TiO2 crystal (with the nonlinear refractive index of ≈5.6 × 10−15 cm2/W) which had a similar nonlinearity as the group of six Kerr plates (15-mm thick sapphire crystals with n2 ≈ 1.3 × 10−16 cm2/W and 3-mm thick YAG crystal with n2 ≈ 2.7 × 10−16 cm2/W) [32]. As a consequence, the output coupling ratio under the condition of the same intracavity additional nonlinearity could be increased from 3% to 5% due to the decreased losses. The mode locking was realized under a pump power of 180 W, and 55-fs pulses with an average power of 9.4 W could be obtained. The optical-to-optical efficiency is calculated as 5.2%, which is approximately 50% higher than the configuration with six plates in our previous work.
The measured spectrum was shown in Figure 3 (OSA, Ando AQ-6315A), which spans from 980 nm to 1,070 nm with a width of 24 nm at full width at half-maximum (FWHM). This is 2.5 times wider than the emission bandwidth of the Yb:YAG crystal (9 nm at FWHM). The small peak around 990 nm is speculated to come from the change of the dispersion from the HD mirrors. We used a home-built frequency-resolved optical gating (FROG) apparatus to further characterize the spectrum and the pulse duration of the output pulses, as shown in Figure 4. The retrieved temporal intensity shows a pulse duration of 55 fs. Figure 4C shows a good matching between the measured and the retrieved FROG traces, indicating that our retrieval is reliable. The repetition frequency of the oscillator was characterized with a radio frequency (RF) spectrum analyzer, as illustrated in Figure 5. It shows a high signal-to-noise ratio of 80 dB at a resolution bandwidth of 100 Hz (Agilent, E4447A), implying a stable mode-locked operation of the thin-disk oscillator. Once started, the KLM operation could be maintained for several hours under ambient air conditions. Stable operation for a whole day will be expected with a professionally designed housing. The output pulses have an excellent beam profile with the beam quality factor M2 less than 1.1 (Figure 1).
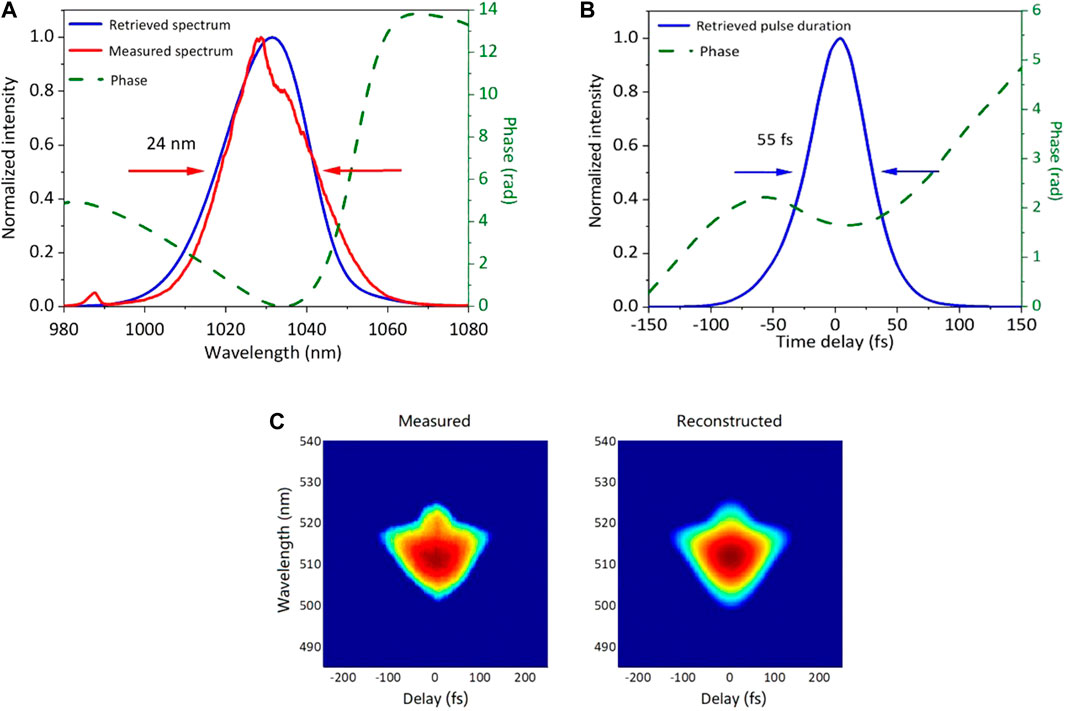
FIGURE 4. Characteristics of the output pulses. (A) Measured and retrieved spectra of the pulses. The measured spectrum extends from 980 to 1,080 nm. (B) Retrieved temporal intensity showing a pulse duration of 55 fs. (C) The comparison of the measured and retrieved FROG traces.
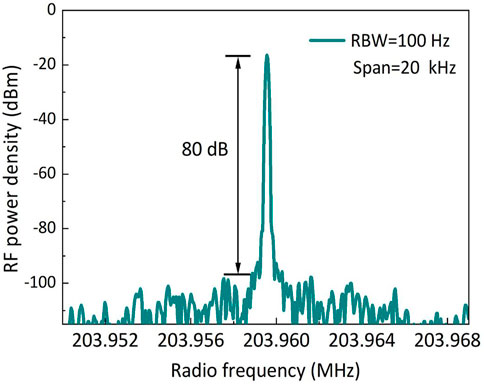
FIGURE 5. Fundamental RF spectrum measured at a resolution bandwidth of 100 Hz showing a signal-to-noise ratio of 80 dB.
For the next step, the improvement could be implemented by building a resonator with even stronger asymmetry, which will help to both enlarge the beam size on the thin disk for higher gain and higher output power and increase the repetition rate while preserving a large pump beam size on the disk. A single-pass configuration with the thin disk placed as an end mirror and a replacement of the concave mirrors by lenses are also beneficial for the cavity arrangement of higher repetition rate. Besides, the current TiO2 crystal is slightly wedged, resulting in residual reflection losses which can be reduced in the future. Higher output power could be expected with a plane-parallel crystal. To further shorten the pulse duration, a thicker TiO2 plate or a plate with higher nonlinear coefficient could be used to further enhance the Kerr lens effect. New thin-disk materials with broader emission spectrum bandwidth such as Yb:Lu2O3 or Yb:CALGO could also be applied with the same method, and new record of the pulse duration would be expected.
Conclusion
In conclusion, we have demonstrated a DKLM Yb:YAG thin-disk oscillator with a single TiO2 plate as the additional Kerr lens. This configuration enhanced the Kerr lens effect while greatly reducing the reflection losses inside the cavity, which enabled a higher output average power and optical-to-optical efficiency compared to our previous report. As a result, 9.4-W pulses with pulse duration of 55 fs at a repetition rate of 203 MHz were generated. Further improvement to the repetition rate, average power and pulse duration will be expected with stronger cavity asymmetry, new thin-disk materials and higher intracavity nonlinearity. The current oscillator combines a high repetition rate (>200 MHz), a high average power (∼10 W) and short pulse duration (∼50 fs) within one resonator, which provides a reliable laser source for the generation of high power optical frequency comb in the near infrared and even mid-infrared region.
Data availability statement
The raw data supporting the conclusion of this article will be made available by the authors, without undue reservation.
Author contributions
The main setup was designed and built by JZ. The data was measured, analyzed and interpreted by HL, TY, JX, QW, HX, and JZ. The experiment was conceived by JZ. All authors reviewed and contributed to the final manuscript.
Funding
This work is supported by the National Natural Science Foundation of China (No. 62075068), the International Science and Technology Cooperation Programme of Hubei Province (No. 2021EHB004) and the Research Project of Aerospace Information Research Institute, Chinese Academy of Sciences (E1Z1D101, E2Z2D101).
Conflict of interest
The authors declare that the research was conducted in the absence of any commercial or financial relationships that could be construed as a potential conflict of interest.
Publisher’s note
All claims expressed in this article are solely those of the authors and do not necessarily represent those of their affiliated organizations, or those of the publisher, the editors, and the reviewers. Any product that may be evaluated in this article, or claim that may be made by its manufacturer, is not guaranteed or endorsed by the publisher.
References
1. Picqué N, Hansch TW. Frequency-comb spectroscopy. Nat Photon (2019) 13:146–57. doi:10.1038/s41566-018-0347-5
2. Wang S, Guo H, Bai X, Zeng X. Broadband Kerr frequency combs and intracavity soliton dynamics influenced by high-order cavity dispersion. Opt Lett (2014) 39:2880–3. doi:10.1364/OL.39.002880
3. Xue B, Tamaruab Y, Fu Y, Yuan H, Lan P, Mücke OD, et al. A custom-tailored multi-TW optical electric field for gigawatt soft-x-ray isolated attosecond pulses. Ultrafast Sci (2021) 2021:1–13. doi:10.34133/2021/9828026
4. Modsching N, Drs J, Brochard P, Fischer J, Schilt S, Wittwer VJ, et al. High-power dual-comb thin-disk laser oscillator for fast high-resolution spectroscopy. Opt Express (2021) 29:15104–13. doi:10.1364/OE.424317
5. Zhao Y, Liang Y, Zhu X. Applications of scanning femtosecond laser-induced ionization microscopy in biological imaging. Front Optoelectron China (2008) 1:201–4. doi:10.1007/s12200-008-0041-1
6. Gaida C, Heuermann T, Gebhardt M, Shestaev E, Butler TP, Gerz D, et al. High-power frequency comb at 2 μm wavelength emitted by a Tm-doped fiber laser system. Opt Lett (2018) 43:5178–81. doi:10.1364/OL.43.005178
7. Cheng H, Wang W, Zhou Y, Qiao T, Lin W, Xu S, et al. 5 GHz fundamental repetition rate, wavelength tunable, all-fiber passively mode-locked Yb-fiber laser. Opt Express (2017) 25:27646–51. doi:10.1364/OE.25.027646
8. Li C, Ma Y, Gao X, Niu F, Jiang T, Wang A, et al. 1 GHz repetition rate femtosecond Yb:fiber laser for direct generation of carrier-envelope offset frequency. Appl Opt (2015) 54:8350–3. doi:10.1364/AO.54.008350
9. Zhou X, Yoshitomi D, Kobayashi Y, Torizuka K. Generation of 28-fs pulses from a mode-locked ytterbium fiber oscillator. Opt Express (2008) 6:7055–9. doi:10.1364/OE.16.007055
10. Baumgartl M, Lecaplain C, Hideur A, Limpert J, Tünnermann A. 66 W average power from a microjoule-class sub-100 fs fiber oscillator. Opt Lett (2012) 37:1640–2. doi:10.1364/OL.37.001640
11. Gao Z, Zhu J, Wang J, Wei Z, Xu X, Zheng L, et al. Generation of 33 fs pulses directly from a Kerr-lens mode-locked Yb:CaYAlO4 laser. Photon Res (2015) 3:335–8. doi:10.1364/PRJ.3.000335
12. Kimura S, Tani S, Kobayashi Y. Kerr-lens mode locking above a 20 GHz repetition rate. Optica (2019) 6:532–3. doi:10.1364/OPTICA.6.000532
13. Zheng L, Tian W, Liu H, Wang G, Bai C, Xu R, et al. 2 GHz watt-level Kerr-lens mode-locked Yb:KGW laser. Opt Express (2021) 29:12950–7. doi:10.1364/OE.424139
14. Yang J, Wang Z, Song J, Lv R, Wang X, Zhu J, et al. Diode-pumped 10 W femtosecond Yb:CALGO laser with high beam quality. High Pow Laser Sci Eng (2021) 9:e33–5. doi:10.1017/hpl.2021.19
15. Tian W, Xu R, Zheng L, Tian X, Zhang D, Xu X, et al. 10-W-scale Kerr-lens mode-locked Yb:CALYO laser with sub-100-fs pulses. Opt Lett (2021) 46:1297–300. doi:10.1364/OL.419370
16. Demirbas U, Thesinga J, Kellert M, Reuter S, Pergament M, Kärtner FX. Semiconductor saturable absorber mirror mode-locked Yb:YLF laser with pulses of 40 fs. Opt Lett (2022) 47:933–6. doi:10.1364/OL.450706
17. Giesen A, Hügel H, Voss A, Brauch U, Opower H. Scalable concept for diode-pumped high-power solid-state lasers. Appl Phys B (1994) 58:365–72. doi:10.1007/BF01081875
18. Brons J, Pervak V, Fedulova E, Bauer D, Sutter D, Kalashnikov V, et al. Energy scaling of Kerr-lens mode-locked thin-disk oscillators. Opt Lett (2014) 39:6442–5. doi:10.1364/OL.39.006442
19. Brons J, Pervak V, Bauer D, Sutter D, Pronin O, Krausz F. Powerful 100-fs-scale Kerr-lens mode-locked thin-disk oscillator. Opt Lett (2016) 41:3567–70. doi:10.1364/OL.41.003567
20. Saraceno CJ, Emaury F, Heckl HO, Bear CRE, Hoffmann M, Schriber C, et al. 275 W average output power from a femtosecond thin disk oscillator operated in a vacuum environment. Opt Express (2012) 20:23535–41. doi:10.1364/OE.20.023535
21. Saltarelli F, Graumann IJ, Bauer D, Phillips CR, Keller U. Power scaling of ultrafast oscillators: 350-W average-power sub-picosecond thin-disk laser. Opt Express (2019) 27:31465–74. doi:10.1364/OE.27.031465
22. Drs J, Fischer J, Modsching N, Labaye F, Wittwer VJ, Südmeyer T. Sub-30-fs Yb:YAG thin-disk laser oscillator operating in the strongly self-phase modulation broadened regime. Opt Express (2021) 29:35929–37. doi:10.1364/OE.440196
23. Fischer J, Drs J, Modsching N, Labaye F, Wittwer VJ, Südmeyer T. Efficient 100-MW, 100-W, 50-fs-class Yb:YAG thin-disk laser oscillator. Opt Express (2021) 29:42075–81. doi:10.1364/OE.442833
24. Saracenon CJ, Heckl OH, Baer CRE, Golling M, Beil K, Kränkel C, et al. Sub-100 femtosecond pulses from a SESAM mode-locked thin disk laser. Appl Phys B (2012) 106:559–62. doi:10.1007/s00340-012-4900-5
25. Modsching N, Paradis C, Labaye F, Gaponenko M, Graumann IJ, Diebold A, et al. Kerr lens mode-locked Yb:CALGO thin-disk laser. Opt Lett (2018) 43:879–82. doi:10.1364/OL.43.000879
26. Kitajima S, Shirakawa A, Yagi H, Yanagitani T. Sub-100 fs pulse generation from a Kerr-lens mode-locked Yb:Lu2O3 ceramic thin-disk laser. Opt Lett (2018) 43:5451–4. doi:10.1364/OL.43.005451
27. Zhang J, Mak KF, Pronin O. Kerr-Lens mode-Locked 2-μm thin-disk lasers. IEEE J Sel Top Quan Electron (2018) 24:1102101–11. doi:10.1109/JSTQE.2018.2814780
28. Tomilov S, Wang Y, Hoffmann M, Heidrich J, Golling M, Keller U, et al. 50-W average power Ho:YAG SESAM mode-locked thin-disk oscillator at 2.1 µm. Opt Express (2022) 30:27662–73. doi:10.1364/OE.460298
29. Zhang J, Mak KF, Nagl N, Seidel M, Bauer D, Pervak V, et al. Multi-mW, few-cycle mid-infrared continuum spanning from 500 to 2250 cm-1. Light Sci Appl (2018) 7:17180. doi:10.1038/lsa.2017.180
30. Zhang J, Brons J, Lilienfein N, Fedulova E, Pervak V, Bauer D, et al. 260-megahertz, megawatt-level thin-disk oscillator. Opt Lett (2015) 40:1627–30. doi:10.1364/OL.40.001627
31. Zhang J, Pӧtzlberger M, Wang Q, Seidel M, Bauer D, Sutter D, et al. Distributed kerr lens mode-Locked Yb:YAG thin-disk oscillator. Ultrafast Sci (2022) 2022:1–8. doi:10.34133/2022/9837892
Keywords: high repetition rate, high power, ultrashort pulses, thin disk, Yb:YAG
Citation: Liu H, Yang T, Xu J, Wang Q, Xuan H and Zhang J (2022) High-power 55-fs Yb:YAG thin-disk oscillator at 200 MHz repetition rate. Front. Phys. 10:1070826. doi: 10.3389/fphy.2022.1070826
Received: 15 October 2022; Accepted: 04 November 2022;
Published: 17 November 2022.
Edited by:
Guoqiang Xie, Shanghai Jiao Tong University, ChinaReviewed by:
Jie Ma, Jiangsu Normal University, ChinaYongguang Zhao, Jiangsu Normal University, China
Jiangfeng Zhu, Xidian University, China
Copyright © 2022 Liu, Yang, Xu, Wang, Xuan and Zhang. This is an open-access article distributed under the terms of the Creative Commons Attribution License (CC BY). The use, distribution or reproduction in other forums is permitted, provided the original author(s) and the copyright owner(s) are credited and that the original publication in this journal is cited, in accordance with accepted academic practice. No use, distribution or reproduction is permitted which does not comply with these terms.
*Correspondence: Hongwen Xuan, xuanhw@aircas.ac.cn; Jinwei Zhang, jinweizhang@hust.edu.cn