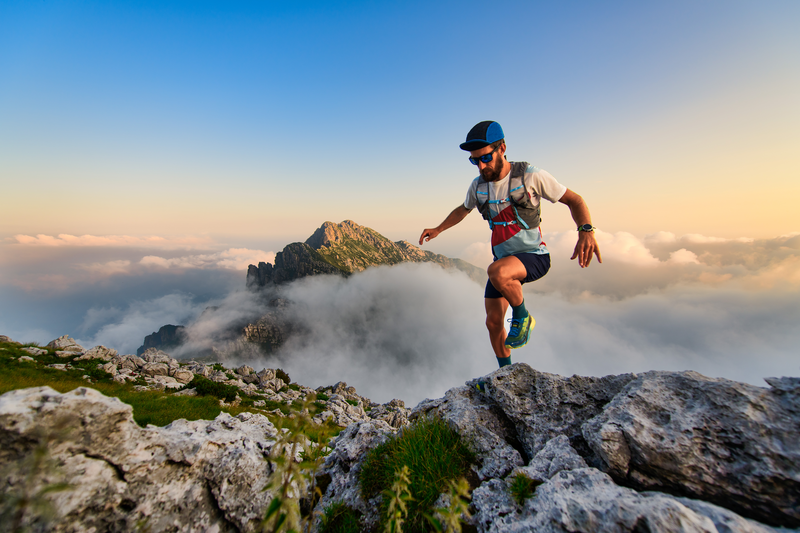
95% of researchers rate our articles as excellent or good
Learn more about the work of our research integrity team to safeguard the quality of each article we publish.
Find out more
BRIEF RESEARCH REPORT article
Front. Phys. , 29 September 2022
Sec. Optics and Photonics
Volume 10 - 2022 | https://doi.org/10.3389/fphy.2022.1013102
This article is part of the Research Topic Physical Model and Applications of High-Efficiency Electro-Optical Conversion Devices, volume II View all 11 articles
Electronic paper display has received increasing attention due to its outstanding properties of wide viewing angle, bistable state and lower energy consumption. Among various electronic paper display technologies, electrowetting e-paper (EWD) is the most promising as it can achieve full-color display by using stacked layers or RGB color filter. However, the EWD is faced with several challenges, including poor color brightness, low contrast ratio and small color gamut. To improve the performance of the EWD, we proposed a new configuration of EWD consisting of three independent PCLC reflection films based on the cholesteric liquid crystal materials. We designed and experimentally fabricated three types of reflection films, and then added the PCLC films to the tri-layered EWD device. We experimentally characterized the reflectivity and color gamut of the sample in the dark room. It is found that the experimental results and simulation results match with each other. It is demonstrated that with the PCLC films, the reflectivity is improved by 20%, while the color gamut is improved by 80%, which is sufficient for the daily display demand of E-paper. The proposed EWD device containing PCLC reflection films provide a new strategy to improve the brightness and color gamut of current EWD device, and is promising for realizing the full-color E-paper display.
Electrowetting display (EWD), which is based on the quick manipulation of microfluid, is a type of reflective electronic paper display technology [1]. Compared with the commercialized electronic paper product of electrophoresis display (EPD), EWD has the same advantages, including low energy consumption, visual healthy and flexibility. Moreover, EWD is capable of realizing “color” and “video” display simultaneously, which is rather difficult to achieve by other reflective display technologies [2]. Therefore, EWD has become one of the mainstreams of electronic paper display in recent decade.
The concept of EWD was pioneered by G. Beni et al. [3, 4] in 1981, who used the electrowetting effect to manipulate the liquid movement in the pixel structure, so as to change the optical spatial coherence in the pixel, and realize display by switching between white and colored state. Fundamentally, electrowetting is based on electrocapillarity, which was first discovered by Gabriel Lippmann in 1875 and raised the famous Young-Lippmann equation [5]. In 1993, Berge proposed the concept of electrowetting based on dielectric layer [6], which became the basis of modern electrowetting devices [7], such as displays, lenses [8], microfluidic platform [9], heat transfer [10], energy conversion [11] etc. In 2003, Robert A. Hayes reported a video-speed electronic paper based on electrowetting [1], and started the research of EWD technology officially.
So far, the performance of EWD devices have been greatly improved in the past 2 decades, including the reflectivity [12], response time [13] and reliability [14, 15]. EWD prototypes with high brightness and video-speed has also been demonstrated. However, the EWD color display is still unsatisfactory, due to the difficulties in achieving high brightness and high color gamut simultaneously [16]. For reflective color display, the additive color mixing with red (R), green (G) and blue (B) color filter is unfavorable, since each of the color subpixels absorbs roughly 2/3 of the incident white light and thus reduce the brightness/gamut performance [17]. By contrast, subtractive color, mixing with vertical cyan (C), magenta (M) and yellow (Y) stack, is more promising for reflective EWD with wide color gamut while maintaining high reflectivity [18–20]. As reported by H. You et al. [18], the CMY color EWD layers can be switched and are responsible for RGB light control independently in the vertical direction. By controlling the opening rate of each layer, the three primary colors of different gray scales can be obtained, and finally achieve full color display.
Currently, although the multi-layered EWD configuration can achieve high brightness in principle, however, it also causes evident light loss due to the excessive number of functional layers and limit the brightness of the device ultimately. To reduce the light loss, this study report a new approach to enhance the optical performance of the EWD device. Selective reflection films made of polymer cholesteric liquid crystal (PCLC) were added under each layer to reflect the light that has been modulated. The reflectance and color gamut of this new EWD device structure were studied by experiment and simulation, and both results show that this method greatly enhanced the reflectivity and color gamut of tri-layers EWD.
The full color tri-layered EWD device is formed by stacking three monolayer EWD devices, which operates independently and regulates part of the visible light. The working principle of monolayer EWD device is shown in Figure 1A. Specifically, when in the off state, the color ink spreads at the bottom of the pixel and absorbs the corresponding band of light, the pixel presents the color of the ink. When in the on state, the wettability and the contact angle between water and insulator are changed after applying voltage to the upper and lower electrodes. The ink is pushed to the corner of the pixel, and thus the area without ink becomes transparent. Moreover, the opening rates of the oil which depends on the contact angle θ near the three-phase contact line, can be achieved by adjusting the voltage and calculated by the Young-Lippmann equation as shown in Eq. 1.
where
FIGURE 1. Schematic diagram of EWD device: (A) the working principle of EWD technology, (B) the vertical subtractive color mixing mechanism, (C) the basic structure and geometry parameters of tri-layers EWD pixels (here, PW: pixel wall, RF: reflector film), (D) selective reflection principle of PCLC films.
In tri-layered EWD device, CMY oils are used to absorb RGB light respectively. As shown in Figure 1B, when the visible light passes through the layer consisting of Y oil, the blue light is absorbed, whereas the red and green light pass through the Y oil layer. Subsequently, the transmitted light continues to pass through the M oil layer, and the green light is absorbed. In this process, only the red light can pass, and is absorbed by Coil finally. Therefore, when the three layers of ink are spread, the pixels show black. By contrast, when the three layers of ink are contracted, the visible light can pass through the open area, thus resulting in white pixels. It can be inferred that the modulation of the three ink layers on the RGB tri-color light is independent. Moreover, by controlling the open rate of each layer of ink, the absorption of RGB light can be adjusted, and the full-color display can finally be realized.
However, it should be emphasized that the aforementioned mechanism to realize color display suffers from strong losses in the process of light passing through the entire device and reflecting back in a three-layer superimposed EWD device, although in principle other ink layers have no effect on the light outside the absorption spectrum. Taking the blue light as an example, the blue light in the reflected spectrum is regulated by the uppermost yellow ink layer, which has already been completed by the first layer of EWD devices. However, the regulated blue light still needs to pass through the lower two layers of the device to reach the reflective film and again go through the three layers of the device before it comes to human eyes. In this process, the intensity of the blue light is weakened by the absorption of the material, the reflection of the interface and the diffraction of the structure, which eventually leads to a significant reduction in the reflectivity of the blue light.
In order to mitigate this problem, we proposed to place a selective reflective layer under each single-layer device, which helps to reflect the light modulated by the upper ink layer as soon as possible, as shown in Figure 1C. Specifically, a reflection layer (RF-B) corresponding to the band of blue light is added below the yellow ink layer that regulates the blue light. The RF-B layer directly reflects the modulated blue light to prevent the loss of blue light, which may be induced by the structure of the following two layers of devices. Similarly, RF-G and RF-R are also effective in preventing the loss of green and red light, respectively. Thereby, the proposal based on adding three reflective layers are promising in improving the overall reflectivity of the device.
In order to realize the selective reflection of the visible spectrum, PCLC films were employed as the dichromatic reflector in this work. Cholesteric liquid crystal (CLC) is a kind of one-dimensional photonic material with a chiral-spiral structure with Bragg reflective properties, which reflect circularly polarized light in the same direction as its chiral spiral (plus references). According to the Bragg reflection, the reflected band λo of CLC is directly related to its pitch P and refractive index, which can be described by following formula:
where
P is the pitch of the CLC, which is the distance at which the liquid crystal molecule rotates in orientation for one circle, as shown in Figure 1D. The reason for choosing the CLC material in this study is that it can adjust the reflection band by adjusting the p value. In principle, the value of P can be realized by adjusting the concentration of the chiral dopant in the liquid crystal material. The pitch of the CLC can be determined by Eq. 4
where c is the concentration of chiral dopant, and HTP is the helical twisting power of chiral dopant in this nematic liquid crystal. Therefore, the reflection band can be adjusted by adjusting the concentration of the dopant.
Another reason for employing the CLC material in this study is that the CLC with optical birefringence has a relatively wide reflective bandwidth, which is compatible with the absorption spectrum of CMY oil. The reflected bandwidth Δλ of the CLC is related to its birefringence Δn, which is calculated as follows:
And
In this study, the polymerizable liquid crystal monomer material is used as the main body to prepare PCLC film, which can be directly attached to the surface of the EWD substrate. Moreover, since CLC materials can only reflect circular polarized light in the same direction as their chiral helix, two PCLC films with right-handed (RH) and left-handed (LH) rotation respectively are prepared. As shown in Figure 1D, after the incident light passes through the RH PCLC, the RH-CPL (circularly polarized light) is reflected, and the LH-CPL transmitted and is reflected again by the PCLC of the second layer LH, so that the total reflection within a certain bandwidth can be realized. Hence, in this article, PCLC was used to fabricate the RGB tri-color light reflective films that match with the oil absorption spectrum so as to improve the reflectivity of the EWD device.
In order to demonstrate the proposed prototype consisting of tri-layer full-color EWD devices and verify the effect of PCLC reflective film on enhancing reflectivity and color gamut, both of simulation and experimental studies are conducted here.
The tri-layer EWD device is fabricated by coupling three separate EWD devices together, as shown in Figure 2A. The detailed fabrication process can be found in our previous publication [20]. The pixel size of the sample is 165 μm × 165 μm with 150 μm working area and 15 μm pixel wall width. The height of the pixel wall grid composed of photoresist SU8 is 5 μm. Amorphous fluoropolymer (FP) Teflon AF1600X is used as the insulator layer. The colored oils are prepared via dissolving self-synthesized dyes in oily solvents. The transmittance spectra of CMY oils are measured and shown in Figure 2B. It can be seen that these oils absorb corresponding RGB light and have good transmittance in other wavelength. The oil and water are filled into the cells with 50 μm height and then the whole device is sealed. After bonding FPC, the three EWD layers can be driven separately.
FIGURE 2. Fabrication of tri-layer EWD device and PCLC film: (A) fabrication process of tri-layer EWD device, (B) transmittance spectrum of CMY oil, (C) LC monomer materials for PCLC films and (D) transmittance spectrum of PCLC films.
Figure 2C depicts the materials used for producing the PCLC film by photo-copolymerizing three liquid crystalline monomers. The concentration of three monomers is adjusted to have suitable ne and no. In order to adjust the selective reflection wavelength, we varied the concentration and chirality of chiral dopant S/R 5011. Moreover, inhibitor was adopted to avoid premature polymerization. The constituents were mixed by dissolving in the dichloromethane, which was evaporated subsequently. The liquid crystal mixtures were capillary filled into the cells with 5 μm gap and parallel alignment layer on both internal surface at 65°C for 15 min. The cells were then cooled down to 50°C for another 15 min before polymerization to achieve better alignment. The samples were polymerized by UV light source at 50°C, post-cured by heat at 125°C for 15 min to ensure full cure of the acrylate monomers, and then cool down to room temperature on the hot plate slowly. Finally, the cells were opened so to take out the PCLC films.
In this work, six PCLC films, which were designed to reflect the RH-CPL and LH-CPL RGB light respectively, were fabricated. Figure 2D shows the transmission spectra of these six films, the RH and LH films after stacking. It reveals that the reflection band of PCLC films fits exactly with the absorption band of CMY. In order to maintain the color gamut of the EWD device, the reflected bandwidth is made slightly narrower than the absorption bandwidth of the ink. Moreover, it can be seen that the reflectivity of the film after stack reaches more than 95% in the band where light should be reflected. On the contrary, the transmittance is higher than 90% in the band where light can pass. These PCLC films are added between the EWD layers, as shown in Figure 1C.
An optical model of tri-layer EWD device is built in software to simulate the effect of adding PCLC films on the reflectivity and color gamut of the device. The side view and equiaxial view of the optical model are shown in Figure 3A. To save computing resource, the model is composed of an array of 50 × 50 pixels. The geometric and optical parameters of each functional layer are set according to the sample in the experiment, where the thickness and refractive index of each layer are shown in Figure 3C. The absorption spectrum of CMY oil and the reflection spectrum of PCLC films are set according to the results in Figures 2B,D. In the simulation model, the reflector used in the model is the standard Lambert diffuse reflective surface, and the light source is the D65 standard white parallel light source, which shines on the device at an angle of 45°. A monitor is set vertically above the device as a sensor to record the light intensity and color coordinates of the reflected light. By establishing the switching states of different membrane layers, the reflected light intensity and the reflected light color coordinates of the device when displaying six primary colors (namely RGB, CMY, black and white) are simulated and calculated.
FIGURE 3. Optical simulation and characterisation of tri-layer EWD device: (A) optical simulation model, (B) optical performance test system, (C) optical and geometrical parameter of functional layers in EWD device.
In order to optically characterize the performance of EWD devices, a system (Figure 3B) was built in the dark room, according to the electronic paper display measurement standard IEC-62679. In the test system, a collimated D65 light source with divergence angle less than 5° and illuminance higher than 65,000 Lux was used to illuminate on the center of the display screen under test. The angle between the lighting direction and the measured EWD device surface is 45°. To measure the brightness and chromaticity coordinates of the six primary colors on EWD device, a colorimeter (Topcon, SR-UL1R) was putted perpendicular to the surface of the EWD device with a measuring distance of 50 cm.
The reflectivity and color gamut of the tri-layer EWD device are measured to evaluate the effect of PCLC films on the optical performance of the EWD device. In principle, the reflectivity of EWD device is defined as the ratio R between the reflectance of the device and the standard reflector, which can be calculated by the following equation:
where Icolor is the intensity of the reflected light of the EWD device under color states, Ireflector is the intensity of the reflected light of standard reflector.
Generally, the color gamut refers to the range of colors that the display device can show. For subtractive color mixing implemented by CMY, the color that can be achieved is the area enclosed by a hexagonal shape on the chromaticity map connected by the color coordinates of the CMYRGB six primary colors. The NTSC color gamut coverage of the display GEWD is usually defined as the ratio between the hexagonal area
The value of the SNTSC is 0.1582, and the color display gamut of the device can be calculated by testing the chromaticity coordinates of the display in the C, M, Y, R, G, B primary colors in the CIE1931 chromaticity space.
Figure 4A depicts the simulated reflectivity of the color state of the tri-layer EWD device with and without the PCLC films. The experimentally captured reflectivity of the color state is shown in Figure 4B. They indicate that the reflectivity of the device can be improved effectively by using the PCLC films. Moreover, some differences between simulation and experimental results can also be identified, no matter whether the device contains the PCLC films or not. This is mainly caused by the model simplification, as the actual effect is slightly worse than the simulation effect. However, the trend of the change in the simulation and experimental data is consistent with each other, hence we think the results are reliable. Similar differences are also found in the simulated and experimentally captured color gamut, as shown in Figures 4C,D.
FIGURE 4. Simulation and experimental results of tri-layers full-color EWD device with and without PCLC films: (A) simulation results of reflectivity, (B) experimental results of reflectivity, (C) simulation result of color gamut, (D) experimental result of color gamut.
As EWD is a type of reflective display technology, the reflectivity which determines the display brightness is an important parameter. It can be seen from both the simulation and experimental results that the reflectivity of the device has been significantly improved by adding the PCLC films. Specifically, the most significant improvement is the reflectivity of the cyan. For cyan display, it is necessary to open the ink of the upper Y and M layers and close the ink of the lower C layer. In such conditions, the red light is absorbed while the blue light and green light are reflected. This is realized by using the two reflective layers of RF-B and RF-G, which help to reflect the blue light and green light subsequently before they go through the tri-layer device. Therefore, the loss of the blue light and green light is greatly minimized, thus significantly improving the reflectivity of cyan. Similarly, the reflectivity of the blue and green has also been significantly improved. Regarding to the blue, it is necessary to absorb the green and red light, while reflect the blue light. This is accomplished by using the RF-B reflective layer to reflect the blue light after it passing through the uppermost device, thus saving the blue light to the greatest extent. For green, it is necessary to absorb the blue and red light, while reflect the green light. It can be seen from Figure 2B that the bottom cyan ink has a certain absorption of green light. Hence, when the RF-G reflective layer does not need to pass through the lowest layer of the device, the green light reflectance is improved more than the blue light. Meanwhile, the results show that the least increase in reflectivity is the red, due to the fact that the cyan ink that regulates the red light is located in the lowest layer, and the red light must pass through the three-layer device anyway, so the presence or absence of RF has little effect on the reflectivity of the red light. Overall, after the addition of PCLC films, the overall reflectivity of the device has been increased by more than 30%, mainly from the increasement of the reflectivity of the blue light and green light. Therefore, by adding a selective reflective layer between the display layers, it is indeed possible to greatly improve the reflectivity of the device by reducing the invalid light loss when light passes through the three layers of the device.
The PCLC films also improves the color gamut of tri-layer EWD device significantly. Without the films, the color gamut of the device is only 32% NTSC in CIE 1931 color space in the simulation and 30% NTSC in the test, which is much lower than the theoretical color gamut that can be achieved through these CMY oils. This can also be attributed to the material absorption caused by the multi-layers structure. Due to the loss of the blue light, the color coordinates of the blue color deviate into to the magenta region. Similarly, due to the loss of the green light, the color coordinates of green color deviate into yellow region. The low reflectivity of cyan results in color coordinates of cyan color deviate towards the central white region. All these make the color gamut of the device greatly reduced.
With the help of the PCLC films, the EWD device acquires a much wider color gamut up to 60% NTSC in the simulation and 54.1% NTSC in the test, mainly due to the significant increasement in the reflectivity of the blue and green light. It can be inferred that the purity of the color region has also been improved. Moreover, the results also clearly indicate that the green-blue area is much wider. The blue color shifts to the right region and the green color became more distinct, due to the enhanced reflection of the blue and green light. For the cyan, its color coordinates are pulled significantly towards the periphery by the increased reflectivity of blue and green light. For the yellow, magenta and red, the color coordinates are almost kept unchanged, mainly because the reflectivity of the red is well maintained. Generally, similar trend can be found in both the simulation and experimental results, in spite of slight difference in the shape of the hexagon. This difference mainly exists in the relative position of the magenta, red and blue. In principle, the six corners of the CMY tri-layer EWD device’s gamut hexagon should be protruding outward, which matches with the experimental results. However, certain deviation can be identified in the simulation results of the magenta and blue color coordinates, which is attributed to the error in the color coordinates when fitting the CIE with limited number of light bars, the simulation results in the fit CIE.
It can be inferred from the above results that the addition of PCLC films with selective reflection characteristics greatly improves the reflectivity of the blue light and green light, via directly reflecting the blue light and green light without passing through the three layers of EWD devices. Both the reflectivity and the color gamut of the tri-layer EWD device have been significantly improved, which can help to mitigate the problems in the current color EWD display device.
We proposed a novel full-color reflective electrowetting display device, consisting of three independent PCLC reflection films that can reflect the red, green and blue circularly polarized light respectively. PCLC films embedded in the device can effectively reduce the reflectance and gamut value. The results of simulation and experiment are in good agreement. It is demonstrated that with the PCLC films, the reflectivity is improved by 20%, while the color gamut is improved by 80%, which is sufficient for the daily display demand of E-paper. The proposed EWD device containing PCLC reflection films provide a new strategy to improve the brightness and color gamut of current EWD device, and is promising for realizing the full-color E-paper display. It is of great significance for the promotion and application of full-color video electro-wetting electronic paper display devices.
The original contributions presented in the study are included in the article/supplementary material, further inquiries can be directed to the corresponding authors.
All authors contributed to the study conception and design. Conceptualization: XZ and DY; Data curation: BZ, XL and LX; Methodology: XL and HY; Writing-original draft preparation: BZ and XZ; Writing-review and editing: BT and HY; Supervision: Dong Yuan; Funding acquisition: BT and DY.
This research was funded by National Key R&D Program of China (2021YFB3600602), Science and Technology Program of Guangzhou (Nos. 202201,010351, 2019,050001), Natural Science Foundation of Guangdong Province (Nos. 2020A1515010715, 2021A1515010623), Program for Chang Jiang Scholars and Innovative Research Teams in Universities (No. IRT_17R40), Program for Guangdong Innovative and Enterpreneurial Teams (No. 2019BT02C241). This work also supported by the Guangdong Provincial Key Laboratory of Optical Information Materials and Technology (No. 2017B030301007), Guangzhou Key Laboratory of Electronic Paper Displays Materials and Devices (201705030007) and the 111 Project.
We would like to express our thanks to the Science and Technology Program for help identifying collaborators for this work. We also thanks to Guangdong Provincial Key Laboratory of Optical Information Materials and Technology for providing sufficient equipment supplements.
The authors declare that the research was conducted in the absence of any commercial or financial relationships that could be construed as a potential conflict of interest.
All claims expressed in this article are solely those of the authors and do not necessarily represent those of their affiliated organizations, or those of the publisher, the editors and the reviewers. Any product that may be evaluated in this article, or claim that may be made by its manufacturer, is not guaranteed or endorsed by the publisher.
1. Hayes RA, Feenstra BJ. Video-speed electronic paper based on electrowetting. Nature (2003) 425(6956):383–5. doi:10.1038/nature01988
2. Feenstra B, Hayes R, Van Dijk R, Boom R, Wagemans M, Giraldo A, et al. (2006).Electrowetting-based displays: bringing microfluidics alive on-screen. in: IEEE International Conference on Micro Electro Mechanical Systems: 22-26 Jan. 2006: Turkey
3. Beni G, Hackwood S. Electro-wetting displays. Appl Phys Lett (1981) 38(4):207–9. doi:10.1063/1.92322
4. Beni G, Tenan MA. Dynamics of electrowetting displays. J Appl Phys (1981) 52(10):6011–5. doi:10.1063/1.329822
5. Lippman G. Relations entre les phenomenes electriques et capillaries. Ann de Chim de Physique (1875) 5(11):494–549.
6. Berge B. Electrocapillarite et mouillage de films isolants par l'eau. Comptes Rendus De Lacademie Des Sci Paris Serie (1993) 317(2):157–63.
7. Mugele F, Baret J-C. Electrowetting: from basics to applications. J Phys : Condens Matter (2005) 17(28):705–74. doi:10.1088/0953-8984/17/28/r01
8. Grilli S, Miccio L, Vespini V, Finizio A, De Nicola S, Ferraro P. Liquid micro-lens array activated by selective electrowetting on lithium niobate substrates. Opt Express (2008) 16(11):8084–93. doi:10.1364/oe.16.008084
9. Mark D, Haeberle S, Roth G, von Stetten F, Zengerle R. Microfluidic lab-on-a-chip platforms: requirements, characteristics and applications. Chem Soc Rev (2010) 39(3):1153–82. doi:10.1039/b820557b
10. Sur A, Lu Y, Pascente C, Ruchhoeft P, Liu D. Pool boiling heat transfer enhancement with electrowetting. Int J Heat Mass Transfer (2018) 120:202–17. doi:10.1016/j.ijheatmasstransfer.2017.12.029
11. Krupenkin T, Taylor JA. Reverse electrowetting as a new approach to high-power energy harvesting. Nat Commun (2011) 2(1):448. doi:10.1038/ncomms1454
12. Wu H, Hayes RA, Li F, Henzen A, Shui L, Zhou G. Influence of fluoropolymer surface wettability on electrowetting display performance. Displays (2018) 53:47–53. doi:10.1016/j.displa.2018.02.002
13. Dou Y, Tang B, Groenewold J, Li F, Yue Q, Zhou R, et al. Oil motion control by an extra pinning structure in electro-fluidic display. Sensors (2018) 18(4):1114. doi:10.3390/s18041114
14. Zhou R, Ye Q, Li H, Jiang H, Tang B, Zhou G. Experimental study on the reliability of water/fluoropolymer/ITO contact in electrowetting displays. Results Phys (2019) 12:1991–8. doi:10.1016/j.rinp.2019.02.037
15. Dou Y, Chen L, Li H, Tang B, Henzen A, Zhou G. Photolithography fabricated spacer arrays offering mechanical strengthening and oil motion control in electrowetting displays. Sensors (2020) 20(2):494. doi:10.3390/s20020494
16. Kuo SW, Chang YP, Cheng WY, Lo KL, Lee DW, Lee HH, et al. 34.3: Novel development of multi-color electrowetting display. SID symposium. Dig Tech Pap (2010) 40(1):483–6.
17. Giraldo A, Aubert J, Bergeron N, Li F, Mvd W, Massard R, et al. 34.2: Transmissive electrowetting-based displays for portable MultiMedia devices. J Soc Inf Disp (2010) 18(4):317–25. doi:10.1889/jsid18.4.317
18. You H, Steckl A. Three-color electrowetting display device for electronic paper. Appl Phys Lett (2010) 97(2):023514. doi:10.1063/1.3464963
19. Wei-Yuan L, Cheng W, Yung-Hsiang C, Liang C-C. A stacking color electrowetting display for the smart window application. SID Int Symp Dig Tech Pap (2011) 42(1):78–81. doi:10.1889/1.3621448
Keywords: electrowetting display, electronic paper, full-color display, polymer cholesteric liquid crystal, color gamut, reflectance
Citation: Zhang B, Liao X, Xie L, Tang B, Zhou X, Ye H and Yuan D (2022) Reflectivity enhancement of full color tri-layer electrowetting display with polymer cholesteric liquid crystal films. Front. Phys. 10:1013102. doi: 10.3389/fphy.2022.1013102
Received: 06 August 2022; Accepted: 22 August 2022;
Published: 29 September 2022.
Edited by:
Qiang Xu, Nanyang Technological University, SingaporeCopyright © 2022 Zhang, Liao, Xie, Tang, Zhou, Ye and Yuan. This is an open-access article distributed under the terms of the Creative Commons Attribution License (CC BY). The use, distribution or reproduction in other forums is permitted, provided the original author(s) and the copyright owner(s) are credited and that the original publication in this journal is cited, in accordance with accepted academic practice. No use, distribution or reproduction is permitted which does not comply with these terms.
*Correspondence: Xin Zhou, NzM1NTg4Mzg1QHFxLmNvbQ==; Huapeng Ye, eWVocEBtLnNjbnUuZWR1LmNu; Dong Yuan, eXVhbmRvbmdAbS5zY251LmVkdS5jbg==
†These authors contributed equally to this work and share first authorship
Disclaimer: All claims expressed in this article are solely those of the authors and do not necessarily represent those of their affiliated organizations, or those of the publisher, the editors and the reviewers. Any product that may be evaluated in this article or claim that may be made by its manufacturer is not guaranteed or endorsed by the publisher.
Research integrity at Frontiers
Learn more about the work of our research integrity team to safeguard the quality of each article we publish.