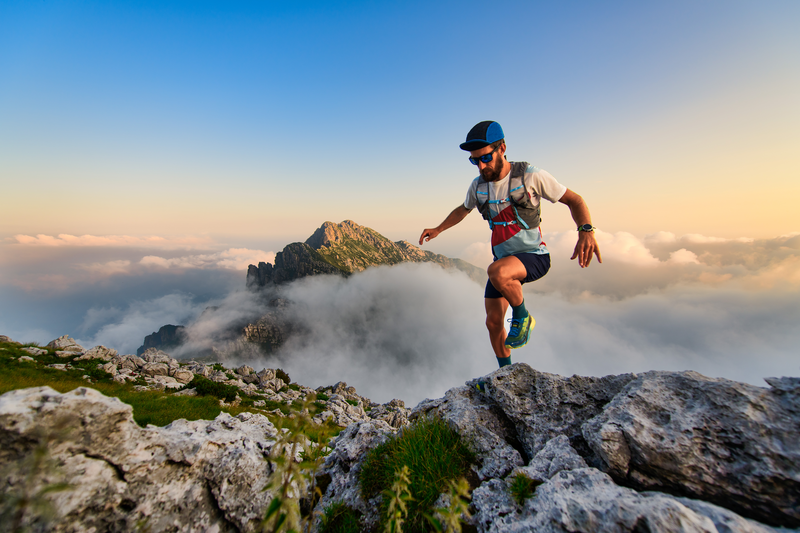
94% of researchers rate our articles as excellent or good
Learn more about the work of our research integrity team to safeguard the quality of each article we publish.
Find out more
EDITORIAL article
Front. Phys. , 01 September 2022
Sec. Soft Matter Physics
Volume 10 - 2022 | https://doi.org/10.3389/fphy.2022.1005146
This article is part of the Research Topic Active Matter in Complex Environments View all 12 articles
Editorial on the Research Topic
Active matter in complex environments
Unlike inert materials in equilibrium, active matter is intrinsically out of equilibrium. Whether as individual or a collective of many self-propelled objects, active matter is often surrounded by physically, chemically, and/or biologically complex environments. Articles of this issue described how such complexities change the behavior of active matter in fascinating and often unexpected ways.
At the level of individuals, theoretical and numerical models are providing new insights into the fundamental physics of motility. For example, Barriuso-Gutierrez et al. developed a numerical algorithm based on dissipative particle hydrodynamics, which properly accounts for hydrodynamic interactions and thermal fluctuations. This approach allowed them to investigate active particles of different swimming mechanisms and shapes in bulk fluids and under confinement. They showed that geometric confinement could substantially vary the translational and orientational dynamics of active spherical colloids and modify the morphology of active polymers. In a related work, Faúndez et al. used simulations to investigate how adhesive active Brownian particles interact with a cylindrical obstacle under an imposed fluid flow. When the flow is strong, particles encounter and adhere to the entire obstacle surface, leading to the formation of multi-layered deposits. Conversely, when the flow is weak, particles directly intercept and adhere to the obstacle in a single-layered deposit. The crossover of these two regimes then defines an optimal flow velocity at which the number of deposited particles is maximized, which provides a useful guide for applications seeking to e.g., filter and retain microswimmers.
Fluid flow also plays an important role in influencing the transport of biological swimmers, such as bacteria. As an example, Savorana et al. experimentally explored how the shear rate and the rheological behavior of polymer solutions affect the motility of Pseudomonas aeruginosa in a Poiseuille flow. They found that in the flow of Newtonian fluids, the bacteria are more concentrated towards the center of the microchannel where the shear rate is minimum. By contrast, in the flow of a shear-thinning fluid, the concentration profile of bacteria is not much different from that in the low-viscosity, Newtonian buffer, despite the zero-shear viscosity of the shear-thinning fluid being two orders of magnitude higher than that of the buffer. Intrigued by the oscillatory motion of cilia and flagella, Tamayo et al. used numerical simulations to study the instabilities and dynamics of a minimal filament-motor system in model viscoelastic fluids. Their model successfully captures some very interesting features. In a Newtonian fluid, the oscillation frequency is determined by motor kinetics and decreases with the increase of fluid viscosity. In viscoelastic fluids that have the same viscosity as the Newtonian fluid, the filament can exhibit stable oscillatory states with larger amplitudes and higher frequencies than in the Newtonian case.
Unlike bacteria, mammalian cells constantly reorganize their cytoskeleton to generate contractility and migrate. Bergeron-Sandoval et al. explored the biological mechanisms of shear stress-mediated cell contractility. They discovered that only cells with dynamic Filamin A (FLNa), a protein critical to cell structure and mechanosensation, and FilGAP, a specific GTPase activating protein (GAP), convert shear stress into GTPase activity to result downstream contractile changes. Yet, in the absence of intact FLNa-FilGAP mechanosensing, the contractile activity of cells can be rescued using pharmaceuticals to manipulate Rho and Rac activity, which are known to mediate cellular migration by directing the formation and organization of actin filaments. These studies clarify a precise mechanomolecular pathway used for cellular force sensing that may play critical roles in e.g., cancer metastasis and cardiovascular disease.
Moving to even more complex environments, Modica et al. used experiments with self-propelled Janus particles, Brownian dynamics simulations, and theory to investigate how active particles navigate through arrays of multiple obstacles, which act as a model of more complex porous media. In this case, accumulation at the obstacle surfaces hinders the long-time effective diffusion of the particles. Remarkably, this hindrance is much larger than the hindrance experienced by passive Brownian particles—highlighting the importance of the coupling between active forces and boundary interactions on the transport properties of active matter.
Two articles of the issue investigated the collective dynamics of active matter and examined their behaviors under geometric confinement. Inspired by the inter-cell interaction mediated by deformed elastic substrates, Bose et al. explored the emergent collective states of active particles with elastic dipolar interactions in simulations. They showed the formation of polar particle clusters and used confinement to modify the collision dynamics of the motile chains. Gulati et al. addressed the important question on how boundary conditions affect the collective dynamics of active liquid crystals. Combining simulations with stability analysis, they showed a series of transitions between different collective states and revealed the interplay between symmetry and geometry in dictating the collective dynamics of active matter. Both works illustrated the great potential of using confinement to tune the dynamics of active systems.
Active materials can also respond to and alter the environments they interact with. As a fascinating example of this point, Pönisch and Zaburdaev computationally studied how bacteria that use surface appendages (“pili”) to self-propel can power the persistent rotation of a micron-sized turbine. Their work is motivated by the observation that many bacteria use pili protrusion and retraction to bind to and crawl along surfaces, and that the molecular motors involved are among the strongest motors known in nature. Indeed, Pönisch and Zaburdaev found that when groups of cells attach to and pull on the turbine, it can rotate persistently, depending on specific changes in pili binding and adhesion. Not only does this work further elucidate active matter-boundary interactions at a fundamental level, but it also suggests a way to put active matter to work!
Going beyond studies in the lab, the mathematical modeling of Vachier and Wettlaufer provided insights into bacterial locomotion in ice under temperature and chemical gradients. The authors considered the interplay between bio-enhanced interfacial premelting, thermal regelation, particle motility and chemotaxis and analyzed its consequence on particle dynamics and nutrient distributions in ice. Their work reveals interesting aspects of life in extreme environments and may be useful for understanding the covariation of life and climate and potential biosignatures in extraterrestrial life.
Finally, moving beyond natural and synthetic microswimmers, Tepermeister et al. reviewed the use of soft responsive materials to make functional devices—highlighting how activity, encoded by material properties, can be exploited for applications. The researchers outlined the physical principles for soft ionic materials and devices, discussed the progress for each of the potential device components, and pointed out opportunities for future research.
All authors contributed equally to the conceptualization and writing of this editorial.
The authors declare that the research was conducted in the absence of any commercial or financial relationships that could be construed as a potential conflict of interest.
All claims expressed in this article are solely those of the authors and do not necessarily represent those of their affiliated organizations, or those of the publisher, the editors and the reviewers. Any product that may be evaluated in this article, or claim that may be made by its manufacturer, is not guaranteed or endorsed by the publisher.
Keywords: active matter, soft matter physics, complex fluids, porous media, responsive materials
Citation: Cai L-H, Datta SS and Cheng X (2022) Editorial: Active matter in complex environments. Front. Phys. 10:1005146. doi: 10.3389/fphy.2022.1005146
Received: 28 July 2022; Accepted: 15 August 2022;
Published: 01 September 2022.
Edited and reviewed by:
Jasper Van Der Gucht, Wageningen University and Research, NetherlandsCopyright © 2022 Cai, Datta and Cheng. This is an open-access article distributed under the terms of the Creative Commons Attribution License (CC BY). The use, distribution or reproduction in other forums is permitted, provided the original author(s) and the copyright owner(s) are credited and that the original publication in this journal is cited, in accordance with accepted academic practice. No use, distribution or reproduction is permitted which does not comply with these terms.
*Correspondence: Li-Heng Cai, bGloZW5nLmNhaUB2aXJnaW5pYS5lZHU=; Sujit S. Datta, c3NkYXR0YUBwcmluY2V0b24uZWR1; Xiang Cheng, eGNoZW5nQHVtbi5lZHU=
†ORCID: Sujit S. Datta, orcid.org/0000-0003-2400-1561; Li-Heng Cai, orcid.org/0000-0002-6806-0566
Disclaimer: All claims expressed in this article are solely those of the authors and do not necessarily represent those of their affiliated organizations, or those of the publisher, the editors and the reviewers. Any product that may be evaluated in this article or claim that may be made by its manufacturer is not guaranteed or endorsed by the publisher.
Research integrity at Frontiers
Learn more about the work of our research integrity team to safeguard the quality of each article we publish.