- 1Wenzhou Key Laboratory of Micro-nano Optoelectronic Devices, Wenzhou University, Wenzhou, China
- 2Key Laboratory of Optoelectronic Materials Chemistry and Physics, Fujian Institute of Research on the Structure of Matter, Chinese Academy of Sciences, Fuzhou, China
Diode end-pumped Nd,La:CaNb2O6 self-Raman laser with acousto-optic Q-switching was successfully demonstrated for the first Stokes wave generation at 1,174 nm. A 1.0 at.% Nd3+ and 1.0 at.% La3+-doped CaNb2O6 crystal in dimensions 3 × 3 × 14.3 mm3 was used as the self-Raman laser crystal. Doping 1 at.% La3+ ions into this crystal could subdue the fluorescence quenching caused by cross-relaxation between Nd3+ ions and finally improve the laser output performance. Under the incident pump power of 9.9 W, the first Stokes wave at 1,174 nm with a maximum output power up to 928 mW was obtained, with the diode to Stokes conversion efficiency of about 9.4%. The results show that the Nd,La:CaNb2O6 is also a promising self-Raman crystal for efficient fundamental and Raman laser operation.
Introduction
As an efficient wavelength conversion technology, stimulated Raman scattering has received extensive attention in recent years. Many solid-state materials have been found as promising Raman gain media for all-solid-state Raman laser design [1–8]. A class of Raman crystals that can be used as excellent laser crystals by doping active ions have been widely concerned, such as vanadate [9–11] and tungstate crystals [12–15]. In addition to these commonly used Raman crystals, calcium niobate (CaNb2O6) is one of the excellent Raman crystals with strong Raman gain and can also be used as an excellent laser crystal by doping rare earth ions.
As early as 1963, Ballman et al. grew CaNb2O6 crystal by the Czochralski technique [16] and observed the laser phenomenon for the first time in CaNb2O6 doped with Nd3+, Ho3+, Pr3+, Er3+, and Tm3+. In 1969, Kaminskii et al. reported the absorption and luminescence spectra of Nd:CaNb2O6 [17]. As the rare earth ion-doped CaNb2O6 crystal can be used as a laser crystal, many research studies on its fundamental laser have been reported [18–22]. In 1977, Husson et al. found that the CaNb2O6 crystal was also an excellent Raman crystal with a primary Raman shift of 904 cm−1, and the polarized Raman spectra in different directions were measured [23]. In 2004, Silva et al. studied the growth and characterization of CaNb2O6 single crystal fiber and measured its Raman spectra [24]. In 2009, Cheng et al. measured the thermal and mechanical properties of Yb3+-doped CaNb2O6 crystal, and the results show that it has larger thermal conductivity and specific heat than those of other materials such as Yb:LuVO4 and Yb:KLu(WO4)2 [25]. In the same year, Kaminskii et al. reported the high-order stimulated Raman scattering phenomenon of pure and Nd3+-doped CaNb2O6 crystals [26] and realized Nd:CaNb2O6 self-Raman laser output. The average output power of 85 mW at 1,174 nm first Stokes wave with a 904 cm−1 Raman shift was obtained [27]. The abovementioned reports confirmed that the rare earth ion-doped CaNb2O6 crystal can be used as a self-Raman crystal and applied to the generation of the new near-infrared laser. However, both the output power and conversion efficiency of the Nd:CaNb2O6 crystal self-Raman laser were very low. In 2019, the laser performances of Nd:CaNb2O6 and Nd,La:CaNb2O6 were compared. It was found that Nd3+ ion-doped CaNb2O6 crystal would lead to fluorescence quenching caused by cross-relaxation between Nd3+ ions and resulted in low laser efficiency [28]. By doping 1.0 at.% La3+ ions into this crystal could subdue the fluorescence quenching and finally improve the laser output performance. Using this modified crystal, 2.6 W fundamental laser and 310 mW first Stokes laser have been obtained, and both results were much higher than those using Nd:CaNb2O6 crystal, respectively. Therefore, the Nd,La:CaNb2O6 crystal has achieved much better performance and higher conversion efficiency. In summary, the excellent Raman characteristics, thermal effect, and physical properties of CaNb2O6 crystal make it possible to obtain efficient self-Raman operation by co-doped with Nd3+ and La3+ ions.
In this article, watt level first Stokes light at 1,174 nm of a diode end-pumped acousto-optic Q-switched Nd,La:CaNb2O6 self-Raman laser was successfully demonstrated. The fluorescence quenching caused by cross-relaxation between Nd3+ ions was subdued by doping La3+ ions into the Nd:CaNb2O6 crystal. An average output power of 928 mW was obtained under the incident pump power of 9.9 W, with the diode-to-Stokes conversion efficiency up to 9.4%.
Crystal characteristics of Nd,La:CaNb2O6 crystal
CaNb2O6 crystal has an orthorhombic symmetry structure with the Pbcn space group [16]. A lot of literature has proved that the crystal has excellent optical properties, thermal performance, and a large Raman gain coefficient. Some of the crystals were doped with a certain amount (0.58–2 at. %) of Nd3+ to realize laser operation, but Nd3+ as an isovalent ion doped into CaNb2O6 will form defects such as NdCa with a positive charge and VCa'' with a negative charge, leading to the formation of Nd3+ clusters, thus cross-relaxation between neodymium ions will take place [28]. This phenomenon leads to fluorescence quenching related to the transition from 4F3/2 multiple peaks. Therefore, the improvement of laser power and efficiency is limited. In order to solve this problem, we proposed that La3+ ions were doped into Nd:CaNb2O6 to form Nd3+-La3+ buffer clusters, so that more efficient lasers were successfully obtained in co-doped Nd,La:CaNb2O6 crystal. Therefore, in order to obtain stable high-power fundamental light and first Stokes light, we used 1.0 at.% Nd3+ and 1.0 at.% La3+ co-doped CaNb2O6 crystal as self-Raman laser crystal in this experiment.
The Raman properties of CaNb2O6 crystals are similar to those of conventional Raman crystals such as vanadates (YVO4 and LuVO4) and tungstates (KLu(WO4)2 and KGd(WO4)2) [29]. The primary Raman shift of the CaNb2O6 crystal is at 904 cm−1 which is similar to that of KGd(WO4)2 at 901 cm−1. The fundamental wavelength at 1,061 nm for the Nd:CaNb2O6 crystal could be shifted to the first Stokes wavelength at 1,174 nm based on the primary Raman shift of 904 cm−1. The Raman gain at 1,064 nm of KGd(WO4)2 is 3.5 cm/GW, whereas it is 2.8 cm/GW for this crystal [27]. However, the thermal conductivity of CaNb2O6 crystal is much higher than that of vanadates and tungstates, which is approx. two times that of KGd(WO4)2 [25, 29]. The good thermal properties will help in achieving higher laser powers.
Experimental setup
The experimental setup for the acousto-optic Q-switched Nd,La:CaNb2O6 self-Raman laser is shown in Figure 1. A fiber-coupled laser diode at 808 nm (fiber core diameter of 200 μm and numerical aperture of 0.22) is re-imaged into the laser crystal with a spot diameter of about 320 μm. A c-cut 1.0 at.% Nd3+ and 1.0 at.% La3+-doped CaNb2O6 crystal with the size of 3 × 3 × 14.3 mm3 was used as the self-Raman laser crystal. The crystal was wrapped in indium foil and mounted on TEC-cooled copper blocks, where the surface temperature was kept at about 20°C, eliminating the thermal generated in the crystal. In order to make the structure more compact, the input side of the self-Raman crystal was worked as a high-reflective cavity mirror with high reflectivity (HR, R>99.9%) for fundamental wavelength at 1,061 nm and first Stokes wavelength at 1,174 nm, and high transmittance (HT, T>95%) coated for pump wavelength at 808 nm. The other side was anti-reflectivity (AR) coated at both 1,061 nm and 1,174 nm. The output mirror was HR (R> 99.9%) coated at 1,061 nm and a partial reflectivity (PR) coated at 1,174 nm (T = 4.1%). The cavity formed by the input side of the self-Raman crystal and the output mirror was shared for both fundamental light and first Stokes light oscillation. The total cavity length was about 6 cm. An acousto-optic Q-switch modulator (AOM, model QS041-10G-GHI2, Gooch and Housego Co.) was installed between the self-Raman laser crystal and the output mirror to achieve pulsed fundamental laser operation.
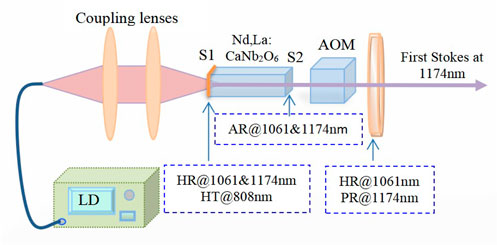
FIGURE 1. Schematic showing the experimental layout of the acousto-optic Q-switched Nd,La:CaNb2O6 self-Raman laser.
Results and discussion
First, the continuous-wave fundamental laser operation at 1,061 nm was investigated with the use of a compact two-mirror cavity. An efficient 1,061 nm laser was obtained using an output mirror with a transmission of 15%. The output power versus the incident pump power and laser spectra of the fundamental laser measured with the maximum incident pump power fixed at 7.8 W are displayed in Figure 2. The center wavelength of fundamental light was 1,061.3 nm, which was measured by the grating monochromator (model Omniλ-500) with a resolution of 0.05 nm. The threshold of the fundamental light output is around 1 W with respect to incident pump power, and the output power increases with the increase of the pump power from the laser threshold. The overall linear relationship is well, and the slope efficiency reaches 48.4%. The maximum continuous-wave output power of 3.3 W is finally achieved at the incident pump power of 7.8 W, and the conversion efficiency reaches 42.3%.
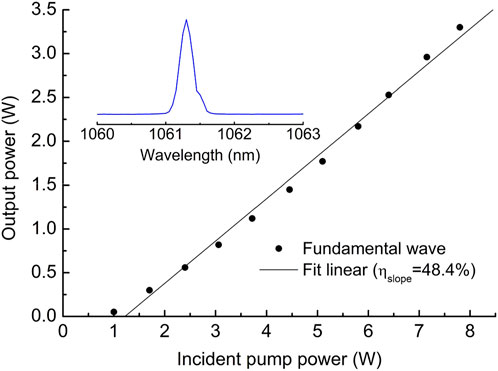
FIGURE 2. Laser spectra (shown in inset) and plot of the power transfer characteristics of the fundamental laser output.
Then, the first Stokes laser operation based on self-Raman conversion with the experimental setup as shown in Figure 1 was studied. With optimization of the focused pump beam spot position in the self-Raman crystal and the repetition rate of the acousto-optic Q-switch modulator, the higher average output power for the first Stokes laser at 1,174 nm was obtained under a higher incident pump power of 9.9 W. The laser spectrum of self-Raman output was also measured by the grating monochromator with the results shown in Figure 3A. The spectrum was scanned from 1,050 to 1,200 nm, and only the wavelength of the first Stokes laser was detected. The center wavelength was 1174 nm, and the line width was about 0.25 nm. As it was converted from the fundamental wave at 1,061.3 nm, the calculated Raman shift was about 904 cm−1, which was well fitted with the measured Raman spectra.
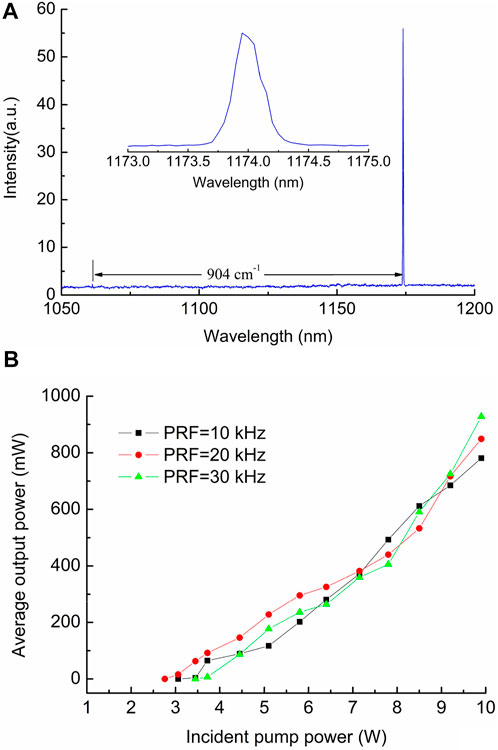
FIGURE 3. Laser spectra (A) and plot of the power transfer characteristics with different pulse repetition frequencies (B) of self-Raman laser output.
Figure 3B shows the relationship between the measured average output power and the incident pump power under different pulse repetition frequencies of 10, 20, and 30 kHz. According to the plot of the power transfer curve, the threshold pump powers were from 2.7 to 3.5 W. As the incident pump power increases, the average output power corresponding to the three repetition frequencies also increases. Under the incident pump power of 9.9 W, a slightly higher average output power of 928 mW was achieved at a pulse repetition frequency of 30 kHz, and the diode-to-Stokes conversion efficiency was 9.4%. As we know, this is the highest first Stokes light output power and conversion efficiency in all studies using the CaNb2O6 crystal as a self-Raman laser crystal.
The laser pulse characteristics of the first Stokes output were detected by an InGaAs free-space photodetector and recorded on a 500 MHz oscilloscope (Model DPO3052B). Under the maximum incident pump power of 9.9 W, the narrowest pulse widths were 14, 17, and 19 ns for different pulse repetition frequencies of 10, 20, and 30 kHz, respectively. The pulse energy and peak power were calculated to be about 31 μJ and 1.63 kW at a full output power of 928 mW, respectively. Though the average output power was only 781 mW for a pulse repetition frequency of 10 kHz, the pulse energy was calculated up to 78 μJ, which was much higher than those of higher pulse repetition frequencies. However, we also see the sub-pulse as the fundamental photons in the cavity re-accumulated and exceeded the Raman threshold again after the principal pulse disappeared at the low pulse repetition frequency. Figure 4 shows the temporal pulse profile and train for the first Stokes laser at the pulse repetition frequency of 10 kHz. The sub-pulse generated also caused instability in the pulse train. We calculated the amplitude fluctuation up to ±9% at 10 kHz pulse repetition frequency.
Conclusion
In conclusion, we have successfully generated a 1,174-nm first Stokes wave with high output power and efficiency in an acousto-optic Q-switched Nd,La:CaNb2O6 self-Raman laser. A c-cut Nd,La:CaNb2O6 (1.0 at. % Nd3+ and 1.0 at. % La3+ doped) in dimensions 3 × 3 × 14.3 mm3 was used as a self-Raman laser crystal. Nd:CaNb2O6 crystal laser performance was improved by doping La3+ ions into this crystal to subdue fluorescence quenching caused by cross-relaxation between Nd3+ ions. Under the incident pump power of 9.9 W, the first Stokes light at 1,174 nm with a maximum output power up to 928 mW and peak power of 1.63 kW was obtained at a pulse repetition frequency of 30 kHz, with the diode-to-Stokes conversion efficiency of about 9.4%. As a new self-Raman crystal, the higher Raman laser operation can be expected by further optimizing Nd3+ and La3+ ion concentration and crystal growth conditions. The results show that the Nd,La:CaNb2O6 is also a promising self-Raman crystal for efficient fundamental and Raman laser operation.
Data availability statement
The original contributions presented in the study are included in the article/Supplementary material; further inquiries can be directed to the corresponding author.
Author contributions
YZ was responsible for the investigation and experiment. WM was responsible for the experiment and writing. XG was responsible for crystal growth and performance analysis. XJ and ZL were responsible for data curation and editing. YH was responsible for the experiment, data curation, and editing. YD was responsible for conceptualization, methodology, writing, and funding acquisition. All authors contributed to the final manuscript.
Funding
This study was supported by the National Natural Science Foundation of China (62075167, 62275200 and 61875199) and the Leading Class of “High-Level Innovation Team” of Wenzhou City.
Conflict of interest
The authors declare that the research was conducted in the absence of any commercial or financial relationships that could be construed as a potential conflict of interest.
Publisher’s note
All claims expressed in this article are solely those of the authors and do not necessarily represent those of their affiliated organizations, or those of the publisher, the editors, and the reviewers. Any product that may be evaluated in this article, or claim that may be made by its manufacturer, is not guaranteed or endorsed by the publisher relationships that could be construed as a potential conflict of interest.
References
1. Zhao H, Dai SB, Zhu SQ, Yin H, Li Z, Chen ZQ. Multifunctional optical crystals for all-solid-state Raman lasers. Crystals (2021) 11(2):114. doi:10.3390/cryst11020114
2. Duan Y, Zhu H, Wang H, Zhang Y, Chen Z. Comparison of 1.15μm Nd:YAG\KTA Raman lasers with 234 and 671 cm-1 shifts. Opt Express (2016) 24(5):5565–71. doi:10.1364/OE.24.005565
3. Gao FL, Zhang XY, Cong ZH, Liu ZJ, Chen XH, Qin ZG, et al. Tunable Stokes laser based on the cascaded stimulated polariton scattering and stimulated Raman scattering in RbTiOPO4 crystal. Opt Lett (2020) 45(4):861–4. doi:10.1364/OL.383885
4. Duan Y, Zhang J, Zhu H, Zhang Y, Xu C, Wang H, et al. Compact passively Q-switched RbTiOPO4 cascaded Raman operation. Opt Lett (2018) 43(19):4550–3. doi:10.1364/OL.43.004550
5. Duan Y, Sun Y, Zhu H, Mao T, Zhang L, Chen X. YVO4 cascaded Raman laser for five-visible-wavelength switchable emission. Opt Lett (2020) 45(9):2564–7. doi:10.1364/OL.392566
6. Yang XZ, Kitzler O, Spence DJ, Bai ZX, Feng Y, Mildren RP. Diamond sodium guide star laser. Opt Lett (2020) 45(7):1898–901. doi:10.1364/OL.387879
7. Bai ZX, Zhang ZP, Wang K, Gao J, Zhang ZD, Yang XZ, et al. Comprehensive thermal analysis of diamond in a high-power Raman cavity based on FVM-FEM coupled method. Nanomaterials (Basel) (2021) 11(6):1572. doi:10.3390/NANO11061572
8. Yang XZ, Bai ZX, Chen DJ, Chen WB, Feng Y, Mildren RP. Widely-tunable single-frequency diamond Raman laser. Opt Express (2021) 29(18):29449–57. doi:10.1364/OE.435023
9. Liu YC, Chen CM, Hsiao JQ, Pan YY, Tsou CH, Liang HC, et al. Compact efficient high-power triple-color Nd:YVO4 yellow-lime-green self-Raman lasers. Opt Lett (2020) 45(5):1144–7. doi:10.1364/OL.388266
10. Ma YY, Lee AJ, Pask HM, Miyamoto K, Omatsu T. Direct generation of 1108 nm and 1173 nm laguerre-Gaussian modes from a self-Raman Nd:GdVO4 laser. Opt Express (2020) 28(16):24095–103. doi:10.1364/OE.400007
11. Duan YM, Zhou YM, Zhu HY, Li ZH, Jin XX, Tang DY. Selective frequency mixing in a cascaded self-Raman laser with a critical phase-matched LBO crystal. J Lumin (2022) 244:244118698. doi:10.1016/J.JLUMIN.2021.118698
12. Tang CY, Zhuang WZ, Su KW, Chen YF. Efficient continuous-wave self-Raman Nd:KGW laser with intracavity cascade emission based on shift of 89 cm-1. IEEE J Sel Top Quan Electron (2015) 21(1):142–7. doi:10.1109/jstqe.2014.2364126
13. Sheng Q, Lee A, Spence D, Pask H. Wavelength tuning and power enhancement of an intracavity Nd:GdVO4-BaWO4 Raman laser using an etalon. Opt Express (2018) 26(24):32145–55. doi:10.1364/OE.26.032145
14. Sheng Q, Li R, Lee AJ, Spence DJ, Pask HM. A single-frequency intracavity Raman laser. Opt Express (2019) 27(6):8540–51. doi:10.1364/oe.27.008540
15. Fan L, Wang XY, Zhao XD, Wang JH, Shen J, Fan HB, et al. First-Stokes and second-Stokes multi-wavelength continuous-wave operation in Nd:YVO4/BaWO4 Raman laser under in-band pumping. Chin Opt Lett (2020) 18(11):111401. doi:10.3788/Col202018.111401
16. Ballman AA, Porto SPS, Yariv A. Calcium niobate Ca(NbO3)2—a new laser host crystal. J Appl Phys (1963) 34(11):3155–6. doi:10.1063/1.1729154
17. Kaminskii AA, Rogov GI, Bagdasarov KS. Stimulated radiation from a Ca(NbO3)2‐Nd3+ crystal laser. Phys Stat Sol (1969) 31(2):K87–K90. doi:10.1002/pssb.19690310241
18. Cheng Y, Xu XD, Xin Z, Yang XB, Xiao XD, Li DZ, et al. Crystal growth, optical properties, and continuous‐wave laser operation of Nd3+-doped CaNb2O6 crystal. Laser Phys Lett (2009) 6(10):740–5. doi:10.1002/lapl.200910065
19. Xie GQ, Qian LJ, Xu XD, Cheng Y, Zhao ZW, Tang DY, et al. Diode-pumped passively mode-locked Nd:CaNb2O6 laser. Laser Phys (2010) 20(6):1331–4. doi:10.1134/S1054660X10110307
20. Li DZ, Xu XD, Xu CW, Zhang J, Tang DY, Cheng Y, et al. Diode-pumped femtosecond Yb:CaNb2O6 laser. Opt Lett (2011) 36(19):3888–90. doi:10.1364/OL.36.003888
21. Li JH, Liu XH, Wu JB, Zhang X, Li YL, Zhang YC, et al. Efficient diode-end-pumped Yb:CaNb2O6 thin‐disk laser at 1003 nm and second-harmonic generation for an emission at 501.5 nm. Laser Phys Lett (2012) 9(3):199–203. doi:10.1002/lapl.201110118
22. Wang Y, Duanmu QD, Sun GC, Dong Y. Diode-pumped continuous-wave dual-wavelength Yb:CaNb2O6 lasers at 1003 nm and 1038 nm. Optik (Stuttg) (2014) 125(9):2041–3. doi:10.1016/j.ijleo.2013.09.086
23. Husson E, Repelin Y, Dao NQ, Brusset H. Normal coordinate analysis for CaNb2O6 of columbite structure. J Chem Phys (1977) 66:5173–80. doi:10.1063/1.433780
24. de Almeida Silva R, de Camargo ASS, Cusatis C, Nunes LAO, Andreeta JP. Growth and characterization of columbite CaNb2O6 high quality single crystal fiber. J Cryst Growth (2004) 262:246–50. doi:10.1016/j.jcrysgro.2003.10.013
25. Cheng Y, Xu XD, Xu J, Zhao CC, Yang XB, Liang XY. Spectroscopic, thermal, and laser properties of Yb: CaNb2O6 crystal. IEEE J Quan Electron (2009) 45(12):1571–6. doi:10.1109/JQE.2009.2025249
26. Kaminskii AA, Dong J, Eichler HJ, Hanuza J, Ueda K, Maczka M, et al. Laser and nonlinear-laser properties of undoped and Nd3+-doped orthorhombic Ca(NbO3)2 single crystals: New stimulated-emission performance and high-order picosecond stimulated Raman scattering covering more than two octave Stokes and anti-Stokes wavelengths. Laser Phys Lett (2009) 6(11):821–32. doi:10.1002/lapl.200910078
27. Kaminskii AA, Dong J, Ueda K, Bettinelli M, Grinberg M, Jaque D. Q-switched nanosecond Nd3+:Ca(NbO3)2 crystalline self-Raman laser with single-step cascade SE (lambda(SE)=1.0615 mu m of 4F3/2 - 4I11/2 channel) -> SRS (lambda(St1)=1.1741 mu m of omega(SRS) approximate to 904 cm-1 promotion vibration mode) wavelength conversion. Laser Phys Lett (2009) 6(11):782–7. doi:10.1002/lapl.200910077
28. Gong XH, Zhu HY, Chen YJ, Huang JH, Lin YF, Luo ZD, et al. Nd3+ cluster Adjustment in Nd3+:CaNb2O6 by codoping La3+ buffers for improvement of fundamental and self-stimulated Raman scattering laser operation: A study case from the perspective of defect chemistry. Cryst Growth Des (2019) 19(12):6963–71. doi:10.1021/acs.cgd.9b00757
Keywords: self-Raman, solid-state laser, acousto-optic Q-switch, Nd,La:CaNb2O6 crystal, diode pumping
Citation: Zhuang Y, Mao W, Gong X, Jin X, Li Z, Huang Y and Duan Y (2022) Diode-pumped, actively Q-switched Nd,La:CaNb2O6 self-Raman laser at 1,174 nm. Front. Phys. 10:1012551. doi: 10.3389/fphy.2022.1012551
Received: 05 August 2022; Accepted: 29 August 2022;
Published: 23 September 2022.
Edited by:
Xuezong Yang, University of Chinese Academy of Sciences, ChinaReviewed by:
Shibo Dai, Jinan University, ChinaBin Xu, Xiamen University, China
Soumya Sarang, University of Central Florida, United States
Copyright © 2022 Zhuang, Mao, Gong, Jin, Li, Huang and Duan. This is an open-access article distributed under the terms of the Creative Commons Attribution License (CC BY). The use, distribution or reproduction in other forums is permitted, provided the original author(s) and the copyright owner(s) are credited and that the original publication in this journal is cited, in accordance with accepted academic practice. No use, distribution or reproduction is permitted which does not comply with these terms.
*Correspondence: Yanmin Duan, eW1kdWFuQHd6dS5lZHUuY24=