- 1Biology Department, Adelphi University, Garden City, NY, United States
- 2Institute of Biology/Applied Genetics, Dahlem Centre of Plant Science, Freie Universität Berlin, Berlin, Germany
- 3Laboratory of Growth Regulators, Faculty of Science, Palacký University and Institute of Experimental Botany, The Czech Academy of Sciences, Olomouc, Czechia
Introduction: Cytokinins, a group of adenine derivatives, are phytohormones that regulate many aspects of the plant's reaction to changes in the abiotic and biotic environment and ensure the correct execution of developmental programs. While the signaling pathway and its effects are very well established for Angiosperms, its origin, and evolution are less well understood.
Methods: The first step in the analysis of the cytokinin signaling pathway is to test if the organism can react to the hormone. Thus, an assay was established, that uses differences in the growth pattern of the Streptophyte alga, Coleochaete scutata, to determine if this algal species reacts to different cytokinins.
Results: Surprisingly not only classical cytokinins, such as trans-zeatin and kinetin, led to a change in the pattern of growth, but also adenine, which is usually used as a negative control.
Discussion: This raises questions about the origin and the functioning of the cytokinin signaling in C. scutata and also in algae in general.
Introduction
The conquest of land by plants is one of the most consequential events in the evolution of life. While there are few fossils of those early land plants, recent analyses place this event around 550 mya ago (Morris et al., 2018; Fürst-Jansen et al., 2020). However, even the first land plants were not alone in this new habitat. The mycorrhizal landing hypothesis predicts that bacteria and fungi were already present in this environment (Delaux et al., 2015). It is believed that the ability to form symbiotic relationships with some of those organisms already present was a prerequisite for the successful establishment of plant life on land (de Vries and Rensing, 2020). The presence of regulatory systems controlled by phytohormones is often considered another requirement for the landfall by plants (Wang et al., 2015; Fürst-Jansen et al., 2020). Phytohormones are small compounds that regulate various aspects of plant life, such as the interaction with abiotic and biotic environments and the control of developmental processes. Phytohormone biology can be roughly divided into three sections: (i) the metabolism, (ii) the transport, and (iii) the signal perception and transduction. (Eklund et al., 2018; Sun et al., 2019; Blázquez et al., 2020; Yang et al., 2020). One of the nine classical groups of plant hormones is cytokinin (Guillory and Bonhomme, 2021). Cytokinins are N6-substituted adenine derivatives, which were originally identified for their role in promoting plant cell growth (Kieber and Schaller, 2010). As nucleic acid derivatives, cytokinins are found in virtually every organism investigated. However, only in cyanobacteria, amoebae, streptophyte algae, and land plants are the amount and type of cytokinin regulated (Gajdosova et al., 2011; Žižková et al., 2017; Aoki et al., 2019).
Cytokinin metabolism
Cytokinin metabolism has been characterized as the balance between synthesis, interconversion, formation of conjugates, and degradation (Heyl et al., 2007). In the synthesis of cytokinins, the rate-limiting step is catalyzed by isopentenyl transferases (IPTs) which either uses tRNA (tRNA pathway) or ATP/ADP/AMP (adenylate pathway) as a substrate (Lindner et al., 2014; Frébortová and Frébort, 2021). Of these two pathways the tRNA pathway is considered to be evolutionary older (Frébort et al., 2011; Frébortová et al., 2015), due to the evolutionary history of its class of IPT genes (Wang et al., 2020; Hluska et al., 2021). Also, cis-zeatin and its derivatives, which are mainly produced by the tRNA pathway, are the predominant form of cytokinins in algae (Stirk et al., 2003). While interconversion and the formation of conjugates are regulated by many different types of enzymes, the irreversible degradation of cytokinins in the land plant is mediated by cytokinin oxidases/dehydrogenases (CKX) (Heyl et al., 2006; Frébort et al., 2011).
Cytokinin transport
While each plant cell should be capable of producing cytokinins, local differences in cytokinin concentration and experimental evidence for long-distance transport highlight the presence of facilitation proteins (Hirose et al., 2007; Matsumoto-Kitano et al., 2008; Zürcher et al., 2016; Osugi et al., 2017). In the last decades, several cytokinin transporters have been identified, mainly belonging to five different protein families: (i) ATP-BINDING CASSETTE TRANSPORTER SUBFAMILY G (ABCG), (ii) PURINE PERMEASE (PUP),(iii) AZA-GUANINE RESISTANT (AGZ), (iv)EQUILIBRATIVE NUCLEOSIDE TRANSPORTER (ENT), and (v)ABC subfamily I (ABCI) (Hu and Shani, 2023). However, more research is needed to better understand the specific functions of many of these transporters in cytokinin biology.
Cytokinin signaling
Cytokinin signaling is mediated by a multi-step His-to-Asp phosphorelay system, based on the bacterial two-component system (Hwang and Sheen, 2001; West et al., 2001). This system is best characterized in Arabidopsis thaliana. Here, the cytokinin interaction with the receptor leads to its autophosphorylation (Inoue et al., 2001). After an intramolecular phosphorelay, the signal is transferred to a histidine phosphotransfer protein (HPt), which continuously shuttles between the cytoplasm and the nucleus (Hutchison et al., 2006; Punwani et al., 2010). In the nucleus, the HPts activate type-B response regulators (RRB). RRBs are transcription factors that activate the transcription of their target genes upon phosphorylation. One group of the target genes is the type A response regulation (RRA). RRAs act as negative regulators of the cytokinin signaling pathway (Hwang and Sheen, 2001; Mason et al., 2004; To et al., 2004; Heyl et al., 2008; Ishida et al., 2008).
Origin and evolution of cytokinin
The increasing number of available genomic and transcriptomic data at the beginning of the century allowed for deeper phylogenetic, studies and one of the first in-depth studies in the field looked at the origin and evolution of cytokinin (Pils and Heyl, 2009). Work on other hormones quickly followed (De Smet et al., 2011; Wang et al., 2015). A detailed analysis including more than 50 Streptophytes revealed different evolutionary patterns for each of the four protein families of cytokinin signaling (Kaltenegger et al., 2018). It was generally assumed that if the respective genes are present in the respective genome, they would work in the same way as in Arabidopsis and rice. However, for several phytohormones, it has been shown that although the members of the signaling pathway are present, they function in different ways (Yasumura et al., 2007; Fürst-Jansen et al., 2020; Feng et al., 2023). This strongly highlights the need for experimental evidence to understand the evolution of a given pathway. Thus, streptophyte algae are gaining more attention as organisms for hormonal research (de Vries and Archibald, 2018; De Vries et al., 2018; de Vries and Rensing, 2020; Feng et al., 2023).
Coleochaete scutata
Coleochaete scutata, a member of the Coleochaetales, has been used as a model for apical growth, quantitative analysis of body plant formation and multicellular morphogenesis, and the functional and structural changes during desiccation (Graham et al., 2012; Leliaert et al., 2012). Coleochaetales belong to the so-called advanced streptophyte algae as they form – just like land plants – phragmoplasts during cell division (McCourt et al., 2023). It also belongs to the group of Streptophyte algae that can rotate the plane of cell division, like what is seen in land plants (Buschmann, 2020). Due to these properties, Coleochaete has been proposed as an emerging model organism (Domozych et al., 2016). We were interested in investigating if C. scutata is utilizing the cytokinin signaling system to regulate growth. Toward this goal, a novel cytokinin response assay was used to test different cytokinins in their ability to change the growth pattern of C. scutata.
Materials and methods
Coleochaete scutata culture, treatment, and analysis
C. scutata stock culture LB 2784 (UTEX Culture Collection of Algae at the University of Texas at Austin, Austin Texas USA) was cultured using Bold 3N media (UTEX). at a constant 20°C in a 16h/8h light/dark cycle. For treatment, 1 ml of three-week-old culture was transferred on a sterile microscope slide and placed in a sterile glass petri dish. The sample was distributed evenly across the slide. The dishes were incubated for four hours at 20°C for adherence of the algae to the slide. After four hours, 11 mL of Bold 3N media were slowly pipetted into the side of the dish to form a complete layer over the entire dish. The chemicals used for treatment were dissolved in 1 μM KOH and were added to the petri dish. The dishes were placed on the same shelf, equidistant from the light source during incubation, and were incubated for one week at 20°C in a 16/8h light/dark cycle. After incubation, the algal growth of each slide was analyzed under 200x magnification using a microscope (Zeiss, Infinity 3 Lumenera, Germany). The first 50 living cells seen were classified by both the growth pattern and the number of cell rows. Cells were characterized in three categories: only 2D or only unstructured growth or a combination of both – here referred to as “mixed”.
The data were analyzed using a generalized linear mixed model. In short, each cell structure was treated as an observation and accounted for the dependence structure in the data by including the microscope slide as a random factor, nested within an experimental trial, in addition to our focal predictor, treatment. First, it was examined whether the random factor explained any variation in the response. If this was not the case, the microscope slide was removed from the model and a generalized linear model was run, which was further simplified by removing the variable trial if it was not significant. A binomial error distribution was specified to test the dichotomous response “dimension” (2D or mixed) and a Poisson error distribution to analyze the number of cell rows. Model diagnostics followed the recommendation by (Zuur and Ieno, 2016). All analyses were carried out using R version 4.3.1 (R Core Team, 2023) and RStudio version 2023.06.1 (Posit team, 2023).
Measurements of endogenous cytokinins
For the quantification of cytokinin content, the axenic culture of C. scutata (Central Collection of Algal Cultures (CCAC) 0493) was used. The culture conditions were the same as described before, only the Wees-H media was used instead of Bold N3 (McFadden and Melkonian, 1986). Quantification of cytokinin metabolites was performed according to the method described by Novák et al. (Novák et al., 2008). 50 mg fresh weight or 1 ml media were extracted in 1 ml and 9 ml of modified Bieleski buffer (60% MeOH, 10% HCOOH, and 30% H2O), respectively, together with a cocktail of stable isotope-labeled internal standards (0.5 pmol of CK bases, ribosides, N-glucosides, and 1.0 pmol of CK O-glucosides, nucleotides per sample added). The extracts were applied onto an Oasis MCX column (30 mg/1 ml, Waters) conditioned with 1 ml each of 100% MeOH and H2O, equilibrated sequentially with 1ml of 50% (v/v) nitric acid, 1 ml of H2O, and 1 ml of 1M HCOOH, and washed with 1 ml of 1M HCOOH and 1 ml 100% MeOH. Analytes were then eluted by two-step elution using 1 ml of 0.35M NH4OH aqueous solution and 2 ml of 0.35M NH4OH in 60% (v/v) MeOH solution. The eluates were then evaporated to dryness in a vacuum and stored at -20°C. Cytokinin levels were determined by an ultra-high performance liquid chromatography-electrospray tandem mass spectrometry (UHPLC-MS/MS) using stable isotope-labeled internal standards as a reference (Rittenberg and Foster, 1940). Separation was performed on an Acquity UPLC® i-Class System (Waters, Milford, MA, USA) equipped with an Acquity UPLC BEH Shield RP18 column (150x2.1 mm, 1.7 μm; Waters), and the effluent was introduced into the electrospray ion source of a triple quadrupole mass spectrometer Xevo™ TQ-S MS (Waters, Milford, MA, USA). Five independent biological replicates were performed, including two technical replicates of each.
Phylogenetic analysis
Members of the cytokinin signaling pathway from Physcomitrum patens were identified using phytozome (CHK, Pp3c16_7610 (CRE2), HPt, Pp3c17_11720, RRB, Pp3c23_7400, RRA, Pp3c8_11160) (Goodstein et al., 2012). These sequences were used as queries to identify components of the cytokinin signaling pathway using BLASTP against C. scutata sequences of the OneKP database (Leebens-Mack et al., 2019). Resulting sequences with an e-value above 5.0e-08 were checked for the presence of the canonical protein domains using PFAM (Mistry et al., 2021), and sequences missing domains were eliminated. RRAs only contain a response regulator domain, a domain also found in RRBs and CHKs. To make sure that identified RRAs are not truncated RRBs or CHKs it is necessary to run a phylogenetic analysis (Heyl et al., 2013). Thus, the CHK, RRB, and RRA sequences were used to generate a phylogenetic tree of the response regulators domains using the Phylogeny program (Dereeper et al., 2008). In short, sequences were aligned using MAFFT, curated using GBLOCKS, and a tree was calculated using the PhyML algorithm (Guindon and Gascuel, 2003; Talavera and Castresana, 2007; Katoh et al., 2018). The branching pattern of the resulting tree was confirmed by using the Bayesian Interference method instead for the calculation of the tree (Ronquist et al., 2012).
Results
C. scutata regulates cytokinin content differently in tissue and in culture media
Any organism that uses a chemical as a signaling molecule must be able to regulate the quantity and quality of that component. For aquatic organisms, this includes not only the body itself but also the immediate surrounding environment – in cultured specimens, the media. Thus, the cytokinin content of C. scutata tissue and the media it was grown in, was measured (Table 1). Four different types of cytokinin and their ribosides were tested – trans-Zeatin (tZ), cis-Zeatin (cZ), Isopentenyl adenine (IP), and Dihydrozeatin (DHZ) and their respective derivatives. Interestingly, many of the types of cytokinin derivatives, which are common in Angiosperms, were not found in C. scutata tissue or its medium (Table 1). While ribosides and O-glycosylates were detected for most of the four types of cytokinin, 7-, and 9-glucosides were not found for any cytokinin variety tested. When comparing the concentration of the detected cytokinins in the tissue with those detected in the media, the types of cytokinin found were very similar. However, there was a 10- to 100-fold difference in the quantity. Generally, the cytokinin concentration in the tissue was much lower than those detected in the media. Exceptions to this trend were iPR5’MP and cZR5’MP, which were both found in trace amounts in the algal tissue but below the limit of detection in the media (Table 1).
Most members of the canonical cytokinin signaling pathway are present in C. scutata
In land plants cytokinin is perceived and the signal is transduced by members of four protein families. Sequences from the cytokinin signaling pathway of Physcomitrium patens were chosen as in this species different aspects of cytokinin biology are very well characterized and it is evolutionary more closely related to C. scutata than other cytokinin model species, such as Arabidopsis or rice (von Schwartzenberg et al., 2007; Lindner et al., 2014; Gruhn et al., 2015; Von Schwartzenberg et al., 2016; Guillory and Bonhomme, 2021). BLASTP searches against the C. scutata sequences of the OneKP database (Carpenter et al., 2019) revealed the presence of putatively one CHK, one HPt, two RRB, and several RRA sequences (Table 2). As the RRA sequence could also represent truncated RRBs, CHKs, or other histidine kinases, a phylogenetic tree was generated using only the response regulator domain (Supplementary Figure 1). This analysis revealed that all putative RRAs from C.scutata cladded with the CHKs, thus are more likely part of a truncated histidine kinase than bone fide RRAs.
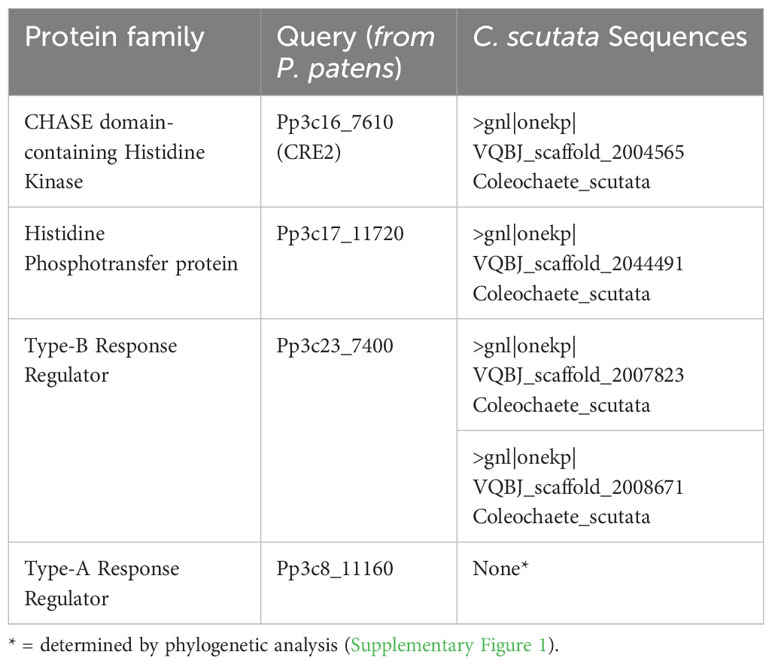
Table 2 Coleochaete scutata sequences with complete domain structure for members of the cytokinin signaling pathway.
Classification of the growth pattern of C. scutata
The signaling molecules, cytokinins, were identified and their content was shown to be regulated (Table 1). Canonical cytokinin receptors and most of the signaling proteins are present (Table 2). This raised the question if C. scutata is able to respond to cytokinin treatment. To answer this question, we first looked at the types of growth patterns found in this Streptophyte algae species. Three distinct growth patterns were detected: (i) a monolayered, discoid body (referred to as 2D), (ii) an unstructured, callus-like body, and (iii) a mixture of the two, with a discoid body with some additional unstructured growth (referred to as “mixed”) (Figure 1).
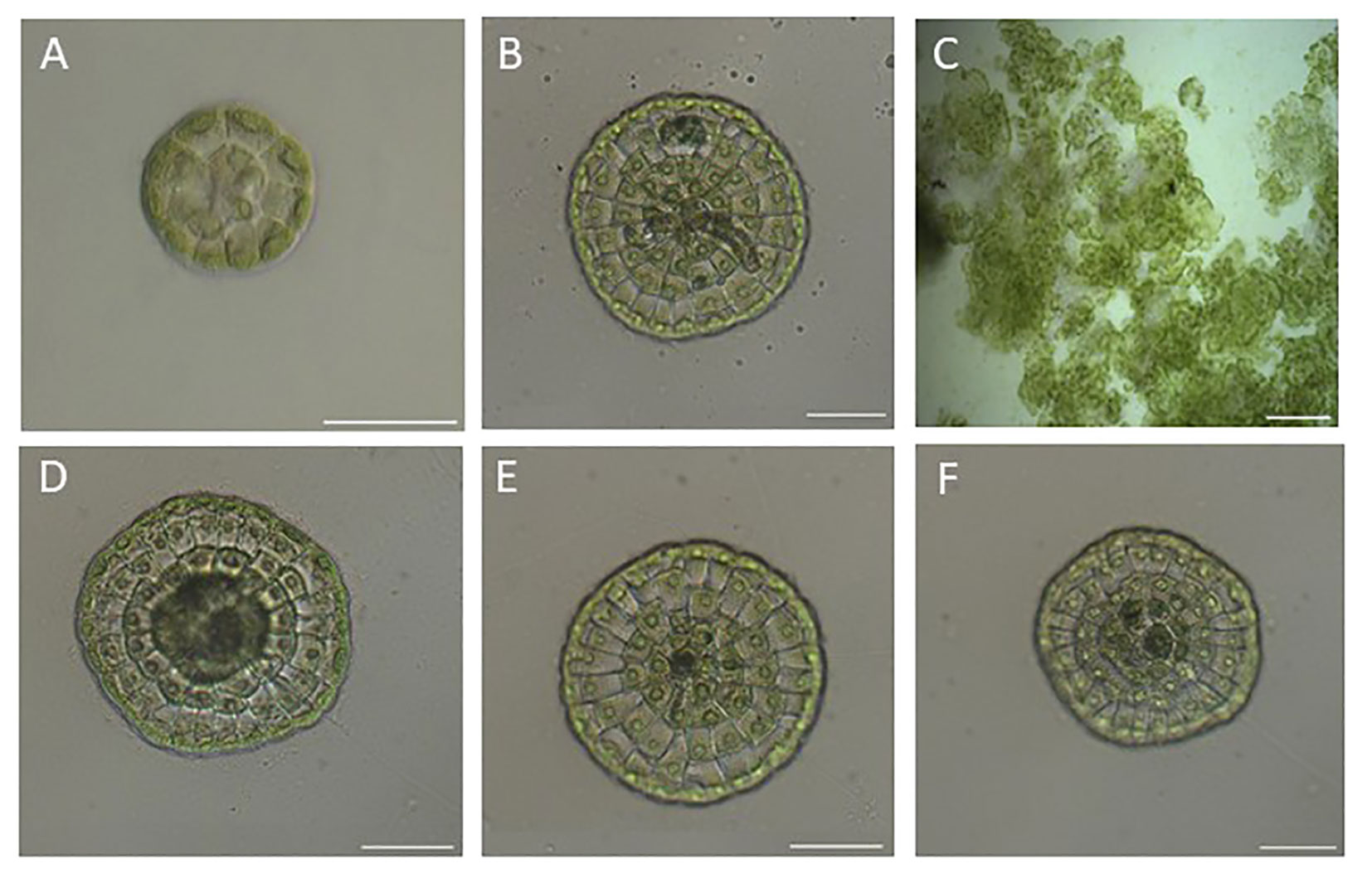
Figure 1 The different types of growth patterns of C scutata observed in this study. Cultures of C scutata were grown on glass slides and analyzed after one week. (A) Represents a disk-type growth (2D) with 1 cell row. (B) Represents a disk-type growth with an unstructured part (mixed) with 5 cell rows. (C) Represent algae with unstructured growth. (D) Represents a mixed growth type with 5 cell rows. (E) Represent a disk growth (2D) with 4 cell rows. (F) Represents a mixed growth type with 4 cell rows. The scale bar represents 200 μm.
The cytokinin iP, but also adenine affects the growth pattern of C. scutata
The effect of cytokinin treatment on the occurrence of those patterns was tested by treating C. scutata, with isopentenyl adenine (iP) at different concentrations. Adenine was used as a negative control as it does not trigger a significant cytokinin response in plants (Skoog et al., 1967; Brandstatter and Kieber, 1998). In the KOH solvent control, most of the C.scutata scored showed unstructured growth. Only a few algae showed the 2D or the mixed phenotype (Figure 2). When compared to the KOH control, iP triggered a significant increase in the size of discoid growth with increasing concentrations of iP causing more growth in the size (number of cell rows) of the discoid structures in both 2D and mixed (Figure 2). Surprisingly, adenine also triggered a change in the growth pattern, which was comparable, but significantly weaker than that of iP for the higher concentrations (100nM, 1µM) (Supplemental Files). Algae treated with the solvent control, KOH, showed only unstructured growth (Figure 2).
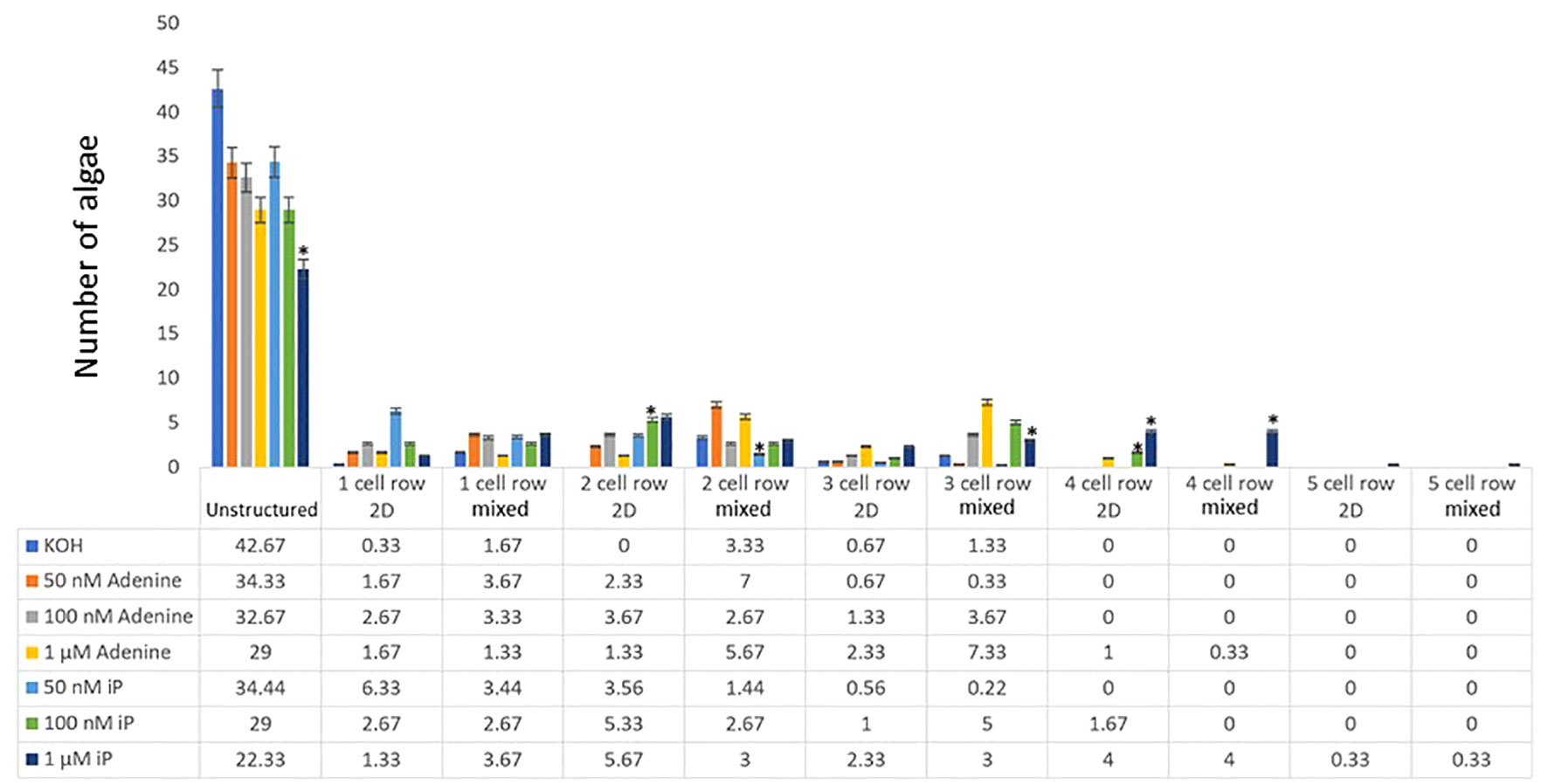
Figure 2 Cell row and dimensional growth after treatment with iP or adenine. C. scutata cells were grown in liquid culture supplemented with 50 nM, 100 nM, or 1 μM adenine or iP, respectively. KOH treatment served as solvent control. After one week, the growth pattern of the first 50 algae was scored for the structure of growth - either disc-like (2D), unstructured, or mixed. In addition, the number of cell rows was counted. The experiment was repeated three times and the average of the three experiments is shown here. The standard deviation is shown as a percentage. * = represents a significant difference (P<0.05) between adenine and iP at the respective concentration.
Different cytokinins lead to more structured growth in C. scutata
In the next step, different types of cytokinins (Kinetin, 6-benzyl-adenine (BA), and trans-eatin were tested in different concentrations. A similar picture as in the iP treatment emerged. The control treatment with KOH led to mainly unstructured growth. The treatment of the algae with adenine led to a significant increase in the number of algae growing in discoid structures as before (Figure 3). Among the different tested cytokinins, tZ had the strongest effect, as seen by the size of the discoid growth it triggered – significantly larger than that observed with adenine. The size of the algae treated with either BA or kinetin was very similar to those treated with adenine, except for adenine-treated cultures with a concentration of 100nM, which resulted in more cell rows than those treated with BA or kinetin (Figure 3; Supplementary Files). See the Supplementary Materials for detailed statistical analysis.
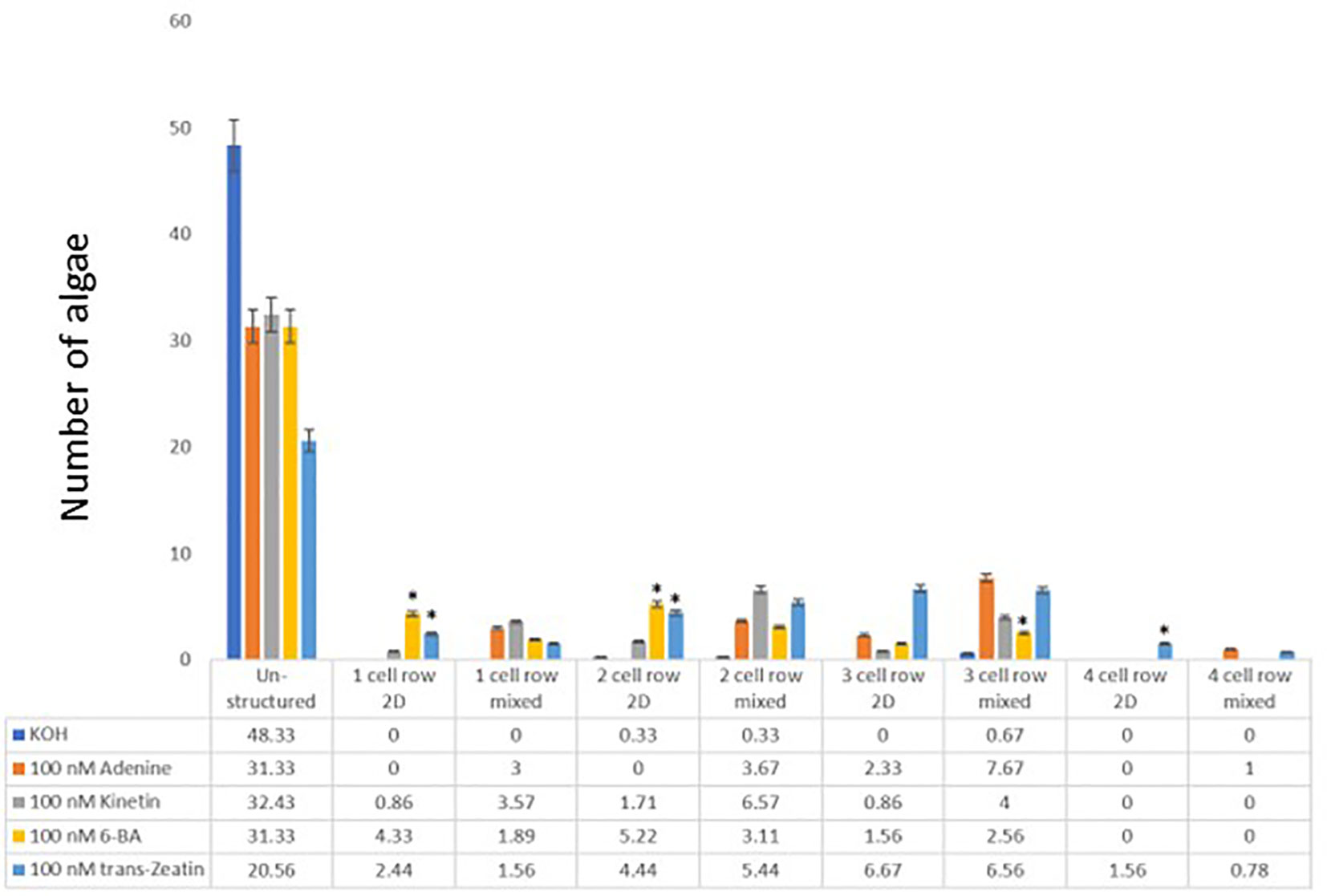
Figure 3 Cell row and dimensional growth after treatment with different cytokinins. C. scutata cells were grown in liquid culture supplement with 100nM adenine, kinetin, BA, or trans-Zeatin, respectively. KOH treatment served as a solvent control. After one week, the growth pattern of the first 50 algae was scored for the structure of growth - either disc-like (2D), unstructured, or mixed. In addition, the number of cell rows was counted. The experiment was repeated three times and the average of the three experiments is shown here. The standard deviation is shown as a percentage. * = represents a significant difference (P<0.05) between adenine and the other chemicals used at the respective concentrations.
Both purines, adenine, and guanine, have an effect on algal growth
With Adenine treatment leading to a change in growth pattern similar or even stronger than that observed with traditional cytokinins, the question arose, if this effect is adenine specific or if other purines would have the same effects. Thus, also guanine, the other purine of nucleic acids, was tested for its effect on the growth of C. scutata. Again, a significant difference was detected between the KOH control on one side and guanine and adenine on the other. The effect of guanine on the growth of C. scutata was statistically indistinguishable from that of adenine (Figure 4; Supplementary Files).
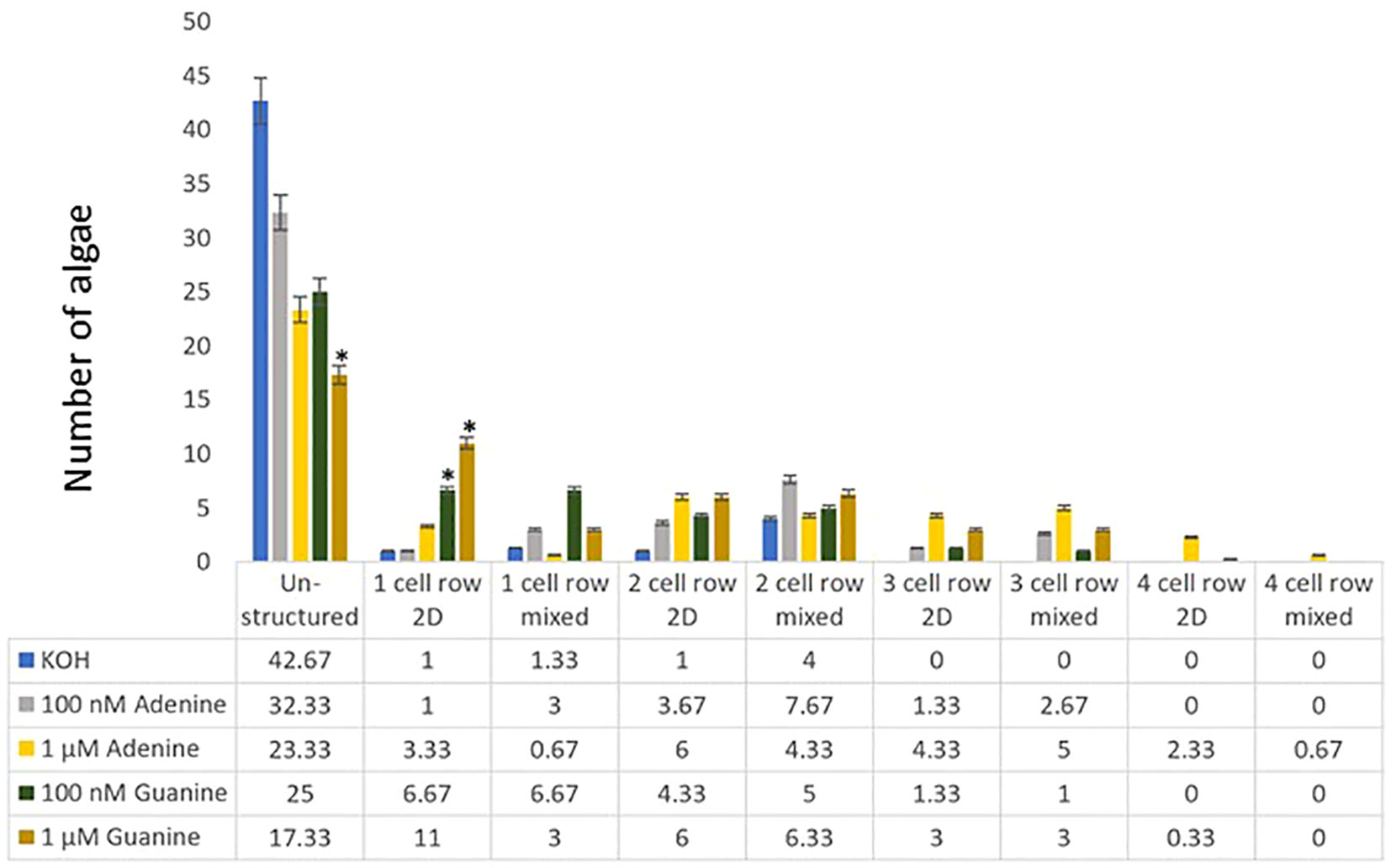
Figure 4 Cell row and dimensional growth after treatment with adenine and guanine. C. scutata cells were grown in liquid culture supplemented with 100 nM, or 1 μM adenine or guanine, respectively. KOH treatment served as a solvent control. After one week, the growth pattern of the first 50 algae was scored for the structure of growth - either disc-like (2D), unstructured, or mixed. In addition, the number of cell rows was counted. The experiment was repeated three times and the average of the three experiments is shown here. The standard deviation is shown as a percentage. * = represents a significant difference (P<0.05) between adenine and guanine at the respective concentration.
Discussion
The types of cytokinins found in C. scutata are comparable to those of other algae
A signaling system consists of a signaling molecule, a receptor, and a signal transduction pathway, which connects the binding of the ligand to a cellular response. Thus, the first analysis was to look at the presence of the signaling molecule. The analysis of the cytokinin content of the algae themselves as well as of the media, showed marked differences, implying that the cytokinins are regulated, both in quantity and quality. Interestingly, when a cytokinin type is present in both, the media usually contains more than 10-fold more of the chemical. This might indicate that C. scutata regulates its cellular cytokinin content by transporting it out of the cell. However, in the aqueous, natural environment the cytokinins would most likely quickly diffuse away and not build up as they do in the artificial situation of a liquid culture.
When compared to the cytokinin content of various macro- and microalgae, Marchantia polymorpha and Physcomitrium patens, the cytokinin content of C. scutata was lower or in the same range (Stirk et al., 2003; Ördög et al., 2004; Stirk et al., 2013; Lindner et al., 2014; Aki et al., 2019a). One of the most interesting aspects of this comparison was the trans-zeatin content. This cytokinin is the most effective cytokinin in experiments in land plants, yet its contents differ wildly from not detectable to being equal to other unmodified cytokinins (Aki et al., 2019a; Gajdosova et al., 2011; Lindner et al., 2014). More research in different species is necessary to understand the relationship between cytokinin content and cytokinin effect.
The cytokinin signaling pathway of C. scutata is similar to other streptophyte algae
The first comprehensive analysis of the members of the cytokinin signaling pathway across the plant kingdom showed that all species investigated had HPts and RRBs genes in their genomes (Pils and Heyl, 2009). During the course of evolution, the classical cytokinin receptors (CHKs) and then the RRAs evolved (Aki et al., 2019b; Gruhn et al., 2015; Wang et al., 2015; Bowman et al., 2017; Kaltenegger et al., 2018; Powell and Heyl, 2023). The sequences obtained from the transcriptome database OneKP seem to fit this model, as only one CHK, one HPt, and two RRBs, but no RRAs were detected (Table 2). However, while these data fit with the current model for the evolution of cytokinin signaling, one should keep in mind that due to a lack of genomic data, this analysis was based on the mRNA sequence approach of the OneKP consortium (Leebens-Mack et al., 2019). As RRA are usually transcribed only after the perception of a cytokinin signal, members of this protein family might be present in the genome, but not transcribed at the time of mRNA collection for the analysis. Thus, only the sequencing of the nuclear genome will ultimately determine, if RRAs are present in C. scutata.
Cytokinins and purines change algal growth patterns
For any signaling system to be functional, its activity must lead to a response of the organism on the cellular, the organ, and/or the organismal level. For cytokinins, as for many other hormones, several bioassays have long been established for land plants (Inoue et al., 2001; Mason et al., 2004; To et al., 2004; Hutchison et al., 2006; Von Schwartzenberg et al., 2016). While for algae no such set of established assays is available, many researchers have investigated the effects of phytohormone on algal growth (Renuka et al., 2017; Piotrowska-Niczyporuk et al., 2018; Guldhe et al., 2019; Piotrowska-Niczyporuk et al., 2020; Singh et al., 2020; Araújo et al., 2021). In this study, the effect of different cytokinins and purines on the growth pattern of C. scutata was investigated. It is well known that cytokinins promote cell division in modern land plants and the molecular mechanism for this apical growth has recently been established for Arabidopsis (Schaller et al., 2015; Yang et al., 2021). As C. scutata has long been proposed to serve as a model organism for apical cell growth (Domozych et al., 2016; Buschmann, 2020), we established a growth here that is easily scorable and showed a clear difference between the solvent control and the cytokinin treatment. It is based on previous work looking at the effect of terrestrial habitats on the growth of C. orbicularis and C. scutata (Graham et al., 2012). In an aqueous mineral media, Coleochaetes grows mainly in a discoid shape. As the cytokinins used in this experiment are dissolved in KOH, this was also used as a control. Interestingly, for unknown reasons, the addition of KOH seems to alter the growth habit of C. scutata into a more unstructured growth. However, considering the results of previous experiments with cytokinin on the algae, it was not surprising that a change in growth pattern was observed upon cytokinin treatment (see above). However, the results for adenine were very surprising. As adenine has been established early on in cytokinin research as not biologically active (Skoog et al., 1967), it is mainly used as a negative control in cytokinin experiments (Brandstatter and Kieber, 1998; Dow et al., 2022). The results of the current study show that adenine is as functional as a growth regulator as classical cytokinins - at least in C. scutata. However, this might be true also for other algae or even early diverging land plants. Only experiments can show. Even more surprising was that guanine was as effective as adenine or cytokinins in changing the way that C. scutata grew. This raises several possible scenarios about cytokinin signaling in C. scutata, which might apply to Streptophyte algae in general:
1.) No cytokinin signaling pathway.
Algae might not respond to cytokinin and related chemicals as a specific signal but rather just use them as additional building blocks. This would explain why all tested cytokinins and purines triggered a similar response. However, it is clear from the cytokinin content measurements that C. scutata is regulating the cytokinin content in its cell as compared to the surrounding media. Furthermore, the low concentrations of cytokinins and purines used in the experiment make it unlikely that they are used just as building blocks for growth. Future experiments replacing the purines adenine and guanine with the pyrimidines cytosine and thymine could determine if the added nitrogenous bases – even in such low concentration – could be used as a building block and cause a change in growth pattern.
2.) The canonical cytokinin signaling pathway is present, but still rather unspecific.
A second possibility is that cytokinin biology in general and cytokinin signaling in particular functions in C. scutata as it does in land plants. The only difference would be in the ligand-binding properties of the receptor. This would fit with a model recently proposed by Lomin and colleagues (Lomin et al., 2021), where cytokinin receptors have been rather unspecific in their binding properties in the beginning of plant evolution. However, in that study, only land plants were investigated. It would be interesting to test the binding properties of the putative cytokinin receptors detected in our analysis (Table 2) or other studies (Wang et al., 2015; De Vries et al., 2018; Nishiyama et al., 2018; Feng et al., 2023).
3.) An alternative pathway of cytokinin signaling.
One could also think of an alternative that has been proposed for phytohormone signaling pathways in general and cytokinin in particular (reviewed by Powell and Heyl, 2023). In this model, the signaling molecule and downstream signaling components existed before the presence of a dedicated receptor. Thus, the hormone might have been perceived by an alternative pathway. Once the complexity of the organism or its environment (e.g., after landfall) demanded a more efficient signaling system a receptor was connected to the existing pathway. During evolution, such a pathway became more specific, and it signals more complex to accommodate the increasing number of programs and processes regulated, Evidence for such a model of phytohormone evolution has been found in different organisms (Kaltenegger et al., 2018 Yasumura et al., 2007; Fürst-Jansen et al., 2020; Hu et al., 2023).
One can imagine that adenine and guanine are picked up by the cells and then converted into cytokinins. However, to our knowledge, no pathway is known that can convert guanine into a type of cytokinin, so this represents a highly unlikely scenario.
In summary, this study starts to shed light on the cytokinin biology of the streptophyte algae C. scutata. It led to surprising results concerning adenine and guanine, which are usually used as negative controls, but show the same effect as classical cytokinins. However, more experimental evidence is needed to understand their role in plant growth regulation in general and the molecular base of the effect of cytokinin on cell division in this streptophyte alga in particular.
Data availability statement
The original contributions presented in the study are included in the article/Supplementary Material. Further inquiries can be directed to the corresponding author.
Author contributions
SP: Conceptualization, Data curation, Investigation, Visualization, Writing – review & editing, Methodology. NT: Conceptualization, Data curation, Investigation, Writing – review & editing, Methodology. KG: Investigation, Resources, Writing – review & editing. PB: Conceptualization, Investigation, Resources, Writing – review & editing. ON: Data curation, Formal Analysis, Investigation, Resources, Writing – review & editing, Writing – original draft. MS: Formal Analysis, Investigation, Resources, Writing – original draft, Writing – review & editing, Supervision. MF: Conceptualization, Formal Analysis, Visualization, Writing – original draft, Writing – review & editing, Investigation, Methodology. AH: Conceptualization, Formal Analysis, Methodology, Project administration, Resources, Supervision, Visualization, Writing – original draft, Writing – review & editing, Investigation.
Funding
The author(s) declare that no financial support was received for the research, authorship, and/or publication of this article.
Acknowledgments
The authors give sincere thanks to Hana Martínková and Petra Amakorová for their help with phytohormone analyses. We also want to thank Cordula Braatz and Dr. John Hunter for their support in handling and maintaining the algal culture. We also want to thank Dr. Burkhard Becker for his help and support in establishing C. scutata as a model organisms in the lab. Sebastien Santini (CNRS/AMU IGS UMR7256) and the PACA Bioinfo platform for the availability and management of the phylogeny.fr website used for the phylogenetic analysis.
Conflict of interest
The authors declare that the research was conducted in the absence of any commercial or financial relationships that could be construed as a potential conflict of interest.
The authors AH and ON declared that they were an editorial board member of Frontiers, at the time of submission. This had no impact on the peer review process and the final decision.
Publisher’s note
All claims expressed in this article are solely those of the authors and do not necessarily represent those of their affiliated organizations, or those of the publisher, the editors and the reviewers. Any product that may be evaluated in this article, or claim that may be made by its manufacturer, is not guaranteed or endorsed by the publisher.
Supplementary material
The Supplementary Material for this article can be found online at: https://www.frontiersin.org/articles/10.3389/fphgy.2023.1275205/full#supplementary-material
References
Aki S. S., Mikami T., Naramoto S., Nishihama R., Ishizaki K., Kojima M., et al. (2019a). Cytokinin signaling is essential for organ formation in marchantia polymorpha. Plant Cell Physiol. 60, 1842–1854. doi: 10.1093/pcp/pcz100
Aki S. S., Nishihama R., Kohchi T., Umeda M. (2019b). Cytokinin signaling coordinates development of diverse organs in Marchantia polymorpha. Plant Signal Behav. 14, 11. doi: 10.1080/15592324.2019.1668232
Aoki M. M., Kisiala A. B., Li S., Stock N. L., Brunetti C. R., Huber R. J., et al. (2019). Cytokinin detection during the dictyostelium discoideum life cycle: Profiles are dynamic and affect cell growth and spore germination. Biomolecules 9, 702. doi: 10.3390/biom9110702
Araújo P. G., Souza J. M. C., Pasqualetti C. B., Yokoya N. S. (2021). Auxin and cytokinin combinations improve growth rates and protein contents in Laurencia catarinensis (Rhodophyta). J. Appl. Phycol. 33, 1071–1079. doi: 10.1007/s10811-020-02334-7
Blázquez M. A., Nelson D. C., Weijers D. (2020). Evolution of plant hormone response pathways. Annu. Rev. Plant Biol. 71, 327–353. doi: 10.1146/annurev-arplant-050718
Bowman J. L., Kohchi T., Yamato K. T., Yotsui I., Zachgo S., Schmutz Correspondence J., et al. (2017). Insights into Land Plant Evolution Garnered from the Marchantia polymorpha Genome. Cell 171, 287–304. doi: 10.1016/j.cell.2017.09.030
Brandstatter I., Kieber J. J. (1998). Two genes with similarity to bacterial response regulators are rapidly and specifically induced by cytokinin in Arabidopsis. Plant Cell 10, 1009–1019. doi: 10.1105/tpc.10.6.1009
Buschmann H. (2020). Into another dimension: How streptophyte algae gained morphological complexity. J. Exp. Bot. 71, 3279–3286. doi: 10.1093/jxb/eraa181
Carpenter E. J., Matasci N., Ayyampalayam S., Wu S., Sun J., Yu J., et al. (2019). Access to RNA-sequencing data from 1,173 plant species: The 1000 Plant transcriptomes initiative (1KP). Gigascience 8 (10), giz126. doi: 10.1093/gigascience/giz126
Delaux P.-M., Radhakrishnan G. V., Jayaraman D., Cheema J., Malbreil M., Volkening J. D., et al. (2015). Algal ancestor of land plants was preadapted for symbiosis. Proc. Natl. Acad. Sci. U.S.A. 112, 201515426. doi: 10.1073/pnas.1515426112
Dereeper A., Guignon V., Blanc G., Audic S., Buffet S., Chevenet F., et al. (2008). Phylogeny.fr: robust phylogenetic analysis for the non-specialist. Nucleic Acids Res. 36, W465–9. doi: 10.1093/nar/gkn180
De Smet I., Voss U., Lau S., Wilson M., Shao N., Timme R. E., et al. (2011). Unraveling the evolution of auxin signaling. Plant Physiol. 155, 209–221. doi: 10.1104/pp.110.168161
de Vries J., Archibald J. M. (2018). Plant evolution: landmarks on the path to terrestrial life. New Phytol. 217, 1428–1434. doi: 10.1111/nph.14975
De Vries J., Curtis B. A., Gould S. B., Archibald J. M. (2018). Embryophyte stress signaling evolved in the algal progenitors of land plants. Proc. Natl. Acad. Sci. U.S.A. 115, E3471–E3480. doi: 10.1073/pnas.1719230115
de Vries J., Rensing S. A. (2020). Gene gains paved the path to land. Nat. Plants 6, 7–8. doi: 10.1038/s41477-019-0579-5
Domozych D. S., Popper Z. A., Sørensen I. (2016). Charophytes: Evolutionary giants and emerging model organisms. Front. Plant Sci. 7. doi: 10.3389/fpls.2016.01470
Dow L., Barrow R. A., White R. G., Mathesius U. (2022). Photolysis of caged cytokinin in single cells of Arabidopsis thaliana. Plant Methods 18, 120. doi: 10.1186/s13007-022-00953-4
Eklund D. M., Kanei M., Flores-Sandoval E., Ishizaki K., Nishihama R., Kohchi T., et al. (2018). An evolutionarily conserved abscisic acid signaling pathway regulates dormancy in the liverwort marchantia polymorpha. Curr. Biol. 28, 3691–3699.e3. doi: 10.1016/j.cub.2018.10.018
Feng X., Zheng J., Irisarri I., Yu H., Zheng B., Ali Z., et al. (2023). Chromosome-level genomes of multicellular algal sisters to land plants illuminate signaling network evolution. bioRxiv [Preprint]. doi: 10.1101/2023.01.31.526407
Frébort I., Kowalska M., Hluska T., Frébortová J., Galuszka P. (2011). Evolution of cytokinin biosynthesis and degradation. J. Exp. Bot. 62, 2431–2452. doi: 10.1093/jxb/err004
Frébortová J., Frébort I. (2021). Biochemical and structural aspects of cytokinin biosynthesis and degradation in bacteria. Microorganisms 9 (6), 1314. doi: 10.3390/microorganisms9061314
Frébortová J., Greplová M., Seidl M. F., Heyl A., Frébort I. (2015). Biochemical characterization of putative adenylate dimethylallyltransferase and cytokinin dehydrogenase from Nostoc sp. PCC 7120. PloS One 10 (9). doi: 10.1371/journal.pone.0138468
Fürst-Jansen J. M. R., De Vries S., De Vries J., De Vries J. (2020). Evo-physio: On stress responses and the earliest land plants. J. Exp. Bot. 71 (11), 3254–3269. doi: 10.1093/jxb/eraa007
Gajdosova S., Spichal L., Kaminek M., Hoyerova K., Novak O., Dobrev P. I., et al. (2011). Distribution, biological activities, metabolism, and the conceivable function of cis-zeatin-type cytokinins in plants. J. Exp. Bot. 62, 2827–2840. doi: 10.1093/jxb/erq457
Goodstein D. M., Shu S., Howson R., Neupane R., Hayes R. D., Fazo J., et al. (2012). Phytozome: A comparative platform for green plant genomics. Nucleic Acids Res. 40, D1178–D1186. doi: 10.1093/nar/gkr944
Graham L. E., Arancibia-Avila P., Taylor W. A., Strother P. K., Cook M. E. (2012). Aeroterrestrial Coleochaete (Streptophyta, Coleochaetales) models early plant adaptation to land. Am. J. Bot. 99, 130–144. doi: 10.3732/ajb.1100245
Gruhn N., Seidl M. F. M. F., Halawa M., Heyl A. (2015). Members of a recently discovered subfamily of cytokinin receptors display differences and similarities to their classical counterparts. Plant Signal Behav. 10, e984512. doi: 10.4161/21659087.2014.984512
Guillory A., Bonhomme S. (2021). Phytohormone biosynthesis and signaling pathways of mosses. Plant Mol. Biol. 107, 245–277. doi: 10.1007/s11103-021-01172-6
Guindon S., Gascuel O. (2003). A simple, fast, and accurate algorithm to estimate large phylogenies by maximum likelihood. Syst. Biol. 52, 696–704. doi: 10.1080/10635150390235520
Guldhe A., Renuka N., Singh P., Bux F. (2019). Effect of phytohormones from different classes on gene expression of Chlorella sorokiniana under nitrogen limitation for enhanced biomass and lipid production. Algal Res. 40, 101518. doi: 10.1016/j.algal.2019.101518
Heyl A., Brault M., Frugier F., Kuderova A., Lindner A.-C. A.-C., Motyka V., et al. (2013). Nomenclature for members of the two-component signaling pathway of plants. Plant Physiol. 161, 1063–1065. doi: 10.1104/pp.112.213207
Heyl A., Ramireddy E., Brenner W. G. G., Riefler M., Allemeersch J., Schmülling T., et al. (2008). The transcriptional repressor ARR1-SRDX suppresses pleiotropic cytokinin activities in arabidopsis. Plant Physiol. 147, 1380–1395. doi: 10.1104/pp.107.115436
Heyl A., Werner T., Schmülling T. (2006). “Cytokinin metabolism and signal transduction,” in Annual plant reviews volume 24: plant hormone signaling. Eds. Hedden P., Thomas S. G. doi: 10.1002/9780470988800.ch4
Hirose N., Takei K., Kuroha T., Kamada-Nobusada T., Hayashi H., Sakakibara H. (2007). Regulation of cytokinin biosynthesis, compartmentalization, and translocation. J. Exp. Bot. 59, 75–83. doi: 10.1093/jxb/erm157
Hluska T., Hlusková L., Emery R. J. N. (2021). The hulks and the deadpools of the cytokinin universe: A dual strategy for cytokinin production, translocation, and signal transduction. Biomolecules 11, 1–40. doi: 10.3390/biom11020209
Hu Y., Shani E. (2023). Cytokinin activity – transport and homeostasis at the whole plant, cell, and subcellular levels. New Phytol. 239 (5), 1603–1608. doi: 10.1111/nph.19001
Hu S., Yu K., Yan J., Shan X., Xie D. (2023). Jasmonate perception: Ligand-receptor interaction, regulation, and evolution. Mol. Plant 16, 23–42. doi: 10.1016/j.molp.2022.08.011
Hutchison C. E., Li J., Argueso C., Gonzalez M., Lee E., Lewis M. W., et al. (2006). The arabidopsis histidine phosphotransfer proteins are redundant positive regulators of cytokinin signaling. Plant Cell 18, 3073–3087. doi: 10.1105/tpc.106.045674
Hwang I., Sheen J. (2001). Two-component circuitry in Arabidopsis cytokinin signal transduction. Nature 413 (6854), 383–389. doi: 10.1038/35096500
Inoue T., Higuchi M., Hashimoto Y., Seki M., Kobayashi M., Kato T., et al. (2001). Identification of CRE1 as a cytokinin receptor from Arabidopsis. Nature 409, 1060–1063. doi: 10.1038/35059117
Ishida K., Yamashino T., Yokoyama A., Mizuno T. (2008). Three type-B response regulators, ARR1, ARR10, and ARR12, play essential but redundant roles in cytokinin signal transduction throughout the life cycle of Arabidopsis thaliana. Plant Cell Physiol. 49, 47–57. doi: 10.1093/pcp/pcm165
Kaltenegger E., Leng S., Heyl A. (2018). The effects of repeated whole genome duplication events on the evolution of cytokinin signaling pathway. BMC Evol. Biol. 18 (1), 76. doi: 10.1186/s12862-018-1153-x
Katoh K., Rozewicki J., Yamada K. D. (2018). MAFFT online service: Multiple sequence alignment, interactive sequence choice, and visualization. Brief Bioinform. 20, 1160–1166. doi: 10.1093/bib/bbx108
Kieber J. J., Schaller G. E. (2010). The perception of cytokinin: a story 50 years in the making. Plant Physiol. 154, 487–492. doi: 10.1104/pp.110.161596
Leebens-Mack J. H., Barker M. S., Carpenter E. J., Deyholos M. K., Gitzendanner M. A., Graham S. W., et al. (2019). One thousand plant transcriptomes and the phylogenomics of green plants. Nature 574, 679–685. doi: 10.1038/s41586-019-1693-2
Leliaert F., Smith D. R., Moreau H., Herron M. D., Verbruggen H., Delwiche C. F., et al. (2012). Phylogeny and molecular evolution of the green algae. CRC Crit. Rev. Plant Sci. 31, 1–46. doi: 10.1080/07352689.2011.615705
Lindner A.-C., Lang D., Seifert M., Podle akova K., Novak O., Strnad M., et al. (2014). Isopentenyltransferase-1 (IPT1) knockout in Physcomitrella together with phylogenetic analyses of IPTs provide insights into the evolution of plant cytokinin biosynthesis. J. Exp. Bot. 65, 2533–2543. doi: 10.1093/jxb/eru142
Lomin S. N., Savelieva E. M., Arkhipov D. V., Pashkovskiy P. P., Myakushina Y. A., Heyl A., et al. (2021). Cytokinin perception in ancient plants beyond angiospermae. Int. J. Mol. Sci. 22, 13077. doi: 10.3390/ijms222313077
Mason M. G., Li J., Mathews D. E., Kieber J. J., Schaller G. E. (2004). Type-B response regulators display overlapping expression patterns in Arabidopsis. Plant Physiol. 135, 927–937. doi: 10.1104/pp.103.038109
Matsumoto-Kitano M., Kusumoto T., Tarkowski P., Kinoshita-Tsujimura K., Václavíková K., Miyawaki K., et al. (2008). Cytokinins are central regulators of cambial activity. Proc. Natl. Acad. Sci. U.S.A. 105, 20027–20031. doi: 10.1073/pnas.0805619105
McCourt R. M., Lewis L. A., Strother P. K., Delwiche C. F., Wickett N. J., de Vries J., et al. (2023). Green land: Multiple perspectives on green algal evolution and the earliest land plants. Am. J. Bot. 110 (5), e16175. doi: 10.1002/ajb2.16175
McFadden G. I., Melkonian M. (1986). Use of Hepes buffer for microalgal culture media and fixation for electron microscopy. Phycologica 25, 551557. doi: 10.2216/i0031-8884-25-4-551.1
Mistry J., Chuguransky S., Williams L., Qureshi M., Salazar G. A., Sonnhammer E. L. L., et al. (2021). Pfam: The protein families database in 2021. Nucleic Acids Res. 49, D412–D419. doi: 10.1093/nar/gkaa913
Morris J. L., Puttick M. N., Clark J. W., Edwards D., Kenrick P., Pressel S., et al. (2018). The timescale of early land plant evolution. Proc. Natl. Acad. Sci. U.S.A. 115 (10), E2274–E2283. doi: 10.1073/pnas.1719588115
Nishiyama T., Sakayama H., de Vries J., Buschmann H., Saint-Marcoux D., Ullrich K. K., et al. (2018). The chara genome: secondary complexity and implications for plant terrestrialization. Cell 174 (2), 448–464.e24. doi: 10.1016/j.cell.2018.06.033
Novák O., Hauserová E., Amakorová P., Doležal K., Strnad M. (2008). Cytokinin profiling in plant tissues using ultra-performance liquid chromatography–electrospray tandem mass spectrometry. Phytochemistry 69, 2214–2224. doi: 10.1016/j.phytochem.2008.04.022
Ördög V., Stirk W. A., Van Staden J., Novák O., Strnad M. (2004). Endogenous cytokinins in three genera of microalgae from the chlorophyta1. J. Phycol. 40, 88–95. doi: 10.1046/j.1529-8817.2004.03046.x
Osugi A., Kojima M., Takebayashi Y., Ueda N., Kiba T., Sakakibara H. (2017). Systemic transport of trans-zeatin and its precursor have differing roles in Arabidopsis shoots. Nat. Plants 3, 17112. doi: 10.1038/nplants.2017.112
Pils B., Heyl A. (2009). Unraveling the evolution of cytokinin signaling. Plant Physiol. 151, 782–791. doi: 10.1104/pp.109.139188
Piotrowska-Niczyporuk A., Bajguz A., Kotowska U., Bralska M., Talarek-Karwel M. (2018). Growth, metabolite profile, oxidative status, and phytohormone levels in the green alga acutodesmus obliquus exposed to exogenous auxins and cytokinins. J. Plant Growth Regul. 37, 1159–1174. doi: 10.1007/s00344-018-9816-9
Piotrowska-Niczyporuk A., Bajguz A., Kotowska U., Zambrzycka-Szelewa E., Sienkiewicz A. (2020). Auxins and cytokinins regulate phytohormone homeostasis and thiol-mediated detoxification in the green alga acutodesmus obliquus exposed to lead stress. Sci. Rep. 10 (1), 10193. doi: 10.1038/s41598-020-67085-4
Powell A. E., Heyl A. (2023). The origin and early evolution of cytokinin signaling. Front. Plant Sci. 14. doi: 10.3389/fpls.2023.1142748
Punwani J., Hutchison C. E., Schaller G. E., Kieber J. J. (2010). The subcellular distribution of the Arabidopsis histidine phosphotransfer proteins is independent of cytokinin signaling. Plant J. 62, 473–482. doi: 10.1111/j.1365-313X.2010.04165.x
R Core Team. (2023). R: a language and environment for statistical computing. Vienna, Austria: R Foundation for Statistical Computing. Available at: https://www.R-project.org/.
Renuka N., Guldhe A., Singh P., Ansari F. A., Rawat I., Bux F. (2017). Evaluating the potential of cytokinins for biomass and lipid enhancement in microalga Acutodesmus obliquus under nitrogen stress. Energy Convers Manag 140, 14–23. doi: 10.1016/j.enconman.2017.02.065
Rittenberg D., Foster G. L. (1940). A new procedure for quantitative analysis by isotope dilution, with application to the determination of amino acids and fatty acids. J. Biol. Chem. 133, 737–744. doi: 10.1016/S0021-9258(18)73304-8
Ronquist F., Teslenko M., van der Mark P., Ayres D. L., Darling A., Höhna S., et al. (2012). Mrbayes 3.2: Efficient Bayesian phylogenetic inference and model choice across a large model space. Syst. Biol. 61, 539–542. doi: 10.1093/sysbio/sys029
Schaller G. E., Bishopp A., Kieber J. J. (2015). The yin-yang of hormones: cytokinin and auxin interactions in plant development. Plant Cell 27, 44–63. doi: 10.1105/tpc.114.133595
Singh J., Jain D., Agarwal P., Singh R. P. (2020). Auxin and cytokinin synergism augmenting biomass and lipid production in microalgae Desmodesmus sp. JS07. Process Biochem. 95, 223–234. doi: 10.1016/j.procbio.2020.02.012
Skoog F., Hamzi H. Q., Szweykowska A. M., Leonard N. J., Carraway K. L., Fujii T., et al. (1967). Cytokinins: structure/activity relationships. Phytochemistry 6, 1169–1192. doi: 10.1016/S0031-9422(00)86080-X
Stirk W., Novák O., Strnad M., Van Staden J. (2003). Cytokinins in macroalgae. Plant Growth Regul. 41, 13–24. doi: 10.1023/A:1027376507197
Stirk W. A., Ördög V., Novák O., Rolčík J., Strnad M., Bálint P., et al. (2013). Auxin and cytokinin relationships in 24 microalgal strains 1. J. Phycol. 49, 459–467. doi: 10.1111/jpy.12061
Sun Y., Harpazi B., Wijerathna-Yapa A., Merilo E., de Vries J., Michaeli D., et al. (2019). A ligand-independent origin of abscisic acid perception. Proc. Natl. Acad. Sci. U. S. A. 116 (49), 24892–24899. doi: 10.1073/pnas.1914480116
Talavera G., Castresana J. (2007). Improvement of phylogenies after removing divergent and ambiguously aligned blocks from protein sequence alignments. Syst. Biol. 56, 564–577. doi: 10.1080/10635150701472164
To J. P. C. J., Haberer G., Ferreira F. F. J., Deruère J., Mason M. G., Schaller G. E., et al. (2004). Type-A Arabidopsis response regulators are partially redundant negative regulators of cytokinin signaling. Plant Cell 16, 658–671. doi: 10.1105/tpc.018978.2
Von Schwartzenberg K., Lindner A. C., Gruhn N., Šimura J., Novák O., Strnad M., et al. (2016). CHASE domain-containing receptors play an essential role in the cytokinin response of the moss Physcomitrella patens. J. Exp. Bot. 67, 667–679. doi: 10.1093/jxb/erv479
von Schwartzenberg K., Núñez M. F., Blaschke H., Dobrev P. I., Novák O., Motyka V., et al. (2007). Cytokinins in the bryophyte Physcomitrella patens: analyses of activity, distribution, and cytokinin oxidase/dehydrogenase overexpression reveal the role of extracellular cytokinins. Plant Physiol. 145, 786–800. doi: 10.1104/pp.107.103176
Wang X., Lin S., Liu D., Gan L., McAvoy R., Ding J., et al. (2020). Evolution and roles of cytokinin genes in angiosperms 1: Do ancient IPTs play housekeeping while non-ancient IPTs play regulatory roles? Hortic. Res. 7, 28. doi: 10.1038/s41438-019-0211-x
Wang C., Liu Y., Li S. S., Han G. Z. (2015). Insights into the origin and evolution of the plant hormone signaling machinery. Plant Physiol. 167, 872–886. doi: 10.1104/pp.114.247403
West A. H., Stock A. M., West A. H. (2001). Histidine kinases and response regulator proteins in two-component signaling systems. Trends Biochem. Sci. 26, 369–376. doi: 10.1016/S0968-0004(01)01852-7
Yang J. F., Chen M. X., Zhang J. H., Hao G. F., Yang G. F. (2020). Genome-wide phylogenetic and structural analysis reveals the molecular evolution of the ABA receptor gene family. J. Exp. Bot. 71, 1322–1336. doi: 10.1093/jxb/erz511
Yang W., Cortijo S., Korsbo N., Roszak P., Schiessl K., Gurzadyan A., et al. (2021). Molecular mechanism of cytokinin-activated cell division in Arabidopsis. Science 371 (6536), 1350–1355. doi: 10.1126/science.abe2305
Yasumura Y., Crumpton-Taylor M., Fuentes S., Harberd N. P. (2007). Step-by-step acquisition of the gibberellin-DELLA growth-regulatory mechanism during land-plant evolution. Curr. Biol. 17, 1225–1230. doi: 10.1016/j.cub.2007.06.037
Žižková E., Kubeš M., Dobrev P. I., Přibyl P., Šimura J., Zahajská L., et al. (2017). Control of cytokinin and auxin homeostasis in cyanobacteria and algae. Ann. Bot. 119, 151–166. doi: 10.1093/aob/mcw194
Zürcher E., Liu J., di Donato M., Geisler M., Müller B. (2016). Plant development regulated by cytokinin sinks. Science 353 (6303), 1027–1030. doi: 10.1126/science.aaf7254
Keywords: cytokinin, C. scutata, signal transduction, evolution, phytohormone, adenine
Citation: Pinto S, Tajeshwar N, Gordon K, Borrero P, Novák O, Strnad M, Foellmer M and Heyl A (2023) Cytokinin Response of the Streptophyte Alga Coleochaete scutata provides a clue to the evolution of cytokinin signaling. Front. Plant Physiol. 1:1275205. doi: 10.3389/fphgy.2023.1275205
Received: 09 August 2023; Accepted: 06 November 2023;
Published: 18 December 2023.
Edited by:
Patrick Laufs, Institut National de Recherche pour l’Agriculture, l’Alimentation et l’Environnement (INRAE), FranceReviewed by:
Anish Malladi, University of Georgia, United StatesMingyuan Zhu, Duke University, United States
Copyright © 2023 Pinto, Tajeshwar, Gordon, Borrero, Novák, Strnad, Foellmer and Heyl. This is an open-access article distributed under the terms of the Creative Commons Attribution License (CC BY). The use, distribution or reproduction in other forums is permitted, provided the original author(s) and the copyright owner(s) are credited and that the original publication in this journal is cited, in accordance with accepted academic practice. No use, distribution or reproduction is permitted which does not comply with these terms.
*Correspondence: Alexander Heyl, YWhleWxAYWRlbHBoaS5lZHU=
†ORCID: Sandra Pinto, orcid.org/0009-0002-1372-9194
Navindra Tajeshwar, orcid.org/0009-0002-8109-2463
Kyana Gordon, orcid.org/0000-0001-6921-9025
Alexander Heyl, orcid.org/0000-0002-8782-1260