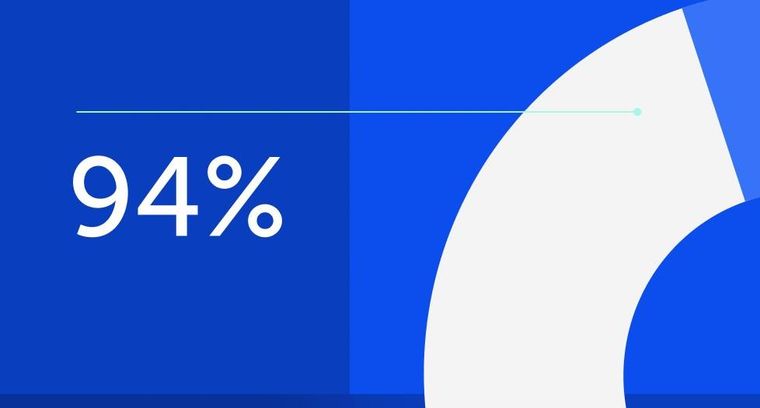
94% of researchers rate our articles as excellent or good
Learn more about the work of our research integrity team to safeguard the quality of each article we publish.
Find out more
REVIEW article
Front. Pharmacol., 19 March 2025
Sec. Ethnopharmacology
Volume 16 - 2025 | https://doi.org/10.3389/fphar.2025.1538311
This article is part of the Research TopicPrevention and Treatment of Infectious Diseases by Herbal MedicineView all 10 articles
Infectious diseases continue to be a major global public health concern, which is exacerbated by the increasing prevalence of antimicrobial resistance. This review investigates the potential of herbal medicine, particularly Chrysanthemum morifolium (CM) and Chrysanthemum indicum (CI), in addressing these challenges. Both herbs, documented in traditional Chinese medicine (TCM) and the Pharmacopoeia of the People’s Republic of China (2020 edition), are renowned for their heat-clearing and detoxifying properties. Phytochemical studies reveal that these botanicals contain diverse bioactive compounds, including flavonoids, terpenoids, and phenylpropanoids, which exhibit antimicrobial, anti-inflammatory, and antioxidant properties, among other effects. Comparative analysis reveals that distinct compound profiles and differential concentrations of core phytochemicals between CM and CI may lead to differentiated therapeutic advantages in anti-infective applications. By systematically examining their ethnopharmacological origins, phytochemical fingerprints, and pharmacological mechanisms, this review highlights their synergistic potential with conventional antimicrobial therapies through multi-target mechanisms, proposing novel integrative approaches for global health challenges.
Infectious diseases continue to pose a persistent and significant threat to global public health, contributing substantially to morbidity and mortality worldwide. The rise of antimicrobial resistance has further complicated the management of these diseases, highlighting the urgent need for alternative therapeutic strategies (Sangeetha Vijayan et al., 2024). Botanical drug, with its extensive historical and cultural heritage, presents a promising avenue for addressing both infectious diseases and the challenges posed by antimicrobial resistance.
Chrysanthemum morifolium (CM, known as “Juhua” in China) refers to the flower head of C. morifolium Ramat. Originating from China, CM has been utilized for over 3,000 years, predominantly as a dietary flower tea for health maintenance and as an integral component of traditional Chinese medicine (TCM) (Yuan et al., 2020). CM is listed in the Pharmacopoeia of the people’s Republic of China (ChP) as a significant botanical drug with properties that include dispersing wind-heat, clearing liver fire, brightening the eyes, and detoxifying (Commission CP, 2020). Chrysanthemum indicum (CI, known as “Yeju” in China) is the flower head of C. indicum Linné. According to the 2020 edition of ChP, CI is recognized for its properties in clearing heat, detoxifying, purging fire, and pacifying the live (Commission CP, 2020). The similar names and appearances of the two botanical drugs frequently cause confusion and misidentification. Although both CM and CI belong to the Compositae family, their plant morphology and phytochemical compositions differ significantly. In TCM, CI is considered more effective for heat-clearing and detoxifying, while CM exhibits a broader range of therapeutic functions. Nonetheless, both botanical drugs show potential in the treatment of infectious diseases.
The pharmacological potential of CM and CI in infectious disease management is underscored by their rich phytochemical composition. These plants contain a plethora of compounds, including flavonoids (Miyazawa and Hisama, 2003; Wang et al., 2010; Wu et al., 2015; Yang et al., 2019a), terpenoids (Commission CP, 2020; Liu et al., 2017), phenolic acids (Yang et al., 2019b; Gao et al., 2008), and others (Guo et al., 2010). Notably, these compounds demonstrate antibacterial (Park and Kang, 2021; Zhang et al., 2024; Wang et al., 2020), antiviral (Shen et al., 2018; Boudou et al., 2024), anti-inflammatory (Boudou et al., 2024; Hamad et al., 2023), antioxidant effects (Boudou et al., 2024; Han et al., 2018), among others (Chen et al., 2024; Yang et al., 2017; Xie et al., 2009; Sun et al., 2012). Due to the differences in the types of compounds and the concentrations of the main compounds between the two, they may have different characteristics and advantages in anti-infection treatment. However, there has been no comprehensive summary or comparison of the effects of CM and CI in the treatment of infectious diseases.
This article aims to explore the main compounds and potential mechanisms by which CM and CI play a role in the treatment of infectious diseases. By delving into the ethnopharmacological origins, major phytochemical compounds, and associated pharmacological activities of these botanicals, we aim to elucidate their potential in complementing conventional anti-infection therapies and addressing the challenges of antimicrobial resistance. Finally, the paper summarizes the current research status and discusses future prospects of CM and CI in the context of infectious diseases.
Both CM and CI belong to the Compositae family and are utilized both medicinally and for environmental applications. The dried flower heads of CM are commonly utilized as medicinal parts for treatment, while both the dried flower heads and whole plants of CI serve as primary medicinal components for treating various diseases. To better differentiate between CM and CI, we conducted a macroscopic identification, as detailed in Table 1.
CM (Juhua) has been utilized in China for over 3,000 years, with historical records dating back to the Qin and Han dynasties (Liang et al., 2014). It was introduced to Japan during the Tang Dynasty (AD 710–784) as a highly regarded spice and subsequently spread Europe and the United States in the 17th century (Li, 1993). The genus CM comprises 41 species, which are widely distributed across Asia, including Mongolia, Russia, China, Japan, and Korea, as well as eastern Europe (Chen et al., 2020; Committee FoCE, 1983). In China, excluding Tibet and the Northwest regions, approximately 21 species are predominantly found in humid areas at middle and low altitudes (Chen et al., 2020). The botanical morphological characteristics of CM are described as follows: 1) Life form: Perennial herb. 2) Stem: Erect, either branched or unbranched, covered with pubescent. 3) Leaves: Ovate to lanceolate in shape, measuring 5–15 cm in length, pinnately lobed or semi-lobed, short-petioled, and white pubescent on the underside. 4) Flowers: The capitulum ranges from 2.5 to 20 cm in diameter. The involucrum consists of multiple layers, with the outer layer being pilose. The ray florets exhibit a variety of colors, whereas the disk florets are yellow. 5) Fruits: Achenes measure approximately 1.3 mm in length, slightly pointed at the apex, flat-wedge shaped, longitudinally ribbed on the surface, and brown in color (Committee FoCE, 1983).
The application of CI (Yeju) in TCM can be traced back to the Qin and Han dynasties (Chen Caiying et al., 2015). The native range of CI includes China, the Eastern Himalayas, Inner Mongolia, Japan, South Korea, Nepal, etc. Additionally, it has a broad distribution across various Asian countries, as well as in several European and South American nations (Committee FoCE, 2011). This plant thrives in wide range of habitats, from grasslands on mountain slopes and thickets to wet areas near rivers, fields, roadsides, saline zones by seashores, and under scrub, typically at altitudes ranging from 100 to 2,900 m (Committee FoCE, 2011). The botanical morphological characteristics of CI are as follows: 1) Life form: Perennial herb. 2) Stem: Long or short procumbent rhizomes, with erect or diffuse stems that branch and are sparsely pilose; lower leaves senesce by anthesis. 3) Leaves: Middle stem leaves have petioles measuring 1–2 cm, with leaf blades ovate to long-ovate or elliptic-ovate, ranging from 3–7 (-10) × 2–4 (-7) cm. Both surfaces are pale green or olive-green, with the adaxial surface being sparsely pubescent and the abaxial surface less densely. The leaves are pinnatifid, pinnatilobed, or inconspicuously divided, with a truncate, somewhat cordate, or broadly cuneate base. 4) Flowers: The synflorescence is a lax terminal flat-topped cyme, bearing numerous or few capitula. Phyllaries are arranged in 5 rows, featuring broad scarious margins that are white or brown, with obtuse or rounded apices. Outer phyllaries are ovate or ovate-triangular, measuring 2.5–3 mm, while middle phyllaries are ovate at 6–8 mm, and inner phyllaries are narrowly elliptic, approximately 1.1 cm. Ray floret laminae are yellow, measuring 1–1.3 cm, with entire or 3-denticulate apices. 5) Fruits: Achenes measure 1.5–1.8 mm (Committee FoCE, 2011). (Figure 1).
Figure 1. Chrysanthemum morifolium Ramat. and Chrysanthemum indicum Linné. (A) Chrysanthemum morifolium (CM) is the flower head of Chrysanthemum morifolium Ramat. (B) Chrysanthemum indicum (CI) is the flower head of Chrysanthemum indicum Linné. Two images are from Guangmin Li and Xinxin Zhu, respectively. Both images are from Plant Data Center of Chinese Academy of Sciences (https://www.plantplus.cn).
CM has a long history as a TCM. The earliest record of CM can be traced back to the Shennong’s Herbal Classic, which described its taste as bitter and neutral, and indicated its primary use for dizziness and pain caused by wind, and other conditions. The Mingyi Bielu noted CM tastes sweet and is non-toxic. According to The Compendium of Materia Medica, Juhua is widely believed to dispel wind-heat, benefit the liver, and tonify Yin”. A Qing Dynasty doctor, Xu Dachun, recorded that prolonged consumption of Juhua can tonify blood and Qi, enhance physical wellbeing, and promote longevity. Since Juhua can survive for a long time, there is a belief that consuming it can extend human life. ChP recorded that CM disperses wind and clears heat, pacifies the liver and brightens the eyes, and detoxifies. Clinically, it is used to treat wind-heat colds, headaches and dizziness, red and swollen eyes, diminished eyesight, sores, and abscess swelling (Commission CP, 2020). CM is extensively utilized in TCM, particularly as a primary ingredient in TCM prescription formulations such as Sangju Ganmao Wan, Huanglian Shangqing Wan, and Jinsang Kaiyin Wan. The 2020 edition of the ChP lists 1605 TCM formula and single-botanical drug preparations, among which 55 containing CM, accounting for approximately 3.4% of the total (Commission CP, 2020).
There are limited records of CI in ancient medical literature. The earliest record of CI can be traced back to the Sheng Nong’s herbal classic and it was recorded as “Jiehua” (Chen Caiying et al., 2015). In the Bencao Qiuzhen, CI is described as having the properties of “entering the lung and liver meridians”. The Bencao Huiyan noted: “CI has a bitter taste, is cold in nature, and slightly toxic. It functions to break up blood stasis, soothe the liver, and detoxify whitlow. Additionally, it can be used as a douche for scabies to dispel wind and kill parasites”. The 2020 edition of ChP recorded that CI has the functions of clearing heat, detoxifying, purging fire, and pacifying the liver, and clinically, it is used to treat conditions such as whitlow, sores, abscesses, and swelling, as well as symptoms like redness of the eyes, headache, and dizziness (Commission CP, 2020). The 2020 edition of ChP includes 16 TCM formula preparations containing CI (Commission CP, 2020). Table 2 provides details on the utilization of CM and CI as monarch medications.
We conducted a comprehensive literature search in PubMed from 2000 to 2024 using the terms “Chrysanthemum morifolium”, “Chrysanthemum morifolium Ramat.”, “Chrysanthemum indicum”, “Chrysanthemum indicum Linné.”, as well as related keywords such as “Juhua”, “Yeju”, and “Yejuhua”. This search yielded a total of 785 articles (CM: n = 582, CI: n = 203.). After removing duplicates (n = 47) and review articles (n = 23), we carefully screened the titles and abstracts of the remaining articles and excluded those (n = 469) not relevant to the pharmacological effects of CM and CI. Ultimately, we included twenty-nine studies that explicitly examined the antimicrobial (n = 10), anti-inflammatory (n = 8), antioxidant (n = 7), and immunomodulatory (n = 4) effects of CM and CI, with a focus on their potential applications in infectious diseases.
With the exploration of the potential applications of CM and CI in food, health products, and cosmetics, research into their chemical components has significantly expanded. They contain a rich array of compounds, including flavonoids, phenylpropanoids, terpenoids, triterpenoids, and others, which may endow them with anti-infective pharmacological effects such as antimicrobial, anti-inflammatory, and antioxidant properties. Our review of studies investigating the potential roles of CM and CI in combating infectious diseases revealed that they possess distinct advantages, which can be attributed to their different content and composition of specific compounds, particularly terpenoids and flavonoids. Consequently, we have summarized the major compounds analyzed in the included studies of CM and CI in Table 3 and illustrated these compounds in Figure 2.
Table 3. The primary compounds in CM and CI that are likely to exert anti-infection-related pharmacological effects.
Figure 2. The chemical structures of the main compounds that may exert anti-infection-related pharmacological effects in CM and CI.
CM and CI extracts exhibit potent antimicrobial effects, demonstrating potential to inhibit a wide range of microorganisms including bacteria, fungi, viruses, mycobacteria, and parasite and others (Kuang et al., 2018; Liu et al., 2022; Youssef et al., 2020; Shunying et al., 2005; Gu et al., 2013; Zhang et al., 2020; Akihisa et al., 2005; Lee et al., 2003). The differences in the types of inhibited microorganisms between CM and CI may be attributed to variations in their primary compounds, such as terpenoids and flavonoids.
Terpenoids have been reported to exhibit significant antibacterial activity by destroying the integrity of bacterial cell membranes or impairing essential bacteria functions (Yamaguchi, 2022; Meenu et al., 2023; Zacchino et al., 2017). Essential oils from CM and CI are rich in terpenoids (Liu et al., 2017; Peng et al., 2020; Shao et al., 2020; Wang Xl et al., 2006; Xue et al., 2018), which suggest that they possess potent antibacterial properties. For instance, the study conducted by Kuang et al. (Kuang et al., 2018) demonstrated that CM essential oil exhibits significant inhibitory effects against five bacterial strains: Pseudomonas aeruginosa (P. aeruginosa), Salmonella enteritids (S. enteritidis), B. subtilis (Bacillus subtilis), Staphylococcus aureus (S. aureus), and Escherichia coli (E. coli). The minimum inhibitory concentrations (MICs) were determined as follows: 0.33% for P. aeruginosa, 0.67% for both S. enteritidis and B. subtilis, and 1.30% for both E. coli and S. aureus. The main compounds isolated from CM essential oil are monoterpenes and sesquiterpenes, including α-curcumene (No.1), α-farnesene (No.2), β-bisabolene (No.3), bisabolol (No.4), n-heptadecane (No.5), nonadecane (No.6) and n-pentacosane (No.7) (Table 3). Notably, α-curcumene (No.1) is the most abundant compound among these compounds, accounting for 12.55% of the total composition (Kuang et al., 2018). These compounds are potentially associated with the antibacterial properties of CM essential oils. Furthermore, in a separate study, the content of α-curcumene (No.1) extracted from CI via hydro-distillation (1.23%) was significantly lower at 1.23% compared to 10.50% obtained from CM (Youssef et al., 2020). This suggests that CI may exhibit a weaker inhibitory effect on the aforementioned five bacteria.
Additionally, camphor (No.8), an oxygenated monoterpene, is the predominant compound in both CM (14.56%) and CI (36.69%) oils (Youssef et al., 2020). Notably, the essential oil of CI demonstrated superior antimicrobial activity against Gram-positive bacteria, including B. subtilis, Streptococcus agalactiae, and Streptococcus pyogenes, compared to the CM essential oil (Youssef et al., 2020). This enhanced activity may be attributed to the higher concentrations of camphor (No.8) and isoborneol (No.9) (7.64%) in CI (Youssef et al., 2020), which have been associated with antibacterial and antiviral properties (Chen et al., 2013; Astani et al., 2011; Costa et al., 2021). Therefore, in this study, essential oils rich in terpenoids demonstrated greater efficacy against Gram-positive bacteria than compared to Gram-negative bacteria (Youssef et al., 2020; Shunying et al., 2005). Moreover, the processed and air-dried flower oils of CI were evaluated against 15 microorganisms (Shunying et al., 2005). The processed flower oil exhibited the strongest antimicrobial activity against Staphylococcus saprophyticus (S. saprophyticus) (MIC: 0.78 mg/mL, minimum bactericidal concentration (MBC): 0.78 mg/mL), and the air-dried flower oil showed the most effective bactericidal activity against E. coli (MIC: 0.39 mg/mL, MBC: 0.39 mg/mL). The two oils possessed good inhibitory effects on S. saprophyticus (MIC: 1.56 mg/mL, MBC: 1.56 mg/mL) (Shunying et al., 2005). Furthermore, the air-dried essential oil showed superior bactericidal activity against E. coli (102 strains) at concentrations ranging from 3 to 56 μg/mL, likely due to its higher content of α-terpineol (No.12) at 3.32%, compared to 2.94% in the processed flower essential oil (Shunying et al., 2005). CM also contains α-terpineol (No.12), but at a lower concentration of only 0.65% (Youssef et al., 2020).
Furthermore, essential oils derived from different nonmedicinal parts of CM exhibit varying degrees of antibacterial activity. Specifically, Juhua demonstrates the most potent antibacterial effect against S. aureus (MIC: 10 mg/mL) compared to the stem and leaf extracts as well as root extracts of CM (Liu et al., 2022). The antibacterial efficacy of Juhua and stem and leaf extracts against Propionibacterium acnes (P. acnes) is comparable (MIC: 25 mg/mL), both of which are significantly higher than that of the root extract (Liu et al., 2022). This may be related to the high content of terpenoids in Juhua oil compared with the other two parts of essential oils. In this study, Juhua essential oil exhibited the highest terpenoid content among processed flowers at different stages (Jumi, Tianju, Juhua) and different parts of CM (Juhua, Stem and Leaf, Root), which likely contributes to its superior antibacterial efficacy. The composition of Juhua essential oil was predominantly oxygenated sesquiterpenes, with α-cadinol being the major constituent (28.62%, No.19). Notably, the content of heterospiroolefins, such as (E)-tibetin spiroether, decreased significantly from 44.81% in Jumi essential oil to 7.27% in Juhua essential oil. Despite this reduction, heterospiroolefins appear to have minimal impact on the antibacterial activity of CM (Liu et al., 2022). It is worth noting that heterospiroolefins were first isolated from CM, yet their specific antibacterial effects remain unclear.
Triterpenoids and essential oils extracted from CM and CI showed significant anti-mycobacterial activity against Mycobacterium tuberculosis (M. tuberculosis) (Youssef et al., 2020; Akihisa et al., 2005). For instance, Akihisa et al. (Akihisa et al., 2005) utilized the microplate Alamar Blue Assay (MABA) to identify twenty-nine 3-hydroxytriterpenoids from unsaponified lipid extracts of CM. Among these compounds, fourteen exhibited MIC values ranging from 4 to 64 μg/mL against M. tuberculosis (as detailed in Tables 3, 4). 3-Epilupeol (MIC: 4 μg/mL, No.48), derived from lupeol (No.38), and maniladiol (MIC: 4 μg/mL, No.44) showed the most potent anti-mycobacterial activity among the tested compounds (Akihisa et al., 2005). Notably, the half maximal inhibitory concentration (IC50) value of three-epilupeol exceeded 62.5 μg/mL, suggesting a selective toxicity towards M. tuberculosis (Akihisa et al., 2005). Although the antitubercular effect of CM and the extract was much lower compared to first-line antitubercular drugs, such as rifampin and isoniazid, these compounds still exhibited substantial activity against M. tuberculosis (Youssef et al., 2020; Akihisa et al., 2005).
A study evaluating the antifungal activities of extracts from 12 cultivars of CM roots against Magnaporthe oryzae, Verticillium dahliae, and Fusarium oxysporum revealed that only “Xiao Huang Ju” exhibited significant inhibitory effects on all tested fungi (Zhang et al., 2020). Table 3 summarizes the key compounds identified in the 12 cultivars through principal component analysis. However, a separate study reported that both CM and CI oils demonstrated weak antifungal activity against Aspergillus fumigatus, Candida albicans, Geotrichum candidum, and Syncephalastrum racemosum, with MIC values exceeding 500 μg/mL (Youssef et al., 2020).
Both CM and CI possess antiviral properties (Youssef et al., 2020; Gu et al., 2013; Kang et al., 2013; Lee et al., 2003; Kim et al., 2012). For instance, the flavonoid compound apigenin 7-O-β-D-(4″-caffeoyl)glucuronide (No.54), isolated from CM, exhibited potent HIV-1 integrase inhibitory activity and anti-HIV effects in HIV-1IIIB-infected MT-4 cells (Lee et al., 2003). Moreover, a unique sesquiterpenoid trimer (Chrysanolide A, No.16), along with its biogenetically related monomer (Chrysanolide B, No.17) and dimer (Chrysanolide C, No.18), isolated from CI, exhibited strong inhibitory activity against the secretion of HBsAg and HBeAg (Gu et al., 2013). Additionally, both CM and CI essential oils showed antiviral activity against vesicular stomatitis virus (VSV), hepatitis A (HAV) and herpes simplex type-1 (HSV-1) (Youssef et al., 2020). Notably, the antiviral activity of CI was dose-dependent, with VSV being the most sensitive to CI’s antiviral effects (Youssef et al., 2020).
Epstein-Barr virus (EBV) latent infection membrane protein 1 (LMP1) plays a critical role in EBV-mediated B lymphocyte transformation, with LMP1-induced nuclear factor kappa-B (NF-κB) activation being crucial for the survival of lymphoblastoid cell lines (LCLs). CI exhibited a potent inhibitory effect on EBV LMP1-induced NF-κB activation and significantly reduced the viability of EBV-transformed LCLs in a dose- and time-dependent manner (Kim et al., 2012). This inhibition likely involves blocking LMP1-induced IKKα and IKKβ activation, without affecting the viability of human foreskin fibroblasts, EBV-negative Burkitt lymphoma cells, or HeLa cells (Kim et al., 2012). Furthermore, both the CH2Cl2 fraction of CI and lupeol (No.38) significantly attenuated LMP1-induced NF-κB activation and reduced the viability of LCLs. Notably, the CH2Cl2 fraction of CI exhibited superior antiviral properties compared to lupeol. Additionally, lupeol may synergistically interact with unidentified compounds in the CH2Cl2 fraction of CI to further diminish LMP1-induced NF-κB activation and LCL viability (Kang et al., 2013).
CM and CI have also exhibited potential antimicrobial effects against various microorganisms, including Helicobacter pylori and trypanosomes (Youssef et al., 2020). For example, the essential oils of CM and CI showed an anti-H. pylori activity with IC50 values of 3.63 μg/mL and 3.78 μg/mL, respectively, which are comparable to those of clarithromycin (Youssef et al., 2020).
The antimicrobial activity of CM and CI is strongly correlated with their terpenoid and triterpenoid content. Terpenes enhance lipophilicity and membrane permeability, leading to significant disruption of oxidative phosphorylation and electron transport chains, thereby severely impairing energy production and inducing autooxidation and peroxidation, ultimately resulting in bacterial lysis (Gamal El-Din et al., 2018). The potency of their antimicrobial effects is also influenced by the concentration of key compounds such as α-curcumene (No.1), camphor (No.8), and α-terpineol (No.12). Studies have demonstrated that while CI and CM exhibit weak antifungal activity, CM shows superior antifungal efficacy when different parts of the extract are used. Furthermore, CI demonstrates a stronger inhibitory effect on specific viruses. Additionally, some studies report antimicrobial activity using only IC50 or MIC values, but not both, which may introduce bias in their results. More details are provided in Table 4. Although the antimicrobial activity of CM and CI has been extensively studied, the detailed mechanism of their antimicrobial action has not been reported.
When an infection occurs, the immune system initiates an inflammatory response to combat invading pathogens (Zhang and Wang, 2014). However, excessive or prolonged inflammation can cause tissue damage and exacerbate disease severity. In severe cases, uncontrolled inflammation may lead to sepsis, widespread tissue injury, and organ failure (Esposito et al., 2017). Reducing inflammation can mitigate pathogen-induced tissue damage and bodily discomfort. Studies have shown that both CM and CI extracts exhibit significant anti-inflammatory effects by modulating inflammation-related pathways (Liu et al., 2020; Lee et al., 2021; Zhou et al., 2023; Yu et al., 2019; Zeng et al., 2020; Wu et al., 2014; Lee et al., 2009; Cheon et al., 2009).
CM and CI extracts demonstrated potential efficacy in treating acute lung injury (ALI). In a mouse model of lipopolysaccharide (LPS)-induced, both extracts significantly alleviated lung histopathological damage, reduced the wet-to-dry lung weight ratio and lung injury score, and were associated with decreased levels of pro-inflammatory cytokines, including IL-6 and tumor necrosis factor (TNF)-α (Liu et al., 2020; Wu et al., 2014). CM extract can attenuate the production of pro-inflammatory cytokines (such as IL-6 and TNF-α), enhance the secretion of anti-inflammatory cytokines (including transforming growth factor-β1 (TGF-β1) and IL-10), and mitigate the oxidative stress by increasing total antioxidant capacity (TAC) and reducing malondialdehyde (MDA) levels in mice with ALI. However, the precise mechanisms underlying these effects remain to be elucidated (Liu et al., 2020). The therapeutic efficacy of CI may be attributed to its ability to downregulate both the Toll-like receptor 4 (TLR4) and MyD88-dependent NF-κB signaling pathways (Wu et al., 2014).
The other TLR4 signaling pathway is MyD88-independent and TRIF-dependent, leading to the phosphorylation and nuclear translocation of IRF3. A newly discovered compound, bisepoxylignan dendranlignan A (BDA), isolated from CM, has been shown to reduce the production of inflammatory cytokines TNF-α, IL-2, and interferon (IFN)-γ in LPS-stimulated H9c2 cells (Zeng et al., 2020). BDA significantly decreased the nuclear translocation and phosphorylation levels of IRF3, the NF-κB heterodimer component p65, and one of the AP-1 components, c-Jun. However, it did not significantly affect the protein expression of TLR4, MyD88, or TRIF, suggesting that BDA inhibits TLR4 signaling downstream of these proteins (Zeng et al., 2020). Additionally, molecular docking studies revealed that BDA can occupy the ligand-binding site of the TLR4-MD2 complex, indicating that it may inhibit inflammation by blocking the TLR4 signaling pathway (Zeng et al., 2020).
Hyaluronidase (HAase) is an endoglycosidase important for the metabolism of hyaluronic acid (HA), a linear acidic mucopolysaccharide. HAase plays a significant role in inflammation by enhancing the production of cytokines IL-1β and TNF-α by macrophages, as well as their allostimulatory capacity (Sudha and Rose, 2014; Jiang et al., 2011; Termeer et al., 2000). Studies have found that inhibiting HAase can prevent HA degradation, thereby mitigating inflammatory responses (Shibata et al., 2002). For example, in an in vitro inflammatory model, Zhou et al. (2023) reported that CM extract dose-dependently inhibited HAase activity. Further screening identified four compounds 56, 61, 62 and 63 as the key inhibitors, and these compounds significantly reduced the production of inflammatory mediators nitric oxide (NO) and IL-6, and suppressed the mRNA expression of inducible NO synthase (iNOS) and IL-1β in both mouse and human macrophages.
In addition, the anti-inflammatory mechanism of CI may be associated with the regulation of apoptosis-associated speck-like protein (ASC) phosphorylation and the MAPKs and NF-κB-dependent signaling pathways (Yu et al., 2019; Cheon et al., 2009). For instance, in vivo studies have demonstrated that CI can inhibit the recruitment of total cells and Ly6G+/F4/80- neutrophils as well as reduce the secretion of inflammatory cytokines in peritonitis mice. In vitro, CI has been shown to inhibit the activation of the nucleotide-binding oligomerization domain (NOD)-like receptor (NLR)3 and the HIN-200 family member absent in melanoma 2 (AIM2) inflammasomes, leading to decreased production of IL-1β and caspase-1 (Yu et al., 2019). The phosphorylation of ASC regulates the activity of inflammasomes such as NLR3 and AIM2 inflammasomes through the formation of ASC specks (Hara et al., 2013). In studies, treatment with CI resulted in reduced translocation formation and inhibited phosphorylation, while JNK phosphorylation was not implicated in this pathway (Yu et al., 2019). Given that JNK phosphorylation is recognized as an upstream regulator of ASC phosphorylation (Hara et al., 2013), the observed effects may be associated with the modulation of ASC phosphorylation. However, the specific pathway through which this occurs remains to be elucidated.
LPS can activate a signaling pathway involving mitogen-activated protein kinases (MAPKs), including ERK1/2, JNK1/2 and p38MAPK (Moens et al., 2013). MAPKs inhibitors have been shown to suppress the regulation of iNOS and cyclooxygenase (COX)-2 genes (Kim and Kim, 2005). Overexpression of iNOS and COX-2 leads to NF-κB activation, resulting in increased production of nitric oxide (NO) and prostaglandin E2 (PGE2), which exacerbates inflammatory responses (D'Acquisto et al., 1997). CI extract significantly inhibited the LPS-induced production of inflammatory mediators NO and PGE2, as well as inflammatory cytokines TNF-α and IL-1β, in RAW 264.7 macrophages in a dose-dependent manner (Cheon et al., 2009). Additionally, CI extract suppressed the mRNA and protein expression of iNOS and COX-2. Further studies revealed that CI can inhibit the nuclear translocation of NF-κB p65 subunits by preventing IκBα phosphorylation and also inhibit the phosphorylation of ERK and JNK, suggesting that the anti-inflammatory effects of CI are mediated through both MAPK and NF-κB pathways (Cheon et al., 2009). More details are provided in Table 5.
Treating oxidative imbalance is crucial in the management of infectious diseases (Ashique et al., 2025). Reactive oxygen species (ROS), which are by-products of cellular metabolism, exhibit a dual nature: they are beneficial to cells at low concentrations but become detrimental at high levels (Sander et al., 2022). For instance, ROS contribute to pathogen destruction; however, their excessive accumulation can induce damage to cellular components such as lipids, proteins, and DNA (Sander et al., 2022). Moreover, overproduction of ROS leads to oxidative stress, resulting in bodily damage, various disease states, impaired immune function, and exacerbated inflammatory responses. This creates a vicious cycle that hinders recovery (Ashique et al., 2025). In contrast, inflammation can result in the recruitment of intravascular neutrophils to the alveolar space and lung parenchyma (Looney et al., 2006), leading to the subsequent release of proteases and generation of ROS. Moreover, ROS are closely associated with lipid peroxidation products such as myeloperoxidase (MPO) and MDA, as well as the modulation of antioxidant enzyme activities, including SOD, CAT, and GPX (Looney et al., 2006). Antioxidants can neutralize ROS, thereby protecting cells from oxidative damage and enhancing the immune system’s ability to combat infections effectively. Essential oils derived from CM and CI exhibit potential as natural preservatives due to their antioxidant properties (Lii et al., 2010; Zheng et al., 2015; Zhan et al., 2022; Hao et al., 2021; Lin et al., 2010; Tian et al., 2019; Zhang et al., 2019; Kim et al., 2017).
CM extract and its flavonoids, apigenin and luteolin, exhibit potent antioxidant properties by resisting oxidative stress (Lii et al., 2010; Zhan et al., 2022; Hao et al., 2021). They enhance the levels of antioxidant enzymes such as SOD, CAT, and GPX, upregulate the expression of the antioxidant gene heme oxygenase-1 (HO-1), and decrease the production of reactive oxygen species (ROS), MDA, MPO, and 2,2-diphenyl-1-picrylhydrazyl (DPPH) radicals (Lii et al., 2010; Zheng et al., 2015; Zhan et al., 2022; Hao et al., 2021; Lin et al., 2010; Tian et al., 2019). Moreover, CM can inhibit cell apoptosis, modulate cell cycle progression, and reduce the expression of pro-apoptotic proteins including Bax, cleaved caspase-3, and cleaved poly (ADP-ribose) polymerase (PARP) (Zheng et al., 2015; Hao et al., 2021; Tian et al., 2019). Further studies suggest that these effects may be mediated through several signaling pathways, such as arginine and purine metabolism, phosphatidylinositol 3-kinase (PI3K)/Akt, PI3K/Akt-mediated nuclear factor erythroid 2-related factor 2 (Nrf2)/HO-1, and Nrf2 signaling pathways (Lii et al., 2010; Zhan et al., 2022; Hao et al., 2021; Tian et al., 2019).
CI also exhibits comparable antioxidant effects to CM, such as significantly enhancing the activities of antioxidant enzymes (Zhang et al., 2019; Kim et al., 2017). In addition, CI could not only inhibit the increased Bax/Bcl-2 ratio and activation of cleaved caspase-3 in the liver and brain but also reduce the levels of inflammatory cytokines, including IL-1β, IL-6, and TNF-α (Zhang et al., 2019). Furthermore, studies have found that both CM and CI exhibit peak antioxidant capacity when heated at 100°C for 45 min (Yu et al., 2023). Detailed information is provided in Table 6. Moreover, the anti-inflammatory effects of CM and CI are discussed in Section 3.2, while their antioxidant properties are summarized in Table 5 (Liu et al., 2020; Lee et al., 2021; Wu et al., 2014; Lee et al., 2009).
The immune system responds to exogenous factors encountered by the body and employs defense mechanisms to counteract these challenges. For instance, LPS, a major component of the outer membrane of Gram-negative bacteria, can bind to TLR4 and activate the MyD88-mediated NF-κB signaling pathway, ultimately leading to inflammatory responses (Hamad et al., 2023). Consequently, LPS is frequently utilized to induce inflammatory models in experimental settings. Moreover, abnormal immune responses are prevalent in infectious diseases such as influenza and COVID-19 (Han et al., 2018; Chen et al., 2024). Furthermore, with the increasing prevalence of antibiotic resistance, immunomodulatory agents have emerged as promising alternatives for treating infectious diseases (Yang et al., 2017). Studies have shown that CM polysaccharides and the butanol-soluble fraction of CI exhibit immunomodulatory effects and hold potential as therapeutic agents for infectious diseases (Xie et al., 2009; Sun et al., 2012; Liang et al., 2014; Li, 1993).
Inflammatory bowel disease (IBD) is an autoimmune disorder characterized by dysregulation of multiple immune-related pathways and cells, including the NF-κB signaling pathway, helper T (Th) cells, and regulatory T (Treg) cells (Chen et al., 2020; Committee FoCE, 1983; Chen Caiying et al., 2015; Committee FoCE, 2011). Numerous studies have demonstrated that inflammatory cytokine genes involved in IBD pathogenesis, such as IL-1β, IL-2, TNF-α, and IL-6, contain NF-κB binding sites and are transcriptionally regulated by NF-κB (Chen et al., 2020; Committee FoCE, 1983). Recent research has shown that CM polysaccharides can mitigate intestinal pathological changes in colitis rats, reduce levels of inflammatory cytokines (e.g., TNF-α, IL-6, and IL-1β), and alleviate oxidative stress responses (e.g., SOD and MPO). Furthermore, these effects are associated with decreased mRNA expression levels of TLR4, Janus kinase (JAK)2, and signal transducer and activator of transcription (STAT)3, as well as reduced protein levels of p65, TLR4, p-STAT3, and p-JAK2. Additionally, improvements were observed in the metabolic profiles of plasma and urine (Xie et al., 2009). All these findings indicate that the NF-κB/TLR4 and IL-6/JAK2/STAT3 signaling pathways play a role in the mechanism by which CM exerts its effects on IBD. Further studies have demonstrated that CM polysaccharides can reduce the production of pro-inflammatory cytokines and promote the production of anti-inflammatory cytokines by regulating the imbalance between Th1/Th2 and Th17/Treg (Sun et al., 2012). Additionally, improvements in gut microbiota have also been observed (Sun et al., 2012). However, while the effects of CM polysaccharides on IBD have been extensively studied, the depth of investigation is limited. Consequently, the specific mechanisms remain unclear, particularly regarding the rigorous validation of the signaling pathways involved.
Moreover, the immunomodulatory abilities of polysaccharides from different varieties of CM vary. Wang et al. (2022) investigated the immunomodulatory effects of polysaccharides from five cultivars: Qiju, Gongju, Boju, Hangbaiju, and Huaiju. The study found that all these polysaccharides could enhance the phagocytosis and proliferation of RAW264.7 cells without significant cytotoxicity, and upregulate the release of TNF-α, IFN-γ, and NO. Notably, polysaccharides from Boju and Hangbaiju exhibited superior immune-enhancing activities, making them more suitable for developing functional foods aimed at boosting immunity. These findings provide a reference for selecting appropriate varieties based on specific immune requirements, which are related to their relative molecular mass, glucuronic acid and arabinose content, and microstructure (Wang et al., 2022). Additionally, the potential role of these polysaccharides as vaccine adjuvants is currently under investigation (Han et al., 2021).
Additionally, the butanol soluble fraction of CI exhibited a significant inhibitory effect on dimethylbenzene-induced ear edema in mice and markedly enhanced the 2, 4-dinitro-fluorobenzene (DNFB)-induced delayed-type hypersensitivity (DTH) response (Cheng et al., 2005). Furthermore, CI was found to elevate the levels of sheep red blood cell (SRBC) antibodies, serum IgG, and IgM, while significantly enhancing the phagocytic function of monocytes in cyclophosphamide (CP)-induced immunosuppressed mice (Cheng et al., 2005). These findings indicate that CI possesses anti-inflammatory properties, as well as humoral and cellular immunomodulatory activities, including enhancement of mononuclear phagocytic function. The presence of flavonoids (53%) may contribute to these effects (Cheng et al., 2005).
Although the diseases examined in the aforementioned studies are not infectious in nature, the findings indicate that CM and CI possess certain immunomodulatory effects, which hold significant reference value for the subsequent management of infectious diseases. Further details are provided in Table 7.
In the acute toxicity study, a single oral dose of 15 g/kg body weight (bw) CM extract was administered to rats, then the rats were observed for 14 days. No treatment-related death was observed, and the maximal tolerance dose estimated was greater than 15 g/kg bw in rats. For long-term toxicity studies, rats were given daily intragastric doses of 320, 640, and 1,280 mg/kg bw/day for 26 weeks, followed by a 4-week recovery period (Li et al., 2010). The results showed that there were no toxicological changes in body weight, food intake, water intake, blood biochemistry, organ weight and histopathological examination in each treatment group. Thus, CM extract is generally safe for rats at limited dose levels. Dosage of annotation in the ChP for 5–10 g (Commission CP, 2020).
Different doses of CI extract prepared in saline were given orally to groups of 10 mice. For 30 days subsequent to treatment, the animals were observed daily, and dead animals were subjected to postmortem examination for determination of the cause of death. No animals died during the acute toxicity test, nor were any adverse effects detected in animals treated with different doses of CI extract. This indicates that CI extract was nearly nontoxic in mice up to an oral dose of 2.0 g/kg body weight (Lee et al., 2009). No studies have investigated the long-term toxicity of CI. Dosage of annotation in the ChP for 9–15 g (Commission CP, 2020). In addition to oral administration, it can also be used for external use, decoction for washing or paste for external application.
CM and CI, two major traditional Chinese herbs recognized in the theory of food and medicine homology, have a long history of medicinal use and demonstrated significant clinical efficacy. By systematically reviewing literature from the perspectives of ethnopharmacology, phytochemistry, and pharmacology, this study aims to provide an updated understanding of the anti-infective effects of CM and CI, thereby facilitating a more comprehensive grasp of their potential clinical applications and current research status in the context of infectious diseases.
A 2020 review reported that over 176 compounds, including 60 flavonoids, 28 phenylpropanoids, 68 triterpenoids, three steroids, and 17 others, were isolated and identified in CM. Notably, the “other” category included a small number of terpenoids (only 20 compounds) (Yuan et al., 2020). Subsequently, Peng et al. (Peng et al., 2020) detected a significant number of terpenoids, primarily monoterpenes, from CM, thereby supplementing the earlier review. Overall, a total of 109 terpenoids, including monoterpenoids, sesquiterpenoids, and unidentified diterpenes, have been identified in CM. Additionally, it has been reported that 191 natural compounds were isolated and identified from CI, comprising 42 flavonoids, 96 terpenoids, 21 phenylpropanoids and phenolic acids, 12 spiro ketones, and 20 other compounds (Shao et al., 2020). In this study, we summarize the main compounds reported in studies on the anti-infection-related antimicrobial, anti-inflammatory, antioxidant, and immunomodulatory effects of CM or CI, including 53 alkenes and terpenoids, 11 flavonoids, 7 phenylpropanoids, and other compounds. These compounds play a crucial role in inhibiting microbial growth, reducing inflammation, combating oxidative stress, and modulating immune responses.
Although CM has been reported to contain a higher number of compounds compared to CI, CI has shown superior antimicrobial effects in certain studies (Youssef et al., 2020). This may be attributed to the high concentration of key compounds in CI. For instance, camphor (No.8), a bicyclic monoterpene, exhibits a wide range of biological activities, including insecticidal, analgesic, antimicrobial, antiviral, anticoccidial, antinociceptive, anticancer, and antitussive properties (Sokolova et al., 2015; Zielińska-Błajet and Feder-Kubis, 2020). Studies have shown that symmetrical compounds containing camphor fragments, two imino groups, and/or a charged quaternary nitrogen atom exhibit potent antiviral activity (Sokolova et al., 2014; Sokolova et al., 2013). Additionally, research evaluating the antiviral activity of camphor-based imine derivatives against the H1N1 influenza virus pdm09 and their inhibitory effects on H5N1 pseudovirus infection revealed that the critical structural units responsible for antiviral activity include the natural camphor skeleton, the presence of an imino group, and an alcohol moiety (Sokolova et al., 2015).
A study demonstrated that CI and CM exhibited limited antifungal efficacy, whereas another study indicated that CM displayed superior antifungal properties (Youssef et al., 2020; Zhang et al., 2020). The discrepancy may be attributed to the different plant parts used for CM extraction; the former study utilized flowers, while the latter employed roots. Specifically, the latter study analyzed 12 cultivars of CM roots, revealing that the primary compounds in root extracts differ from those in flower extracts, which likely explains the varying antifungal effects observed. Additionally, differences in extraction methods and fungal strains examined between the studies may have contributed to these discrepancies. Regarding antiviral activity, CI showed a stronger inhibitory effect on specific viruses such as VSV, HAV, and HSV-1 (Youssef et al., 2020; Gu et al., 2013). However, some studies reported antimicrobial activity using only IC50 values without MIC or vice versa, potentially introducing bias into their results.
Furthermore, both CM and CI extracts exhibit anti-inflammatory properties. CM can regulate inflammation by modulating the AMPK-SIRT1 and TLR4 signaling pathways (Lee et al., 2021; Zeng et al., 2020). CI modulates inflammatory pathways, including the TLR4/MyD88-dependent NF-κB signaling pathway, and MAPKs and NF-κB-dependent pathway, as well as ASC phosphorylation independent of JNK phosphorylation (Yu et al., 2019; Wu et al., 2014; Cheon et al., 2009). Both CM and CI extracts reduce oxidative stress markers such as MDA and increase antioxidant enzyme activities, including SOD, CAT, and GPX (Lii et al., 2010; Zhan et al., 2022; Hao et al., 2021; Tian et al., 2019; Zhang et al., 2019; Kim et al., 2017). The anti-oxidative mechanism of CM involves the arginine and purine metabolic pathways, the PI3K/Akt-mediated Nrf2/HO-1 signaling pathway, and others (Lii et al., 2010; Zhan et al., 2022; Hao et al., 2021; Tian et al., 2019). CM polysaccharides have demonstrated immunomodulatory effects in multiple studies, potentially through the NF-κB/TLR4 and IL-6/JAK2/STAT3 pathways, as well as by balancing Th1/Th2 and Th17/Treg cells (Tao et al., 2018; Tao et al., 2017; Wang et al., 2022). CI can regulate the immune response, which may be attributed to its flavonoid content (Cheng et al., 2005).
In TCM, infectious diseases are often conceptualized as disruptions to the body’s balance caused by pathogenic factors such as “heat”, “dampness”, and “wind” (Wu et al., 2023). To eliminate these influences, botanical drugs with properties that “clear heat” and “detoxify” are utilized (Xu et al., 2019; Fang et al., 2009). The objective is to restore balance and harmony within the body, thereby alleviating both the symptoms and underlying causes of the disease. Specifically, CM and CI are used for their heat-clearing and detoxifying attributes, which effectively eliminate these pathogenic factors (Commission CP, 2020). CM and CI are commonly employed in TCM to alleviate symptoms of infections, including fever, inflammation, swelling, and abscesses, which align with the TCM concepts of “heat” and “toxins”. In contemporary pharmacological research, CM and CI extracts have been shown to effectively alleviate infection severity and prevent associated complications by modulating inflammatory responses, enhancing immune function, and mitigating oxidative stress. However, our review reveals that research on CI’s antimicrobial and anti-inflammatory properties is likely more extensive compared to that on CM, indicating that CI may hold greater potential for treating infectious diseases. Additionally, the efficacy of CM and CI in TCM formulations warrants further attention. Sangju cough mixture, which includes CM, has demonstrated therapeutic efficacy against colds. It can also alleviate symptoms of mycoplasma pneumoniae (MP), expedite the negative conversion time of MP-IgM antibodies, and facilitate patient recovery (Yang et al., 2024; Ji et al., 2019). In addition, Xiasangju is a well-known TCM formula for treating fever and influenza, and its composition includes CI. Increasing evidence suggests its various pharmacological effects on bacterial infections, immune system disorders, and other conditions (Wu et al., 2022). Overall, CM and CI serve as prime examples of how traditional botanical knowledge can be integrated into contemporary therapeutic practices, providing valuable treatments for infectious diseases through their heat-clearing and detoxifying properties.
Moreover, we have not identified any clinical study reports on the use of CM and CI. In TCM, treatments typically involve TCM formulas rather than single botanical drugs. Therefore, conducting clinical studies using only single botanical drug may present challenges. Future research could focus on the application of traditional Chinese medicinal formulas that include CM and CI for treating infectious diseases (Yang et al., 2024; Ji et al., 2019; Wu et al., 2022).
However, comprehensive comparative studies between CM and CI are imperative. Rigorous investigations are required to elucidate the precise molecular mechanisms underlying their antimicrobial, anti-inflammatory, immunomodulatory, and antioxidant effects, including the interactions of specific compounds with cellular targets and signaling pathways. Furthermore, potential synergistic effects among different compounds within CM and CI warrant exploration. Additionally, both CM and CI extracts have demonstrated promising effects in preliminary studies, and further investigations, such as pharmacokinetic studies, could enhance our understanding of their bioavailability. Finally, establishing robust quality control measures and standardization protocols for CM and CI extracts will ensure consistent efficacy and safety in therapeutic applications, ultimately aiding in the fight against infectious diseases.
YL: Writing–original draft. TL: Writing–review and editing. DW: Writing–review and editing. QL: Writing–review and editing.
The author(s) declare that financial support was received for the research and/or publication of this article. This study was supported by the Innovation Team Project of the State Administration of traditional Chinese medicine (No. ZYYCXTD-D-202201).
Acknowledgement for the data support from Plant Data Center of Chinese Academy of Sciences (https://www.plantplus.cn) (Figure 2).
The authors declare that the research was conducted in the absence of any commercial or financial relationships that could be construed as a potential conflict of interest.
The author(s) declare that no Generative AI was used in the creation of this manuscript.
All claims expressed in this article are solely those of the authors and do not necessarily represent those of their affiliated organizations, or those of the publisher, the editors and the reviewers. Any product that may be evaluated in this article, or claim that may be made by its manufacturer, is not guaranteed or endorsed by the publisher.
The Supplementary Material for this article can be found online at: https://www.frontiersin.org/articles/10.3389/fphar.2025.1538311/full#supplementary-material
Akihisa, T., Franzblau, S. G., Ukiya, M., Okuda, H., Zhang, F., Yasukawa, K., et al. (2005). Antitubercular activity of triterpenoids from Asteraceae flowers. Biol. Pharm. Bull. 28 (1), 158–160. doi:10.1248/bpb.28.158
Ashique, S., Mishra, N., Mantry, S., Garg, A., Kumar, N., Gupta, M., et al. (2025). Crosstalk between ROS-inflammatory gene expression axis in the progression of lung disorders. Naunyn Schmiedeb. Arch. Pharmacol. 398 (1), 417–448. doi:10.1007/s00210-024-03392-1
Astani, A., Reichling, J., and Schnitzler, P. (2011). Screening for antiviral activities of isolated compounds from essential oils. Evid. Based Complement. Altern. Med. 2011, 253643. doi:10.1093/ecam/nep187
Boudou, F., Belakredar, A., Keziz, A., Aissani, L., Alsaeedi, H., Cronu, D., et al. (2024). Therapeutic potential of Curcuma longa against monkeypox: antioxidant, anti-inflammatory, and computational insights. Front. Chem. 12, 1509913. doi:10.3389/fchem.2024.1509913
Chen, J., Zhou, Z., Wu, N., Li, J., Xi, N., Xu, M., et al. (2024). Chlorogenic acid attenuates deoxynivalenol-induced apoptosis and pyroptosis in human keratinocytes via activating Nrf2/HO-1 and inhibiting MAPK/NF-κB/NLRP3 pathways. Biomed. Pharmacother. 170, 116003. doi:10.1016/j.biopha.2023.116003
Chen, W., Vermaak, I., and Viljoen, A. (2013). Camphor--a fumigant during the Black Death and a coveted fragrant wood in ancient Egypt and Babylon--a review. Molecules 18 (5), 5434–5454. doi:10.3390/molecules18055434
Chen, X., Wang, H., Yang, X., Jiang, J., Ren, G., Wang, Z., et al. (2020). Small-scale alpine topography at low latitudes and high altitudes: refuge areas of the genus Chrysanthemum and its allies. Hortic. Res. 7, 184. doi:10.1038/s41438-020-00407-9
Chen Caiying, C. D., Zhao, Y., and Han, Z. (2015). Textual research on medicine origin of wild Chrysanthemum. J. Hunan Univ. Chin. Med. 35 (5), 69–72. doi:10.3969/j.issn.1674-070X.2015.05.023
Cheng, W., Li, J., You, T., and Hu, C. (2005). Anti-inflammatory and immunomodulatory activities of the extracts from the inflorescence of Chrysanthemum indicum Linné. J. Ethnopharmacol. 101 (1-3), 334–337. doi:10.1016/j.jep.2005.04.035
Cheon, M. S., Yoon, T., Lee, D. Y., Choi, G., Moon, B. C., Lee, A. Y., et al. (2009). Chrysanthemum indicum Linné extract inhibits the inflammatory response by suppressing NF-kappaB and MAPKs activation in lipopolysaccharide-induced RAW 264.7 macrophages. J. Ethnopharmacol. 122, 3.473–477. doi:10.1016/j.jep.2009.01.034
Commission CP (2020). Pharmacopoeia of the people’s Republic of China. Available online at: https://ydz.chp.org.cn/#/item?bookId=1&entryId=496 (Accessed April 01, 2024).
Committee FoCE (1983). Flora reipublicae popularis sinicae. Available online at: https://www.plantplus.cn/info/Dendranthema%20morifolium?t=z (Accessed May 12, 2024).
Committee FoCE (2011). Flora of China. Available online at: https://www.plantplus.cn/cn/sp/Chrysanthemum%20indicum?t=foc (Accessed May 10, 2024).
Costa, J. P., Sousa, S. A., Galvão, A. M., Mata, J. M., Leitão, J. H., and Carvalho, M. (2021). Key parameters on the antibacterial activity of silver camphor complexes. Antibiot. (Basel) 10, 135. doi:10.3390/antibiotics10020135
D'Acquisto, F., Iuvone, T., Rombolà, L., Sautebin, L., Di Rosa, M., and Carnuccio, R. (1997). Involvement of NF-kappaB in the regulation of cyclooxygenase-2 protein expression in LPS-stimulated J774 macrophages. FEBS Lett. 418 (1-2), 175–178. doi:10.1016/s0014-5793(97)01377-x
Esposito, S., De Simone, G., Boccia, G., De Caro, F., and Pagliano, P. (2017). Sepsis and septic shock: new definitions, new diagnostic and therapeutic approaches. J. Glob. Antimicrob. Resist 10, 204–212. doi:10.1016/j.jgar.2017.06.013
Fang, J., Wan, J., Tang, J., Wang, W., and Liu, Y. (2009). Radix isatidis and infectious diseases caused by viruses. Zhongguo Zhong Yao Za Zhi 34 (24), 3169–3172.
Gamal El-Din, M. I., Youssef, F. S., Ashour, M. L., Eldahshan, O. A., and Singab, A. N. B. (2018). Comparative analysis of volatile constituents of Pachira aquatica Aubl. and Pachira glabra Pasq., their anti-mycobacterial and anti-helicobacter pylori activities and their metabolic discrimination using chemometrics. J. Essent. Oil Bear. Plants, 21. 1550–1567. doi:10.1080/0972060X.2019.1571950
Gao, M. H., Li, H., Zhang, L., and Xiao, S. X. (2008). Studies on chemical constituents from flowers of Chrysanthemum indicum. Zhong Yao Cai 31 (5), 682–684.
Gu, Q. C. Y., Cui, H., Huang, D., Zhou, J. W., Wu, T. Z., Chen, Y. P., et al. (2013). Chrysanolide A, an unprecedented sesquiterpenoid trimer from the flowers of Chrysanthemum indicum L. RSC Adv. 3, 10168–10172. doi:10.1039/c3ra23172k
Guo, Q., Fang, H., and Shen, H. (2010). Determination of chlorogenic acid, caffeic acid and linarin in Flos Chrysanthemi Indici from different places by RP-hPLC. Zhongguo Zhong Yao Za Zhi 35 (9), 1160–1163. doi:10.4268/cjcmm20100917
Hamad, R. S., El Sherif, F., Al Abdulsalam, N. K., and Abd El-Moaty, H. I. (2023). Chlorogenic acid derived from Moringa oleifera leaf as a potential antiinflammatory agent against cryptosporidiosis in mice. Trop. Biomed. 40 (1), 45–54. doi:10.47665/tb.40.1.010
Han, J. M., Song, H. Y., Seo, H. S., Byun, E. H., Lim, S. T., Kim, W. S., et al. (2021). Immunoregulatory properties of a crude extraction fraction rich in polysaccharide from Chrysanthemum zawadskii Herbich var. latilobum and its potential role as a vaccine adjuvant. Int. Immunopharmacol. 95, 107513. doi:10.1016/j.intimp.2021.107513
Han, X., Wu, Y. C., Meng, M., Sun, Q. S., Gao, S. M., and Sun, H. (2018). Linarin prevents LPS-induced acute lung injury by suppressing oxidative stress and inflammation via inhibition of TXNIP/NLRP3 and NF-κB pathways. Int. J. Mol. Med. 42 (3), 1460–1472. doi:10.3892/ijmm.2018.3710
Hao, Y., Li, Y., Liu, J., Wang, Z., Gao, B., Zhang, Y., et al. (2021). Protective effect of Chrysanthemum morifolium cv. Fubaiju hot-water extracts against ARPE-19 cell oxidative damage by activating PI3K/Akt-Mediated Nrf2/HO-1 signaling pathway. Front. Nutr. 8, 648973. doi:10.3389/fnut.2021.648973
Hara, H., Tsuchiya, K., Kawamura, I., Fang, R., Hernandez-Cuellar, E., Shen, Y., et al. (2013). Phosphorylation of the adaptor ASC acts as a molecular switch that controls the formation of speck-like aggregates and inflammasome activity. Nat. Immunol. 14, 12.1247–1255. doi:10.1038/ni.2749
Ji, S., He, D. D., Su, Z. Y., Du, Y., Wang, Y. J., Gao, S. K., et al. (2019). P450 enzymes-based metabolic interactions between monarch drugs and the other constituent herbs: a strategy to explore compatibility mechanism of Sangju-Yin. Phytomedicine 58, 152866. doi:10.1016/j.phymed.2019.152866
Jiang, D., Liang, J., and Noble, P. W. (2011). Hyaluronan as an immune regulator in human diseases. Physiol. Rev. 91 (1), 221–264. doi:10.1152/physrev.00052.2009
Kang, S. C., Lim, S. Y., and Song, Y. J. (2013). Lupeol is one of active components in the extract of Chrysanthemum indicum Linne that inhibits LMP1-induced NF-κB activation. PLoS One 8 (11), e82688. doi:10.1371/journal.pone.0082688
Kim, I. H., Lee, T. K., Cho, J. H., Lee, J. C., Park, J. H., Ahn, J. H., et al. (2017). Pre-treatment with Chrysanthemum indicum Linné extract protects pyramidal neurons from transient cerebral ischemia via increasing antioxidants in the gerbil hippocampal CA1 region. Mol. Med. Rep. 16 (1), 133–142. doi:10.3892/mmr.2017.6591
Kim, J. E., Jun, S., Song, M., Kim, J. H., and Song, Y. J. (2012). The extract of Chrysanthemum indicum Linne inhibits EBV LMP1-induced NF-κB activation and the viability of EBV-transformed lymphoblastoid cell lines. Food Chem. Toxicol. 50, 5.1524–1528. doi:10.1016/j.fct.2012.02.034
Kim, J. W., and Kim, C. (2005). Inhibition of LPS-induced NO production by taurine chloramine in macrophages is mediated though Ras-ERK-NF-kappaB. Biochem. Pharmacol. 70, 9.1352–1360. doi:10.1016/j.bcp.2005.08.006
Kuang, C. L., Lv, D., Shen, G. H., Li, S. S., Luo, Q. Y., and Zhang, Z. Q. (2018). Chemical composition and antimicrobial activities of volatile oil extracted from Chrysanthemum morifolium Ramat. J. Food Sci. Technol. 55, 7.2786–2794. doi:10.1007/s13197-018-3203-1
Lee, D. Y., Choi, G., Yoon, T., Cheon, M. S., Choo, B. K., and Kim, H. K. (2009). Anti-inflammatory activity of Chrysanthemum indicum extract in acute and chronic cutaneous inflammation. J. Ethnopharmacol. 123 (1), 149–154. doi:10.1016/j.jep.2009.02.009
Lee, J. S., Kim, H. J., and Lee, Y. S. (2003). A new anti-HIV flavonoid glucuronide from Chrysanthemum morifolium. Planta Med. 69 (9), 859–861. doi:10.1055/s-2003-43207
Lee, Y., Lee, J., Lee, M. S., Chang, E., and Kim, Y. (2021). Chrysanthemum morifolium flower extract ameliorates obesity-induced inflammation and increases the muscle mitochondria content and AMPK/SIRT1 activities in obese rats. Nutrients 13, 3660. doi:10.3390/nu13103660
Li, L., Gu, L., Chen, Z., Wang, R., Ye, J., and Jiang, H. (2010). Toxicity study of ethanolic extract of Chrysanthemum morifolium in rats. J. Food Sci. 75 (6), T105–T109. doi:10.1111/j.1750-3841.2010.01702.x
Liang, X., Wu, H., and Su, W. (2014). A rapid UPLC-PAD fingerprint analysis of Chrysanthemum morifolium Ramat combined with chemometrics methods. Food Anal. Methods 7 (1), 197–204. doi:10.1007/s12161-013-9618-4
Lii, C. K., Lei, Y. P., Yao, H. T., Hsieh, Y. S., Tsai, C. W., Liu, K. L., et al. (2010). Chrysanthemum morifolium Ramat. reduces the oxidized LDL-induced expression of intercellular adhesion molecule-1 and E-selectin in human umbilical vein endothelial cells. J. Ethnopharmacol. 128 (1), 213–220. doi:10.1016/j.jep.2010.01.018
Lin, G. H., Lin, L., Liang, H. W., Ma, X., Wang, J. Y., Wu, L. P., et al. (2010). Antioxidant action of a Chrysanthemum morifolium extract protects rat brain against ischemia and reperfusion injury. J. Med. Food 13 (2), 306–311. doi:10.1089/jmf.2009.1184
Liu, G., Zheng, Q., Pan, K., and Xu, X. (2020). Protective effect of Chrysanthemum morifolium Ramat. ethanol extract on lipopolysaccharide induced acute lung injury in mice. BMC Complement. Med. Ther. 20 (1), 235. doi:10.1186/s12906-020-03017-z
Liu, L. L., Ha, T. K., Ha, W., Oh, W. K., Yang, J. L., and Shi, Y. P. (2017). Sesquiterpenoids with various carbocyclic skeletons from the flowers of Chrysanthemum indicum. J. Nat. Prod. 80 (2), 298–307. doi:10.1021/acs.jnatprod.6b00694
Liu, X. J., Li, Y., Su, S. L., Wei, D. D., Yan, H., Guo, S., et al. (2022). Comparative analysis of chemical composition andAntibacterial and anti-inflammatory activities of theEssential oils from Chrysanthemum morifolium ofDifferent flowering stages and different parts. Evid. Based Complement. Altern. Med. 2022, 5954963. doi:10.1155/2022/5954963
Looney, M. R., Su, X., Van Ziffle, J. A., Lowell, C. A., and Matthay, M. A. (2006). Neutrophils and their Fc gamma receptors are essential in a mouse model of transfusion-related acute lung injury. J. Clin. Invest. 116 (6), 1615–1623. doi:10.1172/jci27238
Meenu, M., Padhan, B., Patel, M., Patel, R., and Xu, B. (2023). Antibacterial activity of essential oils from different parts of plants against Salmonella and Listeria spp. Food Chem. 404, 134723. Pt B. doi:10.1016/j.foodchem.2022.134723
Miyazawa, M., and Hisama, M. (2003). Antimutagenic activity of flavonoids from Chrysanthemum morifolium. Biosci. Biotechnol. Biochem. 67, 10.2091–2099. doi:10.1271/bbb.67.2091
Moens, U., Kostenko, S., and Sveinbjørnsson, B. (2013). The role of mitogen-activated protein kinase-activated protein kinases (MAPKAPKs) in inflammation. Genes (Basel) 4 (2), 101–133. doi:10.3390/genes4020101
Park, M. Y., and Kang, D. H. (2021). Antibacterial activity of caffeic acid combined with UV-A light against Escherichia coli O157:H7, Salmonella enterica serovar typhimurium, and Listeria monocytogenes. Appl. Environ. Microbiol. 87 (15), e0063121. doi:10.1128/aem.00631-21
Peng, A., Lin, L., and Zhao, M. (2020). Screening of key flavonoids and monoterpenoids for xanthine oxidase inhibitory activity-oriented quality control of Chrysanthemum morifolium Ramat. 'Boju' based on spectrum-effect relationship coupled with UPLC-TOF-MS and HS-SPME-GC/MS. Food Res. Int. 137, 109448. doi:10.1016/j.foodres.2020.109448
Sander, W. J., Fourie, C., Sabiu, S., O'Neill, F. H., Pohl, C. H., and O'Neill, H. G. (2022). Reactive oxygen species as potential antiviral targets. Rev. Med. Virol. 32, 1.e2240. doi:10.1002/rmv.2240
Sangeetha Vijayan, P., Xavier, J., and Valappil, M. P. (2024). A review of immune modulators and immunotherapy in infectious diseases. Mol. Cell Biochem. 479 (8), 1937–1955. doi:10.1007/s11010-023-04825-w
Shao, Y., Sun, Y., Li, D., Chen, Y., and Chrysanthemum indicum, L. (2020). Chrysanthemum indicum L.: a comprehensive review of its botany, phytochemistry and pharmacology. Am. J. Chin. Med. 48 (4), 871–897. doi:10.1142/s0192415x20500421
Shen, J., Wang, G., and Zuo, J. (2018). Caffeic acid inhibits HCV replication via induction of IFNα antiviral response through p62-mediated Keap1/Nrf2 signaling pathway. Antivir. Res. 154, 154.166–173. doi:10.1016/j.antiviral.2018.04.008
Shibata, T., Fujimoto, K., Nagayama, K., Yamaguchi, K., and Nakamura, T. (2002). Inhibitory activity of brown algal phlorotannins against hyaluronidase. Int. J. Food Sci. and Technol. 37 (6), 703–709. doi:10.1046/j.1365-2621.2002.00603.x
Shunying, Z., Yang, Y., Huaidong, Y., Yue, Y., and Guolin, Z. (2005). Chemical composition and antimicrobial activity of the essential oils of Chrysanthemum indicum. J. Ethnopharmacol. 96 (1-2), 151–158. doi:10.1016/j.jep.2004.08.031
Sokolova, A. S., Yarovaya, C. O., Shernyukov, C. A., Pokrovsky, C. E., Pokrovsky, C. A., Lavrinenko, V. A., et al. (2013). New quaternary ammonium camphor derivatives and their antiviral activity, genotoxic effects and cytotoxicity. Bioorg Med. Chem. 21, 21.6690–6698. doi:10.1016/j.bmc.2013.08.014
Sokolova, A. S., Yarovaya, O. C., Korchagina, D. V., Zarubaev, V. V., Tretiak, T. S., Anfimov, P. M., et al. (2014). Camphor-based symmetric diimines as inhibitors of influenza virus reproduction. Bioorg Med. Chem. 22, 7.2141–2148. doi:10.1016/j.bmc.2014.02.038
Sokolova, A. S., Yarovaya, O. I., Shernyukov, A. V., Gatilov, Y. V., Razumova, Y. V., Zarubaev, V. V., et al. (2015). Discovery of a new class of antiviral compounds: camphor imine derivatives. Eur. J. Med. Chem. 105, 263–273. doi:10.1016/j.ejmech.2015.10.010
Sudha, P. N., and Rose, M. H. (2014). Beneficial effects of hyaluronic acid. Adv. Food Nutr. Res. 72, 137–176. doi:10.1016/b978-0-12-800269-8.00009-9
Sun, Y., Ma, X., and Liu, J. (2012). Compounds from fraction with cardiovascular activity of Chrysanthemum indicum. Zhongguo Zhong Yao Za Zhi 37 (1), 61–65.
Tao, J. H., Duan, J. A., Jiang, S., Feng, N. N., Qiu, W. Q., and Ling, Y. (2017). Polysaccharides from Chrysanthemum morifolium Ramat ameliorate colitis rats by modulating the intestinal microbiota community. Oncotarget 8, 46.80790–80803. doi:10.18632/oncotarget.20477
Tao, J. H., Duan, J. A., Zhang, W., Jiang, S., Guo, J. M., and Wei, D. D. (2018). Polysaccharides from Chrysanthemum morifolium Ramat ameliorate colitis rats via regulation of the metabolic profiling and NF-κ B/TLR4 and IL-6/JAK2/STAT3 signaling pathways. Front. Pharmacol. 9.746, 746. doi:10.3389/fphar.2018.00746
Termeer, C. C., Hennies, J., Voith, U., Ahrens, T., Weiss, J. M., Prehm, P., et al. (2000). Oligosaccharides of hyaluronan are potent activators of dendritic cells. J. Immunol. 165 (4), 1863–1870. doi:10.4049/jimmunol.165.4.1863
Tian, Z., Jia, H., Jin, Y., Wang, M., Kou, J., Wang, C., et al. (2019). Chrysanthemum extract attenuates hepatotoxicity via inhibiting oxidative stress in vivo and in vitro. Food Nutr. Res. 63, 63. doi:10.29219/fnr.v63.1667
Wang, J., Chen, D., Liang, L., Xue, P., and Tu, P. (2010). Chemical constituents from flowers of Chrysanthemum indicum. Zhongguo Zhong Yao Za Zhi 35 (6), 718–721. doi:10.4268/cjcmm20100613
Wang, X., Shen, Y., Thakur, K., Han, J., Zhang, J. G., Hu, F., et al. (2020). Antibacterial activity and mechanism of ginger essential oil against Escherichia coli and Staphylococcus aureus. Molecules 25, 3955. doi:10.3390/molecules25173955
Wang, Y., Chen, X., Zhao, P., Ren, L., Li, X., and Gao, W. (2022). Physicochemical characteristics and immunoregulatory activities of polysaccharides from five cultivars of Chrysanthemi Flos. Food Sci. Nutr. 10 (5), 1391–1400. doi:10.1002/fsn3.2720
Wang Xl, P. S., Liang, J. P., and Yu, K. B. (2006). (3β,5α,6β,7β,14β)-eudesmen-3,5,6,11-tetrol methanol solvate: a new sesquiterpenoid fromchrysanthemum indicuml. Acta Crystallogr. Sect. E Struct. Rep. Online 62 (8), o3570–o3571. doi:10.1107/s1600536806028522
Wu, S., Luo, H., Zhong, Z., Ai, Y., Zhao, Y., Liang, Q., et al. (2022). Phytochemistry, pharmacology and quality control of Xiasangju: a traditional Chinese medicine formula. Front. Pharmacol. 13, 930813. doi:10.3389/fphar.2022.930813
Wu, S., Su, W., Fan, Q., Shang, H., Xiao, W., and Wang, Y. (2023). Traditional Chinese medicine for the common cold: evidence and potential mechanisms. Am. J. Chin. Med. 51 (3), 487–515. doi:10.1142/s0192415x23500258
Wu, T., Jiang, C., Wang, L., Morris-Natschke, S. L., Miao, H., Gu, L., et al. (2015). 3,5-Diarylpyrazole derivatives obtained by ammonolysis of the total flavonoids from Chrysanthemum indicum extract show potential for the treatment of alzheimer's disease. J. Nat. Prod. 78 (7), 1593–1599. doi:10.1021/acs.jnatprod.5b00156
Wu, X. L., Feng, X. X., Li, C. W., Zhang, X. J., Chen, Z. W., Chen, J. N., et al. (2014). The protective effects of the supercritical-carbon dioxide fluid extract of Chrysanthemum indicum against lipopolysaccharide-induced acute lung injury in mice via modulating Toll-like receptor 4 signaling pathway. Mediat. Inflamm. 2014, 246407. doi:10.1155/2014/246407
Xie, Y. Y., Yuan, D., Yang, J. Y., Wang, L. H., and Wu, C. F. (2009). Cytotoxic activity of flavonoids from the flowers of Chrysanthemum morifolium on human colon cancer Colon205 cells. J. Asian Nat. Prod. Res. 11 (9), 771–778. doi:10.1080/10286020903128470
Xu, H., He, L., Chen, J., Hou, X., Fan, F., Wu, H., et al. (2019). Different types of effective fractions from Radix Isatidis revealed a multiple-target synergy effect against respiratory syncytial virus through RIG-I and MDA5 signaling pathways, a pilot study to testify the theory of superposition of traditional Chinese Medicine efficacy. J. Ethnopharmacol. 239, 111901. doi:10.1016/j.jep.2019.111901
Xue, G. M., Li, X. Q., Chen, C., Chen, K., Wang, X. B., Gu, Y. C., et al. (2018). Highly oxidized guaianolide sesquiterpenoids with potential anti-inflammatory activity from Chrysanthemum indicum. J. Nat. Prod. 81 (2), 378–386. doi:10.1021/acs.jnatprod.7b00867
Yamaguchi, T. (2022). Antibacterial effect of the combination of terpenoids. Arch. Microbiol. 204, 520. doi:10.1007/s00203-022-03142-y
Yang, P. F., Feng, Z. M., Yang, Y. N., Jiang, J. S., and Zhang, P. C. (2017). Neuroprotective caffeoylquinic acid derivatives from the flowers of Chrysanthemum morifolium. J. Nat. Prod. 80, 4.1028–1033. doi:10.1021/acs.jnatprod.6b01026
Yang, P. F., Yang, Y. N., Feng, Z. M., Jiang, J. S., and Zhang, P. C. (2019b). Six new compounds from the flowers of Chrysanthemum morifolium and their biological activities. Bioorg Chem. 82, 82.139–144. doi:10.1016/j.bioorg.2018.10.007
Yang, P. F., Yang, Y. N., He, C. Y., Chen, Z. F., Yuan, Q. S., Zhao, S. C., et al. (2019a). New caffeoylquinic acid derivatives and flavanone glycoside from the flowers of Chrysanthemum morifolium and their bioactivities. Molecules 24, 850. doi:10.3390/molecules24050850
Yang, X., Liu, Z., Liu, X., Li, Q., Huang, H., and Zhang, Z. (2024). Efficacy and influencing factors of Sangju cough mixture in the adjuvant treatment of adult patients with mycoplasma pneumoniae infection: a retrospective study. Infect. Drug Resist 17, 17.275–282. doi:10.2147/idr.S438202
Youssef, F. S., Eid, S. Y., Alshammari, E., Ashour, M. L., Wink, M., and El-Readi, M. Z. (2020). Chrysanthemum indicum and Chrysanthemum morifolium: chemical composition of their essential oils and their potential use as natural preservatives with antimicrobial and antioxidant activities. Foods 9, 1460. doi:10.3390/foods9101460
Yu, S. H., Sun, X., Kim, M. K., Akther, M., Han, J. H., Kim, T. Y., et al. (2019). Chrysanthemum indicum extract inhibits NLRP3 and AIM2 inflammasome activation via regulating ASC phosphorylation. J. Ethnopharmacol. 239, 111917. doi:10.1016/j.jep.2019.111917
Yu, Y. P., Lin, K. H., Shih, M. C., Chen, C. L., and Lu, C. P. (2023). Optimization of aqueous extraction of antioxidants from Chrysanthemum (C. morifolium Ramat and C. indicum L.) flowers and evaluation of their protection from glycoxidation damage on human αA-crystallin. Exp. Eye Res. 235, 109629. doi:10.1016/j.exer.2023.109629
Yuan, H., Jiang, S., Liu, Y., Daniyal, M., Jian, Y., Peng, C., et al. (2020). The flower head of Chrysanthemum morifolium Ramat. (Juhua): a paradigm of flowers serving as Chinese dietary herbal medicine. J. Ethnopharmacol. 261, 113043. doi:10.1016/j.jep.2020.113043
Zacchino, S. A., Butassi, E., Liberto, M. D., Raimondi, M., Postigo, A., and Sortino, M. (2017). Plant phenolics and terpenoids as adjuvants of antibacterial and antifungal drugs. Phytomedicine 37, 27–48. doi:10.1016/j.phymed.2017.10.018
Zeng, M., Li, M., Chen, Y., Zhang, J., Cao, Y., Zhang, B., et al. (2020). A new bisepoxylignan dendranlignan A isolated from Chrysanthemum Flower inhibits the production of inflammatory mediators via the TLR4 pathway in LPS-induced H9c2 cardiomyocytes. Arch. Biochem. Biophys. 690, 108506. doi:10.1016/j.abb.2020.108506
Zhan, G., Long, M., Shan, K., Xie, C., and Yang, R. (2022). Antioxidant effect of Chrysanthemum morifolium (chuju) extract on H(2)O(2)-treated L-O2 cells as revealed by LC/MS-Based metabolic profiling. Antioxidants (Basel) 11, 1068. doi:10.3390/antiox11061068
Zhang, D., Gao, W., Cui, X., Qiao, R., and Li, C. (2024). Caffeic acid and cyclen-based hydrogel for synergistic antibacterial therapy. ACS Appl. Mater Interfaces 16, 34.44493–44503. doi:10.1021/acsami.4c09037
Zhang, K., Jiang, Y., Zhao, H., Köllner, T. G., Chen, S., Chen, F., et al. (2020). Diverse terpenoids and their associated antifungal properties from roots of different cultivars of Chrysanthemum morifolium Ramat. Molecules 25, 2083. doi:10.3390/molecules25092083
Zhang, L., and Wang, C. C. (2014). Inflammatory response of macrophages in infection. Hepatobiliary Pancreat. Dis. Int. 13 (2), 138–152. doi:10.1016/s1499-3872(14)60024-2
Zhang, X., Wu, J. Z., Lin, Z. X., Yuan, Q. J., Li, Y. C., Liang, J. L., et al. (2019). Ameliorative effect of supercritical fluid extract of Chrysanthemum indicum Linnén against D-galactose induced brain and liver injury in senescent mice via suppression of oxidative stress, inflammation and apoptosis. J. Ethnopharmacol. 234, 44–56. doi:10.1016/j.jep.2018.12.050
Zheng, C., Dong, Q., Chen, H., Cong, Q., and Ding, K. (2015). Structural characterization of a polysaccharide from Chrysanthemum morifolium flowers and its antioxidant activity. Carbohydr. Polym. 130, 130.113–121. doi:10.1016/j.carbpol.2015.05.004
Zhou, H., Zhang, X., Li, B., and Yue, R. (2023). Fast and efficient identification of hyaluronidase specific inhibitors from Chrysanthemum morifolium Ramat. using UF-LC-MS technique and their anti-inflammation effect in macrophages. Heliyon 9 (2), e13709. doi:10.1016/j.heliyon.2023.e13709
Keywords: Chrysanthemum morifolium, Chrysanthemum indicum, anti-infection, major compounds, pharmacological effects
Citation: Liang Y, Liu T, Wang D and Liu Q (2025) Exploring the antimicrobial, anti-inflammatory, antioxidant, and immunomodulatory properties of Chrysanthemum morifolium and Chrysanthemum indicum: a narrow review. Front. Pharmacol. 16:1538311. doi: 10.3389/fphar.2025.1538311
Received: 02 December 2024; Accepted: 27 February 2025;
Published: 19 March 2025.
Edited by:
Yong Guo, University of South China, ChinaCopyright © 2025 Liang, Liu, Wang and Liu. This is an open-access article distributed under the terms of the Creative Commons Attribution License (CC BY). The use, distribution or reproduction in other forums is permitted, provided the original author(s) and the copyright owner(s) are credited and that the original publication in this journal is cited, in accordance with accepted academic practice. No use, distribution or reproduction is permitted which does not comply with these terms.
*Correspondence: Dong Wang, d2FuZ2RvbmdAY2R1dGNtLmVkdS5jbg==
Disclaimer: All claims expressed in this article are solely those of the authors and do not necessarily represent those of their affiliated organizations, or those of the publisher, the editors and the reviewers. Any product that may be evaluated in this article or claim that may be made by its manufacturer is not guaranteed or endorsed by the publisher.
Research integrity at Frontiers
Learn more about the work of our research integrity team to safeguard the quality of each article we publish.