- 1Department of Electrical and Electronics Engineering, Lovely Professional University, Punjab, India
- 2Quanzhou Institute of Equipment Manufacturing, Chinese Academy of Sciences, Jinjiang, China
- 3School of Electrical, Computer and Telecommunication Engineering, University of Wollongong, Wollongong, NSW, Australia
- 4Technological Projects Department, The Libyan Center for Remote Sensing and Space Science, Tripoli, Libya
Free-space optical (FSO) communication systems are being anticipated to offer promising alternatives to existing radio networks in delivering high-speed data access to end-users. Ease of installation, robust features, and cost-effective operation have been the hallmark of FSO systems, and these features will play an obvious role in deciding the ways in which futuristic smart communication models will operate. Despite these arrays of features, FSO links suffer severe performance degradation due to channel-induced impairments caused by atmospheric effects such as rain, haze, and fog. In this work, we have investigated and compared the performance of 40 Gbps FSO links for different channel conditions ranging from clear weather to severe attenuation by incorporating spatial and wavelength diversity as performance booster techniques. The use of an erbium-doped fiber amplifier (EDFA) with FSO links has also been proposed here. Using performance metrics like bit error rate (BER) and eye patterns, it has been found that the use of EDFA not only helps in compensating for the link losses but also aids in realizing an all-optical processing based last-mile access system. The proposed FSO system will be capable of bridging the existing backbone fiber networks with end-users with minimal changes to the existing hardware regime, thereby proving to be extremely cost-effective in sharp contrast to radio-frequency generations which require major infrastructure overhaul.
Introduction
Often labeled as wireless optical fibers, optical wireless communication has huge potential to serve the ever-increasing demand for high-speed data services. Over the last few decades, the per capita demand for data has increased manifolds, and this pattern only seems to become more and more aggressive in the near future [1–3]. The recent surge in popularity of free-space optical (FSO) systems as a commercial alternative to radio-frequency (RF) systems is attributed to a wide range of advantages such as 1) massive bandwidth of the order of THz, 2) adaptability with present-day radio systems as RF/FSO systems [4, 5], 3) license-free spectrum, 4) negligible interference from the adjacent carriers, and 5) plug–play character which makes the FSO systems very convenient to install and relocate [6, 7]. FSO systems have begun witnessing commercial and large-scale deployment in cases of disaster management, LAN connectivity within campuses, vehicle-to-vehicle communication, and backhaul services for futuristic cellular networks [8, 9]. However, it is anticipated that FSO links can play a game-changer role in cost-effective connectivity for far-flung areas, especially in developing countries like India in the fields of e-governance, telemedicine, and education [10]. Figure 1 shows the typical FSO topology for providing broadband services in urban areas where the new installation of optical fiber is not possible. The underlying principle of end-user privacy in contemporary networks is based on data encryption and user authentication achieved through a complex mechanism of exchange of digital keys [11, 12]. The FSO channel link is a line-of-sight (LoS) communication model that requires straight-line alignment of transmitter and receiver for data transmission. This restricts the system from a wide-range broadcast of the information signal.
The role of the transmission channel is, therefore, extremely critical in determining the efficiency and uptime of communication links [13]. Additionally, the selection of the type of transmitter and transmitted wavelength is an important criterion in FSO systems. An optical wireless system consists of a light source that could be LED or LASER for transmitting optically modulated information over the channel, while on the receiver side, a photodiode collects the photons to reproduce the original information. However, it is relevant to point out that the received signal experiences absorption and scattering due to the presence of atmospheric phenomena such as rain, fog, and haze which causes signal degradation, thus leading to loss of transmitted information [4, 6]. Equally devastating is signal fading induced by the inhomogeneities present in the transmitting medium [8, 11]. The presence of these inhomogeneities leads to fluctuations in refractive indices along with the propagating medium, hence leading to a situation known as atmospheric turbulence that causes beam divergence and scintillation effects [7, 14]. Various mitigation techniques like the higher order modulation schemes, diversity combining, channel-coding schemes, and spectrum slicing have been proposed in the past to improve the performance and robustness of the FSO system [15–20]. The transmitter diversity, along with channel-coding techniques, deals with the performance enhancement by reducing the erroneous data induced by the characteristics of the fading channel [17, 21–23]. The use of different modulation schemes can also alter the system performance as it has been reported that higher modulation schemes like binary phase-shift keying (BPSK) are more immune to channel adversities than the conventional on-off keying (OOK) schemes [19, 21]. The diversity in the channel brings in the concept of multiple input multiple output (MIMO), which uses multiple transmitters/receivers to improve the transmission reliability [24, 25]. The adoption of diversity schemes not only proved to be a major cost-effective factor in improving the link reliability in FSO systems but also helps improve bandwidth capacity, quality factor (Q), and reduced latency [26–28]. Additional technology measures such as coherent reception, higher modulation techniques, artificial intelligence, and machine learning have also been suggested in recent literature as catalysts to enhance link performance in FSO systems; however, on the flip side, the technological intricacy and budgetary constraints of these techniques are major deterrents toward their commercial implementation [29, 30]. As a well-evolved and matured technology, spatial diversity and wavelength diversity proved to be efficient measures in countering atmospheric adversities [28]. Diversity schemes are also known for their ability to deliver higher throughput and low bit error rates [30]. The usage of readily available hardware, which is easy to install and comes with improved end factors, cuts down the overall cost for the setup, as the available resources are being shared for multiuser scenarios [31].
The organization of this paper is as follows: Link Design Analytics contains the description of the methodology that has been employed to design the link described in System Description and the analysis of the link for various quality factors is elaborated in Results and Discussion, followed by the conclusive report of the paper in Conclusion.
Link Design Analytics
Conventionally, MIMO systems have been used to increase the data transmission rates; as in FSO systems, the MIMO approach has been used to improve the probability of correct data reception by transmitting the same information through multiple lines-of-sight channels [32–36]. The systematic idea of approach toward MIMO is illustrated in Figure 2, which explains the transmission of information through different atmospheric conditions. The optical carriers used in MIMO systems are tuned at a particular wavelength, and this selection could affect the system properties. Therefore, wavelength selection is a crucial part of the FSO system design [37, 38].
Wavelength diversity refers to the use of different transmission wavelengths and these diverse subcarriers, while propagating through channels, undergo different levels of fading [39, 40]. However, if the information received over different channels is combined at the receiver, the detection probability of correct information increases [37]. The block diagram for the proposed MIMO-FSO system is illustrated in Figure 3, where the different blocks of the system are placed according to their functional positions. Since wavelength diversity and spatial diversity have a proven track as performance enhancement catalysts in the FSO system [24], in this paper, the use of wavelength diversity along with MIMO systems has been proposed to provide seamless last-mile connectivity.
It is important to underline here that FSO signals have to deal with absorption, scattering, and geometrical loss of orientation while propagating. All of these factors contribute toward atmospheric attenuation, which can be summed as follows [32, 38]:
The geometric losses include the attenuation due to beam divergence and the transmitter positioning with the receiver. The attenuation phenomenon can be described using Beer-Lambert law that states the relationship between transmitted wavelength and link distance, which is represented as follows [14, 41]:
where λ is the wavelength, L is the propagation distance, and H is the loss coefficient. Kim’s attenuation model for FSO links to relate attenuation with visibility and operational wavelength is as follows [34, 42]:
where V refers to meteorological visibility, λ is the operating wavelength, and q is the factor that depends on the size distribution of atmospheric particles. When it comes to attenuation that occurs due to rain, the equation based on the rate of rainfall can be given as follows [40]:
Here, the rate of rainfall is represented by R and the attenuation depends proportionally on this factor.
The atmospheric effects that are considered for the FSO channel corresponding to various attenuation values are presented in Table 1, which will be used as a reference for the analysis of the proposed link [19].
Although the inclusion of multiple sub-carrier wavelengths helps improve reception quality, it forms a wideband signal after multiplexing and consumes greater amounts of bandwidth in contrast to the transmission of data over a single carrier [40, 43]. This tradeoff between system resources and performance is an exception for next-generation optical networks as optical links by default possess huge spectral bandwidth [36, 44]. Also, the wavelength diversified MIMO-FSO system could be improved further by incorporating the erbium-doped fiber amplifier (EDFA)–based link compensation [45]. The detailed block diagram of the EDFA optical amplifier working for the input optical signal is shown in Figure 4 [46]. Usage of EDFA is helpful in avoiding the losses that incur due to change of signal from optical to electrical, and vice versa, for the purpose of amplification [47].
The optical signal traveling through the open-air medium is collected at a certain point using the collimating lens setup, which is then filtered with a bandpass filter that restricts the outlier wavelengths and forwards the filter output through a gain optimizer setup. The setup helps amplify the signal by an input from a pump LASER emitting the wavelength that is the same as the output toned by a bandpass filter [48]. The coupled output is passed through erbium-doped fiber that helps in the amplification of optical signals without loss of intended information. At the final stage, the signal may be passed through another optical bandpass filter which gives the final amplified signal as an output [46]. Later, a set of collimating optics can be used to transmit the modulated optical signal for detection and further analysis. This practice not only helps in delivering an all-optical system that is capable of compensating for the loss of signal quality due to atmospheric adversities but also readily integrates with the existing optical backbone networks [48–50].
The novelty and scope of the proposed link analyzed and reported in the paper can be summarized as follows:
• Design of a hybrid MIMO-FSO incorporating wavelength diversity over the adverse channel
• Performance analysis of a hybrid model when extended for the use of multiple users
• Gauging performance behavior of the proposed hybrid FSO when coupled with an EDFA-based all-optical post-amplification
System Description
The proposed link presented in this paper and illustrated in Figure 5 has been designed and analyzed using a specialized link design tool, OptiSystem™ 16.0, which is an industry graded experimental platform [51]. The parameters used for the link design and subsequent investigation have been stated in Table 2. As shown in Figure 5, the pseudo-random bit sequence generator (PRBS) produces a stream of bits at the rate of 10 Gbps which are then modulated using Mach Zehnder (MZ) modulator before being relayed over the FSO channel.
Meanwhile, continuous wave (CW) laser generates four subcarriers of different wavelengths, which act as optical carriers for modulation. The modulated signal is propagated over four different paths (spatial diversity), thus leading to a hybrid diversity FSO link, which includes both wavelength and spatial diversity.
The receiver side uses a power combiner to collect the information received from four independent channels. This process essentially improves the prospectus of recovering reliable information by combining and averaging out power received from different paths [50]. In our investigation, we have set the number of simultaneous users to four while the rest of the procedure related to signal modulation and transmission over diversified wavelengths remains the same. This arrangement helps in incrementing the transmission rates without any significant compromise on the quality of signal reception. The system presented in Figure 5 is capable of attaining transmission rates of up to 40 Gbps (4 × 10 Gbps). The comparative analysis of the proposed link over different sets of test conditions has been presented in Results and Discussion.
As set out in Table 2, the proposed link has been investigated for its performance behavior for the link range varying between 1,000 and 2000 m, while the channel turbulence is characterized by the gamma-gamma model. The PDF of its intensity I is given as follows [24]:
where Г(.) is the gamma function, K (α,β) is the Bessel function of second order and the parameter I is referred to as irradiative intensity of the transmission, while α and β characterize the presence of small and large turbulence cells in the channel and are mathematically related as follows:
where the value of σ2 represents variance is associated with the respective turbulence consideration for the available distribution of channel elements.
The turbulence regime within the channel can be characterized by the parameter Cn2, also known as the refractive index structure parameter. The mathematical formulation for the static turbulence can be represented as follows [1]:
where Ct2 represents the mean square temperature between two points within the link, T is the absolute temperature in Kelvin, and P is pressure in Pascals. The values of Cn2 ≈ 10–15 m−2/3 symbolize a moderate turbulence regime [6]. The received signals are filtered using a Bessel filter of order four and then analyzed for quality reception using a bit error analyzer. Various design properties such as transmission bit rate, symbol rate, choice of wavelength, modulation type, and signal recovery setup act as key influencing factors that dictate the system performance.
Results and Discussion
The proposed link has been investigated for a different set of parameters described previously in Tables 1 and 2. The spectrum of the signal captured at the transmitter and then at the receiver is shown in Figures 6A,B respectively. The captured spectrum also characterizes a hybrid FSO link wherein the data is transmitted over four different optical sub-carriers with wavelengths in the range of 1,550–1,553 nm. In this particular case, the receiver is 2000 m away from the transmitter while the link attenuation is 12 dB/km. Analysis of Figures 6A,B illustrates a comparative decrease in power levels of all four carriers after propagating through the FSO channel.
In contrast to the transmission power, the received signal shows degraded power levels that are attributed to channel losses like scattering and absorption. It is imperative to mention here that the transmission power levels cannot be increased beyond a certain threshold value due to the limitation imposed by underlying opto-electronics. Hence transmission power cannot be used as a mitigation tool to overcome link losses. Moreover, keeping in view the eye and skin safety standards set by different governing agencies, the transmission powers must be within the prescribed human eye safe limits.
Figure 7 indicates the pattern of signal to noise ratio (SNR) observed for the proposed system for variable transmission power while the link length is 1,000 m. It can be seen here that with the increase in transmission power, the performance of the link, i.e., achievable SNR, improves. Previously, transmission power solely cannot be used as a mitigation factor due to eye safety regulation. Hence, for the same reason, we have proposed wavelength and spatial diversity to complement our strategy in delivering cost-viable and high-speed FSO links that can address the needs of last-mile connectivity. From Figure 7, we also observe that for transmission power of 15 dB m, the SNR recorded at the receiver reaches 43 dB (approximately) under ideal channel conditions, while under adverse weather conditions, the recorded SNR nose dives to approximately 4 dB.
Figure 8 highlights the relation between received signal power and link distance for different meteorological conditions. It can be clearly seen here that as on expected lines, the received power degrades as link distance increases. For the link range of 1,000 m, the received power reduces from −21 dB m to −78 dB m (approx.) as link attenuation increases from 0.468 dB/km to 25 dB/km, respectively.
The relationship between link distance and bit error rate (BER) under different atmospheric conditions is presented in Figure 9, where we observe that under clear atmospheric conditions, i.e., when link attenuation is 0.468 dB/km, the BER of the proposed system is recorded to be around 2.62 × 10–28, while the link range is 1,000 m. However, as the link conditions worsen (25 dB/km), the BER increases to 4.3 × 10–5 for the same link conditions. The BER degradation is further confirmed by the eye diagrams for different link conditions shown in Figure 9. The eye-opening patterns determine the quality of the received signal. The improved eye-opening (eye height) then corresponds to a successful reception of the transmitted information. The distortions in the eye diagrams indicate loss of coherence which may lead to possibly erroneous detection.
The BER performance analysis of the proposed system using EDFA-based post-amplification is presented in Figure 10. As seen from Figure 10, a consistently low BER pattern is observed for clear weather conditions for the entire link range of up to 2000 m. This is followed by a near similar performance under moderately adverse channel conditions. However, significant BER performance variation is witnessed when severe channel conditions prevail. For extremely adverse channel conditions (25 dB/km) and forward error correction (FEC)-BER limit of 10–9 as a performance benchmark, it can be seen from Figure 9 that an EDFA-compensated FSO link delivers a link range of approximately 1,520 m. This link range is approximated 600 m more than the uncompensated FSO link shown in Figure 9, which is found to be restricted to a link range of 926 m under similar link conditions. The relation between the signal to noise ratio (SNR dB) and link range is presented in Figure 11 and Figure 12 for various weather conditions. At reference link range of 1,000 m, the uncompensated FSO link shown in Figure 11 is found to deliver SNR (dB) of 54.87 dB, 28.77, and 5.48 dB for clear, moderate, and severe channel conditions, respectively. The performance enhancement in SNR (dB) using the post-amplification using EDFA can be ascertained from Figure 12, wherein SNR value of 40.7 dB can be achieved at the link range of 1,000 m under extremely adverse channel conditions (25 dB/km).
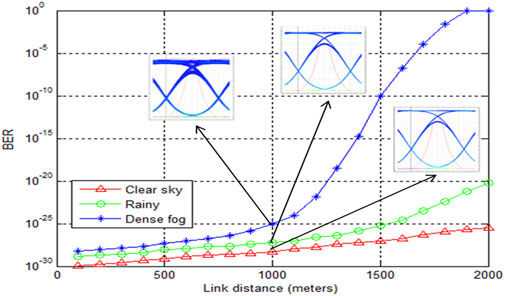
FIGURE 10. Relationship between received BER and link distance at various atmospheric conditions with EDFA.
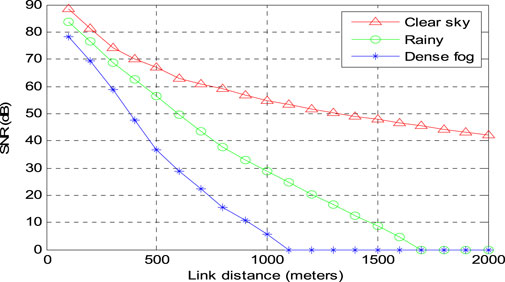
FIGURE 11. Relationship between SNR and link distance for different meteorological conditions without link compensation.
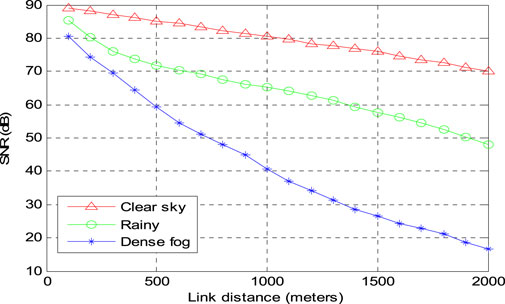
FIGURE 12. Relationship between SNR and link distance for different meteorological conditions while using EDFA.
Thus, a significant improvement in the received SNR compared to the uncompensated FSO link is witnessed here. Moreover, from Figure 12, it can be observed further that for a link distance of 2000 m, the EDFA-compensated link delivers decent SNR while the uncompensated link is rendered useless under similar channel conditions. The detailed comparison of link performances illustrated in Figure 10 Figure 11 has been complied in Table 3. It can be seen from Table 3 that BER of EDFA-compensated FSO links degrades merely by a factor of 10–3 from 10–29 under clear weather conditions to 10–26 for severe conditions. The uncompensated link, however, witnesses massive deviations as BER are found to vary between 10–28 and 10–5. Similar improvements in Q-factor and receiver SNR (dB) can also be observed for compensated FSO links in contrast to non-compensated links over varied atmospheric conditions. The admissible values of FEC (Forward Error Correction) for BER is ∼10–3, and for SNR, it is ∼20 dBm, for which the communication system proves to be working fine. FEC refers to the performance threshold which must be achieved by the system in order to provide end-to-end connectivity for voice and data related services [52, 53]. As mentioned in Table 3, the BER and SNR rates of the proposed link are pretty much beyond the FEC threshold limits.
Conclusion
In this paper, we have presented a holistic analysis of the proposed hybrid FSO link that uses the principle of spatial and wavelength diversity to overcome atmosphere-induced limitations. It has been observed that the link performance degrades as the attenuation levels increase, thus rendering the link useless. However, the inclusion of EDFA-based all-optical compensation plays a critical role in propelling the performance under adverse channel conditions. For attenuation levels as high as 25 dB/km and with the use of EDFA, the proposed link witnesses link range improvement of approximately 600 m over uncompensated FSO links considering the FEC-BER limit as a benchmark. Also, the proposed link incorporates multiple users, thus delivering an effective transmission rate of 40 Gbps. At this data rate, the proposed link is a promising answer to deliver cost-effective, high-speed data access to end-users. The possible future scope of the work could be its inclusion in future mobile generations of 5G and 6G as it is capable of delivering applications like virtual calling and holographic streaming. With aspects of decreased latency and increased reliability, the proposed system could be part of future driverless vehicles in which the response time should be minimal. Open access internet provision at public places with increased range and connectivity could be another possible future implementation of the proposed work.
Data Availability Statement
The raw data supporting the conclusions of this article will be made available by the authors without undue reservation.
Author Contributions
SM: writing original draft, methodology, simulations; RM: writing—review and editing, software, supervision; SC: writing—review and editing, data curation, supervision; FT: visualization, data curation; RR: formal analysis, project administration.
Conflict of Interest
The authors declare that the research was conducted in the absence of any commercial or financial relationships that could be construed as a potential conflict of interest.
Publisher’s Note
All claims expressed in this article are solely those of the authors and do not necessarily represent those of their affiliated organizations, or those of the publisher, the editors and the reviewers. Any product that may be evaluated in this article, or claim that may be made by its manufacturer, is not guaranteed or endorsed by the publisher.
References
1. Khalighi MA, Uysal M. Survey on Free Space Optical Communication: A Communication Theory Perspective. IEEE Commun Surv Tutorials (2014) 16:2231–58. doi:10.1109/COMST.2014.2329501
2. Chaudhary S, Tang X, Sharma A, Lin B, Wei X, Parmar A. A Cost-Effective 100 Gbps SAC-OCDMA-PDM Based Inter-satellite Communication Link. Opt Quant Electron (2019) 51(5):1–10. doi:10.1007/s11082-019-1864-2
3. Arezumand H, Zamiri-Jafarian H, Soleimani-Nasab E. Exact and Asymptotic Analysis of Partial Relay Selection for Cognitive RF-FSO Systems with Non-zero Boresight Pointing Errors. IEEE Access (2019) 7:58611–25. doi:10.1109/ACCESS.2019.2914480
4. Wani MY, Pathak H, Kaur K, Kumar A. Free Space Optical Communication System under Different Weather Conditions. J Opt Commun (2019) 72:52–4. doi:10.1515/joc-2019-0064
5. Upadhya A, Dwivedi VK, Singh G. Multiuser Diversity for Mixed RF/FSO Cooperative Relaying in the Presence of Interference. Opt Commun (2019) 442:77–83. doi:10.1016/j.optcom.2019.02.040
6. Miglani R, Malhotra JS. Investigation on R-S Coded Coherent OFDM Free Space Optical (CO-OFDM-FSO) Communication Link over Gamma-Gamma Channel. Wireless Pers Commun (2019) 109:415–35. doi:10.1007/s11277-019-06571-z
7. Kaur S, Bhardwaj P, Bhardwaj P. Performance of FSO Communication in the Atmospheric Turbulence for Various Modulation Schemes. Ijett (2018) 55(3):142–7. doi:10.14445/22315381/ijett-v55p226
8. Of N, La ADE. Communications. Revolutionary Russia (2008) 21(2):237. doi:10.1080/09546540802461498
9. Jeyaseelan J, Sriram Kumar D, Caroline BE. Disaster Management Using Free Space Optical Communication System. Photon Netw Commun (2019) 39:1–14. doi:10.1007/s11107-019-00865-9
10. Torkestani SS, Sahuguede S, Julien-Vergonjanne A, Cances JP. Indoor Optical Wireless System Dedicated to Healthcare Application in a Hospital. IET Commun (2012) 6(5):541–7. doi:10.1049/iet-com.2010.1116
11. Bhattacharya I. Advances in Optical Science and Engineering. Springer Proc Phys (2015) 166:467–77. doi:10.1007/978-81-322-2367-2
12. Odeyemi KO, Owolawi PA. Physical Layer Security in Mixed Rf/FSO System under Multiple Eavesdroppers Collusion and Non-collusion. Opt Quant Electron (2018) 50:1. doi:10.1007/s11082-018-1565-2
13. Upadhyay KK, Shukla NK, Chaudhary S. A High Speed 100 Gbps MDM-SAC-OCDMA Multimode Transmission System for Short Haul Communication. Optik (2020) 202:163665. doi:10.1016/j.ijleo.2019.163665
14. Ghassemlooy Z, Popoola WO. Terrestrial Free-Space Optical Communications, Mobile and Wireless Communications Network Layer and Circuit Level Design. SA Fares, and F Adachi, Editors. IntechOpen (2010). doi:10.5772/7698
15. Chaudhary S, Tang X, Wei X, Tang X, Wei X. Comparison of Laguerre-Gaussian and Donut Modes for MDM-WDM in OFDM-Ro-FSO Transmission System. AEU - Int J Electronics Commun (2018) 93(6):208–14. doi:10.1016/j.aeue.2018.06.024
16. Amphawan A, Chaudhary S, Chan VWS. 2x20 Gbps - 40 GHz OFDM Ro-FSO Transmission with Mode Division Multiplexing. JEOS:RP (2014) 9:1–6. doi:10.2971/jeos.2014.14041
17. Sharma M, Chadha D, Chandra V. Performance Analysis of MIMO-OFDM Free Space Optical Communication System with Low-Density Parity-Check Code. Photon Netw Commun (2016) 32(1):104–14. doi:10.1007/s11107-015-0579-y
18. Majumdar AK. Mitigation Techniques for Improved Free-Space Optical (FSO) Communications. Springer Ser Opt Sci (2015) 186.
19. Israr A, Israr A, Khan F, Khan F. Optimal Modulation Technique for MIMO FSO Link. Wireless Pers Commun (2019) 109(695)–714. doi:10.1007/s11277-019-06586-6
20. Zou L, Wang L, Xing C, Cui J, Zhao S. Turbulence Mitigation with MIMO Equalization for Orbital Angular Momentum Multiplexing Communication. Int Conf Wirel Commun Signal Process WCSP (2016) 1(1):1–4. doi:10.1109/WCSP.2016.7752455
21. Miglani R, Malhotra JS. Statistical Analysis of Fso Links Employing Multiple Transmitter/receiver Strategy over Double-Generalized and Gamma-Gamma Fading Channel Using Different Modulation Techniques. J Opt Commun (2019) 40(3):295–305. doi:10.1515/joc-2017-0066
22. Das A, Bag B, Bose C, Chandra A. Performance Analysis of MIMO FSO Link with Alamouti Coding and Switch-And-Examine Combining. Photon Netw Commun (2018) 36(3):350–60. doi:10.1007/s11107-018-0790-8
23. Fang J, Bi M, Xiao S, Yang G, Liu L, Zhang Y, et al. Polar-coded MIMO FSO Communication System over Gamma-Gamma Turbulence Channel with Spatially Correlated Fading. J Opt Commun Netw (2018) 10(11):915–23. doi:10.1364/JOCN.10.000915
24. Abou-Rjeily C. Performance Analysis of FSO Communications with Diversity Methods: Add More Relays or More Apertures?. IEEE J Select Areas Commun (2015) 33(9):1890–902. doi:10.1109/JSAC.2015.2432526
25. Vu BT, Thang TC, Pham AT. BER Analysis of MIMO/FSO Systems Using Rectangular QAM over Gamma-Gamma Channels. Int Conf Ubiquitous Futur Networks, ICUFN (2013) 987:370–4. doi:10.1109/ICUFN.2013.6614844
26. Dayal N, Singh P, Kaur P. Long Range Cost-Effective WDM-FSO System Using Hybrid Optical Amplifiers. Wireless Pers Commun (2017) 97:6055–67. doi:10.1007/s11277-017-4826-7
27. Pham TV, Thang TC, Pham AT. Average Achievable Rate of Spatial Diversity MIMO-FSO over Correlated Gamma-Gamma Fading Channels. J Opt Commun Netw (2018) 10(8):662–74. doi:10.1364/JOCN.10.000662
28. Sharda P, Bhatnagar MR. Diversity-Multiplexing Tradeoff for MIMO-FSO System under Different Transmission Scenarios with Limited Quantized Feedback. IEEE Access (2020) 8:114266–86. doi:10.1109/ACCESS.2020.3004135
29. Al-Gailani SA, Mohd Salleh MF, Salem AA, Shaddad RQ, Sheikh UU, Algeelani NA, et al. A Survey of Free Space Optics (FSO) Communication Systems, Links, and Networks. IEEE Access (2021) 9:7353–73. doi:10.1109/ACCESS.2020.3048049
30. Huang L, Liu S, Dai P, Li M, Chang G-K, Shi Y, et al. Unified Performance Analysis of Hybrid FSO/RF System with Diversity Combining. J Lightwave Technol (2020) 38(24):6788–800. doi:10.1109/JLT.2020.3018125
31. Sarangal H, Singh A, Malhotra J, Chaudhary S. A Cost Effective 100 Gbps Hybrid MDM-OCDMA-FSO Transmission System under Atmospheric Turbulences. Opt Quant Electron (2017) 49:184. doi:10.1007/s11082-017-1019-2
32. Dubey A, Prakash D. Performance Improvement of FSO Communication System Using MIMO Technique. Ijitee (2019) 8(10):3470–2. doi:10.35940/ijitee.j9722.0881019
33. Chatti I, Baklouti F, Chekir F, Attia R. Comparative Analysis of MIMO-Based FSO and MIMO-Based MGDM Communications. Opt Rev (2019) 26:631–43. doi:10.1007/s10043-019-00537-z
34. Shaker FK, Ali MAA, Ameer FSA. Utilization of MIMO Concept for Optical Communication System under Fog Condition. Transactions on Electrical Engineering (2019) 17:130–5.
35. Yang P, Zhu J, Xiao Y, Chen Z. Antenna Selection for MIMO System Based on Pattern Recognition. Digital Commun Networks (2019) 5(1):34–9. doi:10.1016/j.dcan.2018.10.001
36. Liu Y, Li H. Research on Transmission Performance of Multi-Input Multi-Output Free Space Optical Communication System Channel. Opt Rev (2019) 26(0):303–9. doi:10.1007/s10043-019-00495-6
37. Shah D, Kothari D. Performance Analysis of Free Space Optical Link with Wavelength Diversity under Weak and Moderate Turbulence Conditions. Sens Lett (2019) 17(2):137–43. doi:10.1166/sl.2019.4058
38. Badar N, Jha RK, Towfeeq I. Performance Analysis of an 80 (8 × 10) Gbps RZ-DPSK Based WDM-FSO System under Combined Effects of Various Weather Conditions and Atmospheric Turbulence Induced Fading Employing Gamma-Gamma Fading Model. Opt Quant Electron (2018) 50(1):1–11. doi:10.1007/s11082-017-1306-y
39. Ding J, Yu S, Fu Y, Ma J, Tan L. New Approximate and Asymptotic Closed-form Expressions for the Outage Probability and the Average BER of MIMO-FSO System with MRC Diversity Technique over Gamma-Gamma Fading Channels with Generalized Pointing Errors. Opt Commun (2020) 456:124633. doi:10.1016/j.optcom.2019.124633
40. Kumar Giri R, Patnaik B. Bit Error Rate Performance Analysis of Hybrid Subcarrier Intensity Modulation-Based Fso with Spatial Diversity in Various Weather Conditions. J Opt Commun (2019) 40(3):307–14. doi:10.1515/joc-2017-0073
41. Chowdhury R, Choyon AKMSJ. Design of 320 Gbps Hybrid AMI-PDM-WDM FSO Link and its Performance Comparison with Traditional Models under Diverse Weather Conditions. J Opt Commun (2021) 1:0135. doi:10.1515/joc-2020-0135
42. Sharoar Jahan Choyon AKM, Chowdhury R. Performance Comparison of Free-Space Optical (FSO) Communication Link under OOK, BPSK, DPSK, QPSK and 8-PSK Modulation Formats in the Presence of Strong Atmospheric Turbulence. J Opt Commun (2020) 1:1. doi:10.1515/joc-2019-0250
43. Dubey V, Chadha D, Chandra V. Experimental Validation of Multiple Gain Combiner in Single-Relay Cooperative FSO Communication Systems. Photon Netw Commun (2019) 37(1):110–9. doi:10.1007/s11107-018-0800-x
44. Miglani R, Malhotra JS. Evaluation of Link-Compensated 32 × 40 Gbit/s DWDM Free Space Optical (FSO) Transmission. J Opt (2018) 47(4):467–74. doi:10.1007/s12596-018-0484-8
45. Ciaramella E, Arimoto Y, Contestabile G, Presi M, D'Errico A, Guarino V, et al. 1.28 Terabit/s (32x40 Gbit/s) Wdm Transmission System for Free Space Optical Communications. IEEE J Select Areas Commun (2009) 27(9):1639–45. doi:10.1109/JSAC.2009.091213
46. Honde V, Mhatre A, Tonde S, Barkul S, Pund P. Performance Analysis of WDM Network Based on EDFA Amplifier with Different Pumping Techniques Performance Analysis of WDM Network Based on EDFA Amplifier with. Int J Recent Innov Trends Comput Commun (2016) 4(4):480–5.
47. Bayaki E, Michalopoulos DS, Schober R. EDFA-based All-Optical Relaying in Free-Space Optical Systems. IEEE Trans Commun (2012) 60(12):3797–807. doi:10.1109/TCOMM.2012.090512.110198
48. Liu H, Huang B, Zacarias JCA, Wen H, Chen H, Fontaine NK, et al. Turbulence-Resistant FSO Communication Using a Few-Mode Pre-amplified Receiver. Sci Rep (2019) 9(1):1–7. doi:10.1038/s41598-019-52698-1
49. Trinh PV, Dang NT, Pham AT. All-Optical Relaying FSO Systems Using EDFA Combined with Optical Hard-Limiter over Atmospheric Turbulence Channels. J Lightwave Technol (2015) 33(19):4132–44. doi:10.1109/JLT.2015.2466432
50. Priyadarshani R, Bhatnagar MR, Bohata J, Zvanovec S, Ghassemlooy Z. Experimental and Analytical Investigations of an Optically Pre-amplified FSO-MIMO System with Repetition Coding over Non-identically Distributed Correlated Channels. IEEE Access (2020) 8:12188–203. doi:10.1109/ACCESS.2020.2964149
51. Zhu J, Xiao X, Luo Y. An experiment Teaching Method Based on the Optisystem Simulation Platform. Proc SPIE (2017) 10452:198. doi:10.1117/12.2269886
52. Nguyen D-N, Bohata J, Komanec M, Zvanovec S, Ortega B, Ghassemlooy Z. Seamless 25 GHz Transmission of LTE 4/16/64-QAM Signals over Hybrid SMF/FSO and Wireless Link. J Lightwave Technol (2019) 37(24):6040–7. doi:10.1109/JLT.2019.2945588
Keywords: MIMO, free-space optics, wavelength diversity, EDFA amplification, cooperative diversity
Citation: Modalavalasa SK, Miglani R, Chaudhary S, Tubbal F and Raad R (2021) Developing Cost-Effective and High-Speed 40 Gbps FSO Systems Incorporating Wavelength and Spatial Diversity Techniques. Front. Phys. 9:744160. doi: 10.3389/fphy.2021.744160
Received: 19 July 2021; Accepted: 27 July 2021;
Published: 27 August 2021.
Edited by:
Santosh Kumar, Liaocheng University, ChinaReviewed by:
A. K. M. Sharoar Jahan Choyon, University of New Mexico, United StatesDharmendra Kumar, Madan Mohan Malaviya University of Technology, India
Copyright © 2021 Modalavalasa, Miglani, Chaudhary, Tubbal and Raad. This is an open-access article distributed under the terms of the Creative Commons Attribution License (CC BY). The use, distribution or reproduction in other forums is permitted, provided the original author(s) and the copyright owner(s) are credited and that the original publication in this journal is cited, in accordance with accepted academic practice. No use, distribution or reproduction is permitted which does not comply with these terms.
*Correspondence: Sushank Chaudhary, c3VzaGFua2NoYXVkaGFyeUBnbWFpbC5jb20=