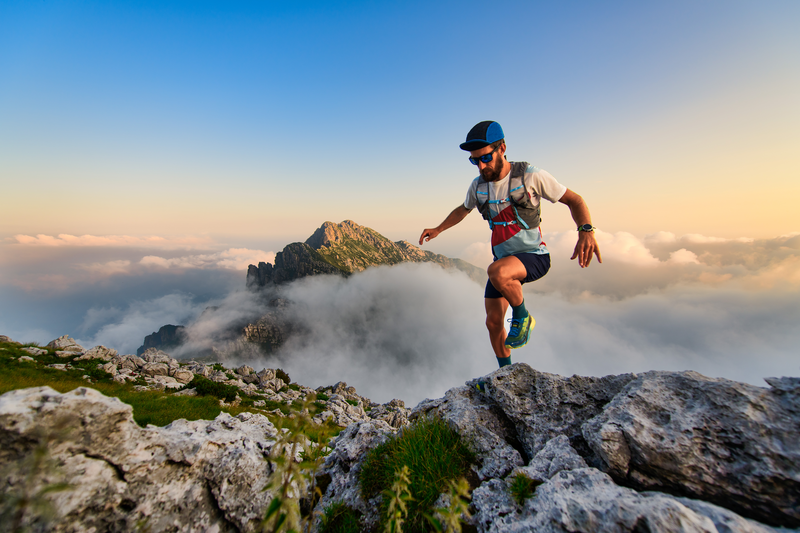
95% of researchers rate our articles as excellent or good
Learn more about the work of our research integrity team to safeguard the quality of each article we publish.
Find out more
REVIEW article
Front. Pharmacol. , 03 March 2025
Sec. Ethnopharmacology
Volume 16 - 2025 | https://doi.org/10.3389/fphar.2025.1521235
This article is part of the Research Topic Reviews in Ethnopharmacology: 2025 View all 10 articles
The Latin name of Wolfiporia cocos is Wolfiporia cocos (F.A. Wolf) Ryvarden & Gilb, it a medicinal and edible mushroom belonging to the family Polyporaceae. Traditional Chinese medicine believes that it can strengthen the spleen, diuretic, tranquillise the mind and dispel dampness. So far, the chemical and active metabolites isolated and extracted from Wolfiporia cocos are mainly polysaccharides, triterpenoids, and sterols. Modern pharmacology has found that these chemical and active metabolites have a wide range of pharmacological effects, including antitumour, antioxidation, anti-inflammatory, immunomodulation, regulation of intestinal flora, regulation of glycolipid metabolism, and improvement of organ function. By applying Poria cocos, Poria, Wolfiporia cocos, Wolfiporia cocos (F.A. Wolf) Ryvarden & Gilb as search terms, we searched all the relevant studies on Poria cocos from Web of Science and PubMed databases and classified these categories of chemical and active metabolites according to the main research content of each literature and summarized its mechanism of action, updated its latest research results, and discussed the direction of further research in the future to provide a better reference for future clinical applications with better therapeutic effects and potential medicinal value.
Wolfiporia cocos (F.A. Wolf) Ryvarden & Gilb. is the current accepted Latin name, and it formerly was known as MacrohyWolfiporia cocos (Schwein.) I. Johans. & Ryvarden., Poria cocos (syn. Wolfiporia cocos), Poria cocos F.A. Wolf, Pachyma cocos (Schwein.) Fr., and Sclerotium cocos Schwein (Li et al., 2022), which is known as “Fuling” in China and is now widely used in China, Japan and other parts of Asia. It is a healthcare edible mushroom belonging to the family Polyporaceae, which grows on the roots of pine trees in China (Nie et al., 2020). Wolfiporia cocos was first recorded in the famous Chinese medical book “Shennong Bencao Jing” and has been used for 2000 years (Li et al., 2019a). It is a kind of traditional Chinese medicine used for both food and medicine, which can strengthen the spleen, diuretic, tranquillize the mind and dispel dampness (Ng et al., 2024). Existing studies have shown that the active metabolites of Wolfiporia cocos are mainly triterpenoids, polysaccharides, sterols, and others, of which the active metabolites have biological functions such as antitumour (Li et al., 2024; Yue et al., 2023), regulation of intestinal flora (Lai et al., 2023), improvement of organ function (Jiang et al., 2022; Wu et al., 2023a), immunomodulation (Zhang W. et al., 2023), anti-inflammatory (Wu et al., 2023b), antioxidation (Fang et al., 2021), and regulation of glycolipid metabolism (Pan et al., 2023). By applying Poria cocos, Poria, Wolfirporia cocos, Wolfiporia cocos (F.A. Wolf) Ryvarden & Gilb as search terms, we searched all the relevant studies on Wolfiporia cocos from Web of Science and PubMed databases and classified these categories of chemical and active metabolites according to the main research content of each literature and summarized its mechanism of action, updated its latest research results, and discussed the direction of further research in the future to provide a better reference for future clinical applications with better therapeutic effects and potential medicinal value.
Polysaccharides refer to a class of high molecular weight metabolites, which are composed of more than 10 monosaccharides and are connected by glycosidic bonds. Wolfiporia cocos polysaccharides, as one of the main active ingredients of Wolfiporia cocos, account for about 84% of the active ingredients in Wolfiporia cocos sclerotia (Li et al., 2019b). Wolfiporia cocos polysaccharides can be divided into two categories based on their structure: glucans and heteropolysaccharides, with heteropolysac-charides mainly consisting of glucose, galactose, and mannose (Huang Q. et al., 2007). Chihara et al. (1970) extracted Pachyman from Wolfiporia cocos, which is mainly composed of β-(1→3)-D-glucan and also contains a small amount of β-(1→6) glycosidic side chains. Narui et al. (Narui et al., 1980) demonstrated through experiments that the structure of Pachyman extracted from Wolfiporia cocos mycelium cultured in the laboratory is almost identical to that extracted from Wolfiporia cocos grown in nature. The research results of Wang et al. (Wang et al., 2004) urther confirmed that the main component of Wolfiporia cocos polysaccharides is β-(1→3)-D-glucan. According to their solubility, Wolfiporia cocos polysaccharides are divided into water soluble polysaccharides (WPCP) whose backbone is composed of (1,6)-α-galactan and (1,3)-β-mannoglucan and alkaline soluble polysaccharides (APCP) whose backbone is composed of (1,3)-β-D-glucan (Zhao et al., 2023). Details are provided in Table 1.
Triterpenoids, as one of the main active ingredients of Wolfiporia cocos, have a basic parent nucleus composed of 30 carbon atoms, and their structure can be regarded as a polymer of six isoprene units (Chen et al., 2018a). So far, more than 100 triterpenes with different skeletons have been discovered, among which pentacyclic triterpenes and tetracyclic triterpenes have the highest content (Andre et al., 2016). The triterpenoids in Wolfiporia cocos are mainly divided into two categories based on their number of rings: tetracyclic triterpenoids and pentacyclic triterpenoids, with tetracyclic triterpenoids dominating. We classified 159 triterpenoids obtained from the literature based on their different molecular backbone characteristics and grouped triterpenoids with similar molecular backbones. Details are provided in Table 2 and Figures 1–5.
Sterol metabolites are a class of steroids, all of which have cyclopentane dihydrophenanthrene as their basic structure and are steroids containing hydroxyl groups (Yalcinkaya et al., 2024). Sterol metabolites mainly contain ergosterol and pregnancy sterols (Chen et al., 2018b). The representative metabolites of ergosterols mainly include ergosta-7.22-dien-3β-ol,ce-revisterol,ergosta-7-en-3β-ol (Jinming et al., 2001), β-sitosterol (Tong et al., 2010) and stigmas-terol (Ni et al., 2019). Representative metabolites of pregnancy sterols include pregn-7-ene-2β,3a,15a,20-tetrol and pregna-7-en-3a,11a,15a,20-quad-roil (Chen et al., 2018b). Details are provided in Table 3.
In addition to polysaccharides, triterpenoids, and sterols, there are also some other types of chemical metabolites in Wolfiporia cocos. Such as tricyclic diterpenes (Shen et al., 2012) and sohiracillinone (Chen et al., 2018a). Organic acids and their esters include protocatechuic acid, palmitic acid, ethyl palmitate, methyl palmitate, trimethyl citrate, dimethyl(R)-malate, di-(2-ethylhexyl) phthalate, dibutyl phthalate, octadecanoic acid, octacosyl acid and pentacosanoic acid (Yang et al., 2019). In addition, 51 proteins were isolated and identified from the fermentation broth of Wolfiporia cocos. Some studies have found that volatile oil metabolites from Wolfiporia cocos (Jie et al., 2014) contain abundant trace elements required by the human body, such as iron, zinc, manganese, potassium, sodium, selenium, calcium and phosphorus. Among them, iron has the highest content, followed by zinc and manganese (Xi and Zhang, 2022).
A large number of studies have found that the anticancer effect of the active ingredients in Wolfiporia cocos on lung cancer (Jiang and Duanmu, 2021), breast cancer (Jeong et al., 2015), gastric cancer (Lu et al., 2018), liver cancer (Huang et al., 2006), pancreatic cancer (Cheng et al., 2013), and kidney cancer (Li et al., 2024) may inhibit tumor cell proliferation and metastasis and induce tumor cell apoptosis by regulating some signal pathways and the expression level of tumor-related cytokines.
Recent pharmacological studies have uncovered the antitumor mechanisms associated with bioactive components derived from Wolfiporia cocos. Pachymic acid (PA) has been shown to disrupt tumor cell architecture and induce apoptosis in renal tumor cells via upregulation of tumor protein p53-inducible nuclear protein 2 (TP53INP2) and tumor necrosis factor receptor-associated factor 6 (TRAF6), alongside activation of pro-apoptotic pathways involving caspase-8, caspase-3, and PARP (Li et al., 2024). Chen et al. (2015) demonstrated that PA inhibits migration and invasion of gallbladder cancer cells in a dose-dependent manner by downregulating tumor-associated proteins including PCNA, ICAM-1, RhoA, p-Akt, and p-ERK1/2, mediated through inhibition of the AKT and ERK pathways. Ling et al. (2011) showed that PA suppresses invasion and metastasis of MDA-MB-231 and MCF-7 breast cancer cells by inhibiting the NF-κB signaling pathway and MMP-9 activity. Wang et al. (2022) demonstrated that PA inhibits gastric cancer (GC) cell viability and proliferation in a concentration-dependent manner. This reduction in GC cell adhesion effectively hampers metastasis and invasion. PA also significantly alters the expression of epithelial-mesenchymal transition (EMT)-related proteins, including E-cadherin, N-cadherin, and Vimentin, while concurrently decreasing the levels of metastasis-related proteins, including matrix metalloproteinases MMP-2 and MMP-9, along with tissue inhibitors of metalloproteinase 1.
Chen et al. (2022) demonstrated that poricoic acid A (PAA) exhibits significant therapeutic effects on T-cell acute lymphoblastic leukemia (T-ALL). Both in vitro and in vivo models showed that PAA markedly reduced T-ALL cell viability, induced G2 phase cell cycle arrest, and triggered apoptosis by exacerbating mitochondrial dysfunction and generating excessive reactive oxygen species (ROS). Additionally, PAA was found to induce autophagy and ferroptosis in T-ALL cells by regulating the AMPK/mTOR and LC3 signaling pathways, thus amplifying its therapeutic effects. Ma et al. (2021a) reported that PAA triggers apoptosis in SKOV3 ovarian cancer cells through mitochondrial and death receptor pathways in a concentration-dependent manner. Its antitumor mechanisms involve inhibition of the mTOR/p70S6K signaling pathway, an increase in LC3-I and LC3-II protein levels, activation of caspase-3, caspase-8, and caspase-9, and modulation of pro-apoptotic and anti-apoptotic protein expression.
Jiang et al. (2022) discovered that Wolfiporia cocos polysaccharides can dose-dependently inhibit the proliferation of lung cancer cells and suppress the migration and invasion of A549 cells by downregulating MMP-2 and MMP-9 through inhibition of the NF-κB signaling pathway. Moreover, neutral polysaccharide metabolites (Chen and Chang, 2004) and triterpenoids (Ukiya et al., 2002) isolated from Wolfiporia cocos have been reported to inhibit the proliferation and differentiation of HL-60 human leukemia cells. Lin et al. (Lin et al., 2020) discovered that the fucose-containing mannoglucan polysaccharide (FMGP) extracted from Wolfiporia cocos significantly inhibits the metastasis of CL1-5 lung cancer cells. FMGP achieves this by inhibiting the TGFβ RI/FAK/AKT signaling pathway and reducing the expression of the metastasis-associated protein Slug. Table 4 summarizes the antitumor bioactivities of Wolfiporia cocos extraction.
The gut microbiota is the largest microbial community in the host’s body, known as the 'invisible organ of the human body'. The metabolic capacity of the human gut microbiota is an important factor in affecting nutrient absorption, immune regulation, the maintenance of health and the triggering of disease (Miao et al., 2016). Studies have demonstrated that carboxymethyl Poria polysaccharides (CMP) extracted from Wolfiporia cocos significantly mitigate colon damage induced by 5-fluorouracil (5-FU). This protective effect is associated with the inhibition of reactive oxygen species (ROS) production, an increase in the levels of catalase (CAT), glutathione peroxidase (GSH Px), and glutathione (GSH), as well as a reduction in the expression of pro-inflammatory markers such as NF-κB, p-p38, and Bax. Simultaneously, CMP enhances the expression of the antioxidant factors Nrf2 and Bcl-2. Moreover, CMP is effective in ameliorating gut microbiota dysbiosis caused by 5-FU, promoting an increase in the proportions of beneficial taxa such as Bacteroidetes, lactobacilli, butyrate-producing bacteria, and acetate-producing bacteria, while restoring overall gut microbiota diversity (Wang et al., 2018). Another investigation indicated that CMP can alleviate the cytotoxic effects of 5-FU, while concurrently enhancing the expression of tight junction proteins and related adhesion molecules, thus strengthening the intestinal barrier against GC (Yin et al., 2022). Yu et al. (2022) reported that Poria cocos polysaccharides (PCP) alleviate Chronic Non-Bacterial Prostatitis by modulating gut microbiota. Notably, after fermentation by the human gut microbiota, there was significant enrichment of Parabacterioides, Fusicatenibacter, and Parasutterella. These bacteria metabolize PCP to produce Haloperidol glucuronide and 7-ketodeoxycholic acid, which promote the expression of Alox15 and Pla2g2f in colon epithelium, while downregulating Cyp1a1 and Hsd17b7, thereby inhibiting inflammatory responses. This suggests that the metabolites Haloperidol glucuronide and 7-ketodeoxycholic acid may act as signaling molecules within the gut-prostate axis.
Lai et al. (2022) found that the water-soluble polysaccharide (PCX), water-insoluble polysaccharide (PCY) and triterpenoid saponin (PCZ) in Poria cocos can increase the number of lactobacilli in the intestine and change the content of short chain peptides in intestinal metabolites. Another study found that PCX, alkali soluble polysaccharide and triterpenoid acids have a protective effect on cisplatin induced intestinal injury, mainly by reducing the relative abundance of pathogenic bacteria such as Proteus mirabilis, cyanobacteria, ruminococcaceae and spirobacteriaceae, and promoting the growth of probiotics such as erysipelotticaae and prevotelacae (Zou et al., 2021). Lai et al. (2023) found that PCX can lower levels of inflammatory cytokines TNF-α and IL-1β, decrease the infiltration of inflammatory cells, and improve intestinal mucosal integrity and barrier function. This was achieved by increasing the relative abundance of beneficial gut microbiota and reducing harmful microbial populations, as manifested by elevated short-chain fatty acid (SCFAs) levels.
Xu et al. (2019) found through experiments that 16α - hydroxytrametinoic acid extracted from Wolfiporia cocos activates glucocorticoid receptor agonists, inhibits the activation of PI3K and Akt, to reduce the phosphorylation of downstream IκB and NF-κB, effectively alleviate TNF - α induced barrier damage in Caco-2 monolayer intestinal epithelial cells. This provides an improved strategy for adjuvant dietary therapy to restore intestinal health. Duan et al. (2023) upregulated the expression of intestinal Occludin and ZO-1, downregulated serum endotoxin, DAO, D-lactate, and intestinal myeloperoxidase (MPO) levels by extracting PCP, enhanced intestinal physical barrier, and increased the expression of MUC2, β-resistin, and SIgA in intestinal tissue, to enhance intestinal biochemical barrier. This indicates that PCP can be used as a functional food to regulate intestinal mucosal function, thereby improving the health of the intestine and host. Moreover, research has found that PCP can not only improve intestinal mucosal barrier function but also increase the diversity of intestinal microbiota to improve antibiotic associated diarrhea in mice (Xu et al., 2023). Table 5 summarizes the bioactivities of Wolfiporia cocos extraction in regulating of intestinal flora.
Oxidation refers to the chemical reaction process between substances and oxygen, oxidative stress is a pathological state in which the redox homeostasis of an organism is imbalanced. It arises from the excessive production of reactive nitrogen species and ROS by the organism when subjected to external or internal stimuli, thereby breaking the original dynamic balance mechanism (Tabei et al., 2023). There are reports proving that supplementing exogenous antioxidants can eliminate free radicals and delay disease progression (Rahbari et al., 2015). However, artificially synthesized antioxidants are harmful to human health, such as liver damage and gout (Wang et al., 2016). Therefore, in this era of pursuing health and wellness, it is necessary to develop natural antioxidants to replace the current artificially synthesized antioxidants.
Recent experimental results have shown that the antioxidant capacity of hydroxymethyl PCP derivatives (PCP-C1, PCP-C2, PCP-C3) is directly related to the degree of carboxymethylation. The results showed that these derivatives possessed free radical scavenging and ferrous ion chelating efficacy, among which PCP-C3 protected renal cells from oxalate-induced oxidative damage, increased cell viability and antioxidant enzyme activities, and reduced the accumulation of harmful oxidative stress products. This suggests that PCP-C3 is a potential anticholinergic drug with great potential (Li CY. et al., 2021). Zhao et al. (2020) found that PCP effectively alleviated oxidative stress induced by oxidised low-density lipoprotein (oxLDL) by decreasing ROS and malondialdehyde (MDA) levels in vascular smooth muscle cells, while increasing superoxide dismutase (SOD) activity. By activating the ERK1/2 signalling pathway, the translocation of Nrf2 and the expression of heme oxygenase-1 were promoted, and the upregulation of Lectin-like oxidised LDL receptor-1 (LOX-1) was inhibited to reduce the uptake of oxLDL, which enhanced the antioxidant capacity of the cells. Fang et al. (2021) found that Wolfiporia cocos extract significantly reduced oxidative stress caused by ROS such as hydrogen peroxide, thereby inhibiting the activity of matrix metalloproteinases and reducing the degradation of collagen. At the same time, it can also upregulate the level of transforming growth factor beta 1 (TGF-β1), promote the regeneration and repair of skin cells, enhance the expression of antioxidant related proteins, and further enhance the antioxidant capacity of skin. This indicates that Wolfiporia cocos extract effectively delays the process of skin aging, providing the strong scientific basis for the development of new anti-aging cosmetics.
Wu et al. (2020) demonstrated through experiments that PCP has significant reducing and good scavenging abilities against DPPH, superoxide anions and hydroxyl radical and may be one of the main material bases for its antioxidant properties. Tang et al. (2014) found that PCP derivatives (PCP-1, PCP-2, and PCP-3) exhibit the ability to scavenge hydroxyl radicals and ABTS radicals, and they function through chelation of ferrous ions, thereby reducing the concentration of free ferrous ions and inhibiting oxidative stress responses. Xu et al. (2020) found that Wolfiporia cocos, an ingredient in Bajitianwan (BJTW), can reduce malondialdehyde (MDA) levels in the brain while simultaneously increasing the concentrations of catalase (CAT) and glutathione peroxidase (GSH Px) in serum. This dual action not only mitigates oxidative stress but also facilitates the upregulation of Forkhead box O1 (FoxO1) expression in bone tissue and enhances the levels of superoxide dismutase 2 (SOD2), thereby providing protection to both the bone and nervous system from oxidative damage. This suggests that BJTW has great potential in the treatment of Alzheimer’s disease and osteoporosis. Table 6 summarizes the bioactivities of Wolfiporia cocos extraction in antioxidation.
Inflammatory responses are known to be present in various disease processes. A study reported that CMP could regulate the balance of pro-inflammatory and anti-inflammatory cytokines in intestinal tissues by decreasing the expression of pro-inflammatory cytokines (TNF-α, IL-1β, IL-6) and increasing the levels of anti-inflammatory cytokines (IL-10, TGF-β), significantly preventing inflammatory bowel disease in mice (Liu et al., 2018). Song et al. (2018) found that PCP inhibits RANKL induced osteoclastogenesis by suppressing the activity of NFATc1 and the phosphorylation of ERK and STAT3. This suggests that PCP prevents and attenuates pathological fractures caused by bone resorption by interfering with the signalling pathway, decreasing osteoclast differentiation, and reducing bone resorption. Wu et al. (2022) established a fungal infection-induced peritonitis (FIP) mouse model and observed that polysaccharide compounds significantly alleviated inflammatory infiltration and cellular apoptosis in the thymus and spleen tissues. This effect is attributed to the reduction of inflammatory cytokines such as TNF-α, IL-6, and IL-1β, effectively ameliorating the inflammatory response. Additionally, PCP was found to decrease the levels of oxidative stress markers, including malondialdehyde (MDA) and myeloperoxidase (MPO), thereby mitigating oxidative damage. Wang et al. (2024) established a mouse model of bleomycin (BLM)-induced pulmonary fibrosis and found that PA inhibited BLM-induced increases in NLRP3, ASC, IL-1 β, P20, and TXNIP, decreased the levels of pro-inflammatory factors (IL -6 and TNF- α), and increased the level of the anti-inflammatory cytokine IL-10 in mouse lung tissue. It also reduced the levels of hydroxyproline and MDA in lung tissue and increased the activities of superoxide dismutase and glutathione peroxidase.
Li W. et al. (2021) explored the potential protective mechanism of PCP on compulsory spondylitis by establishing in the ApoE−/− mice model induced by high-fat diet, and found that PCP can inhibit the increase of serum inflammatory mediators and blood lipids. Through experiments, it was found that PCP can significantly reduce the release of inflammatory mediators TNF - α, IL-6, and NO in serum, thereby protecting blood vessels from inflammatory invasion and reducing the elevation of low-density lipoprotein cholesterol, triglycerides, and total cholesterol in blood lipids. It also inhibits the activation of TLR4/NF-κB pathway in the aorta and blocks the expression of MMP-2 and ICAM-1. This indicates that PCP can intervene in ankylosing spondylitis by reducing inflammatory factors and blood lipid levels. Gui et al. (2021) conducted experiments by establishing a mouse model of fecal - induced peritonitis. They discovered that PA effectively ameliorated the pathological changes in the lung tissue of rats with pneumonia. This was achieved by inhibiting the activation of the NF-κB and MAPK signaling pathways, thereby reducing the release of inflammatory cytokines. Simultaneously, PA could also inhibit cell apoptosis, which further protected the damaged tissues and promoted the resolution of inflammation. These findings revealed the therapeutic potential of PA in inflammatory diseases and provided a scientific basis for the development of new anti-inflammatory drugs. Wu et al. (2023b) established a mouse model of osteoarthritis (OA) and found that PA promotes the expression of SIRT6, which inhibits the activation of the NF-κB signaling pathway. This modulation leads to a reduction in the production of inflammatory mediators such as inducible nitric oxide synthase (iNOS) and prostaglandin E2 (PGE2), as well as the suppression of IL-1β-induced inflammatory responses. Additionally, PA was found to reverse the abnormal upregulation of matrix metalloproteinase-3 and platelet-activating factor-5 in OA chondrocytes, while also downregulating the expression of type II collagen and aggrecan. These findings indicate that PA holds significant potential for the treatment of osteoarthritis. Table 7 summarizes the bioactivities of Wolfiporia cocos extraction in anti-inflammatory.
Wolfiporia cocos has immunomodulatory effects, and its extract can be used as a natural immune agent. There are reports indicating that PCP can increase NO by activating the Ca (2+)/PKC/p38/NF - κ B signalling pathway, TNF-α, IL-1β, IL-6 and intracellular calcium level, thereby enhancing the immune response of RAW 264.7 macrophages (Pu et al., 2019). Liu et al. (2021) found that Wolfiporia cocos derivatives CMP-1 and CMP-2 have a triple helix structure, which can improve the secretion of NO, TNF - α, and IL-6 by increasing the expression of iNOS, TNF–α and IL-6 mRNA, and enhance the immune function of RAW 264.7 macrophages.
Liu et al. (2020) established a model of anthrax protective antigen (APA) by extracting polysaccharide PCP-I from Wolfiporia cocos as an immune adjuvant. They found that PCP-I not only significantly enhanced anthrax specific anti APA antibodies, toxin neutralizing antibodies, anti-APA antibody affinity, as well as IgG1 and IgG2a levels, but also increased the frequency of APA specific memory B cells, increased the proliferation of PA specific spleen cells, significantly stimulated IL-4 secretion, enhanced the activation of dendritic cells in vitro, and improved the survival rate of mice immunized with anthrax lethal toxins. This indicates that polysaccharide PCP-I extracted from Wolfiporia cocos can activate immune signalling pathways, trigger immune synergy, and provide more effective immune responses. PCP-I is a very promising immune adjuvant. Chao et al. (2021) discovered that tumulosic acid, poronic acid C, and three-epi dehydrotumulosic acid—components of lanostane triterpenoids extracted from Wolfiporia cocos—can significantly stimulate the secretion of IFN-γ by mouse spleen cells. Concurrently, these lanostane triterpenoids activate natural killer cells, enhancing non-specific (innate) immunity and promoting the Th1 immune response, which leads to increased IFN-γ secretion. Additionally, they reduce the secretion of IL-4 and IL-5, cytokines associated with allergic reactions and the Th2 immune response. This research demonstrates that extracts from Wolfiporia cocos have the ability to modulate the Th1/Th2 immune response, potentially reducing the incidence of allergic diseases and positioning them as promising candidates for the development of anti-allergic therapies.
Liu et al. (2022) found that PCP significantly increased the activity of four enzymes related to immunity and energy metabolism (phenoloxidase, glucose-6-phosphate dehydrogenase, hexokinase, and fatty acid synthase), thereby significantly enhancing the cellular immunity of silkworms, including the ability of hemocyte phagocytosis, microaggregation and spreading. This indicates that PCP can regulate the immune system by enhancing cellular immunity, modulating immune responses, and regulating the expression levels of physiological metabolism related genes. Zhang W. et al. (2023) found that the polysaccharides PCWPW and PCWPS from Wolfiporia cocos contain some fucose and mannose residues, which could interact with mannose receptor on the surface of macrophages. By experimentally treating the polysaccharides PCWPW and PCWPS with the inhibitors, the secretion of TNFα was inhibited and NF-κB and MAP. Table 8 summarizes the bioactivities of Wolfiporia cocos extraction in immunomodulation.
Wolfiporia cocos regulates metabolism mainly by regulating glucose and lipid metabolism disorders. Glucose metabolism is a complex process of sugar synthesis and decomposition in the body, and abnormal enzymes and other factors involved in synthesis and metabolism will lead to glucose metabolism disorders (Zhang et al., 2022). Genetic, environmental, or pathological conditions can lead to abnormal levels of blood lipids and lipoproteins, resulting in lipid metabolism (Badmus et al., 2022). Studies have shown that crude extracts of Wolfiporia cocos and its triterpenoids such as dehydrotumulosic acid, dehydrotrametinonic acid and pachymic acid can significantly reduce postprandial blood glucose in db/db mice. Further studies on a mouse model treated with streptozotocin showed that the crude extract of Wolfiporia cocos and triterpenoids exhibited insulin sensitizing activity, but not insulin releasing activity. This suggests that the active ingredients of Wolfiporia cocos may enhance insulin sensitivity through a pathway that is not dependent on PPAR-γ, thereby reducing blood glucose levels (Li et al., 2011).
Hyperlipidemia is an important factor leading to atherosclerosis. Some experimental studies have proved that after treatment with Wolfiporia cocos, hyperlipidemia and related lipid metabolite abnormalities were significantly improved (Miao et al., 2016). Kim et al. (2019) found that Poria cocos Wolf (PCW) extract can effectively improve liver steatosis. In vitro HepG2 cell experiments and in vivo high-fat diet mouse models, it was found that PCW can significantly reduce triglyceride levels in cells and mouse liver while affecting the expression of genes related to fat production, fatty acid oxidation, endoplasmic reticulum stress, and autophagy. PCW reduces fat production and promotes fatty acid oxidation by activating AMPK and its downstream pathways while inhibiting endoplasmic reticulum stress and inducing autophagy. These findings indicate that Wolfiporia cocos has the potential to be used for the treatment of hepatic steatosis. Sun et al. (2019) found that PCX extracted from the sclerotia of Wolfiporia cocos can significantly enhance glucose and lipid metabolism, as well as reduce liver steatosis in ob/ob mice. The mechanism of action for PCX involves increasing the abundance of butyrate-producing bacteria in the intestine, which in turn elevates intestinal butyrate levels, enhances the integrity of the intestinal mucosa, and activates the intestinal PPAR-γ pathway. Zhu et al. (2022) by establishing a high-fat diet (HFD) - induced obese mouse model, it was found that Wolfiporia cocos oligosaccharides(PCO) can reverse the imbalance of gut microbiota and changes in microbial metabolites, repair the intestinal barrier, reduce hyperglycemia, glucose tolerance, and insulin resistance in HFD mice, decrease the size of adipocytes, inhibit fat accumulation, and improve the disorder of glucose and lipid metabolism. This indicates that PCO, as a novel prebiotic, has great potential in the treatment of glucose and lipid metabolism diseases. Wang et al. (2023) found that CMP can significantly reduce fat weight and serum lipids, improve glucose tolerance, effectively reduce lipid droplet content in liver tissue, and promote cholesterol and lipid metabolism by reducing the synthesis of liver bile acids. They also found that CMP regulates the metabolism of glucose and lipid and energy balance by enhancing the abundances of Bifidobacterium, Bacteroides, and Akkermansia intestinal microbiota. Pan et al. (2023) found that Wolfiporia cocos acid can alleviate lipid metabolism disorders in mouse primary liver cells induced by OA-palmitic acid by activating SIRT6 signalling pathway. By using molecular docking, it was found that SIRT6/PPAR - α can promote fatty acid oxidation and SIRT6/Nrf2 can enhance antioxidant activity. The interaction between the two is a new target for the treatment of non-alcoholic fatty liver disease. Table 9 summarizes the bioactivities of Wolfiporia cocos extraction in regulating of glycolipid metabolism.
Through research, it has been found that the active ingredients in Wolfiporia cocos have the ability to improve the function of human organs such as the heart (Xie et al., 2023), liver (Jiang et al., 2022) and kidneys (Wu et al., 2023a). Table 10 summarizes the bioactivities of Wolfiporia cocos extraction in improving of organ function.
A study has reported that by establishing a myocardial ischemia (MI/RI) rat model, Wolfiporia cocos polysaccharides reduce the levels of LDH, CK-MB, IL-1 β, IL-18, and MDA in myocardial tissue. At the same time, they reduce the relative expression levels of Bax, cleaved caspase-3, RhoA, ROCK1, and p-MYPT-1 proteins, as well as increase the relative expression levels of SOD and Bcl-2 proteins in myocardial tissue, thereby improving tissue edema and microcirculation disorders, and weakening pathological damage and myocardial cell apoptosis. Meanwhile, by downregulating the levels of RhoA, ROCK1, and downstream signalling factor p-MYPT-1 in MI/RI rat myocardial tissue, the activation of the Rho ROCK signalling pathway is inhibited, the activation of inflammasomes is reduced, and myocardial cell oxidation and inflammatory damage are alleviated, thereby reducing myocardial cell apoptosis (Xie et al., 2023). Liu et al. (2023) found that the triterpenoid compound PA extracted from Wolfiporia cocos can reduce the levels of IL-1 β, IL-6, and TNF-α by inhibiting the pro-inflammatory NF-κB signalling pathway, thereby improving hematopoietic shock (HS) - induced cardiac inflammation. Coincidentally, PA weakens the increase in HS induced cardiac monocyte/macrophage and neutrophil infiltration, as well as inhibits HS induced M1 polarization and exaggerates M2 polarization in myocardial tissue, reducing cardiac damage, inhibiting cell apoptosis, and improving cardiac inflammatory response. Li et al. (2015) found that PA exhibited significant effects in inhibiting lipopolysaccharide (LPS) - induced apoptosis and inflammatory response in H9c2 cardiomyocytes. Through PA treatment, the upregulation and release of TNF-α, IL -1, and IL-6 inflammatory factors in myocardial cells can be significantly reduced. At the same time, PA inhibits LPS induced myocardial cell apoptosis by suppressing the phosphorylation of extracellular regulated kinase (Erk) 1/2 and p38 signalling pathways. This discovery suggests that PA may be a potentially effective drug for treating LPS induced myocarditis and apoptosis, providing a new strategy for treating inflammation related cardiovascular diseases.
In the early stages, research on carboxy methyl Poria cocos polysaccharide (CMPCP) for chronic viral hepatitis has been conducted. Through experiments, it was found that CMPCP can improve liver function and enhance non-specific cell-mediated immune function, without cytotoxic effects. This study was a preliminary investigation of the use of Wolfiporia cocos in the treatment of liver diseases (Guo et al., 1984). With the constant evolution of social times, pressures and other factors have led to an increasing intake of alcohol, gradually making alcoholic liver disease (ALD) the leading chronic liver disease worldwide, placing a heavy burden on the global public health system (Zhang N. et al., 2023). There are research reports that the active Poria cocos polysaccharide (PCP-1C) improves ALD by inhibiting the TLR4/NF-κB and CYP2E1/ROS/MAPK pathways, repairing the intestinal barrier and reducing LPS leakage, thereby reducing liver injury, inflammation, oxidative stress, and intestinal leakage (Jiang et al., 2022). Tan et al. (2022) established a non-alcoholic steatohepatitis (NASH) model by administering methionine and choline deficiency diet to C57BL/6 mice for 4 weeks. They found that Wolfiporia cocos polysaccharides can reshape the composition of intestinal bacteria by significantly increasing the relative abundance of Faecalibaculum and reducing the endotoxin load level from intestinal bacteria. This suggests that Wolfiporia cocos polysaccharides can provide a new potential strategy for the prevention and treatment of NASH. Wu et al. (2019) demonstrated through experiments that PCP can reduce Hsp90 cells, be beneficial for acetaminophen-induced liver cell damage, and enhance its hepatoprotective effect. PCP (Wu et al., 2018) can alleviate liver injury in a dose-dependent manner by downregulating the expression of NF-κB/p65 and IkB α.
Chen et al. (2023) found that inducing renal interstitial fibrosis in rats or mice by establishing unilateral ureteral obstruction (UUO), and PAA from Wolfiporia cocos can promote β-catenin K49 deacetylation, significantly inhibit renal fiber cell activation, and improve renal function. At the same time, Wu et al. (2023a) by establishing a model of diabetes nephropathy (DKD) and extracting PAA from Wolfiporia cocos, found that PAA can significantly reduce the levels of blood sugar and urinary protein in mice, control renal fibrosis, and downregulate FUNDC1 to promote mitosis, thus having a beneficial impact on the damage of capsular cells in DKD and effectively alleviating renal damage. There is experimental evidence (Li Q. et al., 2021) that PAA inhibits the PDGF-C, Smad3, and MAPK pathways to suppress TGF-β1 induced ECM accumulation, fibrosis formation, and proliferation in renal fibroblasts. Fu et al. (2022) found that Wolfiporia cocos polysaccharides can not only induce proliferation and differentiation of bone marrow mesenchymal stem cells, but also reduce the level of pro-inflammatory cytokines to improve kidney morphology, thereby improving chronic kidney disease. Younis et al. (2022) found through experiments that PA has an upregulation effect on renal klotho, thereby inhibiting Wnt/β - catenin reactivation and downregulating RAS gene expression, which brings benefits to the treatment of chronic kidney disease (CKD). At the same time, Wang et al. (2017) confirmed that Poricoic acid ZA extracted from Wolfiporia cocos is used as a renin-angiotensin system inhibitor for the treatment of CKD. It blocks the interaction between Smad2/3-TGF β RI proteins and inhibits Smad2/3 phosphorylation, thereby inhibiting RAS the TGF - β/Smad pathway, ultimately leading to the treatment of chronic kidney disease.
The “Shennong Bencao Jing” describes the traditional Chinese medicine derived from Wolfiporia cocos as being “sweet, smooth, and devoid of toxicity.” Modern studies have confirmed that the hydroalcoholic extract of Wolfiporia cocos has oral and topical anti-inflammatory activity in mice. Two metabolites isolated from it showed strong inhibitory effects and low toxicity on acute TPA edema, and the safe dosage is 6–18 g (Cuellar et al., 1997). The toxicological properties of the water-soluble heteropolysaccharide ac - PCM0 from Wolfiporia cocos were investigated by in vivo acute toxicity test and comparative experiments. The heteropolysaccharide solution with a concentration of 50 mg/mL was intravenously injected into BALB/C mice weighing 201 g. The toxicity and mortality were recorded for seven consecutive days. The LD50 of the polysaccharide was calculated to be higher than 1,250 mg/kg, indicating that the polysaccharide is non-toxic (Zhang et al., 2005). An in vivo toxicity assay was conducted to evaluate the potential toxicity of PAA during the treatment of T-ALL. T-ALL nude mice were randomly divided into three groups: control group, PAA low dose group (5 mg/kg) and PAA high dose group (10 mg/kg); NOD/SCID mice were divided into corresponding control group and PAA treatment group. The PAA treatment group was given an intraperitoneal injection, and the control group was given the same amount of solvent (physiological saline). After 4 weeks of treatment, it was detected that PAA had no significant effect on the levels of alt, AST, bun and Cr in serum. This indicates that PAA has no significant hepatotoxicity or nephrotoxicity (Chen et al., 2022).
In recent years, Wolfiporia cocos has attracted more and more attention from researchers, and many studies have also confirmed its medicinal value. In terms of active ingredients, polysaccharides and terpenoids have been the main research objects. Although researchers have made great efforts in elucidating their chemical structures and biological activities, there are still some limitations. As far as polysaccharides are concerned, the purification process is still a formidable challenge. Most natural polysaccharides are insoluble in water. Researchers mostly use crude extracts or derivatives, which makes the fine structure of polysaccharides unclear, and hinders the accurate understanding of its mechanism of action to a certain extent. It is hoped that the fine structure of Wolfiporia cocos polysaccharide can be described through more advanced technology improvement in the future. On the other hand, the terpenoids in Wolfiporia cocos are mainly triterpenoids, and also contain trace diterpenes. Most of the current research focuses on triterpenoids, while the research on diterpenes is relatively scarce. In the future, if the research on diterpenes can be strengthened, it may open up a new research path for revealing the pharmacological activity of Wolfiporia cocos, and provide a richer scientific basis for the in-depth development and wide application of Wolfiporia cocos.
Wolfiporia cocos, as a traditional Chinese medicine with a wide range of pharmacological mechanisms, has demonstrated in vitro and in vivo experiments the potential for a wide range of applications such as antitumour, antioxidation, anti-inflammatory, immunomodulation, regulation of intestinal flora, regulation of glycolipid metabolism, and improvement of organ function. As shown in Figure 6. In vitro experiments showed that Wolfiporia cocos extracts have antitumour, antioxidation, anti-inflammatory and immunomodulation activities. In the in vivo model, the extract showed antitumour, regulation of intestinal flora, regulation of glycolipid metabolism, and improvement of organ function. Although in vitro experimental studies can precisely regulate the experimental conditions and thus obtain preliminary conclusions faster on the basis of controlled variables, it is difficult to comprehensively simulate the complexity of the in vivo environment, and it is more general for elucidating the mechanism of action of Wolfiporia cocos extracts in detail. As for in vivo experiments, more experiments are currently using mouse models to simulate human beings, although there are many similarities between mice and human beings in physiological mechanisms, mice are still unable to fully reflect the complexity of the human body in vivo. In the future, it is necessary to strengthen clinical research to promote Wolfiporia cocos from the laboratory to clinical application, so that it can truly benefit human health.
In conclusion, in order to provide inspiration for the further study of Wolfiporia cocos, this paper summarizes the research status of Wolfiporia cocos in chemistry, active ingredients and pharmacological mechanism. Although Wolfiporia cocos has shown significant application potential in many fields, its complex biological activity mechanism and fine chemical structure characteristics still need to be further explored and established, so as to fully explore its value in the development of functional food additives and drugs. On this basis, we suggest that the use of modern biotechnology, chemical analysis and computer science and other technologies, in-depth excavation of polysaccharide and terpenoids derivatives and other potential active ingredients in Wolfiporia cocos. Through this way, it is not only expected to find more new compounds with unique biological activities, but also further expand the application scope of Wolfiporia cocos in medicine, food, health products and other fields, laying a solid foundation for the maximum utilization of Wolfiporia cocos resources. We look forward to more researchers joining the research of Wolfiporia cocos in the future to jointly promote the modernization process of this traditional Chinese medicine.
QX: Conceptualization, Writing–original draft. ZL: Conceptualization, Methodology, Writing–review and editing. DY: Project administration, Writing–review and editing. XL: Writing–review and editing, Supervision. WP: Data curation, Writing–review and editing. XY: Software, Writing–review and editing. KJ: Data curation, Writing–review and editing. XW: Supervision, Visualization, Writing–review and editing. YZ: Funding acquisition, Supervision, Writing–review and editing.
The author(s) declare that financial support was received for the research, authorship, and/or publication of this article. This study was supported by the project of Health Digital Research Association of Jilin Province (HDRA.J20230002) and the Program of Science and Technology Research Project of the Education Department of Jilin Province of China (JJKH20241091KJ).
We thank the project of Health Digital Research Association of Jilin Province (HDRA.J20230002) and the Program of Science and Technology Research Project of the Education Department of Jilin Province of China (JJKH20241091KJ) for financial support of this study. The authors would like to thank Figdraw for providing the drawing platform.
Author XW was employed by Jilin Aodong Pharmaceutial Group Co., Ltd.
The remaining authors declare that the research was conducted in the absence of any commercial or financial relationships that could be construed as a potential conflict of interest.
The author(s) declare that no Generative AI was used in the creation of this manuscript.
All claims expressed in this article are solely those of the authors and do not necessarily represent those of their affiliated organizations, or those of the publisher, the editors and the reviewers. Any product that may be evaluated in this article, or claim that may be made by its manufacturer, is not guaranteed or endorsed by the publisher.
Akihisa, T., Mizushina, Y., Ukiya, M., Oshikubo, M., Tai, T., Kimura, Y., et al. (2004). Dehydrotrametenonic acid and dehydroeburiconic acid from Poria cocos and their inhibitory effects on eukaryotic DNA polymerase α and β. Biosci. Biotechnol. and Biochem. 68 (2), 448–450. doi:10.1271/bbb.68.448
Akihisa, T., Nakamura, Y., Tokuda, H., Uchiyama, E., Suzuki, T., Kimura, Y., et al. (2007). Triterpene acids from Poria cocos and their anti-tumor-promoting effects. J. Nat. Prod. 70 (6), 948–953. doi:10.1021/np0780001
Akihisa, T., Uchiyama, E., Kikuchi, T., Tokuda, H., Suzuki, T., and Kimura, Y. (2009). Anti-tumor-promoting effects of 25-methoxyporicoic acid A and other triterpene acids from Poria cocos. J. Nat. Prod. 72 (10), 1786–1792. doi:10.1021/np9003239
Andre, C. M., Legay, S., Deleruelle, A., Nieuwenhuizen, N., Punter, M., Brendolise, C., et al. (2016). Multifunctional oxidosqualene cyclases and cytochrome P450 involved in the biosynthesis of apple fruit triterpenic acids. New Phytol. 211 (4), 1279–1294. doi:10.1111/nph.13996
Badmus, O. O., Hillhouse, S. A., Anderson, C. D., Hinds, T. D., and Stec, D. E. (2022). Molecular mechanisms of metabolic associated fatty liver disease (MAFLD): functional analysis of lipid metabolism pathways. Clin. Sci. (Lond) 136 (18), 1347–1366. doi:10.1042/cs20220572
Cai, T. G., and Cai, Y. (2011). Triterpenes from the fungus Poria cocos and their inhibitory activity on nitric oxide production in mouse macrophages via blockade of activating protein-1 pathway. Chem. Biodivers. 8 (11), 2135–2143. doi:10.1002/cbdv.201100013
Chao, C. L., Huang, H. W., Su, M. H., Lin, H. C., and Wu, W. M. (2021). The lanostane triterpenoids in Poria cocos play beneficial roles in immunoregulatory activity. Life (Basel) 11 (2), 111. doi:10.3390/life11020111
Chen, B., Zhang, J., Han, J., Zhao, R., Bao, L., Huang, Y., et al. (2019). Lanostane triterpenoids with glucose-uptake-stimulatory activity from peels of the cultivated edible mushroom Wolfiporia cocos. J. Agric. Food Chem. 67 (26), 7348–7364. doi:10.1021/acs.jafc.9b02606
Chen, D. Q., Chen, L., Guo, Y., Wu, X. Q., Zhao, T. T., Zhao, H. L., et al. (2023). Poricoic acid A suppresses renal fibroblast activation and interstitial fibrosis in UUO rats via upregulating Sirt3 and promoting β-catenin K49 deacetylation. Acta Pharmacol. Sin. 44 (5), 1038–1050. doi:10.1038/s41401-022-01026-x
Chen, L., Fang, W., Liu, J., Qi, X., Zhao, L., Wang, Y., et al. (2022). Poricoic acid A (PAA) inhibits T-cell acute lymphoblastic leukemia through inducing autophagic cell death and ferroptosis. Biochem. Biophys. Res. Commun. 608, 108–115. doi:10.1016/j.bbrc.2022.03.105
Chen, T., Hua, L., Chou, G., Mao, X., and Zou, X. (2018a). A unique naphthone derivative and a rare 4,5-seco-Lanostane triterpenoid from Poria cocos. Molecules 23 (10), 2508. doi:10.3390/molecules23102508
Chen, T., Kan, Y. J., Chou, G. X., and Zhang, C. G. (2018b). A new highly oxygenated pregnane and two new 5-hydroxymethylfurfural derivatives from the water decoction of Poria cocos. J. Asian Nat. Prod. Res. 20 (12), 1101–1107. doi:10.1080/10286020.2017.1383398
Chen, Y., Lian, P., Liu, Y., and Xu, K. (2015). Pachymic acid inhibits tumorigenesis in gallbladder carcinoma cells. Int. J. Clin. Exp. Med. 8 (10), 17781–17788.
Chen, Y. Y., and Chang, H. M. (2004). Antiproliferative and differentiating effects of polysaccharide fraction from fu-ling (Poria cocos) on human leukemic U937 and HL-60 cells. Food Chem. Toxicol. 42 (5), 759–769. doi:10.1016/j.fct.2004.01.018
Cheng, S., Eliaz, I., Lin, J., Thyagarajan-Sahu, A., and Sliva, D. (2013). Triterpenes from Poria cocos suppress growth and invasiveness of pancreatic cancer cells through the downregulation of MMP-7. Int. J. Oncol. 42 (6), 1869–1874. doi:10.3892/ijo.2013.1902
Cheng, S., Swanson, K., Eliaz, I., McClintick, J. N., Sandusky, G. E., and Sliva, D. (2015). Pachymic acid inhibits growth and induces apoptosis of pancreatic cancer in vitro and in vivo by targeting ER stress. PLoS One 10 (4), e0122270. doi:10.1371/journal.pone.0122270
Cheng, Y., Xie, Y., Ge, J. C., Wang, L., Peng, D. Y., Yu, N. J., et al. (2021). Structural characterization and hepatoprotective activity of a galactoglucan from Poria cocos. Carbohydr. Polym. 263, 117979. doi:10.1016/j.carbpol.2021.117979
Chihara, G., Hamuro, J., Maeda, Y., Arai, Y., and Fukuoka, F. (1970). Antitumor polysaccharide derived chemically from natural glucan (pachyman). Nature 225 (5236), 943–944. doi:10.1038/225943a0
Cuellar, M. J., Giner, R. M., Recio, M. C., Just, M. J., Mañez, S., and Rios, J. L. (1997). Effect of the basidiomycete Poria cocos on experimental dermatitis and other inflammatory conditions. Chem. and Pharm. Bull. 45 (3), 492–494. doi:10.1248/cpb.45.492
Dianpeng, L., Xiaoyan, L., Haishan, C., Yongxin, W., and Chongren, Y. (1998). Studies on the chemical constituents from the surface layer of Yunnan^s Poria cocos. Guangxi Zhiwu 18.
Dong, H., Wu, P., Yan, R., Xu, Q., Li, H., Zhang, F., et al. (2015). Enrichment and separation of antitumor triterpene acids from the epidermis of Poria cocos by pH-zone-refining counter-current chromatography and conventional high-speed counter-current chromatography. J. Sep. Sci. 38 (11), 1977–1982. doi:10.1002/jssc.201500077
Dong, H. J., Xue, Z. Z., Geng, Y. L., Wang, X., and Yang, B. (2017). Lanostane triterpenes isolated from epidermis of Poria cocos. Phytochem. Lett. 22, 102–106. doi:10.1016/j.phytol.2017.09.018
Duan, Y., Huang, J., Sun, M., Jiang, Y., Wang, S., Wang, L., et al. (2023). Poria cocos polysaccharide improves intestinal barrier function and maintains intestinal homeostasis in mice. Int. J. Biol. Macromol. 249, 125953. doi:10.1016/j.ijbiomac.2023.125953
Fan, Y., Sun, L., and Li, D. (2021). Effects of porinic acid on proliferation, apoptosis and cell cycle of tongue squamous cell carcinoma cells CAL-27. Chin. Pat. drug 43 (07), 1909–1914.
Fang, C. L., Paul, C. R., Day, C. H., Chang, R. L., Kuo, C. H., Ho, T. J., et al. (2021). Poria cocos (Fuling) targets TGFβ/Smad7 associated collagen accumulation and enhances Nrf2-antioxidant mechanism to exert anti-skin aging effects in human dermal fibroblasts. Environ. Toxicol. 36 (5), 729–736. doi:10.1002/tox.23075
Fu, M., Wang, L., Wang, X., Deng, B., Hu, X., and Zou, J. (2018). Determination of the five main terpenoids in different tissues of Wolfiporia cocos. Molecules 23 (8), 1839. doi:10.3390/molecules23081839
Fu, Y., Yu, D., Xie, X., Huang, Y., and Li, S. (2022). Protective role of Poria cocos polysaccharide induced differentiation of bone marrow mesenchymal stem cells in chronic kidney disease. Curr. Top. nutraceutical Res. (1), 20.
Gao, A. H., Zhang, L., Chen, X., Chen, Y., Xu, Z. Z., Liu, Y. N., et al. (2015). Inhibition of ovarian cancer proliferation and invasion by pachymic acid. Int. J. Clin. Exp. Pathol. 8 (2), 2235–2241.
Gapter, L., Wang, Z., Glinski, J., and Ng, K. Y. (2005). Induction of apoptosis in prostate cancer cells by pachymic acid from Poria cocos. Biochem. Biophys. Res. Commun. 332 (4), 1153–1161. doi:10.1016/j.bbrc.2005.05.044
Gui, Y., Sun, L., Liu, R., and Luo, J. (2021). Pachymic acid inhibits inflammation and cell apoptosis in lipopolysaccharide (LPS)-induced rat model with pneumonia by regulating NF-κB and MAPK pathways. Allergol. Immunopathol. Madr. 49 (5), 87–93. doi:10.15586/aei.v49i5.468
Guo, D. Z., Yao, P. Z., Fan, X. C., and Zhang, H. Z. (1984). Preliminary observation on carboxyl-methyl Poria cocos polysaccharide (CMPCP) in treating chronic viral hepatitis. J. Tradit. Chin. Med. 4 (4), 282.
Hu, K., Luo, Q., Zhu, X. F., Sun, S. H., Feng, G. L., Liu, Z. Y., et al. (2019). Isolation of homogeneous polysaccharide from Poria cocos and effect of its sulfated derivatives on migration of human breast cancer MDA-MB-231 cells. Zhongguo Zhong Yao Za Zhi 44 (13), 2835–2840. doi:10.19540/j.cnki.cjcmm.20190321.305
Huang, Q., Jin, Y., Zhang, L., Cheung, P. C. K., and Kennedy, J. F. (2007a). Structure, molecular size and antitumor activities of polysaccharides from Poria cocos mycelia produced in fermenter. Carbohydr. Polym. 70 (3), 324–333. doi:10.1016/j.carbpol.2007.04.015
Huang, Q., Zhang, L., Cheung, P. C. K., and Tan, X. (2006). Evaluation of sulfated α-glucans from Poria cocos mycelia as potential antitumor agent. Carbohydr. Polym. 64 (2), 337–344. doi:10.1016/j.carbpol.2005.12.001
Huang, Q. L., Jin, Y., Zhang, L., Cheung, P. C. K., and Kennedy, J. F. (2007b). Structure, molecular size and antitumor activities of polysaccharides from Poria cocos mycelia produced in fermenter. Carbohydr. Polym. 70 (3), 324–333. doi:10.1016/j.carbpol.2007.04.015
Hui, L. I., Shuai, H., Lianhai, S., and Xianli, Z. (2016). Study on triterpenoid acid constituents from the surface layer of Poria cocos. West China Journal of Pharmaceutical Sciences.
Jeong, J. W., Lee, W. S., Go, S. I., Nagappan, A., Baek, J. Y., et al. (2015). Pachymic acid induces apoptosis of EJ bladder cancer cells by DR5 up-regulation, ROS generation, modulation of bcl-2 and IAP family members. Phytother. Res. 29 (10), 1516–1524. doi:10.1002/ptr.5402
Jiang, H. Y., and Duanmu, Z. (2021). “Inhibitory effect of Poria cocos polysaccharides on proliferation, migration, and invasion of lung cancer cells, A549,” in Current topics in nutraceutical research.
Jiang, Y., and Fan, L. (2020). Evaluation of anticancer activities of Poria cocos ethanol extract in breast cancer: in vivo and in vitro, identification and mechanism. J. Ethnopharmacol. 257, 112851. doi:10.1016/j.jep.2020.112851
Jiang, Y. H., Wang, L., Chen, W. D., Duan, Y. T., Sun, M. J., Huang, J. J., et al. (2022). Poria cocos polysaccharide prevents alcohol-induced hepatic injury and inflammation by repressing oxidative stress and gut leakiness. Front. Nutr. 9, 963598. doi:10.3389/fnut.2022.963598
Jie, Z., Jianhua, L., Chen, W., et al. (2014). Chemical constituents of volatile oil from poriae cutis. Chin. J. Exp. Formulary 20 (18), 66–69. doi:10.13422/j.cnki.syfjx.2014180066
Jin, Y., Zhang, L., Chen, L., Chen, Y., Cheung, P. C., and Chen, L. (2003b). Effect of culture media on the chemical and physical characteristics of polysaccharides isolated from Poria cocos mycelia. Carbohydr. Res. 338 (14), 1507–1515. doi:10.1016/s0008-6215(03)00197-6
Jin, Y., Zhang, L., Zhang, M., Chen, L., Cheung, P. C. K., Oi, V. E. C., et al. (2003a). Antitumor activities of heteropolysaccharides of Poria cocos mycelia from different strains and culture media. Carbohydr. Res. 338 (14), 1517–1521. doi:10.1016/s0008-6215(03)00198-8
Jinming, G., Lin, H., and Jikai, L. (2001). A novel sterol from Chinese truffles Tuber indicum. Steroids 66 (10), 771–775. doi:10.1016/s0039-128x(01)00105-2
Kanayama, H., Adachi, N., and Togami, M. (1983). A new antitumor polysaccharide from the mycelia of Poria cocos wolf. Chem. and Pharm. Bull. 31 (3), 1115–1118. doi:10.1248/cpb.31.1115
Kim, J. H., Sim, H. A., Jung, D. Y., Lim, E. Y., Kim, Y. T., Kim, B. J., et al. (2019). Poria cocus wolf extract ameliorates hepatic steatosis through regulation of lipid metabolism, inhibition of ER stress, and activation of autophagy via AMPK activation. Int. J. Mol. Sci. 20 (19), 4801. doi:10.3390/ijms20194801
Lai, K. H., Lu, M. C., Du, Y. C., El-Shazly, M., Wu, T. Y., Hsu, Y. M., et al. (2016). Cytotoxic lanostanoids from Poria cocos. J. Nat. Prod. 79 (11), 2805–2813. doi:10.1021/acs.jnatprod.6b00575
Lai, Y., Deng, H., Fang, Q., Ma, L., Lei, H., Guo, X., et al. (2023). Water-insoluble polysaccharide extracted from Poria cocos alleviates antibiotic-associated diarrhea based on regulating the gut microbiota in mice. Foods 12 (16), 3080. doi:10.3390/foods12163080
Lai, Y., Yu, H., Deng, H., Fang, Q., Lei, H., Liu, L., et al. (2022). Three main metabolites from Wolfiporia cocos (F. A. Wolf) Ryvarden and Gilb regulate the gut microbiota in mice: a comparative study using microbiome-metabolomics. Front. Pharmacol. 13, 911140. doi:10.3389/fphar.2022.911140
Lee, D., Lee, S., Shim, S. H., Lee, H. J., Choi, Y., Jang, T. S., et al. (2017a). Protective effect of lanostane triterpenoids from the sclerotia of Poria cocos Wolf against cisplatin-induced apoptosis in LLC-PK1 cells. Bioorg Med. Chem. Lett. 27 (13), 2881–2885. doi:10.1016/j.bmcl.2017.04.084
Lee, K. Y., and Jeon, Y. J. (2003). Polysaccharide isolated from Poria cocos sclerotium induces NF-kappaB/Rel activation and iNOS expression in murine macrophages. Int. Immunopharmacol. 3 (10-11), 1353–1362. doi:10.1016/s1567-5769(03)00113-9
Lee, S., Choi, E., Yang, S. M., Ryoo, R., Moon, E., Kim, S. H., et al. (2018). Bioactive compounds from sclerotia extract of Poria cocos that control adipocyte and osteoblast differentiation. Bioorg. Chem. 81, 27–34. doi:10.1016/j.bioorg.2018.07.031
Lee, S., Lee, D., Lee, S. O., Ryu, J. Y., Choi, S. Z., Kang, K. S., et al. (2017b). Anti-inflammatory activity of the sclerotia of edible fungus, Poria cocos Wolf and their active lanostane triterpenoids. J. Funct. Foods 32, 27–36. doi:10.1016/j.jff.2017.02.012
León, F., Quintana, J., Rivera, A., Estévez, F., and Bermejo, J. (2004). Lanostanoid triterpenes from Laetiporus sulphureus and apoptosis induction on HL-60 human myeloid leukemia cells. J. Nat. Prod. 67 (12), 2008–2011. doi:10.1021/np049762o
Li, C. Y., Liu, L., Zhao, Y. W., Chen, J. Y., Sun, X. Y., and Ouyang, J. M. (2021a). Inhibition of calcium oxalate formation and antioxidant activity of carboxymethylated Poria cocos polysaccharides. Oxid. Med. Cell Longev. 2021, 6653593. doi:10.1155/2021/6653593
Li, F. F., Yuan, Y., Liu, Y., Wu, Q. Q., Jiao, R., Yang, Z., et al. (2015). Pachymic acid protects H9c2 cardiomyocytes from lipopolysaccharide-induced inflammation and apoptosis by inhibiting the extracellular signal-regulated kinase 1/2 and p38 pathways. Mol. Med. Rep. 12 (2), 2807–2813. doi:10.3892/mmr.2015.3712
Li, G., Xu, M. L., Lee, C. S., Woo, M. H., Chang, H. W., and Son, J. K. (2004). Cytotoxicity and DNA topoisomerases inhibitory activity of constituents from the sclerotium of Poria cocos. Archives pharmacal Res. 27 (8), 829–833. doi:10.1007/bf02980174
Li, L., Zuo, Z. T., and Wang, Y. Z. (2022). The traditional usages, chemical components and pharmacological activities of Wolfiporia cocos: a review. Am. J. Chin. Med. 50 (2), 389–440. doi:10.1142/s0192415x22500161
Li, Q., Ming, Y., Jia, H., and Wang, G. (2021c). Poricoic acid A suppresses TGF-β1-induced renal fibrosis and proliferation via the PDGF-C, Smad3 and MAPK pathways. Exp. Ther. Med. 21 (4), 289. doi:10.3892/etm.2021.9720
Li, S., Zhang, J., Li, S., Liu, C., Liu, S., and Liu, Z. (2017). Extraction and separation of lactate dehydrogenase inhibitors from Poria cocos (Schw.) Wolf based on a hyphenated technique and in vitro methods. J. Sep. Sci. 40 (8), 1773–1783. doi:10.1002/jssc.201700054
Li, T. H., Hou, C. C., Chang, C. L., and Yang, W. C. (2011). Anti-hyperglycemic properties of crude extract and triterpenes from Poria cocos. Evid. Based Complement. Altern. Med. 2011doi, 128402. doi:10.1155/2011/128402
Li, W., Yu, J., Zhao, J., Xiao, X., Zang, L., et al. (2021b). Poria cocos polysaccharides reduces high-fat diet-induced arteriosclerosis in ApoE(-/-) mice by inhibiting inflammation. Phytother. Res. 35 (4), 2220–2229. doi:10.1002/ptr.6980
Li, X., He, A., Liu, C., Li, Y., Luo, Y., Xiong, W., et al. (2024). Pachymic acid activates TP53INP2/TRAF6/caspase-8 pathway to promote apoptosis in renal cell carcinoma cells. Environ. Toxicol. doi:10.1002/tox.24195
Li, X., He, Y., Zeng, P., Liu, Y., Zhang, M., Hao, C., et al. (2019a). Molecular basis for Poria cocos mushroom polysaccharide used as an antitumour drug in China. J. Cell Mol. Med. 23 (1), 4–20. doi:10.1111/jcmm.13564
Li, X., Ma, L., and Zhang, L. (2019b). Molecular basis for Poria cocos mushroom polysaccharide used as an antitumor drug in China. Prog. Mol. Biol. Transl. Sci. 163, 263–296. doi:10.1016/bs.pmbts.2019.02.011
Li, Y. R., Liu, S. T., Gan, Q., Zhang, J., Chen, N., Han, C. F., et al. (2023). Four polysaccharides isolated from Poria cocos mycelium and fermentation broth supernatant possess different activities on regulating immune response. Int. J. Biol. Macromol. 226, 935–945. doi:10.1016/j.ijbiomac.2022.12.077
Lin, T. Y., Lu, M. K., and Chang, C. C. (2020). Structural identification of a fucose-containing 1,3-β-mannoglucan from Poria cocos and its anti-lung cancer CL1-5 cells migration via inhibition of TGFβR-mediated signaling. Int. J. Biol. Macromol. 157, 311–318. doi:10.1016/j.ijbiomac.2020.04.014
Ling, H., Zhang, Y., Ng, K. Y., and Chew, E. H. (2011). Pachymic acid impairs breast cancer cell invasion by suppressing nuclear factor-κB-dependent matrix metalloproteinase-9 expression. Breast Cancer Res. Treat. 126 (3), 609–620. doi:10.1007/s10549-010-0929-5
Ling, H., Zhou, L., Jia, X., Gapter, L. A., Agarwal, R., and Ng, K. Y. (2009). Polyporenic acid C induces caspase-8-mediated apoptosis in human lung cancer A549 cells. Mol. Carcinog. 48 (6), 498–507. doi:10.1002/mc.20487
Liu, F., Liu, Y., Feng, X., Ibrahim, S. A., and Huang, W. (2021). Structure characterization and in vitro immunomodulatory activities of carboxymethyl pachymaran. Int. J. Biol. Macromol. 178, 94–103. doi:10.1016/j.ijbiomac.2021.02.046
Liu, J., Hong, W., Li, M., Xiao, Y., Yi, Y., Liu, Y., et al. (2022). Transcriptome analysis reveals immune and metabolic regulation effects of Poria cocos polysaccharides on Bombyx mori larvae. Front. Immunol. 13, 1014985. doi:10.3389/fimmu.2022.1014985
Liu, K., Yin, Y., Zhang, J., Zai, X., Li, R., Ma, H., et al. (2020). Polysaccharide PCP-I isolated from Poria cocos enhances the immunogenicity and protection of an anthrax protective antigen-based vaccine. Hum. Vaccin Immunother. 16 (7), 1699–1707. doi:10.1080/21645515.2019.1675457
Liu, X., Wang, X., Xu, X., and Zhang, X. (2019). Purification, antitumor and anti-inflammation activities of an alkali-soluble and carboxymethyl polysaccharide CMP33 from Poria cocos. Int. J. Biol. Macromol. 127, 39–47. doi:10.1016/j.ijbiomac.2019.01.029
Liu, X., Yu, X., Xu, X., Zhang, X., and Zhang, X. (2018). The protective effects of Poria cocos-derived polysaccharide CMP33 against IBD in mice and its molecular mechanism. Food Funct. 9 (11), 5936–5949. doi:10.1039/c8fo01604f
Liu, Z., Zhou, W., Liu, Q., Huan, Z., Wang, Q., and Ge, X. (2023). Pachymic acid prevents hemorrhagic shock-induced cardiac injury by suppressing M1 macrophage polarization and NF-[Formula: see text]B signaling pathway. Am. J. Chin. Med. 51 (8), 2157–2173. doi:10.1142/s0192415x23500921
Lu, C., Cai, D., and Ma, J. (2018). Pachymic acid sensitizes gastric cancer cells to radiation therapy by upregulating Bax through hypoxia. Am. J. Chin. Med. 46 (4), 875–890. doi:10.1142/s0192415x18500465
Ma, C., Lu, J., Ren, M., Wang, Q., Li, C., Xi, X., et al. (2023). Rapid identification of α-glucosidase inhibitors from Poria using spectrum-effect, component knock-out, and molecular docking technique. Front. Nutr. 10, 1089829. doi:10.3389/fnut.2023.1089829
Ma, J., Liu, J., Lu, C., and Cai, D. (2015). Pachymic acid induces apoptosis via activating ROS-dependent JNK and ER stress pathways in lung cancer cells. Cancer Cell Int. 15, 78. doi:10.1186/s12935-015-0230-0
Ma, R., Zhang, Z., Xu, J., Liang, X., and Zhao, Q. (2021a). Poricoic acid A induces apoptosis and autophagy in ovarian cancer via modulating the mTOR/p70s6k signaling axis. 54, e11183, doi:10.1590/1414-431X2021e11183
Ma, R., Zhang, Z., Xu, J., Liang, X., and Zhao, Q. (2021b). Poricoic acid A induces apoptosis and autophagy in ovarian cancer via modulating the mTOR/p70s6k signaling axis. Braz J. Med. Biol. Res. 54 (12), e11183. doi:10.1590/1414-431X2021e11183
Miao, H., Zhao, Y. H., Vaziri, N. D., Tang, D. D., Chen, H., Chen, H., et al. (2016). Lipidomics biomarkers of diet-induced hyperlipidemia and its treatment with Poria cocos. J. Agric. Food Chem. 64 (4), 969–979. doi:10.1021/acs.jafc.5b05350
Mizushina, Y., Akihisa, T., Ukiya, M., Murakami, C., Kuriyama, I., Xu, X., et al. (2004). A novel DNA topoisomerase inhibitor: dehydroebriconic acid, one of the lanostane-type triterpene acids from Poria cocos. Cancer Sci. 95 (4), 354–360. doi:10.1111/j.1349-7006.2004.tb03215.x
Narui, T., Takahashi, K., Kobayashi, M., and Shibata, S. (1980). A polysaccharide produced by laboratory cultivation of Poria cocos Wolf. Carbohydr. Res. 87 (1), 161–163. doi:10.1016/s0008-6215(00)85202-7
Ng, C. Y. J., Lai, N. P. Y., Ng, W. M., Siah, K. T. H., Gan, R. Y., and Zhong, L. L. D. (2024). Chemical structures, extraction and analysis technologies, and bioactivities of edible fungal polysaccharides from Poria cocos: an updated review. Int. J. Biol. Macromol. 261 (Pt 1), 129555. doi:10.1016/j.ijbiomac.2024.129555
Ni, F., Xie, X., Wen, J., et al. (2019). Non-polysaccharide chemical constituents of Poria cocos and their anti-complementary activity. Chin. Herb. Med. 50 (11), 2529–2533.
Nie, A., Chao, Y., Zhang, X., Jia, W., Zhou, Z., and Zhu, C. (2020). Phytochemistry and pharmacological activities of Wolfiporia cocos (F.A. Wolf) ryvarden and Gilb. Front. Pharmacol. 11, 505249. doi:10.3389/fphar.2020.505249
Nukaya, H., Yamashiro, H., Fukazawa, H., Ishida, H., and Tsuji, K. (1996). Isolation of inhibitors of TPA-induced mouse ear edema from Hoelen, Poria cocos. Chem. and Pharm. Bull. 44 (4), 847–849. doi:10.1248/cpb.44.847
Pan, Z. S., Chen, Y. L., Tang, K. J., Liu, Z. Z., Liang, J. L., Guan, Y. H., et al. (2023). Pachymic acid modulates sirtuin 6 activity to alleviate lipid metabolism disorders. Exp. Ther. Med. 26 (1), 320. doi:10.3892/etm.2023.12019
Pu, Y., Liu, Z., Tian, H., and Bao, Y. (2019). The immunomodulatory effect of Poria cocos polysaccharides is mediated by the Ca(2+)/PKC/p38/NF-κB signaling pathway in macrophages. Int. Immunopharmacol. 72, 252–257. doi:10.1016/j.intimp.2019.04.017
Rahbari, M., Diederich, K., Becker, K., Krauth-Siegel, R. L., and Jortzik, E. (2015). Detection of thiol-based redox switch processes in parasites - facts and future. Biol. Chem. 396 (5), 445–463. doi:10.1515/hsz-2014-0279
Shen, L., and Weng, D. (2020). Porialic acid regulated and controlled survival and apoptosis in cervical cancer cells through Wnt signaling pathway by inhibiting TRIM29 expression. J. Guangzhou Univ. Traditional Chin. Med. 37 (01), 140–146. doi:10.13359/j.cnki.gzxbtcm.2020.01.026
Shen, Y., Li, J., and Jia, X. (2012). Research progress on chemical constituents of Poria. J. Nanjing Univ. Traditional Chin. Med. 28 (03), 297–300. doi:10.14148/j.issn.1672-0482.2012.03.030
Song, D., Cao, Z., Tickner, J., Qiu, H., Wang, C., Chen, K., et al. (2018). Poria cocos polysaccharide attenuates RANKL-induced osteoclastogenesis by suppressing NFATc1 activity and phosphorylation of ERK and STAT3. Arch. Biochem. Biophys. 647, 76–83. doi:10.1016/j.abb.2018.04.011
Sun, S. S., Wang, K., Ma, K., Bao, L., and Liu, H. W. (2019). An insoluble polysaccharide from the sclerotium of Poria cocos improves hyperglycemia, hyperlipidemia and hepatic steatosis in ob/ob mice via modulation of gut microbiota. Chin. J. Nat. Med. 17 (1), 3–14. doi:10.1016/s1875-5364(19)30003-2
Tabei, Y., Abe, H., Suzuki, S., Takeda, N., Arai, J. I., and Nakajima, Y. (2023). Sedanolide activates KEAP1-NRF2 pathway and ameliorates hydrogen peroxide-induced apoptotic cell death. Int. J. Mol. Sci. 24 (22), 16532. doi:10.3390/ijms242216532
Tai, T., Akahori, A., and Shingu, T. (1993). Triterpenes of Poria cocos. Phytochemistry 32 (5), 1239–1244. doi:10.1016/s0031-9422(00)95099-4
Tai, T., Shingu, T., Kikuchi, T., Tezuka, Y., and Akahori, A. (1995). Triterpenes from the surface layer of Poria cocos. Phytochemistry 39 (5), 1165–1169. doi:10.1016/0031-9422(95)00110-s
Tan, Y. Y., Yue, S. R., Lu, A. P., Zhang, L., Ji, G., Liu, B. C., et al. (2022). The improvement of nonalcoholic steatohepatitis by Poria cocos polysaccharides associated with gut microbiota and NF-κB/CCL3/CCR1 axis. Phytomedicine 103, 154208. doi:10.1016/j.phymed.2022.154208
Tang, J., Nie, J., Li, D., Zhu, W., Zhang, S., Ma, F., et al. (2014). Characterization and antioxidant activities of degraded polysaccharides from Poria cocos sclerotium. Carbohydr. Polym. 105, 121–126. doi:10.1016/j.carbpol.2014.01.049
Tong, X. G., Liu, J. L., and Cheng, Y. X. (2010). A new pregnane steroid from Poria cum radix pini. J. Asian Nat. Prod. Res. 12 (5), 419–423. doi:10.1080/10286021003762010
Ukiya, M., Akihisa, T., Tokuda, H., Hirano, M., Oshikubo, M., Nobukuni, Y., et al. (2002). Inhibition of tumor-promoting effects by poricoic acids G and H and other lanostane-type triterpenes and cytotoxic activity of poricoic acids A and G from Poria cocos. J. Nat. Prod. 65 (4), 462–465. doi:10.1021/np0103721
Wang, C., Yang, S., Gao, L., Wang, L., and Cao, L. (2018). Carboxymethyl pachyman (CMP) reduces intestinal mucositis and regulates the intestinal microflora in 5-fluorouracil-treated CT26 tumour-bearing mice. Food Funct. 9 (5), 2695–2704. doi:10.1039/c7fo01886j
Wang, G., Chen, X., Sun, C., et al. (2023). Gut microbiota and metabolite insights into anti-obesity effect of carboxymethyl pachymaran in high-fat diet mice. Article. J. Funct. Foods, 111105898. doi:10.1016/j.jff.2023.105898
Wang, H., Luo, Y., Chu, Z., Ni, T., Ou, S., Dai, X., et al. (2022). Poria acid, triterpenoids extracted from Poria cocos, inhibits the invasion and metastasis of gastric cancer cells. Molecules 27 (11), 3629. doi:10.3390/molecules27113629
Wang, L. Y., Wan, H. J., Chen, L. X., Zhang, H. M., and Yu, Z. Y. (1993). Studies on chemical constituents from solvent extracts of Poria cocos (Schw.) Wolf. Zhongguo Zhong Yao Za Zhi 18 (10), 613–614, 639.
Wang, M. (2019). Effective constituents and mechanism of the surface layer of Poria cocos against renal fibrosis.
Wang, M., Chen, D. Q., Wang, M. C., Chen, H., Chen, L., Liu, D., et al. (2017). Poricoic acid ZA, a novel RAS inhibitor, attenuates tubulo-interstitial fibrosis and podocyte injury by inhibiting TGF-β/Smad signaling pathway. Phytomedicine 36, 243–253. doi:10.1016/j.phymed.2017.10.008
Wang, N., Zhang, Y., Wang, X., Huang, X., Fei, Y., Yu, Y., et al. (2016). Antioxidant property of water-soluble polysaccharides from Poria cocos Wolf using different extraction methods. Int. J. Biol. Macromol. 83, 103–110. doi:10.1016/j.ijbiomac.2015.11.032
Wang, S., Tan, W., Zhang, L., and Jiang, H. (2024). Pachymic acid protects against bleomycin-induced pulmonary fibrosis by suppressing fibrotic, inflammatory, and oxidative stress pathways in mice. Appl. Biochem. Biotechnol. 196 (6), 3344–3355. doi:10.1007/s12010-023-04686-5
Wang, Y., Zhang, M., Ruan, D., Shashkov, A. S., Kilcoyne, M., Savage, A. V., et al. (2004). Chemical components and molecular mass of six polysaccharides isolated from the sclerotium of Poria cocos. Carbohydr. Res. 339 (2), 327–334. doi:10.1016/j.carres.2003.10.006
Wen, H., Wu, Z., Hu, H., Wu, Y., Yang, G., Lu, J., et al. (2018). The anti-tumor effect of pachymic acid on osteosarcoma cells by inducing PTEN and Caspase 3/7-dependent apoptosis. J. Nat. Med. 72 (1), 57–63. doi:10.1007/s11418-017-1117-2
Wu, K., Fan, J., Huang, X., Wu, X., and Guo, C. (2018). Hepatoprotective effects exerted by Poria cocos polysaccharides against acetaminophen-induced liver injury in mice. Int. J. Biol. Macromol. 114, 137–142. doi:10.1016/j.ijbiomac.2018.03.107
Wu, K., Guo, C., Yang, B., Wu, X., and Wang, W. (2019). Antihepatotoxic benefits of Poria cocos polysaccharides on acetaminophen-lesioned livers in vivo and in vitro. J. Cell Biochem. 120 (5), 7482–7488. doi:10.1002/jcb.28022
Wu, P., Tan, H., Zhan, J., Wang, W., Hu, T., and Li, S. (2020). Optimization of bioprocess extraction of Poria cocos polysaccharide (PCP) with Aspergillus Niger β-glucanase and the evaluation of PCP antioxidant property. Molecules 25 (24), 5930. doi:10.3390/molecules25245930
Wu, Y., Deng, H., Sun, J., Tang, J., Li, X., and Xu, Y. (2023a). Poricoic acid A induces mitophagy to ameliorate podocyte injury in diabetic kidney disease via downregulating FUNDC1. J. Biochem. Mol. Toxicol. 37 (12), e23503. doi:10.1002/jbt.23503
Wu, Y., Li, D., Wang, H., and Wan, X. (2022). Protective effect of Poria cocos polysaccharides on fecal peritonitis-induced sepsis in mice through inhibition of oxidative stress, inflammation, apoptosis, and reduction of treg cells. Front. Microbiol. 13, 887949. doi:10.3389/fmicb.2022.887949
Wu, Y., Li, S., Li, H., Zhao, C., Ma, H., Zhao, X., et al. (2016). Effect of a polysaccharide from Poria cocos on humoral response in mice immunized by H1N1 influenza and HBsAg vaccines. Int. J. Biol. Macromol. 91, 248–257. doi:10.1016/j.ijbiomac.2016.05.046
Wu, Y., Ying, J., Zhu, X., Xu, C., and Wu, L. (2023b). Pachymic acid suppresses the inflammatory response of chondrocytes and alleviates the progression of osteoarthritis via regulating the Sirtuin 6/NF-κB signal axis. Int. Immunopharmacol. 124 (Pt A), 110854. doi:10.1016/j.intimp.2023.110854
Xi, Z., and Zhang, Y. (2022). The content of heavy metals and trace element was determined in Poria. Electron. J. Mod. Med. Health Res. 6 (23), 18–20.
Xie, J., Wang, Y. Y., Li, J. X., and Gao, F. M. (2023). Effect and mechanism of Poria cocos polysaccharides on myocardial cell apoptosis in rats with myocardial ischemia-reperfusion injury by regulating Rho-ROCK signaling pathway. Zhongguo Zhong Yao Za Zhi 48 (23), 6434–6441. doi:10.19540/j.cnki.cjcmm.20230816.401
Xu, H., Wang, S., Jiang, Y., Wu, J., Chen, L., Ding, Y., et al. (2023). Poria cocos polysaccharide ameliorated antibiotic-associated diarrhea in mice via regulating the homeostasis of the gut microbiota and intestinal mucosal barrier. Int. J. Mol. Sci. 24 (2), 1423. doi:10.3390/ijms24021423
Xu, H., Wang, Y., Jurutka, P. W., Wu, S., Chen, Y., Cao, C., et al. (2019). 16α-Hydroxytrametenolic acid from Poria cocos improves intestinal barrier function through the glucocorticoid receptor-mediated PI3K/Akt/NF-κB pathway. J. Agric. Food Chem. 67 (39), 10871–10879. doi:10.1021/acs.jafc.9b04613
Xu, W., Liu, X., He, X., Jiang, Y., Zhang, J., Zhang, Q., et al. (2020). Bajitianwan attenuates D-galactose-induced memory impairment and bone loss through suppression of oxidative stress in aging rat model. J. Ethnopharmacol. 261, 112992. doi:10.1016/j.jep.2020.112992
Yalcinkaya, A., Öztaş, Y. E., and Sabuncuoğlu, S. (2024). Sterols in inflammatory diseases: implications and clinical utility. Adv. Exp. Med. Biol. 1440, 261–275. doi:10.1007/978-3-031-43883-7_13
Yang, C. H., Zhang, S. F., Liu, W. Y., Zhang, Z. J., and Liu, J. H. (2009). Two new triterpenes from the surface layer of Poria cocos. Helvetica Chim. Acta 92, 660–667. doi:10.1002/hlca.200800360
Yang, P. F., Liu, C., Wang, H. Q., Li, J. C., Wang, Z. Z., Xiao, W., et al. (2014). Chemical constituents of Poria cocos. Zhongguo Zhong yao za zhi = Zhongguo zhongyao zazhi = China J. Chin. materia medica 39 (6), 1030–1033.
Yaoita, Y., Kikuchi, M., and Kikuchi, M. (2002). Sterol constituents from Poria cocos. Nat. Med. 56.
Yasukawa, K., Kaminaga, T., Kitanaka, S., Tai, T., Nunoura, Y., Natori, S., et al. (1998). 3 beta-p-hydroxybenzoyldehydrotumulosic acid from Poria cocos, and its anti-inflammatory effect. Phytochemistry 48 (8), 1357–1360. doi:10.1016/s0031-9422(97)01063-7
Yang, P. F., Tao, H., Dong, W., Zhi-Wei, Z., Gao-Lei, X., and Zhi-Fei, C. (2019). Phytochemical and chemotaxonomic study of Poria cocos (schw.) wolf. Biochem. Syst. Ecol. 83, 54–56. doi:10.1016/j.bse.2019.01.002
Yin, L., Huang, G., Khan, I., Su, L., Xia, W., Law, B. Y. K., et al. (2022). Poria cocos polysaccharides exert prebiotic function to attenuate the adverse effects and improve the therapeutic outcome of 5-FU in Apc(Min/+) mice. Chin. Med. 17 (1), 116. doi:10.1186/s13020-022-00667-8
Younis, N. N., Mohamed, H. E., Shaheen, M. A., Abdelghafour, A. M., and Hammad, S. K. (2022). Potential therapeutic efficacy of pachymic acid in chronic kidney disease induced in rats: role of Wnt/β-catenin/renin-angiotensin axis. J. Pharm. Pharmacol. 74 (1), 112–123. doi:10.1093/jpp/rgab129
Yu, J., Hu, Q., Liu, J., Luo, J., Liu, L., and Peng, X. (2022). Metabolites of gut microbiota fermenting Poria cocos polysaccharide alleviates chronic nonbacterial prostatitis in rats. Int. J. Biol. Macromol. 209 (Pt B), 1593–1604. doi:10.1016/j.ijbiomac.2022.04.029
Yue, S., Feng, X., Cai, Y., Ibrahim, S. A., Liu, Y., and Huang, W. (2023). Regulation of tumor apoptosis of poriae cutis-derived lanostane triterpenes by AKT/PI3K and MAPK signaling pathways in vitro. Nutrients 15 (20), 4360. doi:10.3390/nu15204360
Zhang, L., Chen, L., Xu, X., Zeng, F., and Cheung, P. C. (2005). Effect of molecular mass on antitumor activity of heteropolysaccharide from Poria cocos. Biosci. Biotechnol. Biochem. 69 (3), 631–634. doi:10.1271/bbb.69.631
Zhang, M., Chiu, L. C., Cheung, P. C., and Ooi, V. E. (2006). Growth-inhibitory effects of a beta-glucan from the mycelium of Poria cocos on human breast carcinoma MCF-7 cells: cell-cycle arrest and apoptosis induction. Oncol. Rep. 15 (3), 637–643.
Zhang, N., Xue, F., Wu, X.-N., Zhang, W., Hou, J. J., Xiang, J. X., et al. (2023b). The global burden of alcoholic liver disease: a systematic analysis of the global burden of disease study 2019. Alcohol Alcohol. 58 (5), 485–496. doi:10.1093/alcalc/agad046
Zhang, W., He, J., Zheng, D., Zhao, P., Wang, Y., Zhao, J., et al. (2023a). Immunomodulatory activity and its mechanisms of two polysaccharides from Poria cocos. Molecules 29 (1), 50. doi:10.3390/molecules29010050
Zhang, W., Jia, X., Xu, Y., Xie, Q., Zhu, M., Zhang, H., et al. (2022). Effects of coix seed extract, Bifidobacterium BPL1, and their combination on the glycolipid metabolism in obese mice. Front. Nutr. 9, 939423. doi:10.3389/fnut.2022.939423
Zhang, Y. H., Zhang, Y., Li, X. Y., Feng, X. D., Jian, W., and Li, R. Q. (2017). Antitumor activity of the pachymic acid in nasopharyngeal carcinoma cells. Ultrastruct. Pathol. 41 (3), 245–251. doi:10.1080/01913123.2017.1296522
Zhao, J., Niu, X., Yu, J., Xiao, X., Li, W., Zang, L., et al. (2020). Poria cocos polysaccharides attenuated ox-LDL-induced inflammation and oxidative stress via ERK activated Nrf2/HO-1 signaling pathway and inhibited foam cell formation in VSMCs. Int. Immunopharmacol. 80, 106173. doi:10.1016/j.intimp.2019.106173
Zhao, M., Guan, Z., Tang, N., and Cheng, Y. (2023). The differences between the water- and alkaline-soluble Poria cocos polysaccharide: a review. Int. J. Biol. Macromol. 235, 123925. doi:10.1016/j.ijbiomac.2023.123925
Zheng, Y., and Yang, X. W. (2008). Poriacosones A and B: two new lanostane triterpenoids from Poria cocos. J. Asian Nat. Prod. Res. 10 (7-8), 645–651. doi:10.1080/10286020802133597
Zhu, L., Ye, C., Hu, B., Xia, H., Bian, Q., Liu, Y., et al. (2022). Regulation of gut microbiota and intestinal metabolites by Poria cocos oligosaccharides improves glycolipid metabolism disturbance in high-fat diet-fed mice. J. Nutr. Biochem. 107, 109019. doi:10.1016/j.jnutbio.2022.109019
Zou, Y. (2019). Characterization of chemical constituents of Poria cocosand primary study of their protective effects AgainstCisplatin-induced intestinal injury.
Zou, Y., Xu, J., Long, F., Zhang, Y., and Li, S. (2019). Evaluation of chemical consistency of triterpene acids in ethanol extracts ofPoria and acetic ether extracts thereof by UPLC-QTOF-MS/MS with full scanand mimic MRM mode. J. Pharm. 54 (01), 130–137. doi:10.16438/j.0513-4870.2018-0670
Keywords: Wolfiporia cocos (F.A. Wolf) Ryvarden & Gilb, polysaccharide, triterpenoid metabolites, active metabolites, mechanism of action
Citation: Xiong Q, Li Z, Yang D, Liu X, Pu W, Yue X, Jia K, Wan X and Zou Y (2025) Progress in the study of bioactivity, chemical composition and pharmacological mechanism of action in Wolfiporia cocos (F.A. Wolf) Ryvarden & Gilb. Front. Pharmacol. 16:1521235. doi: 10.3389/fphar.2025.1521235
Received: 01 November 2024; Accepted: 06 February 2025;
Published: 03 March 2025.
Edited by:
Rajeev K. Singla, Sichuan University, ChinaReviewed by:
Filipa Pinto-Ribeiro, University of Minho, PortugalCopyright © 2025 Xiong, Li, Yang, Liu, Pu, Yue, Jia, Wan and Zou. This is an open-access article distributed under the terms of the Creative Commons Attribution License (CC BY). The use, distribution or reproduction in other forums is permitted, provided the original author(s) and the copyright owner(s) are credited and that the original publication in this journal is cited, in accordance with accepted academic practice. No use, distribution or reproduction is permitted which does not comply with these terms.
*Correspondence: Xilin Wan, d2FueGlsaW4xOTg3QDE2My5jb20=; Yuanjun Zou, em91eWpAY2N1Y20uZWR1LmNu
†These authors have contributed equally to this work
Disclaimer: All claims expressed in this article are solely those of the authors and do not necessarily represent those of their affiliated organizations, or those of the publisher, the editors and the reviewers. Any product that may be evaluated in this article or claim that may be made by its manufacturer is not guaranteed or endorsed by the publisher.
Research integrity at Frontiers
Learn more about the work of our research integrity team to safeguard the quality of each article we publish.