- 1Tianjin Fire Research Institute of Ministry of Emergency Management, Tianjin, China
- 2The Extreme Optoelectromechanics Laboratory, School of Physics and Materials Science, East China Normal University, Shanghai, China
We experimentally investigated clean optical emissions from multiple combustion intermediates including free radicals C2, CH, and CN at multiple wavelengths induced by ultrashort 1,030-nm laser pulses. We systematically study the evolution of the fluorescence emissions induced by the femtosecond laser filament in the combustion field with the parameters such as the laser pulse energy, pulse duration, and focal length. Compared with the previous work, we promote that the fluorescence emissions of the combustion product can be manipulated effectively by controlling the femtosecond laser characteristics including pulse energy, duration, and the focusing conditions. This process helps to optimize its signal-to-noise ratio, which provides a further application of the femtosecond laser pulses to sense the combustion intermediates.
Introduction
In recent years, the rapid development of the ultrafast laser technology has made it easier to obtain high power femtosecond laser pulses, and novel nonlinear effects triggered by the femtosecond laser filamentation process have attracted widespread attention, such as laser power density clamping in the filament, super-continuous white light, THz generation, and self-steepening effects [1–3]. Based on these unique nonlinear effects, ultrafast strong-field laser filament formation has shown great promise for applications in atmospheric environmental sensing, air lasers, artificial weather control, and other applied research fields [4–9]. In the field of combustion, the ultrafast intense-field laser filament-induced nonlinear spectroscopy can be used for combustion diagnosis. On the one hand, ultrafast strong-field lasers can be used to measure combustion intermediate radical products with ultrashort lifetime in the combustion field and reveal various ultrafast complex physicochemical processes existing in the combustion field [7]. On the other hand, the high clamping intensity of the femtosecond filament can be used for multi-photon excitation of combustion intermediates to achieve simultaneous multi-component measurements and to solve the limitation of tuning the laser wavelength for detecting different combustion components [4, 9].
The combustion process of fuels creates a very complex environment with a large number of intermediate products and many chemical reactions taking place. Although filamentation parameters of ultrafast intense-field laser transmission have been measured in flames and it has been demonstrated that ultrafast intense-field laser filamentation-induced nonlinear spectroscopy can be used for simple flame combustion diagnostics [10–20], this research is still in its infancy. Some of the main current advances in the diagnostic analysis of combustion fields using ultrafast intense-field laser filamentation include the verification of the universality of ultrafast intense-field laser filamentation nonlinear spectroscopy for combustion diagnosis of different fuels, the proposed use of ultrafast intense-field laser filamentation-induced excitation phenomena to solve the fluorescence quenching problem in combustion fields, the viable pump–probe approach to measure ultrafast processes of the soot particles in flames with femtosecond time resolution [21], the demonstration of the minimum ignition energy can decrease to the sub-mJ level using an ultrashort femtosecond laser [22], and ultrafast combustion diagnosis using femtosecond filamentation-induced flame nonlinear spectroscopy. This study is intended to systematically investigate the optimization of the fluorescence emissions of ultrafast strong-field laser filament-induced spectroscopy in ethanol flames using the ultrafast laser at the center wavelength of 1,030 nm with changeable pulse energy, duration, and the focusing conditions, with the aim of better application to remote combustion diagnostics.
Experiment setup
As shown in Figure 1, the experiment was conducted with a Yb: the KGW femtosecond laser system (PHAROS, Light Conversion), which delivers 190 fs (FWHM) pluses with a central wavelength at 1,030 nm and a maximum pulse energy of 2 mJ at 10 kHz repetition frequency. The pulse energy and pulse duration of the laser were adjusted, respectively, by an electrically operated attenuator and pulse chirp modulator installed inside the PHAROS laser. The output beam from the laser system was reflected by a dichroic mirror with high reflectivity of 800–1,200 nm and high transmission for UV and visible light at a 45° incidence angle. The femtosecond laser pulse was focused into the flame on an alcohol burner array by the lens (L1) with different focal lengths to generate a bright filamentation as shown in the inset of Figure 1. The alcohol burner array was set on a stage with an adjustable position to ensure that the filament can pass through the core of the flame when changing different focal lengths. The total length of the alcohol burner array with five burner wicks is approximately 40 mm. The fluorescence generated from the filament was collected and collimated in a backward direction by the same lens. After passing through the two dichroic mirrors, the fluorescence in the UV and visible range was focused into an imaging spectrometer with a grating of 1,200 grooves/mm (Kymera 328i, Andor) by an f = 6 cm lens (L2). A damper was used to collect the pump beam and forward emissions such as third harmonic and white light, which was aligned to prevent the forward radiation scattering back to the spectrometer.
Result and discussion
Figure 2 shows the backward fluorescence spectra of with/without the ethanol/air flame generated by the femtosecond laser pulse filament with laser energy of 2 mJ. The spectra are acquisitioned using the spectrometer and the background noise has been subtracted. In addition, the spectra signal of the flame without the interaction of the femtosecond laser mainly originated from the thermal noise and flame light which is two orders of magnitude weaker compared with the fluorescence signal. As the femtosecond filament transmits through the air without ethanol/air flame, the fluorescence spectra mainly show the spectral lines of
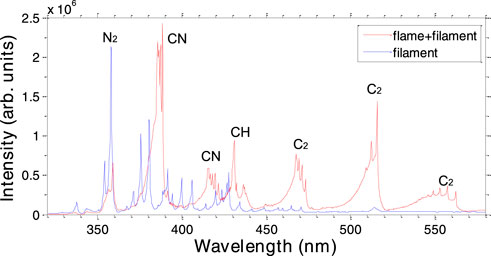
FIGURE 2. Backward fluorescence spectra obtained when the femtosecond filament transmits through air (blue line) and flame (red line).
In addition, to further optimize the free-radical CN, C2, and CH fluorescence emission in the flame, we measured the intensity of the fluorescence emission at different focal lengths. The laser pulse energy and repetition frequency were fixed at 2 mJ and 10 kHz, respectively. As shown in Figure 3, the intensity of the three free-radical fluorescence emissions decreases with the increasing focal length. The conditions of the focal length mainly affect the interaction length and intensity, that is, the longer focal length, longer interaction length, and lower intensity. The results indicated that the optical intensity plays an important role in fluorescence generation compared to the interaction length. In addition, the 388 nm which corresponds to the CN radical fluorescence emission decay the fastest with an increasing focal length, which shows that the CN molecule fluorescence is more sensitive to the focusing condition. Therefore, as a result, a tighter focus can be used to improve the fluorescence intensity and improve the fluorescence emissions.
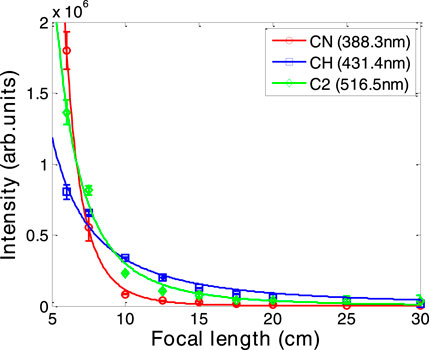
FIGURE 3. Dependence of intensity of the fluorescence from CN (red circle), C2 (green diamond), and CH (blue square) radicals on the focal length. The experimental data are fitted with the power exponential function.
Furthermore, we changed the pulse energy from 0 to 1 mJ by adjusting an electrically operated attenuator installed inside the laser, then the pump laser beam is focused by a spherical lens (f = 75 mm) into the flame. As shown in Figure 4, we measured the intensity of CN radical emission at 388.3 nm (red), that of CH at 431.4 nm (blue), and C2 at 516.5 nm (green) as a function of incident laser energy. It can be seen that the three signals display a superliner dependence on incident laser energy. The experimental data can be well fitted by a power dependence which gives a value of b = 2.078, 2.357, and 3.394, respectively. Obviously, the fluorescence emission of the three free radicals overcomes the quenching effect in combustion diagnosis. In addition, the two-photon effect of the 1,030 nm femtosecond laser pulses can improve the excitation efficiency of C2 radiation emission at 516.5 nm.
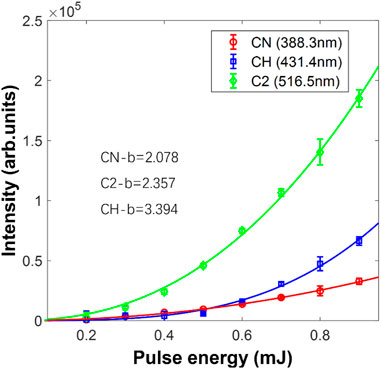
FIGURE 4. Measured (circle) and fitted (solid line) dependence of the emission intensity of CN at 388.3 nm (red), that of CH at 431.4 nm (blue), and C2 at 516.5 nm (green) on the input laser energy.
Last, we changed the chirp of the pump laser pulse by adjusting a pulse chirp modulator installed inside the laser, here; the chirp of the pump laser pulse is defined by the quadratic phase term ϕʺ via the relation
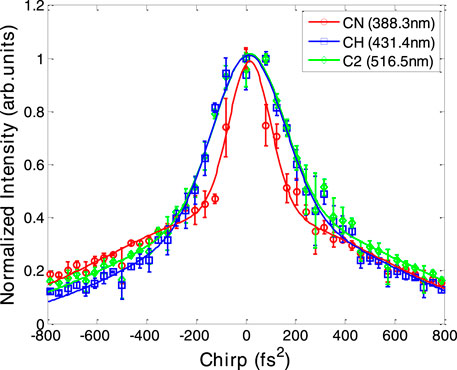
FIGURE 5. Measured (circle) and fitted (solid line) dependence of the emission intensity of CN at 388.3 nm (red), that of CH at 431.4 nm (blue), and C2 at 516.5 nm (green) on the chirp of the pump laser pulse.
In conclusion, we systematically investigate generation of the fluorescence emission of the multiple combustion intermediates from the femtosecond filament with the ultrashort laser pulse at the wavelength of 1,030 nm. By studying the evolution of the fluorescence emission with the focusing conditions, pulse energy, and pulse duration, we proposed an optimizing rule to improve the signal-to-noise ratio of the fluorescence emission intensity in the ethanol/air flame using femtosecond laser filament excitation, which will be promoting a further application of the femtosecond laser pulses to simultaneously monitoring the multiple combustion intermediates.
Data availability statement
The original contributions presented in the study are included in the article/Supplementary Material; further inquiries can be directed to the corresponding authors.
Author contributions
ZTL, JC, and YaC conceived the study and wrote the manuscript. Writing assistance was provided by ZXL and YXC. Optical design support was provided by YeC and YiL.
Funding
This work was supported by the National Natural Science Foundation of China (Grants Nos 11904315, 12004221, and 12074063).
Acknowledgments
We acknowledge the support from the Tianjin Fire Research Institute of the Ministry of Emergency Management. We acknowledge Extreme Optoelectromechanics Laboratory, School of Physics and Materials Science, East China Normal University for optical laboratory and device support.
Conflict of interest
The authors declare that the research was conducted in the absence of any commercial or financial relationships that could be construed as a potential conflict of interest.
Publisher’s note
All claims expressed in this article are solely those of the authors and do not necessarily represent those of their affiliated organizations, or those of the publisher, the editors, and the reviewers. Any product that may be evaluated in this article, or claim that may be made by its manufacturer, is not guaranteed or endorsed by the publisher.
References
1. Liu W. Intensity clamping during femtosecond laser filamentation. Chin J Phys (2014) 52(1-II):465–89. doi:10.6122/CJP.52.465
2. Couairon A, Mysyrowicz A. Femtosecond filamentation in transparent media. Phys Rep (2007) 441:47–189. doi:10.1016/j.physrep.2006.12.005
3. Chin SL, Hosseini SA, Liu W, Luo Q, Théberge F, AköZbek N, et al. The propagation of powerful femtosecond laser pulses in opticalmedia: Physics, applications, and new challenges. Can J Phys (2005) 83:863–905. doi:10.1139/p05-048
4. Xu H, Cheng Y, Chin SL, Sun HB. Femtosecond laser ionization and fragmentation of molecules for environmental sensing. Laser Photon Rev. (2015) 9:275–93. doi:10.1002/lpor.201400208
5. Yao J, Zeng B, Xu H, Li G, Chu W, Ni J, et al. High-brightness switchable multiwavelength remote laser in air. Phys Rev A (Coll Park) (2011) 84:051802. doi:10.1103/PhysRevA.84.051802
6. Rohwetter P, Kasparian J, Stelmaszczyk K, Hao Z, Henin S, Lascoux N, et al. Laser-induced water condensation in air. Nat Photon (2010) 4:451–6. doi:10.1038/nphoton.2010.115
7. Kasparian J, Rodríguez M, Méjean G, Yu J, Salmon E, Wille H, et al. White-light filaments for atmospheric analysis. Science (2003) 301:61–4. doi:10.1126/science.1085020
8. Guandalini A, Eckle P, Anscombe M, Schlup P, Biegert J, Keller U. 5.1 fs pulses generated by filamentation and carrier envelope phase stability analysis. J Phys B: Mol Opt Phys (2006) 39:S257–64. doi:10.1088/0953-4075/39/13/S01
9. Chin SL, Xu HL, Luo Q, Théberge F, Liu W, Daigle JF, et al. Filamentation “remote” sensing of chemical and biological agents/pollutants using only one femtosecond laser source. Appl Phys B (2009) 95:1–12. doi:10.1007/s00340-009-3381-7
10. Li HL, Xu HL, Yang BS, Chen QD, Zhang T, Sun HB. Sensing combustion intermediates by femtosecond filament excitation. Opt Lett (2013) 38:1250–2. doi:10.1364/OL.38.001250
11. Li H, Wei C, Zang H, Xu H, Chin SL. Critical power and clamping intensity inside a filament in a flame. Opt Express (2016) 24:3424. doi:10.1364/OE.24.003424
12. Li H, Chu W, Xu H, Cheng Y, Chin SL, Yamanouchi K, et al. Simultaneous identification of multi-combustion-intermediates of alkanol-air flames by femtosecond filament excitation for combustion sensing. Sci Rep (2016) 6:27340–7. doi:10.1038/srep27340
13. Li S, Li Y, Shi Z, Sui L, Li H, Li Q, et al. Fluorescence emission induced by the femtosecond filament transmitting through the butane/air flame. Spectrochimica Acta A: Mol Biomol Spectrosc (2018) 189:32–6. doi:10.1016/j.saa.2017.08.006
14. Zang HW, Li HL, Su Y, Fu Y, Hou MY, Baltuška A, et al. Third-harmonic generation and scattering in combustion flames using a femtosecond laser filament. Opt Lett (2018) 43:615–8. doi:10.1364/OL.43.000615
15. Li Z, Chen J, Liu Z, Yao J, Yu J, Li Y, et al. Nonintrusive temperature measurement of a combustion field by femtosecond laser-induced third harmonic generation. J Phys B: Mol Opt Phys (2022) 55:075401. doi:10.1088/1361-6455/ac60ad
16. Chu W, Li H, Ni J, Zeng B, Yao J, Zhang H, et al. Lasing action induced by femtosecond laser filamentation in ethanol flame for combustion diagnosis. Appl Phys Lett (2014) 104:091106. doi:10.1063/1.4867503
17. Zhou YS, Wang MM, Lu Y, Silvain JF, Lu YF. Spectroscopic Sensing of O2–C2H2–C2H4 flames for diamond growth using femtosecond filamentation. Cryst Growth Des (2017) 17:3443–9. doi:10.1021/acs.cgd.7b00385
18. Kotzagianni M, Couris S. Femtosecond laser induced breakdown for combustion diagnostics. Appl Phys Lett (2012) 100:264104. doi:10.1063/1.4731781
19. Baudelet M, Guyon L, Yu J, Wolf JP, Amodeo T, Fréjafon E, et al. Spectral signature of native CN bonds for bacterium detection and identification using femtosecond laser-induced breakdown spectroscopy. Appl Phys Lett (2006) 88:063901. doi:10.1063/1.2170437
20. Yuan S, Wang T, Lu P, Leang Chin S, Zeng H. Humidity measurement in air using filament-induced nitrogen monohydride fluorescence spectroscopy. Appl Phys Lett (2014) 104:091113. doi:10.1063/1.4867267
21. Zang H, Fu Y, Hou M, Li H, Yamanouchi K, Xu H. Ultrafast swelling and shrinking of soot in alkanol–air flames induced by femtosecond laser filamentation. Combustion and Flame (2020) 212:345–51. doi:10.1016/j.combustflame.2019.11.009
Keywords: femtosecond filament, combustion field, combustion intermediates, fluorescence emissions, combustion diagnostics
Citation: Li Z, Chen J, Liu Z, Li Y, Chu Y, Chen Y and Cheng Y (2022) Free-radical fluorescence emissions induced by 1,030 nm femtosecond laser filamentation in ethanol flame. Front. Phys. 10:1029954. doi: 10.3389/fphy.2022.1029954
Received: 28 August 2022; Accepted: 13 September 2022;
Published: 30 September 2022.
Edited by:
Weifeng Yang, Hainan University, ChinaReviewed by:
Huailiang Xu, Jilin University, ChinaYi Liu, University of Shanghai for Science and Technology, China
Copyright © 2022 Li, Chen, Liu, Li, Chu, Chen and Cheng. This is an open-access article distributed under the terms of the Creative Commons Attribution License (CC BY). The use, distribution or reproduction in other forums is permitted, provided the original author(s) and the copyright owner(s) are credited and that the original publication in this journal is cited, in accordance with accepted academic practice. No use, distribution or reproduction is permitted which does not comply with these terms.
*Correspondence: Jinming Chen, am1jaGVuQHBoeS5lY251LmVkdS5jbg==; Zhaoxiang Liu, enhsaXVAcGh5LmVjbnUuZWR1LmNu; Ya Cheng, eWEuY2hlbmdAc2lvbS5hYy5jbg==