- School of Electronic and Information Engineering, Beihang University, Beijing, China
Single-cavity, dual-comb lasers are those specially designed mode-locked lasers that can emit more than one, asynchronous ultrashort pulse trains with stable repetition frequency difference between them. Unlike the long-studied, widely-used femtosecond lasers generating one stable pulse train, systematic investigation on them and their potential dual-comb applications only began, based on the fiber laser platform, around a decade ago, despite sporadic and limited reports of similar lasing phenomena since the beginning of the mode-locked laser studies. From a historic perspective, the birth of this novel technology is the lucky outcome of the timely collision of perpetual search for novel pulsing laser dynamics and concerted pursuit of open-minded solutions for out-of-lab dual-comb systems in the 2010s. In this review article, first, the current schemes to implement single-cavity dual optical frequency comb fiber lasers and their applications are summarized, based on the concept of multiplexed mode-locked lasers. The characteristics of reported single-cavity, dual-comb fiber lasers are discussed as well as their applications in spectroscopy, ranging, Terahertz (THz) spectroscopy, and asynchronous optical sampling (ASOPS). Finally, the more recent development of single-cavity, multi-comb lasers is presented.
1 Introduction
Optical frequency comb (OFC) has discrete and equally spaced ‘comb teeth’ in the optical frequency domain, while their tooth spacing falls into the microwave frequency range. Realized by femtosecond pulsed lasers with octave spanning spectra and precisely controlled carrier envelope offset frequency (fceo), the frequency stabilized OFC technology [1, 2] has found applications in many areas of high precision measurement. Dual optical frequency comb scheme was proposed to further simplify the system configuration for broadband measurement while retaining the advantages of OFC [3]. It uses two optical frequency combs with slightly different mode spacing. The coherent beating frequencies from the two combs are well within the radio-frequency range and can be easily picked up and processed by the existing electronic system. Thus, fast, large bandwidth and high resolution measurement can be realized with low-bandwidth electronics. Therefore, it was applied to time/frequency transferring [4], time synchronization [5], optical spectroscopy [6–8] and absolute-distance ranging [9]. It should be noted that, as the technology gained greater popularity, the concept of OFC had evolved into a broader one by covering other types of light sources, including microcavities and modulated continuous wave lasers, with sometimes limited bandwidth and less regulated optical frequency components. Also, along with the drastically growing interests in dual-comb techniques over the past decade, the focus of many studies had been shifted towards their applicability to the in-the-field applications and those that are less demanding in performance but more in system complexity. In spite of its great success in laboratory demonstrations, the complex and expensive phase-locked OFC laser systems, essential for the dual-comb techniques, has become a major obstacle for real-world applications. How to reduce the complexity of the dual-comb source while maintaining the mutual coherence of the dual combs had become one of the important issues for further development of the dual-comb technology [10].
Fiber mode-locked lasers are favored by many OFC systems due to its simple structure and low cost. In recent years, single-cavity dual-comb fiber laser technology that generates two OFC pulse trains at the same time has become a new type of low-complexity dual-comb light source. It could drastically reduce the complexity of a dual-comb source to that of a single fiber mode-locked laser, and thus has become a hotly investigated research topic.
2 Single-cavity dual-comb technology
The development of the optical frequency comb has been closely correlated with the evolution of ultrafast mode-locked laser technologies. During its early days in 1990s, the OFC source had been mostly built based on the then-already-mature solid-state lasers, such as Ti: sapphire lasers [2]. However, in the ensuing two decades, ultrafast fiber lasers have been growing at a fast pace with a number of innovations with the aim of improving mode-locking stability, pulse energy and pulse width/spectral bandwidth. They’d been quickly adopted as the mainstream platform for OFC generation with their OFC noise performance rivaling that from other lasers, because of their additional advantages in the system compactness, ruggedness, and optical-fiber-compatible spectral window. In the first decade into the 21st century, most dual-comb demonstration had been carried out by fiber-laser-based, frequency-stabilized OFC systems.
Unlike their solid-state counterparts that was once plagued by the unwanted bi-directional oscillation issue in their early stages of development [11], it had been much easier to control the light path for pulses in the passively mode-locked fiber lasers, thanks to the availability of a plethora of single-mode, fiber-optic components like isolators. In the 90s, the research community in general had its eyes set on bringing the output power, bandwidth and other characteristics of these lasers on par with those of others. Except for scant reports of peculiar dual-wavelength lasing observation [12–15], little attention had been given to the multi-pulse lasing in fiber lasers. A few studies with revived interests on bi-directional mode-locking in solid-state lasers had been carried out, targeting the laser gyroscope applications [16–18]. Additional attention had been diverted to more complex pulsing phenomena in the 00s, as the fiber laser technology matured and its studies became more widely spread. There had been several reports of picosecond, dual-wavelength pulse generation in the 1,560 nm spectral window from passively mode-locked Erbium fiber lasers [19–21]. It was later observed in 2009 that these pulses could have different periods due to dispersion from a normal dispersion laser [22]. In 2008, it was also demonstrated that bidirectional mode-locking can be realized in a ring fiber laser by removing the isolator and repetition rate difference on the order of tens of Hz was also observed [23].
Coincidentally, demonstrations of the dual-comb techniques, first proposed in 2002 [3], had been achieved for several important applications around the same period of time [6, 7, 9, 24]. Built on the momentum and following the same technical path of the hugely successful fully-frequency-stabilized OFC technology, they quickly garnered great attention as one of the most promising OFC techniques. Nevertheless, mutual coherence of two combs, instead of frequency stabilities of each comb, is now the most critical factor affecting the dual-comb performance, and this is readily ensured for the systems based on the traditional OFC sources. This minute yet important change could spur the dual-comb source technologies to a major shift in paradigm in realizing dual-comb light sources, in order to address growing concerns about the complexity challenges faced by dual-comb systems. Several alternative approaches, such as post compensation by digital processing [25] and electro-optic frequency combs [26], began to emerge.
Dual-wavelength generation of subpicosecond pulses with a stable repetition rate difference from an Erbium fiber laser was realized by tuning the spectral profile of the gain fiber and utilizing both the 1,530 and 1,560 nm windows in 2011 [27]. Realizing the potential to replace the sophisticated dual-comb sources at the time with such a simple free-running laser, the aforementioned laser was soon applied to demonstrations of asynchronous optical sampling (ASOPS) [28] and coherent-detection dual-comb ranging [29]. A universal approach to achieve stable asynchronous ultrashort pulse was proposed by introducing the concept of “multiplexing” into the field of ultrafast lasers [30]. In such a Multiplexed Mode-Locked Laser (M2L2), the cavity is designed to allow the simultaneous oscillation of pulses with different characteristics in one of the propagation dimensions. In the following years, numerous single-cavity dual-comb lasers and dual-comb systems based on them have been achieved, and so far they can still be categorized into the following four categories: wavelength-multiplexed, polarization-multiplexed [31], direction-multiplexed, and pulseshape-multiplexed [32] lasers corresponding to the four dimensions of light propagation, just as envisioned by the concept of M2L2 [30].
On the other hand, as the emergence of single-cavity dual-comb laser opens up a new possibility for realizing low-complexity dual-comb systems, it is still necessary to verify the applicability of such simple light source to dual-comb applications, due to their significantly different characteristics to those of standard comb sources. Along with the continuous improvement of the dual-comb fiber lasers over recent years, successful demonstrations in many important dual-comb applications have been carried out, as shown in Figure 1.
2.1 Single-cavity dual-comb fiber lasers
Multiplexing principle of single-cavity dual-comb lasers as shown in Figure 2. Simultaneous lasing at multiple wavelengths in the laser gain spectrum is often realized by introducing spectral filtering [27–29, 33–62]. This scheme, firstly applied to many continuous-wave lasers, has a long history, but little attention had been paid to the characteristics of the repetition frequencies of the output pulses [12, 13]. There had been conflicting reports of asynchronous or synchronous pulsing phenomena. On the other hand, when splitting gain spectrum into more than one sub-windows, the resulting mode-locked spectral bandwidth is often severely limited, and the long pulsewidth would render the output less useful for most dual-comb applications [19–21, 63]. The ability of controlling the gain spectrum shape and bandwidth [27] enables the generation of femtosecond pulses in dual-wavelength mode-locked fiber lasers. Due to the difference in cavity group velocity dispersion (GVD) at the wavelengths of the mode-locked pulses, the pulses would oscillate in the laser cavity asynchronously with slightly different repetition rate [22, 27]. The repetition frequency difference can then be designed by changing the intracavity dispersion. Various optical filtering schemes based on Lyot filtering [28, 29, 33–44, 46, 48, 56, 59, 60], Sagnac filtering [45, 47, 49, 50, 55], multimode interference [51, 52], spatial light filtering [53, 54, 62, 64] had been later applied to realize single-cavity dual-wavelength mode-locking. Studies on the self-starting process of the wavelength-multiplexed laser source [41, 51], their environmental stability [41, 46, 49, 54], tunability in the repetition frequency difference [47, 48] and pulse dynamics [57, 58, 61] has further carried out.
As to the spatial multiplexing scheme, for the fiber lasers constructed of single-mode fibers, propagation direction in the cavity would be the only possible variable to differentiate different pulses in this physical dimension. In the laser cavity based on the free-space optical path, the phenomenon of bidirectional simultaneous oscillation of pulses was found in early laser research [11, 65]. In order to suppress phenomena such as spatial hole burning, optical isolators had been routinely used to achieve unidirectional operation of laser in the cavity. An all-fiber bidirectional mode-locked laser based on carbon nanotube saturable absorber was realized by removing the intracavity optical isolator [23]. Later on, simultaneous mode-locking in the clockwise and counterclockwise directions in the ring cavity has become the common implementation of the bi-directional lasing [23, 30, 66–84]. Furthermore, in order to avoid the effects of competition between bidirectional pulses, there are also a variety of bidirectional fiber laser structures that share only part of the optical cavity [79, 83–86]. Unlike the wavelength-multiplexed cases, it is thought that the difference in repetition rates between the two pulse trains likely originates from direction-dependent birefringence, non-linear phase shift, and the GVD at different lasing wavelengths [78]. In a study on a bidirectional, dual-wavelength mode-locked laser, it is revealed that the seemingly different types of laser implementations, like the bidirectional or multi-wavelength ones, could be regarded as examples in different transmission dimensions of a family of M2L2. Furthermore, the idea of “multiplexing” was introduced into the design of a mode-locked laser cavity in order to realize asynchronous dual-comb generation [30]. The possibility of these multiplexed mode-locked laser as a dual-comb laser source was laid out as well [30]. Dual-comb spectroscopy measurements based on a bidirectional dual-comb laser were demonstrated [66], and further studies on directional multiplexed lasers in the 2 μm wavelength [67, 73], generation regimes of mode-locked [77, 82] and with high output power [72] had been carried out. Other designs adopting a partially shared-cavity structure to improve the self-starting and polarization-maintaining properties of the laser had also been explored [69, 70, 74, 87, 88], sometimes at the expense of mutual stabilities of the dual combs.
Polarization-multiplexed lasers are to generate two pulse trains with orthogonal polarization states from one laser cavity [31, 89–97]. Conventional fiber mode-locked laser structures are either composed entirely of single-mode fibers or polarization-maintaining fibers. Concerned about the bandwidth limiting effect that may be introduced by birefringence, the former hopes to keep the birefringence in the optical cavity as low as possible, while the latter is designed to maintain a single polarization state operation. After the concept of M2L2 was proposed, it is natural to look beyond the above two physical dimensions, and the scheme to realize polarization multiplexed fiber lasers was investigated. By introducing a short section of birefringent fiber into the cavity to break the degenerate transmission of polarization states in the cavity, it is demonstrated that two vector soliton pulse sequences with different repetition rates and orthogonal polarizations can be emitted stably [31]. The spectra of polarization-multiplexed mode-locked lasers overlap with each other fairly well, and can be directly applied to dual-comb measurements without non-linear spreading [89, 91]. Based on this concept, various studies, like dual-comb lasing utilizing residual birefringence in FP fiber cavities [98], polarization-multiplexed dissipative soliton generation [90], spectroscopy measurement applications using polarization-multiplexed fiber laser [92] had been carried out. Polarization-maintaining, polarization multiplexing schemes had been also developed based on partially shared-cavity structures [94, 99, 100].
Multiplexing based on temporal pulseshapes seems to be an even more daunting task. In order to enable pulses with distinct temporal profiles to mode-lock in the same cavity, unlike conventional lasers, multiple mechanisms that can contribute to the pulse shaping process need to be introduced. In a cavity with a physical saturable absorber, both polarization-dependent loss and birefringence can be introduced by using a polarizer with a polarization-maintaining fiber pigtail. In this way, the birefringence and polarization-dependent loss produce the Lyot filtering effect, which has a narrowing effect on the pulse bandwidth; on the other hand, the polarizer and the non-linear effect in the cavity can form the non-linear polarization rotation effect under sufficient pulse energy. It, combined with the physical mode-locking device, as hybrid mode-locking mechanism, can expand the pulse spectrum. Thereby, a pulse-energy-dependent, pulse shape/spectrum control scheme can be achieved. For the pulse with low pulse energy in the cavity, the linear filtering plays a more significant role, and the mode-locked spectrum is narrow. On the other hand, for the pulse with a sufficiently large pulse energy, the non-linear effect is more prominent, which significantly broadened its spectrum. The laser realized the simultaneous generation of ultrashort pulses with different energies and time-domain characteristics. Two output pulses have overlapped spectra, albeit with very different bandwidths, and slightly different repetition frequencies due to a small center wavelength offset. The beat signal of the dual-comb also proves that the two pulses have good mutual coherence [32].
Repetition frequency difference (Δf) and repetition frequency (f) are the keys in dual-comb application. Δf affects the sampling rate, and f/Δf is the scaling factor in the dual-comb application [10]. Figure 3 shows the relationship between f/Δf and Δf for various types of so-far-reported single-cavity dual-comb lasers with a completely shared cavity. Δf is determined by the cavity dispersion, either chromatic or polarization-dependent, based on the types of dual-comb lasers, and varying the cavity parameters, such as GVD, birefringence can, thus, change Δf of the laser output. Due to the relatively large GVD or birefringence one can apply to a cavity, the wavelength-multiplexed and polarization-multiplexed lasers can realize relatively large Δf, which is beneficial for those measurement applications requiring a higher update rate. On the other hand, Δf of the other two types of lasers are generally much lower, which gives them certain advantages in realizing larger f/Δf factors. A larger f/Δf factor, i.e. a larger down-conversion ratio could be beneficial for those applications with a limited radio frequency (RF) detection bandwidth while targeting a broader frequency range, like THz spectroscopy [38, 39]. For partially-shared cavities, on the other hand, tuning Δf can be done by directly adjusting the lengths of corresponding light paths.
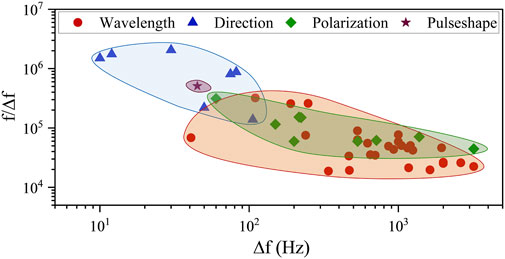
FIGURE 3. Relationship between f/Δf and Δf in the single-cavity dual-comb lasers with wavelength-multiplexed [27–29, 34–50, 53–56, 60, 61, 64], direction-multiplexed [24, 65, 67, 72, 75, 77, 80], polarization-multiplexed [31, 89–93, 95–97] and pulseshape-multiplexed [32] schemes.
The pulse energy is the another key parameter in many dual-comb applications. Figure 4 summarizes the reported performance of dual-comb fiber lasers from that perspective. It can be seen that the average output power for most of the single-cavity dual-comb lasers falls around 1 mW, with one exception of a normal-dispersion, directional-multiplexed with an about 70 mW output. This is mainly due to the limitation posed by the intracavity pulse interactions, and could be ameliorated by extra-cavity optical amplification at moderate costs of system complexity. Since this power level falls short of the requirements of many applications, there had been quite a few demonstrations of amplified dual-comb sources for optical spectroscopy [34, 35, 37] and THz spectroscopy [38, 39, 42] with sufficient mutual coherence and stability, despite the expected impact from the non-linear and spontaneous emission noise from the optical amplification scheme.
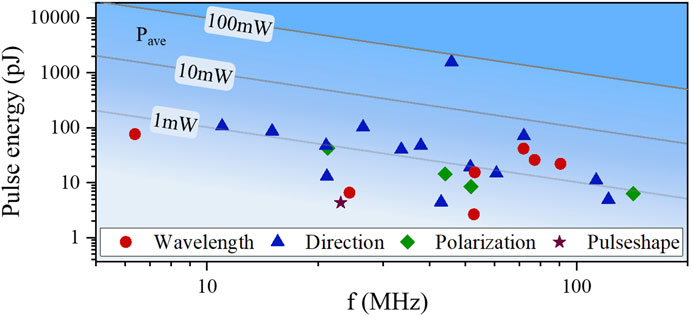
FIGURE 4. Relationship between pulse energy and f in the single-cavity dual-comb lasers with wavelength-multiplexed [34, 41, 45, 48, 54, 55], direction-multiplexed [24, 30, 65–68, 70–72, 74, 77, 81], polarization-multiplexed [90, 92, 94, 100] and pulseshape-multiplexed [32] schemes.
It is also noted that, since the demonstrations of the proposed single-cavity, dual-comb fiber lasers and their dual-comb applications, dual-comb lasers based on other laser platforms had also emerged. Bi-directional Ti: sapphire lasers [101–103], spatially-multiplexed thin-disk laser [104], polarization-multiplexed thin-disk lasers [105–110], polarization-multiplexed solid lasers [111–116] and even bi-directional-pumped microresonator [117] had been investigated. Compared to fiber lasers, some of them can achieve higher repetition frequency or higher output power, and they help to broaden the spectrum and scope of dual-comb laser technology.
2.2 Applications of single-cavity dual-comb lasers
The application schemes of single-cavity dual-comb lasers are shown in Figure 5, and can be roughly divided into two types: time-domain and frequency-domain measurements. The time-domain measurement includes ASOPS and ranging, and the frequency-domain measurement includes THz spectrum and optical spectrum measurements.
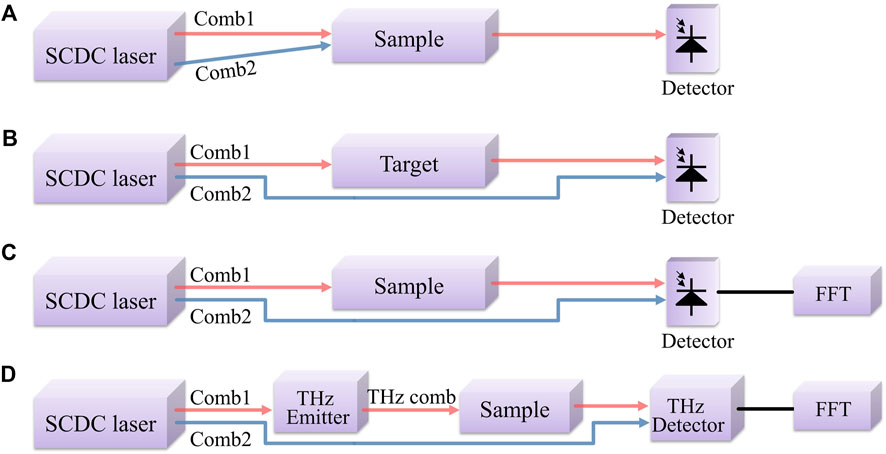
FIGURE 5. Applications of a single-cavity dual-comb (SCDC) laser. (A) ASOPS; (B) Ranging; (C) Optical spectroscopy; (D) THz spectroscopy.
ASOPS pump-probe application was the first demonstration realized by a single-cavity dual-comb laser source for dual-comb applications. In 2012, the dual-wavelength dual-comb laser was used to demonstrate the measurement of the carrier dynamics of a semiconductor optical amplifier (SOA) with over 10 nanoseconds scanning range, sub-picosecond time domain sampling step, and hundreds of Hz update speed [28]. It was realized with a very simple experimental setup without the mechanical delay line. Pump-probe experiments were also performed using a bidirectionally mode-locked laser [118]. A polarization-multiplexed solid-state laser was used to measure the time constants in the SESAM dynamic model, which were consistent with the classical model [119].
Ranging, as another typical time-domain measurement, was soon preliminarily demonstrated sequentially using wavelength-multiplexed and polarization-multiplexed dual-comb lasers to achieve coherent distance measurement [29, 89]. Low-power dual-comb distance measurement based on incoherent reception was also realized [33, 120]. With a dual-wavelength dual-comb fiber laser with improved performance, ranging accuracy over a distance range of 70 m was determined to be on the order of μm in a measurement time of 1 s [44]. Several related improved techniques [55, 110, 121] had been developed.
High-quality dual-comb spectroscopy measurement using a free-running laser was a more challenging task considering the various factors affecting the quality of the spectroscopic result. While the previous studies had obtained temporal interferogram patterns indicating likely promising mutual coherence between pulses from a dual-comb laser, good-quality spectroscopy results could not be obtained until a dual-comb fiber laser with sufficient passive mutual stability performance was developed. After several years of incessant improvement on the fiber laser setup, a dual-wavelength fiber laser achieved about 250 Hz mutual linewidth between the dual combs, lower than the repetition frequency difference of 1,250 Hz, and mHz fluctuations in the repetition rate difference under free-running [34]. Through the measurement of acetylene gas cell and high-Q micro-ring resonators, a picometer spectral resolution was demonstrated, with well-matched spectroscopic results with the known database. Spectroscopic measurement of hydrogen cyanide gas was also carried out using a bi-directional fiber laser [66, 101]. Later on, doppler-limited hydrogen cyanide absorption spectra were measured at low pressures by polarization-multiplexed dual-comb laser [92]. These demonstrations verified the applicability of free-running single-cavity dual-comb lasers to spectroscopy applications with modest resolution requirements. Though fceo’s of the two combs are not actively controlled, passive coherence illustrated by the good-quality spectroscopy results suggests the existence of correlated fceo variations, in contrast to the random drifts between them if two independent lasers are used. Since then, the spectral measurement technology based on single-cavity dual-comb technology in the 1 μm [107, 108], 1.6 μm [35], and 2 μm [45, 67] bands have also attracted the attention of many researchers. In addition, the advantages of the low complexity of the single-cavity dual-comb laser source system have also render it more useful to new applications stressing the low-cost or on-site requirements, such as multi-component gas concentrations measurement in combustion [36] and fiber grating sensing system [37, 40, 68, 93].
Different from the spectroscopy measurement technology in the optical frequency band, the carrier-envelope offset frequency of the optical comb will not be converted to the terahertz or RF frequency band in the spectral measurement at those frequencies. Therefore, the requirement for the frequency stability of the carrier-envelope offset of the dual-comb light source is more relaxed. THz frequency measurement scheme based on the dual-wavelength laser source [38] and the THz time-domain spectroscopy measurement using the wavelength-multiplexing [42] and direction multiplexing [76] dual-comb lasers have achieved GHz spectral resolution. Further, an adaptive-sampling dual-THz comb spectral measurement method based on a dual-wavelength laser greatly expands the averaging time window [39]. The absorption peaks of the methane cyanide gas in the vibrationally excited state in the low-pressure methane cyanide and nitrogen gas mixture can be distinguished, thanks to the MHz-level ultrahigh THz spectral resolution. Recently, the application of coherent anti-Stokes Raman scattering spectroscopy and microscopy with a single cavity dual optical frequency comb was reported [71, 75].
3 Single-cavity multi-comb fiber lasers and their applications
If additional combs can be introduced to a dual-comb system, such a tri-comb or multi-comb system could further expand the capability for certain applications [122]. However, a tri-comb system based on three frequency-stabilized optical frequency combs would be even more complex and expensive. Therefore, single-cavity multi-comb technology could be a more attractive alternative. However, from the perspective of multiplexing schemes, it is challenging to realize the generation of triple-comb or multi-comb in other physical dimensions, except for wavelength. Wavelength -multiplexed laser would also be limited by the finite accessible spectrum width, which strongly limits the performance of multi-comb signals. The concept of Multi-dimensional Multiplexed Mode-Locked Laser (M3L2) was proposed [123]. Multiple combinations in the wavelength- and polarization-dimensions in the optical cavity were leveraged to realize a single-cavity, three-comb or even multi-comb light source [123]. On the application side, dead-zone-free RF frequency measurement was demonstrated with a single-cavity tri-comb laser with wavelength multiplexing [124]. A real-time ranging scheme based on a multi-wavelength tri-comb laser demonstrated an extended ambiguity range to the order of tens of kilometers [125]. A dual-asynchronous sampling scheme based on wavelength-multiplexed tri-comb source has realized the de-modulation of fast-varying spectral modulations [126]. It should be noted that these demonstrations had been carried out with marginal increase in system complexity, due to the application of multi-comb fiber lasers.
4 Conclusion
We have reviewed the history and summarized the recent progress of single-cavity dual-comb fiber lasers and their applications. While hardly-known or recognized over a decade ago, single-cavity, dual-comb fiber lasers have become an attractive topic for researchers around the world. The essence of this technology is removing the “smart” feedback control system and replacing it with a properly designed, “dumb” yet self-stabilized physical system based on a good understanding of laser physics. This renders the unique advantages of the technology in system complexity, power consumption, cost, and compactness, which make it an alternative over the more universal, powerful yet more complicated frequency-stabilized OFC solutions. Such lasers could also play an important role in broadening the reach of the dual-comb techniques by unlocking those application areas that are highly sensitive to system complexity or size. On the other hand, it is not unexpected that, as such lasers are based on somewhat unconventional laser designs, there could be some performance limitations like the adjustability and stability of the repetition frequency difference, the pulse energy as well as intracavity phenomena unique to these lasers. While new mode-locked laser technologies based on more sophisticated ultrafast pulse dynamics or new functional devices keep on emerging, the dual-comb lasers, by far, mostly are still based on the traditional cavity and conventional soliton configurations. With further investigations into the dual-comb dynamics in the cavity and better understanding of ways to control and manipulate them, it is expected that more innovative solutions that further overcome the current performance limitations would be found in the near future. It is foreseeable that more would delve into further investigating or solving these issues.
Author contributions
JY: wrote the initial manuscript, made graph, and revised the second drafts. XZ: obtained funding, designed and reviewed the manuscript. LZ: contributed sections of the initial drafts. ZZ: critically read, discussed, and edited the content prior to submission. All authors read and approved the manuscript.
Funding
This work was supported in part by the National Key Research and Development Program of China (2021YFB2800605) and in part by the National Natural Science Foundation of China (62127814).
Conflict of interest
The authors declare that the research was conducted in the absence of any commercial or financial relationships that could be construed as a potential conflict of interest.
Publisher’s note
All claims expressed in this article are solely those of the authors and do not necessarily represent those of their affiliated organizations, or those of the publisher, the editors and the reviewers. Any product that may be evaluated in this article, or claim that may be made by its manufacturer, is not guaranteed or endorsed by the publisher.
References
1. Hänsch TW. Nobel lecture: Passion for precision[J]. Rev Mod Phys (2006) 78(4):1170–87. doi:10.1002/cphc.200600195
2. Jones DJ, Diddams SA, Ranka JK, Stentz A, Windeler RS, Hall JL, et al. Carrier-envelope phase control of femtosecond mode-locked lasers and direct optical frequency synthesis. Science (2000) 288(5466):635–9. doi:10.1126/science.288.5466.635
3. Schiller S. Spectrometry with frequency combs. Opt Lett (2002) 27(9):766–8. doi:10.1364/ol.27.000766
4. Giorgetta FR, Swann WC, Sinclair LC, Baumann E, Coddington I, Newbury NR Optical two-way time and frequency transfer over free space[J]. Nat Photon (2013) 7(6):435. doi:10.1038/nphoton.2013.69
5. Bergeron H, Sinclair LC, Swann WC, Nelson CW, Deschenes JD, Baumann E, et al. Tight real-time synchronization of a microwave clock to an optical clock across a turbulent air path. Optica (2016) 3(4):441–7. doi:10.1364/optica.3.000441
6. Coddington I, SwannNewbury WCNR. Coherent multiheterodyne spectroscopy using stabilized optical frequency combs. Phys Rev Lett (2008) 100(1):013902. doi:10.1103/physrevlett.100.013902
7. Baumann E, Giorgetta FR, Swann WC, Zolot AM, Coddington I, Newbury NR. Spectroscopy of the methaneν3band with an accurate midinfrared coherent dual-comb spectrometer. Phys Rev A (2011) 84(6):062513. doi:10.1103/physreva.84.062513
8. Newbury NR, CoddingtonSwann IW. Sensitivity of coherent dual-comb spectroscopy. ] Opt Express (2010) 18(8):7929–45. doi:10.1364/oe.18.007929
9. Coddington I, Swann WC, Nenadovic L, Newbury NR. Rapid and precise absolute distance measurements at long range. Nat Photon (2009) 3(6):351–6. doi:10.1038/nphoton.2009.94
10. Coddington I, NewburySwann NW. Dual-comb spectroscopy. Optica (2016) 3(4):414–26. doi:10.1364/optica.3.000414
11. Buholz N, Chodorow M. 3.2 - acoustic wave amplitude modulation of a multimode ring laser. IEEE J Quan Electron (1967) 3(11):454–9. doi:10.1109/jqe.1967.1074407
12. Matsas V, Newson T, Richardson D, Payne D. Selfstarting passively mode-locked fibre ring soliton laser exploiting nonlinear polarisation rotation. Electron Lett (1992) 28(15):1391–3. doi:10.1049/el:19920885
13. Noske DU, Guy MJ, Rottwitt K, Kashyap R, Taylor J. Dual-wavelength operation of a passively mode-locked “figure-of-eight” ytterbium-erbium fibre soliton laser. Opt Commun (1994) 108(4):297–301. doi:10.1016/0030-4018(94)90666-1
15. Zhao Y, Shu C. A fiber laser for effective generation of tunable single- and dual-wavelength mode-locked optical pulses. Appl Phys Lett (1998) 72(13):1556–8. doi:10.1063/1.121113
16. Bohn MJ, Jason JonesDiels RJ-C. Mutual kerr-lens mode-locking. Opt Commun (1999) 170(1):85–92. doi:10.1016/s0030-4018(99)00441-1
17. Guandalini A, Diels J-C, Reali G, Kubecek V, Zhang YS. Bidirectional operation of a diode pumped vanadate laser(C). In: Advanced solid-state lasers. Seattle, Washington: Optica Publishing Group.
18. Meng XM, Diels J-C, Kuehlke D, Batchko R, Byer R. Bidirectional, synchronously pumped, ring optical parametric oscillator. Opt Lett (2001) 26(5):265–7. doi:10.1364/ol.26.000265
19. Gong YD, Tian XL, Tang M, Shum P, Chia M, Paulose V, et al. Generation of dual wavelength ultrashort pulse outputs from a passive mode locked fiber ring laser. ] Opt Commun (2006) 265(2):628–31. doi:10.1016/j.optcom.2006.04.065
20. Chen WC, LuoXu ZCWC. The interaction of dual wavelength solitons in fiber laser. Laser Phys Lett (2009) 6(11):816–20. doi:10.1002/lapl.200910074
21. Zhi Chao L, Ai PingWen Cheng LX, Wen-Cheng Xu . Tunable and switchable multiwavelength passively mode-locked fiber laser based on SESAM and inline birefringence comb filter. IEEE Photon J (2011) 3(1):64–70. doi:10.1109/jphot.2010.2102012
22. Zhang H, Tang DY, Wu X, Zhao LM. Multi-wavelength dissipative soliton operation of an erbium-doped fiber laser. Opt Express (2009) 17(15):12692–7. doi:10.1364/oe.17.012692
23. Kieu K, Mansuripur M. All-fiber bidirectional passively mode-locked ring laser. Opt Lett (2008) 33(1):64–6. doi:10.1364/ol.33.000064
24. Yang H-J, NybergRiles SK. High-precision absolute distance measurement using dual-laser frequency scanned interferometry under realistic conditions. Nucl Instr Methods Phys Res Section A: Acc Spectrometers, Detectors Associated Equipment (2007) 575(3):395–401. doi:10.1016/j.nima.2007.02.101
25. Giaccari P, Deschenes JD, Saucier P, Genest J, Tremblay P. Active Fourier-transform spectroscopy combining the direct RF beating of two fiber-based mode-locked lasers with a novel referencing method. Opt Express (2008) 16(6):4347–65. doi:10.1364/oe.16.004347
26. Cundiff ST, Weiner AM. Optical arbitrary waveform generation. Nat Photon (2010) 4(11):760–6. doi:10.1038/nphoton.2010.196
27. Zhao X, Zheng Z, Lu ZT, Liu Y, Jiang Y, Yang X, et al. Switchable, dual-wavelength passively mode-locked ultrafast fiber laser based on a single-wall carbon nanotube modelocker and intracavity loss tuning. Opt Express (2011) 19(2):1168–73. doi:10.1364/oe.19.001168
28. Zhao X, Zheng Z, Liu L, Wang Q, Chen H, Liu J. Fast, long-scan-range pump-probe measurement based on asynchronous sampling using a dual-wavelength mode-locked fiber laser. ] Opt Express (2012) 20(23):25584–9. doi:10.1364/oe.20.025584
29. Zhao X, Zheng Z, Liu Y, Guan J, Liu L, Sun Y. High-resolution absolute distance measurement using a dual-wavelength, dual-comb, femtosecond fiber laser(C). In: Conference on Lasers and Electro-Optics (CLEO), San Jose, CA, United States, May 6–11, 2012. San Jose, CA, United states: Optical Society of American OSA (2012). CM2J.4.
30. Zhao X, Zheng Z, Liu Y, Hu G, Liu J. Dual-wavelength, bidirectional single-wall carbon nanotube mode-locked fiber laser. IEEE Photon Technol Lett (2014) 26(17):1722–5. doi:10.1109/lpt.2014.2332000
31. Gong Z, Zhao X, Hu GQ, Liu J, Zheng Z Polarization multiplexed, dual-frequency ultrashort pulse generation by a birefringent mode-locked fiber laser(C). In: Conference on Lasers and Electro-Optics (CLEO), San Jose, California, June 8–13, 2014. San Jose, California: Optical Society of America (2014). JTh2A.20.
32. Liu Y, Zhao X, Hu GQ, Li C, Zhao B, Zheng Z. Unidirectional, dual-comb lasing under multiple pulse formation mechanisms in a passively mode-locked fiber ring laser. Opt Express (2016) 24(19):21392–8. doi:10.1364/oe.24.021392
33. Zheng Z, Zhao X. High-resolution, dual-comb asynchronous sampling enabled by dual-wavelength ultrafast fiber lasers and its applications. In: Conference on Lasers and Electro-Optics Pacific Rim (CLEO-PR, Kyoto, Japan, June 30–July 4, 2013. Japan: Institute of Electrical and Electronics Engineers Inc. WF3-2.
34. Zhao X, Hu GQ, Zhao BF, Li C, Pan Y, Liu Y, et al. Picometer-resolution dual-comb spectroscopy with a free-running fiber laser. Opt Express (2016) 24(19):21833–45. doi:10.1364/oe.24.021833
35. Chen J, Zhao X, Yao ZJ, Li T, Li Q, Xie S, et al. Dual-comb spectroscopy of methane based on a free-running Erbium-doped fiber laser. Opt Express (2019) 27(8):11406–12. doi:10.1364/oe.27.011406
36. Xu K, Ma LH, Chen J, Zhao X, Wang Q, Kan R, et al. Dual-comb spectroscopy for laminar premixed flames with a free-running fiber laser[J]. Combustion Sci Technol (2021) 1
37. Zhao X, Li Q, Yin SY, Chen J, Zheng Z. Dual-comb dynamic interrogation of fiber bragg grating with one mode-locked fiber laser. IEEE Sensors J (2018) 18(16):6621–6. doi:10.1109/jsen.2018.2852846
38. Hu GQ, Mizuguchi T, Zhao X, Minamikawa T, Mizuno T, Yang Y, et al. Measurement of absolute frequency of continuous-wave terahertz radiation in real time using a free-running, dual-wavelength mode-locked, erbium-doped fibre laser. Scientific Rep (2017) 7(1):42082. doi:10.1038/srep42082
39. Chen J, Nitta K, Zhao X, Mizuno T, Minamikawa T, Hindle F, et al. Adaptive-sampling near-Doppler-limited terahertz dual-comb spectroscopy with a free-running single-cavity fiber laser. Adv Photon (2020) 2(3):1. doi:10.1117/1.ap.2.3.036004
40. Zhao X, Yang JJ, Liu JS, Shao H, Zhang X, Li Q, et al. Dynamic quasi-distributed ultraweak fiber bragg grating array sensing enabled by depth-resolved dual-comb spectroscopy. IEEE Trans Instrumentation Meas (2020) 69(8):5821–7. doi:10.1109/tim.2019.2959754
41. Wang RL, Zhao X, Bai WN, Chen J, Pan Y, Zheng Z. Polarization-maintaining, dual-wavelength, dual-comb mode-locked fiber laser(C). In: Conference on Lasers and Electro-Optics (CLEO), San Jose, CA, United States, May 13–18, 2018, 139. San Jose, CA, United states: Institute of Electrical and Electronics Engineers Inc. (2018).
42. Hu GQ, Mizuguchi T, Oe R, Nitta K, Zhao X, Minamikawa T, et al. Dual terahertz comb spectroscopy with a single free-running fibre laser. Scientific Rep (2018) 8(1):11155. doi:10.1038/s41598-018-29403-9
43. Hu DT, Wu ZL, Cao H, Shi Y, Li R, Tian H, et al. Dual-comb absolute distance measurement of non-cooperative targets with a single free-running mode-locked fiber laser. Opt Commun (2021) 482:126566. doi:10.1016/j.optcom.2020.126566
44. Lin BK, Zhao X, He MZ, Pan Y, Chen J, Cao S, et al. Dual-comb absolute distance measurement based on a dual-wavelength passively mode-locked laser. IEEE Photon J (2017) 9(6):1–8. doi:10.1109/jphot.2017.2772292
45. Liao RY, Song YJ, Liu W, Shi H, Chai L, Hu M. Dual-comb spectroscopy with a single free-running thulium-doped fiber laser. Opt Express (2018) 26(8):11046–54. doi:10.1364/oe.26.011046
46. Luo X, Tuan TH, Saini TS, Nguyen HPT, Suzuki T, Ohishi Y. Tunable and switchable all-fiber dual-wavelength mode locked laser based on Lyot filtering effect. ] Opt Express (2019) 27(10):14635–47. doi:10.1364/oe.27.014635
47. Guo ZR, Liu TT, Peng JS, Zhu Y, Huang K, Zeng H. Self-started dual-wavelength mode-locking with well-controlled repetition rate difference. J Lightwave Technol (2021) 39(11):3575–81. doi:10.1109/jlt.2021.3068785
48. Zhu YJ, Cui ZK, Sun XN, Shirahata T, Jin L, Yamashita S, et al. Fiber-based dynamically tunable Lyot filter for dual-wavelength and tunable single-wavelength mode-locking of fiber lasers. ] Opt Express (2020) 28(19):27250–7. doi:10.1364/oe.402173
49. Li RM, Shi HS, Tian HC, Li Y, Liu B, Song Y, et al. All-polarization-maintaining dual-wavelength mode-locked fiber laser based on Sagnac loop filter. Opt Express (2018) 26(22):28302–11. doi:10.1364/oe.26.028302
50. Luo X, Tuan TH, Saini TS, Nguyen HPT, Suzuki T, Ohishi Y. Switchable dual-wavelength mode-locked fiber laser using Saganc loop mirror. Opt Commun (2020) 463:125457. doi:10.1016/j.optcom.2020.125457
51. Chen J, Wang R, Li T, Li C, Pan Y, Zhao X, et al. Self-starting, turn-key dual-comb mode-locked fiber laser with a few-mode fiber filter(C). In: Conference on Lasers and Electro-Optics (CLEO), San Jose, California, May 14–19, 2017. San Jose, California: Optical Society of America. JW2A.5.
52. Lin JQ, Dong ZP, Dong TH, Zhang Y, Dai C, Yao P, et al. Wavelength switchable all-fiber mode-locked laser based on nonlinear multimode interference. Opt Laser Technol (2021) 141:107093. doi:10.1016/j.optlastec.2021.107093
53. Fellinger J, Winkler G, Mayer AS, Steidle LR, Heckl OH. Tunable dual-color operation of Yb:fiber laser via mechanical spectral subdivision. Opt Express (2019) 27(4):5478–86. doi:10.1364/oe.27.005478
54. Fellinger J, Mayer AS, Winkler G, Grosinger W, Truong GW, Droste S, et al. Tunable dual-comb from an all-polarization-maintaining single-cavity dual-color Yb:fiber laser. Opt Express (2019) 27(20):28062–74. doi:10.1364/oe.27.028062
55. Fellinger J, Winkler G, Aldia PEC, Mayer AS, Shumakova V, Perner LW, et al. Simple approach for extending the ambiguity-free range of dual-comb ranging. Opt Lett (2021) 46(15):3677–80. doi:10.1364/ol.427816
56. Hu GQ, Pan YL, Zhao X, Yin S, Zhang M, Zheng Z. Asynchronous and synchronous dual-wavelength pulse generation in a passively mode-locked fiber laser with a mode-locker. Opt Lett (2017) 42(23):4942–5. doi:10.1364/ol.42.004942
57. Zhou Y, Ren YX, Shi JW, Wong KKY. Dynamics of dissipative soliton molecules in a dual-wavelength ultrafast fiber laser. Opt Express (2022) 30(12):21931–42. doi:10.1364/oe.461092
58. Liu TT, Yan M, Guo ZR, Zeng H. Mechanisms of dual-wavelength dissipative soliton mode-locking in net-normal dispersion fiber lasers. Results Phys (2022) 36:105464. doi:10.1016/j.rinp.2022.105464
59. Guo Z, Liu T, Zeng H. Real-time observation of the formation of dual-wavelength mode locking[J]. Wiley: Advanced Photonics Research (2000).
60. Gu XR, Chen HD, Li Y, Cao XR, Wang CS, Liu YW. Ultrashort pulse duration and broadband dual-comb laser system based on a free-running passively mode-locked Er-fiber oscillator. Laser Phys Lett (2021) 18(12):125101. doi:10.1088/1612-202x/ac308e
61. Liu M, Li TJ, Luo AP, Xu WC, Luo ZC. “Periodic” soliton explosions in a dual-wavelength mode-locked Yb-doped fiber laser. Photon Res (2020) 8(3):246–51. doi:10.1364/prj.377966
62. Pawliszewska M, Dużyńska A, Zdrojek M, Sotor J. Wavelength- and dispersion-tunable ultrafast holmium-doped fiber laser with dual-color operation. Opt Lett (2020) 45(4):956–9. doi:10.1364/ol.383788
63. Xie GQ, Tang DY, Luo H, Zhang HJ, Yu HH, Wang JY, et al. Dual-wavelength synchronously mode-locked Nd:CNGG laser. Opt Lett (2008) 33(16):1872–4. doi:10.1364/ol.33.001872
64. Tao JN, Lin QM, Yan L, Hou L, Lu B, Bai J. Asynchronous vector solitons based dual-comb in a fiber laser mode-locked by GO-COOH SA. Opt Laser Technol (2022) 154:108308. doi:10.1016/j.optlastec.2022.108308
65. Bohn MJ, Diels J-C. Bidirectional Kerr-lens mode-locked femtosecond ring laser. ] Opt Commun (1997) 141(1):53–8. doi:10.1016/s0030-4018(97)00210-1
66. Mehravar S, Norwood RA, Peyghambarian N, Kieu K. Real-time dual-comb spectroscopy with a free-running bidirectionally mode-locked fiber laser. Appl Phys Lett (2016) 108(23):231104. doi:10.1063/1.4953400
67. Li YM, Yin K, Zhang X, Zheng X, Cheng X, Jiang T. All-fiber bidirectional mode-locked ultrafast fiber laser at 2 μm. IEEE Photon J (2019) 11(6):1–8. doi:10.1109/jphot.2019.2957167
68. Guo JJ, Ding YH, Xiao XS, Kong L, Yang C. Multiplexed static FBG strain sensors by dual-comb spectroscopy with a free running fiber laser. Opt Express (2018) 26(13):16147–54. doi:10.1364/oe.26.016147
69. Nakajima Y, HataMinoshima YK. High-coherence ultra-broadband bidirectional dual-comb fiber laser. Opt Express (2019) 27(5):5931–44. doi:10.1364/oe.27.005931
70. Kayes MI, Abdukerim N, Rekik A, Rochette M. Free-running mode-locked laser based dual-comb spectroscopy. Opt Lett (2018) 43(23):5809–12. doi:10.1364/ol.43.005809
71. Mizuno T, Nakajima Y, Hata Y, Tsuda T, Asahara A, Kato T, et al. Computationally image-corrected dual-comb microscopy with a free-running single-cavity dual-comb fiber laser. ] Opt Express (2021) 29(4):5018–32. doi:10.1364/oe.415242
72. Li BW, Xing J, Kwon D, Xie Y, Prakash N, Kim J, et al. Bidirectional mode-locked all-normal dispersion fiber laser. Optica (2020) 7(8):961–4. doi:10.1364/optica.396304
73. Olson J, Ou YH, Azarm A, Kieu K. Bi-directional mode-locked thulium fiber laser as a single-cavity dual-comb source. IEEE Photon Technol Lett (2018) 30(20):1772–5. doi:10.1109/lpt.2018.2868940
74. Liu XY, Li Z, Pan DH, Li Q, Fu HY. All-polarization-maintaining bidirectional dual-comb fiber laser by nonlinear polarization evolution(C). In: 26th Optoelectronics and Communications Conference, Hong Kong, July 3–7, 2021. Hong Kong: Optica Publishing Group (2021). T3C.5.
75. Qin Y, Cromey B, Batjargal O, Kieu K. All-fiber single-cavity dual-comb for coherent anti-Stokes Raman scattering spectroscopy based on spectral focusing. ] Opt Lett (2021) 46(1):146–9. doi:10.1364/ol.413431
76. Baker RD, Yardimci NT, Ou Y-H, Kieu K, Jarrahi M. Self-triggered asynchronous optical sampling terahertz spectroscopy using a bidirectional mode-locked fiber laser. Scientific Rep (2018) 8(1):14802. doi:10.1038/s41598-018-33152-0
77. Chernysheva M, Araimi MA, Kbashi H, Arif R, Sergeyev SV, Rozhin A. Isolator-free switchable uni- and bidirectional hybrid mode-locked erbium-doped fiber laser. Opt Express (2016) 24(14):15721–9. doi:10.1364/oe.24.015721
78. Zeng C, LiuYun XML. Bidirectional fiber soliton laser mode-locked by single-wall carbon nanotubes. Opt Express (2013) 21(16):18937–42. doi:10.1364/oe.21.018937
79. Ouyang CM, Shum P, Wu K, Wong JH, Lam HQ, Aditya S. Bidirectional passively mode-locked soliton fiber laser with a four-port circulator. Opt Lett (2011) 36(11):2089–91. doi:10.1364/ol.36.002089
80. Zhou Y, Ren YX, Shi JW, Wong KKY. Breathing dissipative soliton molecule switching in a bidirectional mode-locked fiber laser. Adv Photon Res (2022) 3(4):2100318. doi:10.1002/adpr.202100318
81. Kudelin I, SugavanamChernysheva SM. Build-up dynamics in bidirectional soliton fiber lasers. Photon Res (2020) 8(6):776–80. doi:10.1364/prj.388988
82. Krylov AA, Chernykh DS, Arutyunyan NR, Grebenyukov VV, Pozharov AS, Obraztsova ED. Generation regimes of bidirectional hybridly mode-locked ultrashort pulse erbium-doped all-fiber ring laser with a distributed polarizer. Appl Opt (2016) 55(15):4201–9. doi:10.1364/ao.55.004201
83. Mamidala V, Woodward RI, Yang Y, Liu HH, Chow KK. Graphene-based passively mode-locked bidirectional fiber ring laser. ] Opt Express (2014) 22(4):4539–46. doi:10.1364/oe.22.004539
84. Liu HH, Chow KK. Operation-Switchable bidirectional pulsed fiber laser incorporating carbon-nanotube-based saturable absorber. IEEE J Selected Top Quan Electron (2014) 20(5):278–82. doi:10.1109/jstqe.2014.2304141
85. Cui Y, Liu X. Graphene and nanotube mode-locked fiber laser emitting dissipative and conventional solitons. Opt Express (2013) 21(16):18969–74. doi:10.1364/oe.21.018969
86. Yun L, Liu X. Observation of vector- and scalar-pulse in a nanotube-mode-locked fiber laser. Opt Express (2014) 22(5):5442–7. doi:10.1364/oe.22.005442
87. Liu B, Luo Y, Xiang Y, Xiao X, Sun Q, Liu D, et al. Multiplexed ultrafast fiber laser emitting multi-state solitons. Opt Express (2018) 26(21):27461–71. doi:10.1364/oe.26.027461
88. Yang R, Sun H, Hu P, Yang H, Fu H, Fan Z, et al. Experimental exploration of mode-locking evolution mechanism in dual-ring fiber laser. Optik (2020) 208:163899. doi:10.1016/j.ijleo.2019.163899
89. Zhao X, Gong Z, Liu Y, Liu JS, Zheng Z. Coherent asynchronous sampling distance measurement using a single polarization-multiplexed ultrafast laser(C). In: Conference on Lasers and Electro-Optics (CLEO), San Jose, California, June 8–13, 2014. San Jose, California: Optical Society of America (2014). STh4O.2.
90. Zhao X, Li T, Liu Y, Li Q, Zheng Z. Polarization-multiplexed, dual-comb all-fiber mode-locked laser. Photon Res (2018) 6(9):853–7. doi:10.1364/prj.6.000853
91. Liu Y, Zhao X, Zhao BF, Yao Z, Gong Z, Yasui T, et al. High-resolution, dual-comb spectroscopy enabled by a polarization-multiplexed, dual-comb femtosecond fiber laser(C). In: Conference on Lasers and Electro-Optics (CLEO), San Jose, California, June 5–10, 2016. San Jose, California: Optical Society of America (2016). AM4K.5.
92. Sterczewski Ł A, Przewłoka A, Kaszub W, Sotor J. Computational Doppler-limited dual-comb spectroscopy with a free-running all-fiber laser. APL Photon (2019) 4(11):116102. doi:10.1063/1.5117847
93. Guo JJ, Zhao KJ, Zhou BQ, Ning W, Jiang K, Yang C, et al. Wearable and skin-mountable fiber-optic strain sensors interrogated by a free-running, dual-comb fiber laser. Adv Opt Mater (2019) 7(12):1900086. doi:10.1002/adom.201900086
94. Nakajima Y, HataMinoshima YK. All-polarization-maintaining, polarization-multiplexed, dual-comb fiber laser with a nonlinear amplifying loop mirror. Opt Express (2019) 27(10):14648–56. doi:10.1364/oe.27.014648
95. Cuevas AR, Kbashi HJ, Stoliarov D, Sergeyev S. Polarization dynamics, stability and tunability of a dual-comb polarization-multiplexing ring-cavity fiber laser[J] (2022). arXiv preprint arXiv:2208.04385.
96. Zhao KJ, Gao CX, Xiao XS, Yang C. Buildup dynamics of asynchronous vector solitons in a polarization-multiplexed dual-comb fiber laser. Opt Lett (2020) 45(14):4040–3. doi:10.1364/ol.398323
97. Zhao KJ, Gao CX, Xiao XS, Yang C. Real-time collision dynamics of vector solitons in a fiber laser. Photon Res (2021) 9(3):289–98. doi:10.1364/prj.413855
98. Akosman AE, Sander MY. Dual comb generation from a mode-locked fiber laser with orthogonally polarized interlaced pulses. ] Opt Express (2017) 25(16):18592–602. doi:10.1364/oe.25.018592
99. Vedala G, Hui R. Auto-start mechanism of a polarization-multiplexed dual-frequency femtosecond fiber laser(C). In: Conference on Lasers and Electro-Optics (CLEO), San Jose, CA, United States, May 13–18, 2018, 147. San Jose, CA, USA: IEEE, JW2A (2018).
100. Nakajima Y, KusumiMinoshima YK. Mechanical sharing dual-comb fiber laser based on anall-polarization-maintaining cavity configuration. Opt Lett (2021) 46(21):5401–4. doi:10.1364/ol.440818
101. Ideguchi T, Nakamura T, Kobayashi Y, Goda K. Kerr-lens mode-locked bidirectional dual-comb ring laser for broadband dual-comb spectroscopy. Optica (2016) 3(7):748–53. doi:10.1364/optica.3.000748
102. Lomsadze B, FradetArnold KMRS. Elastic tape behavior of a bi-directional Kerr-lens mode-locked dual-comb ring laser. ] Opt Lett (2020) 45(5):1080–3. doi:10.1364/ol.386160
103. Galtier S, Pivard C, Morville J, Rairoux P. High-resolution dual comb spectroscopy using a free-running, bidirectional ring titanium sapphire laser. Opt Express (2022) 30(12):21148–58. doi:10.1364/oe.455963
104. Pupeikis J, Willenberg B, Camenzind SL, Benayad A, Camy P, Phillips CR, et al. Spatially multiplexed single-cavity dual-comb laser. Optica (2022) 9(7):713–6. doi:10.1364/optica.457787
105. Fritsch K, Hofer T, Brons J, Iandulskii M, Mak KF, Chen Z, et al. Dual-comb thin-disk oscillator. Nat Commun (2022) 13(1):2584. doi:10.1038/s41467-022-30078-0
106. Link SM, KlennerKeller AU. Dual-comb modelocked lasers: Semiconductor saturable absorber mirror decouples noise stabilization. Opt Express (2016) 24(3):1889–902. doi:10.1364/oe.24.001889
107. Link SM, Maas D, Waldburger D, Keller U. Dual-comb spectroscopy of water vapor with a free-running semiconductor disk laser. Science (2017) 356(6343):1164–8. doi:10.1126/science.aam7424
108. Modsching N, Drs J, Brochard P, Fischer J, Schilt S, Wittwer VJ, et al. High-power dual-comb thin-disk laser oscillator for fast high-resolution spectroscopy. Opt Express (2021) 29(10):15104–13. doi:10.1364/oe.424317
109. Nurnberg J, Alfieri CGE, Chen Z, Waldburger D, Picque N, Keller U. An unstabilized femtosecond semiconductor laser for dual-comb spectroscopy of acetylene. ] Opt Express (2019) 27(3):3190–9. doi:10.1364/oe.27.003190
110. Nurnberg J, Willenberg B, Phillips CR, Keller U. Dual-comb ranging with frequency combs from single cavity free-running laser oscillators. Opt Express (2021) 29(16):24910–8. doi:10.1364/oe.428051
111. Kowalczyk M, Sterczewski LA, Zhang X, Petrov V, Sotor J. Dual-dispersion-regime dual-comb mode-locked laser. Opt Lett (2022) 47(7):1762–5. doi:10.1364/ol.453653
112. Camenzind SL, Koenen D, Willenberg B, Pupeikis J, Phillips CR, Keller U. Timing jitter characterization of free-running dual-comb laser with sub-attosecond resolution using optical heterodyne detection. Opt Express (2022) 30(4):5075–94. doi:10.1364/oe.448274
113. Kowalczyk M, Ł S, Zhang X, Petrov V, Wang Z, Sotor J. Dual-comb femtosecond solid-state laser with inherent polarization-multiplexing. Laser Photon Rev (2021) 15(8):2000441. doi:10.1002/lpor.202000441
114. Liang HC, Wu CS. Diode-pumped orthogonally polarized self-mode-locked Nd:YLF lasers subject to gain competition and thermal lensing effect. ] Opt Express (2017) 25(12):13697–704. doi:10.1364/oe.25.013697
115. Pupeikis J, Willenberg B, Bruno F, Hettich M, Nussbaum-Lapping A, Golling M, et al. Picosecond ultrasonics with a free-running dual-comb laser. Opt Express (2021) 29(22):35735–54. doi:10.1364/oe.440856
116. Willenberg B, Pupeikis J, Kruger LM, Koch F, Phillips CR, Keller U. Femtosecond dual-comb Yb:CaF2 laser from a single free-running polarization-multiplexed cavity for optical sampling applications[J]. Opt Express (2020) 28(20):30275–88. doi:10.1364/oe.403072
117. Bao CY, SuhVahala M-GK. Microresonator soliton dual-comb imaging. Optica (2019) 6(9):1110–6. doi:10.1364/optica.6.001110
118. Kolano M, Boidol O, Molter D, Von Freymann G. Single-laser, polarization-controlled optical sampling system. Opt Express (2018) 26(23):30338–46. doi:10.1364/oe.26.030338
119. Nussbaum-Lapping A, Pupeikis J, Willenberg B, Phillips CR, Keller U Complete SESAM characterization via equivalent time sampling using a free-running dual-comb laser(C). In: CLEO: Science and Innovations,, San Jose, California, May 9–14, 2021. Optica Publishing Group (2021). STu2E. 5.
120. Liu L, Zhao X, Wang Q, Gong Z, Liu J, Zheng Z. Fast, asynchronous sampling distance ranging using an SOA gate and a dual-wavelength mode-locked fiber laser(C). In: Conference on Lasers and Electro-Optics Pacific Rim (CLEO-PR), Kyoto, June 30–July 4, 2013. Optical Society of America (2013).
121. Li YP, Cai YW, Li RM, Shi H, Tian H, He M, et al. Large-scale absolute distance measurement with dual free-running all-polarization-maintaining femtosecond fiber lasers. Chin Opt Lett (2019) 17(9):091202. doi:10.3788/col201917.091202
122. Lomsadze B, SmithCundiff BCST. Tri-comb spectroscopy. Nat Photon (2018) 12(11):676–80. doi:10.1038/s41566-018-0267-4
123. Li T, Zhao X, Chen J, Li Q, Xie S, Zheng Z. Tri-comb and quad-comb generation based on a multi-dimensional multiplexed mode-locked laser. J Lightwave Technol (2019) 37(20):5178–84. doi:10.1109/jlt.2019.2929821
124. Zhao X, Li C, Li T, Hu G, Li R, Bai M, et al. Dead-band-free, high-resolution microwave frequency measurement using a free-running triple-comb fiber laser. IEEE J Selected Top Quan Electron (2018) 24(3):1–8. doi:10.1109/jstqe.2017.2770098
125. Li T, Zhao X, Chen J, Yang JJ, Li Q, Li YH, et al. Absolute distance measurement with a long ambiguity range using a tri-comb mode-locked fiber laser(C). In: Conference on Lasers and Electro-Optics (CLEO). San Jose, CA, USA: IEEE, SM2H. (2019).
Keywords: single-cavity dual-comb, optical frequency comb, dual-comb, fiber laser, femtosecond laser
Citation: Yang J, Zhao X, Zhang L and Zheng Z (2023) Single-cavity dual-comb fiber lasers and their applications. Front. Phys. 10:1070284. doi: 10.3389/fphy.2022.1070284
Received: 14 October 2022; Accepted: 13 December 2022;
Published: 06 January 2023.
Edited by:
Hainian Han, Institute of Physics (CAS), ChinaReviewed by:
Ming Yan, East China Normal University, ChinaZuxing Zhang, Nanjing University of Posts and Telecommunications, China
Yige Lin, National Institute of Metrology, China
Copyright © 2023 Yang, Zhao, Zhang and Zheng. This is an open-access article distributed under the terms of the Creative Commons Attribution License (CC BY). The use, distribution or reproduction in other forums is permitted, provided the original author(s) and the copyright owner(s) are credited and that the original publication in this journal is cited, in accordance with accepted academic practice. No use, distribution or reproduction is permitted which does not comply with these terms.
*Correspondence: Xin Zhao, x.zhao@buaa.edu.cn