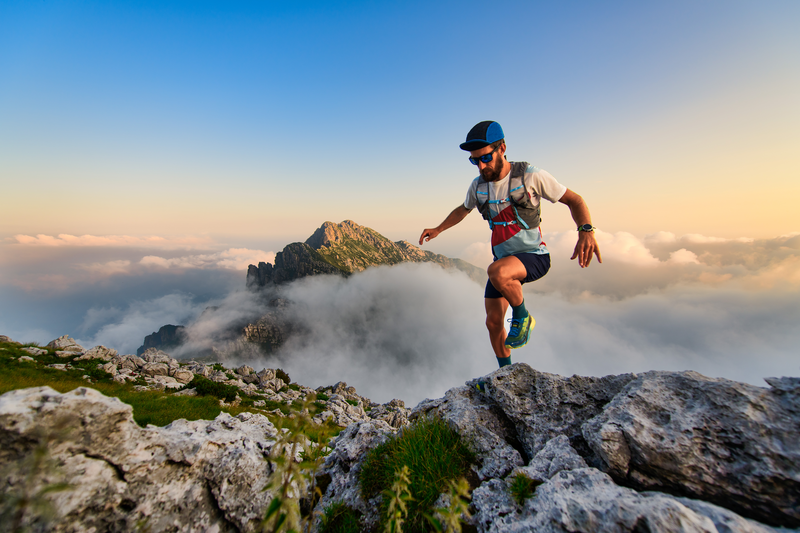
95% of researchers rate our articles as excellent or good
Learn more about the work of our research integrity team to safeguard the quality of each article we publish.
Find out more
REVIEW article
Front. Pharmacol. , 27 January 2025
Sec. Neuropharmacology
Volume 16 - 2025 | https://doi.org/10.3389/fphar.2025.1544795
This article is part of the Research Topic Neuropharmacological Intervention for Severe Mental Illness and Suicide Prevention View all 11 articles
Mood disorders, such as major depressive disorder and bipolar disorder, are among the most common mental illnesses and a leading cause of disability worldwide. Key symptoms of these conditions include a depressed mood or anhedonia, sleep and psychomotor disturbances, changes in appetite or weight, and fatigue or loss of energy. Prolonged cognitive disturbances further impair the ability to think or concentrate and are often accompanied by persistent feelings of worthlessness or excessive guilt. Collectively, these symptoms underscore depression as a serious, long-term global health issue. In addition, clinical studies indicate a growing number of patients experiencing difficulties in responding to treatment, even in the long term. This phenomenon poses significant challenges for healthcare professionals, families, and patients alike. As a result, there is an urgent need for therapies that are both rapid-acting and safe. This review aims to summarize the prevailing trends in research on novel antidepressants, emphasizing their diversity and multi-directional mechanisms of action. The development of rapid-acting drugs is increasingly focused on achieving high efficacy, particularly for treatment-resistant depression. Such advances offer the potential for rapid therapeutic effects without the prolonged and often tedious administration of older generation antidepressants. Findings from studies using animal models of depression continue to play a crucial role in predicting and designing new therapeutic strategies. These models remain indispensable for understanding the physiological effects of newly developed compounds, thereby guiding the creation of innovative treatments.
Major depressive disorder (MDD) is a common and growing global health concern, affecting over 264 million people, with an estimated lifetime risk of 15%–18% (Murray et al., 2012; Bromet et al., 2011). Unlike short-lived, everyday mood fluctuations, depression represents a severe and persistent health condition. Patients report many comorbidities that reduce quality of life and life expectancy (Berk et al., 2023).
According to the DSM-5 (Diagnostic and Statistical Manual of Mental Disorders, Fifth Edition), a diagnosis of a major depressive episode requires the presence of five or more symptoms for at least 2 weeks. Key symptoms include a depressed mood or anhedonia, sleep and psychomotor disturbances (agitation or retardation), changes in appetite or weight, and fatigue or loss of energy. Cognitive impairments, such as difficulties with concentration or decision-making, often accompany persistent feelings of worthlessness or excessive guilt.
The World Health Organization (WHO), in the 11th revision of the International Classification of Diseases and Related Health Problems (ICD-11), conceptualizes depression as a syndrome characterized by a clinically recognizable set of reported experiences (symptoms) and observed behaviors (signs) associated with distress and impaired functioning (Cuijpers et al., 2023; International Advisory Group for the Revision of ICD-10 Mental and Behavioural Disorders, 2011). At its most severe, depression can lead to suicide, which the WHO identifies as the second leading cause of death among individuals aged 15–29, accounting for approximately 800,000 deaths annually (Hawkins et al., 2018; Jensen et al., 2023; Murphy et al., 2021).
The discovery and widespread use of the first antidepressants revolutionized depression treatment. Grounded in the widely accepted monoamine theory of depression, first- and second-generation antidepressants, including serotonin and norepinephrine reuptake inhibitors, provided significant insights into mechanisms of action and treatment options. However, clinical trials have shown that these compounds are not equally effective in patients (Nunez et al., 2022; Salzman, 1996; Price et al., 1987). Furthermore, they often require weeks or months to achieve full effectiveness, underscoring the need for more effective and rapid-acting treatments for MDD (U.S. Food and Drug Administration (FDA) 2021; European Medicines Agency (EMA) 2013; Rush 2007; Mourilhe and Stokes, 1998).
In addition, one of the major challenges in treating depression is that many people who are prescribed antidepressants do not fully respond to pharmacotherapy. About 30% of patients treated for major depression do not respond satisfactorily to initial treatment. A significant number of patients have a poor prognosis at follow-up, with up to 20% still affected 2 years after onset (Fava et al., 2003). The STAR*D trial reported that one in three patients with unipolar depression did not achieve symptomatic remission after multiple antidepressant trials (Trivedi et al., 2006), and patients with bipolar depression did not benefit from antidepressant treatment combined with mood stabilizers (Bowden et al., 2012). These data indicate that a significant number of patients experience a phenomenon known as treatment-resistant depression (TRD) or refractory major depressive disorder (Berlim and Turecki, 2007; Tundo et al., 2023; Fu et al., 2024). Several authors have suggested distinguishing between difficult-to-treat and treatment-resistant depression on the basis of the longitudinal course of the illness. However, the common distinction indicates a clinical state resulting from a lack of response to treatment. As evidenced by the fact that several definitions have been proposed, the construct of TRD is very heterogeneous (Murphy et al., 2021).
Current treatments for MDD are predominantly based on the monoaminergic system, as the monoamine hypothesis of depression suggests that a decrease in serotonin, norepinephrine, and dopamine levels in the central nervous system underlies the condition’s pathophysiology. The effectiveness of antidepressants supports this hypothesis, as drugs that increase the levels of these neurotransmitters in the brain have consistently been shown to reduce symptoms of depression.
Four main classes of monoamine-targeting antidepressants have been developed: first-generation antidepressants (monoamine oxidase inhibitors [MAOIs] and tricyclic antidepressants [TCAs]), second-generation antidepressants (selective serotonin reuptake inhibitors [SSRIs] and serotonin-norepinephrine reuptake inhibitors [SNRIs]), and atypical antidepressants. Among these, SSRIs are the most commonly used today, as they primarily address the hypoactivity of monoamine neurotransmitter systems (Sharp and Collins, 2023). Guidelines from the National Institute for Health and Care Excellence (Leichsenring et al., 2023) and the American Psychiatric Association (APA, 2019) recommend selective serotonin reuptake inhibitors (SSRIs), among other options, as first-line treatments for moderate to severe major depression. They are generally better tolerated and safer in overdose situations compared to TCAs and other antidepressants like noradrenergic and specific serotonin antidepressants or MAOIs (Olfson and Marcus, 2009; NHS Information Centre, 2011). However, an important limitation of all these treatments is their delayed onset of action, often taking 3 weeks or more to produce noticeable effects. During this time, especially in younger populations, SSRIs may exacerbate pre-existing anxiety or suicidality (Walter et al., 2022; Jack et al., 2023; Lagerberg et al., 2023).
Antidepressants acting via the monoaminergic system include partial agonists targeting serotonin receptors such as 5-HT1A, 5-HT1B, 5-HT2A, 5-HT2C, 5-HT4, and 5-HT7. Other mechanisms involve serotonin transporter inhibitors, 5-HT1A receptor “biased” agonists, triple monoamine reuptake inhibitors, and MAO-A inhibitors. Psychedelics, such as psilocin, also act within this system (Lochmann and Richardson, 2018; Weilburg, 2004; Papp et al., 2024). Psilocin, the most extensively studied psychedelic, primarily targets serotonin receptors but ultimately enhances glutamate release and sustained excitatory neurotransmission in pyramidal neurons. This promotes a prolonged state of enhanced neural plasticity in corticolimbic circuits, a key factor in its antidepressant effects.
The antidepressant effects of psilocin are thought to be mediated through activation of the 5-HT2A-mGlu2 receptor complex, which is also crucial for the hallucinogenic behaviors induced by 5-HT2A receptor agonists (López-Giménez and González-Maeso, 2018; Erritzoe et al., 2024). Activation of 5-HT2A receptors promotes glutamate release in pyramidal neurons of the prefrontal cortex, leading to both antidepressant and anxiolytic effects. Research on mitigating the adverse acute effects of psilocybin suggests these effects are related to the occupancy of 5-HT2A receptors (Madsen et al., 2019; Lagerberg et al., 2023). Interestingly, chronic administration of SSRIs or SNRIs has been shown to lead to downregulation of 5-HT2A receptors in both animal and human studies (Kumar et al., 2019; Klimek et al., 1994; Meyer et al., 2001).
Among newer, faster-acting pharmacological treatment strategies, those targeting the glutamatergic and GABAergic systems have shown considerable promise for the treatment of MDD and TRD. A particularly notable example is (R,S)-ketamine, whose rapid improvement in depressive symptoms after administration (Swainson et al., 2019; Berman et al., 2000; Barbara et al., 2024) has significantly renewed interest in compounds that modulate glutamatergic receptors, sparking a wave of research and drug development in this area. (R,S)-Ketamine acts as an NMDA receptor antagonist. Its S (+) isomer, esketamine, has been approved in the European Union, United Kingdom, and United States for adults with TRD and for the rapid reduction of depressive symptoms in psychiatric emergencies related to MDD. Ketamine’s effects, while mechanistically distinct from those of psilocin, share similarities in their activation of intracellular signaling pathways. These pathways produce long-lasting changes in synaptic function and morphology (neuroplasticity), proposed as a common mechanism underlying the therapeutic effects of ketamine and classical psychedelics (Aleksandrova and Phillips, 2021). While newly developed neuroplasticity-based strategies do not directly target glutamate modulation, they focus on activating the intracellular mammalian target of rapamycin complex 1 (mTORC1) signaling cascade. This pathway links glutamate modulation to the induction of neuroplastic changes.
Specific enantiomers, racemic mixtures or metabolites of ketamine have different affinities for NMDAR and have shown antidepressant activity in preclinical and clinical studies. Metabolites of ketamine such as S-norketamine, 2S,6S-hydroxynorketamine, 2S,6R-hydroxynorketamine and 2R,6S-hydroxynorketamine have shown antidepressant-like effects in animal models (Zanos et al., 2016; Yokoyama et al., 2020). Differences between (R)-ketamine and (S)-ketamine have been observed at behavioural and biochemical levels. Some preclinical studies also show that (R)-ketamine has stronger and longer-lasting effects and is safer than ketamine or (S)-ketamine. That suggest that (R)-ketamine could be a promising molecule and, like some metabolites, should be tested in clinical trials (Pochwat et al., 2022).
Among the many compounds under investigation, NMDA and metabotropic glutamate receptor antagonists and negative allosteric modulators appear particularly promising due to their ability to inhibit glutamatergic neurotransmission. These include broad-spectrum glutamatergic modulators, like some of the non-NMDA receptor agonists such as glycine site NMDAR partial agonists, NR2B antagonists, non-selective low-trapping NMDAR channel blockers or non-competitive NMDAR antagonists are active in models of depression (Machado-Vieira et al., 2017). NMDAR antagonists alone or as an adjunct to imipramine/SSRIs have produced antidepressant effects in animal models of depression (Poleszak et al., 2011).
Regarding metabotropic glutamate receptors, all three groups show antidepressant effects, especially under experimental conditions. For group I mGlu receptors, promising ligands include mGlu1 receptor antagonists, mGlu5 and partial mGlu5 receptor negative allosteric modulators, but also mGlu5 receptor inverse agonists. For group II mGlu receptors, antidepressant-like activity has been demonstrated by mGlu2/3 receptor agonists, mGlu2/3 receptor antagonists, and mGlu2/3 and selective mGlu2 and mGlu3 receptor negative allosteric modulators. To a lesser extent, group III mGlu receptors are being investigated. Group III mGlu receptor agonists, mGlu7 receptor negative allosteric modulators, mGlu7 receptor allosteric agonists and mGlu8 receptor agonists (Dogra and Conn, 2021). As shown, metabotropic glutamate receptor ligands excert antidepressant potential and, as some suggest, could be used as adjuncts to reduce the side effects of rapid-acting antidepressants (discussed in Pochwat et al., 2022).
Some experimental compounds exhibit intriguing multimodal mechanisms of action, including NMDA receptor antagonism combined with α-1 adrenergic receptor agonism. Additionally, a positive allosteric GABAA modulator is currently in phase III clinical trials (Clayton et al., 2023). It should be emphasised that a large number of NMDAR antagonists are effective in preclinical models of depression, but have been unsuccessful in clinical trials (Kishimoto et al., 2016). However novel glutamatergic modulators for the treatment of mood disorders encompass a diverse range of approaches. These advances are yielding new insights and opportunities for the development of rapid-acting treatments for MDD (for reviews, see Henter et al., 2021; Serretti, 2024).
Treatment-resistant depression is a significant clinical challenge. While some studies report that over 35% of patients with depression are resistant to antidepressant treatment (Nemeroff, 2007; Thase, 2011; Thomas et al., 2013), more recent data highlight an even greater burden. According to Heerlein et al. (2021); Heerlein et al. (2022), approximately 69% of patients with difficult-to-treat depression in Europe did not respond to treatment within 1 year. Despite low remission rates, 60% of these patients remained on unchanged treatment regimens for extended periods. Some studies reported that in some populations even one-fourth of all depressive patients are affected by TRD (Gałecki et al., 2022). Mortality, particularly due to suicide and accidents, as well as comorbid conditions, is significantly higher in TRD patients (Döme et al., 2021; Reutfors et al., 2018; DiBernardo et al., 2018). Non-lethal self-harm is also more frequent in TRD patients compared to those without this condition (Parker and Graham, 2015). Furthermore, TRD is associated with worse disease outcomes and elevated rates of general and psychiatric comorbidities relative to other patients treated for depression.
Among recently introduced TRD treatments, esketamine has a special place. Recent studies confirm the efficacy, safety and safety and tolerability of esketamine in adults with TRD. In patients with comorbidities in the REAL-ESK study reported the later response, as well as the non-inferiority in effectiveness present novel and interesting findings (Martinotti et al., 2022). It should be noted that esketamine is only approved for TRD and emergency suicidality. It represents an important novelty in the pharmacological treatment of patients with depression, but its specific mechanism of action may be associated with craving behaviour and additional addictive potential (Leichsenring et al., 2023). A narrative review of the available literature on the most common clinical “misconceptions” and “stereotypes” about esketamine has recently been presented by Di Vincenzo et al. (2024).
Despite ongoing research, there is no universally effective treatment strategy for TRD. Current guidelines recommend several approaches, including dose optimization, switching antidepressants, or augmenting therapy with other pharmacological agents (Gabriel et al., 2023; Davies et al., 2019). The high heterogeneity in the treatment of patients with TRD and the urgent need for standardised strategies and treatments specifically approved for TRD are highlighted. In addition to esketamine, some new studies describe the benefits of adding lithium or antipsychotics to adjuvant therapies for patients with TRD (Maina et al., 2023). Strong recommendations also exist for disorder-specific psychological therapies, such as cognitive analytic therapy, cognitive behavioral therapy (including internet-based CBT), and interpersonal therapy for depression (Ijaz et al., 2018). However, long-term treatment often proves discouraging for both patients and their families, emphasizing the urgent need for alternative therapeutic approaches.
The latest guidelines advocate for first addressing pseudo-resistance by ensuring adherence to treatment and optimizing antidepressant dose and duration. For combination therapies, the pairing of monoamine reuptake inhibitors with presynaptic α2-adrenoceptor antagonists has shown promise in improving efficacy in TRD patients. Additionally, targeting the opioid system with µ-receptor agonists, mixed (µ/ĸ) receptor agonists, or ĸ-receptor antagonists may provide novel avenues for treatment (Borbely et al., 2021). Agonism of the 5-HT1A receptor is another promising strategy for achieving rapid and sustained therapeutic effects in TRD (Smith et al., 2023; Papp et al., 2023).
Among augmentation strategies, second-generation antipsychotics and lithium remain the most evidence-supported options for managing TRD. Novel therapies, particularly those targeting the glutamatergic system, have garnered significant attention. Intravenous ketamine and intranasal S-ketamine are at the forefront of these developments. Since intranasal S-ketamine was approved by the U.S. FDA and the EMA in 2019, it has been introduced as a novel adjunctive therapy for TRD with specific indications and under laboratory control. These therapies offer hope for addressing the limitations of traditional treatment approaches. We identified three primary clinical approaches to optimize treatment for TRD: neurostimulation therapy, metabolic modulation, and ion level modulation.
Neuromodulation is a growing area of research interest based on clinical and preclinical reports (Hamani and Nóbrega, 2010; Figee et al., 2022; Papp et al., 2018). Non-invasive techniques include electroconvulsive therapy (ECT), magnetic seizure therapy (MST), transcranial direct current stimulation (tDCS), and repetitive transcranial magnetic stimulation (rTMS). Invasive procedures include neurosurgical vagus nerve stimulation (VNS) and deep brain stimulation (DBS), in which electrodes are implanted in discrete brain targets under MRI guidance. For affective disorders, as recommended by the Canadian Agency for Drugs and Technologies in Health, 2014, DBS for MDD is still under investigation. Acute and maintenance efficacy, as well as safety and tolerability, were classified at level 3 in 2016 (Milev et al., 2016). This classification means that recommendations are based primarily on epidemiological or risk factor studies, observational studies, randomized controlled trials with small samples, non-randomized controlled prospective studies or case series, or high-quality retrospective studies.
The unadaptable stress response with the prolonged energy mobilization promotes dysglycaemia and insulin resistance that in turn alter mitochondrial structure and function generating oxidative stress, and inflammation leading to cellular damage (Picard et al., 2014). This mechanism likely underlies distinguished recently metabolic subtype of MDD (Krupa et al., 2023) and justifies the frequent co-occurrence of type 2 diabetes (TMD2) and depression (Guerrero Fernández de Alba et al., 2020). Insulin resistance has been considered as a factor responsible for more severe depressive symptoms and as a predictor of lack of response to antidepressant drugs. It should be noted that both in the clinics and preclinical studies the insulin-sensitizing interventions improved the effectiveness of antidepressive treatments. The antidepressive mechanism of insulin applied in combined MDD therapy seems to be related to the direct modulatory tone of insulin into serotoninergic and dopaminergic neurotransmission, which is known to be disrupted in patients with insulin resistance (see Krupa et al., 2023).
Cellular metabolic overactivity during exposure to stressor(s) augments the cellular (mainly mitochondrial) production and accumulation of oxygen reactive species (ROS) which in physiological state are neutralized by antioxidant defensive system primarily consisting of superoxide dismutase (SOD), catalase (CAT), glutathione peroxidase (GPX), glutathione reductase (GR), and glutathione S-transferase (GST) enzymes. Oxidative stress, defined as an imbalance between production and accumulation of ROS in cells and tissues is considered as an important factor implicated in the pathomechanism of MDD (Bell et al., 2024; Lievanos-Ruiz and Fenton-Navarro, 2024; Correia et al., 2023). Recent data, performed by proton magnetic resonance spectroscopy methodology revealed reduced levels of GSH in the occipital region of the cortex of patients with MDD (Bell et al., 2024). Although the precise pattern of depression evoked changes in the level and/or function of the antioxidant defensive system is not clear, strategies reducing the oxidative stress components seem to be effective in the reduction of depressive symptoms in the clinics (Bajpai, 2014; Zhang et al., 2022) and in the preclinical studies (see Correia et al., 2023).
All biochemical processes necessary for the proper intracellular signal transduction, protein synthesis, activity, and other metabolic pathways require homeostasis of trace elements including zinc (Zn2+), magnesium (Mg2+), calcium (Ca2+), copper (Cu2+), iron (Fe2+), and manganese (Mn2+) ions (Meng et al., 2024). The monitoring of trace elements may serve as a diagnostic panel and provide information about the effectiveness of antidepressive therapy applied to patients. Clinical observations regarding the serum levels of trace elements in depression patients pointed out decreased Zn2+, and Mg2+ serum levels as potential markers of depression and evidenced the beneficial effects of Zn2+, and Mg2+ supplementation in the treatment or prevention of depression (Szewczyk et al., 2018). Moreover, in the case of TRD, Zn2+ supplementation was shown to augment the efficacy of antidepressant pharmacotherapy (Siwek et al., 2010; Styczeń et al., 2016). Multiple preclinical studies supported clinical observation regarding the role of Zn2+, and Mg2+ deficiency in the expression of depressive-like behaviours (Szewczyk et al., 2018). Regarding the potential antidepressive mechanism of Zn2+, its role in direct activation of metabotropic GPR39 receptor is postulated, and modulation of GPR39 downstream CREB/BDNF/TrkB signalling and related neuroplasticity (Meng et al., 2024). In turn, antidepressive properties of Mg2+ seem to be related mainly to the blockade of the NMDA receptors overactivated in the forebrain in the presence of physical or anticipated stressors (Zelek-Molik et al., 2025). Additionally, Mg2+'s role in the regulation of the HPA axis, inflammation and oxidative stress, regulation of the glutamatergic, 5HT, dopamine and norepinephrine signalling and the sleep-wake cycle has been recognized. Available data suggest that neither depression nor antidepressant treatment affects the Fe2+ and Mn2+ levels. However, the concentration of Ca2+ and Cu2+ in the blood and brain of MDD patients was shown to be upregulated. Moreover, a positive correlation between the patient’s severity of depression and Ca2+ and Cu2+ levels was detected (Meng et al., 2024). Typically, the antidepressive treatment with SSRIs was shown to alleviate the augmented level of Cu2+, however, treatment with lithium additionally increased Ca2+ concentration and may lead to hypercalcemia, recognized as a harmful side effect after lithium treatment.
To assess the latest approaches in studying molecular targets and testing antidepressive strategies in preclinical models of depression, we performed a literature search using the PubMed database with keywords such as “depressive-like behavior” and “pharmacotherapy”. This search yielded 191 review articles published within the last 5 years up to September 2024. After excluding irrelevant publications, non-English articles, and those restricted by open-access limitations, 64 scientific articles were selected, each describing currently tested targets and potential pharmacotherapies for depression.
Among the selected articles, a significant portion (43 articles) focused on the modulation of neuroinflammation, gut-brain axis activity, and BDNF related neuroplasticity processes in the forebrain. Additionally, ten articles explored the therapeutic potential of bioactive plant compounds, reflecting the growing interest in leveraging the therapeutic properties of plants in medicine.
Below, we summarize the most relevant findings from these reviews and provide references to the literature where readers can find detailed analyses and links to original studies.
Impaired inflammatory responses have been identified as a significant factor in the pathomechanism of depression (e.g., Wang et al., 2024; Bielawski et al., 2023; Belzeaux et al., 2012). As mentioned above, depression-related neuroinflammation is connected to the excessive metabolic processes and oxidative stress occurrence (e.g., Correia et al., 2023). Treatment-resistant depression (TRD) and depressive-like behavior are associated with glial dysregulation, particularly in the frontal cortex and hippocampus (Sanadgol et al., 2023; Fang et al., 2023; Bansal et al., 2024; Furuyashiki et al., 2019). This dysregulation manifests as elevated levels of proinflammatory cytokines (Il1β, Il6, TNFα) in the blood and brain and overactivation of immune receptors (TNFR1 and TLR-4). These changes lead to the upregulation of the NF-κB pathway, heightened inflammatory responses, and depressive-like symptoms (Sanadgol et al., 2023; Sharma et al., 2024).
Additionally, the synthesis of proinflammatory lipid mediators such as prostaglandins and cysteinyl leukotrienes, linked to abnormal arachidonic acid turnover in the brain, has been observed in depressive-like behavior (Furuyashiki et al., 2019). Available antidepressants are largely ineffective in reducing neuroinflammation in TRD patients (Belzeaux et al., 2012) and in preclinical models (Duda et al., 2017; 2019), emphasizing the need to identify new therapeutic targets for depression-induced neuroinflammation.
One promising approach is targeting the NLRP3 inflammasome and its P2X7 receptor, which regulate the release of proinflammatory cytokines and are under investigation as potential antidepressive pharmacotherapy targets (Mokhtari and Uludag, 2023; Roy et al., 2023; Qi et al., 2023). Animal models have also shown that depressive-like behavior is accompanied by increased activity of fatty acid amide hydrolase (FAAH), an enzyme in the endocannabinoid system. FAAH inhibitors have demonstrated therapeutic potential without the side effects associated with direct CB1 agonists (Rafiei and Kolla, 2021). Furthermore, studies have revealed that increasing omega-3 fatty acid intake or its derivatives, such as resolvins, can attenuate neuroinflammation-related depression (Furuyashiki et al., 2019).
The gut microbiota is a key site of neurotransmitter synthesis, such as serotonin (5-HT), norepinephrine (NE), and dopamine (DA), which are impaired in depression (Bhatt et al., 2022). It is widely accepted that depression and depressive behaviours are associated with gut dysbiosis induced by excessive activation of the HPA axis and increased levels of cortisol/corticosterone in the blood. This results in increased intestinal permeability and increased translocation of gut bacteria and their metabolites (including short-chain fatty acids, neurotransmitters, and cytokines) into the circulation (a process also known as “leaky gut”) (Halvorson et al., 2024). A leaky gut causes increased inflammation, changes in gut bacteria (including reduced levels of Bifidobacterium and Lactobacillus), and accompanying symptoms of depression, which can be alleviated by taking probiotics and prebiotics (Sharma et al., 2024; Sjöstedt et al., 2021). Depression often accompanies irritable bowel syndrome (IBS), which is directly related to the occurrence of gut dysbiosis, effectively treated with SSRIs. The underlying mechanism of the therapeutic interaction between the microbiota and psychotic treatment is not clear, however. In contrast to the aforementioned therapeutic effects observed in SSRI-treated IBS, preclinical evidence shows that the metabolic disturbances observed with olanzapine are due to the drug-microbiota interaction, as the use of antibiotics that eliminate gut bacteria reversed the adverse side effects of olanzapine (Sjöstedt et al., 2021). Antidepressant therapy can also affect the composition of the microbiota. For example, MAO-A inhibitors (iproniazid) or SSRIs (sertraline, fluoxetine, paroxetine) have been shown to act like antibiotics, leading to microbial dysbiosis, which is associated with weight gain reported as a side effect after long-term SSRI use. In turn, vortioxetine, a 5-HT receptor modulator and SERT inhibitor (Sanchez et al., 2015) seems to exert its antidepressant effects by regulating the gut microbiota in patients with MDD (Ye et al., 2021). Understanding the precise mechanism linking dysbiosis with depression and dysbiosis induced by some antidepressants seems important for the development of improved antidepressants.
Currently, it is evidenced that gut microbiome communicates with the brain through the gut-brain axis, regulating stress responses, inflammation, and emotions (Guerrero-Hreins et al., 2021; Pinna, 2023). Glucagon-like peptide 1 (GLP-1), released from the colon, reaches the brain via the vagal afferent pathway and activates GLP-1 receptors (GLP-1R), which are widely expressed in limbic structures. This pathway is implicated in regulating feeding and emotional behaviors.
Clinical observations suggest that pharmacotherapy with GLP-1R agonists may induce demotivation and anhedonia with prolonged use (Dumiaty et al., 2024; Detka and Głombik, 2021). Preclinical studies have recently highlighted reelin, an extracellular matrix protein expressed in the brain and intestines, as a promising antidepressive compound (Halvorson et al., 2024). In the brain, reelin supports synaptic plasticity, while in the intestines, it interacts with receptors such as VLDLR, ApoER2, EphB2, and α3β1-integrins. Similarly to ketamine, reelin was shown to activate mTORC1, without NMDA receptors antagonism, suggesting that rescuing reelin level may offer rapid antidepressant effects avoiding the side (dissociative) effects related to therapy with ketamine (Johnston et al., 2023).
Epigenetic modifications of the gut microbiome and its metabolites, induced by diet, prebiotics, probiotics, specific antibiotics, fecal microbiota transplantation, or antidepressants, may have beneficial antidepressive effects (Nohesara et al., 2023). For example, augmentation of allopregnanolone (AP) signaling through PPAR-α (peroxisome proliferator-activated receptor α) in the colon has been shown to reduce gut dysbiosis and depressive symptoms (Pinna, 2023). Similarly, gastrointestinal peptides like ghrelin have emerged as potential targets for modulating dysbiosis-related depressive-like behavior (Gajewska et al., 2023).
Dysregulation of neural plasticity is a well-established factor implicated in the pathophysiology of depression and is characterized by hypoactivation, synaptic loss and weakening, reduced connectivity, and decreased levels of neurotrophic factors (reviewed in Matar et al., 2024). Brain-derived neurotrophic factor (BDNF) is considered a critical mediator between antidepressant activity and the neuroplastic changes responsible for therapeutic effects. Impaired BDNF-TrkB signalling and its regulatory influence on NMDA and AMPA receptors in the forebrain leads to structural and functional changes in neurons and the development of psychiatric diseases, including depression (McGinty et al., 2015; Sun et al., 2014; Sharma et al., 2024; Zelek-Molik et al., 2021). Glutamatergic modulation of AMPA and NMDA receptors on cortical pyramidal neurons leads to upregulation of BDNF expression. Through this mechanism, glutamate release and AMPA receptor activation enhance BDNF binding to TrkB. This activates mTOR and ERK signalling, intensifying the expression of neuroplasticity-related genes, protein synthesis of synaptic components, and mechanisms that result in rapid and long-lasting local synaptogenesis (Pochwat et al., 2022).
Antidepressants enhance neuroplasticity through several pathways. By modulating the TrkB receptor and increasing the excitability of pyramidal neurons, these treatments amplify excitatory transmission. Receptor activity of antidepressants influences mTORC1 through serotonin transporters (as seen in classical antidepressants), NMDA, mGluR2, or AMPA receptors (in rapid-acting antidepressants like ketamine and its metabolites), 5HT2A/5HT1A receptors (as in psychedelics such as psilocin or lysergic acid diethylamide), or even through heterodimer formation, which potentiates neuroplasticity effects between receptor types (Brunello et al., 2024; Ilchibaeva et al., 2022). Further, downstream effectors including mTORC1, ERK, CREB, and elongation factor 2 (EF2) mediate intracellular effects of antidepressants by inducing plasticity-related genes and BDNF expression. These neuroplasticity-driven processes significantly reduce depressive symptoms by promoting synaptic remodelling and enhanced connectivity (Page et al., 2024; Moliner et al., 2023; Casarotto et al., 2021; Saarelainen et al., 2003). Although BDNF does not cross the blood-brain barrier (BBB), its levels in the brain have been shown to increase through physical exercise. This effect is mediated by the myokine irisin, a protein upregulated in muscle during exercise, which is cleaved from its precursor (FNDC5), secreted into circulation, and capable of crossing the BBB to stimulate BDNF synthesis in the hippocampus. This process supports neuroplasticity and related therapeutic outcomes (Colucci-D’Amato et al., 2020; Liu et al., 2024).
BDNF exerts its effects not only through high-affinity binding to TrkB receptors but also through low-affinity interactions with the neurotrophin receptor p75NTR and its co-receptor sortilin. These interactions activate a wide range of intracellular signalling pathways involved in cytoskeletal remodelling and plasticity. Recently identified downstream effectors of BDNF signalling that may serve as potential targets for new antidepressant therapies include non-receptor tyrosine kinases such as SFK/JAK/FAK (Wang et al., 2022) and FYN (Zelek-Molik et al., 2025); lysophosphatidic acid (LPA) and its receptors (Li and Li, 2024); the small GTPase RhoA (Rafa-Zabłocka et al., 2021); and adhesion G protein-coupled receptor GPR65 (Qi and Guan, 2024).
Recent studies have highlighted the antidepressant potential of various phytochemicals, including gintonin (Kim H-J et al., 2017), ferulic acid (Dong and Zhao, 2023), ginsenoside Rg1 (Yang et al., 2022), flavonols (Jazvinšćak Jembrek et al., 2023), flavonoids (German-Ponciano et al., 2022; Ko et al., 2020), mitragynine (Johnson et al., 2020), and terpenoids such as linalool, linalyl acetate, geraniol, citronellol, and limonene (Muller et al., 2021; Agatonovic-Kustrin et al., 2020). While the precise mechanisms of antidepressant action and their clinical usefulness require further research, it has been observed that their effects often combine anti-inflammatory properties, improvements in brain-gut axis function, and positive effects on neuronal plasticity.
The anti-inflammatory effects of plant compounds have been linked to downregulation of the proinflammatory MAPK and NF-κB pathways (Wang et al., 2024; Dong and Zhao, 2023), upregulation of the heme oxygenase (HO) system via nuclear transcription factor erythroid 2-related factor 2 (Nrf2) (Wang et al., 2024), inhibition of MAO-A activity in the forebrain, and suppression of microglial activation and proinflammatory cytokine release (Dong and Zhao, 2023).
In addition to their anti-inflammatory properties, active plant compounds like ferulic acid, ginsenoside Rg1, and peptides from soybeans, leaves, and grains (e.g., pEL, rubimetide, and soy-deprestatin) have demonstrated antianxiety and antidepressant effects via gut-brain regulation (Dong and Zhao, 2023; Yang et al., 2022; Mizushige, 2021). These peptides are thought to activate serotonin, dopamine, and GABA systems in the brain through their interaction with the gastrointestinal tract and vagus nerves. Ferulic acid and ginsenoside Rg1 have also been shown to reduce blood corticosterone levels and brain glycogen, as well as alleviate dysbiosis in the colon by modulating microbiome composition and microbial metabolism.
Regarding their effects on impaired neuroplasticity in limbic structures, flavonoids have been found to exhibit antidepressant properties by increasing BDNF and 5-HT levels in these regions (German-Ponciano et al., 2022). Ginsenoside Rg1, ginkgolide A, curcumin, cannabidiol, lavender oil, extracts from Hericium erinaceus mushrooms, and other traditional African medicinal plants have been shown to regulate BDNF/cAMP/CREB signaling in the forebrain (Yang et al., 2022; Gutiérrez-Rodelo et al., 2023; Muller et al., 2021). Lavender oil, in particular, enhances dendritic complexity in primary neurons compared to corticosterone-treated neurons. Interestingly, lavender oil exerts its antidepressant effects without binding to monoamine transporters, neurotransmitter receptors, or GABAergic properties. Its mechanism includes moderate inhibition of voltage-dependent calcium channes (VDCC), which differentiates it from classical antidepressants (Muller et al., 2021).
As discussed above, antidepressive pharmacotherapies are primarily focused on monoaminergic and glutamatergic neurotransmission, which have been shown to influence neuroplasticity in brain limbic structures and mitigate depressive symptoms. However, the clinical utility of currently available antidepressants is limited by insufficient effectiveness and numerous side effects in patients. This highlight the necessity to identify new molecular targets involved in the pathogenesis of depression and to discover compounds with greater therapeutic potential than those offered by current pharmacotherapy.
Animal models provide a unique opportunity to evaluate the effects of potential drugs in the context of a wide range of biological and behavioural actions and play a crucial role in drug development, as well as being used to determine drug efficacy and predict antidepressant efficacy. Rodent models of depression that satisfy critical criteria of face, predictive, and construct validity are most often based on acute, chronic, or social stress paradigms, or genetic predispositions (Duman, 2010; Willner et al., 2019). Traditionally, animal models have been employed to assess drug efficacy and predict antidepressant potential. However, the increasing global burden of TRD requires the development and validation of new preclinical models tailored to this disease.
The Lancet-World Psychiatric Association Commission on Depression (2022) has emphasised that the development and testing of therapies targeting early intervention and novel disease mechanisms is a critical priority. In this regard, variants of the diathesis-stress animal model, also referred to as the vulnerability-stress model, are consistent with these recommendations. The diathesis-stress model posits that an individual predisposed to vulnerability (biological or psychological) may develop depression following exposure to an acute stressor. This vulnerability may encompass biological factors (e.g., genetic predispositions, endocrine imbalances, inflammatory responses, or altered brain connectivity) or psychological traits (e.g., temperament, personality, past experiences, or beliefs) (Monroe and Simons, 1991). These elements are increasingly utilized in animal models with high translational potential to generate novel, rapid-acting antidepressant drug candidates (Papp and Willner, 2023; Papp et al., 2024).
Vulnerability factors stemming from early life stress, social stressors, genetics, or experimental manipulations contribute to the development of depression-like symptoms in animal models. These conditions are associated with alterations in energy metabolism, hypothalamic-pituitary-adrenal (HPA) axis dysfunction, genetic factors, and behavioral disturbances, all of which are relevant to the pathophysiology of depression in humans (Virijevic et al., 2024; Brivio et al., 2022; Willner and Belzung, 2015). While animal models cannot fully replicate the complexity of human depressive disorders, they successfully simulate core symptoms of depression, elucidate underlying mechanisms, and allow the testing of specific hypotheses regarding novel molecular targets. Moreover, animal models are critical for evaluating the potential of newly discovered mechanisms and for assessing the efficacy of drug candidates. Although limitations exist, these models remain invaluable for advancing our understanding of depression and the development of innovative therapies.
In their 2022 review, Borbély et al. highlighted that many phase II clinical trials focus on the effects of drug candidates in treatment-resistant depression (TRD), emphasizing the urgent need for therapies with efficacy in this challenging population. This trend is mirrored in preclinical studies, where antidepressant candidates are often tested in TRD models. Demonstrating efficacy in such models significantly increases the likelihood of success in treating mood disorders. It appears that neuroplasticity-based mechanisms, such as TrkB dimerization and BDNF binding, likely represent a common pathway for many antidepressant therapies, underscoring the essential role of plasticity mechanisms in the long-term efficacy of pharmacotherapy. Understanding and enhancing these mechanisms may be key to improving antidepressant outcomes.
Interestingly, the mechanisms of action of many novel antidepressant drugs have shifted focus to the glutamatergic-GABAergic interplay rather than traditional monoaminergic neurotransmitter systems. New drug candidates include GABA-A potentiators, NMDA receptor antagonists or modulators, and mGlu2/3 receptor modulators. Psilocybin formulations and synthetic psychedelics targeting 5-HT2A receptor agonism are also being explored; however, concerns regarding their safety, including potential risks of addiction and other adverse effects, remain a critical area of debate.
Evidence supports the efficacy of rapid-acting therapies in providing a rapid reduction in depressive symptoms, which could improve patients' quality of life and reduce healthcare costs. However, no glutamatergic modulator tested to date has matched the rapid, robust and sustained antidepressant effects of (R,S)-ketamine and esketamine. In addition, the breadth of therapeutic effects of ketamine, such as its antisuicidal properties, anti-anhedonic effects or broader therapeutic potential, remains unmatched. The investigation of novel compounds for these specific indications remains a high priority.
One of the significant challenges in developing new antidepressants is the heterogeneity of mood disorders. Despite significant progress, the pathophysiology of depression remains idiopathic and incompletely understood, complicating efforts to identify universally effective treatments.
Key questions for future research include:
Neuroplasticity and Treatment Response: How can neuroplasticity be effectively enhanced to improve treatment outcomes? What are the most promising molecular targets to stimulate plasticity mechanisms?
Mechanistic Contributions: How do intracellular signaling pathways, network activity, neuroinflammatory and neuroendocrine effects, early-life factors, and the microbiota system contribute to the clinical efficacy of antidepressants?
Combination Therapies: What are the additive or synergistic effects of pharmacotherapy when combined with psychotherapy or other interventions, and how can these combinations be optimized?
Addressing these questions will require an integrated approach, combining insights from neurobiology, pharmacology, genetics, and clinical practice. Continued exploration of novel compounds, combined therapies, and personalized treatment strategies, offers the best path forward to advancing the treatment of depression and TRD.
AZ-M: Conceptualization, Writing–original draft, Writing–review and editing. EL: Conceptualization, Writing–original draft, Writing–review and editing.
The author(s) declare that financial support was received for the research, authorship, and/or publication of this article. Supported by Grant Intramural (received by EL) statutory funds (AZ-M.) from the Maj Institute of Pharmacology, Polish Academy of Sciences, Kraków, Poland.
The authors thank Professor Mariusz Papp for his constructive comments on manuscript content.
The authors declare that the research was conducted in the absence of any commercial or financial relationships that could be construed as a potential conflict of interest.
The author(s) declare that no Generative AI was used in the creation of this manuscript.
All claims expressed in this article are solely those of the authors and do not necessarily represent those of their affiliated organizations, or those of the publisher, the editors and the reviewers. Any product that may be evaluated in this article, or claim that may be made by its manufacturer, is not guaranteed or endorsed by the publisher.
Agatonovic-Kustrin, S., Kustrin, E., Gegechkori, V., and Morton, D. W. (2020). Anxiolytic terpenoids and aromatherapy for anxiety and depression. Adv. Exp. Med. Biol. 1260, 283–296. doi:10.1007/978-3-030-42667-5_11
Aleksandrova, L. R., and Phillips, A. G. (2021). Neuroplasticity as a convergent mechanism of ketamine and classical psychedelics. Trends Pharmacol. Sci. 42, 929–942. doi:10.1016/j.tips.2021.08.003
American Psychological Association (APA) (2019). Clinical practice guideline for the treatment of depression across three age cohorts. Apa. Org. Available at: https://www.apa.org/depression-guideline.
Bajpai, A., Verma, A. K., Srivastava, M., and Srivastava, R. (2014). Oxidative stress and major depression. J. Clin. Diagnostic Res. 8, CC04–CC7. doi:10.7860/jcdr/2014/10258.5292
Bansal, Y., Codeluppi, S. A., and Banasr, M. (2024). Astroglial dysfunctions in mood disorders and rodent stress models: consequences on behavior and potential as treatment target. Int. J. Mol. Sci. 25, 6357. doi:10.3390/ijms25126357
Barbara, A. M., Xie, W., Mahood, Q., and Hamson, A. (2024). Ketamine for adults with treatment-resistant depression or posttraumatic stress disorder: a 2023 update: rapid review cadth. Available at: https://pubmed.ncbi.nlm.nih.gov/38564542/.
Bell, C. J., Mehta, M., Mirza, L., Young, A. H., and Beck, K. (2024). Glutathione alterations in depression: a meta-analysis and systematic review of proton magnetic resonance spectroscopy studies. Psychopharmacology. doi:10.1007/s00213-024-06735-1
Belzeaux, R., Bergon, A., Jeanjean, V., Loriod, B., Formisano-Tréziny, C., Verrier, L., et al. (2012). Responder and nonresponder patients exhibit different peripheral transcriptional signatures during major depressive episode. Transl. Psychiatry 2, e185. doi:10.1038/tp.2012.112
Berk, M., Ole, K.-F., Turner, M. C., Penninx, B. W. J. H., Wrobel, A. L., Firth, J., et al. (2023). Comorbidity between major depressive disorder and physical diseases: a comprehensive review of epidemiology, mechanisms and management. World Psychiatry 22, 366–387. doi:10.1002/wps.21110
Berlim, M. T., and Turecki, G. (2007). What is the meaning of treatment resistant/refractory major depression (TRD)? A systematic review of current randomized trials. Eur. Neuropsychopharmacol. 17, 696–707. doi:10.1016/j.euroneuro.2007.03.009
Berman, R. M., Cappiello, A., Anand, A., Oren, D. A., Heninger, G. R., Charney, D. S., et al. (2000). Antidepressant effects of ketamine in depressed patients. Biol. Psychiatry 47, 351–354. doi:10.1016/s0006-3223(99)00230-9
Bhatt, S., Kanoujia, J., Mohanalakshmi, S., Patil, C., Gupta, G., Chellappan, D. K., et al. (2022). Role of brain-gut-microbiota Axis in depression: emerging therapeutic avenues. CNS and Neurological Disord. - Drug Targets 21, 276–288. doi:10.2174/1871527321666220329140804
Bielawski, A., Zelek-Molik, A., Rafa-Zabłocka, K., Kowalska, M., Gruca, P., Papp, M., et al. (2023). Elevated expression of HSP72 in the prefrontal cortex and Hippocampus of rats subjected to chronic mild stress and treated with imipramine. Int. J. Mol. Sci. 25, 243. doi:10.3390/ijms25010243
Borbély, É., Simon, M., Fuchs, E., Wiborg, O., Czéh, B., and Helyes, Z. (2022). Novel drug developmental strategies for treatment-resistant depression. Br. J. Pharmacol. 179, 1146–1186. doi:10.1111/bph.15753
Bowden, C. L., Perlis, R. H., Thase, M. E., Ketter, T. A., Ostacher, M. M., Calabrese, J. R., et al. (2012). Aims and results of the NIMH systematic treatment enhancement program for bipolar disorder (STEP-BD). CNS Neurosci. and Ther. 18, 243–249. doi:10.1111/j.1755-5949.2011.00257.x
Brivio, P., Audano, M., Gallo, M. T., Gruca, P., Lason, M., Litwa, E., et al. (2022). Metabolomic signature and mitochondrial dynamics outline the difference between vulnerability and resilience to chronic stress. Transl. Psychiatry 12, 87. doi:10.1038/s41398-022-01856-7
Bromet, E., Andrade, L. H., Hwang, I., Sampson, N. A., Alonso, J., de Girolamo, G., et al. (2011). Cross-national epidemiology of DSM-IV major depressive episode. BMC Med. 9, 90. doi:10.1186/1741-7015-9-90
Brunello, C. A., Cannarozzo, C., and Castrén, E. (2024). Rethinking the role of TRKB in the action of antidepressants and psychedelics. Trends Neurosci. 47, 865–874. doi:10.1016/j.tins.2024.08.011
Canadian Agency for Drugs and Technologies in Health (2014). Intravenous ketamine for the treatment of mental health disorders: a review of clinical effectiveness and guidelines. Available at: https://pubmed.ncbi.nlm.nih.gov/25411673/(Accessed July 30, 2024).
Casarotto, P. C., Girych, M., Fred, S. M., Kovaleva, V., Moliner, R., Enkavi, G., et al. (2021). Antidepressant drugs act by directly binding to TRKB neurotrophin receptors. Cell 184, 1299–1313.e19. doi:10.1016/j.cell.2021.01.034
U.S. Food and Drug Administration (FDA) (2021). 2018 first generic drug approvals. FDA. Available at: https://www.fda.gov/drugs/first-generic-drug-approvals/2018-first-generic-drug-approvals.
Clayton, A. H., Lasser, R., Nandy, I., Sankoh, A. J., Jonas, J., and Kanes, S. J. (2023). Zuranolone in major depressive disorder: results from MOUNTAIN-A phase 3, multicenter, double-blind, randomized, placebo-controlled trial. J. Clin. Psychiatry 84, 22m14445. doi:10.4088/jcp.22m14445
Colucci-D’Amato, L., Speranza, L., and Volpicelli, F. (2020). Neurotrophic factor BDNF, physiological functions and therapeutic potential in depression, neurodegeneration and brain cancer. Brain Cancer. Int. J. Mol. Sci. 21, 7777. doi:10.3390/ijms21207777
Correia, A. S., Cardoso, A., and Vale, N. (2023). Oxidative stress in depression: the link with the stress response, neuroinflammation, serotonin, neurogenesis and synaptic plasticity. Antioxidants 12, 470. doi:10.3390/antiox12020470
Cuijpers, P., Javed, A., and Bhui, K. (2023). The WHO world mental health report: a call for action. Br. J. Psychiatry 222, 227–229. doi:10.1192/bjp.2023.9
Davies, P., Ijaz, S., Williams, C. J., Kessler, D., Lewis, G., and Wiles, N. (2019). Pharmacological interventions for treatment-resistant depression in adults. Cochrane Database Syst. Rev. 12. doi:10.1002/14651858.cd010557.pub2
Detka, J., and Głombik, K. (2021). Insights into a possible role of glucagon-like peptide-1 receptor agonists in the treatment of depression. Pharmacol. Rep. 73, 1020–1032. doi:10.1007/s43440-021-00274-8
DiBernardo, A., Lin, X., Zhang, Q., Xiang, J., Lu, L., Jamieson, C., et al. (2018). Humanistic outcomes in treatment resistant depression: a secondary analysis of the STAR*D study. BMC Psychiatry 18, 352. doi:10.1186/s12888-018-1920-7
Di Vincenzo, M., Martiadis, V., Della Rocca, B., Arsenio, E., D’Arpa, A., Volpicelli, A., et al. (2024). Facts and myths about use of esketamine for treatment-resistant depression: a narrative clinical review. Front. Psychiatry 15, 1394787. doi:10.3389/fpsyt.2024.1394787
Dogra, S., and Conn, P. J. (2021). Targeting metabotropic glutamate receptors for the treatment of depression and other stress-related disorders. Neuropharmacology 196, 108687. doi:10.1016/j.neuropharm.2021.108687
Döme, P., Kunovszki, P., Takács, P., Fehér, L., Balázs, T., Dede, K., et al. (2021). Clinical characteristics of treatment-resistant depression in adults in Hungary: real-world evidence from a 7-year-long retrospective data analysis. PLOS ONE 16, e0245510. doi:10.1371/journal.pone.0245510
Dong, X., and Zhao, D. (2023). Ferulic acid as a therapeutic agent in depression: evidence from preclinical studies. CNS Neurosci. and Ther. 29, 2397–2412. doi:10.1111/cns.14265
Duda, W., Curzytek, K., Kubera, M., Connor, T. J., Fagan, E. M., Basta-Kaim, A., et al. (2019). Interaction of the immune-inflammatory and the kynurenine pathways in rats resistant to antidepressant treatment in model of depression. Int. Immunopharmacol. 73, 527–538. doi:10.1016/j.intimp.2019.05.039
Duda, W., Kubera, M., Kreiner, G., Katarzyna, C., Detka, J., Głombik, K., et al. (2017). Suppression of pro-inflammatory cytokine expression and lack of anti-depressant-like effect of fluoxetine in lipopolysaccharide-treated old female mice. Int. Immunopharmacol. 48, 35–42. doi:10.1016/j.intimp.2017.04.021
Duman, C. H. (2010). Models of depression. Hormones Limbic Syst. 82, 1–21. doi:10.1016/s0083-6729(10)82001-1
Dumiaty, Y., Underwood, B. M., Phy-Lim, J., and Chee, M. J. (2024). Neurocircuitry underlying the actions of glucagon-like peptide 1 and peptide YY3–36 in the suppression of food, drug-seeking, and anxiogenesis. Neuropeptides 105, 102427. doi:10.1016/j.npep.2024.102427
Erritzoe, D., Barba, T., Spriggs, M. J., Rosas, F. E., Nutt, D. J., and Carhart-Harris, R. (2024). Effects of discontinuation of serotonergic antidepressants prior to psilocybin therapy versus escitalopram for major depression. J. Psychopharmacol. 38, 458–470. doi:10.1177/02698811241237870
European Medicines Agency (EMA) (2013). Guideline on clinical investigation of medicines for depression. European Medicines Agency. Available at: https://www.ema.europa.eu/en/news/european-medicines-agency-publishes-guideline-clinical-investigation-medicines-depression (Accessed January 16, 2025).
Fang, S., Wu, Z., Guo, Y., Zhu, W., Wan, C., Yuan, N., et al. (2023). Roles of microglia in adult hippocampal neurogenesis in depression and their therapeutics. Front. Immunol. 14, 1193053. doi:10.3389/fimmu.2023.1193053
Fava, M., Papakostas, G. I., Petersen, T., Mahal, Y., Quitkin, F., Stewart, J., et al. (2003). Switching to bupropion in fluoxetine-resistant major depressive disorder. Ann. Clin. psychiatry official J. Am. Acad. Clin. Psychiatrists 15, 17–22. doi:10.1023/a:1023224525400
Figee, M., Riva-Posse, P., Choi, K. S., Bederson, L., Mayberg, H. S., and Kopell, B. H. (2022). Deep brain stimulation for depression. Neurotherapeutics 19, 1229–1245. doi:10.1007/s13311-022-01270-3
Fu, C. H. Y., Antoniades, M., Erus, G., Garcia, J. A., Fan, Y., Arnone, D., et al. (2024). Neuroanatomical dimensions in medication-free individuals with major depressive disorder and treatment response to SSRI antidepressant medications or placebo. Nat. Ment. Health 2, 164–176. doi:10.1038/s44220-023-00187-w
Furuyashiki, T., Akiyama, S., and Kitaoka, S. (2019). Roles of multiple lipid mediators in stress and depression. Int. Immunol. 31, 579–587. doi:10.1093/intimm/dxz023
Gabriel, F. C., Stein, A. T., Melo, D. de O., Fontes-Mota, G. C. H., dos Santos, I. B., Rodrigues, C. da S., et al. (2023). Guidelines’ recommendations for the treatment-resistant depression: a systematic review of their quality. PLOS ONE 18, e0281501. doi:10.1371/journal.pone.0281501
Gajewska, A., Strzelecki, D., and Gawlik-Kotelnicka, O. (2023). Ghrelin as a biomarker of “immunometabolic depression” and its connection with dysbiosis. Nutrients 15, 3960. doi:10.3390/nu15183960
Gałecki, P., Samochowiec, J., Mikułowska, M., and Szulc, A. (2022). Treatment-resistant depression in Poland—epidemiology and treatment. J. Clin. Med. 11, 480. doi:10.3390/jcm11030480
German-Ponciano, L. J., Rosas-Sánchez, G. U., Cueto-Escobedo, J., Fernández-Demeneghi, R., Guillén-Ruiz, G., Soria-Fregozo, C., et al. (2022). Participation of the serotonergic system and brain-derived neurotrophic factor in the antidepressant-like effect of flavonoids. Int. J. Mol. Sci. 23, 10896. doi:10.3390/ijms231810896
Guerrero Fernández de Alba, I., Gimeno-Miguel, A., Poblador-Plou, B., Gimeno-Feliu, L. A., Ioakeim-Skoufa, I., Rojo-Martínez, G., et al. (2020). Association between mental health comorbidity and health outcomes in type 2 diabetes mellitus patients. Sci. Rep. 10, 19583. doi:10.1038/s41598-020-76546-9
Guerrero-Hreins, E., Goldstone, A. P., Brown, R. M., and Sumithran, P. (2021). The therapeutic potential of GLP-1 analogues for stress-related eating and role of GLP-1 in stress, emotion and mood: a review. Prog. Neuro-Psychopharmacology Biol. Psychiatry 110, 110303. doi:10.1016/j.pnpbp.2021.110303
Gutiérrez-Rodelo, C., Martínez-Tolibia, S. E., Morales-Figueroa, G. E., Velázquez-Moyado, J. A., Olivares-Reyes, J. A., and Navarrete-Castro, A. (2023). Modulating cyclic nucleotides pathways by bioactive compounds in combatting anxiety and depression disorders. Mol. Biol. Rep. 50, 7797–7814. doi:10.1007/s11033-023-08650-8
Halvorson, C. S., Sánchez-Lafuente, C. L., Johnston, J. N., Kalynchuk, L. E., and Caruncho, H. J. (2024). Molecular mechanisms of reelin in the enteric nervous system and the microbiota-gut-brain Axis: implications for depression and antidepressant therapy. Int. J. Mol. Sci. 25, 814. doi:10.3390/ijms25020814
Hamani, C., and Nóbrega, J. N. (2010). Deep brain stimulation in clinical trials and animal models of depression. Eur. J. Neurosci. 32, 1109–1117. doi:10.1111/j.1460-9568.2010.07414.x
Hawkins, M., Schaffer, A., Sinyor, M., Nishikawa, Y., Herrmann, N., Lanctôt, K. L., et al. (2018). Suicide deaths by intentional self-poisoning in people with cardiovascular disease. General Hosp. Psychiatry 52, 41–47. doi:10.1016/j.genhosppsych.2018.03.005
Heerlein, K., De Giorgi, S., Degraeve, G., Frodl, T., Hagedoorn, W., Oliveira-Maia, A. J., et al. (2022). Real-world evidence from a European cohort study of patients with treatment resistant depression: healthcare resource utilization. J. Affect. Disord. 298, 442–450. doi:10.1016/j.jad.2021.11.004
Heerlein, K., Young, A. H., Otte, C., Frodl, T., Degraeve, G., Hagedoorn, W., et al. (2021). Real-world evidence from a European cohort study of patients with treatment resistant depression: baseline patient characteristics. J. Affect. Disord. 283, 115–122. doi:10.1016/j.jad.2020.11.124
Henter, I. D., Park, L. T., and Zarate, C. A. (2021). Novel glutamatergic modulators for the treatment of mood disorders: current status. CNS Drugs 35, 527–543. doi:10.1007/s40263-021-00816-x
Ijaz, S., Davies, P., Williams, C., Kessler, D., Lewis, G., and Wiles, N. (2018). Psychological therapies for treatment-resistant depression in adults. Cochrane database Syst. Rev. 5. doi:10.1002/14651858.CD010558.pub2
Ilchibaeva, T., Tsybko, A., Zeug, A., Müller, F. E., Guseva, D., Bischoff, S., et al. (2022). Serotonin receptor 5-ht2a regulates TrkB receptor function in heteroreceptor complexes. Cells 11, 2384. doi:10.3390/cells11152384
International Advisory Group for the Revision of ICD-10 Mental and Behavioural Disorders (2011). A conceptual framework for the revision of the ICD-10 classification of mental and behavioural disorders. World Psychiatry 10, 86–92. doi:10.1002/j.2051-5545.2011.tb00022.x
Jack, R. H., Joseph, R. M., Hollis, C., Hippisley-Cox, J., Butler, D., Waldram, D., et al. (2023). Seasonal trends in antidepressant prescribing, depression, anxiety and self-harm in adolescents and young adults: an open cohort study using English primary care data. BMJ Ment. Health 26, e300855. doi:10.1136/bmjment-2023-300855
Jazvinšćak Jembrek, M., Oršolić, N., Karlović, D., and Peitl, V. (2023). Flavonols in action: targeting oxidative stress and neuroinflammation in major depressive disorder. Int. J. Mol. Sci. 24, 6888. doi:10.3390/ijms24086888
Jensen, K. J., Osler, M., Bødker, N., Riise, J., and Petersen, J. (2023). Healthcare resource utilization prior to suicide death or suicide attempt in patients with major depressive disorder—a Danish registry-based cohort study. Suicide Life-Threatening Behav. 53, 399–414. doi:10.1111/sltb.12953
Johnson, L. E., Balyan, L., Magdalany, A., Saeed, F., Salinas, R., Wallace, S., et al. (2020). The potential for kratom as an antidepressant and antipsychotic. Yale J. Biol. Med. 93, 283–289. Available at: https://pubmed.ncbi.nlm.nih.gov/32607089/.
Johnston, J. N., Allen, J., Shkolnikov, I., Sanchez-Lafuente, C. L., Reive, B. S., Scheil, K., et al. (2023). Reelin rescues behavioral, electrophysiological, and molecular metrics of a chronic stress phenotype in a similar manner to ketamine. ENeuro 10, 0106–0123. doi:10.1523/eneuro.0106-23.2023
Kim, H.-J., Park, S.-D., Lee, R. M., Lee, B.-H., Choi, S.-H., Hwang, S.-H., et al. (2017). Gintonin attenuates depressive-like behaviors associated with alcohol withdrawal in mice. J. Affect. Disord. 215, 23–29. doi:10.1016/j.jad.2017.03.026
Kishimoto, T., Chawla, J. M., Hagi, K., Zarate, C. A., Kane, J. M., Bauer, M., et al. (2016). Single-dose infusion ketamine and non-ketamine N-methyl-d-aspartate receptor antagonists for unipolar and bipolar depression: a meta-analysis of efficacy, safety and time trajectories. Psychol. Med. 46, 1459–1472. doi:10.1017/s0033291716000064
Klimek, V., Zak-Knapik, J., and Mackowiak, M. (1994). Effects of repeated treatment with fluoxetine and citalopram, 5-HT uptake inhibitors, on 5-HT1A and 5-HT2 receptors in the rat brain. J. psychiatry and Neurosci. JPN 19, 63–67. Available at: https://pubmed.ncbi.nlm.nih.gov/8148368/(Accessed August 1, 2024).
Ko, Y.-H., Kim, S.-K., Lee, S.-Y., and Jang, C.-G. (2020). Flavonoids as therapeutic candidates for emotional disorders such as anxiety and depression. Archives Pharmacal Res. 43, 1128–1143. doi:10.1007/s12272-020-01292-5
Krupa, A. J., Dudek, D., and Siwek, M. (2023). Consolidating evidence on the role of insulin resistance in major depressive disorder. Curr. Opin. psychiatry 37, 23–28. doi:10.1097/yco.0000000000000905
Kumar, S., Gal, Z., Gonda, X., Huse, R. J., Juhasz, G., Bagdy, G., et al. (2019). Transcriptomic changes following chronic administration of selective serotonin reuptake inhibitors: a review of animal studies. Neuropsychopharmacol. Hung. A Magyar Pszichofarmakologiai Egyesulet Lapja = Official J. Hung. Assoc. Psychopharmacol. 21, 26–35. Available at: https://pubmed.ncbi.nlm.nih.gov/30962407/.
Lagerberg, T., Virtanen, S., Kuja-Halkola, R., Hellner, C., Lichtenstein, P., Fazel, S., et al. (2023). Predicting risk of suicidal behaviour after initiation of selective serotonin reuptake inhibitors in children, adolescents and young adults: protocol for development and validation of clinical prediction models. BMJ open 13, e072834. doi:10.1136/bmjopen-2023-072834
Leichsenring, F., Steinert, C., Rost, F., Abbass, A., Heim, N., and Ioannidis, J. P. A. (2023). A critical assessment of NICE guidelines for treatment of depression. World Psychiatry 22, 43–45. doi:10.1002/wps.21039
Li, N., and Li, Y. (2024). Lysophosphatidic acid (LPA) and its receptors in mood regulation: a systematic review of the molecular mechanisms and therapeutic potential. Int. J. Mol. Sci. 25 (13), 7440. doi:10.3390/ijms25137440
Lievanos-Ruiz, F. J., and Fenton-Navarro, B. (2024). Enzymatic biomarkers of oxidative stress in patients with depressive disorders. A systematic review. Clin. Biochem. 130, 110788. doi:10.1016/j.clinbiochem.2024.110788
Liu, Y., Fu, X., Zhao, X., Cui, R., and Yang, W. (2024). The role of exercise-related FNDC5/irisin in depression. Front. Pharmacol. 15, 1461995. doi:10.3389/fphar.2024.1461995
Lochmann, D., and Richardson, T. (2018). Selective serotonin reuptake inhibitors. Antidepressants 250, 135–144. doi:10.1007/164_2018_172
López-Giménez, J. F., and González-Maeso, J. (2018). Hallucinogens and serotonin 5-ht2a receptor-mediated signaling pathways. Curr. Top. Behav. Neurosci. 36, 45–73. doi:10.1007/7854_2017_478
Machado-Vieira, R., Henter, I. D., and Zarate, C. A. (2017). New targets for rapid antidepressant action. Prog. Neurobiol. 152, 21–37. doi:10.1016/j.pneurobio.2015.12.001
Madsen, M. K., Fisher, P. M., Burmester, D., Dyssegaard, A., Stenbæk, D. S., Kristiansen, S., et al. (2019). Psychedelic effects of psilocybin correlate with serotonin 2A receptor occupancy and plasma psilocin levels. Neuropsychopharmacology 44, 1328–1334. doi:10.1038/s41386-019-0324-9
Maina, G., Adami, M., Ascione, G., Bondi, E., Domenico, De B., Delmonte, D., et al. (2023). Nationwide consensus on the clinical management of treatment-resistant depression in Italy: a Delphi panel. Ann. General Psychiatry 22, 48. doi:10.1186/s12991-023-00478-7
Martinotti, G., Vita, A., Fagiolini, A., Maina, G., Bertolino, A., Dell’Osso, B., et al. (2022). Real-world experience of esketamine use to manage treatment-resistant depression: a multicentric study on safety and effectiveness (REAL-ESK study). J. Affect. Disord. 319, 646–654. doi:10.1016/j.jad.2022.09.043
Matar, D., Serhan, A., El Bilani, S., Faraj, R. A., Hadi, B. A., and Fakhoury, M. (2024). Psychopharmacological approaches for neural plasticity and neurogenesis in major depressive disorders. Adv. Exp. Med. Biol. 1456, 27–48. doi:10.1007/978-981-97-4402-2_2
McGinty, J. F., Zelek-Molik, A., and Sun, W.-L. (2015). Cocaine self-administration causes signaling deficits in corticostriatal circuitry that are reversed by BDNF in early withdrawal. Brain Res. 1628, 82–87. doi:10.1016/j.brainres.2014.09.050
Meng, Y., Liu, S., Yu, M., Liang, H., Tong, Y., Song, J., et al. (2024). The changes of blood and CSF ion levels in depressed patients: a systematic review and meta-analysis. Mol. Neurobiol. 61, 5369–5403. doi:10.1007/s12035-023-03891-x
Meyer, J. H., Kapur, S., Eisfeld, B., Brown, G. M., Houle, S., DaSilva, J., et al. (2001). The effect of paroxetine on 5-HT2AReceptors in depression: an [18F]setoperone PET imaging study. Am. J. Psychiatry 158, 78–85. doi:10.1176/appi.ajp.158.1.78
Milev, R., Giacobbe, P., Kennedy, S., Blumberger, D., Daskalakis, Z., Downar, J., et al. (2016). Canadian network for mood and anxiety treatments (CANMAT) 2016 clinical guidelines for the management of adults with major depressive disorder: section 4. Neurostimulation treatments. Canadian journal of psychiatry. Rev. Can. Psychiatr. doi:10.1177/0706743716660033
Mizushige, T. (2021). Neuromodulatory peptides: orally active anxiolytic-like and antidepressant-like peptides derived from dietary plant proteins. Peptides 142, 170569. doi:10.1016/j.peptides.2021.170569
Mokhtari, T., and Uludag, K. (2023). Role of NLRP3 inflammasome in post-spinal-cord-injury anxiety and depression: molecular mechanisms and therapeutic implications. ACS Chem. Neurosci. 15, 56–70. doi:10.1021/acschemneuro.3c00596
Moliner, R., Girych, M., Brunello, C. A., Kovaleva, V., Biojone, C., Enkavi, G., et al. (2023). Psychedelics promote plasticity by directly binding to BDNF receptor TrkB. Nat. Neurosci. 26, 1032–1041. doi:10.1038/s41593-023-01316-5
Monroe, S. M., and Simons, A. D. (1991). Diathesis-stress theories in the context of life stress research: implications for the depressive disorders. Psychol. Bull. 110, 406–425. doi:10.1037/0033-2909.110.3.406
Mourilhe, P., and Stokes, P. E. (1998). Risks and benefits of selective serotonin reuptake inhibitors in the treatment of depression. Drug Saf. 18, 57–82. doi:10.2165/00002018-199818010-00005
Muller, W. E., Sillani, G., Schuwald, A., and Friedland, K. (2021). Pharmacological basis of the anxiolytic and antidepressant properties of Silexan®, an essential oil from the flowers of lavender. Neurochem. Int. 143, 104899. doi:10.1016/j.neuint.2020.104899
Murphy, S. E., Capitão, L. P., Giles, S. L. C., Cowen, P. J., Stringaris, S., and Harmer, C. J. (2021). The knowns and unknowns of SSRI treatment in young people with depression and anxiety: efficacy, predictors, and mechanisms of action. Lancet Psychiatry 8, 824–835. doi:10.1016/S2215-0366(21)00154-1
Murray, A. D., Staff, R. T., McNeil, C. J., Salarirad, S., Phillips, L. H., Starr, J., et al. (2012). Depressive symptoms in late life and cerebrovascular disease: the importance of intelligence and lesion location. Depress. Anxiety 30, 77–84. doi:10.1002/da.22022
Nemeroff, C. B. (2007). Prevalence and management of treatment-resistant depression. J. Clin. Psychiatry 68 (Suppl. 8), 17–25. Available at: https://pubmed.ncbi.nlm.nih.gov/17640154/.
NHS Information Centre (2011). Attitudes to mental illness - 2011, survey report. NHS Digital. Available at: https://digital.nhs.uk/data-and-information/publications/statistical/attitudes-to-mental-illness/attitudes-to-mental-illness-2011-survey-report.
Nohesara, S., Abdolmaleky, H. M., Zhou, J.-R., and Thiagalingam, S. (2023). Microbiota-induced epigenetic alterations in depressive disorders are targets for nutritional and probiotic therapies. Genes 14, 2217. doi:10.3390/genes14122217
Nunez, N. A., Joseph, B., Pahwa, M., Kumar, R., Resendez, M. G., Prokop, L. J., et al. (2022). Augmentation strategies for treatment resistant major depression: a systematic review and network meta-analysis. J. Affect. Disord. 302, 385–400. doi:10.1016/j.jad.2021.12.134
Olfson, M., and Marcus, S. C. (2009). National patterns in antidepressant medication treatment. Archives General Psychiatry 66, 848–856. doi:10.1001/archgenpsychiatry.2009.81
Page, C. E., Page, C. E., Page, C. E., Page, C. E., and Page, C. E. (2024). Beyond the serotonin deficit hypothesis: communicating a neuroplasticity framework of major depressive disorder. Mol. psychiatry 29, 3802–3813. doi:10.1038/s41380-024-02625-2
Papp, M., Gruca, P., Lason, M., Katarzyna, T.-G., Niemczyk, M., Litwa, E., et al. (2018). Rapid antidepressant effects of deep brain stimulation of the pre-frontal cortex in an animal model of treatment-resistant depression. J. Psychopharmacol. 32, 1133–1140. doi:10.1177/0269881118791737
Papp, M., Gruca, P., Lason, M., Litwa, E., Newman-Tancredi, A., and Depoortère, R. (2023). The 5-HT1A receptor biased agonists, NLX-204 and NLX-101, display ketamine-like RAAD and anti-TRD activities in rat CMS models. Psychopharmacology 240, 2419–2433. doi:10.1007/s00213-023-06389-5
Papp, M., Gruca, P., Litwa, E., Lason, M., Newman-Tancredi, A., and Depoortère, R. (2024). The 5-HT1A receptor biased agonists, NLX-204 and NLX-101, like ketamine, elicit rapid-acting antidepressant activity in the rat chronic mild stress model via cortical mechanisms. J. Psychopharmacol. Oxf. Engl. 38, 661–671. doi:10.1177/02698811241254832
Papp, M., and Willner, P. (2023). Models of affective illness: chronic mild stress in the rat. Curr. Protoc. 3, e712. doi:10.1002/cpz1.712
Parker, G., and Graham, R. (2015). Determinants of treatment-resistant depression: the salience of benzodiazepines. J. Nerv. Ment. Dis. 203, 659–663. doi:10.1097/nmd.0000000000000348
Picard, M., Juster, R.-P., and McEwen, B. S. (2014). Mitochondrial allostatic load puts the “gluc” back in glucocorticoids. Nat. Rev. Endocrinol. 10, 303–310. doi:10.1038/nrendo.2014.22
Pinna, G. (2023). Role of PPAR-allopregnanolone signaling in behavioral and inflammatory gut-brain Axis communications. Biol. Psychiatry 94, 609–618. doi:10.1016/j.biopsych.2023.04.025
Pochwat, B., Krupa, A. J., Siwek, M., and Szewczyk, B. (2022). New investigational agents for the treatment of major depressive disorder. Expert Opin. Investigational Drugs 31, 1053–1066. doi:10.1080/13543784.2022.2113376
Poleszak, E., Wlaź, P., Szewczyk, B., Wlaź, A., Kasperek, R., Wróbel, A., et al. (2011). A complex interaction between glycine/NMDA receptors and serotonergic/noradrenergic antidepressants in the forced swim test in mice. J. Neural Transm. (Vienna) 118, 1535–1546. doi:10.1007/s00702-011-0630-9
Price, L. H., Charney, D. S., and Heninger, G. R. (1987). Reserpine augmentation of desipramine in refractory depression: clinical and neurobiological effects. Psychopharmacology 92, 431–437. doi:10.1007/bf00176473
Qi, Q., and Guan, G. (2024). GPR56: a potential therapeutic target for neurological and psychiatric disorders. Biochem. Pharmacol. 226, 116395. doi:10.1016/j.bcp.2024.116395
Qi, W., Jin, X., and Guan, W. (2023). Purinergic P2X7 receptor as a potential therapeutic target in depression. Biochem. Pharmacol. 219, 115959. doi:10.1016/j.bcp.2023.115959
Rafa-Zabłocka, K., Zelek-Molik, A., Tepper, B., Chmielarz, P., Kreiner, G., Wilczkowski, M., et al. (2021). Chronic restraint stress induces changes in the cerebral Galpha 12/13 and Rho-GTPase signaling network. Pharmacol. Rep. 73, 1179–1187. doi:10.1007/s43440-021-00294-4
Rafiei, D., and Kolla, N. J. (2021). Elevated brain fatty acid amide hydrolase induces depressive-like phenotypes in rodent models: a review. Int. J. Mol. Sci. 22, 1047. doi:10.3390/ijms22031047
Reutfors, J., Andersson, T. M.-L., Brenner, P., Brandt, L., DiBernardo, A., Li, G., et al. (2018). Mortality in treatment-resistant unipolar depression: a register-based cohort study in Sweden. J. Affect. Disord. 238, 674–679. doi:10.1016/j.jad.2018.06.030
Roy, S., Arif Ansari, M., Choudhary, K., and Singh, S. (2023). NLRP3 inflammasome in depression: a review. Int. Immunopharmacol. 117, 109916. doi:10.1016/j.intimp.2023.109916
Rush, A. J. (2007). The varied clinical presentations of major depressive disorder. J. Clin. Psychiatry Suppl 8, 4–10. Available at: https://pubmed.ncbi.nlm.nih.gov/17640152/.
Saarelainen, T., Hendolin, P., Lucas, G., Koponen, E., Sairanen, M., MacDonald, E., et al. (2003). Activation of the TrkB neurotrophin receptor is induced by antidepressant drugs and is required for antidepressant-induced behavioral effects. J. Neurosci. Official J. Soc. Neurosci. 23, 349–357. doi:10.1523/JNEUROSCI.23-01-00349.2003
Salzman, C. (1996). Heterogeneity of SSRI response. Harv. Rev. Psychiatry 4, 215–217. doi:10.3109/10673229609030546
Sanadgol, N., Feriz, A. M., Lisboa, S. F., and Joca, S. R. L. (2023). Putative role of glial cells in treatment resistance depression: an updated critical literation review and evaluation of single-nuclei transcriptomics data. Life Sci. 331, 122025. doi:10.1016/j.lfs.2023.122025
Sanchez, C., Asin, K. E., and Artigas, F. (2015). Vortioxetine, a novel antidepressant with multimodal activity: review of preclinical and clinical data. Pharmacol. and Ther. 145, 43–57. doi:10.1016/j.pharmthera.2014.07.001
Serretti, A. (2024). A critical view on new and future antidepressants. Clin. Psychopharmacol. neuroscience/Clinical Psychopharmacol. Neurosci. 22, 201–210. doi:10.9758/cpn.23.1145
Sharma, S., Chawla, S., Kumar, P., Ahmad, R., and Verma, P. K. (2024). The chronic unpredictable mild stress (CUMS) Paradigm: bridging the gap in depression research from bench to bedside. Brain Res. 1843, 149123. doi:10.1016/j.brainres.2024.149123
Sharp, T., and Collins, H. (2023). Mechanisms of SSRI therapy and discontinuation. Curr. Top. Behav. Neurosci. 66, 21–47. doi:10.1007/7854_2023_452
Siwek, M., Dudek, D., Schlegel-Zawadzka, M., Morawska, A., Piekoszewski, W., Opoka, W., et al. (2010). Serum zinc level in depressed patients during zinc supplementation of imipramine treatment. J. Affect. Disord. 126, 447–452. doi:10.1016/j.jad.2010.04.024
Sjöstedt, P., Enander, J., and Isung, J. (2021). Serotonin reuptake inhibitors and the gut microbiome: significance of the gut microbiome in relation to mechanism of action, treatment response, side effects, and tachyphylaxis. Front. Psychiatry 12, 682868. doi:10.3389/fpsyt.2021.682868
Smith, A. L., Harmer, C. J., Cowen, P. J., and Murphy, S. E. (2023). The serotonin 1A (5-ht1a) receptor as a pharmacological target in depression. CNS Drugs 37, 571–585. doi:10.1007/s40263-023-01014-7
Styczeń, K., Sowa-Kućma, M., Siwek, M., Dudek, D., Reczyński, W., Szewczyk, B., et al. (2016). The serum zinc concentration as a potential biological marker in patients with major depressive disorder. Metab. Brain Dis. 32, 97–103. doi:10.1007/s11011-016-9888-9
Sun, W.-L., Eisenstein, S. A., Zelek-Molik, A., and McGinty, J. F. (2014). A single brain-derived neurotrophic factor infusion into the dorsomedial prefrontal cortex attenuates cocaine self-administration-induced phosphorylation of synapsin in the nucleus accumbens during early withdrawal. Int. J. Neuropsychopharmacol. 18, pyu049. doi:10.1093/ijnp/pyu049
Swainson, J., Thomas, R. K., Archer, S., Chrenek, C., MacKay, M.-A., Baker, G., et al. (2019). Esketamine for treatment resistant depression. Expert Rev. Neurother. 19, 899–911. doi:10.1080/14737175.2019.1640604
Szewczyk, B., Szopa, A., Serefko, A., Poleszak, E., and Nowak, G. (2018). The role of magnesium and zinc in depression: similarities and differences. Magnesium Res. 31, 78–89. doi:10.1684/mrh.2018.0442
Thase, M. E. (2011). The small specific effects of antidepressants in clinical trials: what do they mean to psychiatrists? Curr. Psychiatry Rep. 13, 476–482. doi:10.1007/s11920-011-0235-x
Thomas, L., Kessler, D., Campbell, J., Morrison, J., Peters, T. J., Williams, C., et al. (2013). Prevalence of treatment-resistant depression in primary care: cross-sectional data. Br. J. General Pract. 63, e852–e858. doi:10.3399/bjgp13x675430
Trivedi, M. H., Rush, A. J., Wisniewski, S. R., Nierenberg, A. A., Warden, D., Ritz, L., et al. (2006). Evaluation of outcomes with citalopram for depression using measurement-based care in STAR*D: implications for clinical practice. Am. J. Psychiatry 163, 28–40. doi:10.1176/appi.ajp.163.1.28
Tundo, A., Betrò, S., Rocco, de F., Marchetti, F., Nacca, D., Necci, R., et al. (2023). Pramipexole augmentation for treatment-resistant unipolar and bipolar depression in the real world: a systematic review and meta-analysis. Life 13, 1043. doi:10.3390/life13041043
Virijevic, K., Spasojevic, N., Stefanovic, B., Ferizovic, H., Jankovic, M., Vasiljevic, P., et al. (2024). Chronic mild stress-induced dysregulation of MAPK and PI3K/AKT signaling in the hippocampus and medial prefrontal cortex of WKY female rats. Neurosci. Lett. 825, 137709. doi:10.1016/j.neulet.2024.137709
Walter, H. J., Abright, A. R., Bukstein, O. G., Diamond, J., Keable, H., Ripperger-Suhler, J., et al. (2022). Clinical practice guideline for the assessment and treatment of children and adolescents with major and persistent depressive disorders. J. Am. Acad. Child and Adolesc. Psychiatry 62, 479–502. doi:10.1016/j.jaac.2022.10.001
Wang, J., Behl, T., Rana, T., Sehgal, A., Wal, P., Saxena, B., et al. (2024). Exploring the pathophysiological influence of heme oxygenase-1 on neuroinflammation and depression: a study of phytotherapeutic-based modulation. Phytomedicine 127, 155466. doi:10.1016/j.phymed.2024.155466
Wang, J. Q., Derges, J. D., Bodepudi, A., Pokala, N., and Mao, L. (2022). Roles of non-receptor tyrosine kinases in pathogenesis and treatment of depression. J. Integr. Neurosci. 21, 025. doi:10.31083/j.jin2101025
Weilburg, J. B. (2004). An overview of SSRI and SNRI therapies for depression. Manag. Care (Langhorne, Pa.) 13, 25–33. Available at: https://pubmed.ncbi.nlm.nih.gov/15293768/(Accessed July 30, 2024).
Willner, P., and Belzung, C. (2015). Treatment-resistant depression: are animal models of depression fit for purpose? Psychopharmacology 232, 3473–3495. doi:10.1007/s00213-015-4034-7
Willner, P., Gruca, P., Lason, M., Tota-Glowczyk, K., Litwa, E., Niemczyk, M., et al. (2019). Validation of chronic mild stress in the Wistar-Kyoto rat as an animal model of treatment-resistant depression. Behav. Pharmacol. 30, 239–250. doi:10.1097/fbp.0000000000000431
Yang, S., Wang, J., Cheng, P., Chen, L., Hu, J., and Zhu, G. (2022). Ginsenoside Rg1 in neurological diseases: from bench to bedside. Acta Pharmacol. Sin. 44, 913–930. doi:10.1038/s41401-022-01022-1
Ye, X., Wang, D., Zhu, H., Wang, D., Li, J., Tang, Y., et al. (2021). Gut microbiota changes in patients with major depressive disorder treated with vortioxetine. Front. Psychiatry 12, 641491. doi:10.3389/fpsyt.2021.641491
Yokoyama, R., Higuchi, M., Tanabe, W., Tsukada, S., Naito, M., Yamaguchi, T., et al. (2020). (S)-norketamine and (2S,6S)-hydroxynorketamine exert potent antidepressant-like effects in a chronic corticosterone-induced mouse model of depression. Pharmacol. Biochem. Behav. 191, 172876. doi:10.1016/j.pbb.2020.172876
Zanos, P., Moaddel, R., Morris, P. J., Georgiou, P., Fischell, J., Elmer, G. I., et al. (2016). NMDAR inhibition-independent antidepressant actions of ketamine metabolites. Nature 533, 481–486. doi:10.1038/nature17998
Zelek-Molik, A., Bobula, B., Gądek-Michalska, A., Chorązka, K., Bielawski, A., Kuśmierczyk, J., et al. (2021). Psychosocial crowding stress-induced changes in synaptic transmission and glutamate receptor expression in the rat frontal cortex. Biomolecules 11, 294. doi:10.3390/biom11020294
Zelek-Molik, A., Gądek-Michalska, A., Wilczkowski, M., Bielawski, A., Maziarz, K., Kreiner, G., et al. (2025). Restraint stress effects on glutamate signaling protein levels in the rats’ frontal cortex: does β1 adrenoceptor activity matter? Front. Pharmacol. 15. doi:10.3389/fphar.2024.1451895
Keywords: depression, animal models, RAAD, TRD, antidepressants, ketamine, psylocin
Citation: Zelek-Molik A and Litwa E (2025) Trends in research on novel antidepressant treatments. Front. Pharmacol. 16:1544795. doi: 10.3389/fphar.2025.1544795
Received: 13 December 2024; Accepted: 10 January 2025;
Published: 27 January 2025.
Edited by:
Francisco Lopez-Munoz, Camilo José Cela University, SpainReviewed by:
Marcin Siwek, Jagiellonian University, PolandCopyright © 2025 Zelek-Molik and Litwa. This is an open-access article distributed under the terms of the Creative Commons Attribution License (CC BY). The use, distribution or reproduction in other forums is permitted, provided the original author(s) and the copyright owner(s) are credited and that the original publication in this journal is cited, in accordance with accepted academic practice. No use, distribution or reproduction is permitted which does not comply with these terms.
*Correspondence: Ewa Litwa, bGl0d2FAaWYtcGFuLmtyYWtvdy5wbA==
Disclaimer: All claims expressed in this article are solely those of the authors and do not necessarily represent those of their affiliated organizations, or those of the publisher, the editors and the reviewers. Any product that may be evaluated in this article or claim that may be made by its manufacturer is not guaranteed or endorsed by the publisher.
Research integrity at Frontiers
Learn more about the work of our research integrity team to safeguard the quality of each article we publish.