- 1Department of Radiation Oncology, Yonsei Cancer Center, Yonsei University College of Medicine, Seoul, South Korea
- 2Department of Radiation Oncology, Samsung Medical Center, Sungkyunkwan University School of Medicine, Seoul, South Korea
- 3Department of Radiation Oncology, Yonsei Cancer Center, Seoul, South Korea
The aim of this study was to evaluate the dosimetric characteristics of a semi-customized tongue displacement device (SCTDD) fabricated using a 3D printer for patients receiving unilateral head and neck irradiation with intensity-modulated proton therapy (IMPT) and assess its feasibility as a proton beam stopper compared to a standard mouthpiece (SMP). Seven consecutive patients, three with tonsil cancer, and four with oral cavity cancer were included in this retrospective study. Planning computed tomography (CT) images of each patient were acquired for each device. Both the SCTDD and SMP plans were generated using a single-field optimized IMPT. The clinically relevant dose-volume parameters for the organs at risk (OARs), especially the tongue, were compared between the SCTDD and SMP plans. Additionally, to assess the feasibility of SCTDD as a proton beam stopper, the dose to the contralateral oral mucosa (COM) was compared with that from sMP use. The use of scTDD resulted in a statistically significant decrease in the radiation dose to the tongue and COM compared to sMP. The median mean dose to the tongue was significantly reduced with SCTDD (18.3 Gy(RBE)) compared to the SMP (22.9 Gy(RBE)) (p = 0.016). The percentages of tongue volume receiving doses between 15 and 60 Gy(RBE) were significantly lower with SCTDD. In the COM, SCTDD resulted in a significantly lower median mean (2.9 Gy(RBE) vs. 7.9 Gy(RBE), p = 0.018) and maximum dose (39.1 Gy(RBE) vs. 41.6 Gy(RBE), p = 0.018) doses compared to SMP. The SCTDD effectively decreased the radiation dose to the tongue compared to SMP in patients undergoing unilateral head and neck irradiation with IMPT and acted as a proton beam stopper to protect normal tissues located behind the target volume, such as the COM.
Introduction
Radiation therapy (RT) is an essential treatment modality for head and neck squamous cell carcinoma. Precision external-beam RT techniques, such as intensity-modulated radiation therapy (IMRT) [1, 2] and intensity-modulated proton therapy (IMPT) [3–5] can provide highly conformal dose distributions to the target volume and minimize the dose to the surrounding normal tissues for head and neck cancers (HNCs). However, dose reduction to the organs at risk (OARs) near the target remains a challenge because the planning target volumes (PTVs) are often very close to or overlap with several OARs, including the brainstem, spinal cord, optic apparatus, major salivary glands, pharyngeal musculature, oral tongue, and mucosa of the oral cavity (OC). Although intensity modulation based on inverse planning algorithms has contributed greatly to lowering the dose of the OC around the target, it is still difficult to be free from the threshold dose of radiation-induced toxicities in both IMRT and IMPT.
The tongue, a subsite of the OC, plays an important role in taste, saliva production, speech, and swallowing. Delivery of high-dose radiation to the OC causes radiation-induced toxicities, including oral mucositis, taste alterations, xerostomia, and swallowing dysfunction during or after RT [6–8]. Furthermore, these can lead to weight loss, which can cause significant dosimetric changes in target volumes and OARs in IMPT and poor quality of life after RT [9].
IMPT has a dosimetric advantage over IMRT, owing to the rapid dose fall-off behind the Bragg peak, it can deliver a highly conformal dose to the target while reducing the dose to adjacent OARs [3–5]. However, due to its unique physical properties of dose deposition, protons are more sensitive to uncertainties than photons [10]. In particular, in unilateral head and neck (HN) RT using IMPT, it is not easy to spare the OC including the tongue because the OC is very close to the target volume. Therefore, even in unilateral IMPT, it is necessary to separate the tongue from the target volume to further reduce the dose to the adjacent tongue.
Intraoral devices (IODs) are widely used to minimize setup uncertainty of the tongue and reduce the dose of the OC and tongue. In clinical practice, tongue depressors or bite blocks, mainly those that press the entire tongue, have been commonly used as IODs in bilateral HN RT [11–13]. However, these techniques are less effective when applying RT for unilateral HNCs, especially OC and tonsil cancers. Forced displacement of the tongue from the target using IODs is an effective and reliable approach to minimize the radiation dose to the tongue in unilateral HN RT using either IMRT or IMPT. For this reason, there is a great need for customized IOD in RT for HNC because of the anatomical complexity of the HN region. There have been a few reports regarding the effectiveness of custom-made mouthpieces as tongue depressors and elevators for carbon-ion RT [14–16]. However, no studies have investigated whether 3D-printed semi-customized IODs can be used to displace the tongue laterally from the target volume in unilateral HN IMPT. A unique, semi-customized intraoral tongue displacement device (SCTDD) treated with helical tomotherapy (HT) was previously developed and showed a significant decrease in the radiation dose to the tongue compared with a standard mouthpiece (SMP) in HT for OC and tonsil cancer [17]. Most HNCs are candidates for IMRT or IMPT alone or in combination [11]. Most centers use the same IODs for both proton and photon therapy. Therefore, it is very important to evaluate the usefulness and dosimetric effect of IODs in IMPT because proton beams behave completely differently from megavoltage photon beams [18, 19].
Proton beam ranges are also susceptible to tissue density heterogeneities, and it is difficult to stop protons within a specific range inside low-density materials, especially air cavities [20, 21]. An IOD can be used to effectively displace the tongue from the target. However, it can alter the anatomical structure or create air cavities in the OC. In particular, if the PTV contains or is adjacent to an air cavity in the OC, it may be difficult to optimize the dose to the target volume while protecting the tongue and contralateral oral mucosa (COM) behind the target volume because the proton beam range will be extended over the target. Consequently, proton beam overshoot caused by range uncertainty and setup error will lead to an unnecessary high dose to the tongue and COM beyond the target volume. Thus, evaluation of the dosimetric characteristics of IODs is essential for IMPT for HNC.
The aim of this study was to investigate the usefulness and dosimetric effects of SCTDD in unilateral HN IMPT. For the first time, we also assessed whether an SCTDD could act as a proton beam stopper in the OC.
Materials and Methods
Patients and Simulation
We designed and developed a unique patient-specific intraoral device, the scTDD, using a 3D printer (3DP) for use in patients with unilateral HNC (Figure 1). A detailed description of the SCTDD has been previously published [17]. Briefly, to reduce the radiation dose to the tongue, an SCTDD was designed to displace the tongue to the contralateral side of the target. In terms of functionality, the scTDD consists of an airway, tongue displacer, and tongue position verification window. When the patient bites the scTDD and sticks out his tongue until the tongue tip touches the tongue position verification window, the tongue is naturally displaced along the guide groove of the displacer to the contralateral side of the target (blue dotted arrows in Figure 1). The patient can breathe comfortably during RT through the airway, and the radiation therapist can simply check the reproducibility of the patient’s tongue-tip position through the verification window during the RT session. The two connectors were designed to attach and secure to the thermoplastic immobilization mask to improve the reproducibility of the patient setup. To ensure that every patient’s tongue would fit in the device and account for the variety of jaw-opening dimensions that naturally occur, we fabricated 16 different sizes of the SCTDD with varying combinations of thicknesses (5–20 mm, in 5 mm increments) and lengths (40–70 mm, in 10 mm increments). The scTDD was printed using a fused deposition modeling (FDM) 3DP (3DISON Multi, Rokit, Korea) with a biocompatible 3DP material (Kitchen and Deco, Rokit, Korea). Printing was performed at an extruder temperature of 260°C, a layer height of 0.3 mm, and infill of 100% at a printing speed of 60 mm/s. The manufacturing process using 3DP is quite similar to that reported in previous studies [17, 22].
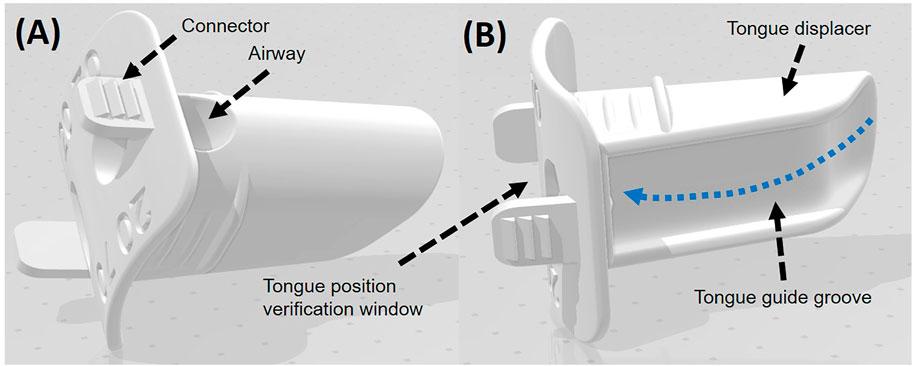
FIGURE 1. Top (A) and bottom (B) views of the three-dimensional model for the semi-customized tongue displacement device (scTDD). The scTDD consists of airway, tongue displacer and tongue position verification window.
Following approval from the institutional review board, seven consecutive patients with histologically proven HN squamous cell carcinoma, three with tonsil cancer and four with OC cancer, who underwent unilateral HN RT at a single institution between June and October of 2016 were included in this study (Table 1). Before the actual simulation, each patient was able to estimate the appropriate size of the SCTDD by measuring the distances between the upper and lower incisors (thickness) and the length of the OC with their mouths open comfortably. For adaptation and training, reproducibility and suitability were judged by confirming that each patient was able to maintain the posture for approximately 10 min by applying the selected size of scTDD. This retrospective study generated IMPT plans using patient data, including planning computed tomography (CT) and contours of the targets and OARs, which were used in a previous study [17]. Briefly, all patients were immobilized in the supine position using individual thermoplastic masks (Aquaplast RTTM, Q-fix, USA). Two sets of planning CT images were acquired: one with an SCTDD (CTSCTDD) and one with an SMP (CTSMP), for each patient, and these images were transferred to the treatment planning system (TPS, RayStation, RaySearch Laboratories AB, Sweden).
Proton Planning
The targets and OARs were delineated on CTSCTDD and CTSMP. In the three patients with tonsil cancer treated with definitive RT, the gross tumor volume (GTV) and clinical target volume (CTV) were delineated on both CT image sets, whereas the CTV was delineated only for the four patients with OC cancer treated with adjuvant RT. The PTVs (P_GTV and P_CTV) were created by a 3-mm isotropic expansion from the GTV and CTV, respectively, which were edited in consideration of actual anatomic boundaries, such as the spinal cord and skin surface. Furthermore, the following OARs were delineated on both CT image sets according to internal guidelines based on a reference: the parotid glands, spinal cord, brain stem, and tongue [23, 24]. Additionally, the COM surface was delineated to assess the feasibility of the SCTDD as a proton beam stopper. The planning volume for the spinal cord (p-cord) was generated by adding a 3-mm margin to the actual spinal cord.
IMPT plans were generated using one or two single-field optimization beams [4, 25] based on line scanning with a range shifter (4 cm water equivalent thickness) for both the SCTDD and SMP (Table 1). The Sumitomo Proton machine (Sumitomo Proton Therapy System, Sumitomo Heavy Industries, Ltd.) model was used to create the IMPT plans. Generally, anterior and posterior oblique beams are employed to optimize the target dose coverage while minimizing uncertainties along the beam path by avoiding the beam passing through the mouth (teeth) and shoulder penetrations. For this reason, a small couch kick angle (10°) was applied in two cases. In addition, because we did not use the beam direction traversed through SCTDD, the stopping power ratio (SPR) of the mouthpiece in the dose calculation was determined directly from the CT number using a CT-number-to-SPR conversion table.
All doses were defined in gray (Gy) as the relative biological effectiveness (RBE), assuming an RBE of 1.1 for protons. The same target coverage and OAR dose constraints were used for both plans based on internal guidelines as follows: a total dose of 66 Gy(RBE) and 60 Gy(RBE) were prescribed to 99% of the GTV and CTV, respectively, in 33 fractions using the simultaneous integrated boost technique for tonsil cancer. A total dose of 59.4 Gy(RBE) was prescribed to 99% of the CTV in 27 fractions for OC cancer. To achieve the most uniform dose distribution within the GTV and CTV, 99% of the GTV and CTV should receive at least 95% of the prescribed dose, and the volume receiving ≥110% of the prescribed dose should not be >1 cm3. The mean doses (Dmean) for the parotid glands and tongue were limited to ≤26 Gy(RBE) and ≤30 Gy(RBE), respectively, and the maximum dose (Dmax) to the p-cord and brainstem did not exceed 45 Gy(RBE) and 54 Gy(RBE), respectively. Robust optimization was performed using GTV and CTV as the target volumes. The robustness parameters were setup errors of ±3 mm in three dimensions and range uncertainties of ±3.5% of the nominal range, considering a total of 21 different scenarios. For both plans, the same number of iterations was used during the dose optimization process. During inverse planning, once the GTV and CTV constraints were reached, optimization was continued for dose reduction to the OARs until the iteration limit was reached while maintaining the GTV and CTV doses. A pencil beam algorithm was used for the final dose calculation, and the dose calculation for all IMPT plans was performed on a 2 × 2 × 2 mm3 dose grid resolution. For a fair dosimetric comparison, all plans were normalized such that 99% of the GTV and CTV received the prescribed dose.
Dosimetric Comparison
Dosimetric characteristics were obtained from both plans to compare the quality of the two plans. For the PTV, the minimum doses received by 98% (D98), 50% (D50), and 2% (D2) of the PTVs were compared. The dose homogeneity index (HI = [D2–D98]/D50) was calculated to assess the uniformity of the dose distribution within the PTV. An HI value of 1 is ideal and indicates a uniform dose distribution within the target. The following parameters were used for dosimetric comparison of the OARs: the mean dose (Dmean) of the parotid glands; maximum point dose (Dmax) of the p-cord and brain stem; percentage volume receiving 15 Gy(RBE) [V15Gy(RBE)], 30 Gy(RBE) [V30Gy(RBE)], 35 Gy(RBE) [V35Gy(RBE)], 45 Gy(RBE) [V45Gy(RBE)], and 60 Gy(RBE) [V60Gy(RBE)], and Dmax and Dmean for the tongue. In addition, the doses to the COM were analyzed as follows: the percentage volumes receiving 5 Gy(RBE) [V5Gy(RBE)], 10 Gy(RBE) [V10Gy(RBE)], 15 Gy(RBE) [V15Gy(RBE)], 30 Gy(RBE) [V30Gy(RBE)], and 39 Gy(RBE) [V39Gy(RBE)]; and Dmean and Dmax.
Quantitative statistical analysis of SCTDD and SMP data was performed using the Wilcoxon signed-rank test (SPSS, IBM Corporation, New York, United States). Statistical significance was set at p < 0.05.
Results
Proton beams provided good dose distribution, in which a high dose was focused around the target in both the SMP and SCTDD plans for all patients (Figure 2). In particular, the Bragg peak was effective at limiting the dose to the OARs near the target regions while maintaining the target dose coverage. For the target dose coverage, there was no significant difference in the D2, D98, and D50 of all target volumes between the SMP and SCTDD plans (p > 0.05). Furthermore, no significant differences were observed in the Dmean of the parotid glands [13.9 vs 13.7 Gy(RBE), p = 0.938] and Dmax of the p-cord (14.6 vs. 11.1 Gy(RBE), p = 0.469) and brain stem [3.0 vs. 3.1 Gy(RBE), p = 0.438] between the two groups (Supplementary Table S1).
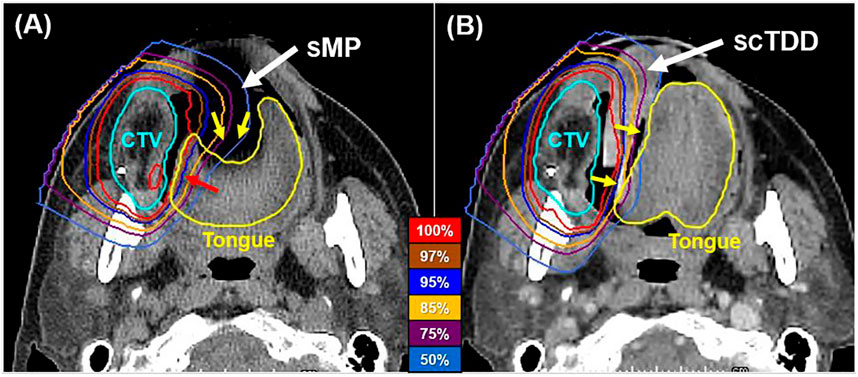
FIGURE 2. Axial dose distributions of the SMP (A) and SCTDD (B) in a representative patient. The SCTDD reduced the dose to the tongue by effectively separating the tongue from the target volume. The yellow arrows indicate the tongue, which was depressed (left) and laterally displaced (right) when using the SMP and SCTDD, respectively.
The SCTDD effectively displaced the tongue to the contralateral side of the PTV, while the SMP depressed the tongue down without displacement in all patients (Figure 1, yellow arrows). This led to differences in the dosimetric characteristics of the tongue, specifically, a reduction in the volume of the tongue that received high radiation doses (near the prescribed dose) (Figure 2, red arrow). The median Dmean of the tongue was significantly reduced with an SCTDD [18.3 Gy(RBE), interquartile range (IQR): 16.6–23.1] compared to that with the SMP [22.9 Gy(RBE), IQR: 21.2–25.2] (p = 0.016) (Table 2). In addition, the median V15Gy(RBE), V30Gy(RBE), V35Gy(RBE), V45Gy(RBE), and V60Gy(RBE) were significantly lower with an SCTDD (37.6, 27.4, 24.8, 19.8, and 7.3%, respectively) compared to those with the SMP (46.1, 36.2, 33.6, 28.2, and 10.3%, respectively) (all p < 0.05). However, no significant difference in the median Dmax of the tongue was observed between the SMP [62.0 Gy(RBE), IQR: 61.8–67.3] and SCTDD [62.2 Gy(RBE), IQR: 61.9–67.4].
An air cavity was formed in the OC as the SMP depressed the tongue (Figure 3). It extended the proton beam range so that a low radiation dose reached the COM. In contrast, no air cavity was created when SCTDD was used. Furthermore, it played an important role as a proton beam stopper, absorbing unwanted radiation in the penumbra region. These effects are well represented in the dosimetric profile of the COM (Figure 4; Table 3). The median Dmean of the COM was significantly reduced with SCTDD use [2.9 Gy(RBE), IQR: 2.1–5.0] compared to SMP [7.9 Gy(RBE), IQR: 3.6–8.9] (p = 0.018). SCTDD resulted in a significantly lower maximum dose to the COM than SMP [39.1 Gy(RBE) vs. 41.6 Gy(RBE), p = 0.018]. Moreover, significant dose reductions were achieved for the V5Gy(RBE), V10Gy(RBE), V15Gy(RBE), V30Gy(RBE), and V39Gy(RBE) with SCTDD (16.3, 10.4, 6.4, 0.5, and 0.0%, respectively) compared to SMP (48.6, 33.8, 14.7, 2.0, and 0.1%, respectively) (all p < 0.05). Patients with OC cancer, who had larger air pockets in the OC, showed higher median dose-volume histograms (DVHs) in the COM as well as higher dose reduction effects using an SCTDD compared to tonsil cancer patients (Figure 4).
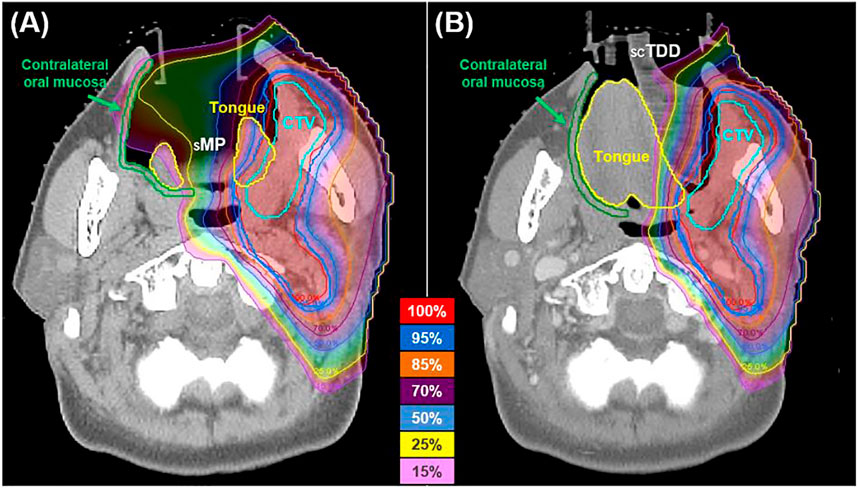
FIGURE 3. Axial dose distributions of the SMP (A) and SCTDD (B) in a patient with oral cavity cancer. The SCTDD acted as a proton beam stopper to protect the contralateral oral mucosa (green).
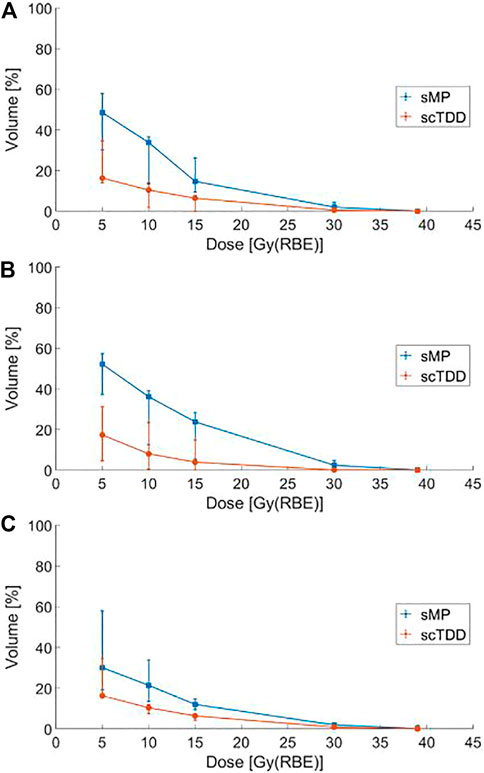
FIGURE 4. Median dose-volume histogram (DVH) for the contralateral oral mucosa with the SMP and SCTDD in all patients (A), patients with oral cavity cancer (B), and patients with tonsil cancer (C). The bars represent the first (25%) and third (75%) quartiles for each dose.
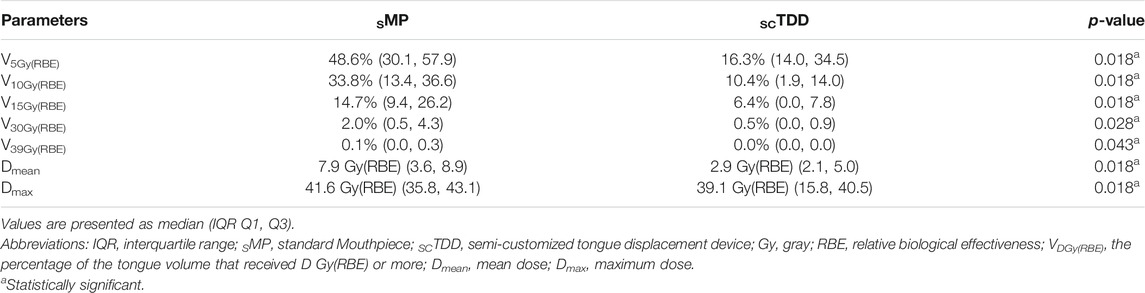
TABLE 3. Comparison of dosimetric characteristics for contralateral oral mucosa between the SMP and SCTDD.
Discussion
Various types of IODs are widely used to minimize setup errors and reduce the dose to the OC and its subsites [14–16, 26–28]. However, tongue depressor-type IODs are not effective for lateralized HNC RT. In addition, patient-specific customization of the IOD is important considering the presence of various types of OC conditions for HN RT patients. New types of patient-specific IODs have recently been reported [14–16, 28]. However, proper creation of a patient-specific IOD requires skill and sufficient manufacturing time, and can be difficult in busy clinical settings. To solve these issues, we developed a unique SCTDD through a semi-customization process using the same design but with various sizes. Manufacturing SCTDD has been made possible using 3D printing technology. The patient only needs to select an SCTDD that fits his/her oral conditions.
Despite the development of advanced RT techniques, radiation-induced oral mucositis is one of the most common obstacles in HNC RT [6, 29]. Furthermore, although the use of IODs can significantly decrease the tongue and mucosa dose in patients receiving IMRT for lateralized tumors [17, 30], it is not clear whether IODs are effective in lowering the OC dose, including the tongue, in unilateral HN IMPT. Manzar et al. demonstrated that IMPT resulted in significantly lower doses to the OC, and improved mucositis, dry mouth, and sticky saliva in patients treated unilaterally compared with IMRT; however, no IOD information was provided [31]. Grant et al. reported that the use of tongue-deviating stents in the unilateral treatment of tonsil cancer is likely to have no dosimetric benefit for patients receiving IMPT compared to IMRT [30]. However, through this study, we were able to confirm that as a unique IOD, an SCTDD was very effective in reducing the tongue dose in IMPT [Dmean: 22.9 Gy(RBE) to 18.3 Gy(RBE)] compared to the use of SMP. This difference seems to be due to differences in structure and principle of operation of the IOD. This is a valuable outcome in clinical practice because most HNCs are candidates for IMRT or IMPT, and some patients receive both treatment modalities for adaptive RT with the same IOD [11].
The development of radiation-induced tongue mucositis is associated with tongue volumes that receive low-and high-dose radiation. Musha et al. reported that patients receiving 16.0–73.0 Gy to the tongue were susceptible to grade 2–3 acute radiation tongue toxicity [32]. Therefore, a decrease in the tongue volume that receives low-dose radiation may be effective in preventing tongue mucositis. Our results showed that SCTDD use led to a statistically significant reduction in the dose to the tongue in terms of the mean dose, V15Gy(RBE), V30Gy(RBE), V35Gy(RBE), V45Gy(RBE), and V60Gy(RBE) compared to SMP use. These results indicate that it is possible to reduce the rate of grade 0—1 and 2—3 acute radiation tongue mucositis using an SCTDD.
Radiation-induced oral mucositis was related to a cumulative radiation dose to the OC between 15 Gy (V15Gy) and 39 Gy (V39Gy) [32, 33]. In patients using an SMP, the V15Gy(RBE) and V30Gy(RBE) of the COM were higher than those using the SCTDD (14.7 vs. 6.4%, and 2.0 vs. 0.5%, respectively). These differences seem to be due to the presence of air cavities formed by the sMP depressing the tongue in the OC (Figure 3). That is, more proton beams reached the COM because the proton beam range was increased in the air cavity compared to typical tissue with equivalent water density. In contrast, the use of an scTDD did not result in the formation of an air cavity, and it worked as a beam stopper in the OC. Although these values are small, they are included in the potential risk range for oral mucositis and may increase when the proton beam overshoots due to range uncertainty caused by tumor shrinkage, weight loss, or setup error [34]. Furthermore, if the PTV boundary is too close to the anatomical boundaries of the air volume in the OC and the air volume is large [21, 35], a large amount of unnecessary radiation will be delivered to the contralateral side of the target, such as the tongue and COM. In addition, there is a significant increase in RBE >1.1 at the end of the proton beam range [36, 37]. This can lead to a greater incidence of normal tissue complications in contralateral oral structures. From this point of view, SCTDD use may be effective at reducing the dose to contralateral oral structures because it acts as a proton beam stopper as well as a displacement device for the tongue by filling the air volume in the OC.
Prior to the clinical use of in-house IODs in particle therapy of HNC, it is necessary to conduct several investigations. Most importantly, the effects of the IOD material on the dose calculation of the particle beams must be considered. In CT-based planning, the composition of the IODs is not properly converted to SPR in the TPS, which can lead to range errors of particle beams in patients. Therefore, the validation of the SPR estimate for IODs materials should be clarified by comparing the converted value with the measurements [38]. When the incident beam passes through the IOD, it is recommended that the IODs be overwritten with the measured SPR value according to the treatment planning procedure. If it is not available, it would be useful to use a treatment planning strategy in which the incident beam does not pass through the IODs. Because we did not use the beam direction through the IODs in this study, we did not consider overwriting and correcting the IOD material with proper Hounsfield units or proton SPR. Therefore, although the SPR error of IODs did not affect the dose calculation of the target, there may be a difference in the dosimetric results of COM.
Second, because particle therapy is sensitive to patient setup uncertainty, validation of the positional stability and reproducibility of IODs during treatment is necessary. To ensure robust positioning reproducibility, the scTDD design introduces a connector to the thermoplastic immobilization mask and a tongue-tip position guide bar with a verification window. Further studies are needed to evaluate setup reproducibility by analyzing daily setup images. In addition, patients should be able to tolerate IODs easily. According to the results of a previous study [17], sMP could not be applied because it was difficult to open the jaw sufficiently for patients with postoperative RT, whereas scTDD with a thickness of 1 cm and a length of 6.5 cm could be effectively applied to the patient. As the course of RT progresses, oral mucositis lesions are extremely painful and may lead to oral discomfort. For this reason, scTDD requires a rounded corner design with a smooth surface.
In lateralized cancers such as buccal or retromolar trigone cancer, the CTV generally does not include the tongue, considering anatomic boundaries and tumor invasion patterns. However, with the use of usual tongue depressor-type IODs, it is often difficult to distinguish the anatomical boundary between the lateral tongue and buccal mucosa or gingiva. The use of scTDD helps to identify the anatomical space between the tongue and the gingiva/buccal mucosa. Clinicians should delineate CTV considering these changes.
This study has several limitations. First, this was a single-institution retrospective study including a small number of patients and is subject to the inherent biases of the study design. Second, we hypothesized that the tongue depressor was the standard tongue immobilization device for unilateral HNCs [39], and this study focused on comparing SCTDD with the tongue depressor. Although SCTDD is readily applicable to patients and has advantages as a proton beam stopper, comparisons with currently commercialized tongue-deviating IODs were not included in this study. Finally, further study is needed to assess whether the dosimetric benefits obtained using SCTDD can also reduce RT-associated acute and late toxicities.
Conclusion
We developed a unique SCTDD using 3DP technology, which was designed to separate the tongue from the target volume. It can be used during unilateral IMPT to reduce the radiation dose to the tongue and the COM. The SCTDD not only dramatically reduced the dose to the tongue compared to SMP, but also played a very important role as a proton beam stopper for the COM by preventing proton overshoot within the OC. Furthermore, it is expected to play an important role in minimizing radiation-induced toxicity of the tongue and oral mucosa in unilateral IMPT.
Data Availability Statement
The original contributions presented in the study are included in the article/supplementary material, further inquiries can be directed to the corresponding author.
Ethics Statement
The studies involving human participants were reviewed and approved by The institutional review board at Samsung Medical Center (file no. SMC 2020-02-134-001, April 20, 2020). Written informed consent for participation was not required for this study in accordance with the national legislation and the institutional requirements.
Author Contributions
Conception, design, and drafting the manuscript were performed by SJ, C-SH, and DO. Data collection and interpreting were performed by YA, Y-bK, SP, and WL. All authors have read and agreed to the published version of the manuscript.
Funding
This work was supported by the research program, NRF-2018R1D1A1B07043445 (South Korea).
Conflict of Interest
The authors declare that the research was conducted in the absence of any commercial or financial relationships that could be construed as a potential conflict of interest.
Publisher’s Note
All claims expressed in this article are solely those of the authors and do not necessarily represent those of their affiliated organizations, or those of the publisher, the editors and the reviewers. Any product that may be evaluated in this article, or claim that may be made by its manufacturer, is not guaranteed or endorsed by the publisher.
Acknowledgments
This idea is protected by a Korean patent (No. 10–1828053).
Supplementary Material
The Supplementary Material for this article can be found online at: https://www.frontiersin.org/articles/10.3389/fphy.2021.680820/full#supplementary-material
References
1. Dragan T, Beauvois S, Moreau M, Paesmans M, Vandekerkhove C, Cordier L, et al. Clinical Outcome and Toxicity after Simultaneous Integrated Boost IMRT in Head and Neck Squamous Cell Cancer Patients. Oral Oncol (2019) 98:132–40. doi:10.1016/j.oraloncology.2019.09.012
2. Gupta T, Agarwal J, Jain S, Phurailatpam R, Kannan S, Ghosh-Laskar S, et al. Three-dimensional Conformal Radiotherapy (3D-CRT) versus Intensity Modulated Radiation Therapy (IMRT) in Squamous Cell Carcinoma of the Head and Neck: a Randomized Controlled Trial. Radiother Oncol (2012) 104(3):343–8. doi:10.1016/j.radonc.2012.07.001
3. Romesser PB, Cahlon O, Scher E, Zhou Y, Berry SL, Rybkin A, et al. Proton Beam Radiation Therapy Results in Significantly Reduced Toxicity Compared with Intensity-Modulated Radiation Therapy for Head and Neck Tumors that Require Ipsilateral Radiation. Radiother Oncol (2016) 118(2):286–92. doi:10.1016/j.radonc.2015.12.008
4. Blanchard P, Garden AS, Gunn GB, Rosenthal DI, Morrison WH, Hernandez M, et al. Intensity-modulated Proton Beam Therapy (IMPT) versus Intensity-Modulated Photon Therapy (IMRT) for Patients with Oropharynx Cancer - A Case Matched Analysis. Radiother Oncol (2016) 120(1):48–55. doi:10.1016/j.radonc.2016.05.022
5. McDonald MW, Liu Y, Moore MG, Johnstone PAS. Acute Toxicity in Comprehensive Head and Neck Radiation for Nasopharynx and Paranasal Sinus Cancers: Cohort Comparison of 3D Conformal Proton Therapy and Intensity Modulated Radiation Therapy. Radiat Oncol (2016) 11:32. doi:10.1186/s13014-016-0600-3
6. Maria OM, Eliopoulos N, Muanza T. Radiation-Induced Oral Mucositis. Front Oncol (2017) 7:89. doi:10.3389/fonc.2017.00089
7. Sapir E, Tao Y, Feng F, Samuels S, El Naqa I, Murdoch-Kinch CA, et al. Predictors of Dysgeusia in Patients with Oropharyngeal Cancer Treated with Chemotherapy and Intensity Modulated Radiation Therapy. Int J Radiat Oncology*Biology*Physics (2016) 96(2):354–61. doi:10.1016/j.ijrobp.2016.05.011
8. Wentzel A, Hanula P, van Dijk LV, Elgohari B, Mohamed ASR, Cardenas CE, et al. Precision Toxicity Correlates of Tumor Spatial Proximity to Organs at Risk in Cancer Patients Receiving Intensity-Modulated Radiotherapy. Radiother Oncol (2020) 148:245–51. doi:10.1016/j.radonc.2020.05.023
9. Kurz C, Nijhuis R, Reiner M, Ganswindt U, Thieke C, Belka C, et al. Feasibility of Automated Proton Therapy Plan Adaptation for Head and Neck Tumors Using Cone Beam CT Images. Radiat Oncol (2016) 11:64. doi:10.1186/s13014-016-0641-7
10. Paganetti H. Range Uncertainties in Proton Therapy and the Role of Monte Carlo Simulations. Phys Med Biol (2012) 57(11):R99–R117. doi:10.1088/0031-9155/57/11/R99
11. Park SG, Ahn YC, Oh D, Noh JM, Ju SG, Kwon D, et al. Early Clinical Outcomes of Helical Tomotherapy/intensity‐modulated Proton Therapy Combination in Nasopharynx Cancer. Cancer Sci (2019) 110(9):2867–74. doi:10.1111/cas.14115
12. Mesko S, Wang H, Tung S, Wang C, Pasalic D, Chapman BV, et al. Estimating PTV Margins in Head and Neck Stereotactic Ablative Radiation Therapy (SABR) through Target Site Analysis of Positioning and Intrafractional Accuracy. Int J Radiat Oncology*Biology*Physics (2020) 106(1):185–93. doi:10.1016/j.ijrobp.2019.09.010
13. Zhao B, Maquilan G, Jiang S, Schwartz DL. Minimal Mask Immobilization with Optical Surface Guidance for Head and Neck Radiotherapy. J Appl Clin Med Phys (2018) 19(1):17–24. doi:10.1002/acm2.12211
14. Ikawa H, Koto M, Ebner DK, Takagi R, Hayashi K, Tsuji H, et al. A Custom-Made Mouthpiece Incorporating Tongue Depressors and Elevators to Reduce Radiation-Induced Tongue Mucositis during Carbon-Ion Radiation Therapy for Head and Neck Cancer. Pract Radiat Oncol (2018) 8(2):e27–e31. doi:10.1016/j.prro.2017.10.009
15. Musha A, Saitoh J-i., Shirai K, Kubota Y, Shimada H, Abe T, et al. Customized Mouthpieces Designed to Reduce Tongue Mucositis in Carbon-Ion Radiotherapy for Tumors of the Nasal and Paranasal Sinuses. Phys Imaging Radiat Oncol (2017) 3:1–4. doi:10.1016/j.phro.2017.07.003
16. Ikawa H, Koto M, Ebner DK, Hayashi K, Takagi R, Tonogi M, et al. The Efficacy of a Custom-Made Mouthpiece with Spacer to Reduce Osteoradionecrosis in Carbon-Ion Radiation Therapy for Tongue-Base Tumor. Adv Radiat Oncol (2019) 4(1):15–9. doi:10.1016/j.adro.2018.08.016
17. Hong C-S, Oh D, Ju SG, Ahn YC, Na CH, Kwon DY, et al. Development of a Semi-customized Tongue Displacement Device Using a 3D Printer for Head and Neck IMRT. Radiat Oncol (2019) 14(1):79. doi:10.1186/s13014-019-1289-x
18. Blanchard P, Gunn GB, Lin A, Foote RL, Lee NY, Frank SJ. Proton Therapy for Head and Neck Cancers. Semin Radiat Oncol (2018) 28(1):53–63. doi:10.1016/j.semradonc.2017.08.004
19. Mohan R, Das IJ, Ling CC. Empowering Intensity Modulated Proton Therapy through Physics and Technology: An Overview. Int J Radiat Oncology*Biology*Physics (2017) 99(2):304–16. doi:10.1016/j.ijrobp.2017.05.005
20. Malyapa R, Lowe M, Bolsi A, Lomax AJ, Weber DC, Albertini F. Evaluation of Robustness to Setup and Range Uncertainties for Head and Neck Patients Treated with Pencil Beam Scanning Proton Therapy. Int J Radiat Oncology*Biology*Physics (2016) 95(1):154–62. doi:10.1016/j.ijrobp.2016.02.016
21. Fukumitsu N, Ishikawa H, Ohnishi K, Terunuma T, Mizumoto M, Numajiri H, et al. Dose Distribution Resulting from Changes in Aeration of Nasal Cavity or Paranasal Sinus Cancer in the Proton Therapy. Radiother Oncol (2014) 113(1):72–6. doi:10.1016/j.radonc.2014.08.024
22. Ju SG, Ahn YC, Kim Y-b., Park SG, Choi Y-m., Na CH, et al. Development of a Tongue Immobilization Device Using a 3D Printer for Intensity Modulated Radiation Therapy of Nasopharyngeal Cancer Patients. Cancer Res Treat (2021) 53(1):45–54. doi:10.4143/crt.2020.572
23. Dean JA, Welsh LC, Gulliford SL, Harrington KJ, Nutting CM. A Novel Method for Delineation of Oral Mucosa for Radiotherapy Dose-Response Studies. Radiother Oncol (2015) 115(1):63–6. doi:10.1016/j.radonc.2015.02.020
24. Brouwer CL, Steenbakkers RJHM, Bourhis J, Budach W, Grau C, Grégoire V, et al. CT-based Delineation of Organs at Risk in the Head and Neck Region: DAHANCA, EORTC, GORTEC, HKNPCSG, NCIC CTG, NCRI, NRG Oncology and TROG Consensus Guidelines. Radiother Oncol (2015) 117(1):83–90. doi:10.1016/j.radonc.2015.07.041
25. Gunn GB, Garden AS, Ye R, Ausat N, Dahlstrom KR, Morrison WH, et al. Proton Therapy for Head and Neck Cancer: A 12-Year, Single-Institution Experience. Int J Part Ther (2021) 8(1):108–18. doi:10.14338/IJPT-20-00065.1
26. Zaid M, Bajaj N, Burrows H, Mathew R, Dai A, Wilke CT, et al. Creating Customized Oral Stents for Head and Neck Radiotherapy Using 3D Scanning and Printing. Radiat Oncol (2019) 14(1):148. doi:10.1186/s13014-019-1357-2
27. Verrone JR, Alves Fd. A, Prado JD, Boccaletti KW, Sereno MP, Silva MLG, et al. Impact of Intraoral Stent on the Side Effects of Radiotherapy for Oral Cancer. Head Neck (2013) 35(7):E213–E217. doi:10.1002/hed.23028
28. Huang Y-M, Lee J-C, Chu C-M, Tai H-C, Hou T-C, Chen F, et al. Three-Dimensional Printed Silicone Bite Blocks for Radiotherapy of Head and Neck Cancer-A Preliminary Study. Appl Sci (2020) 10(5):1688. doi:10.3390/app10051688
29. Elting LS, Cooksley CD, Chambers MS, Garden AS. Risk, Outcomes, and Costs of Radiation-Induced Oral Mucositis Among Patients with Head-And-Neck Malignancies. Int J Radiat Oncology*Biology*Physics (2007) 68(4):1110–20. doi:10.1016/j.ijrobp.2007.01.053
30. Grant S, Williamson T, Fuller CD, Rosenthal D, Frank SJ, Garden AS, et al. A Dosimetric Comparison of Oral Cavity Sparing in the Unilateral Treatment of Early Stage Tonsil Cancer: IMRT, IMPT, and Tongue Deviating Oral Stents. Int J Radiat Oncology*Biology*Physics (2019) 5(6):103. doi:10.1016/s0360-3016(19)30489-4
31. Manzar GS, Lester SC, Routman DM, Harmsen WS, Petersen MM, Sloan JA, et al. Comparative Analysis of Acute Toxicities and Patient Reported Outcomes between Intensity-Modulated Proton Therapy (IMPT) and Volumetric Modulated Arc Therapy (VMAT) for the Treatment of Oropharyngeal Cancer. Radiother Oncol (2020) 147:64–74. doi:10.1016/j.radonc.2020.03.010
32. Musha A, Fukata K, Saitoh J-I, Shirai K, Abe T, Mizukami T, et al. Tongue Surface Model Can Predict Radiation Tongue Mucositis Due to Intensity-Modulated Radiation Therapy for Head and Neck Cancer. Int J Oral Maxillofac Surg (2020) 49(1):44–50. doi:10.1016/j.ijom.2019.06.012
33. Narayan S, Lehmann J, Coleman MA, Vaughan A, Yang CC, Enepekides D, et al. Prospective Evaluation to Establish a Dose Response for Clinical Oral Mucositis in Patients Undergoing Head-And-Neck Conformal Radiotherapy. Int J Radiat Oncology*Biology*Physics (2008) 72(3):756–62. doi:10.1016/j.ijrobp.2008.01.060
34. Musha A, Shimada H, Shirai K, Saitoh J-i., Yokoo S, Chikamatsu K, et al. Prediction of Acute Radiation Mucositis Using an Oral Mucosal Dose Surface Model in Carbon Ion Radiotherapy for Head and Neck Tumors. PLoS One (2015) 10(10):e0141734. doi:10.1371/journal.pone.0141734
35. Shusharina N, Fullerton B, Adams JA, Sharp GC, Chan AW. Impact of Aeration Change and Beam Arrangement on the Robustness of Proton Plans. J Appl Clin Med Phys (2019) 20(3):14–21. doi:10.1002/acm2.12503
36. Rabus H, Ngcezu SA, Braunroth T, Nettelbeck H. "Broadscale" Nanodosimetry: Nanodosimetric Track Structure Quantities Increase at Distal Edge of Spread-Out Proton Bragg Peaks. Radiat Phys Chem (2020) 166:108515. doi:10.1016/j.radphyschem.2019.108515
37. Paganetti H, Giantsoudi D. Relative Biological Effectiveness Uncertainties and Implications for Beam Arrangements and Dose Constraints in Proton Therapy. Semin Radiat Oncol (2018) 28(3):256–63. doi:10.1016/j.semradonc.2018.02.010
38. Kawamura M, Maeda Y, Takamatsu S, Tameshige Y, Sasaki M, Asahi S, et al. The Usefulness of Vinyl Polysiloxane Dental Impression Material as a Proton Beam Stopper to Save normal Tissue during Irradiation of the Oral Cavity: Basic and Clinical Verifications. Med Phys (2013) 40(8):081707. doi:10.1118/1.4813300
39. Yoon HG, Ahn YC, Oh D, Noh JM, Park SG, Nam H, et al. Early Clinical Outcomes of Intensity Modulated Radiation Therapy/Intensity Modulated Proton Therapy Combination in Comparison with Intensity Modulated Radiation Therapy Alone in Oropharynx Cancer Patients. Cancers (2021) 13(7):1549. doi:10.3390/cancers13071549
Keywords: tongue displacement device, proton beam stopper, intensity-modulated proton therapy, head and neck cancer, oral mucosa, 3D printing
Citation: Hong C-S, Oh D, Ju S, Ahn Y, Kim Y-b, Park S and Lee W (2021) Tongue Displacement Device in Decreasing the Radiation Dose to Tongue and Preventing Proton Beam Overshoot in Proton Therapy for Unilateral Head and Neck Cancer. Front. Phys. 9:680820. doi: 10.3389/fphy.2021.680820
Received: 15 March 2021; Accepted: 21 September 2021;
Published: 13 October 2021.
Edited by:
Eliana Maria Vasquez Osorio, The University of Manchester, United KingdomReviewed by:
Andrew Green, The University of Manchester, United KingdomDaniel Scandurra, University Medical Center Groningen, Netherlands
Copyright © 2021 Hong, Oh, Ju, Ahn, Kim, Park and Lee. This is an open-access article distributed under the terms of the Creative Commons Attribution License (CC BY). The use, distribution or reproduction in other forums is permitted, provided the original author(s) and the copyright owner(s) are credited and that the original publication in this journal is cited, in accordance with accepted academic practice. No use, distribution or reproduction is permitted which does not comply with these terms.
*Correspondence: Sang Gyu Ju, sg.ju@samsung.com
†These authors have contributed equally to this work and share first authorship