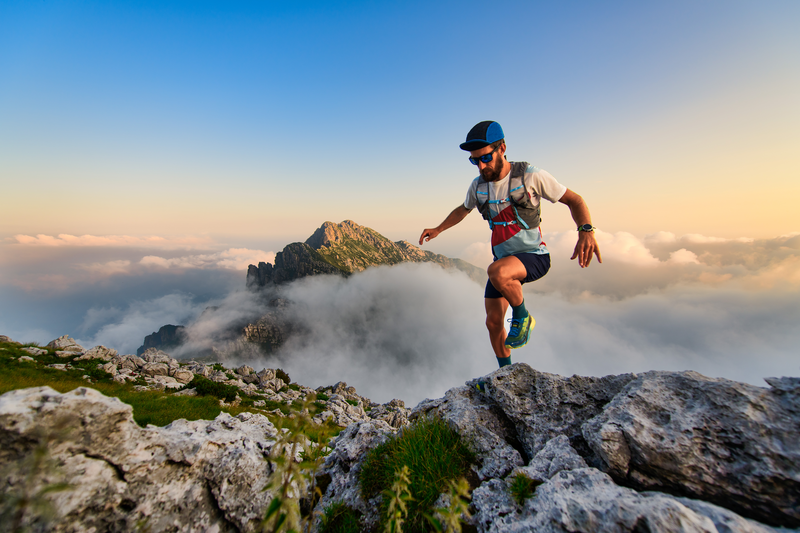
94% of researchers rate our articles as excellent or good
Learn more about the work of our research integrity team to safeguard the quality of each article we publish.
Find out more
REVIEW article
Front. Pharmacol. , 12 February 2025
Sec. Ethnopharmacology
Volume 16 - 2025 | https://doi.org/10.3389/fphar.2025.1535555
This article is part of the Research Topic Food Derived Bioactive Metabolites: Unlocking their Potential Health Benefits and Medical Potential View all 6 articles
Luteolin is a flavonoid widely found in plants, including vegetables, botanical drugs, and fruits. Owing to its diverse pharmacological activities, such as anticancer, oxidative stress protection, anti-inflammatory, and neuron-preserving effects, luteolin has attracted attention in research and medicine. Luteolin exhibits therapeutic effects on various pulmonary disease models through multiple molecular mechanisms; these include inhibition of activation of the PI3K/Akt-mediated Nuclear Factor kappa-B (NF-κB) and MAPK signaling pathways, as well as the promotion of regulatory T cell (Treg) function and enhancement of alveolar epithelial sodium channel (ENaC) activity (alleviating inflammation and oxidative stress responses). Luteolin has therapeutic effects on chronic obstructive pulmonary disease (COPD), acute lung injury/acute respiratory distress syndrome (ALI/ARDS), pulmonary fibrosis, allergic asthma, and lung cancer. Luteolin, a naturally occurring polyphenol, is poorly water-soluble. The oral route may be ineffective because the gut poorly absorbs this type of flavonoid. Therefore, although luteolin exhibits significant biological activity, its clinical application is limited by challenges associated with its poor water solubility and low bioavailability, which are critical factors for its efficacy and pharmacological application. These challenges can be addressed by modifying the chemical structure and enhancing pharmaceutical formulations. We summarized the research advancements in improving the solubility and bioavailability of luteolin, as well as the effects of luteolin on various pulmonary diseases and their related mechanisms, with the aim of providing new ideas for researchers.
Pulmonary diseases are one of the major health issues worldwide, with high incidence and mortality rates (Chen and Li, 2024; Meng et al., 2025; GBDCRD, 2023). According to the World Health Organization, COPD, asthma, pulmonary fibrosis, lung cancer, and other pulmonary diseases lead to millions of deaths each year (Roh, 2024; Wang et al., 2025; Podolanczuk and Raghu, 2024; Lu et al., 2025). With the aging of the population and the intensification of environmental issues, the burden of pulmonary diseases is expected to increase further (Faniyi et al., 2022). Although there are various drugs available for the treatment of pulmonary diseases, there are still many challenges, such as drug side effects, drug resistance, and the lack of effective treatments for certain diseases (Mazumder et al., 2024; Dorababu and Maraswami, 2023; Bandyopadhyay and Mirsaeidi, 2023).
Against this backdrop, the potential of natural products in the treatment of pulmonary diseases is increasingly gaining attention. Natural products, with their rich chemical diversity and biological activity, offer valuable resources for drug development. Compared with traditional chemical drugs, natural products often have better biocompatibility and lower toxicity, and have shown unique advantages in regulating the body’s immune system, anti-inflammatory, and antioxidant aspects (Li C. et al., 2024; Wang et al., 2023). From traditional Chinese medicine to modern botanical drugs, the application of natural products in the treatment of pulmonary diseases has a long history, and new research results are constantly emerging (Rahman et al., 2022).
Currently, there are numerous narrative reports about luteolin in various diseases (Zhang et al., 2024; Shi et al., 2024; Huang L. et al., 2023; Diniz et al., 2022), but most of the research on pulmonary diseases focuses on describing its therapeutic effects, with few exploring and summarizing its potential mechanisms. This study summarizes the effects of luteolin on various pulmonary diseases and related mechanisms, aiming to provide new insights for researchers and to promote further research and application of luteolin in the field of pulmonary disease treatment.
Over the last 2 decades, there has been mounting emphasis on utilizing metabolites derived from medicinal plants to treat and manage a diverse array of diseases (Xiong et al., 2021; Ahmad et al., 2020; Singh et al., 2023; Ramadaini et al., 2024). Luteolin, an active flavonoid metabolite isolated from Lonicera japonica, exhibits many biological activities. It is also known for its potent antioxidant and anti-inflammatory characteristics (Xiong et al., 2024; Jia et al., 2023). Plants abundant in luteolin have been used in traditional Chinese medicine for treating a wide array of illnesses, including hypertension (Gao et al., 2023), various inflammatory conditions (Attiq et al., 2021), and cancer (Jiang et al., 2021). In addition to its medicinal use, luteolin is also present in various vegetables, such as fresh Spinacia oleracea, Petroselinum crispum, and Brassica oleracea var. Capitata, and a range of fruits and botanical drugs (Manzoor et al., 2019; Almatroodi et al., 2024). Flavonoids—prevalent polyphenolic metabolites within the plant kingdom—have demonstrated health benefits in humans at particular concentrations (Wen et al., 2021). These metabolites are biosynthesized via the phenylpropanoid pathway in plants (Cocuron et al., 2019). Luteolin has a classic flavonoid structure featuring four hydroxyl groups at 5′, 7′, 3′, and 4′positions. The structural hallmark of luteolin includes two aromatic rings, A and B, which are linked to an oxygenated pyran ring labeled as C. The biological activities of luteolin are potentially contingent upon the presence of its hydroxyl groups and the double bond that exists between carbons 2 and 3. Typically, luteolin is encountered in a glycosylated form, where one or more of its hydroxyl groups have been replaced by diverse glycosyl groups (Huang L. et al., 2023). Similar to other flavonoids, luteolin is an antioxidant and can prevent damage to DNA, lipids, and proteins induced by reactive oxygen species (ROS) (Zou et al., 2021). Molecules such as hydrogen peroxide (H2O2), singlet oxygen, and hydroxyl radicals are highly reactive with numerous biological targets and play crucial roles in neuromodulation, immunomodulation, ion transport, and apoptosis (Mittler, 2017). Luteolin influences the activities of various enzymes associated with oxidative stress. Among these, oxidases responsible for the generation of ROS, such as xanthine oxidase, are inhibited by luteolin (Liu X. et al., 2022). Luteolin also inhibits the activity of enzymes that catalyze the oxidation of cellular constituents, including cyclooxygenases and lipoxygenases (Han et al., 2024; Ren et al., 2021). Additionally, luteolin can be oxidized and serves as a scavenger of ROS—an activity that stems from the chemical structure it shares with other flavonoids, enabling it to provide hydrogen atoms from the hydroxyl groups present on its aromatic rings (Ramesh et al., 2021). Despite the promising outlook on the therapeutic characteristics of luteolin, it is imperative to consider its possible toxic effects and broader health implications (Galati and O'Brien, 2004; Khan et al., 2021). Understanding the full spectrum of its effects on human biology is crucial for its effective use, as shown in Figure 1.
Figure 1. An illustration of the main source and anti-inflammatory, anticancer potential, antioxidant properties, antiviral activity and antiapoptotic characteristics of luteolin, created using Biorender.com.
The in vivo bioavailability of luteolin is relatively low owing to its poor stability and low absorption rate, which consequently diminishes its therapeutic effectiveness. The bioavailability of total luteolin, including free and bound forms, has been reported to be 53.9%. Despite an apparent good absorption rate, the level of free luteolin present in the systemic circulation is lower than that of its metabolites, with the actual bioavailability of free luteolin being merely 17.5%. This suggests that luteolin is promptly absorbed upon entering the rat intestinal system and undergoes comprehensive metabolism in the intestinal and/or hepatic cellular structures, which is the reason for its low bioavailability (Yao et al., 2023; Deng et al., 2017). Following oral administration, luteolin achieves its peak plasma concentration within 1–2 h and is retained in circulation for several hours thereafter. Its metabolism is sufficiently slow to allow the metabolite to exhibit biological activity in vivo (Ali and Siddique, 2019). When comparing the absorption efficiency of a single oral dose of pure luteolin or luteolin derived from peanut hull extract, the jejunum and duodenum had higher absorption rates than the colon and ileum. Furthermore, the bioavailability of luteolin from the peanut hull extracts significantly surpassed that of pure luteolin (Zhou et al., 2008). Upon absorption, luteolin is rapidly taken up, and can be detected in the plasma as luteolin, luteolin monoglucoside, and luteolin glucuronide, along with sulfate conjugates of luteolin aldehyde and O-methylated luteolin (Yasuda et al., 2015; Shimoi et al., 1998). Certain co-occurring metabolites, such as eriodictyol, enhance the absorption of luteolin in the intestine, thereby improving its bioavailability (Zhou et al., 2008). Moreover, various pharmaceutical techniques, including cyclodextrin complexation, luteolin-loaded nanocarriers, polymeric micelles, phospholipid complexation, RA (rosmarinic acid)-SS-mPEG, and combined hydroxyethyl starch luteolin nanocrystals have been used to augment the solubility and bioavailability of luteolin. These methods slow the degradation of luteolin in the bloodstream, thereby prolonging its circulation time (Liu et al., 2013; Khan et al., 2016; Qing et al., 2017; Kar et al., 2024; Luo et al., 2024; Lu et al., 2023; Pittol et al., 2022). Recently, Imam and colleagues prepared luteolin-loaded nanovesicles (NVs) using the solvent evaporation method, and antioxidant activity results demonstrated that luteolin-NVs exhibited greater activity than pure luteolin. Cytotoxicity studies have shown that the IC50 value of luteolin NVs was lower than that of pure luteolin (Imam et al., 2022). Miao et al. formulated luteolin-loaded methoxy poly (ethylene glycol)-polylactide micelles (luteolin/MPEG-PLA) to enhance the bioavailability of luteolin in pulmonary infectious diseases (Miao et al., 2021). Ren et al. improved the bioavailability of luteolin four-fold using a cyclodextrin MOF-modified dry powder inhaler to treat fibrotic interstitial lung disease (Ren et al., 2023). These studies illustrate that strategies for improving the solubility and bioavailability of luteolin through structural modifications and formulation advancements are likely to facilitate its use in the treatment of diseases in the future.
In the plant kingdom, luteolin is commonly found in a glycosylated state. During absorption, these glycosides are hydrolyzed to yield the free form of luteolin (Wang Z. et al., 2021). Glycosylation of the aglycone moiety primarily occurs via two distinct mechanisms: free hydroxyl groups to form O-glycosides and forming a carbon-carbon bond to create C-glycosides (Figure 2) (Hostetler et al., 2017; Punia Bangar et al., 2023). Following absorption, luteolin and its metabolites are rapidly and widely distributed throughout the major organs and tissues of the body. Throughout the rat circulatory system, luteolin is largely present in conjugated forms, with luteolin-glucuronide being the most prevalent metabolite circulating in most tissues (Hayasaka et al., 2018). In the current study, luteolin was highly concentrated in the liver, kidneys, gastrointestinal tract, and lungs in conjunction with its multiple metabolites. Importantly, luteolin can cross the blood-brain barrier, suggesting its potential for treating central nervous system diseases (Daily et al., 2021). These findings support the action of luteolin on these medicinal targets (Chen et al., 2010; Domitrović et al., 2009; Lu et al., 2015; Xu et al., 2015). Because of their ability to cross the placenta, these substances may be toxic to the fetus in the womb (Skibola and Smith, 2000).
Figure 2. An illustration of structural formulas (through the free hydroxyl groups to form O-glycosides and through the formation of a carbon-carbon bond to create C-glycosides) of metabolites related to luteolin, created using Biorender.com.
After absorption, flavonoids undergo metabolic processes in the liver, including glucuronidation, sulfation, and methylation. In addition, they can be metabolized into smaller phenolic metabolites (Bravo, 1998). Specifically, luteolin is first metabolized through glucuronidation or methylation and converted into a methylated glucuronide form. The principal Phase II metabolite has been confirmed to be luteolin-3′-O-glucuronide (Boersma et al., 2002). Luteolin-3′-O-glucuronide is the predominant metabolite in these metabolic pathways (Kure et al., 2016). Furthermore, two novel diglucuronide conjugates of luteolin were separated by incubation with uridine diphosphate glucuronosyltransferase (UGTs). Methylation has also been identified as a significant metabolic pathway for luteolin. This methylation process produces chrysoeriol and diosmetin, a reaction facilitated by catechol-O-methyltransferase in rats (Wu et al., 2015; Chen et al., 2011; Wang et al., 2017). Upon oral ingestion, luteolin is quickly absorbed into the systemic circulation and undergoes extensive metabolic processing within the rat body. The recovered metabolites included five isolates from the urine and two from the bile. Luteolin is rapidly metabolized, likely in the intestinal lining and/or the liver cells throughout the first-pass metabolism after oral administration (Boersma et al., 2002). Its metabolite, luteolin-3′-O-glucuronide is primarily excreted in the urine. The feces showed no evidence of conjugated metabolites, whereas approximately 5.8% of the starting dose was recovered as free luteolin. A second peak of luteolin and most metabolites occurred between 2 and 8 h after administration, suggesting the possible involvement of enterohepatic recirculation. Biliary excretion is the main route of elimination of orally administered luteolin. In particular, metabolites with 7′-O-glucuronidation show widespread metabolism and extensive distribution of luteolin in rats (Figure 3) (Shi et al., 2024; Wu et al., 2022).
Figure 3. An illustration of absorption, distribution, and metabolism of luteolin. Luteolin is absorbed from the intestine and enters the liver via the portal vein. During the first phase of metabolism, it is metabolized in the intestinal wall and/or by liver cells. In the second phase of metabolism, it undergoes enterohepatic recirculation. It is then distributed to various organs and tissues throughout the body via the bloodstream and is finally excreted through urine and bile, created using Biorender.com.
Luteolin has demonstrated a variety of pharmacological influences in both in vitro and in vivo studies (Huang L. et al., 2023), possessing characteristics of antioxidant (Seelinger et al., 2008a), anti-tumor (Ganai et al., 2021; Seelinger et al., 2008b), anti-microbial (El-Shiekh et al., 2023), anti-viral (Men et al., 2023), anti-inflammatory (Conti et al., 2021; Aziz et al., 2018), anti-apoptotic (Han et al., 2023), anti-allergic (Liang et al., 2020), anti-diabetic (Pradhan and Kulkarni, 2024), chemopreventive (Nguyen et al., 2021), renoprotective (Diniz et al., 2022; Oyagbemi et al., 2021; Xiong et al., 2020), cardioprotective (Liu Z. et al., 2022; Xu et al., 2012) and neuroprotective (Nabavi et al., 2015; Ashaari et al., 2018) properties. Additionally, luteolin has shown anti-anxiety (Gadotti and Zamponi, 2019), antidepressant-like (Mokhtari et al., 2023), antipruritic (Ueda et al., 2005), hepatoprotective (Yao et al., 2023) and antithrombotic (Choi et al., 2015). Numerous studies have indicated that the toxicity of luteolin is minimal, showing no significant harmful effects in clinical trials (Terzo et al., 2023; Gelabert-Rebato et al., 2019; Taliou et al., 2013) (Alzaabi et al., 2022). This included a study by Terzo et al., where 50 obese individuals completed the research protocol. Studies have shown that luteolin’s effect in pre-obesity is safe and has no significant toxic side effects at normal dosages (Terzo et al., 2023). Therefore, the safety of luteolin provides a foundation for further exploration of its use in treating human pulmonary diseases. Some scholars have studied various aspects of luteolin, such as luteolin concentration, lowest toxic dose, and determination of interference effects (Rameshbabu et al., 2024). In terms of Lipinski’s rule of drug-likeness, luteolin meets Lipinski’s Rule of Five, demonstrating drug-like properties (Rameshbabu et al., 2024).
Interestingly, several studies have explored the potential of luteolin to protect lungs across various models. Luteolin also activates the Treg function (Zhang ZT. et al., 2021) and enhances transepithelial sodium transport (Hou et al., 2022) to alleviate inflammation-associated ALI. Here, we discuss the various roles of luteolin in improving dysfunction caused by pulmonary diseases.
ALI/ARDS has consistently been a primary cause of acute respiratory exhaustion in seriously ill patients, with high incidence and mortality rates over the past 20 years (Fielding-Singh et al., 2018). According to the “International Sepsis Consensus 5.0 Guidelines” (fifth edition, 2021) (Evans et al., 2021), sepsis is a multi-organ dysfunction caused by a dysregulated host inflammatory response to infection (Cavaillon and Chrétien, 2019; Cecconi et al., 2018). The lungs are the most affected and vulnerable target organs in the process of multiple organ dysfunction caused by sepsis (Fan and Fan, 2018). The pathophysiological process of sepsis-associated acute lung injury (SALI) involves disruption in epithelial and endothelial cell function, excessive accumulation and activation of immune cells, inflammatory response, oxidative stress, programmed cell death, and mobilization of coagulation pathways (Sun et al., 2023). Given the relatively high mortality rate and medical burden, ARDS interventions have always been at the forefront of critical care. Because current treatments for ALI are limited and face additional challenges, the introduction of alternative or complementary treatment methods is necessary (Patel et al., 2018). Fortunately, several “conventional” pharmacological therapies for ALI have been found to reduce mortality. Considering that luteolin is expected to have good effects on ALI, numerous studies have utilized luteolin to explore various cell and animal models induced by Lipopolysaccharide (LPS), demonstrating that luteolin exerts its effects on LPS-induced ALI by acting on multiple pathways involved in the pathogenesis of LPS-induced ALI (Figure 4).
Figure 4. An illustration of the signaling pathways by which luteolin affects ALI/ARDS, created using Biorender.com. The symbol “——|” represents inhibition, and “——>” represents promotion, “----→” represents indirect promotion (PI3K, phosphatidylinositol 3-kinase; GSH, Glutathione, Reduced; STAT3, signal transducer and activator of transcription 3; AKT, Protein kinase B; IL-18, interleukin-18; IL-1β, interleukin-1 beta; IL-10, interleukin-10; TNF-α, tumor necrosis factor; MDA, malondialdehyde; CNP, C-type natriuretic peptide; NLRP3, NOD-like receptor protein 3; SOD, serum superoxide dismutase; CAT, catalase; NF-κB, nuclear factor kappa-B; GSDMD-N, gasdermin D-N).
Studies using animal models of severe sepsis have shown that inflammation plays a key role in the pathophysiology of sepsis. Sepsis is associated with the activation of pro-inflammatory mediators, among which, NF-κB is an important pro-inflammatory transcription factor that mediates the upregulation of several pro-inflammatory cytokines and chemokines, such as tumor necrosis factor (TNF-α), interleukin-6 (IL-6), interleukin-8 (IL-8), and IL-1β, leading to further amplification of inflammatory damage. Luteolin exhibits anti-inflammatory effects by blocking the activity of Hsp90 in macrophages (Malhotra et al., 2001; Yeo et al., 2004; Chen et al., 2014). Thus, luteolin reduces the triggering of LPS-induced MAPK and NF-κB pathways. IL-10, an anti-inflammatory cytokine, can increase sepsis scores and mortality by diminishing inflammatory defenses and dampening immune responses (Levy et al., 2001; Sabat et al., 2010). The anti-inflammatory effects of luteolin are associated with reduced IL-10 levels. Luteolin protects lung tissue from SALI and reduces the excessive accumulation of IL-10 in the lungs during sepsis (Rungsung et al., 2018; Wang X. et al., 2021). Luteolin is a protective antagonist against LPS-induced ALI in mice. Pretreatment of septic mice significantly reduced the mRNA expression of ICAM-1. There is a protective impact on mice with SALI by curbing the ICAM-1, NF-κB, and specific inducible nitric oxide synthase (iNOS) signaling cascades and reducing the levels of pulmonary IL-6, IL-1β, and TNF-α (Rungsung et al., 2018). Luteolin activates Tregs to promote IL-10 expression and alleviates caspase-11-dependent pyroptosis in SALI, thereby significantly inhibiting inflammation and mitigating cecal ligation and puncture (CLP)-induced lung injury (Zhang Z. T. et al., 2021). Luteolin also regulates AKT1/iNOS levels to promote pyroptosis (Zhang et al., 2023). Therefore, luteolin has immense potential for treating ALI, and the pathways above can serve as therapeutic targets for reducing the levels of inflammatory cytokines induced by sepsis (Table 1).
Oxidative stress, characterized by an imbalance between ROS production and the antioxidant defense system, plays a critical role in inflammation-related diseases. Excessive ROS can induce cellular damage, impair normal physiological functions, trigger the release of inflammatory mediators, exacerbate inflammatory responses, and ultimately lead to irreversible tissue damage. Gu et al. coordinated Ce ions with luteolin to synthesize cerium-luteolin nanocomplexes (CeLutNCs). The prepared CeLutNCs can effectively eliminate excess ROS, prevent apoptosis, downregulate the levels of important inflammatory cytokines, modulate the response of inflammatory macrophages, and inhibit the activation of the NF-κB pathway. This confirmed the therapeutic potential of CeLutNCs in an ALI model (Gu et al., 2024). Luteolin alleviates ALI in a mouse model of CLP-induced sepsis through its antioxidant effects (Rungsung et al., 2018; Liu and Su, 2023). Elevated levels of TNF-α can increase free radicals (Cinar et al., 2019; Kutlu et al., 2020), and early studies have shown that luteolin can reduce TNF-α levels in sepsis and other inflammatory experimental models (Rungsung et al., 2018; Basu et al., 2016; Móritz et al., 2024). Celebi and colleagues found that luteolin mainly reduces the expression of TNF-α mRNA, decreases oxidative stress, and reduces the biochemical and histopathological damage occurring in lung tissue of CLP-induced septic rats, which is attributed to the antioxidant properties of luteolin. An increase in malondialdehyde (MDA) caused by free radicals formed during sepsis was normalized by luteolin treatment, which improved glutathione (GSH) levels, CAT, and superoxide dismutase (SOD) activity, and reduced MDA levels. Luteolin is a potent antioxidant even under intense free radical production conditions, such as sepsis (Rungsung et al., 2018; Xiong et al., 2017). Through the mechanisms above, luteolin can affect pulmonary oxidative stress and enhance the activity of antioxidant enzymes, including CAT, glutathione peroxidase, and superoxide dismutase, thereby counteracting lipid peroxidation.
The main characteristic of ALI/ARDS is pulmonary edema. Rungsung et al. treated mice with luteolin, which not only reduced the wet-to-dry weight ratio and protein concentration in the bronchoalveolar lavage fluid (BALF) but also alleviated lung edema in LPS-challenged ALI mice [77]. ENaC, located on the epithelial cell membrane, is a major participant in sodium transport across the alveoli and a primary determinant of alveolar fluid clearance (AFC). ENaC is crucial in effectively clearing excess alveolar edema fluid, which is vital for restoring gas exchange and reducing damage to peripheral tissues. Luteolin enhances alveolar sodium transport, which is reduced by LPS in H441 cell monolayers, strongly suggesting that luteolin increases sodium transport associated with ENaC activity. Inhibition of cyclic guanosine monophosphate (cGMP) or PI3K can counteract the luteolin-induced increase in ENaC protein expression in the plasma membrane, indicating that the regulation of ENaC by luteolin is related to the cGMP/PI3K pathway. The downstream signaling cascade of PI3K is associated with several effector proteins, including AKT, serum, and glucocorticoid-induced kinase (SGK), both of which can upregulate cellular ENaC by inhibiting the ubiquitination of Nedd4-2 (Hou et al., 2022; Lamothe and Zhang, 2013). Therefore, luteolin alleviates SALI/ARDS by activating alveolar ENaCs via the cGMP/PI3K pathway. Additionally, Chen et al., by knocking down STAT3, demonstrated that luteolin decreases phosphorylation in the JAK/STAT pathway while elevating SOCS3 levels, thereby eliminating the suppressive effect of LPS-induced ENaC expression, indicating that luteolin can alleviate ALI/ARDS by enhancing transepithelial sodium transport through the JAK/STAT pathway (Chen et al., 2023). In summary, luteolin can exert ameliorative effects on LPS-induced ALI through various pathways, such as acting as an anti-inflammatory drug (that inhibits the activity of Hsp90, reduces IL-10 levels, and inhibits NF-κB and MAPK pathways) and an antioxidant (that eliminates excess ROS and enhances antioxidant enzyme activity), as well as improving the function of the pulmonary alveolar ENaC (that activates alveolar ENaC and regulates JAK/STAT pathway) in LPS-induced ALI. Research on luteolin in the treatment of ALI/ARDS provides new insights into the pathophysiology of ALI/ARDS and offers a scientific basis for developing new therapeutic strategies. As our research progresses, we hope to identify luteolin as a novel treatment option for patients with ALI/ARDS.
Respiratory viruses are a substantial threat to human wellbeing, and respiratory syncytial virus (RSV) is the primary cause of respiratory infections. Studies by Weifeng Li et al. have shown that luteolin exerts therapeutic effects on RSV pneumonia through various mechanisms. Luteolin mitigates lung inflammation in mice, curbs RSV proliferation in the lungs, elevates pulmonary IFN-β and interferon-stimulated genes (ISG) expression, and modulates murine glucose metabolism. AMs constitute the chief producers of IFN-β in the lungs at the beginning of RSV infection, and the absence of AMs can exacerbate RSV-induced lung tissue damage. Luteolin predominantly targets AMs. By safeguarding the mitochondria in AMs and curbing oxidative stress within cells, luteolin can prevent RSV-induced apoptosis and stimulate IFN-β secretion by AMs (Li W. et al., 2024). Luteolin exerts anti-inflammatory effects on lung tissue in rat models of acute pneumonia. Results from the study by Kong et al. indicated that luteolin inhibits inflammation by suppressing cAMP-phosphodiesterase (cAMP-PDE) activity and the expression of microvascular endothelial cell adhesion molecules (Kong et al., 2019). S. aureus infections can range from mild skin infections to acute diseases such as pneumonia (David and Daum, 2010). Yuan et al. showed that the expression of genes involved in virulence and biofilm formation in S. aureus strains was downregulated after treatment with luteolin, confirming the potential anti-virulence effects of luteolin (Yuan et al., 2022). Additionally, Yuan et al. found that luteolin treatment mitigated the damage to human alveolar epithelial A549 cells caused by the wild-type (WT) strain and protected mice from pneumonia induced by the WT strain. They suggested that luteolin is a promising metabolite that can interfere with the agr system and can be developed as a novel therapeutic agent for S. aureus infections (Lv et al., 2023). Moreover, docking studies with methicillin-resistant Staphylococcus aureus (MRSA) strains showed that luteolin exhibits good pharmacokinetics, drug-likeness, and high binding energy with MRSA strains. Ligands with good binding energy values, pharmacokinetics, and drug-likeness have been proven to be potential ligands for treating MRSA infections, and luteolin may serve as a potential therapeutic inhibitor of pneumonia caused by MRSA (Nandhini et al., 2023). At the end of 2019, an outbreak of novel acute viral pneumonia caused by severe acute respiratory syndrome coronavirus 2 (SARS-CoV-2) triggered a severe medical and economic crisis. SARS-CoV-2 uses the receptor-binding domain (RBD) of its spike protein to bind to angiotensin-converting enzyme 2 (ACE2) in host cells (Zhu et al., 2023). Therefore, metabolites that bind to human ACE2 or the SARS-CoV-2 spike protein may block the SARS-CoV-2-ACE2 receptor interaction (Day et al., 2021). Luteolin has been shown to bind and significantly inhibit SARS-CoV infection (Stalin et al., 2022). Stalin et al. used computational simulations and virtual screening methods to demonstrate that luteolin engages in uniquely strong interactions with hotspots in the RBD of the SARS-CoV-2 RBD/ACE2 complex, inhibiting the infection of SARS-CoV-2 (Figure 5) (Stalin et al., 2022). In summary, luteolin and its derivatives demonstrate therapeutic potential through multiple mechanisms, such as inhibiting cAMP-PDE activity and the expression of adhesion molecules on microvascular endothelial cells, enhancing pulmonary IFN-β and ISG expression while regulating glucose metabolism in mice, protecting AMs mitochondria and suppressing intracellular oxidative stress to prevent RSV-induced cell apoptosis and stimulate AMs secretion of IFN-β, enhancing the blockade of the RBD/ACE2 complex to inhibit SARS-CoV-2 infection, and downregulating gene expression in S. aureus strains. These actions collectively contribute to anti-inflammatory effects, protection of AMs, inhibition of pathogen proliferation, metabolic regulation, and direct interference with virus-host cell receptor interactions, showing promise in treating respiratory diseases. This not only provides a scientific basis for the development of luteolin as a new type of anti-pneumonia drug but also deepens our understanding of the potential of plant-derived metabolites in modern medicine. We look forward to future clinical trials that can further verify these findings and explore new uses for luteolin in treating pneumonia.
Figure 5. An illustration of the effect of luteolin on the novel coronavirus, created using Biorender.com. The symbol “——|” represents inhibition, and “——>” represents promotion (SARS-CoV-2, Severe Acute Respiratory Syndrome Coronavirus 2; ROS, Reactive Oxygen Species; TNF-α, Tumor Necrosis Factor-alpha; IL-6, Interleukin-6; IL-10, Interleukin-10; IL-8, Interleukin-8; ACE2, Angiotensin-Converting Enzyme 2; TMPRSS2, Transmembrane Protease Serine 2).
Asthma is a common chronic airway disease characterized by airway narrowing, thickening of the airway walls, and increased airway mucus secretion, which leads to restricted airflow. The clinical features include dyspnea, wheezing, chest tightness, and cough. The prevalence and incidence of asthma has increased significantly. Asthma affected approximately 262 million people in 2019 and was responsible for 455,000 deaths that year. The asthma mortality rate is highest in low and middle sociodemographic index (SDI) countries, whereas its prevalence is highest in high-SDI countries (GBD 2019 Diseases and Injuries Collaborators, 2020). During the sensitization process and after sensitization in a model of ovalbumin (OVA)-sensitized airway hyperresponsive male BALB/c mice aged 8–9 weeks, the administration of luteolin (0.1 mg/kg body weight led to a marked reduction in OVA-induced constriction of the airways and bronchial hyperreactivity, in contrast to the control group. Luteolin also reduced the levels of OVA-specific IgE in the serum, increased the levels of interferon-gamma (IFN-γ), and decreased the levels of interleukin-4 (IL-4) and interleukin-5 (IL-5) in the BALF, attenuating the asthmatic characteristics of the experimental mice (Das et al., 2003). Luteolin alleviates bronchoconstriction and airway hyperresponsiveness in OVA-sensitized mice. Luteolin exerts anti-asthmatic activity against OVA-induced pulmonary inflammation by downregulating the transcription of CD4+ T cells with Th2 phenotype cytokines and inhibiting the production of Prostaglandin E2 (PGE2) (Jin et al., 2009). Luteolin attenuates the immediate and late asthmatic responses to nebulized OVA exposure in conscious guinea pigs. Compared to the control group, luteolin, and apigenin significantly reduced specific airway resistance in both immediate and late asthmatic responses. They also reduced the recruitment of leukocytes and release of histamines, as well as the activity of phospholipase A2 (PLA2) and eosinophil peroxidase (EPO) in bronchoalveolar lavage fluid (BALF). Still, the anti-asthmatic activity of luteolin was lower than that of sodium cromoglycate and dexamethasone (Lee et al., 2014). Therefore, luteolin can be used not only as a lead molecule for identifying effective anti-asthmatic therapies but also as a means of identifying novel anti-asthmatic targets (Figure 6). In addition, luteolin reduces autophagy in allergic asthma. They observed that luteolin treatment significantly inhibited OVA-induced inflammatory responses and autophagy in the lung tissue. Moreover, luteolin activated the PI3K/Akt/mTOR pathway in the lung tissues of asthmatic mice and inhibited the Beclin-1-PI3KC3 protein complex. This strategy encompasses the prevention of autophagic processes in allergic asthma via upregulation of the PI3K/Akt/mTOR pathway and downregulation of the Beclin-1-PI3KC3 complex (Wang S. et al., 2021). Asthma often manifests as an excessive buildup of mucus in the airway lining, which can lead to severe clinical outcomes. Although general asthma therapies are effective, specialized treatments designed to limit mucus overproduction in patients with asthma are still lacking. Recent studies have revealed that stimulating the gamma-aminobutyric acid type A receptor (GABAAR) is crucial for promoting excessive mucus secretion by pulmonary airway epithelial cells. Shen and colleagues found that in an OVA-induced asthma mouse model, with a 10 mg/kg administration, luteolin markedly reduced the number of goblet cells within pulmonary tissue and suppressed excessive mucus production. Luteolin hindered GABAAR-induced currents in A549 cells, according to patch-clamp data. Additionally, the suppressive effects of luteolin on goblet cell hyperplasia and excessive mucus secretion induced by OVA are reversed by picrotoxin, a GABAAR antagonist (Shen et al., 2016). In summary, luteolin and its derivatives have demonstrated potential in treating asthma by modulating various cellular signaling pathways and immune responses. These include the activation of the PI3K/Akt/mTOR pathway and inhibition of the Beclin-1-PI3KC3 protein complex. The therapeutic potential is reflected in mechanisms such as reducing airway inflammation, decreasing airway hyperresponsiveness, modulating immune cytokines, reducing eosinophil infiltration, inhibiting autophagy, and suppressing excessive mucus secretion. After evaluating the potential impact of luteolin on asthma, we believe that this metabolite shows promise for improving asthma symptoms by modulating various cellular signaling pathways and immune responses. Nonetheless, we should recognize the complexity of asthma, which necessitates further research to determine the optimal dose of luteolin for asthma treatment. We are confident that with a deeper understanding of the mechanisms of action of luteolin, it could become an important metabolite in future asthma treatment strategies.
Figure 6. An illustration of effects by which luteolin affects asthma, created using Biorender.com. The symbol “↓” indicates downregulation, and “↑” indicates upregulation (mTOR, mammalian Target of Rapamycin; PLA2, Phospholipase A2; GABAAR, γ-Aminobutyric acid sub-type A receptors).
COPD is one of the leading causes of morbidity and mortality worldwide (Buhl et al., 2024) and poses a significant economic and social burden (Alupo et al., 2024). Patients experience progressive deterioration until end-stage COPD, which is characterized by severe airflow limitations, severely compromised lung function, chronic respiratory failure, advanced age, multiple comorbidities, and severe systemic manifestations/complications. COPD is often underdiagnosed and undertreated (Viegi et al., 2007). Intensified oxidative stress and body-wide inflammation are critical elements of the complex pathophysiology of COPD (Austin et al., 2016). Studies have shown that matrix metalloproteinases (MMPs) are associated with COPD progression, and luteolin has been identified as an inhibitor of MMP-2 and MMP-9 (Ende and Gebhardt, 2004). Therefore, Yaghi et al. explored the use of luteolin in their study, measuring ciliary beat frequency (CBF) in nasal cilia from COPD patients as an important indicator of ciliary function in COPD. Compared to the high-risk and control groups, CBF was significantly reduced in individuals with moderate-to-severe COPD. The treatment group observed a significant and sustained increase in CBF in COPD cilia tested with luteolin, increasing the CBF of cilia in patients with COPD, favorably enhancing mucociliary clearance, reducing retention of secretions, and decreasing infections (Yaghi et al., 2012). Li et al. proposed that luteolin inhibits inflammation and oxidative stress in COPD by suppressing the NADPH oxidase 4 (NOX4)-mediated NF-κB signaling pathway. Studies have used cigarette smoke (CS) to induce COPD in mice or A549 cells both in vivo and in vitro. In vivo, luteolin mitigated the inhibitory effects of CS on body weight and the activities of SOD and CAT in COPD model mice and reduced the levels of MDA. Similar results have been obtained in vitro. Li et al. transfected A549 cells with a NOX4 overexpression plasmid to induce NOX4 overexpression. Compared to the control group, the overexpression of NOX4 reversed the downregulation of the ratio of p-p65/p65 and p-IκB/IκB in the luteolin group, upregulation of oxidative stress-related proteins, and reduction of inflammatory-related factors. Luteolin alleviates inflammation, oxidative stress, and activation of the NOX4-mediated NF-κB signaling pathway induced by CS in A549 cells (Li M. et al., 2023). Zhou and colleagues proposed that luteolin alleviates oxidative stress induced by CS in COPD by modulating the transient receptor potential vanilloid type 1 (TRPV1) and cytochrome P450 family 2 subfamily A member 13 (CYP2A13)/nuclear factor erythroid 2-related factor 2 (NRF2) signaling pathways. They observed that luteolin downregulated the expression of TRPV1 and CYP2A13 proteins and upregulated the expression of sirtuin 6 (SIRT6) and NRF2 proteins in CS + LPS-induced COPD mice and A549 cells treated with CS. CS treatment increased intracellular calcium ions, overall ROS, and mitochondria-derived ROS levels in A549 cells. Importantly, luteolin inhibited the influx of Ca2+ into CS-treated A549 cells and mitigated the excessive production of mitochondrial and intracellular ROS. By modulating the TRPV1/SIRT6 and CYP2A13/NRF2 signaling pathways, luteolin exhibits a protective effect by reducing oxidative stress and inflammation in COPD mice induced by CS-and LPS, as well as in A549 cells exposed to CS (Figure 7) (Zhou et al., 2023). In summary, luteolin has the potential to alleviate inflammation and oxidative stress and improve cilia function in COPD by inhibiting NOX4-mediated NF-κB signaling pathway, regulating TRPV1 and CYP2A13/NRF2 signaling pathways, and modulating various signaling pathways and cytokines. However, we are also aware that COPD is a multifactorial disease requiring a comprehensive treatment strategy. Therefore, future research should explore the combined application of luteolin and other drugs to manage COPD effectively.
Figure 7. An illustration of the protective effect of luteolin in CS-induced COPD, created using Biorender.com. The symbol “——|” represents inhibition, and “——>” represents promotion (CS, Cigarette Smoke; NOX4, NADPH oxidase 4; Ca+, Calcium ions; CYP2A13, Cytochrome P450 family 2 subfamily A member 13; Keap1, Kelch-like ECH-associated protein 1; NF-KB, Nuclear factor kappa B; ROS, Reactive Oxygen Species; SIRT6, Sirtuin 6; Nrf2, Nuclear factor erythroid 2-related factor 2; NLRP3, NLR family pyrin domain containing 3; IL-1β, Interleukin-1 beta; IL-18, Interleukin-18; PGC1α, Peroxisome proliferator-activated receptor gamma coactivator 1-alpha; SOD2, Superoxide dismutase 2; SOD1, Superoxide dismutase 1).
Idiopathic pulmonary fibrosis (IPF), classified as progressive fibrosing interstitial lung disease (ILD), leads to a gradual decline in lung function (Raghu et al., 2018). The hallmarks of IPF include a progressive deterioration in lung capacity, exacerbation of fibrosis as depicted on high-resolution computed tomography (HRCT) scans, decline in both symptomatology and quality of life for patients, and a propensity for early death (Travis et al., 2013; Wells et al., 2018; Cottin et al., 2019; Kolb and Vašáková, 2019). Aside from pirfenidone and nintedanib, which are specifically used for treating IPF, no other medications have been approved for treating ILDs (Brown et al., 2020). The predominant pharmacological approach for treating other progressive fibrosing ILDs involves immunosuppression (Cottin et al., 2019). When the fibrotic response of an ILD to lung injury advances to a stage where fibrosis becomes self-perpetuating and continues to progress, targeted anti-fibrotic therapy is essential to mitigate the rate of disease progression. Clinical studies have demonstrated that natural substances extracted from plants possess potent antifibrotic properties and can alleviate dyspnea, a common and debilitating symptom experienced by patients with ILD (Chang et al., 2021). Luteolin is an active flavonoid metabolite isolated from Lonicera japonica and is known for its broad range of biological activities, particularly its antioxidant and anti-inflammatory effects. Ren and colleagues loaded luteolin into γ-cyclodextrin metal-organic frameworks (CD-MOFs) for pulmonary drug delivery using a dry powder inhaler. In vitro lung deposition results showed that luteolin effectively inhibited the progression of ILD in bleomycin (BLM)-induced fibrotic ILD rat model. Compared to the control group, treatment with luteolin significantly protected rats from BLM-induced lung injury. They demonstrated that luteolin possesses potent anti-inflammatory activity and that inhalation of luteolin could be considered a promising new strategy for treating fibrotic ILD (Ren et al., 2023). Oral administration of luteolin (10 mg/kg) in both the early and delayed luteolin treatment groups effectively inhibited the infiltration of neutrophils into BALF and increased TNF-R and IL-6 levels in C57BL/6 mice intubated with BLM. Luteolin also mitigated collagen deposition, TGF-β1 expression, and pulmonary fibrosis following BLM injection. Subsequently, in vitro studies showed that luteolin inhibited the expression of TGF-β1-induced reactive serum myoglobin (R-SMA), type I collagen, and vimentin in primary cultured mouse lung fibroblasts. Luteolin significantly blocked the downregulation of epithelial markers (E-cadherin) and upregulation of mesenchymal cell markers (fibronectin and vimentin) mediated by TGF-β1 and maintained the epithelial morphology of human alveolar epithelial-derived A549 cells. This indicates that luteolin exerts effective antifibrotic activity by inhibiting pulmonary inflammation and suppressing myofibroblast differentiation and the epithelial-mesenchymal transition (EMT) of cells (Chen et al., 2010). Data analysis in informatics implied that luteolin may offer substantial therapeutic benefits in treating IPF and gastroesophageal reflux disease (GERD). By identifying and analyzing differentially expressed genes (DEGs) between IPF and GERD and predicting drug molecules for IPF and GERD, luteolin was recognized as a potential biomarker and promising therapeutic target for both IPF and GERD (Table 2) (Shen et al., 2016). In summary, luteolin demonstrates potential in the treatment of IPF through various mechanisms, including anti-inflammatory effects, anti-fibrotic actions (inhibition of Smad3 phosphorylation in the TGF-β1 signaling pathway), inhibition of myofibroblast differentiation, suppression of EMT, modulation of key signaling pathways, and potential as a drug delivery vehicle. After analyzing the potential applications of luteolin in treating pulmonary fibrosis, we were encouraged by the antifibrotic potential of the metabolite. We believe that luteolin’s anti-inflammatory and anti-fibrotic properties and its role in modulating key signaling pathways make it a strong candidate for treating pulmonary fibrosis. Future studies should focus on determining the optimal route of administration and luteolin dosage to maximize its therapeutic effects.
The GLOBOCAN 2020 estimates from the International Agency for Research on Cancer (IARC) regarding cancer incidence and mortality rates show that carcinoma of the lung stands as the most prevalent cause of death from cancer, with projections indicating approximately 2.2 million new instances and 1.8 million deaths worldwide (Sung et al., 2021). In China, the primary treatment options for lung cancer include surgical intervention, radiation treatment, chemotherapy, precision cancer therapies, immune system treatments, and traditional Chinese medicine (Yang et al., 2018; Chaft et al., 2016). Recent reports suggest that luteolin may be a novel and potent agent against lung cancer with the potential to inhibit disease progression (Imran et al., 2019).
KRAS mutations are known to increase the expression of programmed death-ligand 1 (PD-L1) within tumor cells, enabling the tumor to shun the immune system for detection and subsequent destruction. An in vitro experiment demonstrated that luteolin inhibits the growth of KRAS-mutated cells in a dose-dependent manner. The expression of PD-L1 is a consequence of STAT3 activity, which engages the PD-L1 gene promoter upon phosphorylation. Luteolin inhibits the STAT3 signaling pathway and the expression of PD-L1, thereby exposing tumor cells to T cells of the immune system (Jiang et al., 2021; Watterson and Coelho, 2023). MiR-34a is commonly downregulated in cancer cells, and elevated levels of miR-34a have been shown to suppress tumor growth (Zhang et al., 2019; Fu et al., 2023). Research has indicated that luteolin upregulates the expression of miR-34a, which may subsequently inhibit the proliferation of non-small cell lung cancer (NSCLC) cells (Shi et al., 2014; Jiang et al., 2018). Circular RNAs (circRNAs) are upregulated in lung cancer tissues, and luteolin suppresses lung cancer progression by targeting the circ_0000190/miR-130a-3p/notch-1 signaling pathway (Zheng et al., 2023). Luteolin may inhibit the proliferation and migration of A549 cells by reducing the expression of AR and modulating the phosphorylation of AR receptor sites (Li X. et al., 2023).
The anticancer properties of luteolin have also been demonstrated through the induction of apoptosis in neoplastic cells (Çetinkaya and Baran, 2023). Luteolin induces mitochondria-dependent apoptosis in human lung adenocarcinoma cells (Chen et al., 2012). Luteolin promotes the activation of caspase-9 and caspase-3, decreases the expression of Bcl-2, increases the expression of BAX, increases the BAX/Bcl-2 ratio, and induces apoptosis by activating intrinsic pathways (Chen et al., 2012). Additionally, the accumulation of ROS induced by luteolin can enliven the extrinsic pathways modeled by TNF-induced signaling (Ju et al., 2007). Luteolin has been demonstrated to inhibit EMT and downregulate E-cadherin by suppressing the triggering of key signaling pathways, such as TGF-β1 and the PI3K/AKT-NF-κB-Snail pathways (Chen et al., 2013). Furthermore, Masraksa and colleagues have demonstrated that luteolin can hinder the spread and invasion of lung cancer cells by targeting the signaling pathways of focal adhesion kinase and non-receptor tyrosine kinase (Src/FAK) as well as its downstream pathways involving Ras-related C3 botulinum toxin substrate 1 (Rac1), cell division cycle 42 (Cdc42), and ras homologous gene family member A (RhoA) (Masraksa et al., 2020). Luteolin works synergistically with osimertinib, a third-generation epidermal growth factor receptor tyrosine kinase inhibitor (EGFR-TKI), to overcome acquired resistance in NSCLC cells by suppressing the hepatocyte growth factor (HGF)-MET-Akt signaling cascade, which is involved in MET amplification and hyperactivation (Huang G. et al., 2023). When used alone or in combination with factor-related apoptosis-inducing ligand (TRAIL), a novel and potent anticancer agent, can enhance the expression of death receptor 5 (DR5) and promote dynamin-related protein 1 (Drp1)-dependent mitochondrial fission. By increasing DR5 expression and Drp1-mediated mitochondrial fission, luteolin enhances the sensitivity of NSCLC cells to TRAIL (Wu et al., 2020). Another study indicated that LIM Kinase 1 (LIMK1) is a target of luteolin, which suppresses tumor growth by inhibiting LIMK1 activity (Zhang M. et al., 2021). Beyond its anticancer characteristics, luteolin also possesses the ability to alleviate bone cancer pain (Zhou et al., 2022). Luteolin involves numerous determinants and cascades related to lung cancer progression (Rakoczy et al., 2023). In summary, luteolin has shown potential as a therapeutic agent for lung cancer by affecting multiple key factors in disease progression, and further research into its clinical applications is warranted (Table 3). Luteolin has shown the potential to inhibit lung cancer progression by affecting various key tumor biological pathways. We believe that these mechanisms of action provide a scientific basis for luteolin as a candidate drug for lung cancer treatment. Future research should focus on clinical trials of luteolin and optimizing its efficacy through drug delivery systems.
The multifaceted pharmacological profile of luteolin makes it a potential candidate for further investigation as a natural therapeutic agent for the treatment of pulmonary diseases. However, poor solubility in water and low bioavailability hinder their therapeutic potential. This article emphasizes that strategies to enhance water solubility and bioavailability through chemical modifications and advanced pharmaceutical formulations such as nanovesicles and cyclodextrin complexes hold promise for addressing the challenges associated with luteolin. Balancing the concentration of luteolin, determining the minimum toxic dose, and addressing interference effects in broad-spectrum assays are also issues that deserve attention. While preclinical studies in cellular and animal models are promising, and numerous studies indicate that luteolin has minimal toxicity, this article emphasizes the need for further research, particularly clinical trials in humans and rigorous toxicity experiments that are still required to assess its safety and promote its rational development, to fully understand the efficacy and safety of luteolin in treating pulmonary diseases. Owing to the structural diversity of flavonoids in plants and the inherent limitations of existing technologies, industrial-scale production of flavonoids faces challenges that necessitate the improvement and optimization of current extraction techniques (Li L. et al., 2024). In this context, enhancing the purification efficiency and recovery rate of luteolin is a critical step that is essential for the large-scale production and widespread application of luteolin-based pharmaceuticals. Although there are already commercial luteolin health foods and cosmetic products on the market (Wang Z. et al., 2021), the use of luteolin as a drug for the treatment of other diseases is still in the clinical trial phase (Shi et al., 2024; Di Stadio et al., 2022; Cordaro et al., 2020). Future research should focus on two key areas: optimizing the solvent extraction technology for luteolin and developing advanced pharmaceutical formulations that can improve its solubility and bioavailability. These two tasks are of great significance in overcoming the limitations of luteolin in practical applications, fully exerting its therapeutic effects, and promoting its development for clinical applications.
In this article, we reviewed the therapeutic potential and molecular mechanisms of action of luteolin in various pulmonary diseases. Luteolin has shown significant effects in the treatment of ALI/ARDS, COPD, asthma, pulmonary fibrosis, and lung cancer, owing to its pharmacological actions such as anti-cancer, antioxidant, and anti-inflammatory properties. Despite its notable bioactivity, water-solubility and bioavailability of luteolin limit its clinical applications. These limitations can be overcome through chemical structural modifications and pharmaceutical formulation innovations. This article summarizes the research progress in improving the solubility and bioavailability of luteolin, discusses its multifaceted therapeutic effects in the treatment of pulmonary diseases and provides new directions for future research.
JL: Data curation, Formal Analysis, Project administration, Resources, Supervision, Validation, Writing–original draft. XS: Conceptualization, Methodology, Software, Visualization, Writing–original draft. ZL: Data curation, Formal Analysis, Writing–original draft. DH: Software, Visualization, Writing–original draft. LX: Software, Visualization, Writing–original draft. KZ: Conceptualization, Methodology, Writing–review and editing.
The author(s) declare that financial support was received for the research, authorship, and/or publication of this article. This work was supported by the Ganzhou Science and Technology Bureau, China (2023LNS36644). Jiangxi Provincial Health Commission, China (202410348). Jiangxi Provincial Administration of Traditional Chinese Medicine, China (2024B0031).
The authors thank Editage (www.editage.cn) for English language editing. The figures were created with BioRender.com.
The authors declare that the research was conducted in the absence of any commercial or financial relationships that could be construed as a potential conflict of interest.
The author(s) declare that no Generative AI was used in the creation of this manuscript.
All claims expressed in this article are solely those of the authors and do not necessarily represent those of their affiliated organizations, or those of the publisher, the editors and the reviewers. Any product that may be evaluated in this article, or claim that may be made by its manufacturer, is not guaranteed or endorsed by the publisher.
The Supplementary Material for this article can be found online at: https://www.frontiersin.org/articles/10.3389/fphar.2025.1535555/full#supplementary-material
GBD 2019 Diseases and Injuries Collaborators (2020). Global burden of 369 diseases and injuries in 204 countries and territories, 1990-2019: a systematic analysis for the Global Burden of Disease Study 2019. Lancet 396 (10258), 1204–1222. doi:10.1016/S0140-6736(20)30925-9
Ahmad, S., Zahiruddin, S., Parveen, B., Basist, P., Parveen, A., Gaurav, A., et al. (2020). Indian medicinal plants and formulations and their potential against COVID-19-preclinical and clinical research. Front. Pharmacol. 11, 578970. doi:10.3389/fphar.2020.578970
Ali, F., and Siddique, Y. H. (2019). Bioavailability and pharmaco-therapeutic potential of luteolin in overcoming alzheimer's disease. CNS Neurol. Disord. Drug Targets 18 (5), 352–365. doi:10.2174/1871527318666190319141835
Almatroodi, S. A., Almatroudi, A., Alharbi, H. O. A., Khan, A. A., and Rahmani, A. H. (2024). Effects and mechanisms of luteolin, a plant-based flavonoid, in the prevention of cancers via modulation of inflammation and cell signaling molecules. Molecules 29 (5), 1093. doi:10.3390/molecules29051093
Alupo, P., Baluku, J., Bongomin, F., Siddharthan, T., Katagira, W., Ddungu, A., et al. (2024). Overcoming challenges of managing chronic obstructive pulmonary disease in low- and middle-income countries. Expert Rev. Respir. Med. 18, 873–882. doi:10.1080/17476348.2024.2398639
Alzaabi, M. M., Hamdy, R., Ashmawy, N. S., Hamoda, A. M., Alkhayat, F., Khademi, N. N., et al. (2022). Flavonoids are promising safe therapy against COVID-19. Phytochemistry Rev. Proc. Phytochemical Soc. Eur. 21 (1), 291–312. doi:10.1007/s11101-021-09759-z
Ashaari, Z., Hadjzadeh, M. A., Hassanzadeh, G., Alizamir, T., Yousefi, B., Keshavarzi, Z., et al. (2018). The flavone luteolin improves central nervous system disorders by different mechanisms: a review. J. Mol. Neurosci. 65 (4), 491–506. doi:10.1007/s12031-018-1094-2
Attiq, A., Jalil, J., Husain, K., Mohamad, H. F., and Ahmad, A. (2021). Luteolin and apigenin derived glycosides from Alphonsea elliptica abrogate LPS-induced inflammatory responses in human plasma. J. Ethnopharmacol. 275, 114120. doi:10.1016/j.jep.2021.114120
Austin, V., Crack, P. J., Bozinovski, S., Miller, A. A., and Vlahos, R. (2016). COPD and stroke: are systemic inflammation and oxidative stress the missing links? Clin. Sci. (Lond). 130 (13), 1039–1050. doi:10.1042/CS20160043
Aziz, N., Kim, M. Y., and Cho, J. Y. (2018). Anti-inflammatory effects of luteolin: a review of in vitro, in vivo, and in silico studies. J. Ethnopharmacol. 225, 342–358. doi:10.1016/j.jep.2018.05.019
Bandyopadhyay, D., and Mirsaeidi, M. S. (2023). Sarcoidosis-associated pulmonary fibrosis: joining the dots. Eur. Respir. Rev. 32 (169), 230085. doi:10.1183/16000617.0085-2023
Basu, A., Das, A. S., Majumder, M., and Mukhopadhyay, R. (2016). Antiatherogenic roles of dietary flavonoids chrysin, quercetin, and luteolin. J. Cardiovasc Pharmacol. 68 (1), 89–96. doi:10.1097/FJC.0000000000000380
Boersma, M. G., van der Woude, H., Bogaards, J., Boeren, S., Vervoort, J., Cnubben, N. H., et al. (2002). Regioselectivity of phase II metabolism of luteolin and quercetin by UDP-glucuronosyl transferases. Chem. Res. Toxicol. 15 (5), 662–670. doi:10.1021/tx0101705
Bravo, L. (1998). Polyphenols: chemistry, dietary sources, metabolism, and nutritional significance. Nutr. Rev. 56 (11), 317–333. doi:10.1111/j.1753-4887.1998.tb01670.x
Brown, K. K., Martinez, F. J., Walsh, S. L. F., Thannickal, V. J., Prasse, A., Schlenker-Herceg, R., et al. (2020). The natural history of progressive fibrosing interstitial lung diseases. Eur. Respir. J. 55 (6), 2000085. doi:10.1183/13993003.00085-2020
Buhl, R., Miravitlles, M., Anzueto, A., and Brunton, S. (2024). Long-acting muscarinic antagonist and long-acting β(2)-agonist combination for the treatment of maintenance therapy-naïve patients with chronic obstructive pulmonary disease: a narrative review. Ther. Adv. Respir. Dis. 18, 17534666241279115. doi:10.1177/17534666241279115
Cavaillon, J.-M., and Chrétien, F. (2019). From septicemia to sepsis 3.0—from ignaz semmelweis to louis pasteur. Genes and Immun. 20 (5), 371–382. doi:10.1038/s41435-019-0063-2
Cecconi, M., Evans, L., Levy, M., and Rhodes, A. (2018). Sepsis and septic shock. Lancet 392 (10141), 75–87. doi:10.1016/S0140-6736(18)30696-2
Çetinkaya, M., and Baran, Y. (2023). Therapeutic potential of luteolin on cancer. Vaccines (Basel) 11 (3), 554. doi:10.3390/vaccines11030554
Chaft, J. E., Dunphy, M., Naidoo, J., Travis, W. D., Hellmann, M., Woo, K., et al. (2016). Adaptive neoadjuvant chemotherapy guided by (18)F-fdg PET in resectable non-small cell lung cancers: the NEOSCAN trial. J. Thorac. Oncol. 11 (4), 537–544. doi:10.1016/j.jtho.2015.12.104
Chang, R. Y. K., Chow, M. Y. T., Khanal, D., Chen, D., and Chan, H. K. (2021). Dry powder pharmaceutical biologics for inhalation therapy. Adv. Drug Deliv. Rev. 172, 64–79. doi:10.1016/j.addr.2021.02.017
Chen, C. Y., Peng, W. H., Wu, L. C., Wu, C. C., and Hsu, S. L. (2010). Luteolin ameliorates experimental lung fibrosis both in vivo and in vitro: implications for therapy of lung fibrosis. J. Agric. food Chem. 58 (22), 11653–11661. doi:10.1021/jf1031668
Chen, D., Bi, A., Dong, X., Jiang, Y., Rui, B., Liu, J., et al. (2014). Luteolin exhibits anti-inflammatory effects by blocking the activity of heat shock protein 90 in macrophages. Biochem. biophysical Res. Commun. 443 (1), 326–332. doi:10.1016/j.bbrc.2013.11.122
Chen, K. C., Chen, C. Y., Lin, C. R., Yang, T. Y., Chen, T. H., Wu, L. C., et al. (2013). Luteolin attenuates TGF-β1-induced epithelial-mesenchymal transition of lung cancer cells by interfering in the PI3K/Akt-NF-κB-Snail pathway. Life Sci. 93 (24), 924–933. doi:10.1016/j.lfs.2013.10.004
Chen, L., Yu, T., Zhai, Y., Nie, H., Li, X., and Ding, Y. (2023). Luteolin enhances transepithelial sodium transport in the lung alveolar model: integrating network pharmacology and mechanism study. Int. J. Mol. Sci. 24 (12), 10122. doi:10.3390/ijms241210122
Chen, Q., Liu, S., Chen, J., Zhang, Q., Lin, S., Chen, Z., et al. (2012). Luteolin induces mitochondria-dependent apoptosis in human lung adenocarcinoma cell. Nat. Prod. Commun. 7 (1), 29–32. doi:10.1177/1934578x1200700111
Chen, S., and Li, Y. (2024). Global health inequalities in the burden of interstitial lung disease and pulmonary sarcoidosis from 1990 to 2021. BMC Public Health 24 (1), 2892. doi:10.1186/s12889-024-20430-y
Chen, Z., Chen, M., Pan, H., Sun, S., Li, L., Zeng, S., et al. (2011). Role of catechol-O-methyltransferase in the disposition of luteolin in rats. Drug Metab. Dispos. 39 (4), 667–674. doi:10.1124/dmd.110.037333
Choi, J. H., Kim, Y. S., Shin, C. H., Lee, H. J., and Kim, S. (2015). Antithrombotic activities of luteolin in vitro and in vivo. J. Biochem. Mol. Toxicol. 29 (12), 552–558. doi:10.1002/jbt.21726
Cinar, I., Sirin, B., Aydin, P., Toktay, E., Cadirci, E., Halici, I., et al. (2019). Ameliorative effect of gossypin against acute lung injury in experimental sepsis model of rats. Life Sci. 221, 327–334. doi:10.1016/j.lfs.2019.02.039
Cocuron, J. C., Casas, M. I., Yang, F., Grotewold, E., and Alonso, A. P. (2019). Beyond the wall: high-throughput quantification of plant soluble and cell-wall bound phenolics by liquid chromatography tandem mass spectrometry. J. Chromatogr. A 1589, 93–104. doi:10.1016/j.chroma.2018.12.059
Conti, P., Caraffa, A., Gallenga, C. E., Ross, R., Kritas, S. K., Frydas, I., et al. (2021). Powerful anti-inflammatory action of luteolin: potential increase with IL-38. Biofactors 47 (2), 165–169. doi:10.1002/biof.1718
Cordaro, M., Cuzzocrea, S., and Crupi, R. (2020). An update of palmitoylethanolamide and luteolin effects in preclinical and clinical studies of neuroinflammatory events. Antioxidants Basel, Switz. 9 (3), 216. doi:10.3390/antiox9030216
Cottin, V., Wollin, L., Fischer, A., Quaresma, M., Stowasser, S., and Harari, S. (2019). Fibrosing interstitial lung diseases: knowns and unknowns. Eur. Respir. Rev. 28 (151), 180100. doi:10.1183/16000617.0100-2018
Daily, J. W., Kang, S., and Park, S. (2021). Protection against Alzheimer's disease by luteolin: role of brain glucose regulation, anti-inflammatory activity, and the gut microbiota-liver-brain axis. Biofactors 47 (2), 218–231. doi:10.1002/biof.1703
Das, M., Ram, A., and Ghosh, B. (2003). Luteolin alleviates bronchoconstriction and airway hyperreactivity in ovalbumin sensitized mice. Inflamm. Res. 52 (3), 101–106. doi:10.1007/s000110300021
David, M. Z., and Daum, R. S. (2010). Community-associated methicillin-resistant Staphylococcus aureus: epidemiology and clinical consequences of an emerging epidemic. Clin. Microbiol. Rev. 23 (3), 616–687. doi:10.1128/CMR.00081-09
Day, C. J., Bailly, B., Guillon, P., Dirr, L., Jen, F. E., Spillings, B. L., et al. (2021). Multidisciplinary approaches identify compounds that bind to human ACE2 or SARS-CoV-2 spike protein as candidates to block SARS-CoV-2-ACE2 receptor interactions. mBio 12 (2), e03681. doi:10.1128/mBio.03681-20
Deng, C., Gao, C., Tian, X., Chao, B., Wang, F., Zhang, Y., et al. (2017). Pharmacokinetics, tissue distribution and excretion of luteolin and its major metabolites in rats: metabolites predominate in blood, tissues and are mainly excreted via bile. J. Funct. Foods 35, 332–340. doi:10.1016/j.jff.2017.05.056
Diniz, L. R. L., Elshabrawy, H. A., Souza, M. T. S., Duarte, A. B. S., Madhav, N., and de Sousa, D. P. (2022). Renoprotective effects of luteolin: therapeutic potential for COVID-19-associated acute kidney injuries. Biomolecules 12 (11), 1544. doi:10.3390/biom12111544
Di Stadio, A., D'Ascanio, L., Vaira, L. A., Cantone, E., De Luca, P., Cingolani, C., et al. (2022). Ultramicronized palmitoylethanolamide and luteolin supplement combined with olfactory training to treat post-COVID-19 olfactory impairment: a multi-center double-blinded randomized placebo- controlled clinical trial. Curr. Neuropharmacol. 20 (10), 2001–2012. doi:10.2174/1570159X20666220420113513
Domitrović, R., Jakovac, H., Tomac, J., and Sain, I. (2009). Liver fibrosis in mice induced by carbon tetrachloride and its reversion by luteolin. Toxicol. Appl. Pharmacol. 241 (3), 311–321. doi:10.1016/j.taap.2009.09.001
Dorababu, A., and Maraswami, M. (2023). Recent advances (2015-2020) in drug discovery for attenuation of pulmonary fibrosis and COPD. Molecules 28 (9), 3674. doi:10.3390/molecules28093674
El-Shiekh, R. A., Elhemely, M. A., Naguib, I. A., Bukhari, S. I., and Elshimy, R. (2023). Luteolin 4'-neohesperidoside inhibits clinically isolated resistant bacteria in vitro and in vivo. Molecules 28 (6), 2609. doi:10.3390/molecules28062609
Ende, C., and Gebhardt, R. (2004). Inhibition of matrix metalloproteinase-2 and -9 activities by selected flavonoids. Planta Med. 70 (10), 1006–1008. doi:10.1055/s-2004-832630
Evans, L., Rhodes, A., Alhazzani, W., Antonelli, M., Coopersmith, C. M., French, C., et al. (2021). Surviving sepsis campaign: international guidelines for management of sepsis and septic shock 2021. Intensive Care Med. 47 (11), 1181–1247. doi:10.1007/s00134-021-06506-y
Fan, E. K. Y., and Fan, J. (2018). Regulation of alveolar macrophage death in acute lung inflammation. Respir. Res. 19 (1), 50. doi:10.1186/s12931-018-0756-5
Faniyi, A. A., Hughes, M. J., Scott, A., Belchamber, K. B. R., and Sapey, E. (2022). Inflammation, ageing and diseases of the lung: potential therapeutic strategies from shared biological pathways. Br. J. Pharmacol. 179 (9), 1790–1807. doi:10.1111/bph.15759
Fielding-Singh, V., Matthay, M. A., and Calfee, C. S. (2018). Beyond low tidal volume ventilation: treatment adjuncts for severe respiratory failure in acute respiratory distress syndrome. Crit. Care Med. 46 (11), 1820–1831. doi:10.1097/CCM.0000000000003406
Fu, J., Imani, S., Wu, M. Y., and Wu, R. C. (2023). MicroRNA-34 family in cancers: role, mechanism, and therapeutic potential. Cancers 15 (19), 4723. doi:10.3390/cancers15194723
Gadotti, V. M., and Zamponi, G. W. (2019). Anxiolytic effects of the flavonoid luteolin in a mouse model of acute colitis. Mol. Brain 12 (1), 114. doi:10.1186/s13041-019-0539-z
Galati, G., and O'Brien, P. J. (2004). Potential toxicity of flavonoids and other dietary phenolics: significance for their chemopreventive and anticancer properties. Free Radic. Biol. Med. 37 (3), 287–303. doi:10.1016/j.freeradbiomed.2004.04.034
Ganai, S. A., Sheikh, F. A., Baba, Z. A., Mir, M. A., Mantoo, M. A., and Yatoo, M. A. (2021). Anticancer activity of the plant flavonoid luteolin against preclinical models of various cancers and insights on different signalling mechanisms modulated. Phytother. Res. 35 (7), 3509–3532. doi:10.1002/ptr.7044
Gao, H. L., Yu, X. J., Feng, Y. Q., Yang, Y., Hu, H. B., Zhao, Y. Y., et al. (2023). Luteolin attenuates hypertension via inhibiting NF-κB-Mediated inflammation and PI3K/akt signaling pathway in the hypothalamic paraventricular nucleus. Nutrients 15 (3), 502. doi:10.3390/nu15030502
Gbdcrd, C. (2023). Global burden of chronic respiratory diseases and risk factors, 1990-2019: an update from the Global Burden of Disease Study 2019. EClinicalMedicine 59, 101936. doi:10.1016/j.eclinm.2023.101936
Gelabert-Rebato, M., Wiebe, J. C., Martin-Rincon, M., Galvan-Alvarez, V., Curtelin, D., Perez-Valera, M., et al. (2019). Enhancement of exercise performance by 48 hours, and 15-day supplementation with mangiferin and luteolin in men. Nutrients 11 (2), 344. doi:10.3390/nu11020344
Gu, J., Zhang, P., Li, H., Wang, Y., Huang, Y., Fan, L., et al. (2024). Cerium-luteolin nanocomplexes in managing inflammation-related diseases by antioxidant and immunoregulation. ACS Nano 18 (8), 6229–6242. doi:10.1021/acsnano.3c09528
Han, M., Lu, Y., Tao, Y., Zhang, X., Dai, C., Zhang, B., et al. (2023). Luteolin protects pancreatic β cells against apoptosis through regulation of autophagy and ROS clearance. Pharm. (Basel) 16 (7), 975. doi:10.3390/ph16070975
Han, Z., Batudeligen, C. H., Narisu, A., Xu, Y., Anda, A., Xu, Y., et al. (2024). Luteolin attenuates CCl4-induced hepatic injury by inhibiting ferroptosis via SLC7A11. BMC complementary Med. Ther. 24 (1), 193. doi:10.1186/s12906-024-04486-2
Hayasaka, N., Shimizu, N., Komoda, T., Mohri, S., Tsushida, T., Eitsuka, T., et al. (2018). Absorption and metabolism of luteolin in rats and humans in relation to in vitro anti-inflammatory effects. J. Agric. food Chem. 66 (43), 11320–11329. doi:10.1021/acs.jafc.8b03273
Hostetler, G. L., Ralston, R. A., and Schwartz, S. J. (2017). Flavones: food sources, bioavailability, metabolism, and bioactivity. Adv. Nutr. (Bethesda, Md) 8 (3), 423–435. doi:10.3945/an.116.012948
Hou, Y., Li, J., Ding, Y., Cui, Y., and Nie, H. (2022). Luteolin attenuates lipopolysaccharide-induced acute lung injury/acute respiratory distress syndrome by activating alveolar epithelial sodium channels via cGMP/PI3K pathway. J. Ethnopharmacol. 282, 114654. doi:10.1016/j.jep.2021.114654
Huang, G., Liu, X., Jiang, T., Cao, Y., Sang, M., Song, X., et al. (2023b). Luteolin overcomes acquired resistance to osimertinib in non-small cell lung cancer cells by targeting the HGF-MET-Akt pathway. Am. J. Cancer Res. 13 (9), 4145–4162.
Huang, L., Kim, M. Y., and Cho, J. Y. (2023a). Immunopharmacological activities of luteolin in chronic diseases. Int. J. Mol. Sci. 24 (3), 2136. doi:10.3390/ijms24032136
Imam, S. S., Alshehri, S., Altamimi, M. A., Hussain, A., Alyahya, K. H., Mahdi, W. A., et al. (2022). Formulation and evaluation of luteolin-loaded nanovesicles: in vitro physicochemical characterization and viability assessment. ACS omega 7 (1), 1048–1056. doi:10.1021/acsomega.1c05628
Imran, M., Rauf, A., Abu-Izneid, T., Nadeem, M., Shariati, M. A., Khan, I. A., et al. (2019). Luteolin, a flavonoid, as an anticancer agent: a review. Biomed. Pharmacother. 112, 108612. doi:10.1016/j.biopha.2019.108612
Jia, Q., Wen, J., Yang, Q., Liu, S., Zhang, J., Wang, T., et al. (2023). Lonicera japonica Thunb extract ameliorates lipopolysaccharide-induced acute lung injury associated with luteolin-mediated suppression of NF-κB signaling pathway. J. Inflamm. (Lond). 20 (1), 44. doi:10.1186/s12950-023-00372-9
Jiang, Z. B., Wang, W. J., Xu, C., Xie, Y. J., Wang, X. R., Zhang, Y. Z., et al. (2021). Luteolin and its derivative apigenin suppress the inducible PD-L1 expression to improve anti-tumor immunity in KRAS-mutant lung cancer. Cancer Lett. 515, 36–48. doi:10.1016/j.canlet.2021.05.019
Jiang, Z. Q., Li, M. H., Qin, Y. M., Jiang, H. Y., Zhang, X., and Wu, M. H. (2018). Luteolin inhibits tumorigenesis and induces apoptosis of non-small cell lung cancer cells via regulation of MicroRNA-34a-5p. Int. J. Mol. Sci. 19 (2), 447. doi:10.3390/ijms19020447
Jin, M., Yang, J. H., Lee, E., Lu, Y., Kwon, S., Son, K. H., et al. (2009). Antiasthmatic activity of luteolin-7-O-glucoside from Ailanthus altissima through the downregulation of T helper 2 cytokine expression and inhibition of prostaglandin E2 production in an ovalbumin-induced asthma model. Biol. Pharm. Bull. 32 (9), 1500–1503. doi:10.1248/bpb.32.1500
Ju, W., Wang, X., Shi, H., Chen, W., Belinsky, S. A., and Lin, Y. (2007). A critical role of luteolin-induced reactive oxygen species in blockage of tumor necrosis factor-activated nuclear factor-kappaB pathway and sensitization of apoptosis in lung cancer cells. Mol. Pharmacol. 71 (5), 1381–1388. doi:10.1124/mol.106.032185
Kar, B., Rout, S. R., Halder, J., Mahanty, R., Mishra, A., Saha, I., et al. (2024). The recent development of luteolin-loaded nanocarrier in targeting cancer. Curr. Pharm. Des. 30, 2129–2141. doi:10.2174/0113816128313713240628063301
Khan, J., Deb, P. K., Priya, S., Medina, K. D., Devi, R., Walode, S. G., et al. (2021). Dietary flavonoids: cardioprotective potential with antioxidant effects and their pharmacokinetic, toxicological and therapeutic concerns. Molecules 26 (13), 4021. doi:10.3390/molecules26134021
Khan, J., Saraf, S., and Saraf, S. (2016). Preparation and evaluation of luteolin-phospholipid complex as an effective drug delivery tool against GalN/LPS induced liver damage. Pharm. Dev. Technol. 21 (4), 475–486. doi:10.3109/10837450.2015.1022786
Kolb, M., and Vašáková, M. (2019). The natural history of progressive fibrosing interstitial lung diseases. Respir. Res. 20 (1), 57. doi:10.1186/s12931-019-1022-1
Kong, X., Huo, G., Liu, S., Li, F., Chen, W., and Jiang, D. (2019). Luteolin suppresses inflammation through inhibiting cAMP-phosphodiesterases activity and expression of adhesion molecules in microvascular endothelial cells. Inflammopharmacology 27 (4), 773–780. doi:10.1007/s10787-018-0537-2
Kure, A., Nakagawa, K., Kondo, M., Kato, S., Kimura, F., Watanabe, A., et al. (2016). Metabolic fate of luteolin in rats: its relationship to anti-inflammatory effect. J. Agric. Food Chem. 64 (21), 4246–4254. doi:10.1021/acs.jafc.6b00964
Kutlu, Z., Celik, M., Bilen, A., Halıcı, Z., Yıldırım, S., Karabulut, S., et al. (2020). Effects of umbelliferone isolated from the Ferulago pauciradiata Boiss. and Heldr. Plant on cecal ligation and puncture-induced sepsis model in rats. Biomed. Pharmacother. 127, 110206. doi:10.1016/j.biopha.2020.110206
Lamothe, S. M., and Zhang, S. (2013). The serum- and glucocorticoid-inducible kinases SGK1 and SGK3 regulate hERG channel expression via ubiquitin ligase Nedd4-2 and GTPase Rab11. J. Biol. Chem. 288 (21), 15075–15084. doi:10.1074/jbc.M113.453670
Lee, J. Y., Kim, J. M., and Kim, C. J. (2014). Flavones derived from nature attenuate the immediate and late-phase asthmatic responses to aerosolized-ovalbumin exposure in conscious Guinea pigs. Inflamm. Res. 63 (1), 53–60. doi:10.1007/s00011-013-0670-8
Levy, B. D., Clish, C. B., Schmidt, B., Gronert, K., and Serhan, C. N. (2001). Lipid mediator class switching during acute inflammation: signals in resolution. Nat. Immunol. 2 (7), 612–619. doi:10.1038/89759
Li, C., Li, Y., Huang, X., Li, S., Sangji, K., and Gu, R. (2024a). Traditional Tibetan medicine: therapeutic potential in lung diseases. Front. Pharmacol. 15, 1365911. doi:10.3389/fphar.2024.1365911
Li, L., Zhang, S., Yu, B., Liu, S. Q., and Xiong, Y. (2024c). Fractionating the flavonoids in lonicerae japonicae flos and lonicerae flos via solvent extraction coupled with automated solid-phase extraction. Foods Basel, Switz. 13 (23), 3861. doi:10.3390/foods13233861
Li, M., Wang, H., Lu, Y., and Cai, J. (2023a). Luteolin suppresses inflammation and oxidative stress in chronic obstructive pulmonary disease through inhibition of the NOX4-mediated NF-κB signaling pathway. Immun. Inflamm. Dis. 11 (4), e820. doi:10.1002/iid3.820
Li, W., Wang, X., Chen, Y., Ding, Y., Ling, X., Yuan, B., et al. (2024b). Luteolin-7-O-glucoside promotes macrophage release of IFN-β by maintaining mitochondrial function and corrects the disorder of glucose metabolism during RSV infection. Eur. J. Pharmacol. 963, 176271. doi:10.1016/j.ejphar.2023.176271
Li, X., Tang, Y., Liang, P., Sun, M., Li, T., Shen, Z., et al. (2023b). Luteolin inhibits A549 cells proliferation and migration by down-regulating androgen receptors. Eur. J. Med. Res. 28 (1), 353. doi:10.1186/s40001-023-01302-4
Liang, K. L., Yu, S. J., Huang, W. C., and Yen, H. R. (2020). Luteolin attenuates allergic nasal inflammation via inhibition of interleukin-4 in an allergic rhinitis mouse model and peripheral blood from human subjects with allergic rhinitis. Front. Pharmacol. 11, 291. doi:10.3389/fphar.2020.00291
Liu, B., Li, W., Zhao, J., Liu, Y., Zhu, X., and Liang, G. (2013). Physicochemical characterisation of the supramolecular structure of luteolin/cyclodextrin inclusion complex. Food Chem. 141 (2), 900–906. doi:10.1016/j.foodchem.2013.03.097
Liu, B., and Su, H. (2023). Luteolin improves vasoconstriction function and survival of septic mice via AMPK/NF-κB pathway. Heliyon 9 (2), e13330. doi:10.1016/j.heliyon.2023.e13330
Liu, X., Wu, D., Liu, J., Li, G., Zhang, Z., Chen, C., et al. (2022a). Characterization of xanthine oxidase inhibitory activities of phenols from pickled radish with molecular simulation. Food Chem. X 14, 100343. doi:10.1016/j.fochx.2022.100343
Liu, Z., Gao, S., Bu, Y., and Zheng, X. (2022b). Luteolin protects cardiomyocytes cells against lipopolysaccharide-induced apoptosis and inflammatory damage by modulating Nlrp3. Yonsei Med. J. 63 (3), 220–228. doi:10.3349/ymj.2022.63.3.220
Lu, J., Li, G., He, K., Jiang, W., Xu, C., Li, Z., et al. (2015). Luteolin exerts a marked antitumor effect in cMet-overexpressing patient-derived tumor xenograft models of gastric cancer. J. Transl. Med. 13, 42. doi:10.1186/s12967-015-0398-z
Lu, J., Zhao, X., and Gan, S. (2025). Global, regional and national burden of tracheal, bronchus, and lung cancer attributable to ambient particulate matter pollution from 1990 to 2021: an analysis of the global burden of disease study. BMC Public Health 25 (1), 108. doi:10.1186/s12889-024-21226-w
Lu, Z., Liu, J., Zhao, L., Wang, C., Shi, F., Li, Z., et al. (2023). Enhancement of oral bioavailability and anti-colitis effect of luteolin-loaded polymer micelles with RA (rosmarinic acid)-SS-mPEG as carrier. Drug Dev. Ind. Pharm. 49 (1), 17–29. doi:10.1080/03639045.2023.2175850
Luo, H., Wang, X., Fang, M., Yu, H., Gui, L., Wu, Z., et al. (2024). Combined hydroxyethyl starch luteolin nanocrystals for effective anti-hyperuricemia effect in mice model. Int. J. Nanomedicine 19, 5139–5156. doi:10.2147/IJN.S464948
Lv, R., Wang, L., Maehara, A., Matsumura, M., Guo, X., Samady, H., et al. (2023). Combining IVUS + OCT data, biomechanical models and machine learning method for accurate coronary plaque morphology quantification and cap thickness and stress/strain index predictions. J. Funct. Biomater. 14 (1), 41. doi:10.3390/jfb14010041
Malhotra, V., Shanley, T. P., Pittet, J. F., Welch, W. J., and Wong, H. R. (2001). Geldanamycin inhibits NF-kappaB activation and interleukin-8 gene expression in cultured human respiratory epithelium. Am. J. Respir. Cell Mol. Biol. 25 (1), 92–97. doi:10.1165/ajrcmb.25.1.4384
Manzoor, M. F., Ahmad, N., Ahmed, Z., Siddique, R., Zeng, X. A., Rahaman, A., et al. (2019). Novel extraction techniques and pharmaceutical activities of luteolin and its derivatives. J. Food Biochem. 43 (9), e12974. doi:10.1111/jfbc.12974
Masraksa, W., Tanasawet, S., Hutamekalin, P., Wongtawatchai, T., and Sukketsiri, W. (2020). Luteolin attenuates migration and invasion of lung cancer cells via suppressing focal adhesion kinase and non-receptor tyrosine kinase signaling pathway. Nutr. Res. Pract. 14 (2), 127–133. doi:10.4162/nrp.2020.14.2.127
Mazumder, S., Bindu, S., Debsharma, S., and Bandyopadhyay, U. (2024). Induction of mitochondrial toxicity by non-steroidal anti-inflammatory drugs (NSAIDs): the ultimate trade-off governing the therapeutic merits and demerits of these wonder drugs. Biochem. Pharmacol. 228, 116283. doi:10.1016/j.bcp.2024.116283
Men, X., Li, S., Cai, X., Fu, L., Shao, Y., and Zhu, Y. (2023). Antiviral activity of luteolin against pseudorabies virus in vitro and in vivo. Anim. (Basel) 13 (4), 761. doi:10.3390/ani13040761
Meng, K., Chen, X., Chen, Z., and Xu, J. (2025). Burden of chronic obstructive pulmonary disease in adults aged 70 years and older, 1990-2021: findings from the Global Burden of Disease Study 2021. PLoS One 20 (1), e0316135. doi:10.1371/journal.pone.0316135
Miao, J., Lin, F., Huang, N., and Teng, Y. (2021). Improving anti-inflammatory effect of luteolin with nano-micelles in the bacteria-induced lung infection. J. Biomed. Nanotechnol. 17 (6), 1229–1241. doi:10.1166/jbn.2021.3101
Mittler, R. (2017). ROS are good. Trends Plant Sci. 22 (1), 11–19. doi:10.1016/j.tplants.2016.08.002
Mokhtari, T., Lu, M., and El-Kenawy, A. E. (2023). Potential anxiolytic and antidepressant-like effects of luteolin in a chronic constriction injury rat model of neuropathic pain: role of oxidative stress, neurotrophins, and inflammatory factors. Int. Immunopharmacol. 122, 110520. doi:10.1016/j.intimp.2023.110520
Móritz, A. V., Kovács, H., Jerzsele, Á., Psáder, R., and Farkas, O. (2024). Flavonoids in mitigating the adverse effects of canine endotoxemia. Front. veterinary Sci. 11, 1396870. doi:10.3389/fvets.2024.1396870
Nabavi, S. F., Braidy, N., Gortzi, O., Sobarzo-Sanchez, E., Daglia, M., Skalicka-Woźniak, K., et al. (2015). Luteolin as an anti-inflammatory and neuroprotective agent: a brief review. Brain Res. Bull. 119 (Pt A), 1–11. doi:10.1016/j.brainresbull.2015.09.002
Nandhini, P., Gupta, P. K., Mahapatra, A. K., Das, A. P., Agarwal, S. M., Mickymaray, S., et al. (2023). In-Silico molecular screening of natural compounds as a potential therapeutic inhibitor for Methicillin-resistant Staphylococcus aureus inhibition. Chem. Biol. Interact. 374, 110383. doi:10.1016/j.cbi.2023.110383
Nguyen, H. T., Couture, R., Touaibia, M., and Martin, L. J. (2021). Transcriptome modulation following administration of luteolin to bleomycin-etoposide-cisplatin chemotherapy on rat LC540 tumor Leydig cells. Andrologia 53 (2), e13960. doi:10.1111/and.13960
Oyagbemi, A. A., Adejumobi, O. A., Ajibade, T. O., Asenuga, E. R., Afolabi, J. M., Ogunpolu, B. S., et al. (2021). Luteolin attenuates glycerol-induced acute renal failure and cardiac complications through modulation of kim-1/NF-κB/Nrf2 signaling pathways. J. Diet. Suppl. 18 (5), 543–565. doi:10.1080/19390211.2020.1811442
Patel, V. J., Biswas Roy, S., Mehta, H. J., Joo, M., and Sadikot, R. T. (2018). Alternative and natural therapies for acute lung injury and acute respiratory distress syndrome. BioMed Res. Int. 2018, 2476824. doi:10.1155/2018/2476824
Pittol, V., Veras, K. S., Doneda, E., Silva, A. D., Delagustin, M. G., Koester, L. S., et al. (2022). The challenge of flavonoid/cyclodextrin complexation in a complex matrix of the quercetin, luteolin, and 3-O-methylquercetin. Pharm. Dev. Technol. 27 (6), 625–634. doi:10.1080/10837450.2022.2098326
Podolanczuk, A. J., and Raghu, G. (2024). Idiopathic pulmonary fibrosis mortality: update on trends in the modern treatment era. Eur. Respir. J. 64 (2), 2401305. doi:10.1183/13993003.01305-2024
Pradhan, G., and Kulkarni, Y. A. (2024). Diabetes and its complications: role of luteolin, A wonder chemical from the natural source. Curr. Diabetes Rev. 21, e290224227537. doi:10.2174/0115733998285798240217084632
Punia Bangar, S., Kajla, P., Chaudhary, V., Sharma, N., and Ozogul, F. (2023). Luteolin: a flavone with myriads of bioactivities and food applications. Food Biosci. 52, 102366. doi:10.1016/j.fbio.2023.102366
Qing, W., Wang, Y., Li, H., Ma, F., Zhu, J., and Liu, X. (2017). Preparation and characterization of copolymer micelles for the solubilization and in vitro release of luteolin and luteoloside. AAPS PharmSciTech 18 (6), 2095–2101. doi:10.1208/s12249-016-0692-y
Raghu, G., Remy-Jardin, M., Myers, J. L., Richeldi, L., Ryerson, C. J., Lederer, D. J., et al. (2018). Diagnosis of idiopathic pulmonary fibrosis. An official ATS/ERS/JRS/ALAT clinical practice guideline. Am. J. Respir. Crit. Care Med. 198 (5), e44–e68. doi:10.1164/rccm.201807-1255ST
Rahman, M. M., Bibi, S., Rahaman, M. S., Rahman, F., Islam, F., Khan, M. S., et al. (2022). Natural therapeutics and nutraceuticals for lung diseases: traditional significance, phytochemistry, and pharmacology. Biomed. Pharmacother. 150, 113041. doi:10.1016/j.biopha.2022.113041
Rakoczy, K., Kaczor, J., Sołtyk, A., Szymańska, N., Stecko, J., Sleziak, J., et al. (2023). Application of luteolin in neoplasms and nonneoplastic diseases. Int. J. Mol. Sci. 24 (21), 15995. doi:10.3390/ijms242115995
Ramadaini, T., Sumiwi, S. A., and Febrina, E. (2024). The anti-diabetic effects of medicinal plants belonging to the liliaceae family: potential alpha glucosidase inhibitors. Drug Des. Devel Ther. 18, 3595–3616. doi:10.2147/DDDT.S464100
Ramesh, P., Jagadeesan, R., Sekaran, S., Dhanasekaran, A., and Vimalraj, S. (2021). Flavonoids: classification, function, and molecular mechanisms involved in bone remodelling. Front. Endocrinol. (Lausanne) 12, 779638. doi:10.3389/fendo.2021.779638
Rameshbabu, S., Alehaideb, Z., Alghamdi, S. S., Suliman, R. S., Almourfi, F., Yacoob, S. A. M., et al. (2024). Identification of anastatica hierochuntica L. Methanolic-Leaf-Extract-Derived metabolites exhibiting xanthine oxidase inhibitory activities: in vitro and in silico approaches. Metabolites 14 (7), 368. doi:10.3390/metabo14070368
Ren, P., Cao, J. L., Lin, P. L., Cao, B. Y., Chen, J. L., Gao, K., et al. (2021). Molecular mechanism of luteolin regulating lipoxygenase pathway against oxygen-glucose deprivation/reperfusion injury in H9c2 cardiomyocytes based on molecular docking. Zhongguo Zhong Yao Za Zhi 46 (21), 5665–5673. doi:10.19540/j.cnki.cjcmm.20210805.701
Ren, X., Yang, T., Zhang, K., Liu, Y., Wang, C., Wu, L., et al. (2023). Cyclodextrin MOFs modified dry powder inhalers quadruple bioavailability of luteolin to ameliorate fibrosing interstitial lung disease. Int. J. Pharm. 645, 123405. doi:10.1016/j.ijpharm.2023.123405
Roh, E. J. (2024). Comparison and review of international guidelines for treating asthma in children. Clin. Exp. Pediatr. 67 (9), 447–455. doi:10.3345/cep.2022.01466
Rungsung, S., Singh, T. U., Rabha, D. J., Kumar, T., Cholenahalli Lingaraju, M., Parida, S., et al. (2018). Luteolin attenuates acute lung injury in experimental mouse model of sepsis. Cytokine 110, 333–343. doi:10.1016/j.cyto.2018.03.042
Sabat, R., Grütz, G., Warszawska, K., Kirsch, S., Witte, E., Wolk, K., et al. (2010). Biology of interleukin-10. Cytokine Growth Factor Rev. 21 (5), 331–344. doi:10.1016/j.cytogfr.2010.09.002
Seelinger, G., Merfort, I., and Schempp, C. M. (2008a). Anti-oxidant, anti-inflammatory and anti-allergic activities of luteolin. Planta Med. 74 (14), 1667–1677. doi:10.1055/s-0028-1088314
Seelinger, G., Merfort, I., Wölfle, U., and Schempp, C. M. (2008b). Anti-carcinogenic effects of the flavonoid luteolin. Molecules 13 (10), 2628–2651. doi:10.3390/molecules13102628
Shen, M. L., Wang, C. H., Lin, C. H., Zhou, N., Kao, S. T., and Wu, D. C. (2016). Luteolin attenuates airway mucus overproduction via inhibition of the GABAergic system. Sci. Rep. 6, 32756. doi:10.1038/srep32756
Shi, M., Chen, Z., Gong, H., Peng, Z., Sun, Q., Luo, K., et al. (2024). Luteolin, a flavone ingredient: anticancer mechanisms, combined medication strategy, pharmacokinetics, clinical trials, and pharmaceutical researches. Phytother. Res. 38 (2), 880–911. doi:10.1002/ptr.8066
Shi, Y., Liu, C., Liu, X., Tang, D. G., and Wang, J. (2014). The microRNA miR-34a inhibits non-small cell lung cancer (NSCLC) growth and the CD44hi stem-like NSCLC cells. PLoS One 9 (3), e90022. doi:10.1371/journal.pone.0090022
Shimoi, K., Okada, H., Furugori, M., Goda, T., Takase, S., Suzuki, M., et al. (1998). Intestinal absorption of luteolin and luteolin 7-O-beta-glucoside in rats and humans. FEBS Lett. 438 (3), 220–224. doi:10.1016/s0014-5793(98)01304-0
Singh, K., Maurya, H., Singh, P., Panda, P., Behera, A. K., Jamal, A., et al. (2023). DISPEL: database for ascertaining the best medicinal plants to cure human diseases. Database (Oxford) 2023, 2023. doi:10.1093/database/baad073
Skibola, C. F., and Smith, M. T. (2000). Potential health impacts of excessive flavonoid intake. Free Radic. Biol. Med. 29 (3-4), 375–383. doi:10.1016/s0891-5849(00)00304-x
Stalin, A., Lin, D., Senthamarai, K. B., Feng, Y., Wang, Y., Zhao, W., et al. (2022). An in-silico approach to identify the potential hot spots in SARS-CoV-2 spike RBD to block the interaction with ACE2 receptor. J. Biomol. Struct. Dyn. 40 (16), 7408–7423. doi:10.1080/07391102.2021.1897682
Sun, B., Lei, M., Zhang, J., Kang, H., Liu, H., and Zhou, F. (2023). Acute lung injury caused by sepsis: how does it happen? Front. Med. 10, 1289194. doi:10.3389/fmed.2023.1289194
Sung, H., Ferlay, J., Siegel, R. L., Laversanne, M., Soerjomataram, I., Jemal, A., et al. (2021). Global cancer statistics 2020: GLOBOCAN estimates of incidence and mortality worldwide for 36 cancers in 185 countries. CA Cancer J. Clin. 71 (3), 209–249. doi:10.3322/caac.21660
Taliou, A., Zintzaras, E., Lykouras, L., and Francis, K. (2013). An open-label pilot study of a formulation containing the anti-inflammatory flavonoid luteolin and its effects on behavior in children with autism spectrum disorders. Clin. Ther. 35 (5), 592–602. doi:10.1016/j.clinthera.2013.04.006
Terzo, S., Amato, A., Magán-Fernández, A., Castellino, G., Calvi, P., Chianetta, R., et al. (2023). A nutraceutical containing chlorogenic acid and luteolin improves cardiometabolic parameters in subjects with pre-obesity: a 6-month randomized, double-blind, placebo-controlled study. Nutrients 15 (2), 462. doi:10.3390/nu15020462
Travis, W. D., Costabel, U., Hansell, D. M., King, T. E., Lynch, D. A., Nicholson, A. G., et al. (2013). An official American Thoracic Society/European Respiratory Society statement: update of the international multidisciplinary classification of the idiopathic interstitial pneumonias. Am. J. Respir. Crit. Care Med. 188 (6), 733–748. doi:10.1164/rccm.201308-1483ST
Ueda, Y., Oku, H., Iinuma, M., and Ishiguro, K. (2005). Antianaphylactic and antipruritic effects of the flowers of Impatiens textori MIQ. Biol. Pharm. Bull. 28 (9), 1786–1790. doi:10.1248/bpb.28.1786
Viegi, G., Pistelli, F., Sherrill, D. L., Maio, S., Baldacci, S., and Carrozzi, L. (2007). Definition, epidemiology and natural history of COPD. Eur. Respir. J. 30 (5), 993–1013. doi:10.1183/09031936.00082507
Wang, L., Chen, Q., Zhu, L., Li, Q., Zeng, X., Lu, L., et al. (2017). Metabolic disposition of luteolin is mediated by the interplay of UDP-glucuronosyltransferases and catechol-O-methyltransferases in rats. Drug Metab. Dispos. 45 (3), 306–315. doi:10.1124/dmd.116.073619
Wang, L., Zhang, Y., Song, Z., Liu, Q., Fan, D., and Song, X. (2023). Ginsenosides: a potential natural medicine to protect the lungs from lung cancer and inflammatory lung disease. Food Funct. 14 (20), 9137–9166. doi:10.1039/d3fo02482b
Wang, S., Wuniqiemu, T., Tang, W., Teng, F., Bian, Q., Yi, L., et al. (2021c). Luteolin inhibits autophagy in allergic asthma by activating PI3K/Akt/mTOR signaling and inhibiting Beclin-1-PI3KC3 complex. Int. Immunopharmacol. 94, 107460. doi:10.1016/j.intimp.2021.107460
Wang, X., Wang, L., Dong, R., Huang, K., Wang, C., Gu, J., et al. (2021b). Luteolin ameliorates LPS-induced acute liver injury by inhibiting TXNIP-NLRP3 inflammasome in mice. Phytomedicine Int. J. phytotherapy Phytopharm. 87, 153586. doi:10.1016/j.phymed.2021.153586
Wang, Z., Lin, J., Liang, L., Huang, F., Yao, X., Peng, K., et al. (2025). Global, regional, and national burden of chronic obstructive pulmonary disease and its attributable risk factors from 1990 to 2021: an analysis for the Global Burden of Disease Study 2021. Respir. Res. 26 (1), 2. doi:10.1186/s12931-024-03051-2
Wang, Z., Zeng, M., Wang, Z., Qin, F., Chen, J., and He, Z. (2021a). Dietary luteolin: a narrative review focusing on its pharmacokinetic properties and effects on glycolipid metabolism. J. Agric. food Chem. 69 (5), 1441–1454. doi:10.1021/acs.jafc.0c08085
Watterson, A., and Coelho, M. A. (2023). Cancer immune evasion through KRAS and PD-L1 and potential therapeutic interventions. Cell Commun. Signal 21 (1), 45. doi:10.1186/s12964-023-01063-x
Wells, A. U., Brown, K. K., Flaherty, K. R., Kolb, M., and Thannickal, V. J.IPF Consensus Working Group (2018). What's in a name? That which we call IPF, by any other name would act the same. Eur. Respir. J. 51 (5), 1800692. doi:10.1183/13993003.00692-2018
Wen, K., Fang, X., Yang, J., Yao, Y., Nandakumar, K. S., Salem, M. L., et al. (2021). Recent research on flavonoids and their biomedical applications. Curr. Med. Chem. 28 (5), 1042–1066. doi:10.2174/0929867327666200713184138
Wu, B., Xiong, J., Zhou, Y., Wu, Y., Song, Y., Wang, N., et al. (2020). Luteolin enhances TRAIL sensitivity in non-small cell lung cancer cells through increasing DR5 expression and Drp1-mediated mitochondrial fission. Archives Biochem. biophysics 692, 108539. doi:10.1016/j.abb.2020.108539
Wu, L., Liu, J., Han, W., Zhou, X., Yu, X., Wei, Q., et al. (2015). Time-dependent metabolism of luteolin by human UDP-glucuronosyltransferases and its intestinal first-pass glucuronidation in mice. J. Agric. Food Chem. 63 (39), 8722–8733. doi:10.1021/acs.jafc.5b02827
Wu, W., Li, K., Zhao, C., Ran, X., Zhang, Y., and Zhang, T. (2022). A rapid HPLC-MS/MS method for the simultaneous determination of luteolin, resveratrol and their metabolites in rat plasma and its application to pharmacokinetic interaction studies. J. Chromatogr. B, Anal. Technol. Biomed. life Sci. 1191, 123118. doi:10.1016/j.jchromb.2022.123118
Xiong, C., Wu, Q., Fang, M., Li, H., Chen, B., and Chi, T. (2020). Protective effects of luteolin on nephrotoxicity induced by long-term hyperglycaemia in rats. J. Int. Med. Res. 48 (4), 300060520903642. doi:10.1177/0300060520903642
Xiong, J., Wang, K., Yuan, C., Xing, R., Ni, J., Hu, G., et al. (2017). Luteolin protects mice from severe acute pancreatitis by exerting HO-1-mediated anti-inflammatory and antioxidant effects. Int. J. Mol. Med. 39 (1), 113–125. doi:10.3892/ijmm.2016.2809
Xiong, L., Liu, Y., Wang, Y., Zhao, H., Song, X., Fan, W., et al. (2024). The protective effect of Lonicera japonica Thunb. against lipopolysaccharide-induced acute lung injury in mice: modulation of inflammation, oxidative stress, and ferroptosis. J. Ethnopharmacol. 331, 118333. doi:10.1016/j.jep.2024.118333
Xiong, Y., Gao, M., van Duijn, B., Choi, H., van Horssen, F., and Wang, M. (2021). International policies and challenges on the legalization of traditional medicine/herbal medicines in the fight against COVID-19. Pharmacol. Res. 166, 105472. doi:10.1016/j.phrs.2021.105472
Xu, T., Li, D., and Jiang, D. (2012). Targeting cell signaling and apoptotic pathways by luteolin: cardioprotective role in rat cardiomyocytes following ischemia/reperfusion. Nutrients 4 (12), 2008–2019. doi:10.3390/nu4122008
Xu, Y., Zhang, J., Liu, J., Li, S., Li, C., Wang, W., et al. (2015). Luteolin attenuate the D-galactose-induced renal damage by attenuation of oxidative stress and inflammation. Nat. Prod. Res. 29 (11), 1078–1082. doi:10.1080/14786419.2014.981181
Yaghi, A., Zaman, A., Cox, G., and Dolovich, M. B. (2012). Ciliary beating is depressed in nasal cilia from chronic obstructive pulmonary disease subjects. Respir. Med. 106 (8), 1139–1147. doi:10.1016/j.rmed.2012.04.001
Yang, L., Xie, N., Huang, J., Huang, H., Xu, S., Wang, Z., et al. (2018). SIK1-LNC represses the proliferative, migrative, and invasive abilities of lung cancer cells. Onco Targets Ther. 11, 4197–4206. doi:10.2147/OTT.S165278
Yao, C., Dai, S., Wang, C., Fu, K., Wu, R., Zhao, X., et al. (2023). Luteolin as a potential hepatoprotective drug: molecular mechanisms and treatment strategies. Biomed. and Pharmacother. = Biomedecine and Pharmacother. 167, 115464. doi:10.1016/j.biopha.2023.115464
Yasuda, M. T., Fujita, K., Hosoya, T., Imai, S., and Shimoi, K. (2015). Absorption and metabolism of luteolin and its glycosides from the extract of Chrysanthemum morifolium flowers in rats and caco-2 cells. J. Agric. Food Chem. 63 (35), 7693–7699. doi:10.1021/acs.jafc.5b00232
Yeo, M., Park, H. K., Lee, K. M., Lee, K. J., Kim, J. H., Cho, S. W., et al. (2004). Blockage of HSP 90 modulates Helicobacter pylori-induced IL-8 productions through the inactivation of transcriptional factors of AP-1 and NF-kappaB. Biochem. Biophys. Res. Commun. 320 (3), 816–824. doi:10.1016/j.bbrc.2004.05.214
Yuan, Q., Feng, W., Wang, Y., Wang, Q., Mou, N., Xiong, L., et al. (2022). Luteolin attenuates the pathogenesis of Staphylococcus aureus by interfering with the agr system. Microb. Pathog. 165, 105496. doi:10.1016/j.micpath.2022.105496
Zhang, D., Li, Y., Jiang, W., Li, W., Yuan, X., and Lin, Z. (2023). A network pharmacology-based treatment analysis of luteolin for regulating pyroptosis in acute lung injury. Shock 60 (2), 306–314. doi:10.1097/SHK.0000000000002168
Zhang, L., Liao, Y., and Tang, L. (2019). MicroRNA-34 family: a potential tumor suppressor and therapeutic candidate in cancer. J. Exp. and Clin. cancer Res. CR 38 (1), 53. doi:10.1186/s13046-019-1059-5
Zhang, M., Wang, R., Tian, J., Song, M., Zhao, R., Liu, K., et al. (2021b). Targeting LIMK1 with luteolin inhibits the growth of lung cancer in vitro and in vivo. J. Cell. Mol. Med. 25 (12), 5560–5571. doi:10.1111/jcmm.16568
Zhang, Z., Wang, J., Lin, Y., Chen, J., Liu, J., and Zhang, X. (2024). Nutritional activities of luteolin in obesity and associated metabolic diseases: an eye on adipose tissues. Crit. Rev. Food Sci. Nutr. 64 (12), 4016–4030. doi:10.1080/10408398.2022.2138257
Zhang, Z. T., Zhang, D. Y., Xie, K., Wang, C. J., and Xu, F. (2021a). Luteolin activates Tregs to promote IL-10 expression and alleviating caspase-11-dependent pyroptosis in sepsis-induced lung injury. Int. Immunopharmacol. 99, 107914. doi:10.1016/j.intimp.2021.107914
Zheng, H., Zhu, X., Gong, E., Lv, Y., Li, Y., and Cai, X. (2023). Luteolin suppresses lung cancer progression through targeting the circ_0000190/miR-130a-3p/notch-1 signaling pathway. J. Chemother. 35 (4), 330–342. doi:10.1080/1120009X.2022.2102303
Zhou, L., Jian, T., Wan, Y., Huang, R., Fang, H., Wang, Y., et al. (2023). Luteolin alleviates oxidative stress in chronic obstructive pulmonary disease induced by cigarette smoke via modulation of the TRPV1 and CYP2A13/NRF2 signaling pathways. Int. J. Mol. Sci. 25 (1), 369. doi:10.3390/ijms25010369
Zhou, P., Li, L. P., Luo, S. Q., Jiang, H. D., and Zeng, S. (2008). Intestinal absorption of luteolin from peanut hull extract is more efficient than that from individual pure luteolin. J. Agric. Food Chem. 56 (1), 296–300. doi:10.1021/jf072612+
Zhou, Y. S., Cui, Y., Zheng, J. X., Quan, Y. Q., Wu, S. X., Xu, H., et al. (2022). Luteolin relieves lung cancer-induced bone pain by inhibiting NLRP3 inflammasomes and glial activation in the spinal dorsal horn in mice. Phytomedicine 96, 153910. doi:10.1016/j.phymed.2021.153910
Zhu, J., Yan, H., Shi, M., Zhang, M., Lu, J., Wang, J., et al. (2023). Luteolin inhibits spike protein of severe acute respiratory syndrome coronavirus-2 (SARS-CoV-2) binding to angiotensin-converting enzyme 2. Phytother. Res. 37 (8), 3508–3521. doi:10.1002/ptr.7826
Zou, Y., Luo, X., Feng, Y., Fang, S., Tian, J., Yu, B., et al. (2021). Luteolin prevents THP-1 macrophage pyroptosis by suppressing ROS production via Nrf2 activation. Chem. Biol. Interact. 345, 109573. doi:10.1016/j.cbi.2021.109573
PGE2 Prostaglandin E2
NF-κB Nuclear Factor kappa-B
COPD Chronic Obstructive Pulmonary Disease
ALI Acute Lung Injury
ARDS Acute Respiratory Distress Syndrome
ENaC Epithelial Sodium Channel
AFC Alveolar Fluid Clearance
cGMP Cyclic Guanosine Monophosphate
LPS Lipopolysaccharide
IL-6 Interleukin-6
TNF-α Tumor Necrosis Factor alpha
CLP Cecal Ligation and Puncture
ROS Reactive Oxygen Species
GSH Glutathione
SOD Superoxide Dismutase
MDA Malondialdehyde
HMGB1 High-Mobility Group Box 1
iNOS Inducible Nitric Oxide Synthase
GSDMD-N Gasdermin D-N gasdermin D
TRAIL Tumor Necrosis Factor-related Apoptosis-inducing Ligand
DR5 Death Receptor five
NLRP3 NOD-like Receptor Protein 3
LIMK1 LIM Kinase 1
NSCLC Non-Small Cell Lung Cancer
EGFR Epidermal Growth Factor Receptor
PD-L1 Programmed Death-Ligand 1
STAT3 Signal Transducer and Activator of Transcription 3
miR-34a MicroRNA-34a
circRNA Circular RNA
Tregs regulatory T cells
IPF Idiopathic pulmonary fibrosis
ILD interstitial lung disease
PLA2 Phospholipase A2
GABAAR γ-Aminobutyric acid sub-type A receptors
CS Cigarette Smoke
NOX4 NADPH oxidase four
Ca+ Calcium ions
CYP2A13 Cytochrome P450 family 2 subfamily A member 13
Keap1 Kelch-like ECH-associated protein 1
SIRT6 Sirtuin 6
Nrf2 Nuclear factor erythroid 2-related factor 2
PGC1α Peroxisome proliferator-activated receptor gamma coactivator 1-alpha
SOD2 Superoxide dismutase 2
SOD1 Superoxide dismutase 1
ACE2 angiotensin-converting enzyme 2
TMPRSS2 Transmembrane Protease serine 2
ISG interferon-stimulated genes
MRSA methicillin-resistant staphylococcus aureus
SARS-CoV-2 severe acute respiratory syndrome coronavirus.
Keywords: luteolin, pulmonary diseases, molecular mechanisms, bioavailability, pharmacokinetics, pharmacological activities
Citation: Lv J, Song X, Luo Z, Huang D, Xiao L and Zou K (2025) Luteolin: exploring its therapeutic potential and molecular mechanisms in pulmonary diseases. Front. Pharmacol. 16:1535555. doi: 10.3389/fphar.2025.1535555
Received: 27 November 2024; Accepted: 22 January 2025;
Published: 12 February 2025.
Edited by:
Ruyu Yao, Chinese Academy of Sciences (CAS), ChinaReviewed by:
Gang Fan, Chengdu University of Traditional Chinese Medicine, ChinaCopyright © 2025 Lv, Song, Luo, Huang, Xiao and Zou. This is an open-access article distributed under the terms of the Creative Commons Attribution License (CC BY). The use, distribution or reproduction in other forums is permitted, provided the original author(s) and the copyright owner(s) are credited and that the original publication in this journal is cited, in accordance with accepted academic practice. No use, distribution or reproduction is permitted which does not comply with these terms.
*Correspondence: Kang Zou, em91a2FuZ0BnbXUuZWR1LmNu; Li Xiao, Mzc2MjY0MDkzQGdtdS5lZHUuY24=
†ORCID: Kang Zou, orcid.org/0000-0002-4959-1951
Disclaimer: All claims expressed in this article are solely those of the authors and do not necessarily represent those of their affiliated organizations, or those of the publisher, the editors and the reviewers. Any product that may be evaluated in this article or claim that may be made by its manufacturer is not guaranteed or endorsed by the publisher.
Research integrity at Frontiers
Learn more about the work of our research integrity team to safeguard the quality of each article we publish.