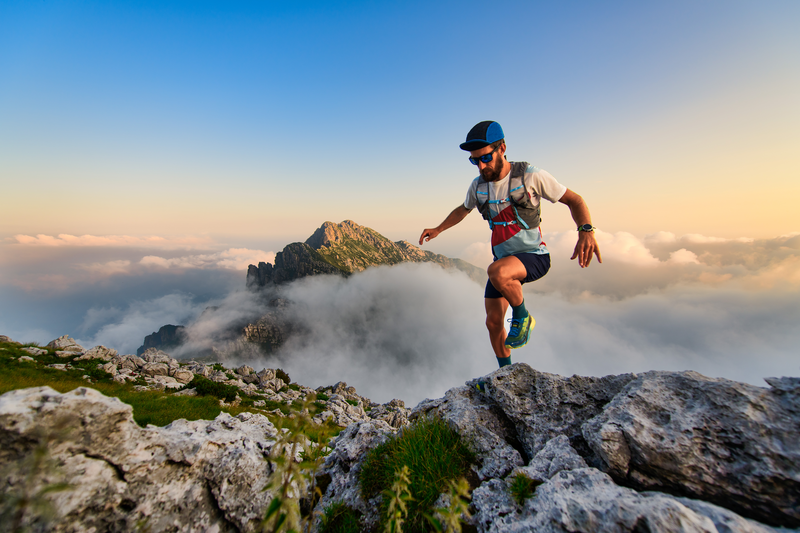
95% of researchers rate our articles as excellent or good
Learn more about the work of our research integrity team to safeguard the quality of each article we publish.
Find out more
ORIGINAL RESEARCH article
Front. Phys. , 07 September 2021
Sec. Optics and Photonics
Volume 9 - 2021 | https://doi.org/10.3389/fphy.2021.756232
This article is part of the Research Topic Optical Fiber-based Plasmonics Biosensors for Biomedical Applications View all 18 articles
High-speed data demand in sensitive locations has prompted new wireless technologies to grow in areas like hospitals for bio-sensor data transmission between doctors and patients. However, interference of electromagnetic spectrum or highly sensitive medical equipment in such locations can prevent radio waves which can further compromise the health of patients. Radio over Free Space Optics (Ro-FSO) can fulfil high-speed data demand in such locations without any such interference. However, the Ro-FSO performance is highly influenced by different adverse weather conditions, particularly haze and rainfall, which further cause attenuation in the transmission path of Ro-FSO systems. These atmospheric turbulences mainly affect the transmission link range of Ro-FSO systems. In this work, Ro-FSO system is designed by incorporating hybrid mode division multiplexing (MDM) and polarization division multiplexing (PDM) schemes to deliver four independent channels, each carrying 10 Gbps data upconverted to 40 GHz radio signal, over 3.4 km free space optical link operating under clear weather conditions. In addition to this, the proposed Ro-FSO link is subjected to different weather conditions, particularly partially hazy/rainy and dense fog/very rainy. The reported results indicate the achievement of acceptable bit error rate (BER≈10–3) for all channels up to 3400m FSO link under clear weather conditions, 1000m under partially haze/rain and 620 m under dense fog/heavy rain.
The current pandemic situation of COVID-19 has not only affected global healthcare in general but also raised questions on the effectiveness of epidemic response mechanisms across the world. In terms of screening the infected individuals and frontline healthcare personnel, communication and data exchange systems have a crucial role to play. Particularly in high-density countries like Thailand, China, and India, it is essential to update such systems for controlling the ongoing pandemic outbreak. Telecommunication operators can install high-speed broadband networks in hospitals by using innovative applications to drive emergency response mechanisms digitally and accurately. Both high and low frequencies can be used by broadband networks to provide highest speeds on millimeter waves with frequencies between 30 and 300 GHz. However, these radio millimeter waves are not permitted in sensitive medical locations since it can interfere with crucial medical equipment which can impact patients’ health. Therefore, such sensitive locations are always considered as challenging locations to connect with broadband services. Moreover, growing population has caused substantial increase in data demand which further compels researchers to explore new-generation communication technologies. According to a survey by Ericsson, annual mobile data traffic saw an increase of 65% in 2015 which is assumed to grow ten times bigger by the end of 2021 [1]. The issue of bandwidth increase of the existing wireless radio networks can be resolved by decreasing the cell size to accommodate more users. Spectral congestions can be mitigated by operating frequency bands in microwave or millimeter bands [2]. Because of this, the service area requires multiple base stations resulting in its complexed structure and higher cost. Free Space Optics (FSO) has thrived in application in high-speed wireless networks due to its features for smooth traffic functioning in optical fibers [3–5]. Moreover, another FSO-based study [6] used point-to-point laser signals for negligible interception to secure transmission. Studies [7–9] have also reported features of FSO including its high capacity, less power consumption, light weight, smaller size and cost-effective implementation charges. Radio over Free Space Optical (Ro-FSO) communication can be an excellent solution to fulfill the demands of high-speed data by combining radio technology with optical wireless technology at lower cost [10, 11]. As modern cities are growing bigger and bigger, digging of lands to install optical fiber cables is not feasible. Thus, Ro-FSO can be a potential carrier to deliver various high-speed digital services such as high-speed internet, video on demand, and Triple Play Services (TPS) without using optical fiber cables [12, 13] as shown in Figure 1. Ro-FSO can handle the increasing mobile subscribers by transmitting RF signals through high-speed optical carrier that does not cost much or need any licensing.
Some of the major features of Ro-FSO include no license requirement, low power consumption and ease of deployment; however, its performance is limited in atmospheric turbulences such as fog, rain, scintillations, and fog [4, 14, 15]. Atmospheric turbulences cause temporal random fluctuations of the refractive index through the optical channel due to temperature, wind variations, and pressure [16]. This fluctuation in refractive index further results in phase shift of optical signals transmitting through the atmosphere leading to attenuation in the wave front. To improve the performance, many researchers have proposed orthogonal frequency division multiplexing (OFDM) scheme by compromising the complexity and cost of FSO systems. In 2016 [17], authors have transmitted 1.6 Gbps data over 1.8 km FSO link by employing OFDM scheme under heavy rainfall conditions. In 2017 [18], authors have used 16 QAM-OFDM encoding scheme to transmit 20 Gbps data over 10 km single mode fiber link and 300 mm FSO link. In 2018 [19], authors have proposed OFDM-based FSO link by incorporating non line-of-sight assisted relay. Recently in 2019 [20], authors have proposed hybrid polarization division multiplexing (PDM) and OFDM schemes to transmit data over 5 km FSO link. OFDM can improve the FSO system performance by providing high spectral bandwidth, firmness against multipath path fading effects and narrowband interferences. However, this increases the cost and complexity of FSO transmitter and receiver. On-off key encoding schemes can be an attractive solution to reduce the cost of and simplify FSO communication system. But it also needs to compromise the transmission capacity and distance. Whereas mode division multiplexing (MDM) becomes an attractive solution to increase the transmission capacity of multimode transmission systems by simultaneously transmitting different channels on different modes. The last few years have witnessed remarkable adoption of MDM scheme in FSO systems. It uses eigen modes instead of wavelength which can enhance the capacity of Ro-FSO. Eigen modes can be generated by using mechanisms such as Photonic Crystal Fibers (PCF) or Spatial Light Modulators (SLM). The spectral bandwidth of Ro-FSO can further be increased by incorporating PDM [21] with MDM.
Figure 2 shows how the hybrid MDM-PDM scheme is designed for transmitting four independent channels by using OptiSystem™ software. Each channel can support the data rate of 10 Gbps upconverted to 40 GHz mm carrier. A continuous wavelength (CW) laser with the wavelength of 1,552.5 nm is used to excite the particular donut mode by using donut mode generator. The power of laser is set at 0dBm. Transmitter 1 is operated on donut mode 0 and Transmitter 2 is operated on donut mode 1. The output of these transmitters is integrated. The state of polarization controller with azimuth of 00 is used for maintaining the polarization state (X Polarization). Similarly, transmitters 3 and 4 are operated on donut modes 0 and 1, respectively. The output of transmitters 3 and 4 is combined and a polarization state (Y Polarization) is maintained by providing azimuth of 900 through the state of polarization controller. The output of the states of polarization controllers is combined and transmitted through free space link. The output of link is amplified by using optical amplifier with the gain of 18dB. Figure 3 shows the maintained polarization states for X and Y polarizations. X and Y polarizations are separated by using polarization splitter at the receiver side. The polarization states are further split into two parts corresponding to two modes transmitted at the transmitter side. Mode selector is used to select the particular mode required for the receiver side.
Figure 4 shows the schematic diagram of transmitter and receiver in the proposed MDM-PDM-Ro-FSO system. 10 Gbps data are encoded by employing low-cost NRZ encoding scheme and then upconverted to 40 GHz mm wave by using mixer as shown in Figure 5. Sine wave generator is used to generate the 40 GHz mm signal.
FIGURE 4. Schematic representation of Transmitter and Receive. rThe FSO link can be mathematically described as.
Lithium Niobate optical modulator is used for modulating the 40 GHz upconverted wave through optical carrier. The receiver consists of PIN photodiode which further converts the optical signal back to the corresponding electrical signal. The output of photodetector is then mixed with 40 GHz radio signal to attain the process of down conversion.
After the down conversion process, low-pass filter is used to recover the original baseband signal.
In this FSO link equation,
In this equation, “λ” refers to wavelength, “L” the propagation distance and “H” the loss coefficient. Another model on FSO attenuation that depends upon visibility factor known as Kim’s attenuation model can be represented mathematically as [23]:
where v refers to meteorological visibility, λ operating wavelength and q a factor depending on the size distribution of atmospheric particles.
Under the impact of different rainfall, the following equation can calculate the attenuation [24]:
where R is the amount of rainfall. Table 1 shows the different values of atmospheric turbulences [25] and Table 2 shows the parameters to model the proposed MDM-PDM-Ro-FSO link.
The results obtained from the modeling of the proposed MDM-PDM-Ro-FSO system are presented and discussed in this section. Figure 6 depicts the measured BERs for channels 1 and 2 operated on donut modes 0 and 1, respectively with X polarization as well as for channels 3 and 4 with Y polarization state with respect to Ro-FSO link distance under clear weather conditions. As shown in Figure 6A, the computed BER values for Channel 1 and Channel 2 are 10−9, 10−7 and 10−5 at the FSO transmission link of 2,700, 3,200, and 3,400 m. Similarly, for Channel 3 and Channel 4, the BER values are computed as 10−9, 10−6 and 10−5 at the FSO transmission link of 2,700 m, 3,200 m and 3,400 m as shown in Figure 6B.
FIGURE 6. Computed BER (clear weather conditions) (A) Channel 1 and Channel 2 (X Polarization) (B) Channel 3 and Channel 4 (Y Polarization).
Therefore, under clear weather conditions, all channels achieved the BER of 10−4 which is below the range of acceptable BER≈10−3 (FCC limit) at the FSO link of 3400 m with required opening of eye diagrams. However, Channel 2 and Channel 4 operated on donut mode 1 are slightly affected more as compared to Channel 1 and Channel 3 operated on donut mode 0. In another case, the Ro-FSO link is subjected to partially hazy/rainy atmospheric conditions and computed BER is shown in Figure 7. The values of BER for Channel 1 are computed as 10−7, 10−5 and 10−3 at the FSO transmission link of 850, 940 and 1000 m. Similarly for Channel 2, it is computed as 10−9, 10−5 and 10−3 at the FSO transmission link of 850, 940 and 1000 m. As shown in Figure 7B, the values of computed BER for Channel 3 and Channel 4 are 10−9, 10−5 and 10−3 at the FSO transmission link of 850, 940 and 1000 m.
FIGURE 7. Measured BER under partially hazy/rainy atmospheric condition (A) Channel 1 and Channel 2 (B) Channel 3 and Channel 4
Figure 8 shows the measured BER for all channels under the influence of dense fog/very hazy atmospheric conditions. For Channel 1 and Channel 2, the values of BER are computed as 10−7, 10−5, and 10−4 at the FSO transmission link of 570, 600 and 620 m.
FIGURE 8. Computed BER (dense fog/very hazy atmospheric conditions) (A) Channel 1 and Channel 2 (X Polarization) (B) Channel 3 and Channel 4 (Y Polarization).
Similarly as shown in Figure 8B, for Channel 3 and Channel 4, the values of BER are computed as 10−7, 10−5 and 10−4 at the FSO transmission link of 570, 600 and 620 m.
Thus, when the proposed Ro-FSO link is operated in clear weather conditions, the computed BER shows transmission of all the channels up to 3400 m FSO link with acceptable BER ≈10−3 and eye diagrams. After the FSO transmission distance, BER value increases beyond the FCC limit. Moreover, when the proposed Ro-FSO link is operated in partial haze/rain, the FSO transmission distance reduces to 1000 m with acceptable BER and eye diagrams. Furthermore, when the proposed Ro-FSO link is operated in dense fog/heavy rain, the FSO transmission distance reduces to 620 m with acceptable BER and eye diagrams.
In this work, Ro-FSO link is designed for transmission of broadband services by employing hybrid MDM and PDM schemes. Donut modes 0 and 1 were used for MDM scheme whereas NRZ scheme was used for encoding the pseudorandom data. The designed MDM-PDM-Ro-FSO link could transmit four independent channels each with 10 Gbps data upconverted to 40 GHz radio signal. Under the clear weather condition, the proposed MDM-PDM-Ro-FSO link can transmit 40 Gbps data up to 3400 m with acceptable BER ≈10−3. However, when the weather condition changed to partial haze/rain, the proposed MDM-PDM-Ro-FSO link could withstand only up to 1000 m due to attenuation. Furthermore, when the atmospheric condition changed from partial haze/rain to dense fog/heavy rain, the proposed link could withstand up to 650 m only with acceptable BER.
The raw data supporting the conclusion of this article will be made available by the authors, without undue reservation.
SC: Conceptualization, methodology, investigation, writing—original draft. LW: supervision, resources, project administration, funding acquisition. JN and XT: supervision, funding acquisition. MS , SA, and AA: Methodology, Funding Acquisition, resources. AS and SC: data curation and visualization.
This research project is supported by the Second Century Fund (C2F), Chulalongkorn University, Thailand. This research work is also funded by TSRI Fund (CU_FRB640001_01_21_8).
The authors declare that the research was conducted in the absence of any commercial or financial relationships that could be construed as a potential conflict of interest.
All claims expressed in this article are solely those of the authors and do not necessarily represent those of their affiliated organizations, or those of the publisher, the editors and the reviewers. Any product that may be evaluated in this article, or claim that may be made by its manufacturer, is not guaranteed or endorsed by the publisher.
The authors also would like to thank Taif University Researchers supporting project number (TURSP-2020/228), Taif University, Taif, Saudi Arabia.
1. de la Fuente A, Leal RP, Armada AG. New Technologies and Trends for Next Generation Mobile Broadcasting Services. IEEE Commun Mag (2016) 54:217–23. doi:10.1109/mcom.2016.1600216rp
2. Zin A, Bongsu M, Idrus S, Zulkifli N. An Overview of Radio-Over-Fiber Network Technology. In International Conference On Photonics (2010). p. 1–3. doi:10.1109/icp.2010.5604429
3. Henniger H, Wilfert O. An Introduction to Free-Space Optical Communications. Radioengineering (2010) 19:203–12.
4. Malik A, Singh P. Free Space Optics: Current Applications and Future Challenges. Int J Opt (2015) 2015. doi:10.1155/2015/945483
5. Lim C, Wang K, Nirmalathas A. Optical Wireless Communications for High-Speed In-Building Personal Area Networks. In Transparent Optical Networks (ICTON). Kuching, Sarawak, Malaysia.: International Conference on (20162016). p. 1–4. doi:10.1109/icton.2016.7550662
6. Majumdar AK. Fundamentals of Free-Space Optical (FSO) Communication System. In Advanced Free Space Optics (FSO). Springer (2015). p. 1–20. doi:10.1007/978-1-4939-0918-6_1
7. Siegel T, Chen S-P. Investigations of Free Space Optical Communications under Real-World Atmospheric Conditions. Wireless Pers Commun (2021) 116:475–90. doi:10.1007/s11277-020-07724-1
8. Sarangal H, Singh A, Malhotra J, Chaudhary S. A Cost Effective 100 Gbps Hybrid MDM-OCDMA-FSO Transmission System under Atmospheric Turbulences. Opt Quant Electron (2017) 49:184. doi:10.1007/s11082-017-1019-2
9. Anandkumar D, Sangeetha R. A Survey on Performance Enhancement in Free Space Optical Communication System through Channel Models and Modulation Techniques. Opt Quan Elect (2021) 53:1–39. doi:10.1007/s11082-020-02629-6
10. Amphawan A, Chaudhary S, Neo T-K, Kakavand M, Dabbagh M. Radio-over-free Space Optical Space Division Multiplexing System Using 3-core Photonic crystal Fiber Mode Group Multiplexers. Wireless Netw (2021) 27:211–25. doi:10.1007/s11276-020-02447-4
11. Kuppusamy PG, Rajkumar K, Maheswar R, Rani SS, Amiri IS. A Long-Reach Radio over Free Space Optics (Ro-FSO) System Using Hybrid Orthogonal Frequency Division Multiplexing (OFDM)-multibeam Concept with Enhanced Detection. J Opt Commun 1:2020.
12. Mandal GC, Mukherjee R, Das B, Patra AS. Next-generation Bidirectional Triple-Play Services Using RSOA Based WDM Radio on Free-Space Optics PON. Opt Commun (2018) 411:138–42. doi:10.1016/j.optcom.2017.11.033
13. Chaudhary S, Choudhary S, Tang X, Wei X. Empirical Evaluation of High-Speed Cost-Effective Ro-FSO System by Incorporating OCDMA-PDM Scheme under the Presence of Fog. J Opt Commun (2020) 1. doi:10.1515/joc-2019-0277
14. Chaudhary S, Amphawan A. The Role and Challenges of Free-Space Optical Systems. J Opt Commun (2014) 35:327–34. doi:10.1515/joc-2014-0004
15. Chaudhary S, Lin B, Tang X, Wei X, Zhou Z, Lin C, et al. 40 Gbps-80 GHz PSK-MDM Based Ro-FSO Transmission System. Opt Quant Electron (2018) 50:321. doi:10.1007/s11082-018-1592-z
16. Xiaoming Zhu X, Kahn JM. Free-space Optical Communication through Atmospheric Turbulence Channels. IEEE Trans Commun (2002) 50:1293–300. doi:10.1109/tcomm.2002.800829
17. Rashidi F, He J, Chen L. Performance Investigation of FSO–OFDM Communication Systems under the Heavy Rain Weather. J Opt Commun (2017) 39:37–42. doi:10.1515/joc-2016-0085
18. Perez J, Chicharro F, Ortega B, Mora J, "On the Evaluation of an Optical OFDM Radio over FSO System with IM-DD for High-Speed Indoor Communications," in 2017 19th International Conference on Transparent Optical Networks (ICTON), 2017, pp. 1–4.doi:10.1109/icton.2017.8025112
19. Kumar P, Thakor S. Performance of OFDM-FSO Link with Analog Network Coding. Photon Netw Commun (2018) 35:210–24. doi:10.1007/s11107-017-0730-z
20. Kaur G, Srivastava D, Singh P, Parasher Y. Development of a Novel Hybrid PDM/OFDM Technique for FSO System and its Performance Analysis. Opt Laser Tech (2019) 109:256–62. doi:10.1016/j.optlastec.2018.08.008
21. Sumathi K, Balasaraswathi M, Boopathi C, Singh M, Malhotra J, Dhasarathan V. Design of 3.84 Tbps Hybrid WDM–PDM Based Inter-satellite Optical Wireless Communication (IsOWC) System Using Spectral Efficient Orthogonal Modulation Scheme. J Ambient Intelligence Humanized Comput (2020) 1–9.
22. Gebhart M, Leitgeb E, Muhammad SS, Flecker B, Chlestil C, Al Naboulsi M, et al. Measurement of Light Attenuation in Dense Fog Conditions for FSO Applications. In Atmospheric Optical Modeling. Measurement: and Simulation (2005). p. 58910K. doi:10.1117/12.614830
23. Shakir F, Ali MAA, Ameer F. Utilization of MIMO Concept for Optical Communication System under Fog Condition. ECTI-EEC (2019) 17:130–5. doi:10.37936/ecti-eec.2019172.219183
24. Kumar Giri R, Patnaik B. Bit Error Rate Performance Analysis of Hybrid Subcarrier Intensity Modulation-Based FSO with Spatial Diversity in Various Weather Conditions. J Opt Commun (2019) 40:307–14. doi:10.1515/joc-2017-0073
Keywords: radio over free space optics, mode division multiplexing, polarization division multiplex, partially hazy, dense fog
Citation: Chaudhary S, Wuttisittikulkij L, Nebhen J, Tang X, Saadi M, Al Otaibi S, Althobaiti A, Sharma A and Choudhary S (2021) Hybrid MDM-PDM Based Ro-FSO System for Broadband Services by Incorporating Donut Modes Under Diverse Weather Conditions. Front. Phys. 9:756232. doi: 10.3389/fphy.2021.756232
Received: 10 August 2021; Accepted: 23 August 2021;
Published: 07 September 2021.
Edited by:
Santosh Kumar, Liaocheng University, ChinaReviewed by:
Tony Jose, Karunya Institute of Technology and Sciences, IndiaCopyright © 2021 Chaudhary, Wuttisittikulkij, Nebhen, Tang, Saadi, Al Otaibi, Althobaiti, Sharma and Choudhary. This is an open-access article distributed under the terms of the Creative Commons Attribution License (CC BY). The use, distribution or reproduction in other forums is permitted, provided the original author(s) and the copyright owner(s) are credited and that the original publication in this journal is cited, in accordance with accepted academic practice. No use, distribution or reproduction is permitted which does not comply with these terms.
*Correspondence: Sushank Chaudhary, c3VzaGFua2NoYXVkaGFyeUBnbWFpbC5jb20=; Lunchakorn Wuttisittikulkij, THVuY2hha29ybi5XQGNodWxhLmFjLnRo
Disclaimer: All claims expressed in this article are solely those of the authors and do not necessarily represent those of their affiliated organizations, or those of the publisher, the editors and the reviewers. Any product that may be evaluated in this article or claim that may be made by its manufacturer is not guaranteed or endorsed by the publisher.
Research integrity at Frontiers
Learn more about the work of our research integrity team to safeguard the quality of each article we publish.