- 1Department of Engineering and Technology, Guru Nanak Dev University, Jalandhar, India
- 2Department of Electronics and Communication Engineering, SIET, Amritsar, India
- 3Center for Interdisciplinary Programs, Indian Institute of Technology Hyderabad, Telangana, India
- 4Department of Computer Science and Engineering, Punjab Engineering College (Deemed to be University), Chandigarh, India
- 5Department of Electronics and Communication Engineering, Shaheed Bhagat Singh State University, Ferozepur, India
- 6Department of Computer Application, ABES Engineering College, Ghaziabad, India
- 7Department of Electronics and Communication Engineering, Vivekanandha College of Technology for Women, Tiruchengode, India
- 8Department of Engineering and Technology, Guru Nanak Dev University, Gurdaspur, India
The requirement of high data rate information transmission is rising exponentially for supporting different services including social networking, web streaming, and biomedical sensor data transmission. Such services required high channel bandwidth with secure information transmission and immunity to electromagnetic interference. Radio over free space optics (RoFSO) is witnessed as a promising technological solution to provide high data rate transmission over free space channel. We report on the design of a 2
Introduction
In the present-day telecommunications sector, the base transceiver station (BTS)-BTS connection is established through fiber optic trunk cables in which voice/data are transmitted at high speed using radio over fiber (RoF) technology. Also, in order to establish a BTS-user communication link, a radio-frequency (RF) network is deployed. Nevertheless, fiber installation constrictions both in urban and rural areas, excessive infrastructure costs, and hefty spectrum pricing in the case of RF technology make such approaches unattractive and unfavorable for the establishment of next-generation wireless networks. To overcome this trouble, a radio over free space optics (RoFSO) link, where the RF signal will be transmitted between BTS-BTS or BTS-user using an optical carrier (up-conversion) and air/free space as the medium, is a viable solution. RoFSO technology provides unlicensed spectrum operation, interference, and interception-free transmission within a short deployment period and little expense [1]. To enhance the spectral efficiency and information-carrying capacity of RoFSO links, the mode division multiplexing (MDM) technique is incorporated in which independent RF signals are transmitted over distinct spatial modes of a single frequency laser beam [2–6]. Recently, Satea et al. proposed that FSO systems employing multiple channels with erbium-doped fiber amplifiers (EDFAs) work efficiently under high attenuation environments such as heavy fog and dust [7]. Alaa et al. analyzed the FSO system performance in fog and sandstorm conditions by changing the operating wavelength, receiver diameter aperture, photodetector type, and the modulation technique. They found that both the dense fog and dust triggered 87 and 95% power loss, respectively, at a transmission range of 1 km [8]. Maged et al. put forth an experimental evaluation of an all-optical hybrid FSO/RF link carrying 5G signals in a dust channel. They reported that the RF link with low bandwidth works well in dense dust and the FSO operates once the weather improves beyond a definite threshold. As a consequence, the FSO/RF parallel link can be a first-rate choice towards overcoming dust effects [9]. Matthew et al. proposed a novel visibility and dust absorption model after using visibility and absorption measurements made farther from the source (10–100 km) to demonstrate the impact of reduced dust particle size over the regional distance from the source [10]. Haichao et al. described that in addition to wavelength, particle sizes of sand and dust affect the laser beam attenuation [11]. Zabih et al. reported that the FSO link performance can be improved by increasing the launched optical power and selecting adequate line speed under sandstorm conditions [12]. The current research in RoFSO is mainly focused on fog- and smoke-induced attenuation [13–16]. Intensive investigation and modeling of the dust effects on the RoFSO channel are yet to grab the attention of researchers. Very limited or basically no attempt has been made in this course, and the development and investigation of MDM-based RoFSO transmission for dust environment has not been reported till date. In arid and semiarid regions, the effect of dust will remain paramount for any upcoming installation of the RoFSO network in 5G and smart city applications [17]. It is straightforward that the propagation prediction under dust storms regarding signal attenuation, maximum reachable distance, and error performance will be the solemn challenge for the setting up of the RoFSO communication network.
This work reports the designing and performance evaluation of an MDM-RoFSO transmission for varying levels of dust and clear climate conditions. This research article is structured as follows: FSO Evaluation in Dust Channel discusses the numerical model for dust storm characteristics and attenuation in FSO. Simulation Setup Description describes the proposed simulation setup and parameters. Results and Discussion presents the simulation results, and Conclusion concludes the paper.
FSO Evaluation in Dust Channel
In FSO, the chosen signal wavelength belongs to the atmospheric transmission window which provides low molecular absorption since absorption cross-section
The second approach used in the literature for the calculation of attenuation in FSO for real-world applications rests on experimental remark [22]. This technique is based on experiential models established by means of visibility range statistics to identify the propagation path characteristics [23]. Link visibility information can be acquired from meteorological stations situated near the setup area. In [24], visual range is described as the distance to an entity at which the picture distinction falls to 2% of the original ocular contrast (100%) along the transmission distance usually referred to as the Koschmieder law. This 2% falloff value recognized as the visual threshold
Depending on the visibility, dust storms are classified into four groups as specified in Table 1. Type 1 is dust haze (very light dust) wherein widespread dust particles drift up and happen to remain suspended evenly in the atmosphere as a result of dust storm that begins at a large distance from the monitoring point. The visibility associated with this occasion is about 10 km or less. Type 2 is blowing dust (light dust) in which the dust is blown in the direction of monitoring position by the action of winds. As a result, the visibility drops between 1 and 10 km. Type 3 is dust storm (moderate dust) where strong winds bluster additional dust elements inside the observation area and the visibility decreases between 0.2 and 1 km. Finally, type 4 is severe dust storm (dense dust) which arises after a large volume of dust particles are blown by violent winds, and the visibility further drops below 0.2 km. The visibility
where
Moreover in [28], the FSO signal attenuation model as a function of the visibility for desert environment that is prone to frequent dust storms is obtained as:
Eq. 3 holds under the condition that the operating wavelength used in FSO communication is 1,550 nm since it provides lowest atmospheric attenuation and minor absorption loss, besides its technology is mature in terms of fabrication of optoelectronic devices.
Simulation Setup Description
The proposed MDM-based RoFSO link setup employing RZ and NRZ binary digital optical modulation is illustrated in Figure 1, which has been designed using the Optisystem™ photonic software. Two independent 10 GHz RF signals are modulated over different HG mode beams, that is, HG00 and HG01 and transported over the FSO channel. Eq. 4 mathematically describes the intensity profiles of HG modes (Figures 2A,B) as [29]:
where
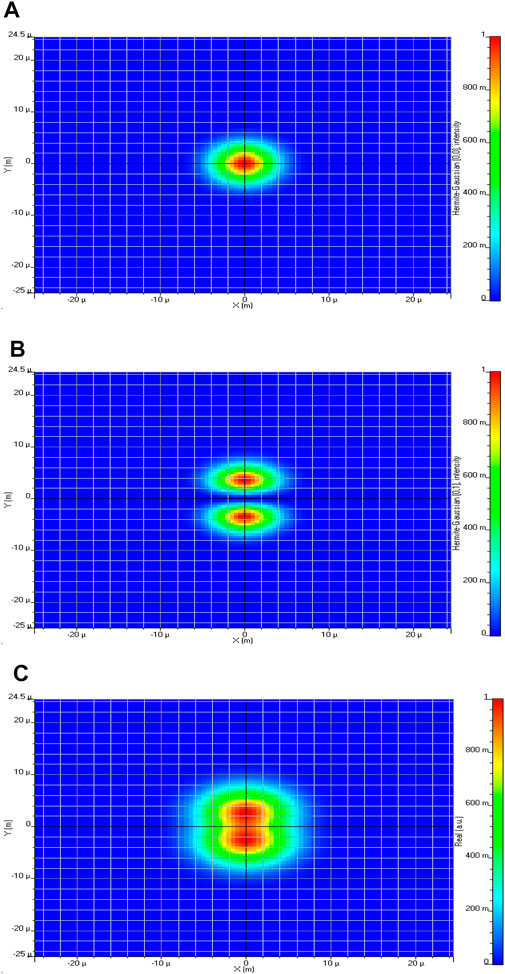
FIGURE 2. Spatial intensity pattern of the (A) HG00 mode, (B) HG01 mode, and (C) transmitted MDM signal.
A 10 Gb/s information-carrying signal is produced by a pseudorandom bit sequence generator (PRBS) for distinct channels and encoded using RZ/NRZ schemes. In RZ-encoded signal, bit “1” is transported by an optical pulse with half bit period. Whereas in NRZ-encoded signal, bit “1” is transported through an optical signal of entire bit period, while in place of bit “0”, no optical signal is conveyed. A Mach–Zehnder modulator (MZM) is utilized to superimpose the encoded information onto a 10-GHz RF signal and a distinct HG mode. This information signal is subsequently communicated into the FSO channel through HG00 and HG01 modes of the 193.1 THz frequency channel.
Mathematically, the signal received after FSO transmission can be modeled as [32]:
where
Results and Discussion
Firstly, we compare the performance of NRZ and RZ modulation-based MDM-RoFSO link under a clear climate. Secondly, we investigate the proposed MDM-RoFSO link utilizing NRZ modulation for four different dust storm conditions. The performance analysis has been carried out with regard to BER, transmission range, and eye diagram.
Figure 3 presents RZ/NRZ-MDM-RoFSO transmission system performance in terms of BER and range under clear weather conditions where each HG mode (HG00 and HG01) carries independent 10 Gb/s-10 GHz information. Though BER of the system degrades with increasing transmission distance for both the channels, it can be found from Figure 3A, using NRZ modulation scheme, the maximum achievable link range is 14.5 km while it decreases to 10 km for RZ modulation in Figure 3B under acceptable performance criteria [log (BER)
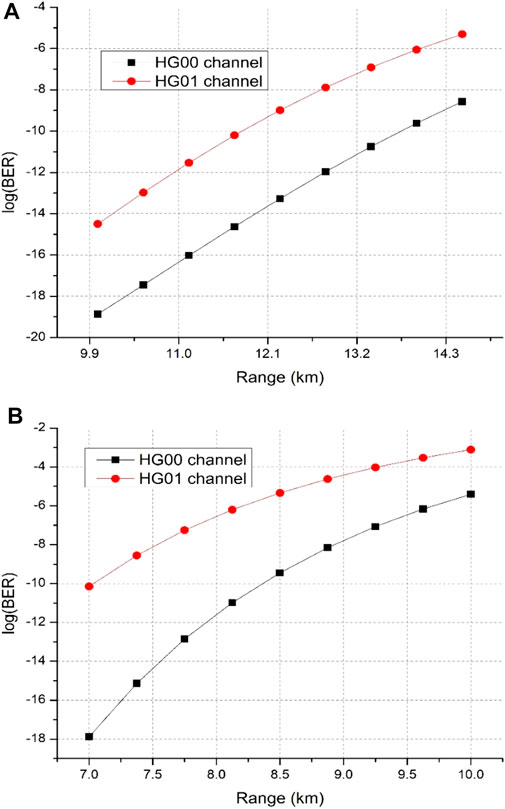
FIGURE 3. log (BER) v/s transmission distance for clear weather using (A) NRZ modulation and (B) RZ modulation.
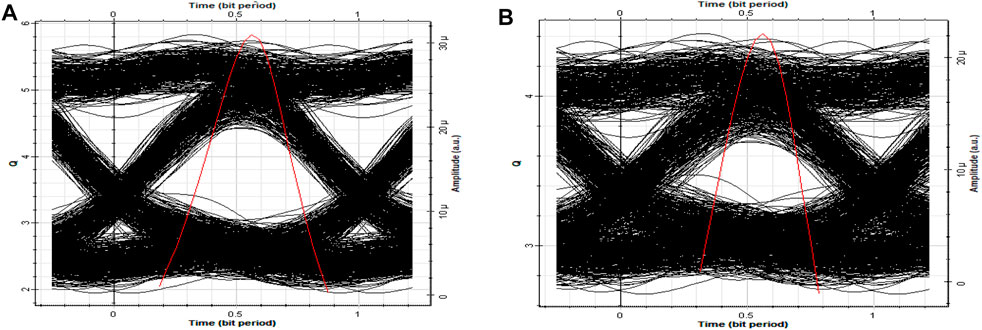
FIGURE 4. Eye diagram using the proposed NRZ-based MDM-RoFSO link at 14.5 km transmission distance under clear weather for the (A) HG00 channel and (B) HG01 channel.
To determine how far and well the proposed link can function under four different dust storm situations, we draw the log of BER versus maximum reachable distance graphs. In addition, to demonstrate the reliability of our observations, we report the eye diagrams using the proposed system for each maximum reachable range with regard to specific dust conditions for both the channels.
Figure 5 shows a log of BER versus range curve under very light dust conditions, that is,
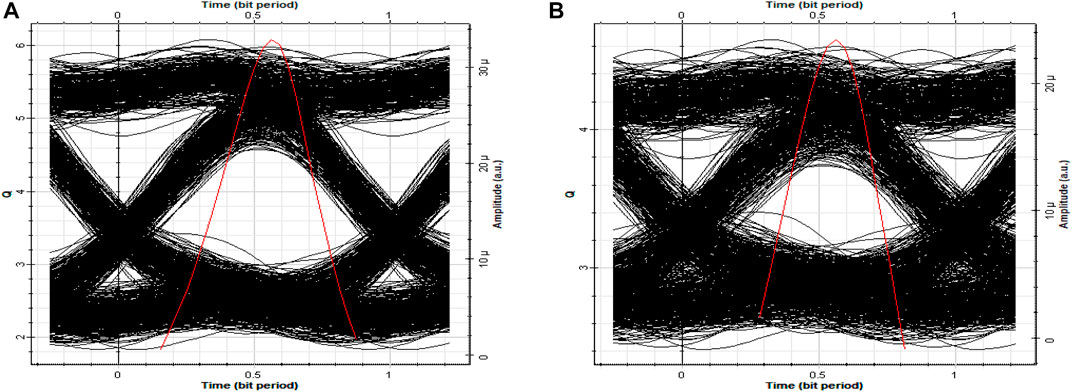
FIGURE 6. Eye diagram using the proposed NRZ-based MDM-RoFSO system at 2,500 m transmission distance under very light dust for the (A) HG00 channel and (B) HG01 channel.
The proposed NRZ-based MDM-RoFSO system performance in light dust conditions (i.e.,
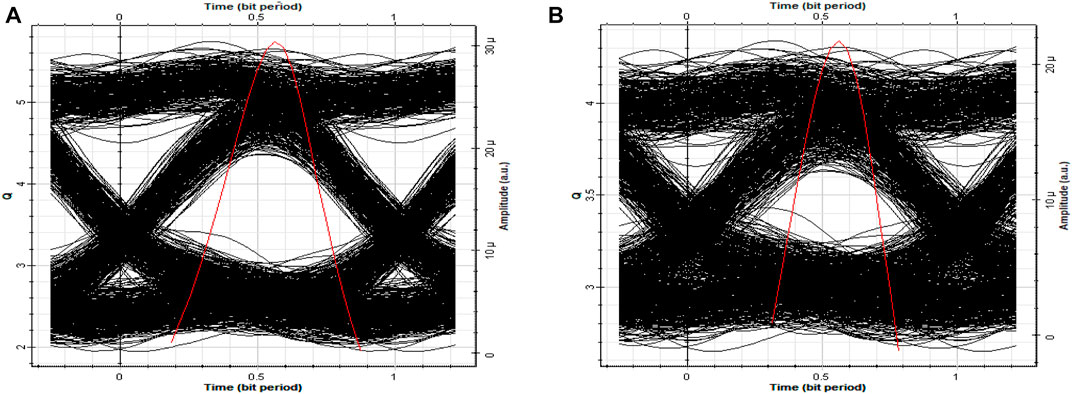
FIGURE 8. Eye diagram using the proposed NRZ-based MDM-RoFSO link at 950 m transmission distance under light dust conditions for the (A) HG00 channel and (B) HG01 channel.
Figure 9 illustrates the log (BER) versus range graph under moderate dust conditions (i.e., 0.2 km < V < 1 km). As can be seen, the BER performance of the received signals reduces from a log (BER) of
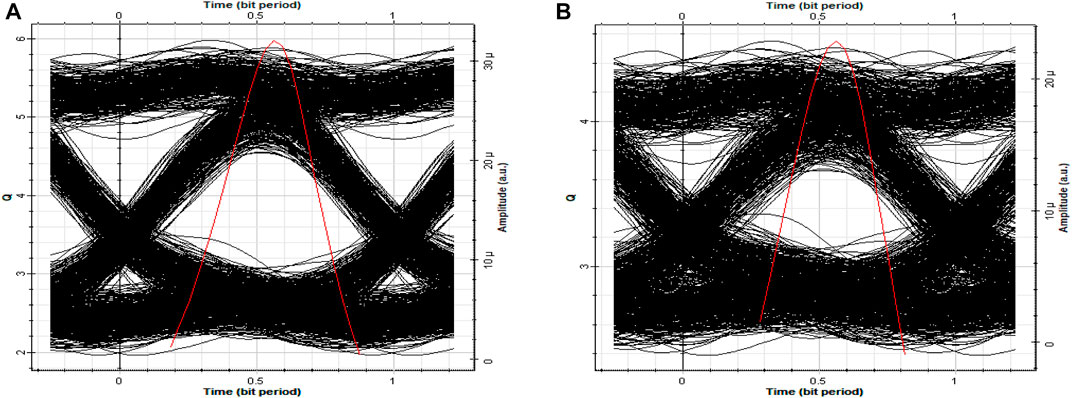
FIGURE 10. Eye diagram using the proposed NRZ-based MDM-RoFSO system at 275 m transmission distance under moderate dust conditions for the (A) HG00 channel and (B) HG01 channel.
The transmission performance of the proposed system under dense dust (i.e., V < 0.2 km) as a function of the log (BER) and transmission range is shown in Figure 11. As can be noticed, the BER performance of the received signal deteriorates from a log (BER) of
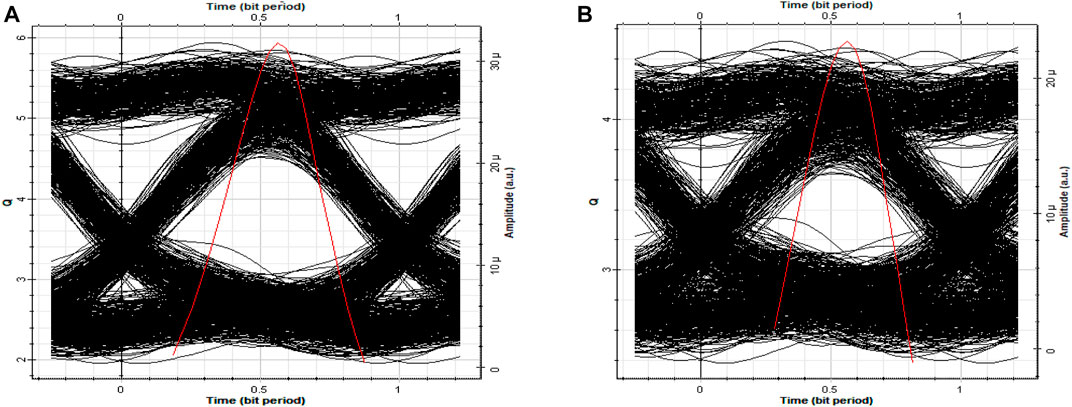
FIGURE 12. Eye diagram using the proposed NRZ-based MDM-RoFSO link at 108 m transmission distance under dense dust for the (A) HG00 channel and (B) HG01 channel.
Table 3 compares the transmission performance of the proposed system with recent works and demonstrates that the proposed system achieves higher information capacity. The higher range in recent works can be attributed to the fact that the authors have considered higher transmission power, that is, 22 dBm (Ref [7, 23]) and 50 dBm (Ref. [33]), as compared to 14 dBm in this work.
Conclusion
RoFSO transmission systems integrated with MDM technology can provide a viable solution for providing secure biomedical sensor data transmission in medical facilities. In this work, an NRZ/RZ digital optical modulation schemes based MDM-RoFSO transmission system is proposed and investigated. The outcomes presented demonstrate that NRZ modulation performs significantly better in terms of providing extra 4.5 km coverage as compared to the RZ modulation format. We first investigated the performance reliability and availability of a NRZ-based MDM-RoFSO transmission system in a dust environment. The results obtained show the likelihood of establishing short FSO networks under light and moderate dust circumstances. Nevertheless, rarely encountered dense dust scenarios can significantly affect the performance of MDM-RoFSO links. Additionally, because the 5G network uses ultra-compact cells with modest separation, the proposed NRZ-based MDM-RoFSO link can prove to be a promising substitute to fiber cables in the fronthaul and backhaul sectors of next-generation wireless networks. In future works, the transmission capacity of the proposed system can further be enhanced by incorporating polarization multiplexing along with higher-order modulation schemes.
Data Availability Statement
The original contributions presented in the study are included in the article/Supplementary Material, and further inquiries can be directed to the corresponding author.
Author Contributions
MS: Conceptualization, methodology, investigation, writing—original draft, supervision, resources, project administration. SP: Methodology, investigation, writing—original draft, supervision. S: Methodology, investigation, data curation, visualization. SD: Investigation, data curation, writing—original draft. R: Investigation, data curation, visualization. AG: Writing—review and editing, validation, conceptualization. AM: Investigation, data curation, visualization. AM: Writing—review and editing, validation, conceptualization.
Conflict of Interest
The authors declare that the research was conducted in the absence of any commercial or financial relationships that could be construed as a potential conflict of interest.
Publisher’s Note
All claims expressed in this article are solely those of the authors and do not necessarily represent those of their affiliated organizations, or those of the publisher, the editors, and the reviewers. Any product that may be evaluated in this article, or claim that may be made by its manufacturer, is not guaranteed or endorsed by the publisher.
References
1. Singh M, Malhotra J. Performance Comparison of M-QAM and DQPSK Modulation Schemes in a 2 × 20 Gbit/s-40 GHz Hybrid MDM-OFDM-Based Radio over FSO Transmission System. Photon Netw Commun (2019) 38(3):378–89. doi:10.1007/s11107-019-00861-z
2. Singh M, Malhotra J. Performance Comparison of High-Speed Long-Reach Mode Division Multiplexing-Based Radio over Free Space Optics Transmission System Using Different Modulation Formats under the Effect of Atmospheric Turbulence. Opt Eng (2019) 58(4):1. doi:10.1117/1.OE.58.4.046112
3. Sharma A, Malhotra J, Chaudhary S, Thappa V. Analysis of 2 × 10 Gbps MDM Enabled Inter Satellite Optical Wireless Communication under the Impact of Pointing Errors. Optik (2021) 227:165250. doi:10.1016/j.ijleo.2020.165250
4. Amphawan A, Chaudhary S, Ghassemlooy Z, Neo T-K. 2×2-channel Mode-Wavelength Division Multiplexing in Ro-FSO System with PCF Mode Group Demultiplexers and Equalizers. Opt Commun (2020) 467:125539. doi:10.1016/j.optcom.2020.125539
5. Amphawan A, Chaudhary S, Chan V. Optical Millimeter Wave Mode Division Multiplexing of LG and HG Modes for OFDM Ro-FSO System. Opt Commun (2019) 431:245–54. doi:10.1016/j.optcom.2018.07.054
6. Chaudhary S, Amphawan A. Solid Core PCF-Based Mode Selector for MDM-Ro-FSO Transmission Systems. Photon Netw Commun (2018) 36:263–71. doi:10.1007/s11107-018-0778-4
7. Alnajjar SH, Noori AA, Moosa AA. Enhancement of FSO Communications Links under Complex Environment. Photonic Sens (2017) 7(2):113–22. doi:10.1007/s13320-017-0336-1
8. Algamal AA, Fayed HA, Mahmoud M, Aly MH. Reliable FSO System Performance Matching Multi-Level Customer Needs in Alexandria City, Egypt, Climate: Sandstorm Impact with Pointing Error. Opt Quant Electron (2020) 52(18):1. doi:10.1007/s11082-020-02468-5
9. Esmail MA, Ragheb AM, Fathallah HA, Altamimi M, Alshebeili SA. 5G-28 GHz Signal Transmission over Hybrid All-Optical FSO/RF Link in Dusty Weather Conditions. IEEE Access (2019) 7:24404–10. doi:10.1109/ACCESS.2019.2900000
10. Baddock MC, Strong CL, Leys JF, Heidenreich SK, Tews EK, Mctainsh GH. A Visibility and Total Suspended Dust Relationship. Atmos Environ (2014) 89:329–36. doi:10.1016/j.atmosenv.2014.02.038
11. Zhong H, Zhou J, Du Z, Xie L. A Laboratory Experimental Study on Laser Attenuations by Dust/sand Storms. J Aerosol Sci (2018) 121:31–7. doi:10.1016/j.jaerosci.2018.04.004
12. Ghassemlooy Z, Perez J, Leitgeb E. On the Performance of FSO Communications Links under Sandstorm Conditions. In: Proceedings of the 12th International Conference on Telecommunications, 2013, June 26-28, Zagreb, Croatia. Zagreb (2013). p. 53–8.
13. Chaudhary S, Lin B, Tang X, Wei X, Zhou Z, Lin C, et al. 40 Gbps-80 GHz PSK-MDM Based Ro-FSO Transmission System. Opt Quant Electron (2018) 50:321. doi:10.1007/s11082-018-1592-z
14. Chaudhary S, Amphawan A. High-speed MDM-Ro-FSO System by Incorporating Spiral-Phased Hermite Gaussian Modes. Photon Netw Commun (2018) 35:374–80. doi:10.1007/s11107-017-0752-6
15. Chaudhary S, Amphawan A. High-speed Millimeter Communication through Radio-Over-Free-Space-Optics Network by Mode-Division Multiplexing. Opt Eng (2017) 56(11):1. doi:10.1117/1.OE.56.11.116112
16. Chaudhary S, Tang X, Wei X. Comparison of Laguerre-Gaussian and Donut Modes for MDM-WDM in OFDM-Ro-FSO Transmission System. AEU - Int J Elect Commun (2018) 93:208–14. doi:10.1016/j.aeue.2018.06.024
17. Kumar A, Krishnan P. Performance Analysis of RoFSO Links with Spatial Diversity over Combined Channel Model for 5G in Smart City Applications. Opt Commun (2020) 466:125600. doi:10.1016/j.optcom.2020.125600
18. Bohren CF, Huffman DR. Absorption and Scattering of Light by Small Particles. Germany: A Wiley-Interscience publication (1998). doi:10.1002/9783527618156
19. Kim II, Mcarthur B, Korevaar EJ, Street R, Diego S. Comparison of Laser Beam Propagation at 785 Nm and 1550 Nm in Fog and Haze for Optical Wireless Communications. In: Proceeding SPIE 4214, Optical Communications III, 2001, February 6, Boston, MA, United States (2001). doi:10.1117/12.417512
20. Khalighi MA, Uysal M, Marseille C, Engineering E. Survey on Free Space Optical Communication: A Communication Theory Perspective. IEEE Commun Surv Tutorials (2014) 16(4):2231–58. doi:10.1109/COMST.2014.2329501
21. Hemmati H, Djordjevic IB, Biswas A. Deep-Space Optical Communications: Future Perspectives and Applications. Proc IEEE (2011) 99(11):2020–39. doi:10.1109/JPROC.2011.2160609
22. Ijaz M, Ghassemlooy Z, Pesek J, Fiser O, Le Minh H, Bentley E. Modeling of Fog and Smoke Attenuation in Free Space Optical Communications Link under Controlled Laboratory Conditions. J Lightwave Technol (2013) 31(11):1720–6. doi:10.1109/JLT.2013.2257683
23. Esmail MA, Fathallah H, Alouini M-S. Effect of Dust Storms on FSO Communications Links. In: 2016 4th International Conference on Control Engineering & Information Technology (CEIT), 2016, December 16-18, Hammamet, Tunisia. Hammamet (2016). 1–6. doi:10.1109/CEIT.2016.7929046
24. Pierce RM, Ramaprasad J, Eisenberg EC. Optical Attenuation in Fog and Clouds. In: Proceeding SPIE, 4530, Optical Wireless Communications IV, 2001, November 27, Denver, CO, United States (2001). 58–71. doi:10.1117/12.449815
25. Prokes A. Atmospheric Effects on Availability of Free Space Optics Systems. Opt Eng (2009) 48(6):066001. doi:10.1117/1.3155431
26. Cao M, Wang H, Yao Y, Hou S. Performance Evaluation of FSO Communications under Sand-Dust Conditions. Int J Antennas Propagation (2019) 2019:1–11. doi:10.1155/2019/2046896
27. Ricklin JC, Hammel SM, Eaton FD, Lachinova SL. Atmospheric Channel Effects on Free-Space Laser Communication. J Optic Comm Rep (2006) 3:111–58. doi:10.1007/s10297-005-0056-y
28. Esmail MA, Fathallah H, Alouini M-S. An Experimental Study of FSO Link Performance in Desert Environment. IEEE Commun Lett (2016) 20(9):1888–91. doi:10.1109/LCOMM.2016.2586043
29. Ghatak A, Thyagarajan K. An Introduction to Fiber Optics. Cambridge: Cambridge University Press (1998). doi:10.1017/CBO9781139174770
30. Nyugen D-N, Bohata J, Spáčil J, Komanec M, Stevens N, Ghassemlooy Z, et al. Polarization Division Multiplexing-Based Hybrid Microwave Photonic Links for Simultaneous mmW and Sub 6-GHz Wireless Transmissions. IEEE Photon J (2020) 12(6):1–16. doi:10.1109/JPHOT.2020.3036440
31. Kakati D, Arya SC. A Full-Duplex Optical Fiber/wireless Coherent Communication System with Digital Signal Processing at the Receiver. Optik (2018) 171:190–9. doi:10.1016/j.ijleo.2018.05.140
32. Ghassemlooy Z, Popoola WO. Terrestrial Free Space Optical Communications. In: S A Fares, and F Adachi, editors. Mobile and Wireless Communication Network Layer and Circuit Level Design. London, United Kingdom: InTech (2010). doi:10.5772/7698
33. Ali MAA, Shaker FK, Kadhum HA. Investigation and Analysis of Data Rate for Free Space Optical Communications System under Dust Conditions. Wirel Pers Commun (2020) 113:2327–38. doi:10.1007/s11277-020-07328-9
34. Mourka A, Mazilu M, Wright EM, Dholakia K. Modal Characterization Using Principal Component Analysis: Application to Bessel, Higher-Order Gaussian Beams and Their Superposition. Sci Rep (2013) 3:1422. doi:10.1038/srep01422
35. Amphawan A, O'Brien D. Modal Decomposition of Output Field for Holographic Mode Field Generation in a Multimode Fiber Channel. In: International Conference on Photonics, 2010, July 5-7, Langkawi, Malaysia. Langkawi (2010). 1–5. doi:10.1109/ICP.2010.5604377
Keywords: HG, biomedical sensor data, MDM, RoFSO, 5G technology
Citation: Singh M, Pottoo SN, Suvidhi , Dewra S, Rishabh , Grover A, Manikandan A and Sheetal A (2021) Performance Investigation of a High Data Rate Mode Division Multiplexed-Free Space Optics Link Under Harsh Weather Conditions. Front. Phys. 9:743545. doi: 10.3389/fphy.2021.743545
Received: 18 July 2021; Accepted: 27 July 2021;
Published: 31 August 2021.
Edited by:
Santosh Kumar, Liaocheng University, ChinaReviewed by:
Sushank Chaudhary, Quanzhou Institute of Equipment manufacturing, Chinese academy of Sciences, China, ChinaDr. Shyam Akashe, ITM University, India
Copyright © 2021 Singh, Pottoo, Suvidhi, Dewra, Rishabh, Grover, Manikandan and Sheetal. This is an open-access article distributed under the terms of the Creative Commons Attribution License (CC BY). The use, distribution or reproduction in other forums is permitted, provided the original author(s) and the copyright owner(s) are credited and that the original publication in this journal is cited, in accordance with accepted academic practice. No use, distribution or reproduction is permitted which does not comply with these terms.
*Correspondence: Amit Grover, YW1pdGdyb3ZlcjMyMUBnbWFpbC5jb20=