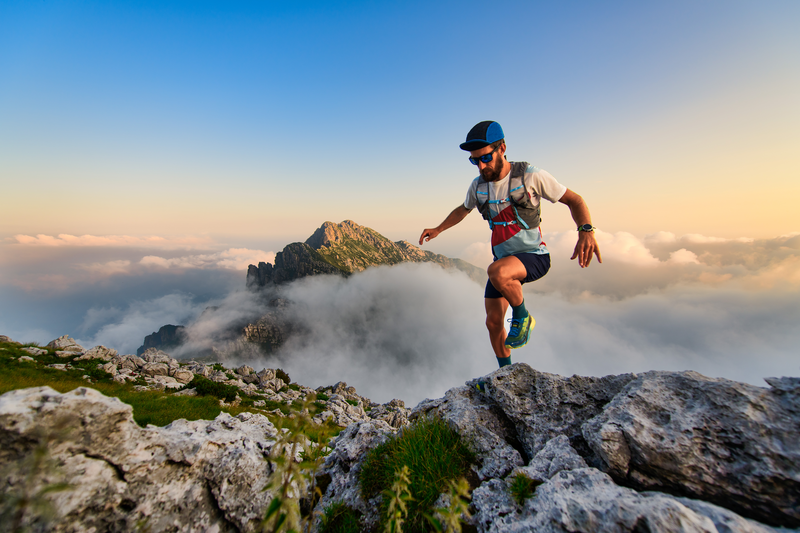
95% of researchers rate our articles as excellent or good
Learn more about the work of our research integrity team to safeguard the quality of each article we publish.
Find out more
ORIGINAL RESEARCH article
Front. Phys. , 12 May 2021
Sec. Stellar and Solar Physics
Volume 9 - 2021 | https://doi.org/10.3389/fphy.2021.679780
This article is part of the Research Topic The Magnetic Structures and Their Role in The Evolution of Coronal Mass Ejections View all 14 articles
During solar eruptions, many closed magnetic flux ropes are ejected into interplanetary space, which contribute to the heliospheric magnetic field and have important space weather effect because of their coherent magnetic field. Therefore, understanding the evolution of these closed flux ropes in the interplanetary space is important. In this paper, we examined all the magnetic and plasma data measured in 1997 by the Wind spacecraft and identified 621 reconnection exhausts. Of the 621 reconnection events, 31 were observed at the boundaries of magnetic flux ropes and were thought to cause the opening or disconnection magnetic field lines of the adjacent ropes. Of the 31 magnetic reconnection events, 29 were interchange reconnections and the closed field lines of these related flux ropes were opened by them. Only 2 of the 31 magnetic reconnection events disconnected the opened field lines of the original flux ropes. These observations indicate that interchange reconnection and disconnection may be two important mechanisms changing the magnetic topology of the magnetic flux ropes during their propagation during the interplanetary space.
• Of the 621 reconnection events, 31 magnetic reconnection events are interchange reconnections, and the closed field lines of these related flux ropes were opening by them.
• Only 2 of the 31 magnetic reconnection events were disconnecting the opened field lines of flux ropes.
• These observations indicate that the interplanetary magnetic reconnections near the boundaries of flux ropes make important contributions to their evolution in the interplanetary space.
During solar eruptions, many magnetic flux ropes are ejected from the corona in the form of coronal mass ejections (CMEs). The magnetic field lines of flux ropes are attached to the Sun at both ends and are referred to as closed flux ropes [1]. The durations of these closed ropes vary from tens of minutes (e.g., the small scale interplanetary flux ropes) to a few days (e.g., magnetic clouds) [2, 3]. The ejected flux ropes have significant effect on the space weather because of their coherent magnetic structures [4–6]. Besides, the magnitude of the magnetic field in the heliosphere will increase as the closed flux ropes ceaselessly ejected into the interplanetary space, which is not consistent with observations. Therefore, understanding the evolution of the closed flux ropes as they propagate in the interplanetary space is important.
As Crooker et al. [7] pointed out, without change in magnetic topology, the magnetic field magnitude in the heliosphere would increase indefinitely because of the ceaseless ejection of closed flux ropes, leading to a “magnetic field magnitude catastrophe” [8, 9]. Magnetic reconnection is an important mechanism changing the topology of the magnetic field. Crooker and Owens [10] summarized two possibilities to avoid the “magnetic field magnitude catastrophe”: (1) that closed magnetic field lines of a rope are gradually opened through interchange reconnection between one of its legs and the surrounding open magnetic field lines near the Sun [7, 11]; (2) that open magnetic field lines of ropes are reconnected with opposite polarity open magnetic field lines, thus disconnecting them from the Sun [7, 12]. Previous studies provide some supports for these possibilities [e.g., [13–15]].
A large number of interplanetary magnetic reconnections associated with magnetic flux ropes have been observed [16, 17]. Although the interplanetary magnetic reconnection is not assumed to play any role in the heliospheric magnetic magnitude budget [18], it is thought important in changing the magnetic topology of the interplanetary flux ropes. For example, Ruffenach et al. [19] thought that magnetic reconnection near the boundary of magnetic clouds can erode them causing an imbalance in the azimuthal flux. Wang et al. [17] reported a small flux rope associated with a magnetic reconnection exhaust, which had closed field lines that were opened by an interplanetary magnetic reconnection. The reconnection exhaust and the following small magnetic flux rope were observed on February 2nd, 2002 by Wind and ACE. Their observations provided direct evidence that closed field lines can be opened by interplanetary interchange magnetic reconnection. In 2018, Feng et al. [20] reported a flux rope associated with a reconnection exhaust. In that study, some field lines before the rear boundary of the flux rope were opened, and the opened magnetic field lines were merging with the open field lines after the rope to produce disconnected field lines. The observations of Feng et al. [20] clearly indicate that the open and disconnection of flux ropes can happen in no particular order, supporting the suggestion by Wang et al. [17]. Since the reported interplanetary reconnection exhausts associated with opened (or disconnected) field lines have been very rare, it is uncertain whether interplanetary reconnection is important in the openness and/or disconnection of the magnetic field of the interplanetary flux ropes.
In this study, we aimed to search for interplanetary flux ropes that were opened or disconnected from the Sun by interplanetary magnetic reconnections. We examined all the magnetic and plasma data measured in 1997 by the Wind spacecraft and identified hundreds of interplanetary reconnection exhausts. Further analysis showed that 30 flux ropes were opened or disconnected by 31 reconnection exhausts. Our observations confirmed that interplanetary magnetic reconnections do play important role in the openness and/or disconnection of the magnetic field of the interplanetary flux ropes.
The data used in this study were obtained from two instruments on board the Wind spacecraft. The magnetic field and plasma data with time resolutions of 3 s are, respectively, from the Fluxgate Magnetometer experiment [21] and the Three-Dimension Plasma (3DP) instrument [22]. The suprathermal electron data were also obtained from the 3DP analyzer. The electrons were measured by the 3DP at 8 pitch angles as spectrograms in terms of energy. The suprathermal electrons used in our analysis were about 220 eV, and their angular and time resolutions were 22.5° and 48 s, respectively. If not specified, the GSE coordinate system (the Geocentric Solar Ecliptic coordinate system in which the x-axis directs from the Earth to the Sun, the z-axis points north, perpendicular to the ecliptic plane, the y-axis completes the right-handed coordinate system) is used in this study.
Suprathermal electron strahls in the solar wind come from the Sun, and they are focused along magnetic field lines [23–28]. Therefore, the suprathermal electron signatures of flux ropes are used to judge the state of their magnetic fields. The presence of counter-streaming suprathermal electron strahls indicates that the magnetic field lines are still attached to the Sun at both ends, unidirectional strahls indicate that the field lines are open, and the disappearance of strahls indicates that the field lines are completely disconnected from the Sun. Magnetic flux ropes are field topologies characterized by bundles of helical magnetic field lines collectively spiraling around a common axis, and their essential observational properties are enhanced magnetic field strength and smooth rotations [29, 30]. Hence, flux ropes were identified to have enhanced magnetic field strength and smooth rotating magnetic field components. This study used the criterion of Gosling et al. [18] to identify interplanetary reconnection exhausts. This criterion requires them to satisfy the following conditions: (1) a roughly Alfvénic jet, (2) an enhancement of proton density and temperature (not all interplanetary reconnection exhausts), and (3) a depression of the magnetic-field strength.
Using the magnetic reconnection exhaust criteria of Gosling et al. [18], the high-resolution (3 s) plasma and magnetic field data from Wind were examined, and 621 magnetic reconnection exhausts were identified. We then investigated magnetic field characteristics and suprathermal electron signatures near these magnetic reconnection exhausts and found 31 reconnection exhausts may open the closed flux ropes or disconnect field lines of flux ropes from the Sun. Table 1 lists the 31 magnetic reconnection exhausts and their related flux ropes. The second and third columns show the front and rear boundaries of the reconnection exhausts, and the fourth and fifth columns list the start and end times of the flux ropes. The sixth column shows the flux ropes that were opened or disconnected from the Sun by the related magnetic reconnections (“O” indicates opened and “D” indicates disconnected. The use of “opened” and “disconnection” meant that these ropes were assumed originally closed). In this section, we will discuss two flux ropes, and their related reconnection exhausts, as examples to exhibit the process of open or disconnection. The first is the flux rope observed on November 16, 1997, whose closed field lines were thought to reconnect to the adjacent open solar wind magnetic field lines at the front and rear boundaries. The other is a flux rope that was observed on November 9, 1997, but whose open field lines were disconnected by reconnecting to the disconnected solar wind magnetic field lines before the flux rope.
Figure 1 shows the suprathermal electron pitch angle distributions, the magnetic field, and the plasma data measured by the Wind spacecraft during the November 16, 1997 flux rope passage. The top panel shows the distributions for the suprathermal electron pitch angle of 228.17 eV. The subsequent panels display the magnitude of the total magnetic field (|B|); the x, y, z components of the magnetic field (Bx, By, Bz); and the x, y, z components of the proton speed (Vx, Vy, Vz), proton density (NP), and the proton temperature (Tp). In Figure 1, there is a small duration (labeled by two vertical dashed lines) that possessed the flux-rope signature of the enhanced magnetic field strength and smoothly rotating magnetic fields. More specifically, the bipolar field appeared in the By component, and the core field appeared in the Bz components. The suprathermal electron pitch angle distribution shows that (1) counter-streaming suprathermal electron strahls were present almost throughout the duration of the small flux rope, but (2) all parallel (0°) suprathermal electron strahls were absent before and after the rope. As mentioned above, the presence of suprathermal electron strahls indicates magnetic connection to the Sun, whereas the absence of suprathermal electron strahls indicates magnetic disconnection from the Sun [31]. Therefore, these observations indicated that almost all magnetic field lines of the small rope were closed and still were connected to the Sun on both ends. However, the surrounding magnetic field lines of the rope were connected to the Sun only on one end. In Figure 1, we see that near the boundaries of the rope, the magnetic strength exhibited depression, some magnetic field component directions were reversed, the proton density and temperature were enhanced, and the accelerated plasma flow was observed. All these features satisfied the criteria of reconnection exhausts [18], and we further confirmed that these are two reconnection exhausts. We will discuss the observations of the two reconnection exhausts in detail below.
Figure 1. Suprathermal electron pitch angle distributions of 228.17 eV, magnetic field, and plasma data measured by Wind during the November 16th, 1997 flux rope passage. The two vertical dashed lines denote the boundaries of the flux rope.
Figure 2 shows the expanded views of the reconnection exhaust near the front boundary of the flux rope. We can see in this figure that outstanding accelerated plasma flow was observed in the y direction, and the y direction magnetic field component was reversed across the accelerated flow, in which the proton density and temperature were also enhanced. All these features are characteristic of interplanetary reconnection exhausts. The Walén relation is often used to identify whether or not the accelerated plasma flows are Alfvénic waves bounding the exhausts [e.g., [32–35]]. We performed a minimum variance analysis (MVA) of the magnetic field data near the reconnection exhaust, and established the LMN coordinate system, where L, M and N represented the directions of maximum, intermediate, and minimum variance of the field component. L was assumed to be along the reconnection outflow direction and N along the normal direction of the reconnection current sheet. Figure 3 shows the magnetic field and velocity curves measured in LMN coordinate system. As shown in the figure, the enhancement of the plasma velocity was mainly in the L direction. Figure 3 also shows the predicted VL velocity from the Walén relation as a blue dashed curve, and the expected VL velocity curve was consistent with the observed velocity curve. Therefore, the observed accelerated flow was an exhaust of a magnetic reconnection. Figure 2 also shows that the antiparallel suprathermal electron strahls occurred throughout the region and that the parallel suprathermal electron strahls were absent before the front boundary of the exhaust, though the weak strahls appeared after the rear boundary of the exhaust. These observations indicated that the field lines prior to the exhaust were opened, but those after the exhaust were still closed. Namely, an interchange reconnection occurred between open magnetic fields before the flux rope and closed magnetic field lines of the flux ropes, and the closed flux ropes were gradually opened by the interchange reconnection at its front boundary. Although here we adopted the term “interchange reconnection” from Crooker and Owens [10], one should keep remember that the reconnection exhausts discussed in this paper were detected in the interplanetary space. The term “disconnection” should also be interpreted in this way.
Figure 2. Magnified images of the suprathermal electron distributions, magnetic field, and plasma data of the reconnection exhaust around 14:45 UT November 16th measured by Wind. The two vertical dashed lines denote the reconnection exhaust interval.
Figure 3. Magnetic field and velocity curves measured in LMN coordinate system of the reconnection exhaust around 14:45 UT November 16th, and the blue dashed curve presents the VL velocity derived from the Walén relation. The two vertical dashed lines denote the reconnection exhaust interval.
Figure 4 shows the expanded views of the reconnection exhaust near the rear boundary of the flux rope. In this figure, accelerated plasma flow was observed in all the three directions from 16:42:30 UT to 16:46:37 UT, during which time all the magnetic field components were reversed and both proton density and temperature were enhanced. Figure 5 provides the magnetic field and velocity curves of the magnetic reconnection exhaust in the LMN coordinate system, and the exhibited expected VL velocity curve was approximately consistent with the observed velocity curve. The suprathermal electron distributions in Figure 5 show that the field lines prior to the exhaust were closed, while those after the exhaust were open; the magnetic reconnection event was also an interchange reconnection. In the same way, this closed flux rope was gradually opened by the reconnection event at its rear boundary.
Figure 4. The reconnection exhaust around 16:45 UT November 16th measured by Wind. The format of the figure is same as Figure 2.
Figure 5. The reconnection exhaust around 16:45 UT November 16th measured by Wind. The format of the figure is same as Figure 3.
Figure 6 shows the suprathermal electron pitch angle distributions, magnetic field, and plasma data of the November 9, 1997 flux rope passage, as well as the preceding magnetic reconnection exhaust. The top panel indicated that (1) the parallel suprathermal electron strahls occurred almost throughout the duration of the rope except for a few brief intervals, but (2) the antiparallel suprathermal electrons strahls were absent except for a brief interval. These observations indicated that most magnetic field lines of the small flux rope were open, and some magnetic field lines were disconnected from the Sun. Before the small rope, all suprathermal electron strahls disappeared, which meant that all the magnetic field lines before the rope were open. Figure 7 provides expanded views of the magnetic reconnection event, which shows that the accelerated flow from 11:17:13 UT to 11:18:58 UT had all the typical observable characteristics of an interplanetary reconnection exhaust. We also tested if the accelerated flow was a reconnection exhaust using the Walén relation (Figure 8). These observations revealed that the open field lines of the flux rope were reconnected with disconnected field lines to produce new U-shaped field lines with no connection to the Sun. This type of disconnection for flux ropes has never been reported before. The previously reported disconnections associated with reconnection exhausts are very rare and only show disconnection that describes two open field lines reconnecting.
Figure 6. Observations of the November 9th, 1997 flux rope passage. The format of the figure is same as Figure 1.
Figure 7. The reconnection exhaust around 11:18 UT November 16th measured by Wind. The format of the figure is same as Figure 2.
Figure 8. The reconnection exhaust around 11:18 UT November 16th measured by Wind. The format of the figure is same as Figure 3.
In this study, the magnetic reconnection exhaust criteria of Gosling et al. [18] was used to examine the plasma and magnetic field data measured by Wind in 1997. Using these data, 621 reconnection exhausts were identified, and 31 of the 621 reconnection events were observed at the boundaries of magnetic flux ropes that were thought to lead to the open and/or disconnection of the magnetic field lines of the adjacent ropes. The 31 magnetic reconnection exhausts and their related flux ropes are listed in Table 1. The results of Table 1 can be summarized as follows: (1) 29 of the 31 magnetic reconnection events were interchange reconnections (a type of magnetic reconnection between open magnetic fields and closed magnetic field lines where the closed field lines of these related flux ropes were opened by them); (2) only 2 of the 31 magnetic reconnection events disconnected the opened field lines of flux ropes (one reconnection type was reconnecting with the adjacent open field lines and the other was reconnecting with the adjacent disconnected field lines); and (3) the scales of most magnetic flux ropes are small, and the average time scale is only 127 min. Interchange reconnection and disconnection are two mechanisms proposed to balance the heliospheric magnetic flux increased by the ceaselessly ejection of closed flux ropes from the Sun. Although we used the terms “interchange reconnection” and “disconnection” here, we were not sure if the reconnection reported here make contribution to the balance of the heliospheric magnetic flux. Since although reconnection was ongoing when the exhaust was detected, the closed magnetic flux produced by the reconnection could not go back to the Sun.
In summary, the results presented here revealed that: (1) flux ropes with magnetic reconnections at their boundaries are more likely to remain attached to the sun, which is consistent with previously observations that most of the flux ropes at 1 AU have closed magnetic field [e.g., [1, 25, 27]]; (2) few magnetic flux ropes are open near 1 AU and seldom do the open magnetic field lines of these flux ropes disconnect by interplanetary magnetic reconnection; (3) field lines of small magnetic flux ropes are easy to open or disconnect when magnetic flux ropes move from the Sun to the interplanetary space; and (4) the closed magnetic flux ropes can be gradually opened and/or disconnected through interplanetary magnetic reconnections, and the percentage of closed field lines should continually decrease when magnetic flux ropes move away.
Publicly available datasets were analyzed in this study. This data can be found at: http://cdaweb.gsfc.nasa.gov/cdaweb/istp_public/.
HF drafted the manuscript and led the observational analysis. YZ, QL, GZ, and JW conducted analysis of the current layer and flux rope. All authors contributed to the interpretation of the results and helped draft the manuscript.
The authors declare that the research was conducted in the absence of any commercial or financial relationships that could be construed as a potential conflict of interest.
We acknowledge supports from NSFC under Grant Nos. 41974197 and 41804162. We thank NASA/GSFC for the use of data from the Wind. These data can obtain freely from the Coordinated Data Analysis Web (http://cdaweb.gsfc.nasa.gov/cdaweb/istp_public/).
1. Wang J, Zhao Y, Feng H, Tian Z, Li H, Zhao A, et al. Comparison of counterstreaming suprathermal electron signatures of ICMEs with and without magnetic cloud: are all ICMEs flux ropes?. Astron Astrophys. (2019) 632:A129. doi: 10.1051/0004-6361/201936733
2. Moldwin MB, Ford S, Lepping R, Slavin JA, Szabo A. Small-scale magnetic flux ropes in the solar wind. Geophys Res Lett. (2000) 27:57–60.
3. Feng H, Zhao G, Wang JM. Small interplanetary magnetic flux rope. Sci China Tech Sci. (2019) 62:183–94. doi: 10.1007/s11431-018-9481-1
4. Gonzalez WD, Tsurutani BT, Clúa de Gonzalez AL. Interplanetary origin of magnetic storms. Space Sci Rev. (1999) 88:529–62. doi: 10.1023/A:1005160129098
5. Feng H, Chao JK, Lyu LH, Lee LC. The relationship between small interplanetary magnetic flux rope and the substorm expansion phase. J Geophys Res. (2010) 115:A09108. doi: 10.1029/2009JA015191
6. Zhang XY, Moldwin MB, Cartwright M. The geo-effectiveness of interplanetary small-scale magnetic flux ropes. J Atmos Solar-Terr Phys. (2013) 95–96:1–14. doi: 10.1016/j.jastp.2012.12.006
7. Crooker NU, Gosling JT, Kahler SW. Reducing heliospheric magnetic flux from coronal mass ejections without disconnection. J Geophys Res. (2002) 107:1028. doi: 10.1029/2001JA000236
8. Gosling JT. Large scale inhomogeneities in the solar wind of solar origin, Rev Geophys. (1975) 13:1053–8. doi: 10.1029/RG013i003p01053
9. McComas DJ. Tongues, bottles, and disconnected loops: the opening and closing of the interplanetary magnetic field. US. Natl. Rep. Int. Union Geod. Geophys. (1995) 1991–1994, Rev Geophys. (1995) 33:603–8. doi: 10.1029/95RG00124
10. Crooker NU, Owens MJ. Interchange reconnection: remote sensing of solar signature and role in heliospheric magnetic flux budget. Space Sci Rev. (2012) 172:201–8. doi: 10.1007/s11214-011-9748-1
11. Gosling JT, Birn J, Hesse M. Three-dimensional magnetic reconnection and the magnetic topology of coronal mass ejection events. Geophys Res Lett. (1995) 22:869–72. doi: 10.1029/95GL00270
12. Crooker NU, Owens MJ. SOHO-23: understanding a peculiar solar minimum. In: Cranmer SR, Hoeksma T, Kohl JL, editors. ASP Conf. Ser. 428. San Francisco, CA: ASP (2010). p. 279.
13. Baker D, Rouillard AP, van Driel-Gesztelyi1 L, Démoulin P, Harra1 LK, Lavraud B, et al. Signatures of interchange reconnection: STEREO, ACE and Hinode observations combined. AnGeo. (2009) 27:3883. doi: 10.5194/angeo-27-3883-2009
14. Lavraud B, Owens MJ, Rouillard AP. In situ signatures of interchange reconnection between magnetic clouds and open magnetic fields: a mechanism for the erosion of polar coronal holes? Solar Phys. (2011) 270:285. doi: 10.1007/s11207-011-9717-6
15. DeForest CE, Howard TA, McComas DJ. Disconnecting open solar magnetic flux. Astrophys J. (2012) 745:36. doi: 10.1088/0004-637X/745/1/36
16. Tian H, Yao S, Zong Q, Jiansen He J, Qi Y, et al. Signatures of magnetic reconnection at boundaries of interplanetary small-scale magnetic flux ropes. Astrophys J. (2010) 720:454.
17. Wang JM, Feng H, Zhao G. Observations of a small interplanetary magnetic flux rope opening by interchange reconnection. Astrophys J. (2018) 853:94. doi: 10.3847/1538-4357/aaa131
18. Gosling JT, Skoug RM, Smith CW. Direct evidence for magnetic reconnection in the solar wind near 1 AU. J Geophys Res. (2005) 110:A01107. doi: 10.1029/2004JA010809
19. Ruffenach A, Lavraud B, Owens MJ, Sauvaud J-A, Savani NP, Rouillard AP, et al. Multispacecraft observation of magnetic cloud erosion by magnetic reconnection during propagation. J Geophys Res. (2012) 117:A09101. doi: 10.1029/2012JA017624
20. Feng H, Wang JM, Zhao G, Zhao Y. Observations on the magnetic disconnections of a magnetic cloud from the sun through magnetic reconnection. Astrophys J. (2018) 864:101. doi: 10.3847/1538-4357/aad95e
21. Lepping RP, Acuna MH, Burlaga LF, Farrell WM, Slavin JA, Schatten KH, et al. The wind magnetic field investigation, edited by Russell, C. T. Space Sci Rev. (1995) 71:207–29. doi: 10.1007/BF00751330
22. Lin RP, Anderson KA, Ashford S, Carlson C, Curtis D, Ergun R, et al. A three-dimensional plasma and energetic particle investigation for the wind spacecraft. Space Sci Rev. (1995) 71:125–53. doi: 10.1007/BF00751328
23. Feldman WC, Anderson RC, Asbridge JR, Bame SJ, Gosling JT, Zwickl RD, et al. Plasma electron signature of magnetic connection to the Earth's bow shock: ISEE 3. J Geophys Res. (1982) 87:632–42. doi: 10.1029/JA087iA02p00632
24. Larson DE, Lin RP, McTiernan JM, McFadden JP, Ergun RE, McCarthy M, et al. Tracing the topology of the October 18–20, 1995 magnetic cloud with ~0.1–102 keV electrons. Geophys Res Lett. (1997) 24:1911–4. doi: 10.1029/97GL01878
25. Shodhan S, Crooker NU, Kahler SW, Fitzenreiter RJ, Larson DE, Lepping RP, et al. Counterstreaming electrons in magnetic clouds. J. Geophys. Res. (2000) 105:27261–8. doi: 10.1029/2000JA000060
26. Crooker NU, Forsyth R, Rees A, Gosling JT, Kahler SW. Counterstreaming electrons in magnetic clouds near 5 AU. J Geophys Res. (2004) 109:A06110. doi: 10.1029/2004JA010426
27. Feng H, Zhao G, Wang JM. Counterstreaming electrons in small interplanetary magnetic flux ropes. J Geophys Res: Space Phys. (2015) 120:10175–84. doi: 10.1002/2015JA021643
28. Xu M, Shen C, Hu Q, Wang Y, Chi Y. Whether small flux ropes and magnetic clouds have the same origin: a statistical study of small flux ropes in different types of solar wind. Astrophys J. (2020) 904:122. doi: 10.3847/1538-4357/abbe21
29. Feng H, Wu D, Lin C, Chao JK, Lee CL, Lyu LH. Interplanetary small- and intermediate-sized magnetic flux ropes during 1995–2005. J Geophys Res. (2008) 113:A12105. doi: 10.1029/2008JA013103
30. Feng H, Wu D, Chao JK. Comment on “Comparison of small-scale flux rope magnetic properties to large-scale magnetic clouds: evidence for reconnection across the HCS”? by M. L. Cartwright and M. B. Moldwin. J Geophys Res. (2010) 115:A10109. doi: 10.1029/2010JA015588
31. McComas DJ, Gosling JT, Phillips JL, Bame SJ, Luhmann JG, Smith EJ. Electron heat flux dropouts in the solar wind: evidence for interplanetary magnetic field reconnection?. J Geophys Res. (1989) 94:6907–16. doi: 10.1029/JA094iA06p06907
32. Paschmann G, Papamastorakis I, Baumjohann W, Sckopke N, Carlson CW, Sonnerup BUÖ, et al. The magnetopause for large magnetic shear: AMPTE/IRM observations. J Geophys Res. (1986) 91:11099–115. doi: 10.1029/JA091iA10p11099
33. Phan TD, Gosling JT, Davis MS, Skoug RM, Øieroset M, Lin RP, et al. A magnetic reconnection X-line extending more than 390 Earth radii in the solar wind. Nature. (2006) 439:175–8. doi: 10.1038/nature04393
34. Xu X, Wei F, Feng X. Observations of reconnection exhausts associated with large-scale current sheets within a complex ICME at 1 AU. J Geophys Res. (2011) 116:A05105. doi: 10.1029/2010JA01615
Keywords: magnetic flux rope, interchange reconnection, magnetic disconnection, magnetic reconnection, interplanetary space
Citation: Feng H, Zhao Y, Wang J, Liu Q and Zhao G (2021) Observations of Magnetic Flux Ropes Opened or Disconnected From the Sun by Magnetic Reconnection in Interplanetary Space. Front. Phys. 9:679780. doi: 10.3389/fphy.2021.679780
Received: 12 March 2021; Accepted: 14 April 2021;
Published: 12 May 2021.
Edited by:
Xueshang Feng, National Space Science Center (CAS), ChinaReviewed by:
Chenglong Shen, University of Science and Technology of China, ChinaCopyright © 2021 Feng, Zhao, Wang, Liu and Zhao. This is an open-access article distributed under the terms of the Creative Commons Attribution License (CC BY). The use, distribution or reproduction in other forums is permitted, provided the original author(s) and the copyright owner(s) are credited and that the original publication in this journal is cited, in accordance with accepted academic practice. No use, distribution or reproduction is permitted which does not comply with these terms.
*Correspondence: Hengqiang Feng, ZmVuZ2hxOTkyMUAxNjMuY29t
Disclaimer: All claims expressed in this article are solely those of the authors and do not necessarily represent those of their affiliated organizations, or those of the publisher, the editors and the reviewers. Any product that may be evaluated in this article or claim that may be made by its manufacturer is not guaranteed or endorsed by the publisher.
Research integrity at Frontiers
Learn more about the work of our research integrity team to safeguard the quality of each article we publish.