- Department of Microwave Engineering, Harbin Institute of Technology, Harbin, China
Wireless communication has become a standard solution to satisfy the ever-increasing demands of information transfer in our daily life. Furthermore, reconfigurable metasurfaces comprised of multiple tunable unitcells have drawn significant attention due to their superior electromagnetic performance, while the desired electromagnetic response can be controlled by computer. We therefore present a prototype of a wireless communication system based reconfigurable metasurface that works in the microwave frequency range. A 2-D periodical array of a reconfigurable metasurface is loaded with a varactor diode to effectively adjust the in-band transmission and reflection coefficients that maintain different far-field electromagnetic characteristics. The reconfigurable metasurface does not radiate electromagnetic waves and only carries information by adjusting its reflection and transmission coefficients. With this reconfigurable metasurface, a passive communication method can be realized.
1 Introduction
It is foreseen that the commercial service of the fifth-generation (5G) of mobile communications will be launched on a worldwide scale starting in 2020. The application of the Internet of Things as an important part of the fifth-generation of mobile communication has a very broad development prospect [1]. At present, wireless sensors in IoT devices are facing two major problems: power consumption and transmission distance [2]. The server’s increasing demands are driven by various intelligent devices, such as smart meters, telemedicine, virtual reality, and autonomous driving, all of which include a lot of wireless sensor devices. With the growth of these mobile Internet services, the requirements for sensor power consumption have become increasingly higher. Currently, short-range wireless communication methods mainly include near-field communication (NFC), Bluetooth, and Zigbee, and most of these methods mainly work with frequency types such as S-band. A metasurface, composed of sub-wave-length resonators in 2-D plane [3–6], can provide a new way to control electromagnetics (EM) in terms of propagation modes, polarization, and wave-fonts [7–12], and recently an active anisotropic metasurface whose reflection phases can be electrically and independently tuned for two orthogonal polarized waves was reported [13]. Due to their unique EM properties, we propose a passive transmitter, using a reconfigurable metasurface design work in 2.4 GHz, to reduce the transmitter’s power consumption and to make the metasurface compatible with the standard IEEE 802.11ac. IEEE 802.11ac is a wireless networking standard in the 802.11 set of protocols (which is part of the Wi-Fi networking family), providing high-throughput wireless local area networks (WLAN) on the 2.4 GHz and 5 GHz band.
In this paper, a single layer reconfigurable metasurface has been presented with the aim of reducing power consumption. The proposed geometry consists of periodic metallic Patterns imprinted on the top of the dielectric substrate, where varactor diodes are mounted in the center of each unit cell. The reconfigurable metasurface has been constructed such that it can integrate two different characteristics (single band reflection and transmission) with independent control of the biasing states of the varactor diodes. In order to communicate with the passive transmitter a receiver has been designed with a software-defined radio (SDR) [14–18]. A software-defined radio is a radio communication system where components that have been traditionally implemented in hardware are instead implemented by means of software on a personal computer or an embedded system. While the concept of SDR is not new, the receiver in this paper only provided a method to verify this design. The varactors in the reconfigurable metasurface are controlled by the base band signal.
The remainder of this paper is organized as follows. Section 2 presents the proposed design concept for a passive transmitter, then describes the system composition and characteristics of power consumption. Section 3 discusses the theoretical investigation of this design and show the simulation results. Finally, concluding remarks and a comparison of the proposed passive transmitter to the traditional short-range wireless communication methods are presented in the last section.
2 Reconfigurable Metasurface Passive Transmitter
2.1 The Design of Unitcell
The reconfigurable metasurface is a lattice of 2-D subwave-length meta-atoms loaded with varactor diodes [14–23]. The Schematic view of the unitcell is illustrated in Figure 1. It includes one metallic layer that is placed on one substrate (F4B with a dielectric constant of 2.65 and loss tangent of 0.001). On the top layer is a “E” shape metallic strip combined by a varactor diode surrounded by metallic strips. The Structural parameters are chosen as follows: The width of the unitcell
2.2 System Model
A Full structure sample containing
In this passive transmitter radio frequency (RF) source and amplifier is not employed, so the transmission coefficient is obtained as
where
For simplicity, let us assume that a wireless source emits a seemingly random signal
When the metasurface is driven by the EM wave
where
where
In this work, the varactor diodes are working at two states, one is without biasing voltage
3 Simulations and Discussion
To verify the performance of the proposed metasurface, Frequency domain simulations are performed using the CST studio and GNUradio software. In our Simulations, the unitcells are driven by the horizontal polarized wave from 1.5 GHz to 3 GHz. The reflection and transmission coefficient are shown in Figure 4 and Figure 5. As an illustrative example, we find that the reconfigurable metasurface worked at 2.4 GHz by adjusting the biasing voltage, and the EM resonance changes as expected.
Furthermore, we simulated the reconfigurable metasurface’s farfield properties. From the farfield simulation results, it can be intuitively seen that the main lobe of the reconfigurable metasurface covers half of the space and the amplitude of the backward radiation is very low. In this case, its farfield reflection properties can be well modulated.
Particularly, the reflectivity and phase difference of the two elements under the illumination of forward x-polarized incidence are depicted in Figure 6, respectively. It is clearly shown that the complete reflection with direction difference approximately approaching 180°, is well kept in
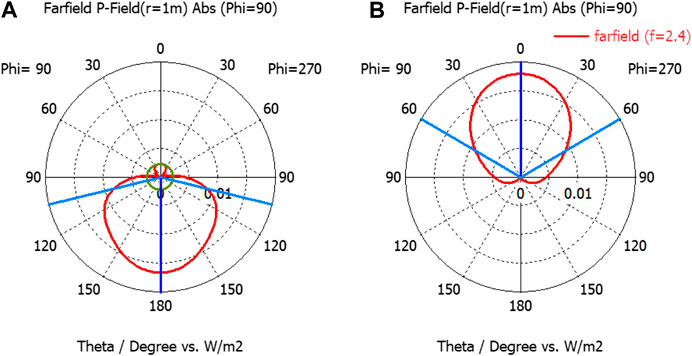
FIGURE 6. Simulation results of the reconfigurable metasurface’s far-field radiation properties. (A) V = 0 V the metasurface working as a reflector. (B) V = 28 V the metasurface working in the transmission state, which can be regarded as an absorber due to the combination with the absorber placed behind the metasurface.
Based on the design proposed above, we can combine it into a new communication system. The reconfigurable metasurface whose biasing voltage are controlled by the base band signal plays the role of transmitter. The continuous wave radar designed by GNUradio is used as a receiver. In this communication system the transmitter does not radiate any EM wave, which is of great significance for EM silence. In IoT applications, a large part of the sensor data transmission is unidirectional, and the power is limited. The passive trans proposed in this paper may solve these problems.
4 Conclusion
In summary, we provided a theoretical framework for modulation, and simulated a prototype system tailored to the use of ambient commodity
Data Availability Statement
The raw data supporting the conclusions of this article will be made available by the authors, without undue reservation.
Author Contributions
SY designed and performed the design and simulation as well as wrote the paper. All authors participated in the data analysis and read the manuscript.
Conflict of Interest
The authors declare that the research was conducted in the absence of any commercial or financial relationships that could be construed as a potential conflict of interest.
References
1. Liu F, Tsilipakos O, Pitilakis A, Tasolamprou AC, Mirmoosa MS, Kantartzis NV, et al. Intelligent metasurfaces with continuously tunable local surface impedance for multiple reconfigurable functions. Phys Review Appl (2019) 11:044024. doi:10.1103/physrevapplied.11.044024
2. Liu H, Sarrazin J, Deshours F, Mavridis T, Petrillo L, Liu Z, et al. Performance assessment of IR-UWB body area network (BAN) based on IEEE 802.15.6 standard. IEEE Antenn Wireless Propag Lett (2016) 15:1645–8. doi:10.1109/LAWP.2016.2520021
3. Li A, Singh S, Sievenpiper D. Metasurfaces and their applications. Nanophotonics (2018) 7:989–1011. doi:10.1515/nanoph-2017-0120
4. Luo Z, Chen MZ, Wang ZX, Zhou L, Li YB, Cheng Q, et al. Digital nonlinear metasurface with customizable nonreciprocity. Adv Funct Mater (2019) 29:1906635. doi:10.1002/adfm.201906635
5. Moccia M, Liu S, Wu RY, Castaldi G, Andreone A, Cui TJ, et al. Coding metasurfaces for diffuse scattering: scaling laws, bounds, and suboptimal design. Adv Opt Mater (2017) 5:1700455. doi:10.1002/adom.201700455
6. Rabinovich O, Epstein A. Analytical design of printed circuit board (pcb) metagratings for perfect anomalous reflection. IEEE Trans Antenn Propag (2018) 66:4086–95. doi:10.1109/tap.2018.2836379
7. Yang R, Li D, Gao D, Zhang A, Hu B, Yang P, et al. Negative reflecting meta-mirrors. Sci Rep (2017) 7:5729. doi:10.1038/s41598-017-06184-1
8. Tao Z, Wan X, Pan BC, Cui TJ. Reconfigurable conversions of reflection, transmission, and polarization states using active metasurface. Appl Phys Lett (2017) 110:121901. doi:10.1063/1.4979033
9. Zhang L, Wan X, Liu S, Yin JY, Zhang Q, Wu HT, et al. Realization of low scattering for a high-gain fabry-perot antenna using coding metasurface. IEEE Trans Antenn Propag (2017) 65:3374–83. doi:10.1109/tap.2017.2700874
10. Zheng Q, Li Y, Zhang J, Ma H, Wang J, Pang Y, et al. Wideband, wide-angle coding phase gradient metasurfaces based on pancharatnam-berry phase. Sci Rep (2017) 7:43543. doi:10.1038/srep43543
11. Su J, Lu Y, Zhang H, Li Z, Yang Y, Che Y, et al. Ultra-wideband, wide angle and polarization-insensitive specular reflection reduction by metasurface based on parameter-adjustable meta-atoms. Sci Rep (2017b) 7:42283. doi:10.1038/srep42283
12. Ratni B, Bochkova E, Piau G-P, de Lustrac A, Lupu A, Burokur SN. Design and engineering of metasurfaces for high-directivity antenna and sensing applications. EPJ Appl Metamater (2016) 3:4. doi:10.1051/epjam/2016008
13. Chen K, Zhang N, Ding G, Zhao J, Jiang T, Feng Y. Active anisotropic coding metasurface with independent real-time reconfigurability for dual polarized waves. Adv Mater Technol (2020) 5:1900930. doi:10.1002/admt.201900930
14. Su J, Kong C, Li Z, Yin H, Yang Y. Wideband diffuse scattering and rcs reduction of microstrip antenna array based on coding metasurface. Electron Lett (2017a) 53:1088–9. doi:10.1049/el.2017.1656
15. Chaimool S, Hongnara T, Rakluea C, Akkaraekthalin P, Zhao Y. Design of a pin diode-based reconfigurable metasurface antenna for beam switching applications. Int J Antenn Propag (2019) 2019:1–7. doi:10.1155/2019/7216324
16. Modi AY, Balanis CA, Birtcher CR, Shaman HN. New class of rcs-reduction metasurfaces based on scattering cancellation using array theory. IEEE Trans Antenn Propag (2019) 67:298–308. doi:10.1109/TAP.2018.2878641
17. Huang H, Omar AA, Shen Z. Low-rcs and beam-steerable dipole array using absorptive frequency-selective reflection structures. IEEE Trans Antenn Propag (2019) 68:2457–62. doi:10.1109/TAP.2019.2943322
18. Zhang XG, Tang WX, Jiang WX, Bai GD, Tang J, Bai L, et al. Light-controllable digital coding metasurfaces. Adv Sci (2018) 5:1801028. doi:10.1002/advs.201801028
19. Vellucci S, Monti A, Barbuto M, Toscano A, Bilotti F. Waveform-selective mantle cloaks for intelligent antennas. IEEE Trans Antenn Propag (2019) 68:1717–25. doi:10.1109/TAP.2019.2948736
20. Phon R, Ghosh S, Lim S. Novel multifunctional reconfigurable active frequency selective surface. IEEE Trans Antenn Propag (2019) 67:1709–18. doi:10.1109/TAP.2018.2889002
21. Wu H, Bai GD, Liu S, Li L, Wan X, Cheng Q, et al. Information theory of metasurfaces. Nat Sci Review (2019) 7:561–71. doi:10.1093/nsr/nwz195
22. Zhang L, Wang ZX, Shao RW, Shen JL, Chen XQ, Wan X, et al. Dynamically realizing arbitrary multi-bit programmable phases using a 2-bit time-domain coding metasurface. IEEE Trans Antenn Propag (2019) 68:2984–921. doi:10.1109/TAP.2019.2955219
Keywords: reconfigurable metasurface, wireless communication, reconfigurable transmission surface, active frequency selective surface, software defined radio
Citation: Yang S, Zhang K, Ding X, Yang G and Wu Q (2021) Theoretical Investigation of the Passive Transmitter Based on Reconfigurable Metasurface. Front. Phys. 9:634906. doi: 10.3389/fphy.2021.634906
Received: 29 November 2020; Accepted: 04 January 2021;
Published: 10 February 2021.
Edited by:
Weiren Zhu, Shanghai Jiao Tong University, ChinaCopyright © 2021 Yang, Zhang, Ding, Yang and Wu. This is an open-access article distributed under the terms of the Creative Commons Attribution License (CC BY). The use, distribution or reproduction in other forums is permitted, provided the original author(s) and the copyright owner(s) are credited and that the original publication in this journal is cited, in accordance with accepted academic practice. No use, distribution or reproduction is permitted which does not comply with these terms.
*Correspondence: Qun Wu, cXd1QGhpdC5lZHUuY24=