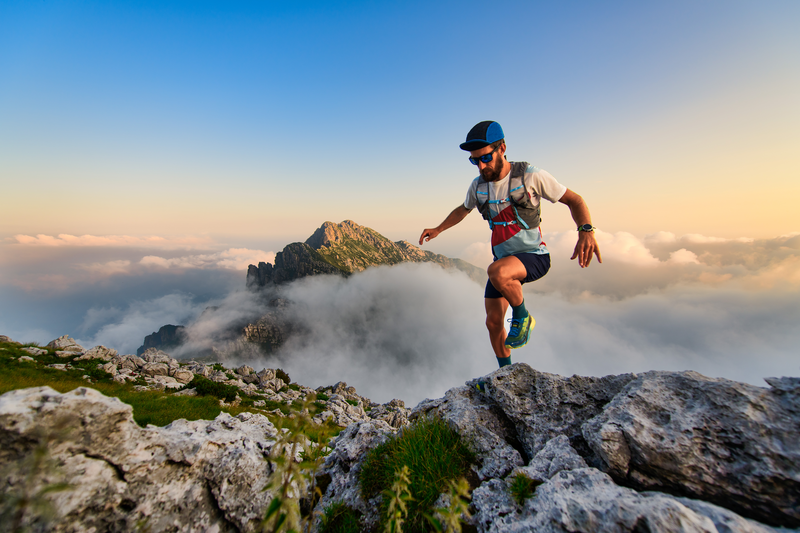
94% of researchers rate our articles as excellent or good
Learn more about the work of our research integrity team to safeguard the quality of each article we publish.
Find out more
REVIEW article
Front. Pharmacol. , 11 December 2024
Sec. Ethnopharmacology
Volume 15 - 2024 | https://doi.org/10.3389/fphar.2024.1510307
Background: Astragalus mongholicus Bunge [Fabaceae; Astragali radix] (AM), a traditional Chinese medicinal (TCM) botanical drug, has been used for centuries and is gaining growing recognition in medical research for its therapeutic potential. The currently accepted scientific name is Astragalus mongholicus Bunge, with Astragalus membranaceus Fisch. ex Bunge recognized as a taxonomic synonym. This review explores the most relevant scientific studies on AM, focusing on its chemical composition, mechanisms of action, and associated health benefits.
Main body: AM is commonly used in clinical practice to treat diabetes mellitus, cardiovascular diseases, oncological processes, lipid metabolism disorders, and ulcerative colitis. Recent research has investigated its potential as a product for anti-aging purposes. These therapeutic effects are attributed to the interactions of bioactive metabolites such as Astragaloside IV, Formononetin, and polysaccharides, with various signaling pathways, leading to the activation or inhibition of gene expression. This review aims to map the signaling pathways affected by these metabolites and their effects on different pathologies. Studies suggest that these metabolites act on signaling pathways such as TLR4/MyD88/NF-κB, PI3K/AKT, RNA expression, and tumor receptors. However, further research is necessary to validate the findings in human trials with better methodological quality.
Conclusion: AM is rich in bioactive metabolites that interact with various signaling pathways, modulating diseases such as diabetes mellitus type 2, cardiovascular diseases, cancer, lipid metabolism disorders, and ulcerative colitis. Although promising, the majority of the studies are conducted in vitro and animal models, and more rigorous human trials are needed to determine the therapeutic potential of AM.
Astragalus mongholicus Bunge (AM), also known as “Huang Qi” in TCM, is a medicinal plant belonging to the legume family (Fabaceae). Astragalus mongholicus Bunge [Fabaceae; Astragali radix] is the species currently accepted according to modern taxonomy. Historically, it has been referred to as Astragalus membranaceus Fisch. ex Bunge in various publications. Native to Asia, particularly China and Mongolia, this plant has been valued for centuries for its immunomodulatory, antioxidant, and adaptogenic properties. The roots, which are the medicinally used part, contain active metabolites such as saponins, flavonoids, and polysaccharides. In traditional medicine, AM is used to strengthen the immune system, improve physical endurance, and promote longevity and vitality. It is also studied for its potential to reduce fatigue and protect organs from damage caused by oxidative stress (Tang and Huang, 2022; Li et al., 2022; Dong et al., 2023).
AM is often used in clinical practice to treat various pathologies and plays an important role in the international health trade. The composition of AM is quite complex, as it has more than 180 bioactive metabolites, including triterpenoids, saponins, sapogenins, flavonoids, glycosides, polysaccharides, phytosterols, fatty acids, etc. (Su et al., 2021; Li et al., 2018). These metabolites have anti-oxidation, anti-inflammation, immune regulatory, anti-cancer, lipid-lowering, hypoglycemic, anti-fatigue, antiviral, and anti-aging effects (Zhang et al., 2022). These effects are produced by the interaction between the bioactive metabolites of AM and different signaling pathways and the activation or inhibition of gene expression (Su et al., 2021; Jin et al., 2020; Gong et al., 2018; Sheng et al., 2021; Zhong et al., 2022).
Astragaloside IV (AS-IV) belongs to the group of saponins and is the main metabolite in AM (Su et al., 2021; Zheng et al., 2018). It has been shown to have numerous pharmacological properties, such as immunoregulation, antioxidant, anticancer, antiviral (Li et al., 2018), neuroprotection, liver protection, and improved sensitivity to cancer treatment. These effects are due to the interaction of AS-IV with several signaling pathways, including Raf-MEK-ERK, EGFR-Nrf2, Akt/PDE3B, AMPK, NF-κB, Nrf2, PI3K/Akt/mTOR, PKC-α- ERK1/2- NF-κB, IL-11/STAT3, Akt/GSK-3β/β-catenin, JNK/c-Jun/AP-1, PI3K/Akt/NF-κB, miRNA-34a/LDHA, Nox4/Smad2, JNK, and NF-κB/PPARγ (Zhang et al., 2020). Formononetin is a phytoestrogen that is increasingly being studied in the field of cancer, and in vitro studies have shown that it can prevent cancer progression by activating apoptosis, modulating the cell cycle, and modulating certain signaling pathways related to metastasis (Jiang et al., 2019). Other bioactive metabolites found in greater proportions are polysaccharides, which have been shown to have antioxidation, antihypertensive, antitumor, and immunomodulatory properties (Zhang R. et al., 2018).
AM can provide numerous health benefits; however, the exact mechanisms of action remains unknown (Shi et al., 2021). Therefore, the objective of this exploratory review was to map the signaling pathways in which AM bioactive metabolites act and their effects on different pathologies.
A bibliographic search of the PubMed database was performed. The keywords used were “Astragalus membranaceus”, “Huang qi”, “Astragaloside IV”, “Formononetin” and “signaling pathways”. In order for the results to be as up-to-date as possible, the search was filtered for studies published between September 2017 and October 2024, and published in English. 585 results were found. After performing the first filter by reading the title, those that did not have to do with the subject of the review (n = 407) were eliminated, of which 178 articles remained. After reading the abstract and full text, studies in which AM was administered in combination with another drug, studies that did not explain any signaling pathway, and articles whose full text could not be accessed were eliminated. In this bibliographic review, 65 studies were used, of which 28 explained the different signaling pathways in which the active metabolites of AM interact. A flowchart is shown in Figure 1. Table 1 summarize the data obtained in this study. Figure 2 shows a summary of the effects of AM on health.
AM is often used in the treatment of diabetes mellitus type 2 (DM2) owing to its hypoglycemic effect. The metabolites found in AM can interact with the AGE-RAGE and PI3K-Akt signaling pathways, which are related to apoptosis, inflammation and monocyte adhesion. These signaling pathways modulate the evolution of complications during the development of DM2 (Jin et al., 2020), such as the deterioration of renal function. The use of AM in patients with diabetic kidney disease has been shown to decrease the levels of albuminuria, proteinuria and serum creatinine (Zhang L. et al., 2019). The antidiabetic effects of AM are highly associated with the activation of receptor protein tyrosine kinase (RPTK), however, the exact mechanism of action is unknown, since numerous genes are involved in this process. It has also been found to regulate lipid metabolism in DM2 and improve insulin resistance (Li J. et al., 2019).
Protein tyrosine phosphatase 1B (PPT1B) increases the transcriptional activity of SREBP-1c, resulting in lipid deposition in the liver, decreased glucose processing ability, and increased insulin resistance. On the other hand, AS-IV is an inhibitor of PPT1B, which helps to reverse this process. It regulates the metabolism of lipids, and carbohydrates and decreases insulin resistance, in a dose-dependent manner. In addition, according to X. Zhou et al., the lipid regulating effect of AS-IV is similar to that of atorvastatin, although further research is needed to corroborate this finding (Zhou et al., 2021). The development of gestational diabetes poses a risk to both the mother and fetus. This alteration in glucose metabolism strongly correlates with an abnormal inflammatory response. Zhang et al. induced diabetes in pregnant mice and subsequently administered AS-IV. A decrease in glucose levels and an increase in insulin levels were observed. Besides that, the expression of the NLRP3 inflammasome, which is related to insulin resistance, showed a clear decrease, and the expression of IL-6 and TNF-α was also reduced, in a dose-dependent manner (Zhang R. et al., 2019).
Abnormal metabolism in adipose tissue is known to be associated with the development of DM2. Zhang et al. found that A. mongholicus polysaccharides (AMP) can significantly increase insulin sensitivity by activating AMPK in 3T3-L1 adipocytes. This process involves the differentiation and proliferation of 3T3-L1 preadipocytes and the normalization of adipose tissue metabolism (Zhang R. et al., 2018). AMPs can also activate hepatic insulin signaling. Better control of body weight, and blood glucose and lipid levels, liver function, and insulin sensitivity was achieved. AMPs can decrease the levels of proinflammatory cytokines such as TNF-α, IL-6, IL-1β, and leptin. AMPs and metformin can activate insulin production through similar mechanistic pathways (Sun et al., 2019). In contrast, Lee et al. observed that AM isoflavonoids, including calycosin-7-O-β-d-glucoside, formononetin and adenosine, increased the expression of PI3K, AKT, PPARγ and PDX-1. This increased expression increases insulin secretion from pancreatic β-cells and lowers blood glucose (Lee et al., 2019).
AM also has therapeutic effects on pathologies related to the cardiovascular system, including myocardial fibrosis, cardiac hypertrophy, viral myocarditis, cardiomyopathy, hypertension, atherosclerosis, and hypertensive nephropathy (Su et al., 2021; Xu et al., 2022; Zhang et al., 2023; Meng et al., 2023). AS-IV can significantly decrease the size of the myocardial infarction zone, promote angiogenesis, improve circulation, and exert antioxidant, anti-inflammatory, and anti-apoptotic effects (Zheng et al., 2018). TLR4/MyD88 modulates the immune response and activates the expression of NF-κB and proinflammatory cytokines related to acute myocardial infarction. AS-IV has the potential to avert instances of acute myocardial infarction through the suppression of the TLR4/MyD88/NF-κB signaling pathway, a pathway intricately linked to myocarditis and myocardial fibrosis. Its inhibition also decreases the production of collagen I, collagen II, collagen III and HYP (Shi et al., 2021). Apart from that, AS-IV can suppress the TLR4/NF-kB signaling pathway, improve vascular endothelial dysfunction due to hyperglycemia, increase eNOS expression and NO production, prevent myocardial cell apoptosis and reduce the expression of biomarkers of inflammation, such as IL-6, TNF-α, VCAM-1, ICAM-1, TLR4, and NF-kB p65 (Leng et al., 2018; Zhao et al., 2018).
AS-IV may also enhance vasodilation via the PI3K/Akt/eNOS signaling pathway. This signaling pathway increases nitric oxide production, leading to the relaxation and dilation of blood vessels (Lin et al., 2018). AS-IV provides protection against damage to ischemic myocardial cells, suppresses myocardial hypertrophy and fibrosis, enhances myocardial contractility, ameliorates diastolic dysfunction, mitigates vascular endothelial dysfunction, and fosters angiogenesis. The safeguarding impact of AS-IV against ischemia and hypoxia is associated with modulation of the MAPK, PI3K/AKT, Notch1, and NF-κB signaling pathways (Tan et al., 2020). Cardiac hypertrophy can lead to arrhythmia, heart failure, and death. AS-IV protects against cardiac hypertrophy, possibly through the TBK1/PI3K/AKT signaling pathway, which is responsible for organ remodeling, including the heart. In cardiac hypertrophy, AS-IV can attenuate apoptosis and decrease levels of inflammatory cytokines, such as TNF-α, IL-1, and IL-6 in a dose-dependent manner (Liu Z. H. et al., 2018).
Hypoxia-inducible factors (HIFs) are transcription factors activated by changes in oxygen concentration. These transcription factors, including HIF-1α, regulate genes related to adaptation to hypoxia such as vascular endothelial growth factor (VEGF). Under hypoxic conditions, AS-IV can promote angiogenesis by activating HIF-1α and VEGF (Wang et al., 2021). In contrast, the miR-135a-TRPM7-TGF-β/Smad signaling pathway is associated with cardiac fibrosis. AS-IV can inhibit miR-135a, thereby inhibiting the signaling cascade and Collagen I formation, resulting in improvement of the fibrotic state (Wei et al., 2020). It was also confirmed that AS-IV has protective effects in the brain in cases of ischemic stroke since it promotes angiogenesis by activating the BNDF/TrkB signaling pathway. At the same time, neurogenesis in the hippocampus, learning and memory are also promoted after an ischemic stroke event (Ni et al., 2020).
AMPs also exhibits cardioprotective activities. In mice with myocardial ischemia, administration of AMP attenuated the increase in myocardial cell volume and reduced apoptosis. In vitro, it was observed that there was less activity of caspase-3 and greater activity of Bcl-2, which translates into inhibition of apoptosis and reduced production of reactive oxygen species (Liu D. et al., 2018).
The combined use of chemotherapy and TCM provides greater benefits than chemotherapy alone. The combination of AM and chemotherapy achieves a better modulation of the immune system, the patient feels better physically, the survival rate increases, the side effects of chemotherapy are reduced, such as nausea, mucositis, or leukopenia, and there is a lower rate of tumor growth and increased apoptosis of cancer cells (Sheik et al., 2021; Li et al., 2020).
AM promotes apoptosis in colorectal cancer cells by inhibiting the pro-cancer genes CCL2, CXCL8, CXCL10 and PTGS2. This effect could be related to the antioxidant and anti-inflammatory properties of AM (Chu et al., 2021). In addition, in the tumor process, a tolerance response of the immune system is produced towards the tumor cells due to the union of PD-LI and PD-I (Xiong et al., 2024). When the expression of PD-LI decreases, the immune response increases. AM can reduce the expression of PD-LI in the cell membranes of tumor cells. This mechanism of action is related to the AKT/mTOR/p70S6K signaling pathway. Chang et al. observed that AM did not induce apoptosis of tumor cells; however, it slowed the reduction of tumor mass growth (Chang et al., 2020). Activation of the PI3K/AKT/mTOR signaling pathway is related to the formation of the carcinogenic process. AM extract can inhibit the proliferation of breast cancer cells by inhibiting PI3K/AKT/mTOR through its biometabolites with a dose-dependent effect, inducing cytotoxicity and apoptosis in these cells, and suppressing their growth and proliferation (Zhou et al., 2018). On the other hand, lncRNA-ATB promotes the migration, metastasis, and survival of hepatocellular carcinoma cells by activating the IL-11/STAT3 signaling pathway. AS-IV inhibits carcinogenesis by suppressing lncRNAs. Thus, IL-11/STAT3 is inhibited and the proliferation of cancer cells is interrupted (Li et al., 2018).
AMPs regulate the levels of CD3+, CD4+, CD8+ T, and B cells in certain types of tumors. They can also activate cells of the immune system, promote anaerobic metabolism in the tumor area, and induce apoptosis in tumor cells (Yu et al., 2021). In addition, AMPs can modulate the growth of dysbiosis-associated melanomas by modulating gut microbiota. This process could occur because these AMPs stimulate the microbiota to produce glutamate and creatine, which are metabolites that inhibit tumor growth. In contrast, AMPs can inhibit the expression of inflammatory cytokines such as IL-10 and TGF-β and activate CD8+ T cells, which are natural killers of tumor cells (Ding et al., 2021). AMPs can also enhance the activity of miR-195-5p, which is a miRNA responsible for the inhibition of cell invasion and migration of tumor cells (Tao et al., 2022). Besides that, miR-27a is an oncogenic miRNA responsible for the growth and proliferation of cancer cells, whereas FBXW7 is a tumor cell suppressor gene. Therefore, miR-27a and FBXW7 were negatively correlated, as when the expression of miR-27a increased, the activity of FBXW7 decreased, and vice versa. AMPs exhibit the ability to impede the growth, invasion, and migration of cancer cells, inducing apoptosis via the microRNA-27a/FBXW7 signaling pathway in a dose-dependent manner (Guo et al., 2020). AMPs can also activate RAW264.7 macrophages, which are responsible for apoptosis of cancer cell apoptosis by releasing cytotoxins such as nitric oxide or TNF-α (Li W. et al., 2019).
Formononetin exerts anticancer effects by modulating numerous signaling pathways involved in apoptosis, cell cycle, suppression of cell proliferation, and cell invasion (Tay et al., 2019). Formononetin can inhibit the growth and invasion of colon carcinoma cells by inhibiting the PI3K/AKT and STAT3 signaling pathways (Wang et al., 2018). Other studies suggest that formononetin may inhibit the oncological process by suppressing ERK1/2 (Hu et al., 2023). Furthermore, in ovarian cancer cells, it promotes apoptosis by increasing Bax/Bcl-2 expression in a dose-dependent manner (Zhang J. et al., 2018).
VEGF1 genes are responsible for angiogenesis, reducing inflammation and regulating the metabolism of adipose tissue. ESR1 genes activates the expression of VEGF1. On the contrary, CCND1 and AKT1 genes expression promotes the development of obesity. Therefore, VEGF1, ESR1, CCND1, and AKT1 are genes closely associated with lipid metabolism and obesity. AM extract can improve the lipid profile by inhibiting lipogenesis and activating β-oxidation and lipolysis. AM stimulates the expression of VEGF1 and ESR1, and inhibits AKT1 and CCND1 (Wang et al., 2022). Furthermore, the PPARγ/UCP1 signaling pathway is responsible for inducing thermogenesis in adipocytes, especially in brown adipose tissue. AM can activate this process by binding formononetin-PPARγ, which forms a heterodimer with RXR and activates the expression of UCP1. Nie et al. administered formononetin to mice and found that it can lower body weight, thus opening a new avenue for obesity research (Nie et al., 2018). Apart from that, the flavones present in AM can reduce the size of atherosclerotic plaques and improve hepatic steatosis by modulating the lipid profile, inflammation, and monocyte adhesion. Flavones have the capacity to reduce NF-κB activity, lower levels of proinflammatory cytokines, and elevate levels of anti-inflammatory cytokines. A decrease in the expression of miR-33, a post-transcriptional regulator inflammation-related genes has been reported (Ma et al., 2020).
Ulcerative colitis is a disease characterized by excessive inflammation. According to scientific literature, Th17 cells are responsible for activating the inflammatory response, while Treg cells inhibit it. AS-IV may be a possible treatment pathway for this disease by modulating Th17/Treg cells, which are also related to immunosuppression and immune tolerance. Zhong et al., who used mice with ulcerative colitis, observed that in the group administered AS-IV, the response of Th17 cells was inhibited and Treg cells were activated. Also, lower levels of oxidative stress, decreased IL-17A and IL-21 inflammatory cytokines, and increased IL-10 and TGF-β1 anti-inflammatory cytokines were observed. On the other hand, at the macroscopic level, improvement was observed in the typical symptoms of ulcerative colitis, such as less weight loss and fewer ulcerations (Zhong et al., 2022).
Although aging is a biological process, AM extract has been shown in fruit flies (Drosophila melanogaster) to have the ability to slow telomere shortening. Some hypotheses show that the bioactive metabolites of AM can eliminate free radicals due to their antioxidant action. A potential candidate could be formononetin, which can activate the formation of endogenous antioxidants and improve the stabilization of the cellular structure (Zhang et al., 2022). AM polysaccharides, flavonoids and saponins can increase telomerase activity, decrease inflammation levels, modulate the immune system, prevent cancer, lower blood glucose and lipid levels, have hepatoprotective functions and increase diuresis. Besides that, the expression of Bcl-2 is related to the selectivity of these bioactive metabolites when it comes to acting on target cells, reducing the apoptosis of normal cells and promoting the apoptosis of cancer cells. However, the exact mechanism remains unknown (Liu et al., 2017).
Experimental studies involving the use of botanical drugs present inherent challenges due to their complex composition, which consists of a variety of bioactive metabolites whose biological activity is not directed towards a single molecular target. Additionally, the composition of these plants is influenced by multiple factors, such as the preparation method, the species used, the agronomic conditions during cultivation, extraction methods, among others (Heinrich et al., 2022). Furthermore, a major challenge in studies using TCM is the lack of standardized protocols, as these vary depending on practitioners and researchers, making the replication difficult. Additionally, since TCM is a holistic treatment approach, it is challenging to measure and quantify using conventional scientific methods. TCM is primarily practiced in Asian countries, with its presence being more limited in Western countries. As a result, studies using herbal formulas within this framework are scarce. One of the main challenges faced by TCM is the need to reach a consensus in order to make studies more standardized (Zhou et al., 2019). In our study, we have not found human studies that analyze molecular pathways associated with the use of AM. After filtering the articles, we identified very few studies addressing this topic. However, AM is a drug commonly used in clinical practice in several Asian countries, where its therapeutic efficacy has been reported. This situation reflects a significant gap in knowledge that should be explored, not only in relation to AM but also regarding other plants used in TCM (Chen et al., 2019; Zhang et al., 2017).
AM is a TCM botanical drug that contains a wide variety of bioactive metabolites that can interact with various signaling pathways and modulate diseases. However, because phytotherapy is a field of study that is still booming, there are still gaps in the knowledge that needed to be explored. Additionally, most of the research on phytotherapy is carried out in vitro and in animal models, while relatively few studies carried out in humans have a low methodological quality. Although the studies included in this review provide evidences about the usefulness of AM in the treatment of diseases, the signaling pathways in humans are more complex that in cell and animal models (Tan et al., 2020); therefore, there is still a long way to go. This review opens the doors to new ways to treat diseases that are currently highly prevalent; however, additional research is imperative to comprehensively elucidate the complete mechanism of AM effects on human biology (Guo et al., 2020). There is a recognized need for further studies in humans to gain a deeper understanding of the mechanisms of action of AM in this population.
AM influences various molecular pathways associated with diabetes, cardiovascular diseases, oncological processes, among others. However, most of the available studies have been conducted in animal models or in vitro. Further research in humans is needed to gain a more precise understanding of the mechanism of action of AM in this population.
IXL: Conceptualization, Data curation, Formal Analysis, Funding acquisition, Methodology, Validation, Writing–original draft, Writing–review and editing. XY: Software, Visualization, Writing–original draft. QC: Investigation, Methodology, Project administration, Resources, Supervision, Writing–review and editing.
The author(s) declare that no financial support was received for the research, authorship, and/or publication of this article. This research received no external funding.
The authors declare that the research was conducted in the absence of any commercial or financial relationships that could be construed as a potential conflict of interest.
The author(s) declare that no Generative AI was used in the creation of this manuscript.
All claims expressed in this article are solely those of the authors and do not necessarily represent those of their affiliated organizations, or those of the publisher, the editors and the reviewers. Any product that may be evaluated in this article, or claim that may be made by its manufacturer, is not guaranteed or endorsed by the publisher.
Chang, H. L., Kuo, Y. H., Wu, L. H., Chang, C. M., Cheng, K. J., Tyan, Y. C., et al. (2020). The extracts of Astragalus membranaceus overcome tumor immune tolerance by inhibition of tumor programmed cell death protein ligand-1 expression. Int. J. Med. Sci. 17 (7), 939–945. doi:10.7150/ijms.42978
Chen, Y. B., Tong, X. F., Ren, J., Yu, C. Q., and Cui, Y. L. (2019). Current research trends in traditional Chinese medicine formula: a bibliometric review from 2000 to 2016. Evidence-based complementary Altern. Med. eCAM. 2019, 3961395. doi:10.1155/2019/3961395
Chu, X. D., Zhang, Y. R., Lin, Z. B., Zhao, Z., Huangfu, S. C., Qiu, S. H., et al. (2021). A network pharmacology approach for investigating the multi-target mechanisms of Huangqi in the treatment of colorectal cancer. Transl. cancer Res. 10 (2), 681–693. doi:10.21037/tcr-20-2596
Ding, G., Gong, Q., Ma, J., Liu, X., Wang, Y., and Cheng, X. (2021). Immunosuppressive activity is attenuated by Astragalus polysaccharides through remodeling the gut microenvironment in melanoma mice. Cancer Sci. 112 (10), 4050–4063. doi:10.1111/cas.15078
Dong, M., Li, J., Yang, D., Li, M., and Wei, J. (2023). Biosynthesis and pharmacological activities of flavonoids, triterpene saponins and polysaccharides derived from Astragalus membranaceus. Mol. Basel, Switz. 28 (13), 5018. doi:10.3390/molecules28135018
Gong, A. G. W., Duan, R., Wang, H. Y., Kong, X. P., Dong, T. T. X., Tsim, K. W. K., et al. (2018). Evaluation of the pharmaceutical properties and value of astragali radix. Med. (Basel). 5 (2), 46. doi:10.3390/medicines5020046
Guo, Y., Zhang, Z., Wang, Z., Liu, G., Liu, Y., and Wang, H. (2020). Astragalus polysaccharides inhibit ovarian cancer cell growth via microRNA-27a/FBXW7 signaling pathway. Biosci. Rep. 40 (3). doi:10.1042/BSR20193396
Heinrich, M., Jalil, B., Abdel-Tawab, M., Echeverria, J., Kulić, Ž., McGaw, L. J., et al. (2022). Best Practice in the chemical characterisation of extracts used in pharmacological and toxicological research-The ConPhyMP-Guidelines. Front. Pharmacol. 13, 953205. doi:10.3389/fphar.2022.953205
Hu, Y., Zhai, W., Tan, D., Chen, H., Zhang, G., Tan, X., et al. (2023). Uncovering the effects and molecular mechanism of Astragalus membranaceus (Fisch.) Bunge and its bioactive ingredients formononetin and calycosin against colon cancer: an integrated approach based on network pharmacology analysis coupled with experimental validation and molecular docking. Front. Pharmacol. 14, 1111912. doi:10.3389/fphar.2023.1111912
Jiang, D., Rasul, A., Batool, R., Sarfraz, I., Hussain, G., Mateen Tahir, M., et al. (2019). Potential anticancer properties and mechanisms of action of formononetin. BioMed Res. Int. 2019, 5854315. doi:10.1155/2019/5854315
Jin, Q., Hao, X. F., Xie, L. K., Xu, J., Sun, M., Yuan, H., et al. (2020). A network pharmacology to explore the mechanism of Astragalus membranaceus in the treatment of diabetic retinopathy. Evidence-based complementary Altern. Med. eCAM. 2020, 8878569. doi:10.1155/2020/8878569
Lee, D., Lee, D. H., Choi, S., Lee, J. S., Jang, D. S., and Kang, K. S. (2019). Identification and isolation of active compounds from Astragalus membranaceus that improve insulin secretion by regulating pancreatic β-cell metabolism. Biomolecules 9 (10), 618. doi:10.3390/biom9100618
Leng, B., Tang, F., Lu, M., Zhang, Z., Wang, H., and Zhang, Y. (2018). Astragaloside IV improves vascular endothelial dysfunction by inhibiting the TLR4/NF-κB signaling pathway. Life Sci. 209, 111–121. doi:10.1016/j.lfs.2018.07.053
Li, C. X., Liu, Y., Zhang, Y. Z., Li, J. C., and Lai, J. (2022). Astragalus polysaccharide: a review of its immunomodulatory effect. Arch. Pharm. Res. 45 (6), 367–389. doi:10.1007/s12272-022-01393-3
Li, J., Huang, Y., Zhao, S., Guo, Q., Zhou, J., Han, W., et al. (2019a). Based on network pharmacology to explore the molecular mechanisms of astragalus membranaceus for treating T2 diabetes mellitus. Ann. Transl. Med. 7 (22), 633. doi:10.21037/atm.2019.10.118
Li, S., Sun, Y., Huang, J., Wang, B., Gong, Y., Fang, Y., et al. (2020). Anti-tumor effects and mechanisms of Astragalus membranaceus (AM) and its specific immunopotentiation: status and prospect. J. Ethnopharmacol. 258, 112797. doi:10.1016/j.jep.2020.112797
Li, W., Song, K., Wang, S., Zhang, C., Zhuang, M., Wang, Y., et al. (2019b). Anti-tumor potential of astragalus polysaccharides on breast cancer cell line mediated by macrophage activation. Mater Sci. Eng. C Mater Biol. Appl. 98, 685–695. doi:10.1016/j.msec.2019.01.025
Li, Y., Ye, Y., and Chen, H. (2018). Astragaloside IV inhibits cell migration and viability of hepatocellular carcinoma cells via suppressing long noncoding RNA ATB. Biomed. and Pharmacother. = Biomedecine and Pharmacother. 99, 134–141. doi:10.1016/j.biopha.2017.12.108
Lin, X. P., Cui, H. J., Yang, A. L., Luo, J. K., and Tang, T. (2018). Astragaloside IV improves vasodilatation function by regulating the PI3K/Akt/eNOS signaling pathway in rat aorta endothelial cells. J. Vasc. Res. 55 (3), 169–176. doi:10.1159/000489958
Liu, D., Chen, L., Zhao, J., and Cui, K. (2018b). Cardioprotection activity and mechanism of Astragalus polysaccharide in vivo and in vitro. Int. J. Biol. Macromol. 111, 947–952. doi:10.1016/j.ijbiomac.2018.01.048
Liu, P., Zhao, H., and Luo, Y. (2017). Anti-aging implications of Astragalus membranaceus (huangqi): a well-known Chinese tonic. Aging Dis. 8 (6), 868–886. doi:10.14336/AD.2017.0816
Liu, Z. H., Liu, H. B., and Wang, J. (2018a). Astragaloside IV protects against the pathological cardiac hypertrophy in mice. Biomed. and Pharmacother. = Biomedecine and Pharmacother. 97, 1468–1478. doi:10.1016/j.biopha.2017.09.092
Ma, C., Zhang, J., Yang, S., Hua, Y., Su, J., Shang, Y., et al. (2020). Astragalus flavone ameliorates atherosclerosis and hepatic steatosis via inhibiting lipid-disorder and inflammation in apoE(-/-) mice. Front. Pharmacol. 11, 610550. doi:10.3389/fphar.2020.610550
Meng, Z., Zhang, S., Li, W., Wang, Y., Wang, M., Liu, X., et al. (2023). Cationic proteins from eosinophils bind bone morphogenetic protein receptors promoting vascular calcification and atherogenesis. Eur. heart J. 44 (29), 2763–2783. doi:10.1093/eurheartj/ehad262
Ni, G. X., Liang, C., Wang, J., Duan, C. Q., Wang, P., and Wang, Y. L. (2020). Astragaloside IV improves neurobehavior and promotes hippocampal neurogenesis in MCAO rats though BDNF-TrkB signaling pathway. Biomed. and Pharmacother. = Biomedecine and Pharmacother. 130, 110353. doi:10.1016/j.biopha.2020.110353
Nie, T., Zhao, S., Mao, L., Yang, Y., Sun, W., Lin, X., et al. (2018). The natural compound, formononetin, extracted from Astragalus membranaceus increases adipocyte thermogenesis by modulating PPARγ activity. Br. J. Pharmacol. 175 (9), 1439–1450. doi:10.1111/bph.14139
Sheik, A., Kim, K., Varaprasad, G. L., Lee, H., Kim, S., Kim, E., et al. (2021). The anti-cancerous activity of adaptogenic herb Astragalus membranaceus. Phytomedicine Int. J. phytotherapy Phytopharm. 91, 153698. doi:10.1016/j.phymed.2021.153698
Sheng, Z., Jiang, Y., Liu, J., and Yang, B. (2021). UHPLC-MS/MS analysis on flavonoids composition in Astragalus membranaceus and their antioxidant activity. Antioxidants Basel, Switz. 10 (11), 1852. doi:10.3390/antiox10111852
Shi, H., Zhou, P., Gao, G., Liu, P. P., Wang, S. S., Song, R., et al. (2021). Astragaloside IV prevents acute myocardial infarction by inhibiting the TLR4/MyD88/NF-κB signaling pathway. J. Food Biochem. 45 (7), e13757. doi:10.1111/jfbc.13757
Su, H. F., Shaker, S., Kuang, Y., Zhang, M., Ye, M., and Qiao, X. (2021). Phytochemistry and cardiovascular protective effects of huang-qi (astragali radix). Med. Res. Rev. 41 (4), 1999–2038. doi:10.1002/med.21785
Sun, J., Liu, Y., Yu, J., Wu, J., Gao, W., Ran, L., et al. (2019). APS could potentially activate hepatic insulin signaling in HFD-induced IR mice. J. Mol. Endocrinol. 63 (1), 77–91. doi:10.1530/JME-19-0035
Tan, Y. Q., Chen, H. W., and Li, J. (2020). Astragaloside IV: an effective drug for the treatment of cardiovascular diseases. Drug Des. Dev. Ther. 14, 3731–3746. doi:10.2147/DDDT.S272355
Tang, Z., and Huang, G. (2022). Extraction, structure, and activity of polysaccharide from Radix astragali. Biomed. and Pharmacother. = Biomedecine and Pharmacother. 150, 113015. doi:10.1016/j.biopha.2022.113015
Tao, X., Zhang, X., and Feng, F. (2022). Astragalus polysaccharide suppresses cell proliferation and invasion by up-regulation of miR-195-5p in non-small cell lung cancer. Biol. Pharm. Bull. 45 (5), 553–560. doi:10.1248/bpb.b21-00634
Tay, K. C., Tan, L. T., Chan, C. K., Hong, S. L., Chan, K. G., Yap, W. H., et al. (2019). Formononetin: a review of its anticancer potentials and mechanisms. Front. Pharmacol. 10, 820. doi:10.3389/fphar.2019.00820
Wang, A. L., Li, Y., Zhao, Q., and Fan, L. Q. (2018). Formononetin inhibits colon carcinoma cell growth and invasion by microRNA-149-mediated EphB3 downregulation and inhibition of PI3K/AKT and STAT3 signaling pathways. Mol. Med. Rep. 17 (6), 7721–7729. doi:10.3892/mmr.2018.8857
Wang, B., Zhang, C., Chu, D., Ma, X., Yu, T., Liu, X., et al. (2021). Astragaloside IV improves angiogenesis under hypoxic conditions by enhancing hypoxia-inducible factor-1α SUMOylation. Mol. Med. Rep. 23 (4), 244. doi:10.3892/mmr.2021.11883
Wang, L., Zheng, W., Yang, J., Ali, A., and Qin, H. (2022). Mechanism of Astragalus membranaceus alleviating acquired hyperlipidemia induced by high-fat diet through regulating lipid metabolism. Nutrients 14 (5), 954. doi:10.3390/nu14050954
Wei, Y., Wu, Y., Feng, K., Zhao, Y., Tao, R., Xu, H., et al. (2020). Astragaloside IV inhibits cardiac fibrosis via miR-135a-TRPM7-TGF-β/Smads pathway. J. Ethnopharmacol. 249, 112404. doi:10.1016/j.jep.2019.112404
Xiong, W., Cheng, Z., Chen, H., Liang, H., Wang, M., Chen, Y., et al. (2024). Biomimetic tumor cell membraneencapsulated nanoparticles combine NIR-II photothermal therapy and chemotherapy for enhanced immunotherapy in triplenegative breast cancer. Adv. Funct. Mater., 2410841. doi:10.1002/adfm.202410841
Xu, Z., Qian, L., Niu, R., Yang, Y., Liu, C., and Lin, X. (2022). Efficacy of huangqi injection in the treatment of hypertensive nephropathy: a systematic review and meta-analysis. Front. Med. 9, 838256. doi:10.3389/fmed.2022.838256
Yu, J., Dong, X. D., Jiao, J. S., Ji, H. Y., and Liu, A. J. (2021). Antitumor and immunoregulatory activities of a novel polysaccharide from Astragalus membranaceus on S180 tumor-bearing mice. Int. J. Biol. Macromol. 189, 930–938. doi:10.1016/j.ijbiomac.2021.08.099
Zhang, J., Liu, L., Wang, J., Ren, B., Zhang, L., and Li, W. (2018b). Formononetin, an isoflavone from Astragalus membranaceus inhibits proliferation and metastasis of ovarian cancer cells. J. Ethnopharmacol. 221, 91–99. doi:10.1016/j.jep.2018.04.014
Zhang, J., Qiao, Y., Li, D., Hao, S., Zhang, F., Zhang, X., et al. (2022). Aqueous extract from Astragalus membranaceus can improve the function degradation and delay aging on Drosophila melanogaster through antioxidant mechanism. Rejuvenation Res. 25 (4), 181–190. doi:10.1089/rej.2021.0081
Zhang, J., Wu, C., Gao, L., Du, G., and Qin, X. (2020). Astragaloside IV derived from Astragalus membranaceus: a research review on the pharmacological effects. Adv. Pharmacol. 87, 89–112. doi:10.1016/bs.apha.2019.08.002
Zhang, L., Shergis, J. L., Yang, L., Zhang, A. L., Guo, X., Zhang, L., et al. (2019a). Astragalus membranaceus (Huang Qi) as adjunctive therapy for diabetic kidney disease: an updated systematic review and meta-analysis. J. Ethnopharmacol. 239, 111921. doi:10.1016/j.jep.2019.111921
Zhang, Q., Yu, H., Qi, J., Tang, D., Chen, X., Wan, J. B., et al. (2017). Natural formulas and the nature of formulas: exploring potential therapeutic targets based on traditional Chinese herbal formulas. PloS one 12 (2), e0171628. doi:10.1371/journal.pone.0171628
Zhang, R., Qin, X., Zhang, T., Li, Q., Zhang, J., and Zhao, J. (2018a). Astragalus polysaccharide improves insulin sensitivity via AMPK activation in 3T3-L1 adipocytes. Mol. Basel, Switz. 23 (10), 2711. doi:10.3390/molecules23102711
Zhang, R., Zhang, X., Xing, B., Zhao, J., Zhang, P., Shi, D., et al. (2019b). Astragaloside IV attenuates gestational diabetes mellitus via targeting NLRP3 inflammasome in genetic mice. Reprod. Biol. Endocrinol. 17 (1), 77. doi:10.1186/s12958-019-0522-7
Zhang, Y., Liu, T., Deng, Z., Fang, W., Zhang, X., Zhang, S., et al. (2023). Group 2 innate lymphoid cells protect mice from abdominal aortic aneurysm formation via IL5 and eosinophils. Adv. Sci. Weinheim, Baden-Wurttemberg, Ger. 10 (7), e2206958. doi:10.1002/advs.202206958
Zhao, Y., Liu, Z., and Zhang, H. (2018). Astragaloside protects myocardial cells from apoptosis through suppression of the TLR4/NF-κB signaling pathway. Exp. Ther. Med. 15 (2), 1505–1509. doi:10.3892/etm.2017.5535
Zheng, Q., Zhu, J. Z., Bao, X. Y., Zhu, P. C., Tong, Q., Huang, Y. Y., et al. (2018). A preclinical systematic review and meta-analysis of Astragaloside IV for myocardial ischemia/reperfusion injury. Front. physiology 9, 795. doi:10.3389/fphys.2018.00795
Zhong, Y., Liu, W., Xiong, Y., Li, Y., Wan, Q., Zhou, W., et al. (2022). Astragaloside - alleviates ulcerative colitis by regulating the balance of Th17/Treg cells. Phytomedicine Int. J. phytotherapy Phytopharm. 104, 154287. doi:10.1016/j.phymed.2022.154287
Zhou, R., Chen, H., Chen, J., Chen, X., Wen, Y., and Xu, L. (2018). Extract from Astragalus membranaceus inhibit breast cancer cells proliferation via PI3K/AKT/mTOR signaling pathway. BMC complementary Altern. Med. 18 (1), 83. doi:10.1186/s12906-018-2148-2
Zhou, X., Li, C. G., Chang, D., and Bensoussan, A. (2019). Current status and major challenges to the safety and efficacy presented by Chinese herbal medicine. Med. (Basel). 6 (1), 14. doi:10.3390/medicines6010014
Zhou, X., Wang, L. L., Tang, W. J., and Tang, B. (2021). Astragaloside IV inhibits protein tyrosine phosphatase 1B and improves insulin resistance in insulin-resistant HepG2 cells and triglyceride accumulation in oleic acid (OA)-treated HepG2 cells. J. Ethnopharmacol. 268, 113556. doi:10.1016/j.jep.2020.113556
Keywords: Astragalus mongholicus, signaling pathways, astragaloside IV, formononetin, polysaccharides
Citation: Xu Lou I, Yu X and Chen Q (2024) Exploratory review on the effect of Astragalus mongholicus on signaling pathways. Front. Pharmacol. 15:1510307. doi: 10.3389/fphar.2024.1510307
Received: 12 October 2024; Accepted: 27 November 2024;
Published: 11 December 2024.
Edited by:
Alexander George Panossian, Phytomed AB, SwedenReviewed by:
Linlu Zhao, Hainan Medical University, ChinaCopyright © 2024 Xu Lou, Yu and Chen. This is an open-access article distributed under the terms of the Creative Commons Attribution License (CC BY). The use, distribution or reproduction in other forums is permitted, provided the original author(s) and the copyright owner(s) are credited and that the original publication in this journal is cited, in accordance with accepted academic practice. No use, distribution or reproduction is permitted which does not comply with these terms.
*Correspondence: Qilan Chen, Y3FsMTM1ODg3NTA5NDFAcXEuY29t
Disclaimer: All claims expressed in this article are solely those of the authors and do not necessarily represent those of their affiliated organizations, or those of the publisher, the editors and the reviewers. Any product that may be evaluated in this article or claim that may be made by its manufacturer is not guaranteed or endorsed by the publisher.
Research integrity at Frontiers
Learn more about the work of our research integrity team to safeguard the quality of each article we publish.