- 1Prokhorov General Physics Institute of the Russian Academy of Sciences, Moscow, Russia
- 2“Sensorica” National Technological Initiative Center, National Research University of Electronic Technology, Moscow, Russia
The results of a study of the dynamics of the excitation angle of a surface electromagnetic wave (SEW) at the interface between a silver/NaCl aqueous solution of concentrations 10−3, 10−4, 10−6 and 10−10 M are presented. It is shown that the rate of change in the excitation angle of SEW is proportional to the concentration of the solution up to a dilution of 10−10 M. The observed effect is explained by the formation of silver chloride on the surface of the silver film as a result of the interaction of solution ions with nanoscale clusters of the silver film. The proposed technique for measuring the dynamics of the excitation angle of SEW can be used for a comparative analysis of the concentrations of highly dilute solutions, as well as for studying the formation and dynamics of transition layers and physicochemical processes in the near-surface regions.
1 Introduction
The problem of low concentrations measurements is an important area of scientific and applied research [1, 2]. There are many measurement methods based on various physical principles, for example, optical, mass spectrometric, atomic adsorption and atomic fluorescence methods, etc. These methods make it possible to measure the molecular concentration up to 10−9 M [3–6]. However, their high sensitivity is combined with highly complex measurement process.
Along with direct methods for measuring concentrations, methods based on comparing the test and reference solutions are often used. Such methods do not provide an accurate value for the concentration, but they provide a qualitative estimate. The advantage of such methods is their instrumental simplicity and availability of implementation. These methods include, in particular, techniques based on spectroscopy of surface electromagnetic waves. A feature of these techniques is the measurement of the dielectric characteristics of a substance in a narrow near-surface layer at the metal-dielectric interface. The use of SEW spectroscopy methods to determine the concentration of solutions requires knowledge of the functional dependence of the dielectric constant on the concentration of the dissolved substance and the solution of the inverse problem, during which the dielectric parameters of the contacting media must be obtained from the excitation parameters. However, as a rule, the metal–liquid interface has a complex structure, to which the approximation of two semi-infinite media is generally inapplicable. In this regard, the solution to the inverse problem has no unique solution. In this regard, such techniques are used in comparative analyzes with reference solutions.
In the general case, the sensitivity of SEW methods to the dielectric parameters of media is determined by the angular error in determining the dependence of the intensity on the angle of incidence. In the plane wave approximation, the error of Kretchman and Otto's SEW excitation methods can be estimated by the following relationship:
where: np is the refractive index of the incident coupling prism, εm is the permittivity of the metal, n2 is the refractive index of the studied investigated medium, θ is the angle of incidence of the exciting radiation.
According to this equation, the refractive index sensitivity is approximately equal to the accuracy of the excitation angle measurement. At the same time, the accuracy of measuring the excitation angle is limited by the angular divergence of the exciting beam. With a laser beam divergence of 1 mrad, the refractive index determination accuracy will be approximately 10−4 10−3 RIU. This sensitivity is sufficient for studying solutions of concentrations up to about 10−3 M. For the study of highly dilute solutions, this sensitivity is in many cases insufficient, even despite the effect of an increase in the concentration of a dissolved substance in the near-surface layer, called the electric double layer or the Helmholtz layer [7].
However, in the case of using a metal for SEW excitation, which physically or chemically interacts with solution ions, an increase in the sensitivity of the technique can be expected due to the interaction products [8].
The interaction of solution ions with a metal surface can proceed relatively slowly for highly dilute solutions. In this regard, we propose a technique based not on the traditional comparison of the conditions for the excitation of SEW in the compared solutions, but on the comparison of the dynamics of these parameters. In particular, this work presents the results of comparing the dynamics of the excitation angle of SEW for various solutions of the NaCl salt with concentrations from 10−3 to 10−10 M.
2 Methods
A small amount of substance in a solvent causes the refraction index of the solution to change very insignificantly. This change may turn out to be rather low to be measured. However, if physical or chemical interaction occurs between the components of the solution and the metal surface, then these processes can be detected due to the accompanying products of this interaction, which lead to a change in the parameters of the interface.
These interactions, due to low concentrations of the reagents, can last for a long time, creating an accumulation effect. In terms of the SEW excitation technique, it means a slow change in surface wave excitation parameters, which is small enough in the short-term, but detectable over long periods of observation. So the characteristic of this interaction would be the rate of the measured parameters changes, for example the rate of the SEW excitation angle change. We have studied such time-dependent changes of signal in five different media: distilled water and aqueous NaCl solutions at concentrations of 10−3, 10−4, 10−6, and 10−10 М.
An approximately 50 nm thick layer of silver deposited by magnetron sputtering, has a structure of nanosized granules (Figure 1A). The interaction of aqueous NaCl solution with this kind of surface has been studied by the authors of paper [9], where the formation of AgCl on the surface of silver clusters has been demonstrated. In particular, the surface of a 50 nm silver film following interaction with 10−3 M NaCl for 72 h is shown in Figure 1B. Such changes in the structural and phase composition of the film surface have impact on SEW excitation parameters.
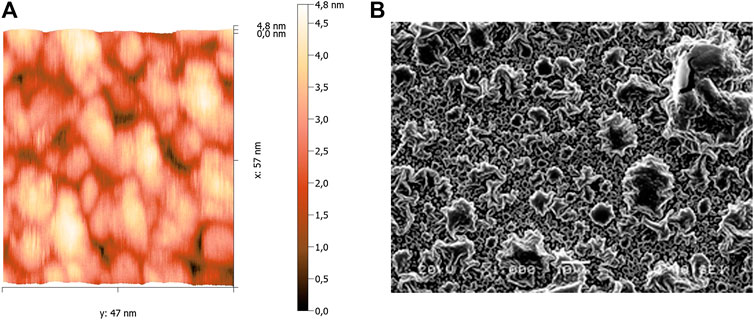
FIGURE 1. An AFM image of the surface of a 50 nm silver film produced by sputter deposition (A), (B): an image of the surface of the 50 nm silver film after interaction with a 10−3 M NaCl solution for 72 h (the image is obtained using a JSM-5900 scanning electron microscope).
The general condition for an excitation of surface plasmon (SP) at interfaces is that the following equation should be satisfied: εm′(ω) ≤ –ε0, where εm′(ω) is the permittivity of the metal, and ε0–is the permittivity of the medium at the interface with which the SP excitation occurs. High sensitivity at interfaces is provided by the localization of the electromagnetic field in the narrow subsurface layer.
The interfacial electrical double layer form on the metal–liquid interfaces The dielectric parameters of this layer differ from the parameters of the bordering media, and SP excitation at such interfaces should be considered as excitation in a three-(or more) layer system.
In particular, when an interfacial layer is formed between liquid and metal surface, the surface electromagnetic waves (SEW) become excited and propagating in a structure–metal/interfacial layer/studied medium [10, 11].
In this case, the adsorption of dissolved molecules on the surface and oxide layer of the metal film should be considered, how it change the characteristics of the electrical double layer and, consequently, the SEW propagation conditions. The equation for an effective wave vector also changes.
We studied the changes in SEW excitation parameters at the interface between a metal and aqueous NaCl solutions at concentrations of 10−3, 10−4, 10−6, and 10−10 M. The SEW was excited by He-Ne laser light (0.6328 nm). The aim of this work was to study the applicability of surface plasmon spectroscopy (SPR) to research the solutions with concentrations lower than 10−3 M. For this aim the distilled water (12 MOm·cm) and NaCl solutions were used.
The experiments were performed using a closed cell setup with a constant water flow. The scheme of device is shown in Figure 2. The system was designed to induce SEM excitation using the Kretschmann configuration at metal-air and metal-liquid interfaces. The system’s controlled parameter is the angular dependence of the intensity of light reflected from an interface. The analysist system allows single and multiple angular measurements with a precision of 0.0125о. Three mutually perpendicular alignment mechanisms (11) are mounted on a rotating table (10) driven by a control unit (14) and capable of rotating at θ = 360о. A laser (1) generates a p-polarized light image on the prism hypotenuse (13) by means of a mirror (4.1–4.3) and polarizer (2). The output light beam is reflected from a mirror (3) and is focused by a lens (5) onto an optical detector (7). Then the signal is digitized (9) and transmitted to a computer (15). The intensity of the incident light is monitored by an optical sensor (6), which receives light from a beam divider (3). The measurement process is automated and run by the computer (15). The main detecting element of the analysis system is the prism having a refractive index n = 1.75. One of its faces is coated with a silver 54 nm thick film. The SPW excitation angle corresponded to θр = 53.4о. The silver layer was coated using sputter deposition carried out in automatic mode, at which time the SP excitation at 650 nm was monitored in real time [12]. The error of prism mounting accuracy was not more than 0.0012о. Scans were recorded over an angle range of 51о to 59о, at a temperature of 22оС. The solutions were introduced into the cell from an external reservoir through a medical silicone tube.
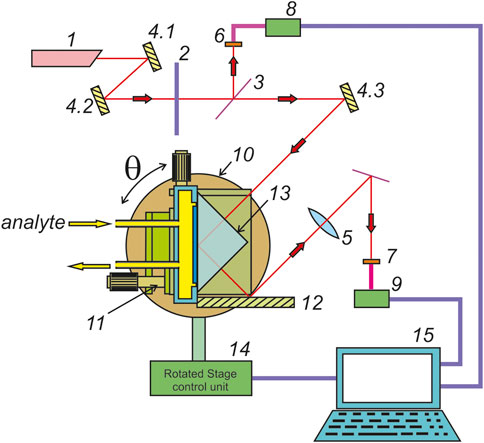
FIGURE 2. A schematic of plasmon resonance analysis system (1–laser; 2–polarizer; 3–beam splitter; 4.x-mirrors; 5– focusing lens; 6, 7– optical detector; 8, 9– optical detection modules; 10– rotating table; 11– alignment elements; 12– corner reflector mirror; 13– prism; 14–electronic control unit of the rotating table; 15– control computer).
3 Results and Discussion
Figure 3 shows shifts in the resonance angle of surface plasmon excitation vs. time for distilled water and aqueous 10−4 M NaCl solution. As can be seen, the shift in resonance observed with distilled water is 0.75° after 3,000 min. This is caused by the oxidation of the silver layer, which is the only reason here for the signal change. Meanwhile, the modified surface remains sensitive enough to the introduction of NaCl diluted to 10−4 M. The shift in resonance minimum rises sharply when the distilled water is replaced with the NaCl solution. The change rate of resonance minimum varies over time. This is associated with the formation of an AgCl layer and changes in SEW propagation conditions at the silver-NaCl solution interface.
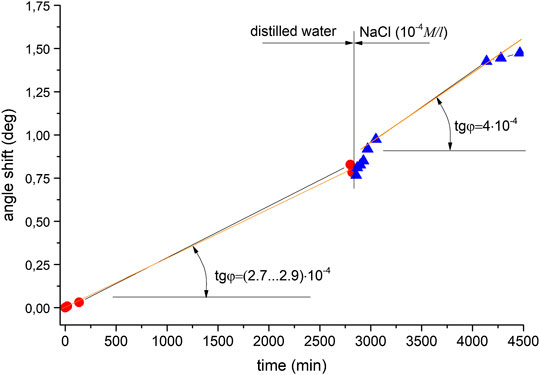
FIGURE 3. Shifts observed in the angle of minimum reflected intensity over time for the following solutions: distilled water, NaCl (10−4 M).
So there are two distinguishable areas in the plot. In the first part, the angle change rate is approximately (2.7 … 2.9)·10−4°/min, which is the average value for distilled water in all of the Ag experiments. In the second part, the change rate is increasing as compared with the first area–to approximately 4⋅10−4°/min. A small decrease in excitation angle change rate to 4.8⋅10−4°/min is caused by decreasing of NaCl concentration and slower AgCl layer formation. Namely, a lower angle change rate corresponds to a lower concentration of substance. There is a characteristic sharp rise in the region of solution replacement corresponding to the change in resonance conditions at the silver-water interface, with a slope of 1.1х10−3, which indicates a sudden modification of the subsurface layer caused by sodium ions interacting with the surface. After that point, the shift is slower and steady until it reaches saturation, where the slope is 4⋅10−4°/min. The dependence of this curve’s slope on concentration was tested by using other aqueous NaCl solution concentrations. Figure 4 shows the results obtained for distilled water and subsequent solutions of concentrations 10−4 M and 10−3 M, where there is also an increase in slope: namely, the transition from a solution with a concentration of 10−4 M to a solution with a concentration of 10−3 M led to an increase in the rate of change in the angle from 4.9 to 7.6·10−4°/min. Figure 5 displays a comparison of solutions with lower concentrations: 10−4 M and 10−6 M. A noticeably smaller difference in terms of curve slope is observed for NaCl(10−6 M) and NaCl(10−4 M), i.e., µ = 0.8 (8*10−4/1*10−3). Therefore, slope changes can be considered proportional to concentration. This difference is reliably detectable by measurements using the analysis system, indicating its high sensitivity. The sequence, of measuring solutions concentrations was changed in the next experimental series–Figure 6. Here distilled water was again measured as the reference. It was followed by 10−6 M NaCl and then a lower concentration–by 10−10 M NaCl. The reverse sequence of solutions also shows differences in observed shifts between different concentrations: the rate of change is greater for higher concentrations than for lower concentrations.
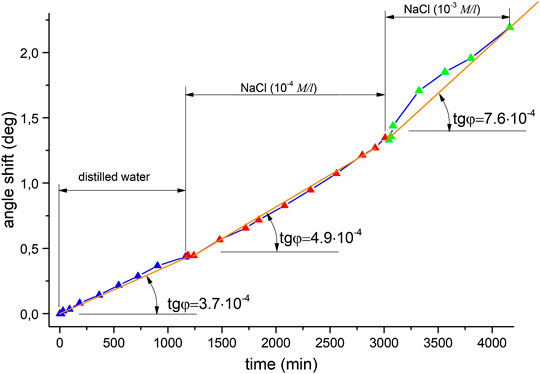
FIGURE 4. Shifts observed in the angle of minimum reflected intensity over time for the following solutions: distilled water, NaCl (10−4 M), and NaCl (10−3 M).
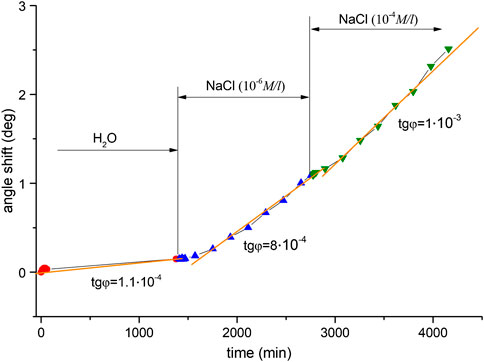
FIGURE 5. Shifts observed in the angle of minimum reflected intensity over time for the following solutions: distilled water, NaCl (10−6 M), and NaCl (10−4 M).
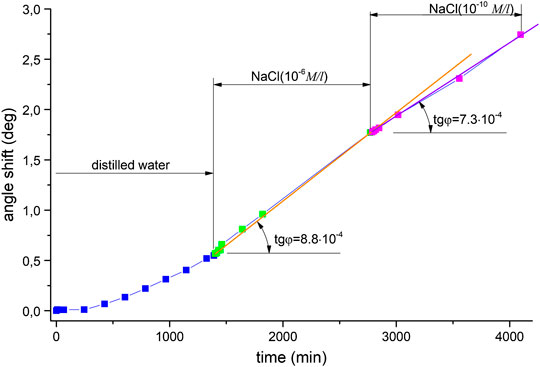
FIGURE 6. The shift observed in the angle of minimum reflected intensity over time for the following solutions: distilled water (7.2Mom), NaCl (10−6 M), and NaCl (10−10 M).
4 Conclusion
The experimental observation of time-dependent changes in the parameters of SEW excitation in low-concentration aqueous solutions has shown that the excitation angle depends on the contact time between aqueous NaCl solution and the silver film. The measured angular shift was 10−4–10−3°/min. It has also been shown that the change rate of SEW excitation angle is dependent on solution concentration.
The observed effect is linked to a modification of the interface, which occurs as the formation of an interfacial layer of solution ions, as well as of an AgCl surface film.
The proposed technique, based on measuring the time dependence of the excitation angle of SEW in solutions of different concentrations, can be extended by studying the time dependences of additional parameters of excitation of surface waves, such as the width at half maximum and the depth of the resonance dip. The study and analysis of the time dependences of the excitation parameters of SEW can be used to investigate interfaces in highly dilute solutions. In particular, to study the formation and dynamics of transition layers, physical and chemical processes in the near-surface regions.
Data Availability Statement
The original contributions presented in the study are included in the article/Supplementary Material, further inquiries can be directed to the corresponding author.
Author Contributions
MAK and VVS conducted experiments. VVS, BAU and VIP participated in the processing of the results and their discussion. VVS and VIP participated in writing the text of the manuscript.
Conflict of Interest
The authors declare that the research was conducted in the absence of any commercial or financial relationships that could be construed as a potential conflict of interest.
Acknowledgments
The authors wish to express their gratitude to Igor Ershov of the Center of Laser Technology and Material Science for his assistance for sample preparing; MMH also acknowledges support from Project No. N0001490J1537.
References
1. Homola J. Surface plasmon resonance sensors for detection of chemical and biological species. Chem Rev (2008) 108(2):462–93. doi:10.1021/cr068107d
2. Eksperiandova LP, Belikov KN, Khimchenko SV, Blank TA. Once again about detection and quantification limits. J Anal Chem (2010) 65(3):229–34. doi:10.1134/S1061934810030020
3. Mermet J-M. Calibration in atomic spectrometry: a tutorial review dealing with quality criteria, weighting procedures and possible curvatures. Spectrochim Acta B (2010) 65:509–23. doi:10.1016/j.sab.2010.05.007
4. Dedushenko KB, Egorov SA, Ershov YA, Likhachev IG. The interferometric fiber optic measurement system. Apparatuses (2002) 7:23.
5. Kazaryan MA, Krasovsky VI, Feofanov IN, Krichevsky DМ, Likhachev IG, Kulikovsky AV, et al. The NO2 fiber optic sensor detector. Photonics (2017) 64(4):92–5. doi:10.22184/1993-7296.2017.64.4.92.95
6. West TS. The determination of very small amounts of materials by the techniques of atomic-absorption and atomic-fluorescence spectroscopy. Pure Appl Chem (1971) 26(1):47. doi:10.1351/pac197126010047
7. Damaskin BB, Frumkin AN. Potentials of zero charge, interaction of metals with water and adsorption of organic substances. Electrochim Acta (1974) 19:173–6. doi:10.1016/0013-4686(74)85058-9
8. Kononov MA, Pustovoy VI, Svetikov VV. Specific features of the excitation of surface plasmons at the interface between metal and aqueous solution of extremely low concentration. Phys Wave Phenom (2020) 28(2):94–7. doi:10.3103/s1541308x20020089
9. Levard C, Mitra S, Yang T, Jew AD, Badireddy AR, Lowry GV, et al. Effect of chloride on the dissolution rate of silver nanoparticles and toxicity to E. coli. Environ Sci Technol (2013) 47:5738–45. doi:10.1021/es400396f
10. Agranovich VM, Mills DL. Surface polaritons: Electromagnetic waves at surfaces and interfaces. Amsterdam: North-Holland Pub. Co. (1982)
11. Schmickler W. Electrical double layers: theory and simulations. Encycl Electrochem (2007) [Epub ahead of print]. doi:10.1002/9783527610426.bard010202
Keywords: surface electromagnetic wave, plasmon, aqueous solution, silver, sodium chloride
Citation: Kononov M, Pustovoy V, Svetikov V and Usievich B (2021) Excitation of Surface Electromagnetic Waves at the Silver/NaCl Aqueous Solution Interface. Front. Phys. 9:636979. doi: 10.3389/fphy.2021.636979
Received: 02 December 2020; Accepted: 11 January 2021;
Published: 15 February 2021.
Edited by:
Nikolai F. Bunkin, Bauman Moscow State Technical University, RussiaReviewed by:
Igor Ageev, Moscow Aviation Institute, RussiaNiravkumar J Joshi, University of Sao Paulo, Brazil
Copyright © 2021 Kononov, Pustovoy, Svetikov and Usievich. This is an open-access article distributed under the terms of the Creative Commons Attribution License (CC BY). The use, distribution or reproduction in other forums is permitted, provided the original author(s) and the copyright owner(s) are credited and that the original publication in this journal is cited, in accordance with accepted academic practice. No use, distribution or reproduction is permitted which does not comply with these terms.
*Correspondence: V.V. Svetikov, vl.svetikov@gmail.com