- 1Department of Agronomy, shahr-e-Qods Branch, Islamic Azad University, Tehran, Iran
- 2Seed and Plant Improvement Institute, Agricultural Research Education and Extension Organization, Karaj, Iran
The usage of microbes and biochar (BC) is fundamental in decreasing the impacts of salinity stress by promoting plant growth and development. The purpose of this study was to determine the impact of phosphate solubilizing bacteria (PSB) and phosphorous (P)-enriched BC on the plant growth and biochemical characteristics for three lines of sunflower (Helianthus annuus L.) in saline soils. The study was designed with salinity at 100 mM NaCl and PSB (Bacillus subtilis) with 109 CFU and BC at 1500 Kg ha-1 in the sunflower lines including RGK38 (salt-sensitive), BGK35 (salt-moderately sensitive), and BGK259 (salt-tolerant) based on completely randomized block design (CRBD) in five replicates. Salinity considerably reduced grain yield, thousand grain weight (TGW), oil content, oil yield, chlorophyll (Chl) content, relative water content (RWC), K, and P, while enhancing malondialdehyde (MDA), electrolyte leakage (EL), Na+, and Cl- in all lines of sunflower with high rate in RGK38. The use of integrated PSB and BC contributed to increases in plant yield. In RGK38 exposed to salinity, PSB+BC raised grain yield (16%), oil yield (27%), RWC (14%), Chl a+b (24%), K (22%), P (127%), but lowered MDA (26%), Na+ (36%), and Cl- (41%). Heat map analysis revealed that RGK38 stood out significantly from the other two sunflower lines with the maximum variability under the treatments. MDA showed the most variability among the lines as an indicator of identifying the susceptibility to salinity, PSB, and BC. This study has encouraging implications for agricultural operations in stress-affected areas by highlighting the potential of combining PSB and BC as environmentally acceptable and sustainable ways to increase plant resilience in salty soils.
1 Introduction
The main issues of the contemporary period for achieving the sustainable development goals (SDGs) of zero hunger, especially for plant biologists, are declining agricultural output and poor food security with a fast eruption in population. Environmental changes that inhibit plant growth, development, and yield are among the harmful stressors to which plants are subjected throughout their life cycle (Kumari et al., 2021). Salt stress were caused by poor irrigation techniques and ongoing environmental changes and poses the greatest danger to agricultural yield worldwide, particularly in arid and semi-arid regions. Salinity may alter the characteristics of soil, changing how water and minerals are absorbed (Hualpa-Ramirez et al., 2024). Salinization affects 1 billion ha of land globally, affecting arable land and 40% of global food production. Improper irrigation practices, limited drainage, and excessive chemical fertilizer use contribute to this issue. Countries like India, Pakistan, China, the US, and Australia report significant crop yield reductions and economic losses (Khondoker et al., 2023). Iran faces significant salinity issues, with 34 million hectares affected, and 50% of its irrigated land affected (Khondoker et al., 2023). Addressing salinization is a priority in Iran’s agricultural sector, with measures like promoting salt-tolerant crops and improving drainage systems. However, water scarcity and reliance on marginal lands continue to pose challenges for sustainable agriculture (Abedi et al., 2021).
Salinity limits the availability of the primary minerals especially phosphorous (P) (Kumar et al., 2023). The management of P in soil is one the most important aspects of controlling soil nutrients under salinity. Agriculture is restricted by intrinsic barriers in areas with salty soil. Due to the significant reduction in P availability caused by salinity, crops are at danger of having insufficient P. Despite the fact that the organic P pool in soil is generally considered inactive and not immediately accessible, it can be a source of available P through mineralization, which is aided by phosphatase (Khosropour et al., 2023). Consequently, by creating methods to control soil P availability and activate refractory P in soils, the requirement for phosphate rocks, a finite and non-renewable resource required for the production of chemical P fertilizers, may be reduced (Alamzeb and Inamullah, 2022; Li et al., 2023). The most affordable and ecologically friendly method to achieve this goal has been suggested to be biological methods that use microorganisms as P activators.
Bacteria are essential to the worldwide phosphorus cycle process, particularly when it comes to the conversion of organic to inorganic P in soils. A significant factor in the lack of fertility in linked soils is the reduced microbial activity brought on by salt stress. Functional bacteria called phosphorus-solubilizing bacteria (PSB) are involved in the cycling of phosphorus in soil (Liu et al., 2023; Rahimi Chegeni et al., 2023). PSB are beneficial bacteria that can remove inorganic P from difficult-to-digest materials. The rhizosphere bacteria’s ability to solubilize P is one of the most important traits connected to plant phosphate feeding. It is commonly accepted that low molecular weight organic acids, whose hydroxyl and carboxyl groups chelate the phosphate-bound cations to make them soluble forms, are released during the process by which PSB strains solubilize mineral phosphate. PSB were being employed as bio-fertilizer. By mineralizing organic P and resolving precipitated P, they significantly increase the amount of P that is available to plants in the soil (Imran and Amanullah, 2023; Sun et al., 2024). PSB excrete protons, organic and mineral acids, phenolic compounds, and protons into the soil, which causes soil acidification. In addition, the use of biochar (BC) as soil amendment are eminent in improving soil and plant under abiotic stress. BC exceptional porosity and high specific surface area give it an excellent capacity for retaining nutrients and water, which is helpful for microbe adhesion and reproduction. The P-enriched BC is an innovative method that can be useful in slowing down the influx of P into the soil (Chen et al., 2023). As a result, BC that has been enhanced with P can enhance not only the physical and chemical properties of the soil but also the availability of P to the soil’s microorganisms, which in turn enhances the availability of P to plants (Khosropour et al., 2022; Lai et al., 2022).
Sunflower (Helianthus annuus L.) is an annual plant with a straight stem, rough and 2 meters high, which is grown as an ornament or for the use of its oil seeds. Its beneficial components include the fruit’s oily interior, the flower, and even the stem. Sunflower is rich in vitamins A and B and is very useful for the liver (Fatemi et al., 2022; Hadidi et al., 2024). The kernel of its seeds contains 40 to 48% oil, which is very pleasant and useful. Sunflower is a tonic for the heart, a solvent, a polisher, an opener of nasal and liver blockages, and beneficial for hemorrhoids, lung ulcers, brain and nerve colds, and a stomach tonic (Singh et al., 2022). Sunflowers are a significant source of vegetable oil globally, with global sunflower seed production reaching 56-60 million metric tons annually. The global cultivation area is estimated at 27-28 million hectares, with fluctuations due to global demand and geopolitical impacts (FAO, 2022; Haliloğlu and Aydogdu, 2024). Sunflower oil ranks as the fourth most significant vegetable oil, following palm, soybean, and rapeseed oils. Iran produces 50000-70000 metric tons of sunflower seeds annually, covering 60000-70000 ha. However, Iran still imports a significant portion of sunflower oil, importing over 400000 tons annually (Iranian Ministry of Agriculture Jihad, 2023). Sunflower is a focus for agricultural expansion to reduce dependency and increase productivity (Hadidi et al., 2024).
Although the negative role of salinity on crop yield have been previously well addressed (Rasool et al., 2022; Damalas and Koutroubas, 2022; Dastorani et al., 2022), the use of PSB by P availability is little. Rahimi Chegeni et al. (2023) demonstrated the beneficial effects of PSB strains in the reduction of salt stress by altering the physiological and biochemical alterations in chickpea plants. Chen et al. (2023) showed that a pig manure BC have a noticeable role in controlling heavy metals so that high extracellular electron transfer rate of microorganisms was accelerated by the good electrical conductivity and organic material adsorption of BC, which further sped up the mineralization and immobilization of Pb2+ and Cd2+ to assure the repair effect of PSB on heavy metals. However, there is no available document on the effect of PSB along with P-enriched BC in modulating salinity on crops particularly sunflower. The research question is on determining whether or not PSB and P-enriched BC are beneficial in modulating salinity via improving the yield of sunflower lines. The objectives of this work are to ascertain the yield, physiological, and biochemical characteristics of three lines of sunflower under salt stress in the presence of PSB and P-enriched BC. The hypothesis is PSB and P-enriched BC used together will notably reduce the detrimental effects of salt stress on sunflower production and growth. PSB and P-enriched BC will contribute to improved nutrient absorption, increased physiological resilience, and improved biochemical performance in sunflower plants in saline circumstances by increasing P availability and improving soil structure. This will improve yield and overall plant health.
2 Materials and methods
2.1 Plan materials, growth conditions, and treatments
Three lines of sunflower, namely RGK38, BGK35, and BGK259 were provided by Administrative Agricultural Research, Education and Extension Organization (AREEO), Tehran, Iran and cultivated during tow growing seasons of 2021-2022. Among the 38 sunflower lines, the three lines RGK38, BGK35, and BGK259 were the main lines selected, showing sensitivity, moderately sensitive, and tolerant to salinity, respectively, in previous tests based on germination and physiological attributes by the authors. Each line represents a candidate for the sensitive, moderately sensitive, and tolerant groups. Therefore, in this experiment, their response to PSB and P-enriched BC under salinity conditions was evaluated. The experiment was designed in a split plot factorial based on a completely randomized block design (CRBD) with five replicates in Isfahan, Iran (1597 m asl., 32° 87´ N and 51° 58´ E). The field soil was a sandy loam with pH: 7.1, and EC: 0.96 dS m-1. N: 0.27%, P: 11.7 mg kg-1; K: 237 mg kg-1. The mean annual temperatures in the first and second years were 15.5 and 15.7°C, respectively. For the first and second years, the annual precipitation was 202 and 199 mm, respectively.
The main plot was salinity stress at 100 mM NaCl; the sub plot was a factorial of inoculation with PSB (Bacillus subtilis) and P-enriched BC of sunflower lines (RGK38, BGK35, and BGK259). Totally, 18 treatments were used in the present study. The seeds were sown on April 4th and the plants were harvested on July 15th. Each plot contained 18 m2, with 25 cm distance between plants and 60 cm row spacing. According to field capacity (FC), the irrigation regime was obtained and the salinity was applied as irrigation water at the stem elongation stage for 40 days.
2.2 Creating suspensions of the studied bacteria
The PSB were provided by Water and Soil institute, Karaj, Iran. Liu et al. (2018) reported that Bacillus subtilis is a type of PSB. Seeds were sterilized with 1.5% sodium hypochlorite for 5 min, then successively washed in sterile water to remove any remaining disinfectant residue. 109 CFU mL-1 of bacteria were introduced into sterilized seeds for 30 min. It was 2.5 kg of inoculant per ha. Uninoculated seeds (control) steeped in LB broth for the same duration. To evaluate the impact of the bacterial treatment, the uninoculated control seeds were steeped in LB broth for the same amount of time. Samples were taken for leaf analysis shortly before the last harvest in order to evaluate important physiological and biochemical characteristics that were impacted by the bacterial infection.
2.3 The preparation of P-enriched BC
The grape residue was used to produce BC through a multi-step process. First, after harvesting, the grape residue was crushed, thoroughly cleaned, and dried in an oven at 75°C for 24 h. The dried residue then underwent pyrolysis, a process where it was heated in an electric furnace at 350°C, with the temperature rising at a rate of 9°C per min for a total of 120 min. After pyrolysis, the furnace was cooled naturally by exchanging heat with the outside environment. To create P-enriched BC, Di-ammonium phosphate ((NH4)2HPO4) was used. The grape-based BC was mixed with Di-ammonium phosphate in a 1:1 ratio. The mixture was shaken continuously for two h to ensure thorough and uniform distribution of P across the surface of the BC. This step is crucial because the even coating of P onto the BC enhances its ability to release the nutrient gradually into the soil, improving its effectiveness as a soil amendment. After the mixing process, the BC was subjected to a drying phase at 75°C for 24 h. This drying step helps remove any excess moisture and further stabilizes the BC, completing the preparation of the P-enriched BC for use in agricultural applications (Chia et al., 2014). 1500 kg of P-enriched BC was applied per ha in this experiment. The soil and BC were properly combined to guarantee uniform dispersion and efficient contact with the plant roots. This was accomplished by evenly distributing the BC across the top soil layer prior to planting, which allowed it to interact with the soil matrix and enhance microbial activity, water retention, and nutrient availability—especially in salty environments.
2.4 Grain yield, thousand grain weight
Grain separation from the inflorescence was followed by a rigorous drying process to guarantee precise weight measurement. Next, in order to give a standardized yield assessment, the total grain yield was computed and documented in terms of grain weight per ha. Additionally, a digital scale was used to precisely measure the TGW, which is a measurement of the average weight of a thousand grains.
2.5 Oil content and oil yield
A dried sample of sunflower seeds was crushed using a commercial blender after seed contaminants were removed, and the seeds were then submitted to cold pressing and Soxhlet extraction. The well-crushed seed samples were put in a paper thimble and fed into a Soxhlet extractor, which was equipped with a 500 mL round bottom flask and a condenser, for the Soxhelt procedure. N-hexane was used as the extraction solvent during the 8-h process to remove the oil. Following extraction, the surplus hexane was vacuum-distilled off at 45°C in a rotating evaporator. Oil was removed, weighed, and the yield was calculated (de Aquino et al., 2019). The oil yield was obtained from grain yield and oil content.
2.6 Relative water content measurement
Three steps were utilized to measure RWC from developed leaves. After determining the fresh weight (FW), they were submerged in distilled water for 24 h to reach their saturation weight (SW). After drying in an oven until the weight remained constant, the samples’ dry weight (DW) was calculated (Khosropour et al., 2022):
2.7 Chlorophyll assay
The Arnon (1949) instruction was followed to determine the amount of leaf Chl. To do this, 8 mL of 80% acetone were used to homogenize 0.2 g of fresh leaves. At 4°C, the mixture was centrifuged for a quarter. A spectrophotometer read the supernatant at 645 and 663 nm.
where A is the absorbance at each wavelength. The final extract volume is V, and the fresh weight in gr is W.
2.8 Malondialdehyde measurement
To calculate leaf MDA content of sunflower lines, 0.5 g of fresh leaves and 4 mL of 20% w/v trichloroacetic acid (TCA) with 0.5% thiobarbituric acid were combined. The mixture was centrifuged for 30 min at 14,000 rpm after 25 min in a hot water bath (95°C). The samples were centrifuged at 10,000 rpm for 10 min after being submerged in a hot water bath for 30 min. The samples were read at a wavelength of 532 nm (Heath and Packer, 1968).
2.9 Electrolyte leakage
The 0.5 cm segments of chickpea leaves were washed with deionized water, and placed in tubes containing 10 mL of deionized water to recoded the electrical conductivity (EC1) after 24 h. In order to discover EC2, the samples were autoclaved at 120°C for 15 min. The EL was calculated as follows: EL (%) = EC1/EC2 × 100 (Lutts et al., 1995).
2.10 Measurement of ion content
Dried leaf and root samples weighing exactly 100 mg each were digested with 2 mL of strong nitric acid. In order to liberate the mineral ions for precise measurement, the plant tissues were broken down using this procedure. After digestion, the samples’ potassium (K+) and sodium (Na+) concentrations were measured using a flame photometer (Model Jenway PFP7, UK) in accordance with Munns et al. (2010) approach. This technique works well for identifying and measuring alkali metals because of the emission characteristics seen during the flame photometry procedure. Furthermore, spectrophotometry was used to determine the samples’ P level at 420 nm using molybdenum blue technique (Burns and Hutsby, 1986). P concentration in plant tissues may be precisely measured using this colorimetric approach, which creates a blue complex when phosphate and molybdate react in an acidic solution.
2.11 Data analysis
SAS software was used to analyze the data after they had been prepared. Mean values were compared by LSD at 5% probability level. The XLSTAT was utilized for principal component analysis (PCA) and agglomerative hierarchical clustering (AHC). The website cimminer created the heat map.
3 Results
3.1 Grain yield and thousand grain weight
Grain yield in all sunflower lines were significantly (P ≤ 0.01) lower in saline soils. However, PSB and Particularly its combined with P enriched BC led to noticeable increases in grain yield. In RGK38, BGK35, and BGK259 without PSB and BC, salinity deceased grain yield by 25, 22, and 20%, respectively. In saline soils, the use of integrated PSB and BC increased grain yield by 16%, 11, and 9% compared to non-PSB and BC for RGK38, BGK35, and BGK259, respectively. The highest grain yield was recorded in sunflower RGK38 that had been inoculated with PBS+BC without salinity, with a 27% improvement over the control. The lowest value was recorded in RGK38 that had been salinized without PSB and BC, with a 25% reduction over the control. Furthermore, the three plant lines showed different reactions to PBS and BC treatments. In particular, under saline conditions, the combined application of PBS and BC resulted in enhanced grain yield of the lines RGK38, BGK35, and BGK259, with increases of 9%, 10%, and 16%, respectively (Figure 1A). Salinity caused a decreases in TGW for the sunflower lines. It ranged from 36.6 g in RGK38 without PSB and BC under salinity stress to 42.3 g in BGK259 inoculated with PBS+BC without salinity. The maximum value showed 15% increase in TGW relative to its minimum amount (Figure 1B).
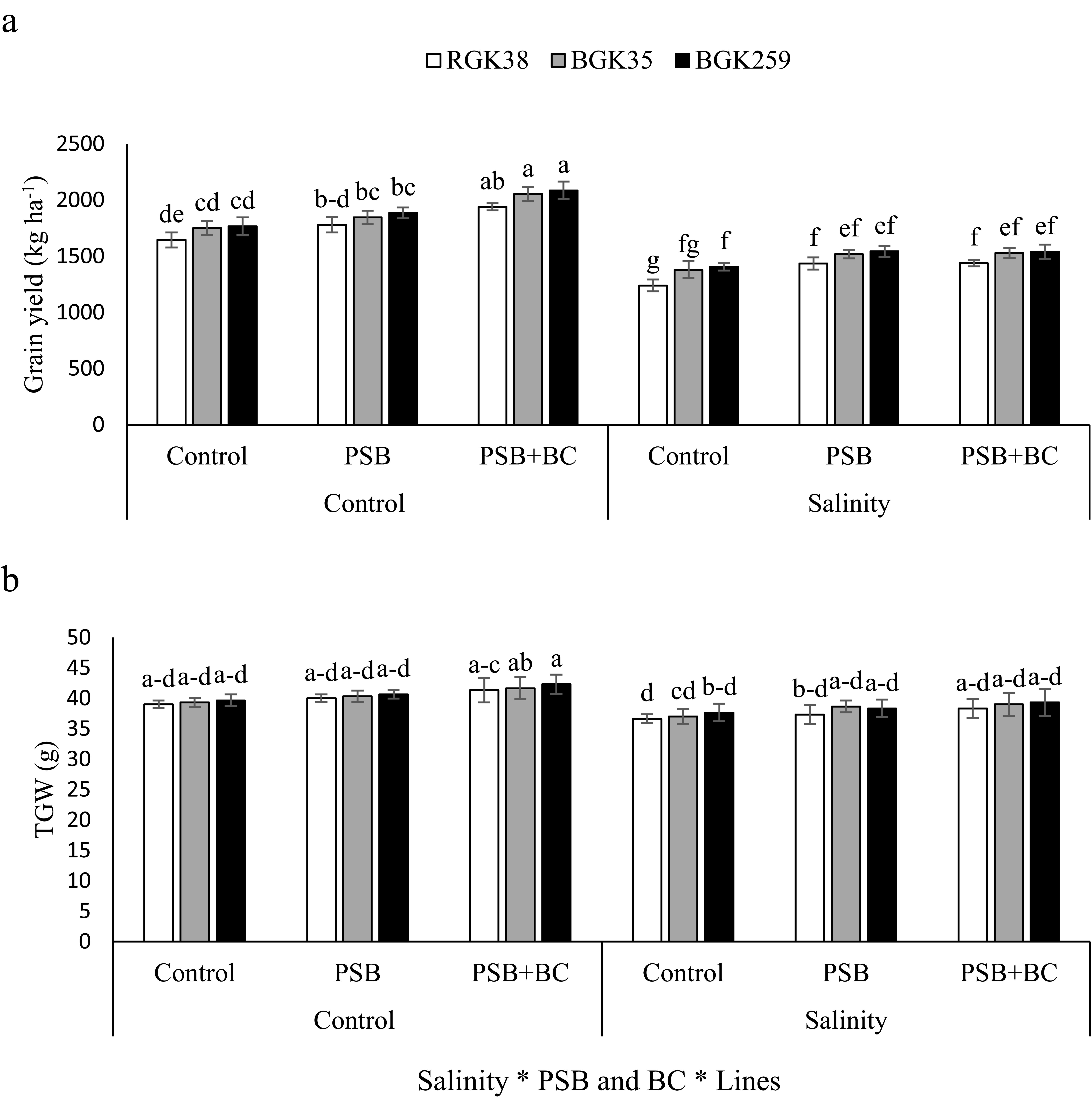
Figure 1. Grain yield (A) and thousand grain weight (TGW, B) of sunflowers lines under phosphate solubilizing bacteria (PSB) and P-enriched biochar (BC) in saline soil. The columns with similar letters have not significant difference at 5% probability level based on LSD test.
3.2 Oil content and oil yield
Oil content and oil yield deceased by salinity (P ≤ 0.05), although PSB and BC represented no significant differences (P>0.05). The maximum oil content (44.4%) was observed in BGK35 treated by PSB+BC without salinity with 10% increase compared to the control (Figure 2A). Additionally, salt stress reduced oil yield, whereas PSB and BC boosted it. Salinity resulted in decreases in oil yield of 31, 27, and 24% for RGK38, BGK35, and BGK259, respectively, when compared to the control (without salinity, PSB and BC). The use of PSB and PSB+BC enhanced oil yield for sunflower RGK38 under salt stress by 19 and 27%, respectively, in comparison to the non-PSB+BC treatment (Figure 2B).
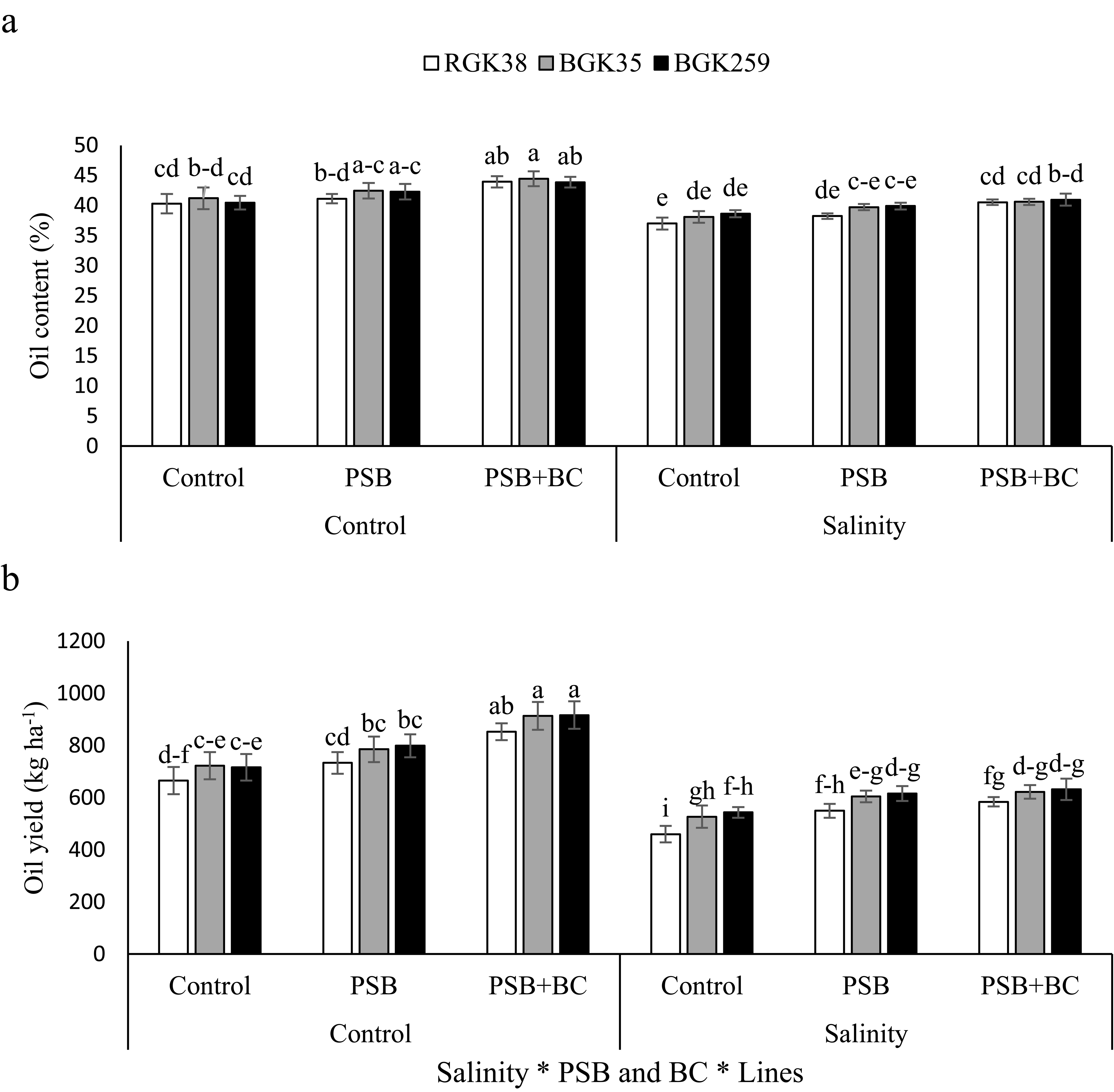
Figure 2. Oil content (A) and oil yield (B) of sunflowers lines under phosphate solubilizing bacteria (PSB) and P-enriched biochar (BC) in saline soil. The columns with similar letters have not significant difference at 5% probability level based on LSD test.
3.3 Relative water content and Chlorophyll content
Salinity caused a considerable drop in RWC and Chl content (P ≤ 0.05). Salinity reduced RWC by 23, 18, and 19% in lines I, BGK35, and III, respectively, in comparison to non-saline circumstances. In comparison to non-PSB and BC, the use of PSB and PSB+BC enhanced RWC of RGK38 by 10 and 14%, respectively, under salt stress (Figure 3A). Similar to RWC, Chl exhibited a reduction with salinity. In RGK38 plants without inoculation of PSB and BC, salt stress led to notable declines of Chl a+b by 41, 31, and 28%, respectively, as compared to non-saline conditions. The combined PSB and BC had a positive role in improving plant growth through improving Chl content. For instance, 12% improvement of Chl content was obtained for BGK259 plants when they were treated by PSB+BC under salt stress (Figure 3B).
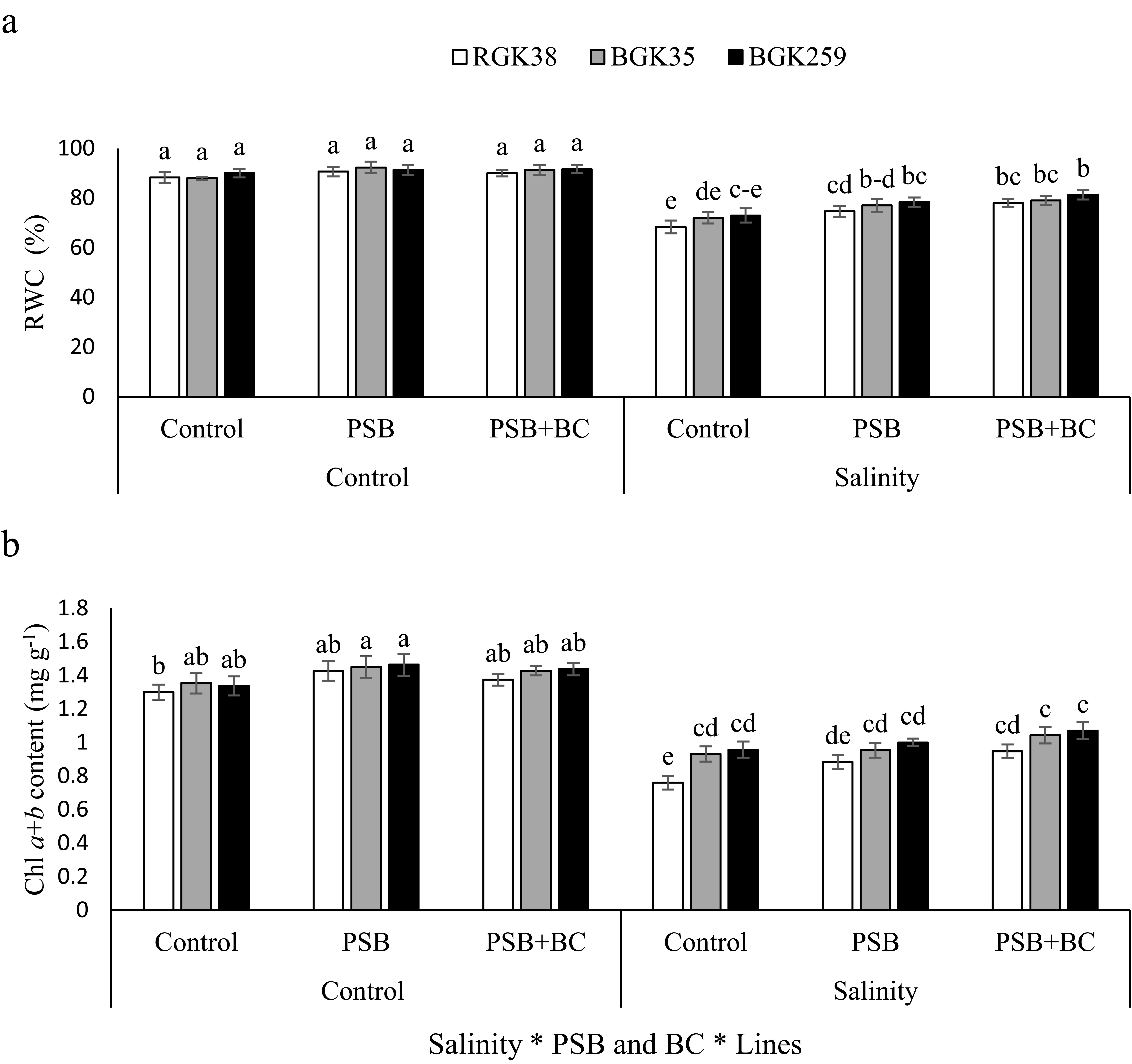
Figure 3. Relative water content (RWC, A) and chlorophyll (Chl) a+b (B) of sunflowers lines under phosphate solubilizing bacteria (PSB) and P-enriched biochar (BC) in saline soil. The columns with similar letters have not significant difference at 5% probability level based on LSD test.
3.4 Malondialdehyde and electrolyte leakage
By increasing MDA accumulation and EL, salinity stress had a detrimental effect on lipid peroxidation, although PSB and BC reduced the parameters (P ≤ 0.05). Salinity raised MDA in RGK38, BGK35, and BGK259 by 65, 43, and 38%, respectively, in the absence of PSB and BC (Figure 4A). In line RGK38 under salt stress, the use of PSB and PSB+BC lowered MDA by 10 and 26%, respectively, relative to the control (Figure 4A). The charges in EL by salinity was shown by 21, 21, and 14% increases for RGK38, BGK35, and BGK259, respectively, relative to the non-saline conditions without PSB application (Figure 4B).
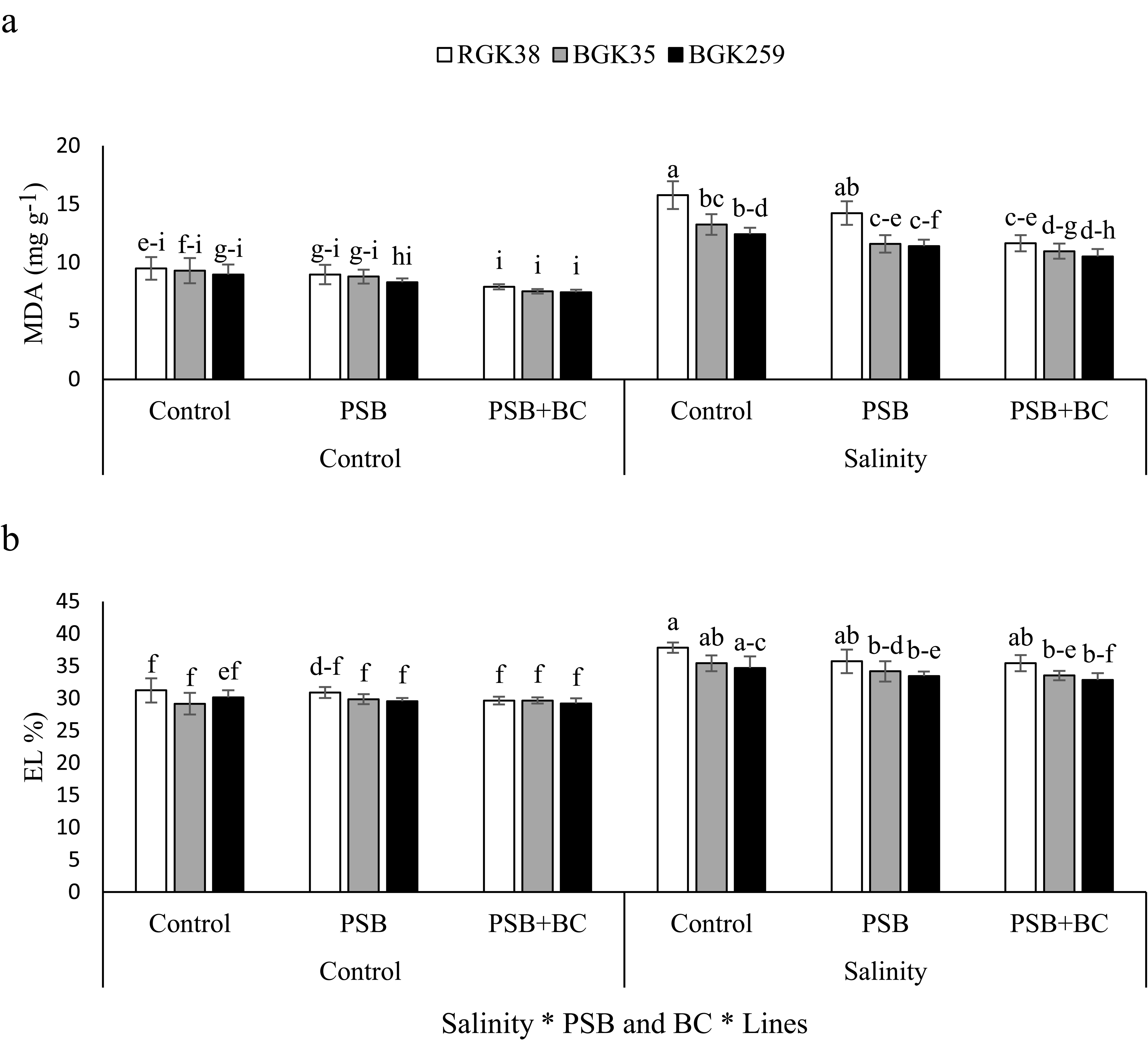
Figure 4. Malondialdehyde (MDA, A) and electrolyte leakage (EL, B) of sunflowers lines under phosphate solubilizing bacteria (PSB) and P-enriched biochar (BC) in saline soil. The columns with similar letters have not significant difference at 5% probability level based on LSD test.
3.5 Minerals
Leaf K significantly decreased by salinity but PSB and BC increased it (P ≤ 0.05). The noticeable role of PSB and BC on modulating salinity through improving K and P were observed. Salinity deceased K and P by 26% and 65%, respectively, in RGK38 without PSB and BC. In these plants, the integrated PSB and BC increased K and P by 22 and 74%, respectively, under salinity stress. Na and Cl noticeably increased by salinity but PSB and BC lowered its accumulation. For example, for RGK38, salinity increased Na and Cl by 6.3 and 4.2-fold relative to the non-saline condition. The role of PSB and BC was eminent in modulating salinity stress by inhibiting Na and Cl accumulation in leaf tissues. In BGK259 plants under salinity, the accumulation of Na and Cl significantly decreased by 34 and 46%, respectively, when compared to the non-PSB and BC application (Table 1).
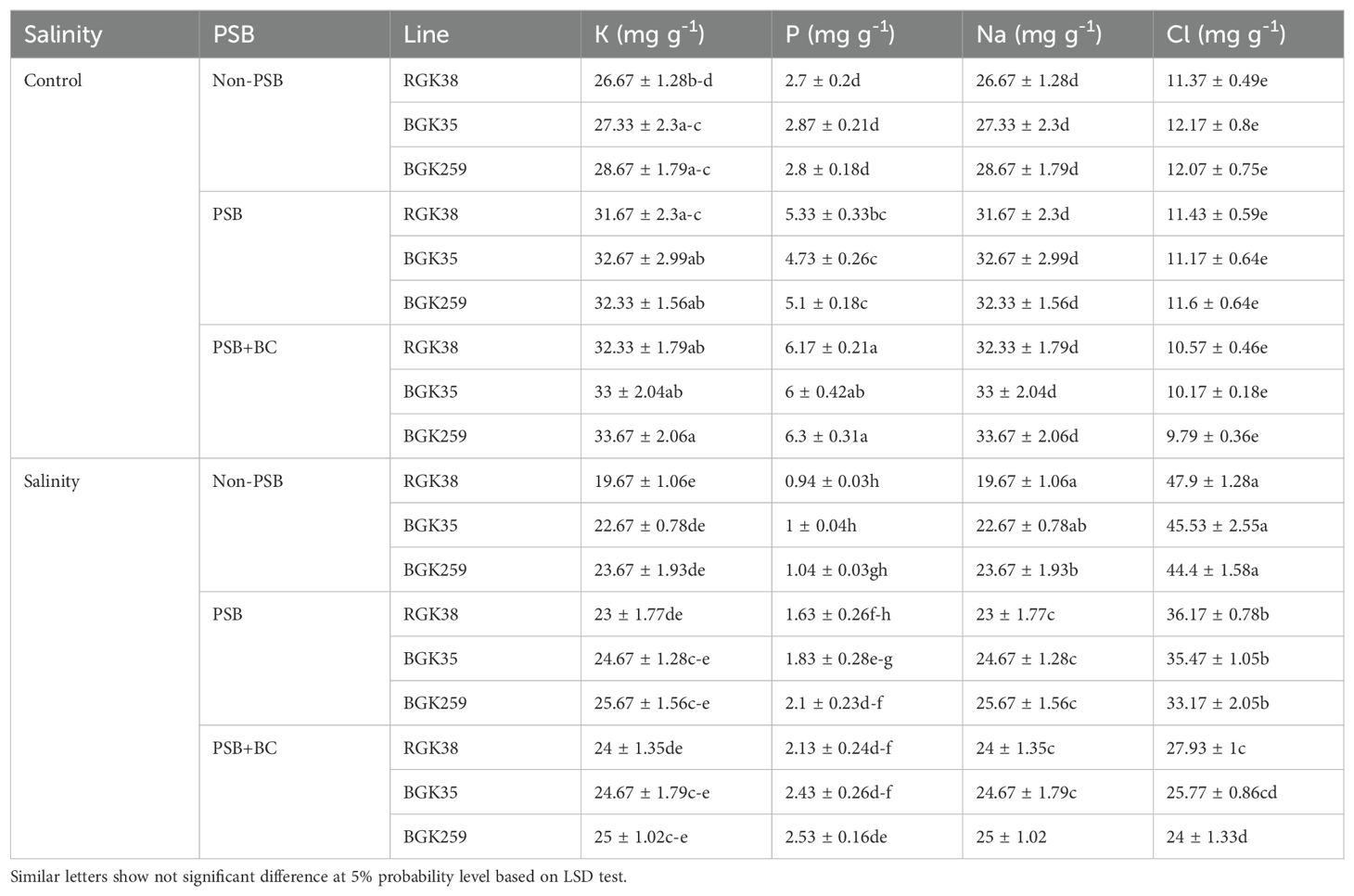
Table 1. Leaf minerals of sunflowers lines under phosphate solubilizing bacteria (PSB) and P-enriched biochar (BC) in saline soil.
3.6 Principal component analysis and heat map
Based on principle PCA, the first principle component (F1) accounted for 93.7% of the total variance, explaining most of the dataset’s variability. The fact that this component largely supported every feature that was assessed shows how important it is in understanding how sunflower lines responded to the experimental setup. The variability shown in lines RGK33 and BGK259 for the sunflower lines under investigation was particularly linked with F1, suggesting that these lines had comparable responses that are reflected by this primary component. Line BGK35, on the other hand, displayed variability via the second main component (F2), indicating different characteristic patterns between this line and RGK33 and BGK259 (Figure 5).
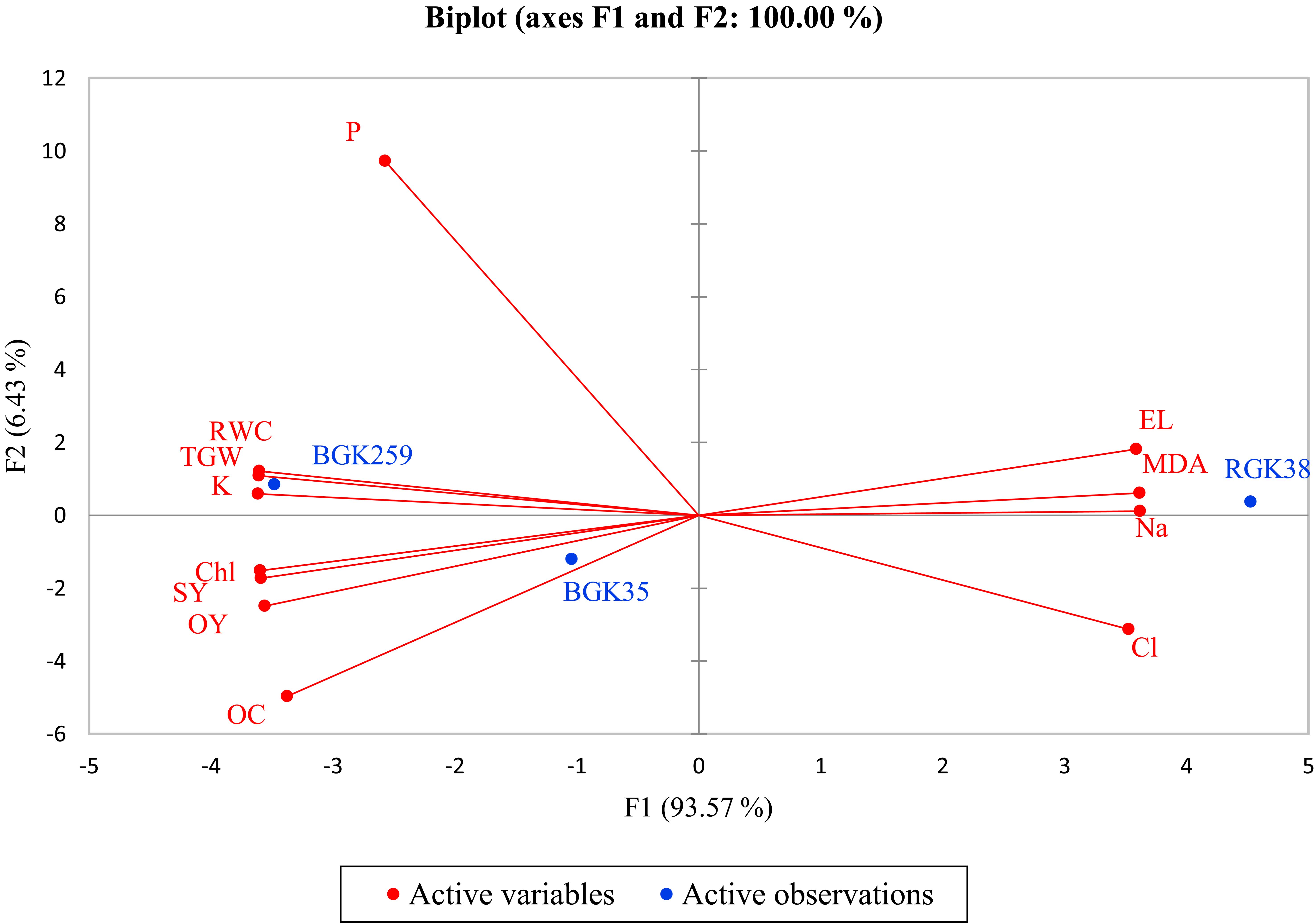
Figure 5. Principal compost analysis (PCA) for traits of sunflowers lines under phosphate solubilizing bacteria (PSB) and P-enriched biochar (BC) in saline soil. RWC, relative water content; OC, oil content; OY, oil yield; SY, Seed yield; Chl, Chlorophyll; TGW, thousand grain yield; MDA, malondialdehyde.
According to heat map, the highest and lowest weights belonged to red and blue, respectively. The analysis showed that sunflower line RGK38 was placed in a separate cluster, indicating distinct characteristics or responses. In contrast, lines BGK35 and BGK259 grouped together in the same cluster, suggesting similarities in their traits or responses under the treatment conditions. Among the traits, MDA, EL, Na+ and Cl- as a separated cluster showed the high variability under the treatments. However, the class containing RWC, Oil content and TGW demonstrated the minimum variability (Figure 6).
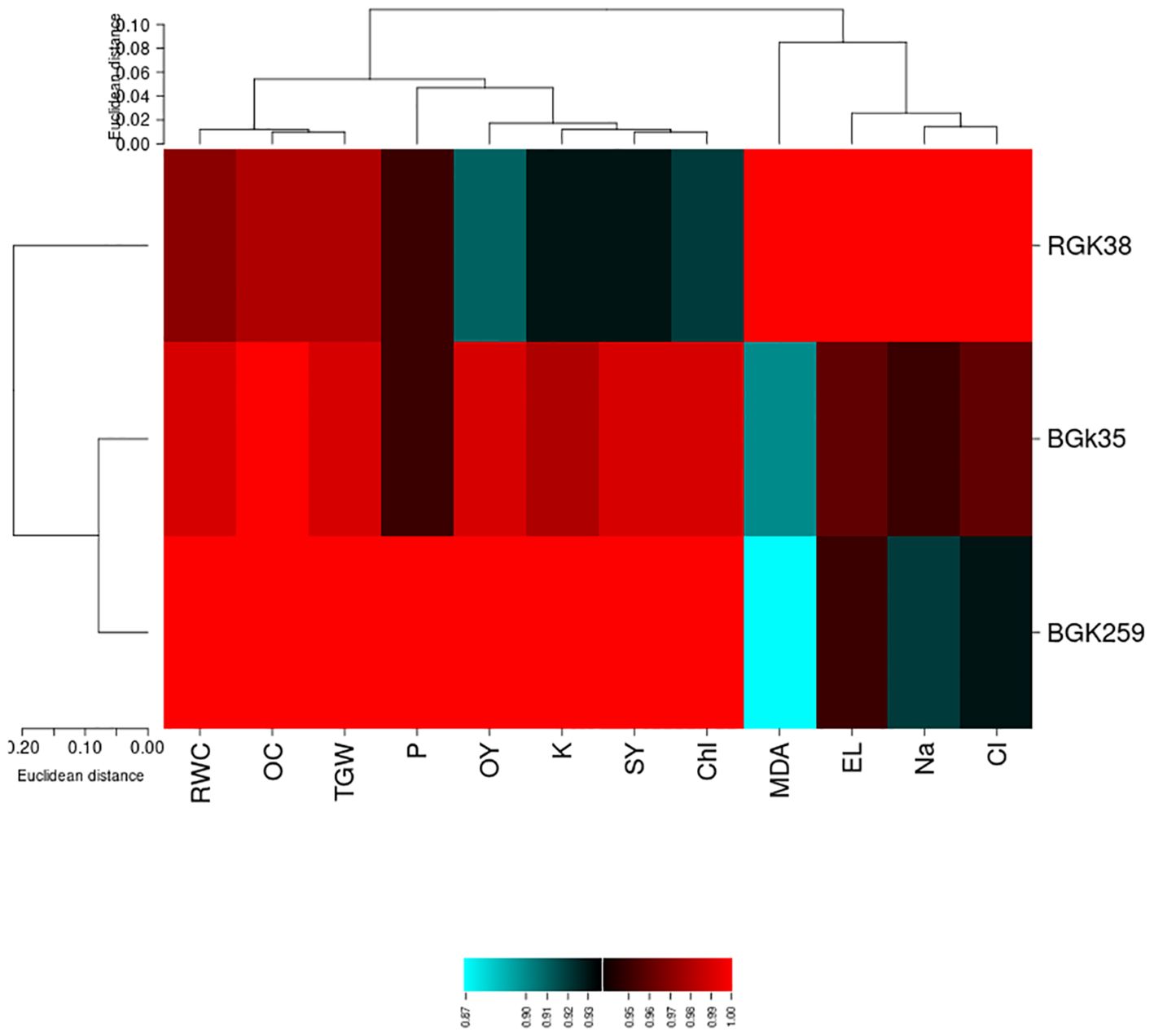
Figure 6. Heat map analysis for traits of sunflowers lines under phosphate solubilizing bacteria (PSB) and P-enriched biochar (BC) in saline soil. RWC, relative water content; OC, oil content; OY, oil yield; SY, Seed yield; Chl, Chlorophyll; TGW, thousand grain yield; MDA, malondialdehyde.
4 Discussion
Grain yield and TGW decreased as a result of salinity. This study found that sunflower lines with varying responses to salinity showed distinct differences in grain yield and TGW under saline conditions. The salt-tolerant line (BGK259) maintained higher yields and TGW, while the sensitive line (RGK38) experienced sharper declines. These differences highlight the genetic differences among lines and their ability to withstand salinity-induced stress on reproductive development and energy allocation (Ghadirnezhad Shiade et al., 2023). Salinity significantly reduces grain yield in sunflower lines, with sensitive lines showing a more significant reduction. This is due to several physiological and biochemical mechanisms. Firstly, salt creates an osmotic imbalance, making it difficult for plants to take up water. This leads to restricted cell expansion, stunted growth, and lower biomass production. Secondly, salt-sensitive lines lack robust osmotic adjustment mechanisms, resulting in a more substantial decline in growth (Ehtaiwesh et al., 2024). Thirdly, salinity stress negatively affects photosynthetic capacity, limiting energy available for grain production. This results in a drastic decrease in assimilate production, leading to fewer resources allocated to grain filling and a sharper decline in grain yield. Fourthly, salinity induces oxidative stress, producing ROS that can damage cellular structures. These physiological limitations amplify the impact of salinity on sensitive lines, leading to a more pronounced yield loss compared to salt-tolerant lines (Choudhary et al., 2023; Ehtaiwesh et al., 2024). Although salt-related significant reductions in grain yield have traditionally been viewed on different plants (Han et al., 2022; Dastorani et al., 2022; Zheng et al., 2023; Masrahi et al., 2023), little is known about how PSB and BC affect salinity and drought through sunflower grain yield. According to Waqar et al. (2022) and Aziz et al. (2023), BC can increase sunflower performance in the face of environmental difficulties. Furthermore, Hassan et al. (2021) documented the beneficial impact of PSB on sunflower yield. In this study, plant weight significantly rose after PSB and BC. It could be related to PSB metabolism and the production of a variety of plant hormones, acids, and vitamins. When P-enriched BC was present, the PSB had a positive impact on growth parameters. Similar findings were made by Adnan et al. (2020) on maize and Masrahi et al. (2023) on barely, who found that the PSB considerably increased the grain yield specially in the present of P. PSB improves developmental traits, which helps to control salt stress when P is present. Plant yield rose dramatically as a result of the usage of microbial inoculants. This may be explained by effective nutrient mobilization from the inaccessible form to the accessible form (Sun et al., 2024). These bacteria could improve nutrient uptake for plant growth by producing physiologically active compounds The higher plant growth might have been caused by the inoculants’ increased N-fixing ability, P-solubilizing capacity, and growth hormone synthesis (Rahimi Chegeni et al., 2023).
Salinity led to deceased in oil content and oil yield. Salinity stress in sunflower lines leads to significant decreases in oil content and yield, with the sensitive line (RGK38) experiencing more significant reductions. This is due to differences in lipid metabolism, carbon assimilation, ion toxicity, seed development, and oxidative stress. Salt-tolerant lines maintain higher oil content due to stable lipid metabolism, while sensitive lines experience more disruption in these processes. Osmotic stress affects seed development more severely in sensitive lines, leading to smaller seeds and less oil accumulation. The tolerant line maintains higher oil yield under salinity, while sensitive lines experience declines (Abd El-Hameid and Sadak, 2020). By modulating the cellular redox state, PSB+BC influences the functioning as a signal transduction route, enhancing Chl production and the photosynthetic processes or inhibiting chlorophyll breakdown and incorporated into primary metabolism. the agricultural yield rose as a result (Alam et al., 2022; Masrahi et al., 2023). It is important to note the impact of PSB+BC treatments on growth regulators such IAA, which appears to promote growth characteristics and results in an increase in oil yield. These increases might be the result of physiological mechanisms including growth regulators and antioxidants, as well as increased assimilate levels and assimilate translocations from leaves to seeds (Rawat et al., 2022). Our findings are in line with recent research that have seen a noticeable rise in grain yield of rice (Rawat et al., 2022) and barely (Masrahi et al., 2023).
Salinity decreased Chl content, whereas it was improved by PSB in the presence of BC. The study revealed that salinity stress causes a decrease in Chl content in three sunflower lines. This degradation affects the plant’s photosynthetic capacity, which is crucial for light absorption and energy capture. Salt-tolerant lines regulate Na+ uptake and compartmentalize excess ions, while sensitive lines experience higher Na+ accumulation, leading to more severe Chl degradation and photosynthetic damage (Emami Bistgani et al., 2023). Salinity stress induces ROS, causing oxidative damage to Chl molecules and cellular components. Tolerant lines have stronger antioxidant defenses, neutralizing ROS more effectively. Chl reduction under salinity stress negatively impacts plant growth and biomass production (Fitzner et al., 2023). Similarly, Rahimi Chegeni et al. (2023) showed that salinity deceased total Chl content of chickpea plants by 32%, whereas two strains of PSB (Bacillus amyloliquefaciens and Bacillus pumilus) noticeably modulated salinity stress through increasing Chl content in the present of P. They claimed that PSB had a significant capacity for supplying the vital nutrients and water required for photosynthesis.
Salty irrigation significantly decreased RWC, a vital physiological property, possibly due to salts disrupting turgor and water uptake, indicating significant variation in RWC under salinity (Seymen et al., 2023). A lower RWC under salinity stress is a sign that salt is negatively affecting the water balance of the plant. By decreasing the amount of water available in the root zone, salinity stress makes it more difficult for plants to absorb water, which leads to osmotic stress. Plants find it more difficult to stay properly hydrated when soil salt levels rise, which lowers RWC. The buildup of Na+ and Cl- ions in plant tissues aggravates this impact by interfering with water absorption processes and disrupting cell turgor pressure. Reduced physiological functioning and damaged cell structure, which impact photosynthesis and nutrition transport, are indicated by lower RWC levels. Therefore, the drop in RWC is a definite sign of the plant’s diminished ability to sustain water homeostasis under salinity. Sofy et al. (2020) showed a noticeable reception of RWC due to higher Na accumulation in plants. In addition, Ansari et al. (2019) showed that alfalfa cultivars have different response in RWC to salinity stress. PSB and BC can improve RWC in plants under salinity stress by enhancing water retention, nutrient availability, and stress tolerance. PSB increases P availability, which enables plants to sustain greater RWC and access deeper water stores. Additionally, PSB generates stress-relieving substances that encourage root development and water absorption, including phytohormones and exopolysaccharides (Osman et al., 2022). The porous nature of BC improves the soil’s ability to retain water, reducing water loss and raising RWC. By adsorbing toxic salt ions, BC lowers osmotic stress and improves plant hydration and water uptake (Ababsa et al., 2023). By efficiently promoting water retention and nutrient balance, these systems lessen the negative impacts of salinity and assist plants in maintaining improved RWC. Similarly increases in RWC have been reported by PSB (Reza et al., 2021; Suhail et al., 2023) and BC (Ababsa et al., 2023).
Lipid peroxidation is an oxidative process of lipid destruction that increases ROS generation. Salt treatment increases ROS concentration and causes the lipid membrane to become peroxidized, which disrupts the membrane’s permeability and causes oxidative stress in plant tissues. In fact, MDA, a product of unsaturated fatty acids oxidation, is widely used as an indicator of oxidative stress (He and Ding, 2020). More oxidative stress markers, such as MDA, were created by sunflower plants, especially sensitive lines, when exposed to salt. This increased EL and affected membrane integrity. It demonstrates how different plants’ antioxidant capacities allow them to scavenge ROS by building up MDA (Sun et al., 2024). Similar to this, Ahsan et al. (2022) have examined how salt negatively impacts sunflower plants’ ability to accumulate more MDA. The PSB and P enriched BC, on the other hand, displayed decreased MDA and EL levels in saline conditions in the current study, which is novel for various sunflower lines. This may be because PSB, especially when combined with BC, regulates membrane activities via lowering ROS production. PSB and BC are essential for plant growth and metabolism by converting insoluble P into forms that plants can absorb. They enhance P availability by producing organic acids and phosphatase enzymes, which improve soil structure and nutrient retention (Cheng et al., 2024). BC improves soil structure and nutrient retention, making phosphorus more accessible to plants over time. Both treatments reduce oxidative stress markers like MDA. PSB produces compounds like siderophores and phytohormones to help plants tolerate stress (Yahya et al., 2021), while BC improves soil water retention and buffers against stress conditions (Wu et al., 2023). These results are also in line with those reported by Reza et al. (2021) and Rahimi Chegeni et al. (2023) who claimed that PSB plays a beneficial effect in regulating oxidative stress by reducing MDA and EL.
Salinity reduced K+ and P while raising Na+ and Cl-. Increasing Na+ under plant tissues causes the equilibrium of salt to be upset, which causes a number of problems with membranes, the inhibition of enzymes, and metabolic disturbances that change cell elongation, cell structure, and cell division. Additionally, salt accumulation obstructs the delivery of nutrients to the leaves and their availability. The two most prevalent soil components, Na+ and Cl, exert ionic and osmotic effects on plant cells. This causes a decrease in osmotic potential, which lowers the availability of water and minerals in root cells (Boshkovski et al., 2020). Salinity raised Na+/K+, and this high rate has negative consequences on plants, including the inactivation of most photosynthesis-related enzymes, which ultimately stunts plant growth. K+ and P are raised while Na+ and Cl- are lowered as a result of the increases in PSB and BC. Due to excessive Na+ inflow K+ outflow, salinity stress impairs plant metabolic and cellular activities and disrupts ionic equilibrium. In turn, this circumstance causes a large drop in cytosolic K+ levels, which ultimately inhibits plant development characteristics. By altering important plant processes, K+ is critical in reducing the effects of salt stress (Kumari et al., 2021). P is one of the essential minerals for the growth of plants. The typical soil P content is 0.05% (w/w); 0.1% of this can be taken by plants as H2PO4 by the amount of 0.1%. The plant’s capacity to use P is constrained by competition between H2PO4 and Cl- when salt stress is present. Under salinity, it prevents P from moving from the plant’s roots to its aerial portions, which slows down plant growth and development (Hajiabadi et al., 2021). Rabbani et al. (2023) previously found that K and P levels were lower while Na+ and Cl- levels were higher in hemp plants. It is widely known that PSB can make more P available to plants through a number of different methods (Dey et al., 2021). Similarly, the significant role of PSB in enhancing P under salinity stress have been reported on wheat plants (Hajiabadi et al., 2021). Additionally, the poticable improvement of leaf P content in chekpea plants have been reported by Rahimi Chegeni et al. (2023) in the presence of P fertilizer in the rhizosphere, which is in line with our results.
According to PCA, grain yield, oil production, and other physiological characteristics essential to plant productivity and health may be negatively correlated with increased levels of EL, MDA, Na+, and Cl-—often connected to stress reactions or cellular damage. Our results are supported by Zadegan et al., (2023) comparable negative connection between MDA and both grain yield and RWC in common beans, which suggests that MDA buildup under stress might affect productive attributes. The significance of maintaining low levels of MDA, EL, Na+, and Cl- to promote increased yield and physiological efficiency in crops is highlighted by this association, which also emphasizes the relevance of these features as possible markers of stress intensity. According to heat map, MDA and EL were among the examined variables that constituted a unique cluster with substantial variability under the experimental treatments. These variables may have served as stress indicators or adaptive responses in the research setting, as shown by their high variability, which also implies that they were more responsive to the treatment circumstances. However, there was little variation in a different set of characteristics, such as EO, TGW, and RWC. This stability suggests that these characteristics are less affected by treatment modifications, which might be because they are less sensitive to environmental influences or have greater genetic control. As seen in Figure 6, the grouping of these qualities demonstrates the variation in responsiveness among attributes and might be a reflection of underlying physiological or biochemical distinctions in response to the treatments. The heat map analysis of the differences across sunflower lines revealed that genetics play a significant impact in the plant’s response to salt, PSB, and BC. It is critical to select the right line of sunflowers, because BGK259 (the tolerant line) demonstrated the least amount of yield decline when exposed to salinity. Ghasemzadeh et al. (2022) and Rabbani et al. (2023) used heat maps to identify the sensitive features, which is similar to the method used in the current study.
5 Conclusions
Salinity stress poses a significant challenge to crop production in saline soils. However, the use of phosphate-solubilizing bacteria in combination with phosphorus-enriched biochar offers a promising solution. The research suggests that phosphate-solubilizing bacteria and phosphorus-enriched biochar can improve soil health and plant resilience in saline soils. These treatments enhance phosphorus availability, water retention, and reduce oxidative stress in plants, enabling crops to thrive in saline conditions. This not only enhances yield but also reduces the need for chemical fertilizers, lowering input costs and environmental impact. Implementing phosphate-solubilizing bacteria and biochar could increase crop productivity and profitability on lands previously constrained by high salinity, contributing to longer-term soil health. The sensitive line demonstrated the greatest reduction in grain yield under saline conditions. However, they also exhibited the most rapid response to treatments with phosphate-solubilizing bacteria and biochar. This finding suggests that selecting different lines based on their responsiveness to phosphate-solubilizing bacteria and biochar under salinity stress could be advantageous for improving grain yield stability in challenging environments. To mitigate the effects of salinity on sunflower production, farmers can benefit from using phosphate-solubilizing bacteria and biochar, each with specific application strategies. For phosphate-solubilizing bacteria, applying approximately 109 CFU per gram (around 2-3 kg of inoculant per hectare) at sowing as a seed coat or soil inoculant can enhance phosphorus availability, a nutrient crucial for plant resilience under salt stress. Biochar, applied at 1000-2000 kg per ha, improves soil structure and reduces salinity by binding excess sodium ions; it is most effective when integrated 2-4 weeks before planting, allowing it to condition the soil. This approach could support sustainable agriculture and food security in regions vulnerable to salinity stress, making it a valuable tool in the global effort to adapt to soil degradation and climate change.
Data availability statement
The original contributions presented in the study are included in the article/supplementary material. Further inquiries can be directed to the corresponding author/s.
Author contributions
SJ: Formal analysis, Methodology, Validation, Writing – original draft. PM: Formal analysis, Supervision, Writing – review & editing. MG: Formal analysis, Software, Writing – review & editing. HM: Formal analysis, Writing – review & editing. BA: Formal analysis, Writing – review & editing.
Funding
The author(s) declare that no financial support was received for the research, authorship, and/or publication of this article.
Conflict of interest
The authors declare that the research was conducted in the absence of any commercial or financial relationships that could be construed as a potential conflict of interest.
Publisher’s note
All claims expressed in this article are solely those of the authors and do not necessarily represent those of their affiliated organizations, or those of the publisher, the editors and the reviewers. Any product that may be evaluated in this article, or claim that may be made by its manufacturer, is not guaranteed or endorsed by the publisher.
References
Ababsa N., Boudjabi S., Chenchouni H. (2023). Biochar amendments changed soil properties and improved cereal crop growth under salt stress. J. Soil Sci. Plant Nutr. 23, 4912–4925. doi: 10.1007/s42729-023-01453-7
Abd El-Hameid A. R., Sadak M. S. (2020). Impact of glutathione on enhancing sunflower growth and biochemical aspects and yield to alleviate salinity stress. Biocatal. Agric. Biotechnol. 29, 101744. doi: 10.1016/j.bcab.2020.101744
Abedi F., Amirian-Chakan A., Faraji M., Taghizadeh-Mehrjardi R., Kerry R., Razmjoue D., et al. (2021). Salt dome related soil salinity in southern Iran: Prediction and mapping with averaging machine learning models. Land Degradation Dev. 32, 1540–1554. doi: 10.1002/ldr.3811
Adnan M., Fahad S., Zamin M., Shah S., Mian I. A., Danish S., et al. (2020). Coupling phosphate-solubilizing bacteria with phosphorus supplements improve maize phosphorus acquisition and growth under lime induced salinity stress. Plants 9, 900. doi: 10.3390/plants9070900
Ahsan M., Zulfiqar H., Farooq M. A., Ali S., Tufail A., Kanwal S., et al. (2022). Strigolactone (GR24) Application positively regulates photosynthetic attributes, stress-related metabolites and antioxidant enzymatic activities of ornamental sunflower (Helianthus annuus cv. Vincent’s Choice) under salinity stress. Agriculture 13, 50. doi: 10.3390/agriculture13010050
Alam F., Khan A., Fahad S., Nawaz S., Ahmed N., Ali M. A., et al. (2022). Phosphate solubilizing bacteria optimize wheat yield in mineral phosphorus applied alkaline soil. J. Saudi Soc Agric. Sci. 21, 339–348. doi: 10.1016/j.jssas.2021.10.007
Alamzeb M., Inamullah D. (2022). Management of phosphorus sources in combination with rhizobium and phosphate solubilizing bacteria improve nodulation, yield and phosphorus uptake in chickpea. Gesun. Pflan. 75, 549–564. doi: 10.1007/s10343-022-00722-2
Ansari M., Shekari F., Mohammadi M. H., Juhos K., Végvári G., Biró B. (2019). Salt-tolerant plant growth-promoting bacteria enhanced salinity tolerance of salt-tolerant alfalfa (Medicago sativa L.) cultivars at high salinity. Acta Physiol. Plant 41, 1–13. doi: 10.1007/s11738-019-2988-5
Arnon D. I. (1949). Copper enzymes in isolated chloroplasts. Polyphenoloxidase in Beta vulgaris. Plant Physiol. 24, 1. doi: 10.1104/pp.24.1.1
Aziz M. A., Wattoo F. M., Khan F., Hassan Z., Mahmood I., Anwar A., et al. (2023). Biochar and polyhalite fertilizers improve soil’s biochemical characteristics and sunflower (Helianthus annuus L.) yield. Agronomy 13, 483. doi: 10.3390/agronomy13020483
Boshkovski B., Tzerakis C., Doupis G., Zapolska A., Kalaitzidis C., Koubouris G. (2020). Relationships of spectral reflectance with plant tissue mineral elements of common bean (Phaseolus vulgaris L.) under drought and salinity stresses. Commun. Soil Sci. Plant Anal. 51, 675–686. doi: 10.1080/00103624.2020.1729789
Burns I. G., Hutsby W. (1986). Critical comparison of the vanadomolybdate and the molybdenum blue methods for the analysis of phosphate in plant sap. Commun. Soil Sci. Plant Anal. 17, 839–852. doi: 10.1080/00103628609367756
Chen H., Min F., Hu X., Ma D., Huo Z. (2023). Biochar assists phosphate solubilizing bacteria to resist combined Pb and Cd stress by promoting acid secretion and extracellular electron transfer. J. Hazar. Mat. 452, 131176. doi: 10.1016/j.jhazmat.2023.131176
Cheng Y., Yuan J., Wang G., Hu Z., Luo W., Zhao X., et al. (2024). Phosphate-solubilizing bacteria improve the antioxidant enzyme activity of Potamogeton crispus L. and enhance the remediation effect on Cd-contaminated sediment. J. Hazard. Mater. 470, 134305. doi: 10.1016/j.jhazmat.2024.134305
Chia C. H., Singh B. P., Joseph S., Graber E. R., Munroe P. (2014). Characterization of an enriched biochar. J. Anal. Appl. Pyrolyisis 108, 26–34. doi: 10.1016/j.jaap.2014.05.021
Choudhary S., Wani K. I., Naeem M., Khan M. M. A., Aftab T. (2023). Cellular responses, osmotic adjustments, and role of osmolytes in providing salt stress resilience in higher plants: polyamines and nitric oxide crosstalk. J. Plant Growth Regul. 42, 539–553. doi: 10.1007/s00344-022-10584-7
Damalas C. A., Koutroubas S. D. (2022). Exogenous application of salicylic acid for regulation of sunflower growth under abiotic stress: a systematic review. Biologia 77, 1685–1697. doi: 10.1007/s11756-022-01020-y
Dastorani M., Albaji M., Nasab S. B. (2022). A comparison of the effect of magnetic drip irrigation and conventional irrigation with different salinity levels on the yield and yield components of sunflower (Helianthus annuus L.). Arab J. Geosci. 15, 1116. doi: 10.1007/s12517-022-10126-1
de Aquino D. S., Fanhani A., Stevanato N., da Silva C. (2019). Sunflower oil from enzymatic aqueous extraction process: Maximization of free oil yield and oil characterization. J. Food Process Eng. 42, e13169. doi: 10.1111/jfpe.13169
Dey G., Banerjee P., Sharma R. K., Maity J. P., Etesami H., Shaw A. K., et al. (2021). Management of phosphorus in salinity-stressed agriculture for sustainable crop production by salt-tolerant phosphate-solubilizing bacteria—A review. Agronomy 11, 1552. doi: https://doi.org/10.3390/agronomy11081552
Ehtaiwesh A., Sunoj V. J., Djanaguiraman M., Prasad P. V. (2024). Response of winter wheat genotypes to salinity stress under controlled environments. Front. Plant Sci. 15. doi: 10.3389/fpls.2024.1396498
Emami Bistgani Z., Barker A. V., Hashemi M. (2023). Review on Physiological and phytochemical responses of medicinal plants to salinity stress. Commun. Soil Sci. Plant Anal. 54, 2475–2490. doi: 10.1080/00103624.2023.2227212
FAO. (2022). World Food and Agriculture Statistical Yearbook 2022. Rome, Italy: Food and Agriculture Organization of the United Nations. doi: 10.4060/cc2211en
Fatemi A., Moaveni P., Daneshian J., Mozafari H., Ghaffari M. (2022). Magnesium nanoparticles improve grain yield, oil percentage, physiological, and biochemical traits of sunflower (Helianthus annuus L.) under drought stress. J. Agric. Sci. Technol. 24, 665–678. Available at: http://jast.modares.ac.ir/article-23-44376-en.html.
Fitzner M., Schreiner M., Baldermann S. (2023). The interaction of salinity and light regime modulates photosynthetic pigment content in edible halophytes in greenhouse and indoor farming. Front. Plant Sci. 14. doi: 10.3389/fpls.2023.1105162
Ghadirnezhad Shiade S. R., Pirdashti H., Esmaeili M. A., Nematzade G. A. (2023). Biochemical and physiological characteristics of mutant genotypes in rice (Oryza sativa L.) contributing to salinity tolerance indices. Gesunde Pflanz 75, 303–315. doi: 10.1007/s10343-022-00701-7
Ghasemzadeh N., Iranbakhsh A., Oraghi-Ardebili Z., Saadatmand S., Jahanbakhsh-Godehkahriz S. (2022). Cold plasma can alleviate cadmium stress by optimizing growth and yield of wheat (Triticum aestivum L.) through changes in physio-biochemical properties and fatty acid profile. Environ. Sci. pollut. Res. 29, 35897–35907. doi: 10.1007/s11356-022-18630-3
Hadidi M., Aghababaei F., McClements D. J. (2024). Sunflower meal/cake as a sustainable protein source for global food demand: Towards a zero-hunger world. Food Hydrocol. 147, 109329. doi: 10.1016/j.foodhyd.2023.109329
Hajiabadi A. A., Arani A. M., Ghasemi S., Rad M. H., Etesami H., Manshadi S. S., et al. (2021). Mining the rhizosphere of halophytic rangeland plants for halotolerant bacteria to improve growth and yield of salinity-stressed wheat. Plant Physiol. Biochem. 163, 139–153. doi: https://doi.org/10.1016/j.plaphy.2021.03.059
Haliloğlu H., Aydogdu A. (2024). The effect of different nitrogen doses on growth attributes of some sunflower (Helianthus annuus L.) cultivars grown as second crop. Akademik Ziraat Dergisi 13, 129–136. doi: 10.29278/azd.1393338
Han X., Kang Y., Wan S., Li X. (2022). Effect of salinity on oleic sunflower (Helianthus annuus Linn.) under drip irrigation in arid area of Northwest China. Agric Water Manage. 259, 107267. doi: 10.1016/j.agwat.2021.107267
Hassan N., Qadir G., Hassan F. U., Akmal M., Sultan T. (2021). Impact of phosphate solubilizing bacteria in combination with di-ammonium phosphate on growth and development of sunflower (Helianthus annus L.). J. Plant Nutr. 44, 2359–2370. doi: 10.1080/01904167.2021.1918158
He M., Ding N. Z. (2020). Plant unsaturated fatty acids: multiple roles in stress response. Front. Plant 11. doi: 10.3389/fpls.2020.562785
Heath R. L., Packer L. (1968). Photoperoxidation in isolated chloroplasts: I. Kinetics and stoichiometry of fatty acid peroxidation. Arch. Biochem. Biophys. 125, 189–198. doi: 10.1016/0003-9861(68)90654-1
Hualpa-Ramirez E., Carrasco-Lozano E. C., Madrid-Espinoza J., Tejos R., Ruiz-Lara S., Stange C., et al. (2024). Stress salinity in plants: New strategies to cope with in the foreseeable scenario. Plant Physiol. Biochem. 208, 108507. doi: 10.1016/j.plaphy.2024.108507
Imran K., Amanullah J. (2023). Integration of peach (Prunus persica L) remnants in combination with beneficial microbes and phosphorus differ phosphorus use efficiency, agronomic efficiency and partial factor productivity in soybean Vs maize crops. J. Plant Nutr. 46, 1745–1756. doi: 10.1080/01904167.2022.2099890
Iranian Ministry of Agriculture Jihad (2023). Iran data portal. Sunflower report. Tehran. Iran: Agriculture Jihad Publication.
Khondoker M., Mandal S., Gurav R., Hwang S. (2023). Freshwater shortage, salinity increase, and global food production: A need for sustainable irrigation water desalination—A scoping review. Earth 4, 223–240. doi: 10.3390/earth4020012
Khosropour E., Hakimi L., Weisany W. (2023). “Role of engineered nanomaterials in biotic stress managements,” in Engineered Nanomaterials for Sustainable Agricultural Production, Soil Improvement and Stress Management (Cambridge, Massachusetts, USA: Academic Press), 257–272. doi: 10.1016/B978-0-323-91933-3.00001-5
Khosropour E., Weisany W., Tahir N. A. R., Hakimi L. (2022). Vermicompost and biochar can alleviate cadmium stress through minimizing its uptake and optimizing biochemical properties in Berberis integerrima bunge. Environ. Sci. pollut. Res. 29, 17476–17486. doi: 10.1007/s11356-021-17073-6
Kumar A., Behera I., Langthasa M., PrakashNaroju S. (2023). Effect of plant growth-promoting rhizobacteria on alleviating salinity stress in plants: A review. J. Plant Nutr. 46, 2525–2550. doi: 10.1080/01904167.2022.2155548
Kumari S., Chhillar H., Chopra P., Khanna R. R., Khan M. I. R. (2021). Potassium: A track to develop salinity tolerant plants. Plant Physiol. Biochemi. 167, 1011–1023. doi: 10.1016/j.plaphy.2021.09.031
Lai W., Wu Y., Zhang C., Dilinuer Y., Pasang L., Lu Y., et al. (2022). Combination of biochar and phosphorus solubilizing bacteria to improve the stable form of toxic metal minerals and microbial abundance in lead/cadmium-contaminated soil. Agronomy 12, 1003. doi: 10.3390/agronomy12051003
Li Z., Wang Y., Liu Z., Han F., Chen S., Zhou W. (2023). Integrated application of phosphorus-accumulating bacteria and phosphorus-solubilizing bacteria to achieve sustainable phosphorus management in saline soils. Sci. Total. Environ. 885, 163971. doi: 10.1016/j.scitotenv.2023.163971
Liu Y., Nessa A., Zheng Q., Hu D., Zhang W., Zhang M. (2023). Inoculations of phosphate-solubilizing bacteria alter soil microbial community and improve phosphorus bioavailability for moso bamboo (Phyllostachys edulis) growth. Appl. Soil Ecol. 189, 104911. doi: 10.1016/j.apsoil.2023.104911
Liu J., Xia B., Du X., Zeng T., Liu Y., Chen L., et al. (2018). Effects of water supplemented with Bacillus subtilis and photosynthetic bacteria on egg production, egg quality, serum immunoglobulins and digestive enzyme activity of ducks. J. Appl. Anim. Res. 46, 322–326. doi: 10.1080/09712119.2017.1299741
Lutts S., Kinet J. M., Bouharmont J. (1995). Changes in plant response to NaCl during development of rice (Oryza sativa L.) varieties differing in salinity resistance. J. Exp. Bot. 46, 1843–1852. doi: 10.1093/jxb/46.12.1843
Masrahi A. S., Alasmari A., Shahin M. G., Qumsani A. T., Oraby H. F., Awad-Allah M. M. (2023). Role of arbuscular mycorrhizal fungi and phosphate solubilizing bacteria in improving yield, yield components, and nutrients uptake of barley under salinity soil. Agriculture 13, 537. doi: 10.3390/agriculture13030537
Munns R., Wallace P. A., Teakle N. L., Colmer T. D. (2010). Measuring soluble ion concentrations (Na+, K+, Cl–) in salt-treated plants. Plant stress tolerance. Methods Protoc. 639, 371–382. doi: 10.1007/978-1-60761-702-0_23
Osman H. S., Rady A. M., Awadalla A., Omara A. E. D., Hafez E. M. (2022). Improving the antioxidants system, growth, and sugar beet quality subjected to long-term osmotic stress by phosphate solubilizing bacteria and compost tea. Int. J. Plant Prod. 16, 119–135. doi: 10.1007/s42106-021-00176-y
Rabbani A., Ardakani M. R., Naghdi Badi H., Rezazadeh. S., Sarajooghi M. (2023). Evaluation of morphophysiological characteristics, nutrient accumulation and fatty acid profile of hemp (Cannabis sativa L.) under different salinity levels. Gesun. Pflan. 75, 1843–1853. doi: 10.1007/s10343-023-00846-z
Rahimi Chegeni A., Fatehi F., Ebrahimi A., Maleki M. (2023). Phosphate-solubilizing bacteria modulated salinity stress in the presence of phosphorous through improving growth, biochemical properties, and gene expression of chickpea (Cicer arientnum L.). J. Soil Sci. Plant Nutr. 23, 4450–4462. doi: 10.1007/s42729-023-01362-9
Rasool A., Shah W. H., Mushtaq N. U., Saleem S., Hakeem K. R., ul Rehman R. (2022). Amelioration of salinity induced damage in plants by selenium application: A review. South Afric J. Bot. 147, 98–105. doi: 10.1016/j.sajb.2021.12.029
Rawat P., Sharma A., Shankhdhar D., Shankhdhar S. C. (2022). Improvement of phosphorus uptake, phosphorus use efficiency, and grain yield of upland rice (Oryza sativa L.) in response to phosphate-solubilizing bacteria blended with phosphorus fertilizer. Pedosphere 32, 752–763. doi: 10.1016/j.pedsph.2022.06.005
Reza D. T. A., Sohrab M., Hossein Ali. A., Masoomeh S. (2021). Role of rhizospheric microorganisms in mitigating the adverse effect of salinity stress in Plantago ovata growth, biochemical and photosynthetic traits. Arch. Agron. Soil Sci. 67, 1060–1074. doi: 10.1080/03650340.2020.1775816
Seymen M., Yavuz D., Eroğlu S., Arı B.Ç., Tanrıverdi Ö.B., Atakul Z., et al. (2023). Effects of different levels of water salinity on plant growth, biochemical content, and photosynthetic activity in cabbage seedling under water-deficit conditions. Gesunde Pflanzen 75, 871–884. doi: 10.1007/s10343-022-00788-y
Singh A., Pandey S., Srivastava R. P., Devkota H. P., Singh L., Saxena G. (2022). “Helianthus annuus L.: traditional uses, phytochemistry, and pharmacological activities,” in Medicinal Plants of the Asteraceae Family: Traditional Uses, Phytochemistry and Pharmacological Activities (Springer Nature Singapore, Singapore), 197–212. doi: 10.1007/978-981-19-6080-2_12
Sofy M. R., Elhawat N., Alshaal T. (2020). Glycine betaine counters salinity stress by maintaining high K+/Na+ ratio and antioxidant defense via limiting Na+ uptake in common bean (Phaseolus vulgaris L.). Ecotoxicol. Environm. Saf. 200, 110732. doi: 10.1016/j.ecoenv.2020.110732
Suhail F., Afzal A., Naseer L., Pervaiz A., Ikram M., Shaheen S., et al. (2023). Influence of phosphate solubilizing bacteria on the growth of mustard grown under drought stress conditions. Agric. Res. 12, 375–386. doi: 10.1007/s40003-023-00656-9
Sun X., Wang W., Yi S., Zheng F., Zhang Z., Alharbi S. A., et al. (2024). Microbial composition in saline and alkaline soils regulates plant growth with P-solubilizing bacteria. Appl. Soil Ecol. 203, 105653. doi: 10.1016/j.apsoil.2024.105653
Waqar M., Habib-ur-Rahman M., Hasnain M. U., Iqbal S., Ghaffar A., Iqbal R., et al. (2022). Effect of slow release nitrogenous fertilizers and biochar on growth, physiology, yield, and nitrogen use efficiency of sunflower under arid climate. Environ. Sci. pollut. Res. 29, 52520–52533. doi: 10.1007/s11356-022-19289-6
Wu Y., Wang X., Zhang L., Zheng Y., Liu X., Zhang Y. (2023). The critical role of biochar to mitigate the adverse impacts of drought and salinity stress in plants. Front. Plant Sci. 14. doi: 10.3389/fpls.2023.1163451
Yahya M., Islam E. U., Rasul M., Farooq I., Mahreen N., Tawab A., et al. (2021). Differential root exudation and architecture for improved growth of wheat mediated by phosphate solubilizing bacteria. Front. Microbiol. 12. doi: 10.3389/fmicb.2021.744094
Zadegan K., Monem R., Pazoki A. (2023). Silicon dioxide nanoparticles improved yield, biochemical attributes, and fatty acid profile of cowpea (Vigna unguiculata [L.] Walp) under different irrigation regimes.J. Soil Sci. Plant Nutr. 23, 3197–3208. doi: https://doi.org/10.1007/s42729-023-01297-1
Zheng C., Liu C., Liu L., Tan Y., Sheng X., Yu D., et al. (2023). Effect of salinity stress on rice yield and grain quality: A meta-analysis. Eur. J. Agron. 144, 126765. doi: https://doi.org/10.1016/j.eja.2023.126765
Keywords: grain oil, phosphorous availability, malondialdehyde, soil salinity, rhizosphere microorganism
Citation: Jahanshahi S, Moaveni P, Ghaffari M, Mozafari H and Alizadeh B (2024) Physio-biochemical responses of three sunflower (Helianthus annuus L.) lines to phosphate solubilizing bacteria and phosphorous-enriched biochar in saline soils. Front. Plant Physiol. 2:1497753. doi: 10.3389/fphgy.2024.1497753
Received: 17 September 2024; Accepted: 31 October 2024;
Published: 20 November 2024.
Edited by:
Valentina Stoian, University of Agricultural Sciences and Veterinary Medicine of Cluj-Napoca, RomaniaReviewed by:
Muhammad Qudrat Ullah Farooqi, University of Western Australia, AustraliaAurel Maxim, University of Agricultural Sciences and Veterinary Medicine of Cluj-Napoca, Romania
Copyright © 2024 Jahanshahi, Moaveni, Ghaffari, Mozafari and Alizadeh. This is an open-access article distributed under the terms of the Creative Commons Attribution License (CC BY). The use, distribution or reproduction in other forums is permitted, provided the original author(s) and the copyright owner(s) are credited and that the original publication in this journal is cited, in accordance with accepted academic practice. No use, distribution or reproduction is permitted which does not comply with these terms.
*Correspondence: Payam Moaveni, cGF5YW0ubW9hdmVuaTIwQGdtYWlsLmNvbQ==; cGF5YW0ubW9hdmVuaUB5YWhvby5jb20=