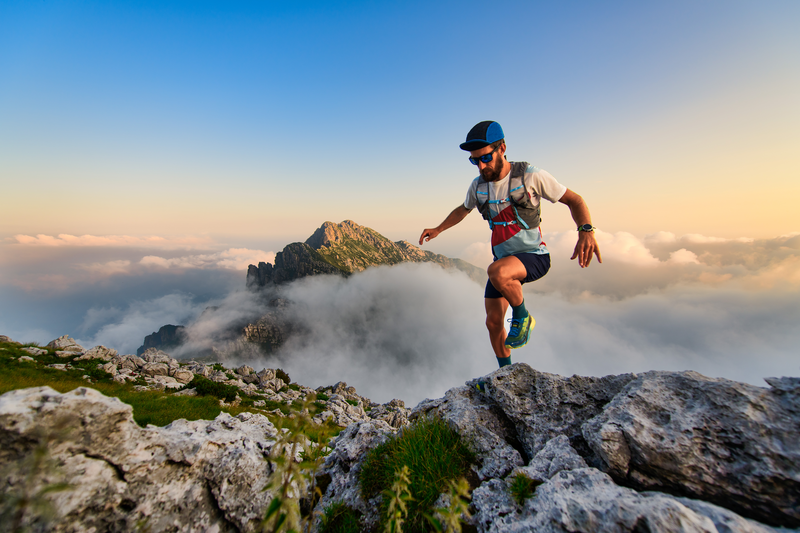
95% of researchers rate our articles as excellent or good
Learn more about the work of our research integrity team to safeguard the quality of each article we publish.
Find out more
ORIGINAL RESEARCH article
Front. Pharmacol. , 24 January 2025
Sec. Pharmacoepidemiology
Volume 16 - 2025 | https://doi.org/10.3389/fphar.2025.1524702
This article is part of the Research Topic Advances in Drug-induced Diseases Volume II View all 43 articles
Objective: Voriconazole is a broad-spectrum antifungal agent. It is used widely for the prevention and treatment of invasive fungal infections in patients with a hematological malignancy, but studies on its safety in this population are scarce. We assessed the adverse drug events (ADEs) of voriconazole in this population based on the US Food and Drug Administration Adverse Event Reporting System (FAERS) database to improve understanding of the safety of voriconazole.
Research design and methods: ADE reports for patients with a hematological malignant tumor using voriconazole between the first quarter of 2004 to the first quarter of 2024 were retrieved. Then, they were classified using the preferred terminology (PT) and system organ category (SOC) in the Medical Dictionary for Regulatory Activities. Data mining was done using reporting odds ratio (ROR), proportional reporting ratio (PRR), Bayesian confidence propagation neural network (BCPNN), and multi-item gamma Poisson shrinker (MGPS).
Results: A total of 605 ADEs were included: 116 (19.17%) in children and 489 (80.83%) in adults. The types of SOC involved in children and adults were 22 and 24, respectively. The only positive SOC signal that satisfied all four algorithms simultaneously in children was “psychiatric disorders”, whereas in adults they were “endocrine disorders” and “hepatobiliary disorders”. At the PT level, the types involved in children and adults were 28 and 74, respectively. The highest ROR signal intensities were found for “hallucinations, mixed” in children and “toxic optic neuropathy” in adults. The median time of onset of the ADE in children and adults was 11 and 8.5 days, respectively.
Conclusion: We used four algorithms (ROR, PRR, BCPNN, MGPS) to mine the signals of voriconazole in patients with a hematological malignant tumor, and compared the differences between children and adults. This study is important for targeting the monitoring, and could help to improve the safety of voriconazole.
According to studies using cell lines involved in tumor transformation, the World Health Organization (WHO) classifies hematological malignant tumors into “myeloid tumours”, “lymphoid tumours”, “mast cell disorders”, and “histiocyte tumours” (Arber et al., 2016; Mertowska et al., 2023; Swerdlow et al., 2016). In patients suffering from a hematological malignancy, due to the fact that their immune system may be suppressed by the disease or treatment, these patients are more susceptible to invasive fungal infections (IFIs). In addition, patients with hematological malignant tumors often require the use of central venous catheters to provide medication, fluids, or nutrition, which also increases the risk of infection. Therefore, these patients are a high-risk group for IFIs and require special prevention and treatment strategies (Jenks et al., 2020; Pagano et al., 2011).
Voriconazole is a broad-spectrum antifungal agent belonging to the triazole class. It inhibits ergosterol biosynthesis in the membranes of fungal cells. Voriconazole is active against a wide range of fungi, including Aspergillus spp, Candida spp, and several others (Fernández Ávila et al., 2021; Purkins et al., 2003; Xie et al., 2023). Voriconazole is used as first-line treatment for invasive aspergillosis. It can also be used for prophylaxis in high-risk patients with an IFI. Voriconazole is active against a wide range of fungi, but it produces adverse effects, including hepatotoxicity, visual disturbances, and rash (Eiden et al., 2007; Yasu et al., 2022; Kim et al., 2017; Mihăilă, 2015; Wu et al., 2021; Xie et al., 2023). Studies have shown that adjustments to dosing regimens for voriconazole based on therapeutic drug monitoring are beneficial for promoting its safety and efficacy (Luong et al., 2016; Valle-T-Figueras et al., 2021).
There are significant differences in physiology and pharmacokinetics between children and adults,but the dosage for children is usually extrapolated from the adult dose (Leroux et al., 2021; Sienkiewicz-Oleszkiewicz et al., 2023; Wei et al., 2019). Children and adults may have different sensitivity and tolerance to drugs, leading to differences in the types and incidence of adverse drug events (ADEs) (Kearns et al., 2003; Pasternak et al., 2019). Therefore, grouping patients in these two age groups can more accurately evaluate the safety and efficacy of voriconazole in patients with hematological malignancies, providing more specific guidance for clinical medication.
The US Food and Drug Administration Adverse Event Reporting System (FAERS) is one of the major databases for the post-marketing surveillance of drugs (Cirmi et al., 2020; Feng et al., 2022). It is updated quarterly and publicly available for free download (Sakaeda et al., 2013). The database is used widely in pharmacovigilance studies to compensate for the limitations of the pre-marketing studies of drugs. FAERS plays an important part in updating drug inserts and releasing information on drug-safety alerts (Raisch et al., 2014).
Herein, we undertook data mining of ADEs with voriconazole in patients with a hematological malignancy using FAERS. We compared the differences between children and adults to provide information on the safety of voriconazole.
This study is based on data from FAERS from the first quarter of 2004 to the first quarter of 2024 (Zhai et al., 2019). FAERS consists of seven American Standard Code for Information Interchange data files: Demographic and Management Information, Adverse Drug Reaction Information, Patient Information, Drug Information, Date of Start and End of Treatment, Reporting Source Information, and Indication of Use/Diagnosis (Yu and Liu, 2024). The most recent FDA_DT with the same CASE ID, or a higher PRIMARY ID when the CASE ID and FDA_DT were identical, was selected to identify and remove duplicate reports (Huang et al., 2024; Zhou et al., 2022).
The search was carried out using the drug names (voriconazole or Vfend) as the primary suspect in the ROLE field. Only patients identified as having a hematological malignant tumor were included. In addition, to compare the differences between adults and children, ADEs with missing ages were excluded (76 cases). The screening process for ADEs is shown in Figure 1.
ADEs were described and classified using the preferred terminology (PT) and the system organ category (SOC) in Medical Dictionary for Regulatory Activities v.26.0 (Romão et al., 2024).
With respect to the safety evaluation of drugs, there are four commonly used signal-mining methods: reporting odds ratio (ROR), proportional reporting ratio (PRR), Bayesian confidence propagation neural network (BCPNN), and multi-item gamma Poisson shrinker (MGPS) (Jiang et al., 2024; Liu et al., 2024; Xiong et al., 2024). The calculation and judgment criteria for these four signal mining methods are shown in Supplementary Table S1. In this study, to remove bias, only those that met all the criteria of the four algorithms were considered to be positive signals (Zhang et al., 2024).
We also assessed the time-to-onset of the ADE, which was defined as the interval between the onset date (EVENT_DT) and start date (START_DT).
Data were analyzed using SPSS 26.0 (IBM, Armonk, NY, United States). Descriptive statistics were used. Variables are presented as numbers and percentages. R 4.3.1 (R Institute for Statistical Computing, Vienna, Austria) was employed for data visualization.
Figure 2 presents the annual distribution of ADEs related to voriconazole use in patients with a hematological malignancy: there was a general upward trend until 2020.
Figure 2. Distribution of reported trends in the use of voriconazole ADEs in patients with a hematological malignant tumor from Q1 2004 to Q1 2024.
The number of children and adults was 116 (19.17%) and 489 (80.83%), respectively (Table 1). The study cohort comprised 214 females (35.37%), 372 males (61.49%), and 19 unspecified cases (3.14%). The country that provided the most ADEs was the USA, followed by France. The main reporter type was “professionals” (physician, pharmacist, health-professional), accounting for 88.26%, which greatly improved the reliability of ADE information.
Table 1. Demographic characteristics of ADEs reported in FAERS (first quarter of 2004 to first quarter of 2024) with voriconazole use as the main suspected drug in patients with a hematological malignancy.
At the SOC level (Tables 2, 3), the number of ADEs caused by voriconazole in children and adults was 22 and 24, respectively. The only positive signal that satisfied all four algorithms simultaneously in children was “psychiatric disorders”, whereas in adults it was “endocrine disorders” and “hepatobiliary disorders”.
Table 2. Frequency and signal intensity of ADEs in children at the level of system organ classifications (SOC).
Table 3. Frequency and signal intensity of ADEs in adults at the level of system organ classifications (SOC).
Figure 3 shows the frequency of each SOC in children and adults as a percentage of the total SOC. The SOCs shown in group 1 were present in children and adults. The SOCs in group 2 were present in children or adults.
Figure 3. Dual-valued histograms plotted to depict the difference in SOCs between children and adults. Note: left: children; right: adult.
Table 4 shows all positive PTs of voriconazole in the child group (ranked by ROR), totaling 28 types involving 13 SOCs. The top-five PTs were “hallucinations, mixed” (ROR: 363.94), “labelled drug-drug interaction medication error” (ROR: 170.43), “photosensitivity reaction” (ROR: 116.47), “steatohepatitis” (ROR: 116.25), and “drug level below therapeutic” (ROR: 109.37).
Table 5 shows all positive PTs of voriconazole in the adult group (ranked by ROR), totaling 74 types involving 17 SOCs. The top-five PTs were “toxic optic neuropathy” (ROR: 2722.79), “drug level below therapeutic” (ROR: 348.91), “vascular access site infection” (ROR: 316.94), “peptic ulcer haemorrhage” (ROR: 308.45), and “drug level decreased” (ROR: 175.69).
We created a volcano plot to visualize the differences in positive PTs between children and adults (Figure 4). The x-axis of the volcano plot showed the magnitude of ROR values (log2), the y-axis height represented statistical significance, and higher points indicated lower p-values (which are more statistically significant results). Each point in the graph represents a relevant PT.
Figure 4. Volcano plot of age-differentiated risk signals for voriconazole in patients with a hematological malignant tumor. Note: Horizontal coordinates indicate log2 ROR (left: children; right: adult) and vertical coordinates indicate −log10-transformed adjusted p-values. Significant signals are highlighted in color. p-values are adjusted using the false discovery rate.
In the children group, the main terms were “hallucinations, mixed”, “photosensitivity reaction”, “drug interaction”, “labelled drug–drug interaction medication error”, “drug level below therapeutic”, “steatohepatitis”, and “cheilitis”. In the adult group, the main terms were “toxic optic neuropathy”, “drug interaction”, “drug ineffective”, “drug level below therapeutic”, “vascular access site infection”, “peptic ulcer haemorrhage”, and “drug level decreased”.
After excluding reports of missing or inaccurate start/onset date, 81 ADEs were collected, with most cases occurring within 0 and 30 days (n = 60, 74.07%), followed by 31–60 days (n = 11, 13.58%). The number of different time periods in children and adults is shown in Figure 5. The median time-of-onset of the ADE in children and adults was 11 and 8.5 days, respectively.
Figure 5. Distribution of time-to-onset of voriconazole-associated adverse reactions in child and adult patients with a hematological malignant tumor.
Voriconazole underwent rigorous pre-marketing clinical trials to ensure its efficacy and safety in treating IFIs. However, the diversity and complexity of the patient population in actual clinical use suggest that there may be ADEs that have not been identified or evaluated fully. We conducted in-depth signal mining and evaluation to explore differences in ADEs between children and adults treated with voriconazole in patients with a hematological malignancy using FAERS. We aimed to provide important information for future clinical use.
Figure 2 showed an upward trend in the number of ADEs related to voriconazole from 2004 to 2020. This trend may have been related to the increased frequency of voriconazole use and increased awareness of ADEs. In particular, the number of reports peaked in 2020, which may have been associated with the coronavirus disease-2019 pandemic (Salmanton-García et al., 2024; Hlaing et al., 2023; Papakonstantinou et al., 2021). Since 2021, there has been a downward trend, which may be related to the development of individualized use of voriconazole in clinical practice.
At the SOC level, commonalities and differences were presented between child and adult groups. The only positive signal that satisfied all four algorithms simultaneously in children was “psychiatric disorders” (Table 2) whereas, in adults, the positive signals were “endocrine disorders” and “hepatobiliary disorders” (Table 3). The percentages of SOC that were significantly higher in children than in adults were “skin and subcutaneous tissue disorders”, “gastrointestinal disorders”, “psychiatric disorders”, and “musculoskeletal and connective tissue disorders” (Figure 3). The percentages of SOC that were significantly higher in adults than in children were “investigations”, “respiratory, thoracic and mediastinal disorders”, “cardiac disorders”, “eye disorders”, and “neoplasms benign, malignant and unspecified (including cysts and polyps)”. In addition, two SOCs were unique to the children group (“ear and labyrinth disorders” and “product issues”), four SOCs were unique to the adult group (“reproductive system and breast disorders”, “congenital, familial and genetic disorders”, “surgical and medical procedures”, and “pregnancy, puerperium and perinatal conditions”). This phenomenon may have been due to the unique physiological state and drug-metabolism characteristics of children (children show greater systemic metabolism of voriconazole than adults) (Leroux et al., 2021).
At the PT level, “photosensitivity reactions” is a more common dermatological complication of voriconazole than in other azole antifungal agents (Malani and Aronoff, 2008). We found that the ROR signal intensity of this PT was higher in children and significant compared with that in adults (Figure 4). This finding provides additional evidence of the need for caution when prescribing voriconazole in children.
In the children group, “hallucinations, mixed” was the PT signal with the highest ROR signal intensity (Table 4). In an observational study of 72 patients aged 14–76 years treated with voriconazole, hallucinations occurred in 12 cases (16.67%). Half of these patients did not report their hallucinations spontaneously. They showed reluctance to describe them, possibly due to embarrassment and other contributing factors (Zonios et al., 2008). A case report and literature review of voriconazole-induced hallucinations and visual disturbances reported 42 cases, three of whom were children (Zheng et al., 2021). In a recent study, a search of multiple databases on drug-induced musical hallucinations identified 27 cases and 21 triggering drugs. Among them, three patients (11.11%) had musical hallucinations induced by voriconazole (Bakewell et al., 2024; Zonios et al., 2008). Voriconazole treatment-related hallucinations may be overlooked by physicians. There are few reports of hallucinations associated with voriconazole in children. However, given the high ROR in the current study, we suggest that whether children experience hallucinations deserves more clinical attention.
In the adult group, “toxic optic neuropathy” had an unusually high ROR signal intensity and high statistical significance (Figure 4). One study revealed six cases of toxic optic neuropathy induced by voriconazole in pharmacovigilance databases (e.g., VigiAcess), and voriconazole was the only drug suspected in two cases (Orssaud et al., 2021). Mounier et al. reported a case of ophthalmic complications possibly caused by toxic optic neuropathy (Mounier et al., 2018). Understanding the mechanism leading to this neuro-ophthalmic adverse effect is crucial for clinical practice. One meta-analysis indicated a trough concentration >3.0 mg/L to be associated with an increased risk of moderate-to-severe hepatotoxicity, and >4.0 mg/L to be associated with an increased risk of neurotoxicity (Jin et al., 2016). Those data suggest a need for close monitoring of the therapeutic concentrations of voriconazole during treatment. Notably, there were “drug interactions”, “drug level below therapeutic”, and “drug level increased” in children and adults in our study. Studies have shown interactions between voriconazole and carbamazepine, cyclophosphamide, aprepitant, tacrolimus, and letermovir, which are related to the induction or inhibition of metabolic enzymes such as CYP2C19, 3A4, 3a5-2D6. Multiple guidelines recommend monitoring the drug concentration during voriconazole treatment to improve safety and efficacy, preferably with prospective dose optimization based on genotype.
We identified new PTs that were not previously listed in the drug label, such as “generalised tonic–clonic seizures” (Table 5) and “disorientation” (Table 4). This finding: (i) suggests that certain patient groups may be at risk; (ii) demonstrates the importance of ongoing post-marketing surveillance and signal mining for ADEs.
Analyses of time-to-onset of the ADE showed that most ADEs occurred within 0 days and 30 days of dosing (Figure 5). The median time-to-onset of the ADE was 11 days in the children group and 8.5 days in the adult group. These data suggested that close monitoring should be carried out during the initial stage of voriconazole treatment. However, one case occurred in the adult group after 1 year of treatment with voriconazole.
Our study had two main limitations. First, FAERS faces inherent challenges, including incomplete, inaccurate, inconsistent, and delayed reporting of ADEs. These factors may have affected the relevance and accuracy of our results. Second, our analysis was affected by the uneven distribution of cases in FAERS, with more adult patients but a significantly smaller number of children. This uneven distribution of cases may have introduced a bias and limited the applicability of our findings. Further prospective clinical studies are needed to overcome these limitations and provide more reliable insights.
We used four algorithms (ROR, PRR, BCPNN, MGPS) to mine the signals of voriconazole in patients with a hematological malignant tumor. We found similarities and differences in SOC/PT signals between children and adults, but also identified some new PT signals not included in the drug label. In the future clinical use, differentiated pharmaceutical monitoring should be carried out for children and adults, and personalized dosing measures, such as therapeutic drug monitoring, should be combined to optimize the dosage, in order to improve the safety of voriconazole in patients with hematological malignancies.
The raw data supporting the conclusions of this article will be made available by the authors, without undue reservation.
HL: Conceptualization, Data curation, Formal Analysis, Methodology, Software, Supervision, Validation, Visualization, Writing–original draft. MJ: Data curation, Methodology, Supervision, Validation, Writing–original draft. XP: Data curation, Formal Analysis, Writing–original draft. LK: Conceptualization, Formal Analysis, Project administration, Writing–review and editing.
The author(s) declare that financial support was received for the research, authorship, and/or publication of this article. This work was supported by the Natural Science Foundation of Bengbu Medical University (No. 2023byzd060), and Anhui Province Key Clinical Specialty Construction Project (No. 2023-320).
The authors declare that the research was conducted in the absence of any commercial or financial relationships that could be construed as a potential conflict of interest.
The author(s) declare that no Generative AI was used in the creation of this manuscript.
All claims expressed in this article are solely those of the authors and do not necessarily represent those of their affiliated organizations, or those of the publisher, the editors and the reviewers. Any product that may be evaluated in this article, or claim that may be made by its manufacturer, is not guaranteed or endorsed by the publisher.
The Supplementary Material for this article can be found online at: https://www.frontiersin.org/articles/10.3389/fphar.2025.1524702/full#supplementary-material
Arber, D. A., Orazi, A., Hasserjian, R., Thiele, J., Borowitz, M. J., Le Beau, M. M., et al. (2016). The 2016 revision to the World Health Organization classification of myeloid neoplasms and acute leukemia. Blood 127 (20), 2391–2405. doi:10.1182/blood-2016-03-643544
Bakewell, B., Johnson, M., Lee, M., Tchernogorova, E., Taysom, J., and Zhong, Q. (2024). Drug-induced musical hallucination. Front. Pharmacol. 15, 1401237. doi:10.3389/fphar.2024.1401237
Cirmi, S., El Abd, A., Letinier, L., Navarra, M., and Salvo, F. (2020). Cardiovascular toxicity of tyrosine kinase inhibitors used in chronic myeloid leukemia: an analysis of the FDA adverse event reporting system database (FAERS). Cancers (Basel) 12 (4), 826. doi:10.3390/cancers12040826
Eiden, C., Peyrière, H., Cociglio, M., Djezzar, S., Hansel, S., Blayac, J.-P., et al. (2007). Adverse effects of voriconazole: analysis of the French Pharmacovigilance Database. Ann. Pharmacother. 41 (5), 755–763. doi:10.1345/aph.1H671
Feng, Z., Li, X., Tong, W. K., He, Q., Zhu, X., Xiang, X., et al. (2022). Real-world safety of PCSK9 inhibitors: a pharmacovigilance study based on spontaneous reports in FAERS. Front. Pharmacol. 13, 894685. doi:10.3389/fphar.2022.894685
Fernández Ávila, D. C., Diehl, M., Degrave, A. M., Buttazzoni, M., Pereira, T., Aguirre, M. A., et al. (2021). Voriconazole-induced periostitis. Reumatismo 73 (1), 44–47. doi:10.4081/reumatismo.2021.1368
Hlaing, K. M., Monday, L. M., Nucci, M., Nouér, S. A., and Revankar, S. G. (2023). Invasive fungal infections associated with COVID-19. J. Fungi 9 (6), 667. doi:10.3390/jof9060667
Huang, F., San, X., Liu, Q., Zhu, H., and Xu, W. (2024). Signal mining and risk analysis of Alprazolam adverse events based on the FAERS database. Sci. Rep. 14 (1), 7489. doi:10.1038/s41598-024-57909-y
Jenks, J. D., Cornely, O. A., Chen, S. C., Thompson, G. R., and Hoenigl, M. (2020). Breakthrough invasive fungal infections: who is at risk? Mycoses 63 (10), 1021–1032. doi:10.1111/myc.13148
Jiang, Y., Lu, R., Zhou, Q., Shen, Y., and Zhu, H. (2024). Analysis of post-market adverse events of istradefylline: a real-world study base on FAERS database. Sci. Rep. 14 (1), 7659. doi:10.1038/s41598-024-58460-6
Jin, H., Wang, T., Falcione, B. A., Olsen, K. M., Chen, K., Tang, H., et al. (2016). Trough concentration of voriconazole and its relationship with efficacy and safety: a systematic review and meta-analysis. J. Antimicrob. Chemother. 71 (7), 1772–1785. doi:10.1093/jac/dkw045
Kearns, G. L., Abdel-Rahman, S. M., Alander, S. W., Blowey, D. L., Leeder, J. S., and Kauffman, R. E. (2003). Developmental pharmacology--drug disposition, action, and therapy in infants and children. N. Engl. J. Med. 349 (12), 1157–1167. doi:10.1056/NEJMra035092
Kim, S. B., Cho, S.-Y., Lee, D.-G., Choi, J.-K., Lee, H.-J., Kim, S.-H., et al. (2017). Breakthrough invasive fungal diseases during voriconazole treatment for aspergillosis: a 5-year retrospective cohort study. Med. Mycol. 55 (3), 237–245. doi:10.1093/mmy/myw067
Leroux, S., Mechinaud-Heloury, F., and Jacqz-Aigrain, E. (2021). Contribution of population pharmacokinetics of glycopeptides and antifungals to dosage adaptation in paediatric onco-hematological malignancies: a review. Front. Pharmacol. 12, 635345. doi:10.3389/fphar.2021.635345
Liu, D., Mao, W., Hu, B., Li, X., Zhao, Q., Zhang, L., et al. (2024). A real-world pharmacovigilance study of polatuzumab vedotin based on the FDA adverse event reporting system (FAERS). Front. Pharmacol. 15, 1405023. doi:10.3389/fphar.2024.1405023
Luong, M.-L., Al-Dabbagh, M., Groll, A. H., Racil, Z., Nannya, Y., Mitsani, D., et al. (2016). Utility of voriconazole therapeutic drug monitoring: a meta-analysis. J. Antimicrob. Chemother. 71 (7), 1786–1799. doi:10.1093/jac/dkw099
Malani, A. N., and Aronoff, D. M. (2008). Voriconazole-induced photosensitivity. Clin. Med. Res. 6 (2), 83–85. doi:10.3121/cmr.2008.806
Mertowska, P., Mertowski, S., Smolak, K., Kita, A., Kita, G., Guz, K., et al. (2023). Immune checkpoint pathway expression in lymphocyte subpopulations in patients with common variable immunodeficiency and chronic lymphocytic leukemia. Cancers (Basel) 15 (21), 5184. doi:10.3390/cancers15215184
Mihăilă, R.-G. (2015). Voriconazole and the liver. World J. Hepatol. 7 (14), 1828–1833. doi:10.4254/wjh.v7.i14.1828
Mounier, A., Agard, E., Douma, I., Chehab, H. E., Vie, A. L., Dot, J. M., et al. (2018). Macular toxicity and blind spot enlargement during a treatment by voriconazole: a case report. Eur. J. Ophthalmol. 28 (4), NP11–NP14. doi:10.1177/1120672117750051
Orssaud, C., Guillemain, R., and Lillo Le Louet, A. (2021). Toxic optic neuropathy due to voriconazole: possible potentiation by reduction of CYP2C19 activity. Eur. Rev. Med. Pharmacol. Sci. 25 (24), 7823–7828. doi:10.26355/eurrev_202112_27628
Pagano, L., Akova, M., Dimopoulos, G., Herbrecht, R., Drgona, L., and Blijlevens, N. (2011). Risk assessment and prognostic factors for mould-related diseases in immunocompromised patients. J. Antimicrob. Chemother. 66 (Suppl. 1), i5–i14. doi:10.1093/jac/dkq437
Papakonstantinou, E., Dragoumani, K., Efthimiadou, A., Palaiogeorgou, A. M., Pierouli, K., Mitsis, T., et al. (2021). Haematological malignancies implications during the times of the COVID-19 pandemic. Oncol. Lett. 22 (6), 856. doi:10.3892/ol.2021.13117
Pasternak, Y., Shechter, N., Loebstein, R., Markovits, N., Gueta, I., Halkin, H., et al. (2019). Voriconazole-induced QTc prolongation in a paediatric population. Acta Paediatr. 108 (6), 1128–1132. doi:10.1111/apa.14652
Purkins, L., Wood, N., Greenhalgh, K., Allen, M. J., and Oliver, S. D. (2003). Voriconazole, a novel wide-spectrum triazole: oral pharmacokinetics and safety. Br. J. Clin. Pharmacol. 56 (Suppl. 1), 10–16. doi:10.1046/j.1365-2125.2003.01993.x
Raisch, D. W., Garg, V., Arabyat, R., Shen, X., Edwards, B. J., Miller, F. H., et al. (2014). Anaphylaxis associated with gadolinium-based contrast agents: data from the Food and Drug Administration’s Adverse Event Reporting System and review of case reports in the literature. Expert Opin. Drug Saf. 13 (1), 15–23. doi:10.1517/14740338.2013.832752
Romão, B. M. S., Duval, F. V., Lima, E. C., da Silva, F. A. B., and de Matos, G. C. (2024). Detection of potential safety signals related to the use of remdesivir and tocilizumab in the COVID era during pregnancy, resorting to open data from the FDA adverse event reporting system (FAERS). Front. Pharmacol. 15, 1349543. doi:10.3389/fphar.2024.1349543
Sakaeda, T., Tamon, A., Kadoyama, K., and Okuno, Y. (2013). Data mining of the public version of the FDA adverse event reporting system. Int. J. Med. Sci. 10 (7), 796–803. doi:10.7150/ijms.6048
Salmanton-García, J., Marchesi, F., Farina, F., Weinbergerová, B., Itri, F., Dávila-Valls, J., et al. (2024). Decoding the historical tale: COVID-19 impact on haematological malignancy patients-EPICOVIDEHA insights from 2020 to 2022. EClinMedicine 71, 102553. doi:10.1016/j.eclinm.2024.102553
Sienkiewicz-Oleszkiewicz, B., Salamonowicz-Bodzioch, M., Słonka, J., and Kałwak, K. (2023). Antifungal drug-drug interactions with commonly used pharmaceutics in European pediatric patients with acute lymphoblastic leukemia. J. Clin. Med. 12 (14), 4637. doi:10.3390/jcm12144637
Swerdlow, S. H., Campo, E., Pileri, S. A., Harris, N. L., Stein, H., Siebert, R., et al. (2016). The 2016 revision of the World Health Organization classification of lymphoid neoplasms. Blood 127 (20), 2375–2390. doi:10.1182/blood-2016-01-643569
Valle-T-Figueras, J. M., Renedo Miró, B., Benítez Carabante, M. I., Díaz-de-Heredia, C., Vima Bofarull, J., Mendoza-Palomar, N., et al. (2021). Voriconazole use in children: therapeutic drug monitoring and control of inflammation as Key points for optimal treatment. J. Fungi 7 (6), 456. doi:10.3390/jof7060456
Wei, X., Zhao, M., Fu, P., and Xiao, X. (2019). Risk factors associated with insufficient and potentially toxic voriconazole plasma concentrations: an observational study. J. Chemother. 31 (7-8), 401–407. doi:10.1080/1120009X.2019.1646974
Wu, J., Chen, N., Yao, Y., Zhou, J., and Zhou, H. (2021). Hyperlipidemia caused by voriconazole: a case report. Infect. Drug Resist. 14, 483–487. doi:10.2147/IDR.S301198
Xie, G.-L., Wang, X.-S., Hu, L.-Y., Wang, Y., Gu, X., and Xu, Y.-Q. (2023). Myelodysplastic syndrome-like response after voriconazole treatment of systemic lupus erythematosus complicated with fungal infection: a case report. Front. Med. (Lausanne) 10, 1286649. doi:10.3389/fmed.2023.1286649
Xiong, S., Gou, R., Liang, X., Wu, H., Qin, S., Li, B., et al. (2024). Adverse events of oral GLP-1 receptor agonist (semaglutide tablets): a real-world study based on FAERS from 2019 to 2023. Diabetes Ther. 15 (8), 1717–1733. doi:10.1007/s13300-024-01594-7
Yasu, T., Nomura, Y., Gando, Y., Matsumoto, Y., Sugita, T., Kosugi, N., et al. (2022). High-performance liquid chromatography for ultra-simple determination of plasma voriconazole concentration. J. Fungi 8 (10), 1035. doi:10.3390/jof8101035
Yu, L., and Liu, L. (2024). Exploration of adverse events associated with risdiplam use: retrospective cases from the US Food and drug administration adverse event reporting system (FAERS) database. PLoS One 19 (3), e0298609. doi:10.1371/journal.pone.0298609
Zhai, Y., Ye, X., Hu, F., Xu, J., Guo, X., Zhuang, Y., et al. (2019). Endocrine toxicity of immune checkpoint inhibitors: a real-world study leveraging US Food and Drug Administration adverse events reporting system. J. Immunother. Cancer 7 (1), 286. doi:10.1186/s40425-019-0754-2
Zhang, W., Wang, Y., Jiang, X., Zhao, H., Jia, X., Wang, Q., et al. (2024). Newly identified adverse events for gemcitabine using the Food and drug administration adverse event reporting system. Expert Opin. Drug Saf. 23 (7), 917–923. doi:10.1080/14740338.2023.2284989
Zheng, R., Li, Y., Guo, C., Pei, Y., Ke, Z., and Huang, L. (2021). Voriconazole induced hallucinations and visual disturbances in a female child: a case report and literature review. Front. Pediatr. 9, 655327. doi:10.3389/fped.2021.655327
Zhou, J., Wei, Z., Xu, B., Liu, M., Xu, R., and Wu, X. (2022). Pharmacovigilance of triazole antifungal agents: analysis of the FDA adverse event reporting system (FAERS) database. Front. Pharmacol. 13, 1039867. doi:10.3389/fphar.2022.1039867
Keywords: data mining, voriconazole, hematological malignant tumor, FAERS, children, adults
Citation: Li H, Jiang M, Pan X and Kong L (2025) Data mining and safety analysis of voriconazole in patients with a hematological malignant tumor based on the FAERS database: differences between children and adults. Front. Pharmacol. 16:1524702. doi: 10.3389/fphar.2025.1524702
Received: 08 November 2024; Accepted: 09 January 2025;
Published: 24 January 2025.
Edited by:
Linan Zeng, McMaster University, CanadaReviewed by:
Daniele Mengato, University Hospital of Padua, ItalyCopyright © 2025 Li, Jiang, Pan and Kong. This is an open-access article distributed under the terms of the Creative Commons Attribution License (CC BY). The use, distribution or reproduction in other forums is permitted, provided the original author(s) and the copyright owner(s) are credited and that the original publication in this journal is cited, in accordance with accepted academic practice. No use, distribution or reproduction is permitted which does not comply with these terms.
*Correspondence: Lingti Kong, a29uZ2xpbmd0aUAxNjMuY29t
Disclaimer: All claims expressed in this article are solely those of the authors and do not necessarily represent those of their affiliated organizations, or those of the publisher, the editors and the reviewers. Any product that may be evaluated in this article or claim that may be made by its manufacturer is not guaranteed or endorsed by the publisher.
Research integrity at Frontiers
Learn more about the work of our research integrity team to safeguard the quality of each article we publish.