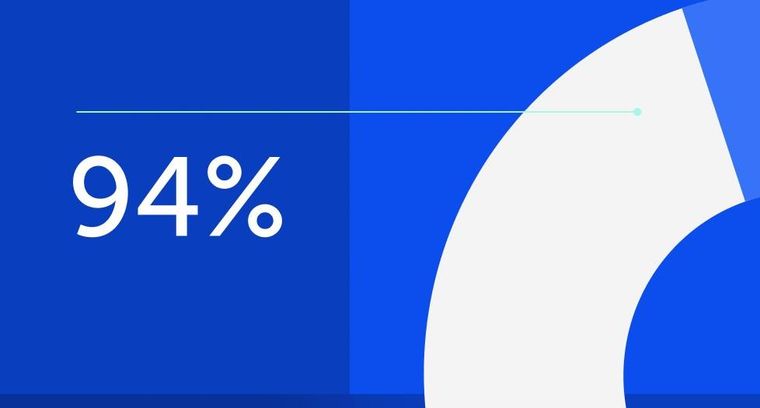
94% of researchers rate our articles as excellent or good
Learn more about the work of our research integrity team to safeguard the quality of each article we publish.
Find out more
SYSTEMATIC REVIEW article
Front. Pharmacol., 07 April 2025
Sec. Neuropharmacology
Volume 16 - 2025 | https://doi.org/10.3389/fphar.2025.1538879
This article is part of the Research TopicTargeting Mitochondrial Dysfunction for the Discovery and Development of Novel CNS Therapies in Rare and Neurodegenerative DiseasesView all 8 articles
Objective: The present review was developed to critically evaluate the neuroprotective effects of edaravone for experimental rat models of spinal cord injury (SCI) and generalize the possible mechanisms.
Methods: Systematic searches were carried out on databases including PubMed, Embase, Web of Science, Scopus, and Cochrane Library from their inception to March 2024. Controlled studies that assessed the neurological roles of edaravone on rats following SCI were selected. The Basso, Beattie, and Bresnahan (BBB) locomotor rating scale, residual white matter area, and malondialdehyde (MDA) level of the SCI rats were systematically searched by two reviewers.
Results: Ten eligible publications were included. Meta-analyses showed increased BBB scores in edaravone-treated rats compared with control ones. The effect size gradually increased from day 7 (seven studies, n = 246, weighted mean difference (WMD) = 1.96, 95% confidence interval (CI) = 1.23 to 2.68, P < 0.00001) to day 28 (seven studies, n = 222, WMD = 4.41, 95% CI = 3.19 to 5.63, P < 0.00001) after injury and then maintained stably in the following time. Meanwhile, edaravone treatment was associated with an amendment in the spared area of white matter and a lowering in the MDA expression in the lesion area. The subgroup analyses revealed that rats treated with edaravone exhibited superior locomotor recovery in compression injury models than contusion ones. In network analyses, the surface under the cumulative ranking curve gradually increased up to a dose of 5–6 mg/(kg·d) of edaravone, after which it plateaued. Mechanism analysis suggested edaravone can ameliorate oxidative stress, mitigate neuroinflammation, and counteract neuron apoptosis and ferroptosis via multiple signaling pathways to exert its neuroprotective effects.
Conclusion: Collectively, with a protective effect and a systematic action mechanism, edaravone warrants further investigation in SCI research and treatment. Nonetheless, in light of the limitations in the included studies, the findings in this review should be interpreted with caution.
Systematic Review Registration: https://www.crd.york.ac.uk/PROSPERO/view/CRD42022374914.
Spinal cord injury (SCI) is a devastating event characterized by a high disability rate (Safdarian et al., 2023). Globally, approximately 20 million individuals are affected by varying degrees of SCI, with approximately 900,000 new cases of SCI occurring annually (Safdarian et al., 2023; James et al., 2019; Guan et al., 2023). Although its incidence is relatively low compared to other types of injury or degenerative disease, the adverse impact on individuals and the consequent societal and familial burden is immeasurable (Safdarian et al., 2023; James et al., 2019). Currently, the standard clinical treatment for SCI primarily comprises surgical interventions, pharmacological treatments (including neuroprotective agents, steroids, vasopressors, and anticoagulation prophylaxis), and rehabilitation therapies. However, the therapeutic benefits of these approaches appear to be limited, and recent evidence has increasingly questioned the efficacy of steroid use in SCI treatment (Jazayeri et al., 2022; Fehlings et al., 2022; Baroudi et al., 2024; Picetti et al., 2022). Consequently, there is an urgent need to develop novel and more effective therapeutic strategies for SCI.
Under physiological conditions, the endogenous antioxidant enzymes effectively counteract the limited number of oxidants primarily derived from cellular energy metabolism (Sies and Jones, 2020; Zaric et al., 2023). However, following SCI, this dynamic balance is broken, with serious, irreversible consequences. The disorders in microcirculatory and organelle function will manifest within a few hours after the initial injury, leading to the accumulation and large generation of reactive oxygen species (ROS) and, ultimately, oxidative stress (Tran et al., 2018; Zrzavy et al., 2021). Subsequently, oxidative stress can persist for an extended period and extensively participate in the pathological processes of secondary injury, initiating a sequential cascade of damage and disrupting the crucial microenvironment for tissue remodeling (Zrzavy et al., 2021; Peng et al., 2023). Therefore, the regulation of excessive oxidative stress always serves as a promising therapeutic strategy for the treatment of SCI.
Edaravone, a synthetic scavenger of ROS, is widely utilized in clinical settings for stroke management (Xu et al., 2019). Meanwhile, due to its proven safety and efficacy in treating amyotrophic lateral sclerosis, this therapeutic intervention has obtained regulatory approval for addressing this challenging disease in both Japan and the United States (Genge et al., 2022). Considering the pivotal role of oxidative stress in SCI, the application scope of edaravone in this field is also being explored. Several pre-clinical studies have investigated the efficacy of edaravone in treating this disease (Ishii et al., 2018; Pang et al., 2022). However, there is currently a paucity of integrated evidence specifically addressing the neuroprotective effects of edaravone for SCI. Furthermore, the mechanism responsible for the neuroprotective role of edaravone remains obscure. In this study, we conducted a comprehensive review to assess the neuroprotective role of edaravone in SCI and summarize the possible molecular mechanisms.
This systematic review was registered on PROSPERO (registration number CRD42022374914). Full details of the rationale and methods of this study were published elsewhere (Wang et al., 2023).
PubMed, Embase, Web of Science, Scopus, and Cochrane Library were systematically searched from their inception to March 2024. The reference lists of the selected studies and reviews were additionally scrutinized to encompass any other pertinent studies. According to the search strategy guidelines (Leenaars et al., 2012), “edaravone,” “MCI 186,” “spinal cord injuries,” “spinal cord injury,” “spinal cord compression,” “spinal cord trauma,” and “rat” were employed as a key term for retrieval (Sng et al., 2022; Zhou et al., 2022). The database search query is provided in Appendix 1 in supplemental material.
The initial database search results were imported into NoteExpress 3.9 software for filtering. Following the prespecified eligibility criteria, two independent evaluators conducted a preliminary screening of the literature based on titles and abstracts, followed by a comprehensive assessment of the full text of potentially eligible studies to determine their inclusion. The senior author made the final decision in the case of any disagreement between the two researchers (Yao et al., 2015).
The inclusion criteria include the following: (1) controlled studies that assessed the neurological effects of edaravone on rat models of SCI without any restrictions on language, publication date, or publication status; (2) laboratory rats of any age, sex, or strain that underwent SCI induced by compression or contusion injury; (3) the experimental group was treated by edaravone with no restriction on dosage, formulation, and administration methods; (4) the control group received either vehicle, saline, saline with diluted dimethyl sulfoxide, or no treatment; and (5) reporting one of the following outcomes: Basso, Beattie, and Bresnahan (BBB) locomotor rating scale, spared white matter area, or malondialdehyde (MDA) (Sng et al., 2022).
The following studies were excluded: (1) case reports, in vitro studies, clinical studies, reviews, and commentaries; (2) studies that used a rat model of SCI induced by non-traumatic ischemia, transection, laceration, traumatic root avulsion, or genetic modification; (3) studies combining edaravone with another treatment, without including a group treated with edaravone alone; (4) absence of control group; and (5) outcome assessments did not include BBB score, spared white matter area, or MDA.
Data extraction was independently conducted by two reviewers. Data collection includes the name of the first author, publication year, details of the study population, injury type and level, specifics of the intervention, and measurements. The proposed mechanisms and changes in related molecules were extracted to investigate the neuroprotective mechanism of edaravone. In studies encompassing multiple intervention groups, data from only the edaravone and negative control groups were extracted for analyses. The mean, standard deviations (SD), and sample sizes were collected from each group of animals. If the study data were represented visually without numerical values, we utilized GetData Graph Digitizer 2.26 (http://getdata-graph-digitizer.com) to extract the relevant statistical data from the graphical representations. In the event of missing data, the authors were contacted on a weekly basis. If no response was received from the original authors within 2 weeks, the related study was included but limited to qualitative analyses only (Yao et al., 2015).
The methodological quality of the included studies was assessed using SYRCLE’s RoB tool, a validated instrument for evaluating the risk of bias in laboratory animal experimentation within systematic reviews (Hooijmans et al., 2014). The checklist evaluates 10 items relating to selectivity bias, implementation bias, measurement bias, missed visit bias, reporting bias, and other biases. In each study, these domains were judged as “yes,” “no,” or “unclear,” indicating a low, high, or unclear risk of bias, respectively. The methodological quality assessment was conducted independently by two investigators, and any disagreements were resolved through consensus or the involvement of a third investigator (Zhou et al., 2022).
The data from the included studies were analyzed using RevMan 5.3 software. In studies with multiple intervention groups, we combined these groups to conduct a single pairwise comparison (Higgins and Green, 2011). The mean, SD, and sample size of animals in each group were used for comparisons. The effects of the interventions in each study were summarized using weighted mean differences (WMDs) or standardized mean differences, along with 95% confidence intervals (CIs). Heterogeneity among the included studies was assessed using the χ2 test and the Cochrane I2 (Higgins and Green, 2011). The random-effect model was employed due to the expected heterogeneity resulting from the exploratory character of the animal studies. Statistical significance was defined as a P value less than 0.05. Linear graphs were constructed using GraphPad Prism software to illustrate the dynamic WMDs of BBB scores and highlight the variation trend in BBB scores of both groups over time.
Subgroup analyses were conducted to investigate the factors that modify BBB scores, including species, gender, injury type, route of administration, and times and administered dosage. Meanwhile, we conducted sensitivity analyses to evaluate the robustness of our findings and to examine the sources of heterogeneity by excluding studies that lacked outcome assessor blinding, small-sample studies, and individual studies.
In order to investigate the optimal dose, we employed a network meta-analysis approach based on the Bayesian method using Stata 12.0 (StataCorp LP, College Station, Texas, USA) to simultaneously compare the therapeutic effects of different treatment regimens by integrating both direct and indirect evidence. We calculated the probability of each treatment regimen occupying a specific rank and subsequently ranked those treatments based on their surface under the cumulative ranking curve (SUCRA) (Owen et al., 2020).
The screening process for the study is shown in Figure 1. We identified 402 potentially relevant references after searching the databases described above. After eliminating duplicates and conducting an initial screening of the titles and abstracts, 11 publications were identified for full-text evaluation. Of them, one publication was excluded due to repetition of data (Zhao Z. J. et al., 2013). Ten publications (Ishii et al., 2018; Pang et al., 2022; Ohta et al., 2005; Ren et al., 2006; Ohta et al., 2011; Ozgiray et al., 2012; Wang et al., 2013; Song et al., 2015; Li et al., 2018; Xu et al., 2023), then, were eligible for this systematic review.
Of the 10 included publications, eight articles were published in English, and the remaining two were in Chinese (Ren et al., 2006; Li et al., 2018). The sample sizes varied between 24 and 191 rats. All studies except two (Pang et al., 2022; Song et al., 2015) used male rats. Eight publications used a weight-drop impactor to induce the SCI model, and the other two demonstrated an SCI model induced by compression of an aneurysm clip (Ishii et al., 2018; Xu et al., 2023). Almost all publications stated a trauma to the spinal cord at the T8–T11 segment. Six studies reported the source of edaravone procurement (Ishii et al., 2018; Pang et al., 2022; Ohta et al., 2005; Ren et al., 2006; Ohta et al., 2011; Xu et al., 2023). Placebo controls included normal saline, phosphate-buffered saline, and no treatment. The administered doses of edaravone ranged from 2 mg/(kg·d) to 75 mg/(kg·d). Four studies used intraperitoneal injection (Pang et al., 2022; Ohta et al., 2011; Li et al., 2018; Xu et al., 2023), five studies chose the intravenous route, with the remaining one not reporting this detail (Wang et al., 2013). Most studies administered edaravone within 2 h post-injury, although four studies included groups that received the initial edaravone injection at 4–24 h after trauma induction or prior to injury (Ishii et al., 2018; Wang et al., 2013; Song et al., 2015; Li et al., 2018). Four studies administered a single dose of edaravone (Ishii et al., 2018; Ohta et al., 2011; özgiray et al., 2012; Wang et al., 2013), while seven studies used repeated administration for 2–7 days after SCI (Pang et al., 2022; Ohta et al., 2005; Ren et al., 2006; Ohta et al., 2011; Song et al., 2015; Li et al., 2018; Xu et al., 2023). Additionally, two studies employed continuous infusion to administer edaravone (Table 1) (Ishii et al., 2018; Ohta et al., 2011).
As presented in Table 2, the included studies adequately addressed the items of “baseline characteristics” and “selective outcome reporting.” The items of “outcome assessor blinding” and “incomplete outcome data” were adequately addressed in 60% and 50% of the included studies, respectively. None or few studies reported on the items of “sequence generation,” “allocation concealment,” “investigator blinding,” “random housing,” and “random outcome assessment.” Additionally, no additional sources of bias were identified in the included studies.
All studies, except one (özgiray et al., 2012), used BBB scores to evaluate locomotor recovery in rats following treatment with edaravone. The meta-analysis of BBB scores and the variation trend of BBB scores in both groups demonstrated that the edaravone-treated rats showed improved BBB scores compared to the control group. The WMDs between the two groups gradually increased from day 7 (seven studies, n = 246, WMD = 1.96, 95% CI = 1.23 to 2.68, P < 0.00001) to day 28 (seven studies, n = 222, WMD = 4.41, 95% CI = 3.19 to 5.63, P < 0.00001) after injury, and then maintained stably in the remaining time points (Figures 2A–C; Table 3).
Figure 2. Overall analyses of the effects of edaravone on dynamic changes in neurological function. (A) Meta-analysis of BBB score at days 28 after SCI. (B) The BBB scores in each group over time. (C) The WMDs of BBB score between edaravone and control groups from day 7 to day 28 after SCI.
Three studies reported the spared white matter areas in the injury epicenter using the Luxol fast blue method (Ohta et al., 2005; Ren et al., 2006; Ohta et al., 2011). All of them presented this outcome as the ratio of spared white matter area to total spinal cord area. Pooled results from those studies indicated an improvement in the spared white matter area in rats after edaravone treatment (three studies, n = 128, WMD = 10.69, 95% CI = 6.24 to 15.13, P < 0.00001; Figure 3A; Table 3).
Figure 3. Overall analyses of the effects of edaravone on tissue sparing and MDA in the lesion area. (A, B) Meta-analysis of spared white matter area ratio and MDA.
Four studies reported the MDA content in the spinal cord tissue (Ishii et al., 2018; Ohta et al., 2005; Ren et al., 2006; özgiray et al., 2012). The pooled results demonstrated that edaravone intervention reduced MDA levels in the spinal cord compared to the placebo group (four studies, n = 71, WMD = −2.07, 95% CI = −3.56 to −0.58. P = 0.007; Figure 3B; Table 3).
Subgroup analyses revealed no significant differences in locomotor recovery between rats based on gender, strain, number of injections (single versus multiple), or injection routes (intravenous versus intraperitoneal); however, locomotor recovery may be influenced by the type of injury (Figures 4, 5; Supplementary Table S1). Dose was argued to critically impact the efficacy of the pharmacological intervention. While our analysis suggested that edaravone at doses of 5–10 mg/(kg·d) only exhibited a weak tendency to improve BBB scores compared with doses of <5 mg/(kg·d), there was no statistically significant difference. Additionally, administering edaravone at doses exceeding 10 mg/(kg·d) did not result in any further improvement in BBB scores (Figure 5C; Supplementary Table S1). The relatively insufficient discrimination in doses may potentially impact the results of subgroup analyses; further analyses are required to explore the appropriate dose.
Figure 4. Subgroup analysis of BBB scores with respect to rat gender, rat strain, and injury model. (A–C) Subgroup analysis concerning rat gender, rat strain, and injury type at day 28 after SCI and the WMDs of BBB score in each subgroup over time. *p < 0.05.
Figure 5. Subgroup analysis of BBB scores with respect to administration details. (A–C) Subgroup analysis concerning administration methods, administration times, and administered dose at day 28 after SCI and the WMDs of BBB score in each subgroup over time.
Because BBB scores trended stably from the fourth week after SCI, we conducted network analysis using BBB score data from day 28 to day 42 post-injury to compare the effects of edaravone at different doses (Zhou et al., 2019). At day 28 after injury, the forest plot indicated 5–6 mg/(kg·d) edaravone increased BBB scores compared with controls, and increasing the dose beyond 5–6 mg/(kg·d) did not produce better results (Figure 6A). The SUCRA values gradually increased as the daily dose increased initially, then trended stably from 5–6 mg/(kg·d) to the maximal dose (Figures 7A, B). The analysis of BBB scores on day 35 and day 42 after SCI yielded highly consistent results (Figure 6B, 7C; Supplementary Figure S1).
Figure 6. Network analysis of the effects of edaravone at different doses. (A) Forest plot of effect size in different edaravone doses according to data at day 28. (B) Forest plot of effect size in different edaravone doses according to data at day 42. a, control; b, 2–3 mg/(kg·d) edaravone; c, 5–6 mg/(kg·d) edaravone; d, 9–10 mg/(kg·d) edaravone; e, 20–23 mg/(kg·d) edaravone; f, 40 mg/(kg·d) edaravone; g, 75 mg/(kg·d) edaravone.
Figure 7. The SUCRA value and ranking probabilities of each treatment dose. (A) SUCRA value ranking of different administered doses according to data at day 28. (B) Histogram of the ranking probability of each treatment dose according to data at day 28. (C) SUCRA value ranking of different administered doses according to data at day 42. (D) Histogram of the ranking probability of each treatment dose according to data at day 42. a, control; b, 2–3 mg/(kg·d) edaravone; c, 5–6 mg/(kg·d) edaravone; d, 9–10 mg/(kg·d) edaravone; e, 20–23 mg/(kg·d) edaravone; f, 40 mg/(kg·d) edaravone; g, 75 mg/(kg·d) edaravone.
Sensitivity analyses were conducted by excluding studies that lacked outcome assessor blinding, small-sample studies, and individual studies. The heterogeneity of the inter-study statistics remained to be moderate or high after using all exclusion methods. The results of sensitivity analyses showed that the improvement of BBB scores after edaravone intervention remained largely unchanged across various exclusion strategies (Supplementary Table S2).
Table 4 shows the proposed mechanisms of edaravone in the included studies. Collectively, the neuroprotective mechanisms of edaravone for SCI primarily involve the mitigation of oxidative stress, suppression of neuroinflammation, reduction in neuronal loss, and attenuation of ferroptosis.
The present systematic review included ten eligible studies to analyze the efficacy and potential mechanism of edaravone in treating SCI. The pairwise meta-analysis revealed a significant increase in BBB scores in rats treated with edaravone compared to the control group. The WMDs of BBB scores between the two groups exhibited a gradual increase from day 7 to day 28 post-injury, after which they stabilized during the subsequent observation period. Meanwhile, rats treated with edaravone showed a higher preservation rate of white matter areas at the lesion site. Additionally, the pooled data indicated that edaravone treatment resulted in a reduction in MDA levels in rats subjected to SCI. Those results demonstrated the positive efficacy of edaravone in enhancing locomotor recovery, reducing tissue damage, and alleviating oxidative stress in experimental SCI models.
The subgroup analyses indicated that the improvement in BBB scores was superior in compression injury models than in contusion models. Analyses with respect to the daily dose revealed no statistically significant differences in locomotor recovery across various daily doses of edaravone. However, the data suggested an initial upward trend followed by a plateau in the improvement of BBB scores as the dose increased. Based on this observation, we conducted a network meta-analysis to determine the optimal dosage of edaravone. The results confirmed the trends identified in the subgroup analysis and provided more comprehensive evidence to predict the appropriate dose of edaravone in treating experimental SCI.
For many drugs, the augment of the therapeutic effect gradually slows with the increase of dosage, and the side effects significantly escalate as the dosage approaches its maximum levels (Riddle, 2000; Huscher et al., 2009). Administration of edaravone at doses of 1–75 mg/(kg·d) produced a protective effect for SCI models in included studies (Ishii et al., 2018; Ohta et al., 2011). Ohta et al. (2011) conducted a comparative study on the neuroprotective effect of different doses of edaravone in rats following SCI. Their findings demonstrated that the intravenous administration of 6 mg/kg edaravone daily resulted in significant improvements in locomotor function and tissue damage compared to control animals, while no further significant improvement was observed with the administration of 20 mg/(kg·d) and 40 mg/(kg·d) edaravone (Ohta et al., 2011). Consistently, our subgroup analysis suggested a tendency of improved BBB scores at doses of 5–10 mg/(kg·d) compared to the lower dose, but administration of the larger dose of edaravone did not result in further improvement in BBB scores. In network analyses, within daily doses of 5–6 mg/kg, the higher dose of edaravone is associated with a superior SUCRA, whereas administration of edaravone at doses of 9–10 mg/(kg·d), 20–23 mg/(kg·d), 40 mg/(kg·d), and 75 mg/(kg·d) did not further increase the SUCRA. Considering the potential side effects associated with high intervention doses, administration of 5–6 mg/(kg·d) edaravone may be considered appropriate for future pre-clinical investigations and could serve as a reference for potential clinical trials.
Different injury mechanisms are associated with various pathological processes and may potentially impact the effectiveness of specific treatment modalities (Geremia et al., 2017). Our subgroup analyses revealed edaravone could result in a superior locomotory recovery in rats with compression injury, implying this agent is more appropriate for SCI induced by compression insult. Actually, this phenomenon is predictable due to the pathological features of compression injury. There are several similarities in the pathological alterations observed in compression and contusion SCIs. Prolonged compression, however, can lead to a reduction in blood flow, blood oxygen level, and glucose supply at the site of compression, thereby inducing electron leakage in mitochondria and the production of free radicals (Abraham et al., 1985; Ueta et al., 1992; Okon et al., 2013). Subsequently, the reperfusion event results in an enlargement of the damaged area and degree by boosting ROS production (Abraham et al., 1985). The processes of ischemia and reperfusion disrupt the metabolism of free radicals and energy at the injury site and powerfully contribute to excessive oxidative stress (Ling et al., 2021). Therefore, compression SCIs may exhibit more pronounced oxidative stress than contusion injuries. As mentioned above, edaravone is well-known for its excellent antioxidant effect. Then, the powerful antioxidant role endows edaravone to elicit superior applicability in compression SCIs. Nevertheless, this hypothesis needs further studies to verify.
Based on the proposed mechanisms outlined in the included studies and relevant supporting evidence, the potential underlying mechanisms of edaravone are as follows:
Following SCI, a substantial accumulation of free radicals occurs rapidly and persistently at the site of injury, triggering a cascade of pathological events during the secondary injury phase (Zrzavy et al., 2021; Peng et al., 2023; Yu et al., 2023). Superoxide dismutase (SOD), a type of metalloenzyme, plays a pivotal role in scavenging free radicals of superoxide anions and serves as a crucial component in the detoxification of ROS (Wang et al., 2018). While MDA is mainly produced as a product of the lipid peroxidation reaction triggered by ROS, it subsequently amplifies the occurrence of secondary harmful reactions and the generation of ROS (Wang et al., 2019). The antioxidative capacity of edaravone has been proved in numerous central nervous system (CNS) diseases (Jiao et al., 2015; Ahmadinejad et al., 2017; Jaiswal, 2019). Five of the included studies reported a decreased level of MDA or an enhanced SOD content in rats with SCI treated with edaravone (Ishii et al., 2018; Ohta et al., 2005; Ren et al., 2006; Ohta et al., 2011; özgiray et al., 2012). The meta-analysis revealed that edaravone resulted in a reduction in MDA levels in the spinal cord compared to the placebo group, verifying the favorable antioxidative role of edaravone in treating SCI.
The combination of a direct and indirect role in free radicals gives edaravone a powerful antioxidative property (Watanabe et al., 2018; Ismail et al., 2020). At a physiological pH, approximately half of edaravone exists as edaravone anions, allowing this agent to directly trap hydroxyl radicals and quench active oxygen by electron transfer or reaction with oxygen molecules (Watanabe et al., 2018; Higashi et al., 2006). In addition, various signaling pathways act as intermediary links for the antioxidant mechanism of edaravone (Ismail et al., 2020; Zhang et al., 2019). Nuclear factor erythroid 2-related factor 2 (Nrf2) and sirtuin 1 (SIRT1) can modulate mitochondrial homeostasis and antioxidant enzyme activity to defend against oxidative stress, while inactivation of these molecules occurs in SCI and related CNS diseases (Samarghandian et al., 2020; Jiang et al., 2023). In a pheochromocytoma cell 12 (PC12) model of neurotoxicity, edaravone was shown to enhance Nrf2 expression, reduce the generation of ROS, and promote cell survival, which was abolished by Nrf2 gene knockdown (Shou et al., 2019). Meanwhile, edaravone treatment has been shown to activate Nrf2, heme oxygenase 1, and SIRT1 in experimental models of traumatic brain injury, amyotrophic lateral sclerosis, and stroke (Ismail et al., 2020; Cui X. et al., 2022). Collectively, the unique structure of edaravone, along with the involvement of Nrf2 and SIRT1, potentially mediates its role in attenuating oxidative stress to mitigate disorders in neurological function and tissue structure in SCI.
Microglia, the resident immune cells in the CNS, respond to the initial injury of the spinal cord within a few hours and facilitate the activation of astrocytes as well as infiltration of peripheral immune cells at the injury site. Subsequently, an inflammatory cascade is initiated (Hu et al., 2023). Scavenging of ROS is of significance for ameliorating neuroinflammatory responses (Zrzavy et al., 2021). Correspondingly, Pang et al. (2022) demonstrated that edaravone effectively suppresses the activation of microglia and astrocytes, thereby mitigating inflammatory responses in SCI. Moreover, the expression of anti-inflammatory factors, such as IL-10 and IL-13, was upregulated by edaravone to effectively attenuate neuroinflammation (Pang et al., 2022). Nuclear transcription factor-κB (NF-κB) and the NOD-like receptor family pyrin domain-containing 3 (NLRP3) inflammasome function as downstream signals of ROS, playing pivotal roles in regulating persistent inflammatory responses during SCI (Gao et al., 2019; Liu et al., 2020). In HT22 cells stimulated by amyloid β or hydrogen peroxide, edaravone was shown to ameliorate inflammatory response and cell apoptosis by diminishing ROS generation, inhibiting NF-κB p65 phosphorylation, and suppressing NLRP3 inflammasome activation (Zhao Z. Y. et al., 2013; Guo et al., 2023). Edaravone reduced the expression of NLRP3, caspase-1, and associated speck-like protein containing a caspase-recruitment domain to blunt the microglial activation induced by lipopolysaccharide (Li J. et al., 2021). Evidence from the in vivo studies confirmed the inhibitory effect of edaravone on ROS generation, NLRP3 inflammasome, and nuclear transcription of NF-κB in multiple neurological disorders (Akiyama et al., 2017; Miao et al., 2020; Meng et al., 2021). Therefore, the suppression of neuroinflammation by edaravone may be attributed to its ability to inhibit free radicals and downstream signaling pathways, including NF-κB and NLRP3 inflammasome.
The generation of large numbers of free radicals and a vigorous inflammatory cascade response following injury can notably disturb the organelle function and homeostasis in neuronal cells, leading to numerous neuron apoptosis (Zrzavy et al., 2021; Sun et al., 2024). In the experimental treatment of SCI, it was reported that edaravone increased the survival rate of neuron cells in the lesion area (Ishii et al., 2018; Pang et al., 2022). Our meta-analysis also revealed edaravone can mitigate tissue damage in the lesion area. The B-cell lymphoma-extra-large (Bcl-XL) and caspases are involved in the cell apoptosis event resulting from oxidative stress (Liu et al., 2022). Administration of edaravone could result in a significant decrease in caspase-3 positive cells and a rise in Bcl-XL levels in rats with SCI (Wang et al., 2013). As mentioned above, Nrf2 and SIRT1 are important regulators of oxidative stress (Samarghandian et al., 2020; Jiang et al., 2023). Interestingly, edaravone was suggested to effectively boost the activation of Nrf2 and SIRT1 to improve the neuron apoptosis caused by excessive generation of ROS during brain injury (Shou et al., 2019; Li X. et al., 2021; Cui X. P. et al., 2022). Collectively, a rational molecular chain comprising Nrf2 (or SIRT1), ROS, and Bcl-XL (or caspase-3) may account for the anti-apoptotic mechanism of edaravone in treating SCI and related CNS diseases.
The rapid and persistent oxidative stress can induce iron overload, impairing the activity of glutathione peroxidase 4 and reinforcing the buildups of ROS in return. This leads to structural damage to the cellular membrane and ultimately causes cell death (Feng et al., 2021; Zhang et al., 2022). Notably, edaravone treatment after SCI was suggested to upregulate anti-ferroptosis proteins, including glutathione peroxidase 4 and system Xc-light chain, and exert inhibitory effects on pro-ferroptosis proteins (Pang et al., 2022), implying that an anti-ferroptosis mechanism participates in the neuroprotective effect of edaravone. The detailed molecular mechanism behind the anti-ferroptosis of edaravone remains to be further illustrated in future studies.
Taken together, we hypothesize that the core mechanism through which edaravone exerts its neuroprotective effects is by attenuating oxidative stress. Partly due to its antioxidant effects, edaravone then counteracts neuroinflammatory responses, attenuates neuronal cell apoptosis, and suppresses ferroptosis through diverse molecular pathways for the treatment of SCI (Figure 8). However, more high-quality studies are required to verify this hypothesis.
Figure 8. Potential action mechanisms of edaravone against SCI. Following the primary insult, large quantities of free radicals will rapidly and persistently accumulate at the site of injury, leading to neuroinflammation, neuronal apoptosis, and cell ferroptosis. Edaravone executes a satisfactory role in blunting oxidative stress by transferring electrons, reacting with oxygen molecules, and activating SIRT1 and Nrf2 pathways. Partly depending on the antioxidant effects, edaravone then inhibits the NLRP3 inflammasome or NF-κB signaling to counteract neuroinflammatory responses, regulate the caspase, Bax, and caspase family proteins to attenuate neuronal cell apoptosis, and modulate the ferroptosis-related proteins to suppress ferroptosis, finally exerting neuroprotective roles in treating SCI rats.
To the best of our knowledge, this meta-analysis represents the first quantitative analysis of edaravone’s therapeutic efficacy in treating SCI. In this review, our primary focus is on the dynamic fluctuations of BBB scores following intervention with edaravone, while also addressing its tissue-protective and antioxidant effects in the context of SCI. The network analyses provide a possible reference for the candidate dose of edaravone. Additionally, we have proposed a mechanistic framework elucidating the neuroprotective effects of edaravone in SCI through an amalgamation of systematic and traditional review approaches, culminating in the construction of a schematic representation depicting these underlying mechanisms. In order to conduct subsequent pre-clinical investigations and assess its potential application in clinical settings, it is essential to comprehend the effectiveness, specifics of administration, and mechanism of action for a particular agent. Our study conducted a comprehensive analysis of the parameters associated with edaravone, aiming to advance basic research on SCI and contribute to the discovery of novel clinical therapies for this condition.
Several limitations should be considered. First, due to the limited data available from studies investigating the efficacy of edaravone in SCI animal models, we selected the outcomes of BBB score, white matter integrity, and MDA for our protocol development and subsequent analyses. Combining these three indicators has certain advantages in demonstrating edaravone’s efficacy by providing complementary validation through objective behavioral scales, pathological assessments, and biochemical measurements. However, these indicators are still insufficient for a comprehensive evaluation of the efficacy of edaravone. Future research is needed to further enrich other dimensions of data and facilitate a more thorough understanding of the effects of edaravone on SCI. Second, we selected data with BBB scores from day 28–42 post-injury for network analysis because BBB scores tended to stabilize after day 28. This approach allows for a focused evaluation of the efficacy of different drug doses on functional recovery at these important timepoints. However, this method was insufficient in reflecting the differential effects of various doses on early and long-term functional recovery following SCI, potentially leading to an incomplete understanding of the efficacy of different doses. Third, although some factors were reported to modulate edaravone’s efficacy, subgroup analyses showed that most did not significantly impact the therapeutic effect of edaravone. It is important to note that subgroup analysis should be seen as a tool for generating hypotheses rather than confirming them. More studies aimed at examining differential effects across subgroups in more detail are needed. Fourth, similar to many other meta-analyses on animal models, our analyses reveal significant heterogeneity in treatment outcomes. Based on the results from subgroup analyses and sensitivity analyses, the high heterogeneity between studies appeared to be associated with the differences in injection dose, administration mode, injury type, rat gender and strain, and the methodological quality of studies. Given the substantial heterogeneity among the studies, the findings of this review should be interpreted with caution.
In conclusion, pooled data analysis from this review supports using edaravone treatment to enhance motor recovery, ameliorate tissue damage, and reduce oxidative stress in rat models of SCI. The recommended dosage is 5–6 mg/kg daily. Based on the literature data, edaravone can alleviate oxidative stress, suppress neuroinflammation, reduce neuronal loss, and counteract ferroptosis in the treatment of SCI. Due to its protective effect and systematic action mechanism, edaravone shows promise as a neuroprotective agent for the research and treatment of SCI. However, considering the limitations in the design of the included studies, further high-quality research is needed to support the effectiveness of edaravone as a therapeutic intervention for SCI.
The original contributions presented in the study are included in the article/Supplementary Material, further inquiries can be directed to the corresponding author.
L-yZ: Data curation, Formal Analysis, Visualization, writing–original draft. X-bW: Data curation, Formal Analysis, Visualization, Writing–original draft. X-qC: Methodology, Data curation, Writing–original draft. RL: Methodology, Writing–original draft; B-bY: Methodology, Validation, Writing–original draft. M-xP: Data curation, Validation, Writing–original draft. LF: Data curation, Validation, Writing–original draft. JL: Data curation, Visualization, Writing–original draft. J-xC: Methodology, Validation, Writing–review and editing. MY: Conceptualization, Supervision, Writing–review and editing. XL: Conceptualization, Supervision, Writing–review and editing
The author(s) declare that financial support was received for the research and/or publication of this article. This study was supported by the National Natural Science Foundation of China (Grant No. 82205148, 82374527, 82374476, 82074454, and 82072546) and the Medical Innovation Team (Grant No. CXZX202222).
The authors declare that the research was conducted in the absence of any commercial or financial relationships that could be construed as a potential conflict of interest.
The author(s) declare that no Generative AI was used in the creation of this manuscript.
All claims expressed in this article are solely those of the authors and do not necessarily represent those of their affiliated organizations, or those of the publisher, the editors and the reviewers. Any product that may be evaluated in this article, or claim that may be made by its manufacturer, is not guaranteed or endorsed by the publisher.
The Supplementary Material for this article can be found online at: https://www.frontiersin.org/articles/10.3389/fphar.2025.1538879/full#supplementary-material
Abraham, J., Balasubramanian, A. S., Theodore, D. R., Nagarajan, S., Apte, C. A., and Chandi, S. (1985). Spinal cord edema, 5-hydroxytryptamine, lipid peroxidation, and lysosomal enzyme release after acute contusion and compression injury in primates. Cent. Nerv. Syst. Trauma 2 (1), 45–60. doi:10.1089/cns.1985.2.45
Ahmadinejad, F., Geir, M. S., Hashemzadeh-Chaleshtori, M., Bidkhori, G., and Jami, M. S. (2017). Molecular mechanisms behind free radical scavengers function against oxidative stress. Antioxidants 6 (3), 51. doi:10.3390/antiox6030051
Akiyama, G., Azuchi, Y., Guo, X., Noro, T., Kimura, A., Harada, C., et al. (2017). Edaravone prevents retinal degeneration in adult mice following optic nerve injury. Invest. Ophthalmol. Vis. Sci. 58 (11), 4908–4914. doi:10.1167/iovs.17-22250
Baroudi, M., Rezk, A., Daher, M., Balmaceno-Criss, M., Gregoryczyk, J. G., Sharma, Y., et al. (2024). Management of traumatic spinal cord injury: a current concepts review of contemporary and future treatment. Injury 55 (6), 111472. doi:10.1016/j.injury.2024.111472
Cui, X., Ye, J., Lin, H., Zhou, H., Ye, S., and Mu, J. (2022). Effects of edaravone combined with Oxiracetam on neuronal apoptosis in rats with cerebral infarction through targeting SIRT1/NF-κB inflammatory pathway. Eur. Rev. Med. Pharmacol. Sci. 26 (1), 218–224. doi:10.26355/eurrev_202201_27771
Cui, X. P., Ye, J. X., Lin, H., Zhou, H., Ye, S., and Mu, J. S. (2022). Effects of edaravone combined with Oxiracetam on neuronal apoptosis in rats with cerebral infarction through targeting SIRT1/NF-κB inflammatory pathway. Eur. Rev. Med. Pharmacol. Sci. 26 (1), 218–224. doi:10.26355/eurrev_202201_27771
Fehlings, M. G., Pedro, K., and Hejrati, N. (2022). Management of acute spinal cord injury: where have we been? Where are we now? Where are we going? J. Neurotrauma 39 (23-24), 1591–1602. doi:10.1089/neu.2022.0009
Feng, Z., Min, L., Chen, H., Deng, W., Tan, M., Liu, H., et al. (2021). Iron overload in the motor cortex induces neuronal ferroptosis following spinal cord injury. Redox Biol. 43, 101984. doi:10.1016/j.redox.2021.101984
Gao, S., Xu, T., Guo, H., Deng, Q., Xun, C., Liang, W., et al. (2019). Ameliorative effects of echinacoside against spinal cord injury via inhibiting NLRP3 inflammasome signaling pathway. Life Sci. 237, 116978. doi:10.1016/j.lfs.2019.116978
Genge, A., Brooks, B. R., Oskarsson, B., Kalin, A., Ji, M., Apple, S., et al. (2022). Analysis of the US safety data for edaravone (Radicava®) from the third year after launch. Drugs R&D. 22 (3), 205–211. doi:10.1007/s40268-022-00391-6
Geremia, N. M., Hryciw, T., Bao, F., Streijger, F., Okon, E., Lee, J., et al. (2017). The effectiveness of the anti-CD11d treatment is reduced in rat models of spinal cord injury that produce significant levels of intraspinal hemorrhage. Exp. Neurol. 295, 125–134. doi:10.1016/j.expneurol.2017.06.002
Guan, B., Anderson, D. B., Chen, L., Feng, S., and Zhou, H. (2023). Global, regional and national burden of traumatic brain injury and spinal cord injury, 1990-2019: a systematic analysis for the Global Burden of Disease Study 2019. BMJ Open 13 (10), e075049. doi:10.1136/bmjopen-2023-075049
Guo, S., Lei, Q., Guo, H., Yang, Q., Xue, Y., and Chen, R. (2023). Edaravone attenuates aβ 1-42-induced inflammatory damage and ferroptosis in HT22 cells. Neurochem. Res. 48 (2), 570–578. doi:10.1007/s11064-022-03782-y
Higashi, Y., Jitsuiki, D., Chayama, K., and Yoshizumi, M. (2006). Edaravone (3-methyl-1-phenyl-2-pyrazolin-5-one), a novel free radical scavenger, for treatment of cardiovascular diseases. Recent Pat. Cardiovasc. drug Discov. 1 (1), 85–93. doi:10.2174/157489006775244191
Higgins, J., and Green, S. (2011). Cochrane handbook for systematic reviews of interventions version 5.1.0. The Cochrane Collaboration. Available at: www.handbook.cochrane.org
Hooijmans, C. R., Rovers, M. M., de Vries, R. B., Leenaars, M., Ritskes-Hoitinga, M., and Langendam, M. W. (2014). SYRCLE's risk of bias tool for animal studies. BMC Med. Res. Methodol. 14, 43. doi:10.1186/1471-2288-14-43
Hu, X., Xu, W., Ren, Y., Wang, Z., He, X., Huang, R., et al. (2023). Spinal cord injury: molecular mechanisms and therapeutic interventions. Signal Transduct. Target. Ther. 8 (1), 245. doi:10.1038/s41392-023-01477-6
Huscher, D., Thiele, K., Gromnica-Ihle, E., Hein, G., Demary, W., Dreher, R., et al. (2009). Dose-related patterns of glucocorticoid-induced side effects. Ann. Rheum. Dis. 68 (7), 1119–1124. doi:10.1136/ard.2008.092163
Ishii, H., Petrenko, A. B., Sasaki, M., Satoh, Y., Kamiya, Y., Tobita, T., et al. (2018). Free radical scavenger edaravone produces robust neuroprotection in a rat model of spinal cord injury. Brain Res. 1682, 24–35. doi:10.1016/j.brainres.2017.12.035
Ismail, H., Shakkour, Z., Tabet, M., Abdelhady, S., Kobaisi, A., Abedi, R., et al. (2020). Traumatic brain injury: oxidative stress and novel anti-oxidants such as mitoquinone and edaravone. Antioxidants 9 (10), 943. doi:10.3390/antiox9100943
Jaiswal, M. K. (2019). Riluzole and edaravone: a tale of two amyotrophic lateral sclerosis drugs. Med. Res. Rev. 39 (2), 733–748. doi:10.1002/med.21528
James, S. L., Theadom, A., Ellenbogen, R. G., Bannick, M. S., Montjoy-Venning, W., Lucchesi, L. R., et al. (2019). Global, regional, and national burden of traumatic brain injury and spinal cord injury, 1990–2016: a systematic analysis for the Global Burden of Disease Study 2016. Lancet Neurology 18 (1), 56–87. doi:10.1016/S1474-4422(18)30415-0
Jazayeri, S. B., Maroufi, S. F., Ghodsi, Z., Ghawami, H., Pourrashidi, A., Amirjamshidi, A., et al. (2022). Adopting clinical practice guidelines for pharmacologic management of acute spinal cord injury from a developed world context to a developing global region. Med 25 (6), 353–359. doi:10.34172/aim.2022.58
Jiang, T., Qin, T., Gao, P., Tao, Z., Wang, X., Wu, M., et al. (2023). SIRT1 attenuates blood-spinal cord barrier disruption after spinal cord injury by deacetylating p66Shc. Redox Biol. 60, 102615. doi:10.1016/j.redox.2023.102615
Jiao, S. S., Yao, X. Q., Liu, Y. H., Wang, Q. H., Zeng, F., Lu, J. J., et al. (2015). Edaravone alleviates Alzheimer's disease-type pathologies and cognitive deficits. Proc. Natl. Acad. Sci. U. S. A. 112 (16), 5225–5230. doi:10.1073/pnas.1422998112
Leenaars, M., Hooijmans, C. R., van Veggel, N., ter Riet, G., Leeflang, M., Hooft, L., et al. (2012). A step-by-step guide to systematically identify all relevant animal studies. Lab. Anim. 46 (1), 24–31. doi:10.1258/la.2011.011087
Li, J., Dai, X., Zhou, L., Li, X., and Pan, D. (2021). Edaravone plays protective effects on LPS-induced microglia by switching M1/M2 phenotypes and regulating NLRP3 inflammasome activation. Front. Pharmacol. 12, 691773. doi:10.3389/fphar.2021.691773
Li, R. B., Yu, G. S., Lin, Y. B., Zhou, J. F., Huang, Y. Q., and Zheng, W. (2018). Edaravone effects on the expression levels of collagen type i and iv after spinal cord injury in rats. Chin. J. Tissue Eng. Res. 22 (8), 1235–1240. doi:10.3969/j.issn.2095-4344.0142
Li, X., Yu, J., Ma, D., and Weng, X. (2021). Edaravone improves the post-traumatic brain injury dysfunction in learning and memory by modulating Nrf2/ARE signal pathway. Clinics 76, e3131. doi:10.6061/clinics/2021/e3131
Ling, X., Lu, J., Yang, J., Qin, H., Zhao, X., Zhou, P., et al. (2021). Non-coding RNAs: emerging therapeutic targets in spinal cord ischemia-reperfusion injury. Front. Neurol. 12, 680210. doi:10.3389/fneur.2021.680210
Liu, Y., Meng, X., Sun, L., Pei, K., Chen, L., Zhang, S., et al. (2022). Protective effects of hydroxy-alpha-sanshool from the pericarp of Zanthoxylum bungeanum Maxim. On D-galactose/AlCl(3)-induced Alzheimer's disease-like mice via Nrf2/HO-1 signaling pathways. Eur. J. Pharmacol. 914, 174691. doi:10.1016/j.ejphar.2021.174691
Liu, Z., Yao, X., Jiang, W., Li, W., Zhu, S., Liao, C., et al. (2020). Advanced oxidation protein products induce microglia-mediated neuroinflammation via MAPKs-NF-κB signaling pathway and pyroptosis after secondary spinal cord injury. J. Neuroinflammation 17 (1), 90. doi:10.1186/s12974-020-01751-2
Meng, W., Wang, X., Lu, L., and Wei, Y. (2021). Effects of edaravone on cerebral ischemia-reperfusion in mice through the TLR4/NF-κB/TNF-α pathway. Panminerva Medica 63 (3), 383–384. doi:10.23736/S0031-0808.19.03693-0
Miao, H., Jiang, Y., Geng, J., Zhang, B., Zhu, G., and Tang, J. (2020). Edaravone administration confers neuroprotection after experimental intracerebral hemorrhage in rats via NLRP3 suppression. Dis. 29 (1), 104468. doi:10.1016/j.jstrokecerebrovasdis.2019.104468
Ohta, S., Iwashita, Y., Kakinoki, R., Noguchi, T., and Nakamura, T. (2011). Effects of continuous intravenous infusion of MCI-186 on functional recovery after spinal cord injury in rats. J. Neurotrauma 28 (2), 289–298. doi:10.1089/neu.2010.1477
Ohta, S., Iwashita, Y., Takada, H., Kuno, S., and Nakamura, T. (2005). Neuroprotection and enhanced recovery with edaravone after acute spinal cord injury in rats. SPINE 30 (10), 1154–1158. doi:10.1097/01.brs.0000162402.79482.fd
Okon, E. B., Streijger, F., Lee, J. H., Anderson, L. M., Russell, A. K., and Kwon, B. K. (2013). Intraparenchymal microdialysis after acute spinal cord injury reveals differential metabolic responses to contusive versus compressive mechanisms of injury. J. Neurotrauma 30 (18), 1564–1576. doi:10.1089/neu.2013.2956
Owen, P. J., Miller, C. T., Mundell, N. L., Verswijveren, S. J. J. M., Tagliaferri, S. D., Brisby, H., et al. (2020). Which specific modes of exercise training are most effective for treating low back pain? Network meta-analysis. Br. J. Sports Med. 54 (21), 1279–1287. doi:10.1136/bjsports-2019-100886
Özgiray, E., Serarslan, Y., öztürk, O. H., Altaş, M., Aras, M., Söǧüt, S., et al. (2012). Protective effects of edaravone on experimental spinal cord injury in rats. Pediatr. Neurosurg. 47 (4), 254–260. doi:10.1159/000335400
Pang, Y., Liu, X., Wang, X., Shi, X., Ma, L., Zhang, Y., et al. (2022). Edaravone modulates neuronal GPX4/ACSL4/5-LOX to promote recovery after spinal cord injury. Front. Cell. Dev. Biol. 10, 849854. doi:10.3389/fcell.2022.849854
Peng, C., Luo, J., Wang, K., Li, J., Ma, Y., Li, J., et al. (2023). Iridium metal complex targeting oxidation resistance 1 protein attenuates spinal cord injury by inhibiting oxidative stress-associated reactive oxygen species. Redox Biol. 67, 102913. doi:10.1016/j.redox.2023.102913
Picetti, E., Iaccarino, C., Coimbra, R., Abu-Zidan, F., Tebala, G. D., Balogh, Z. J., et al. (2022). The acute phase management of spinal cord injury affecting polytrauma patients: the ASAP study. World J. Emerg. Surg. 17 (1), 20. doi:10.1186/s13017-022-00422-2
Ren, X., Yang, Y., and Jiao, J. (2006). The neuroprotection effect of edaravone on spinal cord injury in rats. Chin. J. Rehabilitation Med. 21 (8), 686–688.
Riddle, M. (2000). Combining sulfonylureas and other oral agents. Am. J. Med. 108 (6), 15S–22S. doi:10.1016/S0002-9343(00)00338-7
Safdarian, M., Trinka, E., Rahimi-Movaghar, V., Thomschewski, A., Aali, A., Abady, G. G., et al. (2023). Global, regional, and national burden of spinal cord injury, 1990–2019: a systematic analysis for the Global Burden of Disease Study 2019. Lancet Neurology 22 (11), 1026–1047. doi:10.1016/S1474-4422(23)00287-9
Samarghandian, S., Pourbagher-Shahri, A. M., Ashrafizadeh, M., Khan, H., Forouzanfar, F., Aramjoo, H., et al. (2020). A pivotal role of the Nrf2 signaling pathway in spinal cord injury: a prospective therapeutics study. CNS Neurol. Disord.-Drug Targets 19 (3), 207–219. doi:10.2174/1871527319666200604175118
Shou, L., Bei, Y., Song, Y., Wang, L., Ai, L., Yan, Q., et al. (2019). Nrf2 mediates the protective effect of edaravone after chlorpyrifos-induced nervous system toxicity. Environ. Toxicol. 34 (5), 626–633. doi:10.1002/tox.22728
Sies, H., and Jones, D. P. (2020). Reactive oxygen species (ROS) as pleiotropic physiological signalling agents. Nat. Rev. Mol. Cell Biol. 21 (7), 363–383. doi:10.1038/s41580-020-0230-3
Sng, K. S., Li, G., Zhou, L. Y., Song, Y. J., Chen, X. Q., Wang, Y. J., et al. (2022). Ginseng extract and ginsenosides improve neurological function and promote antioxidant effects in rats with spinal cord injury: a meta-analysis and systematic review. J. Ginseng Res. 46 (1), 11–22. doi:10.1016/j.jgr.2021.05.009
Song, Y. Y., Peng, C. G., and Ye, X. B. (2015). Combination of edaravone and neural stem cell transplantation repairs injured spinal cord in rats. Genet. Mol. Res. 14 (4), 19136–19143. doi:10.4238/2015.December.29.23
Sun, Y., Song, X., Geng, Z., Xu, Y., Xiao, L., Chen, Y., et al. (2024). IL-11 ameliorates oxidative stress damage in neurons after spinal cord injury by activating the JAK/STAT signaling pathway. Int. Immunopharmacol. 127, 111367. doi:10.1016/j.intimp.2023.111367
Tran, A. P., Warren, P. M., and Silver, J. (2018). The biology of regeneration failure and success after spinal cord injury. Physiol. Rev. 98 (2), 881–917. doi:10.1152/physrev.00017.2017
Ueta, T., Owen, J. H., and Sugioka, Y. (1992). Effects of compression on physiologic integrity of the spinal cord, on circulation, and clinical status in four different directions of compression: posterior, anterior, circumferential, and lateral. SPINE 17 (8 Suppl. l), S217–S226. doi:10.1097/00007632-199208001-00002
Wang, J., Guo, G., Wang, W., Tang, Y., Shun, J., Zhou, X., et al. (2013). Effect of methylprednisolone and edaravone administration on spinal cord injury. Eur. Rev. Med. Pharmacol. Sci. 17 (20), 2766–2772.
Wang, X. B., Zhou, L. Y., Chen, X. Q., Li, R., Yu, B. B., Pan, M. X., et al. (2023). Neuroprotective effect and possible mechanism of edaravone in rat models of spinal cord injury: a protocol for a systematic review and meta-analysis. Syst. Rev. 12 (1), 177. doi:10.1186/s13643-023-02306-1
Wang, Y., Branicky, R., Noë, A., and Hekimi, S. (2018). Superoxide dismutases: dual roles in controlling ROS damage and regulating ROS signaling. J. Cell. Biol. 217 (6), 1915–1928. doi:10.1083/jcb.201708007
Wang, Z., He, Z., Emara, A. M., Gan, X., and Li, H. (2019). Effects of malondialdehyde as a byproduct of lipid oxidation on protein oxidation in rabbit meat. Food Chem. 288, 405–412. doi:10.1016/j.foodchem.2019.02.126
Watanabe, K., Tanaka, M., Yuki, S., Hirai, M., and Yamamoto, Y. (2018). How is edaravone effective against acute ischemic stroke and amyotrophic lateral sclerosis? J. Clin. Biochem. Nutr. 62 (1), 20–38. doi:10.3164/jcbn.17-62
Xu, B., Fang, J., Wang, J., Jin, X., Liu, S., Song, K., et al. (2023). Inhibition of autophagy and RIP1/RIP3/MLKL-mediated necroptosis by edaravone attenuates blood spinal cord barrier disruption following spinal cord injury. Biomed. Pharmacother. 165, 115165. doi:10.1016/j.biopha.2023.115165
Xu, J., Wang, Y., Wang, A., Gao, Z., Gao, X., Chen, H., et al. (2019). Safety and efficacy of Edaravone Dexborneol versus edaravone for patients with acute ischaemic stroke: a phase II, multicentre, randomised, double-blind, multiple-dose, active-controlled clinical trial. Stroke Vasc. Neurol. 4 (3), 109–114. doi:10.1136/svn-2018-000221
Yao, M., Yang, L., Wang, J., Sun, Y., Dun, R., Wang, Y., et al. (2015). Neurological recovery and antioxidant effects of curcumin for spinal cord injury in the rat: a network meta-analysis and systematic review. J. Neurotrauma. 32 (6), 381–391. doi:10.1089/neu.2014.3520
Yu, M., Wang, Z., Wang, D., Aierxi, M., Ma, Z., and Wang, Y. (2023). Oxidative stress following spinal cord injury: from molecular mechanisms to therapeutic targets. J. Neurosci. Res. 101 (10), 1538–1554. doi:10.1002/jnr.25221
Zaric, B. L., Macvanin, M. T., and Isenovic, E. R. (2023). Free radicals: relationship to human diseases and potential therapeutic applications. Int. J. Biochem. and Cell Biol. 154, 106346. doi:10.1016/j.biocel.2022.106346
Zhang, L., Guo, Y., Wang, H., Zhao, L., Ma, Z., Li, T., et al. (2019). Edaravone reduces Aβ-induced oxidative damage in SH-SY5Y cells by activating the Nrf2/ARE signaling pathway. Life Sci. 221, 259–266. doi:10.1016/j.lfs.2019.02.025
Zhang, Y., Fang, J., Dong, Y., Ding, H., Cheng, Q., Liu, H., et al. (2022). High-altitude hypoxia exposure induces iron overload and ferroptosis in adipose tissue. Antioxidants 11 (12), 2367. doi:10.3390/antiox11122367
Zhao, Z. J., Sun, Z. M., Zhang, C. Y., Han, J. G., Shi, R. C., and Wang, W. Z. (2013). Edaravone combined with neural stem cell transplantation for treatment of spinal cord injury in rats. Chin. J. Tissue Eng. Res. 17 (10), 1862–1867. doi:10.3969/j.issn.2095-4344.2013.10.024
Zhao, Z. Y., Luan, P., Huang, S. X., Xiao, S. H., Zhao, J., Zhang, B., et al. (2013). Edaravone protects HT22 neurons from H2O2-induced apoptosis by inhibiting the MAPK signaling pathway. CNS Neurosci. Ther. 19 (3), 163–169. doi:10.1111/cns.12044
Zhou, L., Chen, X., Yu, B., Pan, M., Fang, L., Li, J., et al. (2022). The effect of metformin on ameliorating neurological function deficits and tissue damage in rats following spinal cord injury: a systematic review and network meta-analysis. Front. Neurosci. 16, 946879. doi:10.3389/fnins.2022.946879
Zhou, L. Y., Tian, Z. R., Yao, M., Chen, X. Q., Song, Y. J., Ye, J., et al. (2019). Riluzole promotes neurological function recovery and inhibits damage extension in rats following spinal cord injury: a meta-analysis and systematic review. J. Neurochem. 150 (1), 6–27. doi:10.1111/jnc.14686
Keywords: edaravone, spinal cord injury, systematic review, neuroprotective effect, neurological function, action mechanism, administered dose
Citation: Zhou L-y, Wang X-b, Chen X-q, Li R, Yu B-b, Pan M-x, Fang L, Li J, Cui X-j, Yao M and Lu X (2025) Neuroprotective effect and possible mechanism of edaravone in rat models of spinal cord injury: a systematic review and network meta-analysis. Front. Pharmacol. 16:1538879. doi: 10.3389/fphar.2025.1538879
Received: 03 December 2024; Accepted: 06 March 2025;
Published: 07 April 2025.
Edited by:
Peter Lansbury, Harvard Medical School, United StatesReviewed by:
Yuan Zhou, Nanjing University, ChinaCopyright © 2025 Zhou, Wang, Chen, Li, Yu, Pan, Fang, Li, Cui, Yao and Lu. This is an open-access article distributed under the terms of the Creative Commons Attribution License (CC BY). The use, distribution or reproduction in other forums is permitted, provided the original author(s) and the copyright owner(s) are credited and that the original publication in this journal is cited, in accordance with accepted academic practice. No use, distribution or reproduction is permitted which does not comply with these terms.
*Correspondence: Xiao Lu, bHV4aWFvMTk3MkAxNjMuY29t; Min Yao, eWFvbWluMTk4NzEyMjNAMTI2LmNvbQ==
†These authors have contributed equally to this work
Disclaimer: All claims expressed in this article are solely those of the authors and do not necessarily represent those of their affiliated organizations, or those of the publisher, the editors and the reviewers. Any product that may be evaluated in this article or claim that may be made by its manufacturer is not guaranteed or endorsed by the publisher.
Research integrity at Frontiers
Learn more about the work of our research integrity team to safeguard the quality of each article we publish.