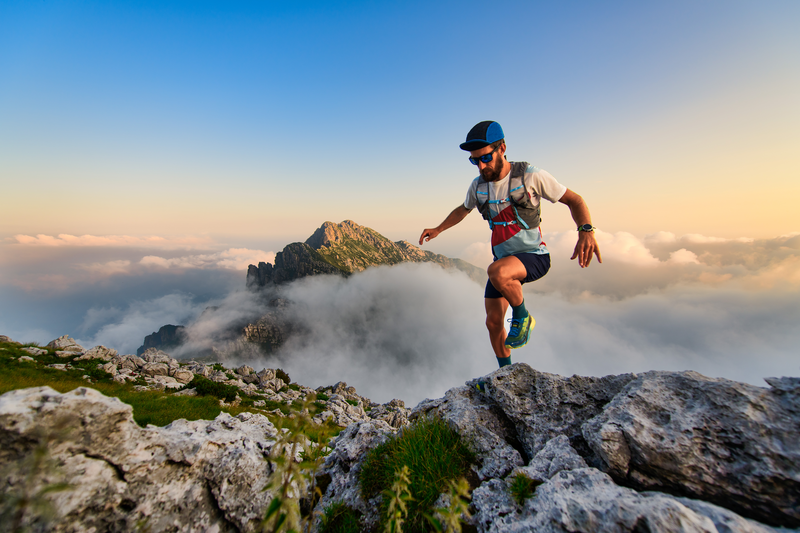
95% of researchers rate our articles as excellent or good
Learn more about the work of our research integrity team to safeguard the quality of each article we publish.
Find out more
REVIEW article
Front. Pharmacol. , 18 December 2024
Sec. Gastrointestinal and Hepatic Pharmacology
Volume 15 - 2024 | https://doi.org/10.3389/fphar.2024.1505027
This article is part of the Research Topic Reviews in Gastrointestinal and Hepatic Pharmacology: 2024 View all 8 articles
F-box and WD repeat domain-containing 7 (FBXW7), formerly known as hCdc4, hAGO Fbw7, or SEL10, plays a specific recognition function in SCF-type E3 ubiquitin ligases. FBXW7 is a well-established cancer suppressor gene that specifically controls proteasomal degradation and destruction of many key oncogenic substrates. The FBXW7 gene is frequently abnormal in human malignancies especially in gastrointestinal cancers. Accumulating evidence reveals that mutations and deletions of FBXW7 are participating in the occurrence, progression and treatment resistance of human gastrointestinal cancers. Considering the current therapeutic challenges faced by gastrointestinal cancers, elucidating the biological function and molecular mechanism of FBXW7 can provide new perspectives and references for future personalized treatment strategies. In this review, we elucidate the key molecular mechanisms by which FBXW7 and its substrates are involved in gastrointestinal cancers. Furthermore, we discuss the consequences of FBXW7 loss or dysfunction in tumor progression and underscore its potential as a prognostic and therapeutic biomarker. Lastly, we propose potential therapeutic strategies targeting FBXW7 to guide the precision treatment of gastrointestinal cancers.
Globally, gastrointestinal cancers have high morbidity and mortality rates, especially in recent years, with a trend toward affecting younger individuals (I et al., 2023; Huang et al., 2023; Ben-Aharon et al., 2023). Gastrointestinal malignancies are estimated to account for 17.7% and 28.5% of all expected newly diagnosed cancer cases and fatalities respectively in the United States in 2024 (Siegel et al., 2024). Gastrointestinal cancers refer to malignant tumors originating from the digestive tract and digestive organs, mainly including esophageal cancer, colorectal cancer, stomach cancer, pancreatic cancer, hepatocellular carcinoma and cholangiocarcinoma (Li et al., 2021; Li et al., 2023a). With the development of medical technology, certain progress has been made in the prognosis of patients with gastrointestinal cancer (Chong et al., 2024). However, gastrointestinal cancers are relatively heterogeneous, and different individuals usually face dramatic differences in outcomes. Molecularly stratified markers are used for patients with gastrointestinal cancers as shown in Figure 1. Precision therapy is the future direction for gastrointestinal cancers, but current therapeutic and prognostic molecular markers are limited. Therefore, to achieve precision management of gastrointestinal cancers, it is necessary to explore novel biomarkers that can improve diagnosis and treatment.
Figure 1. Major molecular markers of gastrointestinal cancers. The key molecular markers for esophageal cancer include HER2 amplification or overexpression, PD-L1, EGFR, and VEGFR (Liu et al., 2020; Zhou and Hofstetter, 2020; Wang et al., 2025). For pancreatic cancer, the main molecular markers are NTRK fusion, BRCA1/2 mutations, KRAS mutations, BRAF mutations, and MSI (Collisson et al., 2019; Buscail et al., 2020; Wang S. et al., 2021; Schlick et al., 2021). Molecular markers for cholangiocarcinoma mainly include MSI, HER-2 amplification or overexpression KRAS mutations,IDH1/2 mutations, FGFR2 fusion and NTRK fusion (Rodrigues et al., 2021; Pavicevic et al., 2022; Kam et al., 2021). The major molecular markers for colorectal cancer include MMR/MSI, RAS/BRAF mutations, NTRK fusion, POLE/POLD1 mutations, PI3K mutations, and HER2 amplification or overexpression (Martelli et al., 2022; Taieb et al., 2019; Sepulveda et al., 2017). The main molecular markers for gastric cancer include MSI, PD-L1,HER-2 amplification or overexpression, Claudin18.2, TMB, and EBV (Guan et al., 2023; Joshi and Badgwell, 2021; Elimova et al., 2015). So far the molecular alterations in hepatocellular carcinoma have not led to effective treatments (Nault and Villanueva, 2021). In the figure we show several promising molecular markers such as ctDNA, ncRNA, TERT promoter mutation and MSI (Nault and Villanueva, 2021; Llovet et al., 2018; Chan et al., 2024a).
The ubiquitin-proteasome system (UPS) regulates many cellular processes such as cell division, cell differentiation, DNA damage repair, and apoptosis by controlling the degradation of a variety of proteins in eukaryotes (Park et al., 2020; Liu et al., 2021a). The protein degradation function of UPS mainly depends on the sequential activation of three enzymes: ubiquitin-activating enzyme E1, ubiquitin couplingase E2 and ubiquitin protein ligase E3 (Tekcham et al., 2020). FBXW7 (also known as Ago, hCdc4, Fbw7, and Sel10) is involved in the formation of the SCF-type E3 enzyme complex and performs specific substrate recognition (Yeh et al., 2018). FBXW7 has been identified as a cancer suppressor gene that frequently malfunctions in a variety of human cancers (Yumimoto and Nakayama, 2020). According to the COSMIC database, the overall mutation rate of FBXW7 in human tumors is 7.79%, with the highest prevalence observed in gynecologic tumors, hematologic malignancies, and gastrointestinal cancers (Fan et al., 2022). Extensive research has demonstrated that abnormal FBXW7 expression contributes to tumor initiation, progression, treatment resistance, and poor prognosis in cancer patients (Qi et al., 2024). While the molecular mechanisms and clinical significance of FBXW7 in gynecologic cancers, breast cancer, and hematologic malignancies have been thoroughly reviewed, a comprehensive analysis of its role in gastrointestinal cancers remains lacking (Di Fiore et al., 2023; Chen et al., 2023; N et al., 2015; Q et al., 2020). Therefore, further investigation into the role of FBXW7 in regulating various signaling pathways and key molecules in gastrointestinal cancers is warranted. It provides a reference for exploring biomarkers and molecular targets for the treatment of gastrointestinal cancers.
The degradation of proteins in eukaryotes requires the coordinated activity of E1, E2, and E3 enzymes (Liu et al., 2017; Hershko and Ciechanover, 1998). First, the E1 enzyme activates and binds to the ubiquitin molecule (Ub) by consuming ATP (Bakos et al., 2018). Subsequently, the Ub bound to the E1 enzyme is transferred to the E2 enzyme (G and T, 2014). The SCF-type E3 enzyme transfers the Ub bound to the E2 enzyme to the specific substrate protein recruited (Pickart, 2001). Finally, the 26s proteasome degrades the target protein-Ub complex and releases Ub for recycling (Xu et al., 2015). F-box proteins are the recognition subunits of the SCF E3 ubiquitin ligase complex, and about 70 F-box proteins have been identified in humans (Ge et al., 2020). Based on their structure, F-box proteins are classified into three families: FBXW (WD40 repeat-containing domains), FBXL (leucine-rich repeats), and FBXO (other structural domains) (Ge et al., 2020; Skaar et al., 2013). FBXW7, due to its involvement in the destruction of many oncogenic proteins, is the most extensively studied F-box protein (Welcker and Clurman, 2008; Zhang et al., 2019). The structure and function of FBXW7 within the UPS are illustrated in Figure 2.
Figure 2. Structure and function of FBXW7. The FBXW7 gene is located on 4q31.3 and encodes three FBXW7 isoforms: FBXW7α, FBXW7β, and FBXW7γ. Despite differences at their N-terminal regions, all three isoforms share dimerization domains, F-box domains, and WD40 repeat domains. FBXW7 primarily targets substrates such as TGF-β, c-Jun, Cyclin E, c-Myc, Notch1, MCL-1, YAP, and mTOR for degradation. Additionally, the expression of FBXW7 is regulated by p53, non-coding RNAs, LSD1, ERK1/2, USP28, HES5, and C/EBPδ.
The FBXW7 gene consists of 13 exons and 4 introns and is located on chromosome 4q31q.3 (Fan et al., 2022; Sailo et al., 2019). As shown in Figure 2, the proteins encoded by the FBXW7 gene contain three domains: F-box, dimerization (D-D), and WD40 (Roling et al., 2022). The F-box domain functions as a linker to the SCF E3 ligase complex by binding to the adaptor protein SKP1 (Welcker and Clurman, 2008; Thirimanne et al., 2022). The WD40 repeat domains fold into β-propeller-like structures, forming binding pockets that specifically recognize and bind phosphorylated substrates (Zhang et al., 2019; Schapira et al., 2017). The dimerization domain contributes to the specificity and stability of FBXW7 and substrate interactions (Welcker et al., 2013). The FBXW7 gene encodes three different mRNAs, which are translated into three isoforms: FBXW7α, FBXW7βand FBXW7γ, differing at the N-terminus (Kitade et al., 2016). These isoforms exhibit distinct subcellular localizations and functional roles.
FBXW7α is distributed in the nucleus and and mediates the degradation of the majority of FBXW7 substrates (Kar et al., 2021). FBXW7β isoforms and FBXW7γ are distributed in the endoplasmic reticulum and nucleolus, respectively (Lee et al., 2020). FBXW7β isoforms are involved in cellular lipid metabolism whereas the function of FBXW7γ is unclear (Wei et al., 2023). The range of substrate proteins degraded by FBXW7 is broad, including Notch1, c-Jun, c-Myc, mTOR, MCL-1, cyclin E, TGF-β, and YAP (Tekcham et al., 2020; Welcker and Clurman, 2008; Sailo et al., 2019; Mao et al., 2008; Bengoechea-Alonso and Ericsson, 2010; Tu et al., 2014). In addition to genetic alterations, the function of FBXW7 is regulated by the P53 , transcriptional (HES5 and C/EBPδ), translational (non-coding RNA), and post-translational regulators (LSD1, ERK1/2, USP28) (Chen et al., 2023). Given the carcinogenic effects of various regulatory proteins and molecules, FBXW7 has a broad and profound role in the occurrence and progression of human cancer (Fan et al., 2022).
FBXW7 plays a pivotal role in various processes, including cancer cell proliferation, metastasis, invasion, apoptosis, and treatment resistance (Davis et al., 2014). Understanding the functional regulatory network of FBXW7 in greater depth is essential to elucidate its tumor-suppressive effects and to identify potential avenues for developing new cancer treatment strategies. The subsequent sections discuss the upstream regulatory mechanisms of FBXW7 and its downstream carcinogenic substrates.
The expression of the FBXW7 gene is regulated by various transcription factors, including TP53, C/EBPδ, PHF1, and Hes5. Mao et al. discovered that the exons of FBXW7 contain p53 binding sites and that heterozygous mutations in TP53 in mouse tumors are frequently accompanied by FBXW7 mutations (Mao et al., 2004). Subsequent studies have confirmed that FBXW7 gene expression depends on the TP53 gene status during tumor development (Perez-Losada et al., 2005; Grim et al., 2012). C/EBPδ directly binds to the FBXW7α promoter to inhibit FBXW7 expression, leading to reduced degradation of mTOR (Balamurugan et al., 2010). Elevated mTOR levels activate HIF-1 protein activity, thereby promoting metastatic tumorigenesis. Similarly, PHF1 regulates FBXW7 transcription by negatively affecting the expression of both the FBXW7 protein and E-cadherin, which promotes tumor proliferation and invasion (Liu et al., 2018a). HES5, a downstream effector of Notch signaling, directly suppresses the transcription of FBW7β, influencing the differentiation patterns of intestinal cells in mice (Sancho et al., 2013). Since Notch is negatively regulated by FBXW7, a significant feedback loop exists between HES5, FBXW7, and Notch. Additionally, the inactivation of the TGF-β signaling pathway is strongly associated with HES5-mediated inhibition of FBXW7 transcription (Chen et al., 2021).
Non-coding RNAs (ncRNAs), which are not translated into proteins, play critical roles in various physiological and pathological processes, primarily by regulating messenger RNA (mRNA) at the post-transcriptional level (Anastasiadou et al., 2018). Several types of ncRNAs, including microRNAs (MiRNAs), Circular RNAs (CircRNAs), and Long non-coding RNAs (LncRNAs), have been implicated in the post-transcriptional regulation of FBXW7 (Lin et al., 2019). In cancer, miRNAs can suppress FBXW7 expression, thereby disrupting its tumor-suppressive functions (Lin et al., 2019; Ling et al., 2016). For instance, MiR-27a-3p and MiR-92a-3p downregulate FBXW7 mRNA expression, promoting tumor cell proliferation and invasion (Wang et al., 2021a; Ben et al., 2020). Similarly, MiRNAs such as MiR-182, MiR-25, and MiR-32 inhibit FBXW7 expression in various cancers, facilitating tumor growth and migration (Chang et al., 2018; Xiang et al., 2015; Xia et al., 2017). LncRNAs often function as MiRNA sponges to regulate FBXW7 expression. For example, LncRNA-MIF acts as a competing endogenous RNA (ceRNA) for miR-586, counteracting miR-586-mediated inhibition of FBXW7, which in turn suppresses c-Myc activity and tumorigenesis (Zhang et al., 2016a). Additionally, circular RNA circPSD3 sponges MiR-25-3p, thereby restoring FBXW7 expression and inhibiting epithelial-mesenchymal transition (EMT) and tumor metastasis (Xie et al., 2022).
The function and expression of FBXW7 are regulated by phosphorylation through several post-translational kinases, including ERK1/2,PI3K, Polo-like kinase-1 and -2 (PLK1/2), and cyclin-dependent kinase 5 (CDK5) (L et al., 2022; D et al., 2016; Cizmecioglu et al., 2012; Ko et al., 2019). ERK1/2 activation induces the phosphorylation of FBXW7 at Thr205, reducing its stability and leading to the accumulation of heat shock factor 1 (HSF1) (Gi et al., 2020). Similarly, PLK family members phosphorylate FBXW7, promoting its ubiquitin-mediated degradation and enhancing the oncogenic potential of Myc (D et al., 2016). The FBXW7α isoform is also phosphorylated in a PI3K-dependent manner at the S227 site (Schülein et al., 2011). Additionally, CDK5 phosphorylates FBXW7 at Ser349 and Ser372, resulting in its ubiquitination and degradation [22]. Phosphorylation by dual-specificity tyrosine-phosphorylation-regulated kinase 2 (DYRK2) has been reported to promote FBXW7 degradation via the proteasome, thereby impairing the turnover of oncogenic proteins (Jiménez-Izquierdo et al., 2023).
At the post-translational level, autoubiquitination and deubiquitinating enzymes (DUBs) collaboratively regulate the levels and functions of the FBXW7 protein (Xu et al., 2016). Sang’s colleagues demonstrated that Pin1 promotes FBXW7 autoubiquitination and degradation by disrupting its dimerization, thereby impairing its function (Min et al., 2012). Concurrently, Pin1 negatively regulates FBXW7-mediated substrate degradation, contributing to tumor development (Min et al., 2012). Additionally, tripartite motif-containing 25 (TRIM25) mediates the ubiquitination and degradation of FBXW7α, leading to increased stability and accumulation of Myc (Zhang et al., 2020). The HECT domain-based E3 ligase thyroid hormone receptor interactor 12 (TRIP12) promotes autoubiquitination and proteasomal degradation of FBXW7 by mediating K11-linked ubiquitination of lysine residues K404 and K412 (Khan et al., 2021a). Beyond genetic alterations, FBXW7 function is modulated by deubiquitinases. USP9X inhibits FBXW7 autoubiquitination, thereby reducing the activity of its downstream substrate c-Myc and suppressing tumor formation in mice (Khan et al., 2018). Similarly, USP28 prevents FBXW7 self-degradation in chronic lymphocytic leukemia (CLL), leading to elevated levels of Notch1 (Close et al., 2018).
FBXW7 forms dimers through its conserved D-domain to maintain stability and functional activity. This structural mechanism enhances the specificity and stability of FBXW7 binding to specific substrates (Welcker et al., 2013; Welcker and Clurman, 2007). Impaired dimerization of FBXW7 not only increases its autoubiquitination but also disrupts substrate ubiquitination and degradation. For instance, as previously discussed, Pin1 promotes FBXW7 autoubiquitination and degradation by interfering with its dimerization (Min et al., 2012). Similarly, LSD1 directly disrupts FBXW7 dimerization, leading to reduced stability and proteasomal degradation (Lan et al., 2019). These findings underscore the critical role of dimerization in regulating FBXW7 stability and its anti-tumor functions.
Cyclin E plays a critical role in the transition from the G1 phase to the S phase and regulates tumorigenesis, cell proliferation, and resistance to anticancer therapies (Chu et al., 2021; T et al., 2004). The normal progression of the cell cycle relies on the proper expression of cyclins and cyclin-dependent kinases (CDKs) (Mammalian cell cycle cyclins, 2024; Targeting cell, 2024). FBXW7 deficiency has been shown to enhance Cyclin E1/CDK activation, contributing to genomic instability and tumorigenesis (Minella et al., 2007; Grim et al., 2008; Takada et al., 2017). Cyclin E is a key downstream substrate of the miR-223/FBXW7 and miR-92a/FBXW7 regulatory pathways (Xu et al., 2010; M et al., 2011). Low levels of miR-223 or miR-27a result in FBXW7 upregulation, thereby inhibiting the ubiquitination and degradation of cyclin E (Xu et al., 2010; M et al., 2011). Consequently, FBXW7 plays a vital role in maintaining Cyclin E levels, and its dysfunction is sufficient to cause Cyclin E dysregulation (Ekholm-Reed et al., 2004).
Notch1, a member of the Notch family, plays a critical role in the initiation and progression of human malignancies (Notch1 in Cancer Therapy, 2024). The Notch signaling pathway is primarily involved in regulating embryonic development, normal cell growth, apoptosis, and differentiation (Notch signaling pathway in cancer, 2024). The intracellular domain of Notch1 (NICD) is a key target for FBXW7-mediated degradation (Kar et al., 2021). In mouse embryonic fibroblasts (MEFs), selective knockout of FBXW7 leads to aberrant activation of the Notch1/NICD signaling pathway, disrupting normal cell cycle regulation (Y et al., 2008). In various hematopoietic and solid tumors, impaired FBXW7-mediated degradation of Notch1 is associated with reduced drug response and poor patient prognosis (Close et al., 2019; Mori et al., 2018).
MCL-1, a member of the anti-apoptotic Bcl-2 protein family, is critically involved in tumor cell proliferation, apoptosis, and drug resistance (Wang et al., 2021b). The function and expression of MCL-1 are tightly regulated by various factors, including VEGF, IL-6, FBXW7, MULE, and MiRNAs (Mojsa et al., 2014; L et al., 2009; Shenoy et al., 2014; CXCR4, 2024; Inuzuka et al., 2011; Pervin et al., 2011). Specific alleles in the CPD domain of MCL-1—Ser159, Thr163, and Ser121—are phosphorylated by glycogen synthase kinase 3β (GSK3β) (Inuzuka et al., 2011; Morel et al., 2009; Senichkin et al., 2020). Subsequently, FBXW7 interacts with phosphorylated MCL-1, facilitating its degradation via the 26S proteasome (Yeh et al., 2018; Tang et al., 2023). Thus, FBXW7 influences multiple malignant processes in cancer, particularly drug resistance, by regulating MCL-1 activity or expression levels (Wu et al., 2024). In oral squamous cell carcinoma, FBXW7 has been reported to negatively regulate MCL-1 expression and autophagy (Sun et al., 2023). Furthermore, reduced FBXW7 expression decreases cisplatin sensitivity in oral squamous cell carcinoma (Yang et al., 2022). These findings suggest that targeting MCL-1 could enhance therapeutic sensitivity in patients with FBXW7-mutated gastrointestinal cancers (Wood, 2020; Mittal et al., 2021).
mTOR is a serine/threonine kinase belonging to the phosphoinositide 3-kinase-related kinase (PIKK) family, playing a pivotal role in tumor growth, metastasis, and drug resistance (Marques-Ramos and Cervantes, 2023; As, 2019). FBXW7 has been reported to recognize and degrade mTOR (Mao et al., 2008). Experiments have shown that the expression level of mTOR depends on the rhythmic change of FBXW7, which means that FBXW7 is a negative regulator of mTOR (Okazaki et al., 2014). This regulatory relationship has been well-demonstrated in breast cancer (Mao et al., 2008). Notably, FBXW7 knockout in tumor cells leads to increased epithelial-mesenchymal transition (EMT), enhanced stem cell properties, and greater migratory potential (Wang et al., 2013). Interestingly, mTOR inhibitors have been shown to suppress EMT and cancer stem cell traits induced by FBXW7 mutations (Wang et al., 2013). Furthermore, in murine tumor models undergoing radiotherapy, mTOR inhibitors delay tumor progression associated with FBXW7 mutations or deletions (Liu et al., 2013).
β-Catenin is a key effector of the canonical Wnt signaling pathway, which regulates cell proliferation, embryonic development, and homeostasis under physiological conditions (Liu et al., 2022; Song et al., 2024; Pai et al., 2017). Dysregulation of the Wnt/β-catenin pathway promotes malignant tumor invasion (Song et al., 2024; Zhang and Wang, 2020). Extensive research has demonstrated that this pathway is frequently overactivated in various cancers, particularly in gastrointestinal malignancies (Is et al., 2022; Bd et al., 2012; X C. et al., 2019a; Wu et al., 2016). FBXW7 plays a critical role in regulating the Wnt/β-catenin pathway by mediating the degradation of β-catenin and associated transcription factors (Yu et al., 2021). Additionally, chromatin domain-helicase-DNA-binding protein 4 (CHD4) promotes Wnt/β-catenin pathway activation, which FBXW7 counteracts by disrupting CHD4 (G et al., 2024). Overexpression of FBXW7 directly ubiquitinates and degrades β-catenin, thereby preventing pathway overactivation and inhibiting tumor growth and invasion (Jiang et al., 2016). Consequently, targeting the Wnt/β-catenin pathway is a promising strategy for treating FBXW7-mutated tumors. However, recent studies reveal resistance to Wnt/β-catenin inhibition therapy in FBXW7-mutant tumors, potentially due to increased activity of alternative FBXW7 substrates, such as Myc (Zhong and Virshup, 2024). This suggests that cancer proliferation and progression driven by FBXW7 mutations are not solely dependent on Wnt/β-catenin signaling and that targeting a single FBXW7 substrate may have limited therapeutic efficacy.
c-Myc is a proto-oncogene encoding a protein implicated in the development of numerous human cancers (Dhanasekaran et al., 2021). Aberrant activation of c-Myc drives hallmark features of cancer, including cell proliferation, growth, differentiation, metabolism, angiogenesis, and tumor microenvironment remodeling, through various genetic mechanisms (M et al., 2014). FBXW7 suppresses the oncogenic effects of c-Myc by ubiquitinating and degrading phosphorylated c-Myc at Thr58 and Ser62 (Yeh et al., 2018; M Y. et al., 2004). At the single-cell level, loss of FBXW7 expression results in the accumulation of several substrates, most notably c-Myc and cyclins (Meyer et al., 2020). This loss leads to the activation of c-Myc, contributing to the progression of various malignancies, particularly hematologic cancers (B et al., 2024; B et al., 2013). Findings by Markus et al. indicate that FBXW7 deletion disrupts control over c-Myc, resulting in rapid tumor cell proliferation (M W. et al., 2004).
FBXW7 mediates the degradation of numerous proteins, and alterations in its expression are strongly associated with cell proliferation, invasion, metastasis, and drug resistance in gastrointestinal cancers. Understanding the molecular mechanisms and clinical significance of FBXW7 in these cancers can aid in the development of targeted therapies and biomarkers. The following sections discuss the role of FBXW7 in the onset and progression of gastrointestinal cancers by tumor type, and summarize the associated molecular mechanisms and signaling pathways, as illustrated in Figure 3.
Figure 3. Interaction of FBXW7 with signaling pathways and key molecules in gastrointestinal cancers. (1) FBXW7 directly targets EGFR and SHOC2, blocking the activation of the MAPK signaling pathway. ERK1/2 promotes FBXW7 phosphorylation, further activating the MAPK pathway. (2) FBXW7 directly targets β-catenin, inhibiting the activation of the Wnt/β-catenin signaling pathway. (3) FBXW7 directly targets Nox1, PTEN, and mTOR, inhibiting the activation of the Akt/mTOR signaling pathway. (4) FBXW7 degrades MCL-1, thereby promoting apoptosis. (5) FBXW7 targets Notch1/NICD, inhibiting MAPK signaling. The degradation of Notch1/NICD inhibits cell proliferation. (6) FBXW7 mediates Cyclin E degradation, maintaining a normal cell cycle. (7) MicroRNA-770, MiR-92a-3p, MiR-27a, and MiR-223 inhibit FBXW7 expression.
CRC is a highly heterogeneous disease, with different molecular alterations and genetic subtypes closely related to the prognosis of CRC (Schell et al., 2016; Linnekamp et al., 2018). Currently, the treatment and prognostic classification of CRC primarily depend on TNM staging, as well as molecular markers such as MMR/MSI, RAS, RAF, and PI3K. Mutations or deletions of FBXW7 are often found in patients with CRC, especially in younger patients (<45 years) (Kothari et al., 2016). A recent meta-analysis of 58 studies found that the overall mutation rate of FBXW7 in 13,974 CRC patients was 10.3% (Afolabi et al., 2024). Thus, investigating the possible role of the FBXW7 gene in CRC is essential. The role of FBXW7 in CRC is shown in Table 1.
FBXW7 influences the growth and proliferation of CRC by regulating the activity of various oncogenic substrates, including Notch, Akt/mTOR, Jun, and DEK (Babaei-Jadidi et al., 2011; Lu et al., 2019; Liu et al., 2021b). Mice with FBXW7 deficiency exhibit an increased likelihood of developing intestinal tumors (Babaei-Jadidi et al., 2011). For instance, the synergistic downregulation of FBXW7 expression by MiR-182 and MiR-503 has been shown to drive the progression of colon adenoma into adenocarcinoma (Li et al., 2014). Ou et al. reported that elevated expression of Polo-like kinase 2 (Plk2) in CRC samples stabilizes Cyclin E by suppressing FBXW7 expression, thereby promoting CRC growth (Ou et al., 2016). Similarly, the Rictor/FBXW7-mediated accumulation of c-Myc and Cyclin E plays a crucial role in CRC cell proliferation (Guo et al., 2012). Additionally, factors such as Serine/threonine/tyrosine interacting protein (STYX), MiR-223, MiR-92b-3p, FAM83D, USP9X, and KDM5c indirectly regulate various oncoproteins by modulating FBXW7 expression, contributing to CRC cell proliferation and apoptosis (Khan et al., 2018; Liu Z. et al., 2021; Gong et al., 2018; He et al., 2019; Mu et al., 2017; Lin H. et al., 2020). Fat metabolism, regulated by FBXW7, is also linked to tumor growth in CRC. The binding of COP9 signalosome subunit 6 (CSN6) to FBXW7β inhibits the degradation of fatty acid synthase (FASN), promoting lipogenic CRC and tumor progression (Wei et al., 2023).
FBXW7 plays a significant role in the invasion and metastasis of CRC. It has been reported to suppress the migration of CRC cells by regulating the HIF1α/CEACAM5 axis (Li et al., 2018). Furthermore, the FBXW7 gene negatively regulates Enolase 1 (ENO1), thereby influencing the growth and metastasis of the CRC cell line HCT116 (163). SHOC2, a conserved protein that binds to RAS and RAF at its N-terminus, facilitates downstream signaling (Xie and Sun, 2019). FBXW7-mediated ubiquitination and degradation of SHOC2 block MAPK pathway activation, thereby inhibiting cancer growth signaling (Xie and Sun, 2019). In FBXW7 knockout CRC cells, increased epithelial-mesenchymal transition (EMT), enhanced stem cell properties, and elevated migration are observed (Wang et al., 2013). The use of mTOR inhibitors can mitigate EMT characteristics and cancer stem cell properties induced by FBXW7 mutations (Wang et al., 2013). Additionally, restoring FBXW7 expression partially suppresses CRC progression driven by the activation of the Wnt/β-catenin signaling pathway (Hu et al., 2019).
Loss of FBXW7 function reduces the sensitivity of CRC cells to anticancer drugs. In CRC cell models, FBXW7 downregulation increases the activity of NADPH oxidase 1 (Nox1) and mTOR, leading to resistance to paclitaxel and cisplatin (Huang and Long, 2023; Wang HP. et al., 2021). Additionally, FBXW7 deletion upregulates ZEB2 protein levels, inducing chemoresistance to 5-fluorouracil and oxaliplatin (Li et al., 2019). Conversely, high FBXW7 expression downregulates Cryptochrome 2 (CRY2), reducing resistance to oxaliplatin treatment (Fang et al., 2015). FBXW7 also plays a critical role in the effectiveness of molecularly targeted therapies for CRC. Sequencing data from CRC patients reveal that mutations in FBXW7 and SMAD4 are common in those resistant to cetuximab or panitumumab (Lupini et al., 2015). Recently, FBXW7 was shown to mediate the ubiquitination and degradation of EGFR, influencing the efficacy of EGFR inhibitors in human CRC-derived organoid lines (Boretto et al., 2024; Nemecek et al., 2016). Moreover, CRC cells harboring FBXW7 mutations exhibit resistance to regorafenib and sorafenib due to MCL-1 accumulation (Tong et al., 2017; Song et al., 2020). However, inhibiting MCL-1 restores the sensitivity of these cells to regorafenib (Tong et al., 2017; Song et al., 2020). Similar mechanisms underlie resistance to trametinib and Hsp90 inhibitors in FBXW7-mutant CRC (Lin L. et al., 2020; J et al., 2017). Interestingly, FBXW7 downregulation has been reported to enhance the therapeutic response of CRC stem cells to anticancer drugs (Honma et al., 2019). CRC stem cells may develop chemoresistance to CPT-11 by upregulating FBXW7, which degrades c-Myc and arrests the cell cycle (Izumi et al., 2017). Thus, further research on FBXW7 could aid in identifying novel predictive markers and treatment strategies for CRC.
The functional status of FBXW7 may be linked to poorer prognosis in CRC patients (Liu H. et al., 2018; Korphaisarn et al., 2017). Specifically, reduced FBXW7 mRNA expression in tumor tissues is inversely associated with CRC prognosis (Iwatsuki et al., 2010). A meta-analysis further confirmed that FBXW7 mutations or low expression levels correlate with advanced T stage, shorter overall survival (OS), and lymph node metastases in CRC patients (Shang et al., 2021). Additionally, CRC patients with liver metastases who have FBXW7 mutations exhibit significantly lower 5-year OS rates compared to those with FBXW7 wild-type tumors (Kawaguchi et al., 2021; Kawashita et al., 2017). However, some studies indicate that the relationship between FBXW7 and patient prognosis may not be strong (Chang et al., 2015). For instance, Liu et al. found that while the R465C hotspot mutation was associated with worse OS, other FBXW7 mutations did not significantly affect survival outcomes (Y et al., 2023). Although FBXW7 appears to influence CRC prognosis, further research is required to establish more conclusive evidence. Nonetheless, FBXW7 shows significant potential as a biomarker for CRC treatment and prognosis.
According to recent statistics, gastric cancer (GC) ranks fifth in cancer-related mortality (Bray et al., 2024). Molecular biomarker-based classification, including markers such as PD-L1, MSI, and HER-2, has enabled some GC patients to benefit from immunotherapy or targeted therapies (Guan et al., 2023). However, there are few treatment options for patients with GC and the prognosis is still relatively poor (Sun et al., 2024). Thus, identifying more effective molecular markers is critical for improving the management of GC. Studies have reported mutations rate of FBXW7 in GC ranging from 9.2% to 18.5% (Li et al., 2016). More and more researchers have paid attention to the role of FBXW7 in GC (Table 2).
Studies have shown that FBXW7-deficient mice exposed to the chemical carcinogen N-methyl-N-nitrosourea (MNU) are more prone to genetic damage and c-Myc accumulation, increasing the risk of gastric cancer (GC) (Jiang et al., 2017). In addition, MiR-223 and MiR-25 can indirectly regulate GC cell proliferation, apoptosis, and invasiveness by controlling the post-transcriptional expression of FBXW7 (191,192). Certain compounds, such as Demethylzeylasteral (a naturally occurring monomer from Tripterygium wilfordii) and Lycorine hydrochloride (LH), can restore FBXW7 expression, thereby reducing the growth and invasiveness of GC cells (Y et al., 2021; Li et al., 2020). Growth factor independent 1 (GFI1) encourages the growth of GC cells and disease progression by inhibiting the transcription of gastric factor-2 (GKN2) (X K. et al., 2019). In GC patients with elevated GFI1 protein levels, FBXW7 function were reduced, leading to rapid GC cell proliferation and disease progression (X K. et al., 2019). Li et al. demonstrated that low FBXW7 expression activates the RhoA pathway in GC, promoting EMT and disease progression (Li et al., 2016). ZC3H15 inhibits FBXW7 expression at the transcriptional level, hindering c-Myc degradation and thereby contributing to GC development and progression (Hou et al., 2022). Higher Brg1 (also known as SMARCA4) expression has been linked to distant and lymph node metastasis in GC patients (Sentani et al., 2001). Huang et al. analyzed human GC tissue samples and found that increased Brg1, due to low FBXW7 expression, is a key mechanism driving GC metastasis (Huang et al., 2018). Moreover, FBXW7 is negatively regulated by lncRNA BDNF-AS and is involved in ferroptosis and peritoneal metastasis of GC in animal models (Huang et al., 2022).
The functional status of FBXW7 also profoundly affects the treatment resistance and prognosis of GC patients. It has been reported that MiR-223 modulates the sensitivity of GC to chemotherapy and molecularly targeted therapy (Zhou et al., 2015; Eto et al., 2015). Mechanistic studies have shown that MiR-223 induces resistance to cisplatin and trastuzumab in GC cells by downregulating FBXW7 expression (Zhou et al., 2015; Eto et al., 2015). The restoration of FBXW7 function by consuming MiR-223 inhibits the resistance of GC cells to cisplatin trastuzumab (Zhou et al., 2015; Eto et al., 2015). Furthermore, in patients with GC, dysregulated FBXW7 is related to more severe clinical characteristics and a worse prognosis (Yokobori et al., 2009). For Chinese patients, the results also suggest that GC patients with low expression of FBXW7 have poor tumor differentiation and worse prognosis (Li MR. et al., 2017). In summary, the function of FBXW7 acts on the whole process of the occurrence and development of GC, which offers an innovative approach for the static treatment of patients.
In ESCC, low FBXW7 expression is related to high aggressiveness, while FBXW7 overexpression significantly inhibits tumor growth and invasion (Gong et al., 2016; Bi et al., 2023). FBXW7 deficiency in ESCC can overactivate the ANXA2-ERK pathway, worsening the tumor’s biological behavior (Li Z. et al., 2023). In addition, FBXW7-MAP4-ERK axis is implicated in ESCC(207). MAP4 overexpression and ERK activation caused by FBXW7 inactivation can promote the growth, invasion and migration of ESCC cells (Pan et al., 2023). Upregulation of MiR-27a-3p in ESCC has been shown to reduce FBXW7 expression, leading to accelerated tumor proliferation and disease progression (Wu et al., 2015). Moreover, the functional status of FBXW7 can also affect the prognosis of ESCC patients (Yu et al., 2015; Y et al., 2010). In ESCC, both FBXW7 mutations and copy number loss affect the prognosis of patients (Yokobori et al., 2012). When the expression of FBXW7 in ESCC is inhibited by MiR-223, the prognosis of patients becomes worse (Kurashige et al., 2012). A retrospective study found that high FBXW7 expression was correlated with a favorable response to chemoradiotherapy (CRT) in advanced ESCC(213). Therefore, the status of FBXW7 is also an important indicator for predicting the efficacy of ESCC treatment (Table 3).
Studies from small samples have reported that the frequency of FBXW7 mutations in hepatocellular carcinoma (HCC) tissues is approximately 7.7% (1/13) (Dl et al., 2014). Patients with HCC have a worse prognosis and a more aggressive tumor when FBXW7 expression is lost (Table 4) (Wang et al., 2015; Zhou et al., 2012; Feng et al., 2022). The functional impairment of FBXW7 in HCC is connected to accumulation of the Notch1 and poorer clinicopathological features (Wang et al., 2015). The Assembly Factor for Spindle Microtubules (ASPM) promotes tumorigenesis in HCC by preventing the binding and degradation of Notch1 by FBXW7 (218). Except for Notch1, FBXW7 can control the invasion ability and prognosis of HCC by regulating the stability of YAP protein and Myc protein (Tu et al., 2014; Zhang et al., 2020). Moreover, the level of FBXW7 in HCC cells was found to be controlled by a variety of regulators. For instance, by controlling FBXW7 expression, FAM83D, MiR-27b, MiR-92a, and MiR-155-3p affect the development and prognosis of HCC (219–222).
Pancreatic cancer (PC) is a high-threat gastrointestinal cancer characterized by its insidious onset and resistance to chemotherapy (Lin et al., 2022). Due to the significant heterogeneity and limited treatment options for PC, the survival rate for patients with advanced disease remains extremely low (Pancreatic Cancer). Therefore, identifying new therapeutic targets and biomarkers is crucial. The mutation rate of FBXW7 in PC has been reported to be approximately 2%–3% (Heestand and Kurzrock, 2015).
FBXW7 plays a crucial role in the differentiation of pancreatic ductal epithelial cells (Sancho et al., 2014). Elevated levels of Ngn3 after FBXW7 inactivation can induce the differentiation potential of pancreatic ductal cells, which may lead to the development of PC (Sancho et al., 2014; Ba and Y, 2014). Zhang et al. showed that deletion of FBXW7 accelerates KRAS G12D-driven pancreatic tumorigenesis (Zhang Q. et al., 2016). Mechanistic studies have shown that FBXW7 loss in mice depends on Yap accumulation to drive pancreatic tumor formation (Zhang Q. et al., 2016). The activation of the KRAS-ERK pathway in PC further suppresses FBXW7 expression, facilitating cancer progression (Ji et al., 2015). This occurs because the ERK pathway degrades FBXW7 in a phosphorylation-dependent manner, and pancreatic cancer cells with defective FBXW7 phosphorylation sites exhibit oncogenic resistance to ERK pathway activation (Ji et al., 2015). FBXW7 has been reported to hinder PC cell growth and invasion by inhibiting Wnt/β-catenin signaling (Jiang et al., 2016; Liu et al., 2019). Protein database analysis suggests that FBXW7 also inhibits the downstream TORC2/AKT signaling pathway by degrading salt-inducible kinase 2 (SIK2), thereby reducing pancreatic cancer cell proliferation (Mx et al., 2020). Moreover, FBXW7 suppresses tumor aggressiveness by degrading other substrates, such as Enhancer of zeste homolog 2 (EZH2) (Jin et al., 2017). The epigenetic regulator protein arginine methyltransferase 5 (PRMT5) stabilizes c-Myc levels in pancreatic cancer cells by downregulating FBXW7, promoting tumor cell proliferation (Qin et al., 2019).
FBXW7 can predict the response of PC to chemotherapy drugs. On one hand, FBXW7 can enhance the therapeutic response to gemcitabine by inhibiting the expression of proteins like stearoyl-CoA desaturase (SCD) or increasing the expression of equilibrative nucleoside transporter 1 (ENT1) (Ye et al., 2020; Q et al., 2017). And on the other hand, the low expression of FBXW7 can promote resistance to gemcitabine and paclitaxel in pancreatic cancer (PC) cells by leading to the accumulation of MCL-1 (240). Consequently, it is anticipated that FBXW7 function restoration will enhance the therapeutic efficacy and prognosis of PC patients (Table 5).
The invasiveness and sensitivity of HCC cells to doxorubicin are also affected by FBXW7 levels. Restoring FBXW7 expression in HCC cells reduces their invasiveness and enhances the efficacy of doxorubicin (Yu et al., 2014). Sorafenib, commonly used in patients with advanced liver cancer, faces significant challenges due to drug resistance. FBXW7 has been implicated in sorafenib resistance in HCC cells (Feng et al., 2022; Tang et al., 2019). This resistance is linked to the downregulation of FBXW7 by MiR-223 and MiR-25, and can be reversed by restoring FBXW7 levels (Feng et al., 2022; Tang et al., 2019). For prognosis, HCC patients with high FBXW7 mRNA expression exhibit better DFS compared to those with low expression (S et al., 2014). Survival analysis indicates that low FBXW7 expression is an independent risk factor for predicting HCC recurrence (S et al., 2014). Therefore, FBXW7 may be a molecular marker in the treatment and prognosis assessment of HCC (Tu et al., 2014; El-Mezayen et al., 2021).
The mutation rate of FBXW7 in extrahepatic cholangiocarcinoma (CCA) has been reported to be 15% (n = 20) (Churi et al., 2014). Wang et al. demonstrated that FBXW7 deletion and AKT pathway activation synergistically upregulated the expression of c-Myc in animal experiment, contributing to the occurrence of intrahepatic cholangiocarcinoma (iCCA) (Wang et al., 2019). Overexpression of FBXW7 enables CCA cells to restore normal c-Myc expression levels, which inhibits cell proliferation and progression (Li M. et al., 2017). Consequently, they proposed a novel therapeutic strategy for iCCA that targets the FBXW7-c-Myc pathway (Wang et al., 2019; Li M. et al., 2017). Besides c-Myc, FBXW7 regulates the progression of CCA cells and their resistance to cisplatin by targeting other substrates, including Notch1 and MCL-1 (Mori et al., 2018). The loss of FBXW7 expression can promote EMT and CSC development in CCA, resulting in tumors with increased malignant potential (H et al., 2015). Studies indicate that FBXW7-deficient CCA exhibits poor differentiation, a propensity for regional lymph node metastasis, and a later tumor stage (H et al., 2015; Enkhbold et al., 2014). Furthermore, FBXW7 expression is closely linked to the prognosis of CCA patients. Patients with low FBXW7 expression have significantly poorer outcomes, indicating that FBXW7 levels are a critical prognostic factor for CCA (Mori et al., 2018; Enkhbold et al., 2014). Recent research has revealed that numerous cytokines and chemokines are upregulated in CCA with FBXW7 mutations, showing favorable responses to immunotherapy (Feng et al., 2024). These findings suggest that FBXW7-mutant CCA may possess a distinct tumor immune microenvironment, making FBXW7 expression status a potential indicator of immunotherapy efficacy.
Approximately 75% of patients with gastrointestinal stromal tumors (GIST) harbor functional mutations in KIT (Shima et al., 2024). Imatinib, a KIT inhibitor, is the primary treatment for inoperable or metastatic GIST. However, the occurrence of drug resistance remains inevitable (Blanke et al., 2008). Current research has emphasized the role of FBXW7 in predicting GIST treatment resistance and prognosis. Both in vivo and in vitro experiments demonstrate that FBXW7 expression enhances sensitivity to imatinib treatment in GIST by downregulating MCL-1 (Wu et al., 2024). Following radical resection, GIST patients with high FBXW7 expression exhibit better prognoses and lower recurrence rates (Koga et al., 2019). The incidence of FBXW7 mutations in rectal neuroendocrine tumors (NETs) is reported to be 25% (Venizelos et al., 2021). In rectal NETs, the levels of FBXW7 mutations and non-coding RNAs may correlate with the Ki67 index, though further research is required to confirm this relationship (Kang et al., 2023). Table 6 illustrates the role of FBXW7 in CCA and other gastrointestinal cancers.
As previously noted, FBXW7 is pivotal in the pathogenesis, progression, treatment resistance, and poor prognosis of gastrointestinal cancers, thereby presenting opportunities for targeted therapy. We propose several promising strategies for the treatment of cancer by FBXW7 as shown in Figure 4. At the same time, we also propose the current challenges of FBXW7 in the treatment of cancer.
Figure 4. Strategies for targeting FBXW7 in the treatment of cancers (A). Inhibition of regulators such as miRNA and ERK can upregulate the expression of FBXW7. Additionally, inhibiting FBXW7 downstream substrates, such as MCL-1 and mTOR, may reduce cancer cell proliferation and drug resistance (B). Inhibiting FBXW7 expression in cancer stem cells (CSCs) can awaken cell cycle-arrested CSCs, making them more sensitive to treatment.
Aberrant FBXW7 expression is a key factor contributing to poor cancer prognosis, drug resistance, and treatment challenges (Khan AQ. et al., 2021). Restoring or increasing FBXW7 expression has been identified as a viable strategy to halt cancer progression and improve treatment outcomes (Figure 4). Several studies have shown that modulating MiR-223 levels can indirectly upregulate FBXW7 expression (Liu Z. et al., 2021; Kurashige et al., 2012). As previously reported, MiR-223-mediated inhibition of FBXW7 leads to GC resistance to trastuzumab and cisplatin, whereas reducing MiR-223 levels reverses this resistance (Zhou et al., 2015; Eto et al., 2015). Notably, genistein has been found to downregulate MiR-223 and upregulate FBXW7 expression (Ma et al., 2013). Elevated FBXW7 expression subsequently inhibits the growth and aggressiveness of PC cells (Ma et al., 2013). Recent findings also suggest that inhibiting ERK, another upstream regulator of FBXW7, improves the sensitivity of CRC cells to chemotherapeutic agents (Ruan et al., 2024). Mechanistically, this is attributed to Clitocine, an adenosine analog, which promotes FBXW7 expression and MCL-1 degradation by preventing the A2B/cAMP/ERK signaling axis (Ruan et al., 2024). Thus, targeting upstream regulators of FBXW7 presents a feasible approach to enhance drug sensitivity in gastrointestinal cancers.
FBXW7 exerts anti-tumor effects by regulating the degradation of various downstream oncoproteins. Thus, developing drugs that target key carcinogenic substrates may counteract the carcinogenic effects resulting from FBXW7 inactivation. For example, selective MCL-1 inhibitors restore the sensitivity of CRC cells with FBXW7 mutations to regorafenib (Tong et al., 2017). It has been found that deguelin can promote the destruction of MCL-1 by FBXW7, which is beneficial to the apoptosis of cancer cells (Gao et al., 2020). Furthermore, FBXW7 deficiency-induced resistance of CRC to Hsp90 inhibitors can be overcome by selective MCL-1 inhibitors (J et al., 2017). Research on MCL-1 inhibitors has garnered significant attention and may be applied in clinical treatment in the near future (Wang H. et al., 2021; Chen and Fletcher, 2017). Alongside MCL-1 inhibitors, mTOR inhibitors also exhibit significant anti-cancer effects on tumors with FBXW7 mutations (Mao et al., 2008). FBXW7 deletion leads to increased EMT and aggressiveness in CRC cell lines, which is mediated by overactivation of the mTOR pathway (Wang et al., 2013). Subsequently, mTOR inhibitors were applied to CRC cells with FBXW7 deficiency, resulting in significant inhibition of EMT and the aggressiveness of cancer cells (Wang et al., 2013). Therefore, inhibiting downstream oncoproteins to treat gastrointestinal cancers with FBXW7 deletions or mutations has the potential to enhance the efficacy of anticancer therapy.
FBXW7 plays a critical role in regulating the differentiation and malignant transformation of various stem/progenitor cells, including pluripotent stem cells, hematopoietic stem cells, intestinal stem cells, and neural stem cells (N et al., 2015; Takeishi and Nakayama, 2014). Additionally, FBXW7 is involved in the characterization of CSCs by modulating key oncoproteins, such as c-Myc and Notch1, in human malignancies (G et al., 2024; Li et al., 2019). For instance, FBXW7 contributes to the self-renewal and stem-like properties of hepatocellular carcinoma (HCC) cells by promoting the degradation of Actin-like 6A (ACTL6A) (X et al., 2022). CSCs, a small subset of tumor cells with the capacity for self-renewal and differentiation, play a pivotal role in tumor recurrence, progression, and resistance to treatment. While cytotoxic drugs target proliferating tumor cells, CSCs in a quiescent state are often resistant to such treatments (Takeishi and Nakayama, 2016). In non-small cell lung cancer (NSCLC), FBXW7 maintains gefitinib-resistant CSCs in a quiescent state by targeting the degradation of c-Myc (Hidayat et al., 2019). Moreover, downregulation of FBXW7 gene expression has been shown to significantly decrease the number of cells in the G0/G1 phase (Hidayat et al., 2019). In chronic myeloid leukemia, FBXW7 preserves dormant leukemia-initiating cells (LICs) through a similar mechanism, and its removal can awaken quiescent LICs, restoring sensitivity to imatinib (Takeishi et al., 2013). Elevated FBXW7 expression has also been detected in drug-resistant colorectal cancer (CRC) stem cells, with FBXW7 silencing enhancing sensitivity to anticancer drugs (Honma et al., 2019). These findings suggest that silencing FBXW7 may help convert CSCs from a quiescent to a proliferative state, potentially improving treatment resistance. However, this effect is primarily directed at CSCs or tumor cells with stem-like properties, which represent only a small portion of the overall tumor.
FBXW7 is frequently inactivated in cancer through mutations, deletions, or promoter hypermethylation (Fan et al., 2022). Therefore, restoring the function of the FBXW7 cancer suppressor gene in tumor cells lacking expression at the molecular and genetic levels represents a potential therapeutic strategy. Evidence from mouse lung cancer models indicates that decitabine promotes FBXW7 expression, leading to MCL-1 degradation and enhanced sensitivity to anticancer drugs (DiNardo et al., 2018). Decitabine, as a DNMT1 inhibitor, induces a shift from hypermethylation to unmethylation of the FBXW7 promoter, thereby facilitating FBXW7 transcription (DiNardo et al., 2018). As discussed in Section 3, FBXW7 protein levels are regulated by autoubiquitination and deubiquitinases (DUBs). USP9X prevents the ubiquitination and degradation of FBXW7 in mouse models and inhibits intestinal tumor development (Khan et al., 2018). In tumors with downregulated FBXW7 expression, it may be possible to counteract FBXW7 self-ubiquitination and degradation by developing deubiquitinase-targeting chimeric (DUBTAC) technology to sustain FBXW7 expression and function (Henning et al., 2022; Willson, 2022). Furthermore, the development of proteolysis-targeting chimeric (PROTAC) technology, where two covalently linked PROTAC molecules recruit specific substrates and FBXW7, could enhance the degradation efficiency of FBXW7 (Li et al., 2022). Although research in this area is limited, the potential significance of DUBTACs and PROTACs will become clearer as the functional mechanisms of FBXW7 are further explored.
The FBXW7 gene is frequently mutated or deleted in human tumors, leading to impaired tumor suppression. Currently, restoring the tumor-suppressive function of FBXW7 in vivo through gene editing or gene transfer therapies remains challenging (Fan et al., 2022). No drug or therapeutic technology currently available targets the FBXW7 gene directly for cancer treatment. While PROTAC and DUBTAC approaches may prove useful in tumors with low FBXW7 activity, they are ineffective in tumors with FBXW7 mutations or deletions. A large network of regulators and oncogenic substrates surrounds FBXW7, offering multiple potential pathways for cancer treatment. However, the tumor-suppressive effects of FBXW7 arise from the integrated function of this regulatory network, making it difficult to predict whether targeting a single pathway will produce the desired therapeutic effect. As noted earlier, there is resistance to Wnt/β-catenin pathway inhibition in FBXW7-mutant tumors (Zhong and Virshup, 2024), which is linked to the activation of other carcinogenic substrates regulated by FBXW7. However, simultaneously targeting multiple oncogenic substrates or regulators may lead to unforeseen toxic side effects. Furthermore, the knockdown of FBXW7 to enhance the sensitivity of dormant cancer stem cells (CSCs) to therapeutic agents presents a dual challenge. CSCs, while a small fraction of the overall tumor, tend to increase as the disease progresses. Whether the enhanced drug sensitivity resulting from FBXW7 knockdown can counterbalance the tumor-suppressive dysfunction caused by FBXW7 deletion requires further investigation.
FBXW7 is a substrate recognition component of the SCF-type E3 ubiquitin ligase complex, frequently inactivated or mutated in human cancers. Given the wide range of FBXW7 functions in cancer, this review focuses on its role in gastrointestinal cancers. As a recognized tumor suppressor gene, the inactivation of FBXW7 promotes tumorigenesis, proliferation, differentiation, invasion, and apoptosis in gastrointestinal tumor cells by interacting with the EGFR-MAPK, Wnt/β-catenin, and PI3K/Akt/mTOR signaling pathways (Wu et al., 2016; Liu Z. et al., 2021; Xie and Sun, 2019; Hu et al., 2019; Huang and Long, 2023; Pan et al., 2023; Ji et al., 2015; Escobar et al., 2022; R et al., 2023). In addition, FBXW7 is involved in the aggressiveness and drug resistance of gastrointestinal cancers by mediating the degradation of downstream substrates such as Cyclin E, MCL-1, and Notch1 (Ou et al., 2016; Guo et al., 2012; Lin et al., 2020b; J et al., 2017; Wang et al., 2015). Therefore, FBXW7 forms a complex and extensive regulatory network in gastrointestinal cancers by modulating various signaling pathways and substrate proteins. Both in vitro and in vivo studies have shown that the loss of FBXW7 expression leads to the accumulation of oncogenic proteins, which is strongly connected to the initiation, development, metastasis, and drug resistance of various gastrointestinal cancers, including CRC, GC, ESCC, HCC, CCA, and PC (Wei et al., 2023; Khan et al., 2018; Mori et al., 2018; Ou et al., 2016; Gong et al., 2018; He et al., 2019; Mu et al., 2017; Lin H. et al., 2020; Li et al., 2018; Zhan et al., 2015; Huang and Long, 2023; Wang et al., 2021c; Kawashita et al., 2017; Jiang et al., 2017; Li et al., 2012; Gong et al., 2015; Y et al., 2021; Li et al., 2020; X et al., 2019b; Hou et al., 2022; Zhou et al., 2015; Eto et al., 2015; Bi et al., 2023; Li et al., 2023b; Wu et al., 2015; Y et al., 2010; Zhou et al., 2012; Feng et al., 2022; Chan et al., 2024b; Yang et al., 2015; Yu et al., 2014; Zhang Q. et al., 2016; Mx et al., 2020; Ye et al., 2020; Wang et al., 2019). Impaired FBXW7 function in gastrointestinal cancers is generally associated with poor patient outcomes (Liu H. et al., 2018; Iwatsuki et al., 2010; Kawaguchi et al., 2021; Yokobori et al., 2009; Li MR. et al., 2017; Gombodorj et al., 2018; S et al., 2014; Feng et al., 2024; Koga et al., 2019). Therefore, strategies aimed at targeting FBXW7 may offer potential therapeutic approaches for cancer treatment. Although no drugs currently directly target FBXW7, promising therapeutic strategies have been proposed based on existing research (Zeng et al., 2024). Future treatments may focus on restoring or replacing FBXW7 expression by targeting its upstream regulators, such as MiRNA and ERK, or by inhibiting downstream oncoproteins such as MCL-1, mTOR, and c-Myc (Zhou et al., 2015; Eto et al., 2015; Ma et al., 2013; Ruan et al., 2024; Gao et al., 2020). The numerous regulators and substrates of FBXW7 provide a wide range of targets for treating gastrointestinal cancers. However, these treatment strategies are often difficult to implement, with uncertain efficacy and significant side effects. To address the key challenges currently faced, the following approaches may be considered: i) Identifying and inhibiting the most critical carcinogenic substrates or upstream regulators of FBXW7 to maximize its tumor-suppressive function. ii) Activating or enhancing the activity of other tumor suppressor genes in the presence of FBXW7 mutations or deletions. iii) Tailoring strategies based on tumor stage and the number of CSCs present to minimize the adverse effects of FBXW7 knockdown.
In conclusion, FBXW7 has the potential to be an important molecular marker for the treatment and prognosis of gastrointestinal cancers. Continued research into the tumor biology and molecular mechanisms of FBXW7 will offer valuable insights for improving the precision treatment of gastrointestinal cancer patients.
WW: Investigation, Project administration, Software, Visualization, Writing–original draft. XL: Investigation, Software, Writing–original draft. LZ: Supervision, Writing–original draft. KJ: Software, Writing–original draft. ZY: Software, Writing–original draft. RY: Investigation, Writing–original draft. WZ: Investigation, Writing–original draft. JC: Conceptualization, Supervision, Writing–review and editing. TL: Conceptualization, Funding acquisition, Resources, Supervision, Validation, Writing–review and editing.
The author(s) declare that financial support was received for the research, authorship, and/or publication of this article. This work was supported by Jilin Provincial Education Department (grant number: 3D5196778428), and Jilin Provincial Finance Department (grant number: 3D5214495428).
The authors declare that the research was conducted in the absence of any commercial or financial relationships that could be construed as a potential conflict of interest.
The author(s) declare that Generative AI was used in the creation of this manuscript. We acknowledge Biorender (https://www.biorender.com/) since Graphical Abstract, Figures 1–4 of this manuscript were made using this software.
All claims expressed in this article are solely those of the authors and do not necessarily represent those of their affiliated organizations, or those of the publisher, the editors and the reviewers. Any product that may be evaluated in this article, or claim that may be made by its manufacturer, is not guaranteed or endorsed by the publisher.
Afolabi, H. A., Salleh, S. M., Zakaria, Z., Seng, C. E., Nafi, N. M., Bin AbdulAziz, A. A., et al. (2024). Targeted variant prevalence of FBXW7 gene mutation in colorectal carcinoma propagation. The first systematic review and meta-analysis. Heliyon 10 (11), e31471. doi:10.1016/j.heliyon.2024.e31471
Anastasiadou, E., Jacob, L. S., and Slack, F. J. (2018). Non-coding RNA networks in cancer. Nat. Rev. Cancer 18 (1), 5–18. doi:10.1038/nrc.2017.99
As, A. (2019). PI3K/Akt/mTOR inhibitors in cancer: at the bench and bedside. Semin. Cancer Biol. 59, 125–132. doi:10.1016/j.semcancer.2019.07.009
B, K., T, T., L, R., L, X., J, M., P, N., et al. (2013). The ubiquitin ligase FBXW7 modulates leukemia-initiating cell activity by regulating MYC stability. Cell 153 (7), 1552–1566. doi:10.1016/j.cell.2013.05.041
B, F., Pa, C., Bj, V. F., El, R., V, R., B, I., et al. (2024). A germline point mutation in the MYC-FBW7 phosphodegron initiates hematopoietic malignancies. Genes Dev. 38 (5–6), 253–272. doi:10.1101/gad.351292.123
Ba, S., and Y, X. (2014). Out of the F-box: reawakening the pancreas. Cell Stem Cell 15 (2), 111–112. doi:10.1016/j.stem.2014.07.006
Babaei-Jadidi, R., Li, N., Saadeddin, A., Spencer-Dene, B., Jandke, A., Muhammad, B., et al. (2011). FBXW7 influences murine intestinal homeostasis and cancer, targeting Notch, Jun, and DEK for degradation. J. Exp. Med. 208 (2), 295–312. doi:10.1084/jem.20100830
Bakos, G., Yu, L., Gak, I. A., Roumeliotis, T. I., Liakopoulos, D., Choudhary, J. S., et al. (2018). An E2-ubiquitin thioester-driven approach to identify substrates modified with ubiquitin and ubiquitin-like molecules. Nat. Commun. 9, 4776. doi:10.1038/s41467-018-07251-5
Balamurugan, K., Wang, J. M., Tsai, H. H., Sharan, S., Anver, M., Leighty, R., et al. (2010). The tumour suppressor C/EBPδ inhibits FBXW7 expression and promotes mammary tumour metastasis. EMBO J. 29 (24), 4106–4117. doi:10.1038/emboj.2010.280
Bd, W., Aj, C., and Dw, D. (2012). Dysregulation of Wnt/β-catenin signaling in gastrointestinal cancers. Gastroenterology 142 (2), 219–232. doi:10.1053/j.gastro.2011.12.001
Ben, W., Zhang, G., Huang, Y., and Sun, Y. (2020). MiR-27a-3p regulated the aggressive phenotypes of cervical cancer by targeting FBXW7. Cancer Manag. Res. 12, 2925–2935. doi:10.2147/CMAR.S234897
Ben-Aharon, I., van Laarhoven, H. W. M., Fontana, E., Obermannova, R., Nilsson, M., and Lordick, F. (2023). Early-onset cancer in the gastrointestinal tract is on the rise-evidence and implications. Cancer Discov. 13 (3), 538–551. doi:10.1158/2159-8290.CD-22-1038
Bengoechea-Alonso, M. T., and Ericsson, J. (2010). Tumor suppressor Fbxw7 regulates TGFβ signaling by targeting TGIF1 for degradation. Oncogene 29 (38), 5322–5328. doi:10.1038/onc.2010.278
Bi, Y., Yang, Y., Zhang, Y., Cheng, C., Tang, P., Xiao, H., et al. (2023). FBXW7 inhibits the progression of ESCC by directly inhibiting the stemness of tumor cells. Neoplasma 70 (6), 733–746. doi:10.4149/neo_2023_230104N8
Blanke, C. D., Rankin, C., Demetri, G. D., Ryan, C. W., von Mehren, M., Benjamin, R. S., et al. (2008). Phase III randomized, intergroup trial assessing imatinib mesylate at two dose levels in patients with unresectable or metastatic gastrointestinal stromal tumors expressing the kit receptor tyrosine kinase: S0033. J. Clin. Oncol. Off. J. Am. Soc. Clin. Oncol. 26 (4), 626–632. doi:10.1200/JCO.2007.13.4452
Boretto, M., Geurts, M. H., Gandhi, S., Ma, Z., Staliarova, N., Celotti, M., et al. (2024). Epidermal growth factor receptor (EGFR) is a target of the tumor-suppressor E3 ligase FBXW7. Proc. Natl. Acad. Sci. U. S. A. 121 (12), e2309902121. doi:10.1073/pnas.2309902121
Bray, F., Laversanne, M., Sung, H., Ferlay, J., Siegel, R. L., Soerjomataram, I., et al. (2024). Global cancer statistics 2022: GLOBOCAN estimates of incidence and mortality worldwide for 36 cancers in 185 countries. CA Cancer J. Clin. 74 (3), 229–263. doi:10.3322/caac.21834
Buscail, L., Bournet, B., and Cordelier, P. (2020). Role of oncogenic KRAS in the diagnosis, prognosis and treatment of pancreatic cancer. Nat. Rev. Gastroenterol. Hepatol. 17 (3), 153–168. doi:10.1038/s41575-019-0245-4
Chan, Y. T., Zhang, C., Wu, J., Lu, P., Xu, L., Yuan, H., et al. (2024a). Biomarkers for diagnosis and therapeutic options in hepatocellular carcinoma. Mol. Cancer 23 (1), 189. doi:10.1186/s12943-024-02101-z
Chan, T. S., Cheng, L. H., Hsu, C. C., Yang, P. M., Liao, T. Y., Hsieh, H. Y., et al. (2024b). ASPM stabilizes the NOTCH intracellular domain 1 and promotes oncogenesis by blocking FBXW7 binding in hepatocellular carcinoma cells. Mol. Oncol. 18 (3), 562–579. doi:10.1002/1878-0261.13589
Chang, C. C., Lin, H. H., Lin, J. K., Lin, C. C., Lan, Y. T., Wang, H. S., et al. (2015). FBXW7 mutation analysis and its correlation with clinicopathological features and prognosis in colorectal cancer patients. Int. J. Biol. Markers 30 (1), e88–e95. doi:10.5301/jbm.5000125
Chang, H., Liu, Y. H., Wang, L. L., Wang, J., Zhao, Z. H., Qu, J. F., et al. (2018). MiR-182 promotes cell proliferation by suppressing FBXW7 and FBXW11 in non-small cell lung cancer. Am. J. Transl. Res. 10 (4), 1131–1142.
Chen, L., and Fletcher, S. (2017). Mcl-1 inhibitors: a patent review. Expert Opin. Ther. Pat. 27 (2), 163–178. doi:10.1080/13543776.2017.1249848
Chen, L. J., Hu, B., Han, Z. Q., Liu, W., Zhu, J. H., Chen, X. X., et al. (2021). Repression of FBXW7 by HES5 contributes to inactivation of the TGF-β signaling pathway and alleviation of endometriosis. FASEB J. Off. Publ. Fed. Am. Soc. Exp. Biol. 35 (2), e20938. doi:10.1096/fj.202000438RRR
Chen, S., Leng, P., Guo, J., and Zhou, H. (2023). FBXW7 in breast cancer: mechanism of action and therapeutic potential. J. Exp. Clin. Cancer Res. CR 42 (1), 226. doi:10.1186/s13046-023-02767-1
Chong, X., Madeti, Y., Cai, J., Li, W., Cong, L., Lu, J., et al. (2024). Recent developments in immunotherapy for gastrointestinal tract cancers. J. Hematol. OncolJ Hematol. Oncol. 17 (1), 65. doi:10.1186/s13045-024-01578-x
Chu, C., Geng, Y., Zhou, Y., and Sicinski, P. (2021). Cyclin E in normal physiology and disease states. Trends Cell Biol. 31 (9), 732–746. doi:10.1016/j.tcb.2021.05.001
Churi, C. R., Shroff, R., Wang, Y., Rashid, A., Kang, H. C., Weatherly, J., et al. (2014). Mutation profiling in cholangiocarcinoma: prognostic and therapeutic implications. PloS One 9 (12), e115383. doi:10.1371/journal.pone.0115383
Cizmecioglu, O., Krause, A., Bahtz, R., Ehret, L., Malek, N., and Hoffmann, I. (2012). Plk2 regulates centriole duplication through phosphorylation-mediated degradation of Fbxw7 (human Cdc4). J. Cell Sci. 125 (4), 981–992. doi:10.1242/jcs.095075
Close, V., Close, W., Kugler, S. J., Reichenzeller, M., Yosifov, D. Y., Bloehdorn, J., et al. (2018). NOTCH1 signaling is activated in CLL by mutations of FBXW7 and low expression of USP28 at 11q23. Blood 132, 946. doi:10.1182/blood-2018-99-114491
Close, V., Close, W., Kugler, S. J., Reichenzeller, M., Yosifov, D. Y., Bloehdorn, J., et al. (2019). FBXW7 mutations reduce binding of NOTCH1, leading to cleaved NOTCH1 accumulation and target gene activation in CLL. Blood 133 (8), 830–839. doi:10.1182/blood-2018-09-874529
Collisson, E. A., Bailey, P., Chang, D. K., and Biankin, A. V. (2019). Molecular subtypes of pancreatic cancer. Nat. Rev. Gastroenterol. Hepatol. 16 (4), 207–220. doi:10.1038/s41575-019-0109-y
CXCR4. CXCR4 inhibitor BL-8040 induces AML blast apoptosis by altering miR-15a/16-1 expression and downregulating ERK, BCL-2, MCL-1, and cyclin-D1 | Leukemia. (2024). Available at: https://www.nature.com/articles/leu201782
D, X., M, Y., H, S., P, R., J, J., F, L., et al. (2016). Polo-like kinase-1 regulates myc stabilization and activates a feedforward circuit promoting tumor cell survival. Mol. Cell 64 (3), 493–506. doi:10.1016/j.molcel.2016.09.016
Davis, R. J., Welcker, M., and Clurman, B. E. (2014). Tumor suppression by the Fbw7 ubiquitin ligase: mechanisms and opportunities. Cancer Cell 26 (4), 455–464. doi:10.1016/j.ccell.2014.09.013
Dhanasekaran, R., Deutzmann, A., Mahauad-Fernandez, W. D., Hansen, A. S., Gouw, A. M., and Felsher, D. W. (2021). The MYC oncogene — the grand orchestrator of cancer growth and immune evasion. Nat. Rev. Clin. Oncol. 19 (1), 23–36. doi:10.1038/s41571-021-00549-2
Di Fiore, R., Suleiman, S., Drago-Ferrante, R., Subbannayya, Y., Suleiman, S., Vasileva-Slaveva, M., et al. (2023). The role of FBXW7 in gynecologic malignancies. Cells 12 (10), 1415. doi:10.3390/cells12101415
DiNardo, C. D., Pratz, K. W., Letai, A., Jonas, B. A., Wei, A. H., Thirman, M., et al. (2018). Safety and preliminary efficacy of venetoclax with decitabine or azacitidine in elderly patients with previously untreated acute myeloid leukaemia: a non-randomised, open-label, phase 1b study. Lancet Oncol. 19 (2), 216–228. doi:10.1016/S1470-2045(18)30010-X
Dl, J., Jj, W., K, H., Am, T., R, Z., J, F., et al. (2014). FBXW7 mutations in patients with advanced cancers: clinical and molecular characteristics and outcomes with mTOR inhibitors. PloS One 9 (2), e89388. doi:10.1371/journal.pone.0089388
Ekholm-Reed, S., Spruck, C. H., Sangfelt, O., van Drogen, F., Mueller-Holzner, E., Widschwendter, M., et al. (2004). Mutation of hCDC4 leads to cell cycle deregulation of cyclin E in cancer. Cancer Res. 64 (3), 795–800. doi:10.1158/0008-5472.can-03-3417
Elimova, E., Wadhwa, R., Shiozaki, H., Sudo, K., Estrella, J. S., Badgwell, B. D., et al. (2015). Molecular biomarkers in gastric cancer. J. Natl. Compr. Cancer Netw. JNCCN 13 (4), e19–e29. doi:10.6004/jnccn.2015.0064
El-Mezayen, H., Yamamura, K., Yusa, T., Nakao, Y., Uemura, N., Kitamura, F., et al. (2021). MicroRNA-25 exerts an oncogenic function by regulating the ubiquitin ligase Fbxw7 in hepatocellular carcinoma. Ann. Surg. Oncol. 28 (12), 7973–7982. doi:10.1245/s10434-021-09778-2
Enkhbold, C., Utsunomiya, T., Morine, Y., Imura, S., Ikemoto, T., Arakawa, Y., et al. (2014). Loss of FBXW7 expression is associated with poor prognosis in intrahepatic cholangiocarcinoma. Hepatol. Res. Off. J. Jpn. Soc. Hepatol. 44 (14), E346–E352. doi:10.1111/hepr.12314
Escobar, D., Bushara, O., Sun, L., Liao, J., and Yang, G. Y. (2022). Clinicopathologic characteristics of FBXW7-mutated colorectal adenocarcinoma and association with aberrant beta-catenin localization. Hum. Pathol. 119, 51–58. doi:10.1016/j.humpath.2021.10.003
Eto, K., Iwatsuki, M., Watanabe, M., Ishimoto, T., Ida, S., Imamura, Y., et al. (2015). The sensitivity of gastric cancer to trastuzumab is regulated by the miR-223/FBXW7 pathway. Int. J. Cancer 136 (7), 1537–1545. doi:10.1002/ijc.29168
Fan, J., Bellon, M., Ju, M., Zhao, L., Wei, M., Fu, L., et al. (2022). Clinical significance of FBXW7 loss of function in human cancers. Mol. Cancer 21 (1), 87. doi:10.1186/s12943-022-01548-2
Fang, L., Yang, Z., Zhou, J., Tung, J. Y., Hsiao, C. D., Wang, L., et al. (2015). Circadian clock gene CRY2 degradation is involved in chemoresistance of colorectal cancer. Mol. Cancer Ther. 14 (6), 1476–1487. doi:10.1158/1535-7163.MCT-15-0030
Feng, X., Zou, B., Nan, T., Zheng, X., Zheng, L., Lan, J., et al. (2022). MiR-25 enhances autophagy and promotes sorafenib resistance of hepatocellular carcinoma via targeting FBXW7. Int. J. Med. Sci. 19 (2), 257–266. doi:10.7150/ijms.67352
Feng, Y., Zhao, M., Wang, L., Li, L., Lei, J. H., Zhou, J., et al. (2024). The heterogeneity of signaling pathways and drug responses in intrahepatic cholangiocarcinoma with distinct genetic mutations. Cell Death Dis. 15 (1), 34. doi:10.1038/s41419-023-06406-7
G, K., and T, M. (2014). Perilous journey: a tour of the ubiquitin-proteasome system. Trends Cell Biol. 24 (6), 352–359. doi:10.1016/j.tcb.2013.12.003
G, X., W, L., J, Y., Z, L., P, W., and H, F. (2024). Fbxw7 suppresses carcinogenesis and stemness in triple-negative breast cancer through CHD4 degradation and Wnt/β-catenin pathway inhibition. J. Transl. Med. 22 (1), 99. doi:10.1186/s12967-024-04897-2
Gao, F., Yu, X., Li, M., Zhou, L., Liu, W., Li, W., et al. (2020). Deguelin suppresses non-small cell lung cancer by inhibiting EGFR signaling and promoting GSK3β/FBW7-mediated Mcl-1 destabilization. Cell Death Dis. 11 (2), 143. doi:10.1038/s41419-020-2344-0
Ge, M. K., Zhang, N., Xia, L., Zhang, C., Dong, S. S., Li, Z. M., et al. (2020). FBXO22 degrades nuclear PTEN to promote tumorigenesis. Nat. Commun. 11, 1720. doi:10.1038/s41467-020-15578-1
Gi, M., E, C., Y, L., and Ys, L. (2020). Decreased expression of FBXW7 by ERK1/2 activation in drug-resistant cancer cells confers transcriptional activation of MDR1 by suppression of ubiquitin degradation of HSF1. Cell Death Dis. 11 (5), 395. doi:10.1038/s41419-020-2600-3
Gombodorj, N., Yokobori, T., Tanaka, N., Suzuki, S., Kuriyama, K., Kumakura, Y., et al. (2018). Correlation between high FBXW7 expression in pretreatment biopsy specimens and good response to chemoradiation therapy in patients with locally advanced esophageal cancer: a retrospective study. J. Surg. Oncol. 118 (1), 101–108. doi:10.1002/jso.25127
Gong, J., Cui, Z., Li, L., Ma, Q., Wang, Q., Gao, Y., et al. (2015). MicroRNA-25 promotes gastric cancer proliferation, invasion, and migration by directly targeting F-box and WD-40 Domain Protein 7, FBXW7. Tumour Biol. J. Int. Soc. Oncodevelopmental Biol. Med. 36 (10), 7831–7840. doi:10.1007/s13277-015-3510-3
Gong, J., Huang, Z., and Huo, J. R. (2016). Involvement of F-box proteins in esophageal cancer (Review). Int. J. Oncol. 48 (3), 886–894. doi:10.3892/ijo.2016.3325
Gong, L., Ren, M., Lv, Z., Yang, Y., and Wang, Z. (2018). miR-92b-3p promotes colorectal carcinoma cell proliferation, invasion, and migration by inhibiting FBXW7 in vitro and in vivo. DNA Cell Biol. 37 (5), 501–511. doi:10.1089/dna.2017.4080
Grim, J. E., Gustafson, M. P., Hirata, R. K., Hagar, A. C., Swanger, J., Welcker, M., et al. (2008). Isoform- and cell cycle–dependent substrate degradation by the Fbw7 ubiquitin ligase. J. Cell Biol. 181 (6), 913–920. doi:10.1083/jcb.200802076
Grim, J. E., Knoblaugh, S. E., Guthrie, K. A., Hagar, A., Swanger, J., Hespelt, J., et al. (2012). Fbw7 and p53 cooperatively suppress advanced and chromosomally unstable intestinal cancer. Mol. Cell Biol. 32 (11), 2160–2167. doi:10.1128/MCB.00305-12
Guan, W. L., He, Y., and Xu, R. H. (2023). Gastric cancer treatment: recent progress and future perspectives. J. Hematol. OncolJ Hematol. Oncol. 16 (1), 57. doi:10.1186/s13045-023-01451-3
Guo, Z., Zhou, Y., Evers, B. M., and Wang, Q. (2012). Rictor regulates FBXW7-dependent c-Myc and cyclin E degradation in colorectal cancer cells. Biochem. Biophys. Res. Commun. 418 (2), 426–432. doi:10.1016/j.bbrc.2012.01.054
H, Y., X, L., Z, L., L, C., Y, X., Y, W., et al. (2015). FBXW7 suppresses epithelial-mesenchymal transition, stemness and metastatic potential of cholangiocarcinoma cells. Oncotarget 6 (8), 6310–6325. doi:10.18632/oncotarget.3355
He, D., Ma, Z., Fang, C., Ding, J., Yang, W., Chen, P., et al. (2019). Pseudophosphatase STYX promotes tumor growth and metastasis by inhibiting FBXW7 function in colorectal cancer. Cancer Lett. 454, 53–65. doi:10.1016/j.canlet.2019.04.014
Heestand, G. M., and Kurzrock, R. (2015). Molecular landscape of pancreatic cancer: implications for current clinical trials. Oncotarget 6 (7), 4553–4561. doi:10.18632/oncotarget.2972
Henning, N. J., Boike, L., Spradlin, J. N., Ward, C. C., Liu, G., Zhang, E., et al. (2022). Deubiquitinase-targeting chimeras for targeted protein stabilization. Nat. Chem. Biol. 18 (4), 412–421. doi:10.1038/s41589-022-00971-2
Hershko, A., and Ciechanover, A. (1998). The ubiquitin system. Annu. Rev. Biochem. 67, 425–479. doi:10.1146/annurev.biochem.67.1.425
Hidayat, M., Mitsuishi, Y., Takahashi, F., Tajima, K., Yae, T., Miyahara, K., et al. (2019). Role of FBXW7 in the quiescence of gefitinib-resistant lung cancer stem cells in EGFR-mutant non-small cell lung cancer. Bosn. J. Basic Med. Sci. 19 (4), 355–367. doi:10.17305/bjbms.2019.4227
Honma, S., Hisamori, S., Nishiuchi, A., Itatani, Y., Obama, K., Shimono, Y., et al. (2019). F-Box/WD repeat domain-containing 7 induces chemotherapy resistance in colorectal cancer stem cells. Cancers 11 (5), E635. doi:10.3390/cancers11050635
Hou, J., Huang, P., Lan, C., Geng, S., Xu, M., Liu, Y., et al. (2022). ZC3H15 promotes gastric cancer progression by targeting the FBXW7/c-Myc pathway. Cell Death Discov. 8 (1), 32. doi:10.1038/s41420-022-00815-x
Hu, J. L., Wang, W., Lan, X. L., Zeng, Z. C., Liang, Y. S., Yan, Y. R., et al. (2019). CAFs secreted exosomes promote metastasis and chemotherapy resistance by enhancing cell stemness and epithelial-mesenchymal transition in colorectal cancer. Mol. Cancer 18 (1), 91. doi:10.1186/s12943-019-1019-x
Huang, G., and Long, K. (2023). Sensitization of colon cancer cells to cisplatin by Fbxw7 via negative regulation of the Nox1-mTOR pathway. Pathol. Res. Pract. 247, 154479. doi:10.1016/j.prp.2023.154479
Huang, L. Y., Zhao, J., Chen, H., Wan, L., Inuzuka, H., Guo, J., et al. (2018). SCFFBW7-mediated degradation of Brg1 suppresses gastric cancer metastasis. Nat. Commun. 9, 3569. doi:10.1038/s41467-018-06038-y
Huang, G., Xiang, Z., Wu, H., He, Q., Dou, R., Lin, Z., et al. (2022). The lncRNA BDNF-AS/WDR5/FBXW7 axis mediates ferroptosis in gastric cancer peritoneal metastasis by regulating VDAC3 ubiquitination. Int. J. Biol. Sci. 18 (4), 1415–1433. doi:10.7150/ijbs.69454
Huang, J., Lucero-Prisno, D. E., Zhang, L., Xu, W., Wong, S. H., Ng, S. C., et al. (2023). Updated epidemiology of gastrointestinal cancers in East Asia. Nat. Rev. Gastroenterol. Hepatol. 20 (5), 271–287. doi:10.1038/s41575-022-00726-3
I, B. A., Hwm van, L., E, F., R, O., M, N., and F, L. (2023). Early-onset cancer in the gastrointestinal tract is on the rise-evidence and implications. Cancer Discov. 13 (3), 538–551. doi:10.1158/2159-8290.CD-22-1038
Inuzuka, H., Shaik, S., Onoyama, I., Gao, D., Tseng, A., Maser, R. S., et al. (2011). SCF(FBW7) regulates cellular apoptosis by targeting MCL1 for ubiquitylation and destruction. Nature 471 (7336), 104–109. doi:10.1038/nature09732
Is, H., A, G., W, S., Ap, K., G, S. M. G., and Garg, M. (2022). The multidimensional role of the Wnt/β-catenin signaling pathway in human malignancies. J. Cell Physiol. 237 (1), 199–238. doi:10.1002/jcp.30561
Ishii, N., Araki, K., Yokobori, T., Gantumur, D., Yamanaka, T., Altan, B., et al. (2017). Reduced FBXW7 expression in pancreatic cancer correlates with poor prognosis and chemotherapeutic resistance via accumulation of MCL1. Oncotarget 8 (68), 112636–112646. doi:10.18632/oncotarget.22634
Iwatsuki, M., Mimori, K., Ishii, H., Yokobori, T., Takatsuno, Y., Sato, T., et al. (2010). Loss of FBXW7, a cell cycle regulating gene, in colorectal cancer: clinical significance. Int. J. Cancer 126 (8), 1828–1837. doi:10.1002/ijc.24879
Izumi, D., Ishimoto, T., Miyake, K., Eto, T., Arima, K., Kiyozumi, Y., et al. (2017). Colorectal cancer stem cells acquire chemoresistance through the upregulation of F-box/WD repeat-containing protein 7 and the consequent degradation of c-myc. Stem Cells Dayt Ohio 35 (9), 2027–2036. doi:10.1002/stem.2668
J, T., S, T., Z, N. C., J, Y., F, Z., and L, Z. (2017). FBW7-Dependent mcl-1 degradation mediates the anticancer effect of Hsp90 inhibitors. Mol. Cancer Ther. 16 (9), 1979–1988. doi:10.1158/1535-7163.MCT-17-0032
Ji, S., Qin, Y., Shi, S., Liu, X., Hu, H., Zhou, H., et al. (2015). ERK kinase phosphorylates and destabilizes the tumor suppressor FBW7 in pancreatic cancer. Cell Res. 25 (5), 561–573. doi:10.1038/cr.2015.30
Jiang, J. X., Sun, C. Y., Tian, S., Yu, C., Chen, M. Y., and Zhang, H. (2016). Tumor suppressor Fbxw7 antagonizes WNT signaling by targeting β-catenin for degradation in pancreatic cancer. Tumour Biol. J. Int. Soc. Oncodevelopmental Biol. Med. 37 (10), 13893–13902. doi:10.1007/s13277-016-5217-5
Jiang, Y., Qi, X., Liu, X., Zhang, J., Ji, J., Zhu, Z., et al. (2017). Fbxw7 haploinsufficiency loses its protection against DNA damage and accelerates MNU-induced gastric carcinogenesis. Oncotarget 8 (20), 33444–33456. doi:10.18632/oncotarget.16800
Jiménez-Izquierdo, R., Morrugares, R., Suanes-Cobos, L., Correa-Sáez, A., Garrido-Rodríguez, M., Cerero-Tejero, L., et al. (2023). FBXW7 tumor suppressor regulation by dualspecificity tyrosine-regulated kinase 2. Cell Death Dis. 14 (3), 202. doi:10.1038/s41419-023-05724-0
Jin, X., Yang, C., Fan, P., Xiao, J., Zhang, W., Zhan, S., et al. (2017). CDK5/FBW7-dependent ubiquitination and degradation of EZH2 inhibits pancreatic cancer cell migration and invasion. J. Biol. Chem. 292 (15), 6269–6280. doi:10.1074/jbc.M116.764407
Joshi, S. S., and Badgwell, B. D. (2021). Current treatment and recent progress in gastric cancer. CA Cancer J. Clin. 71 (3), 264–279. doi:10.3322/caac.21657
Kam, A. E., Masood, A., and Shroff, R. T. (2021). Current and emerging therapies for advanced biliary tract cancers. Lancet Gastroenterol. Hepatol. 6 (11), 956–969. doi:10.1016/S2468-1253(21)00171-0
Kang, H. S., Park, H. Y., Lim, H., Son, I. T., Kim, M. J., Kim, N. Y., et al. (2023). Different miRNAs related to FBXW7 mutations or high mitotic indices contribute to rectal neuroendocrine tumors: a pilot study. Int. J. Mol. Sci. 24 (7), 6329. doi:10.3390/ijms24076329
Kar, R., Jha, S. K., Ojha, S., Sharma, A., Dholpuria, S., Raju, V. S. R., et al. (2021). The FBXW7-NOTCH interactome: a ubiquitin proteasomal system-induced crosstalk modulating oncogenic transformation in human tissues. Cancer Rep. 4 (4), e1369. doi:10.1002/cnr2.1369
Kawaguchi, Y., Newhook, T. E., Tran Cao, H. S., Tzeng, C. W. D., Chun, Y. S., Aloia, T. A., et al. (2021). Alteration of FBXW7 is associated with worse survival in patients undergoing resection of colorectal liver metastases. J. Gastrointest. Surg. Off. J. Soc. Surg. Aliment. Tract. 25 (1), 186–194. doi:10.1007/s11605-020-04866-2
Kawashita, Y., Morine, Y., Ikemoto, T., Saito, Y., Iwahashi, S., Yamada, S., et al. (2017). Loss of Fbxw7 expression is a predictor of recurrence in colorectal liver metastasis. J. Hepato-Biliary-Pancreat Sci. 24 (10), 576–583. doi:10.1002/jhbp.500
Khan, O. M., Carvalho, J., Spencer-Dene, B., Mitter, R., Frith, D., Snijders, A. P., et al. (2018). The deubiquitinase USP9X regulates FBW7 stability and suppresses colorectal cancer. J. Clin. Invest 128 (4), 1326–1337. doi:10.1172/JCI97325
Khan, O. M., Almagro, J., Nelson, J. K., Horswell, S., Encheva, V., Keyan, K. S., et al. (2021a). Proteasomal degradation of the tumour suppressor FBW7 requires branched ubiquitylation by TRIP12. Nat. Commun. 12 (1), 2043. doi:10.1038/s41467-021-22319-5
Khan, A. Q., Al-Tamimi, M., Uddin, S., and Steinhoff, M. (2021b). F-box proteins in cancer stemness: an emerging prognostic and therapeutic target. Drug Discov. Today 26 (12), 2905–2914. doi:10.1016/j.drudis.2021.07.006
Kitade, S., Onoyama, I., Kobayashi, H., Yagi, H., Yoshida, S., Kato, M., et al. (2016). FBXW7 is involved in the acquisition of the malignant phenotype in epithelial ovarian tumors. Cancer Sci. 107 (10), 1399–1405. doi:10.1111/cas.13026
Ko, Y. U., Kim, C., Lee, J., Kim, D., Kim, Y., Yun, N., et al. (2019). Site-specific phosphorylation of Fbxw7 by Cdk5/p25 and its resulting decreased stability are linked to glutamate-induced excitotoxicity. Cell Death Dis. 10 (8), 579. doi:10.1038/s41419-019-1818-4
Koga, Y., Iwatsuki, M., Yamashita, K., Kiyozumi, Y., Kurashige, J., Masuda, T., et al. (2019). The role of FBXW7, a cell-cycle regulator, as a predictive marker of recurrence of gastrointestinal stromal tumors. Gastric Cancer Off. J. Int. Gastric Cancer Assoc. Jpn. Gastric Cancer Assoc. 22 (6), 1100–1108. doi:10.1007/s10120-019-00950-y
Korphaisarn, K., Morris, V. K., Overman, M. J., Fogelman, D. R., Kee, B. K., Raghav, K. P. S., et al. (2017). FBXW7 missense mutation: a novel negative prognostic factor in metastatic colorectal adenocarcinoma. Oncotarget 8 (24), 39268–39279. doi:10.18632/oncotarget.16848
Kothari, N., Teer, J. K., Abbott, A. M., Srikumar, T., Zhang, Y., Yoder, S. J., et al. (2016). Increased incidence of FBXW7 and POLE proofreading domain mutations in young adult colorectal cancers. Cancer 122 (18), 2828–2835. doi:10.1002/cncr.30082
Kurashige, J., Watanabe, M., Iwatsuki, M., Kinoshita, K., Saito, S., Hiyoshi, Y., et al. (2012). Overexpression of microRNA-223 regulates the ubiquitin ligase FBXW7 in oesophageal squamous cell carcinoma. Br. J. Cancer 106 (1), 182–188. doi:10.1038/bjc.2011.509
L, V., O, T., P, V., F, D., G, D., E, C., et al. (2009). Strong correlation between VEGF and MCL-1 mRNA expression levels in B-cell chronic lymphocytic leukemia. Leuk. Res. 33 (12), 1623–1626. doi:10.1016/j.leukres.2009.05.003
L, S., A, C., L, L., L, Y., Y, H., J, X., et al. (2022). NRG1 regulates Fra-1 transcription and metastasis of triple-negative breast cancer cells via the c-Myc ubiquitination as manipulated by ERK1/2-mediated Fbxw7 phosphorylation. Oncogene 41 (6), 907–919. doi:10.1038/s41388-021-02142-4
Lan, H., Tan, M., Zhang, Q., Yang, F., Wang, S., Li, H., et al. (2019). LSD1 destabilizes FBXW7 and abrogates FBXW7 functions independent of its demethylase activity. Proc. Natl. Acad. Sci. U. S. A. 116 (25), 12311–12320. doi:10.1073/pnas.1902012116
Lee, C. J., An, H. J., Kim, S. M., Yoo, S. M., Park, J., Lee, G. E., et al. (2020). FBXW7-mediated stability regulation of signal transducer and activator of transcription 2 in melanoma formation. Proc. Natl. Acad. Sci. U. S. A. 117 (1), 584–594. doi:10.1073/pnas.1909879116
Li, J., Guo, Y., Liang, X., Sun, M., Wang, G., De, W., et al. (2012). MicroRNA-223 functions as an oncogene in human gastric cancer by targeting FBXW7/hCdc4. J. Cancer Res. Clin. Oncol. 138 (5), 763–774. doi:10.1007/s00432-012-1154-x
Li, L., Sarver, A. L., Khatri, R., Hajeri, P. B., Kamenev, I., French, A. J., et al. (2014). Sequential expression of miR-182 and miR-503 cooperatively targets FBXW7, contributing to the malignant transformation of colon adenoma to adenocarcinoma. J. Pathol. 234 (4), 488–501. doi:10.1002/path.4407
Li, H., Wang, Z., Zhang, W., Qian, K., Xu, W., and Zhang, S. (2016). Fbxw7 regulates tumor apoptosis, growth arrest and the epithelial-to-mesenchymal transition in part through the RhoA signaling pathway in gastric cancer. Cancer Lett. 370 (1), 39–55. doi:10.1016/j.canlet.2015.10.006
Li, M. R., Zhu, C. C., Ling, T. L., Zhang, Y. Q., Xu, J., Zhao, E. H., et al. (2017a). FBXW7 expression is associated with prognosis and chemotherapeutic outcome in Chinese patients with gastric adenocarcinoma. BMC Gastroenterol. 17 (1), 60. doi:10.1186/s12876-017-0616-7
Li, M., Ouyang, L., Zheng, Z., Xiang, D., Ti, A., Li, L., et al. (2017b). E3 ubiquitin ligase FBW7α inhibits cholangiocarcinoma cell proliferation by downregulating c-Myc and cyclin E. Oncol. Rep. 37 (3), 1627–1636. doi:10.3892/or.2017.5432
Li, Q., Li, Y., Li, J., Ma, Y., Dai, W., Mo, S., et al. (2018). FBW7 suppresses metastasis of colorectal cancer by inhibiting HIF1α/CEACAM5 functional axis. Int. J. Biol. Sci. 14 (7), 726–735. doi:10.7150/ijbs.24505
Li, N., Babaei-Jadidi, R., Lorenzi, F., Spencer-Dene, B., Clarke, P., Domingo, E., et al. (2019). An FBXW7-ZEB2 axis links EMT and tumour microenvironment to promote colorectal cancer stem cells and chemoresistance. Oncogenesis 8 (3), 13. doi:10.1038/s41389-019-0125-3
Li, C., Deng, C., Pan, G., Wang, X., Zhang, K., Dong, Z., et al. (2020). Lycorine hydrochloride inhibits cell proliferation and induces apoptosis through promoting FBXW7-MCL1 axis in gastric cancer. J. Exp. Clin. Cancer Res. CR 39, 230. doi:10.1186/s13046-020-01743-3
Li, J., Xu, Q., Huang, Z. jian, Mao, N., Lin, Z. tao, Cheng, L., et al. (2021). CircRNAs: a new target for the diagnosis and treatment of digestive system neoplasms. Cell Death Dis. 12 (2), 205–213. doi:10.1038/s41419-021-03495-0
Li, X., Pu, W., Zheng, Q., Ai, M., Chen, S., and Peng, Y. (2022). Proteolysis-targeting chimeras (PROTACs) in cancer therapy. Mol. Cancer 21 (1), 99. doi:10.1186/s12943-021-01434-3
Li, X. P., Qu, J., Teng, X. Q., Zhuang, H. H., Dai, Y. H., Yang, Z., et al. (2023a). The emerging role of super-enhancers as therapeutic targets in the digestive system tumors. Int. J. Biol. Sci. 19 (4), 1036–1048. doi:10.7150/ijbs.78535
Li, Z., Pan, Y., Yao, J., Gao, Y., Qian, Y., Zheng, M., et al. (2023b). ANXA2 as a novel substrate of FBXW7 promoting esophageal squamous cell carcinoma via ERK phosphorylation. Biochem. Biophys. Res. Commun. 649, 93–100. doi:10.1016/j.bbrc.2023.01.082
Lin, M., Xu, Y., Gao, Y., Pan, C., Zhu, X., and Wang, Z. W. (2019). Regulation of F-box proteins by noncoding RNAs in human cancers. Cancer Lett. 466, 61–70. doi:10.1016/j.canlet.2019.09.008
Lin, H., Ma, N., Zhao, L., Yang, G., and Cao, B. (2020a). KDM5c promotes colon cancer cell proliferation through the FBXW7-c-jun regulatory Axis. Front. Oncol. 10, 535449. doi:10.3389/fonc.2020.535449
Lin, L., Ding, D., Xiao, X., Li, B., Cao, P., and Li, S. (2020b). Trametinib potentiates TRAIL-induced apoptosis via FBW7-dependent Mcl-1 degradation in colorectal cancer cells. J. Cell Mol. Med. 24 (12), 6822–6832. doi:10.1111/jcmm.15336
Lin, J., Wang, X., Zhai, S., Shi, M., Peng, C., Deng, X., et al. (2022). Hypoxia-induced exosomal circPDK1 promotes pancreatic cancer glycolysis via c-myc activation by modulating miR-628-3p/BPTF axis and degrading BIN1. J. Hematol. OncolJ Hematol. Oncol. 15 (1), 128. doi:10.1186/s13045-022-01348-7
Ling, H., Pickard, K., Ivan, C., Isella, C., Ikuo, M., Mitter, R., et al. (2016). The clinical and biological significance of mir-224 expression in colorectal cancer metastasis. Gut 65 (6), 977–989. doi:10.1136/gutjnl-2015-309372
Linnekamp, J. F., Hooff, S. R. van, Prasetyanti, P. R., Kandimalla, R., Buikhuisen, J. Y., Fessler, E., et al. (2018). Consensus molecular subtypes of colorectal cancer are recapitulated in in vitro and in vivo models. Cell Death Differ. 25 (3), 616–633. doi:10.1038/s41418-017-0011-5
Liu, Y., Huang, Y., Wang, Z., Huang, Y., Li, X., Louie, A., et al. (2013). Temporal mTOR inhibition protects Fbxw7-deficient mice from radiation-induced tumor development. Aging 5 (2), 111–119. doi:10.18632/aging.100535
Liu, X., Zhao, B., Sun, L., Bhuripanyo, K., Wang, Y., Bi, Y., et al. (2017). Orthogonal ubiquitin transfer identifies ubiquitination substrates under differential control by the two ubiquitin activating enzymes. Nat. Commun. 8, 14286. doi:10.1038/ncomms14286
Liu, R., Gao, J., Yang, Y., Qiu, R., Zheng, Y., Huang, W., et al. (2018a). PHD finger protein 1 (PHF1) is a novel reader for histone H4R3 symmetric dimethylation and coordinates with PRMT5–WDR77/CRL4B complex to promote tumorigenesis. Nucleic Acids Res. 46 (13), 6608–6626. doi:10.1093/nar/gky461
Liu, H., Wang, K., Fu, H., and Song, J. (2018b). Low expression of the ubiquitin ligase FBXW7 correlates with poor prognosis of patients with colorectal cancer. Int. J. Clin. Exp. Pathol. 11 (1), 413–419.
Liu, F., Xia, Z., Zhang, M., Ding, J., Feng, Y., Wu, J., et al. (2019). SMARCAD1 promotes pancreatic cancer cell growth and metastasis through wnt/β-catenin-mediated EMT. Int. J. Biol. Sci. 15 (3), 636–646. doi:10.7150/ijbs.29562
Liu, Y., Zhao, L., Xue, L., and Hou, Y. (2020). Selected updates in molecular and genomic pathology of esophageal cancer. Ann. N. Y. Acad. Sci. 1482 (1), 225–235. doi:10.1111/nyas.14527
Liu, Q., Aminu, B., Roscow, O., and Zhang, W. (2021a). Targeting the ubiquitin signaling cascade in tumor microenvironment for cancer therapy. Int. J. Mol. Sci. 22 (2), 791. doi:10.3390/ijms22020791
Liu, Z., Ma, T., Duan, J., Liu, X., and Liu, L. (2021b). MicroRNA-223-induced inhibition of the FBXW7 gene affects the proliferation and apoptosis of colorectal cancer cells via the Notch and Akt/mTOR pathways. Mol. Med. Rep. 23 (2), 154. doi:10.3892/mmr.2020.11793
Liu, J., Xiao, Q., Xiao, J., Niu, C., Li, Y., Zhang, X., et al. (2022). Wnt/β-catenin signalling: function, biological mechanisms, and therapeutic opportunities. Signal Transduct. Target Ther. 7, 3. doi:10.1038/s41392-021-00762-6
Llovet, J. M., Montal, R., Sia, D., and Finn, R. S. (2018). Molecular therapies and precision medicine for hepatocellular carcinoma. Nat. Rev. Clin. Oncol. 15 (10), 599–616. doi:10.1038/s41571-018-0073-4
Lu, H., Yao, B., Wen, X., and Jia, B. (2019). FBXW7 circular RNA regulates proliferation, migration and invasion of colorectal carcinoma through NEK2, mTOR, and PTEN signaling pathways in vitro and in vivo. BMC Cancer 19 (1), 918. doi:10.1186/s12885-019-6028-z
Lupini, L., Bassi, C., Mlcochova, J., Musa, G., Russo, M., Vychytilova-Faltejskova, P., et al. (2015). Prediction of response to anti-EGFR antibody-based therapies by multigene sequencing in colorectal cancer patients. BMC Cancer 15, 808. doi:10.1186/s12885-015-1752-5
M, Y., S, H., T, K., M, N., R, T., H, I., et al. (2004a). Phosphorylation-dependent degradation of c-Myc is mediated by the F-box protein Fbw7. EMBO J. 23 (10), 2116–2125. doi:10.1038/sj.emboj.7600217
M, W., A, O., J, J., Je, G., Jw, H., Rn, E., et al. (2004b). The Fbw7 tumor suppressor regulates glycogen synthase kinase 3 phosphorylation-dependent c-Myc protein degradation. Proc. Natl. Acad. Sci. U. S. A. 101 (24), 9085–9090. doi:10.1073/pnas.0402770101
M, L., J, L., S, A., A, J., Hf, N., F, A. M., et al. (2011). MiRNA-27a controls FBW7/hCDC4-dependent cyclin E degradation and cell cycle progression. Cell Cycle Georget Tex 10 (13), 2172–2183. doi:10.4161/cc.10.13.16248
M, G., Y, L., and Dw, F. (2014). MYC activation is a hallmark of cancer initiation and maintenance. Cold Spring Harb. Perspect. Med. 4 (6), a014241. doi:10.1101/cshperspect.a014241
Ma, J., Cheng, L., Liu, H., Zhang, J., Shi, Y., Zeng, F., et al. (2013). Genistein down-regulates miR-223 expression in pancreatic cancer cells. Curr. Drug Targets 14 (10), 1150–1156. doi:10.2174/13894501113149990187
Mammalian cell cycle cyclins (2024). Available at: http://pubmed6.0089.lunwenlib.com/32334991/
Mao, J. H., Perez-Losada, J., Wu, D., Delrosario, R., Tsunematsu, R., Nakayama, K. I., et al. (2004). Fbxw7/Cdc4 is a p53-dependent, haploinsufficient tumour suppressor gene. Nature 432 (7018), 775–779. doi:10.1038/nature03155
Mao, J. H., Kim, I. J., Wu, D., Climent, J., Kang, H. C., DelRosario, R., et al. (2008). FBXW7 targets mTOR for degradation and cooperates with PTEN in tumor suppression. Science 321 (5895), 1499–1502. doi:10.1126/science.1162981
Marques-Ramos, A., and Cervantes, R. (2023). Expression of mTOR in normal and pathological conditions. Mol. Cancer 22 (1), 112. doi:10.1186/s12943-023-01820-z
Martelli, V., Pastorino, A., and Sobrero, A. F. (2022). Prognostic and predictive molecular biomarkers in advanced colorectal cancer. Pharmacol. Ther. 236, 108239. doi:10.1016/j.pharmthera.2022.108239
Meyer, A. E., Furumo, Q., Stelloh, C., Minella, A. C., and Rao, S. (2020). Loss of Fbxw7 triggers mammary tumorigenesis associated with E2F/c-Myc activation and Trp53 mutation. Neoplasia N. Y. N. 22 (11), 644–658. doi:10.1016/j.neo.2020.07.001
Min, S. H., Lau, A. W., Lee, T. H., Inuzuka, H., Wei, S., Huang, P., et al. (2012). Negative regulation of the stability and tumor suppressor function of Fbw7 by the Pin1 prolyl isomerase. Mol. Cell 46 (6), 771–783. doi:10.1016/j.molcel.2012.04.012
Minella, A. C., Grim, J. E., Welcker, M., and Clurman, B. E. (2007). p53 and SCFFbw7 cooperatively restrain cyclin E-associated genome instability. Oncogene 26 (48), 6948–6953. doi:10.1038/sj.onc.1210518
Mittal, P., Singh, S., Sinha, R., Shrivastava, A., Singh, A., and Singh, I. K. (2021). Myeloid cell leukemia 1 (MCL-1): structural characteristics and application in cancer therapy. Int. J. Biol. Macromol. 187, 999–1018. doi:10.1016/j.ijbiomac.2021.07.166
Mojsa, B., Lassot, I., and Desagher, S. (2014). Mcl-1 ubiquitination: unique regulation of an essential survival protein. Cells 3 (2), 418–437. doi:10.3390/cells3020418
Morel, C., Carlson, S. M., White, F. M., and Davis, R. J. (2009). Mcl-1 integrates the opposing actions of signaling pathways that mediate survival and apoptosis. Mol. Cell Biol. 29 (14), 3845–3852. doi:10.1128/MCB.00279-09
Mori, A., Masuda, K., Ohtsuka, H., Shijo, M., Ariake, K., Fukase, K., et al. (2018). FBXW7 modulates malignant potential and cisplatin-induced apoptosis in cholangiocarcinoma through NOTCH1 and MCL1. Cancer Sci. 109 (12), 3883–3895. doi:10.1111/cas.13829
Mu, Y., Zou, H., Chen, B., Fan, Y., and Luo, S. (2017). FAM83D knockdown regulates proliferation, migration and invasion of colorectal cancer through inhibiting FBXW7/Notch-1 signalling pathway. Biomed. Pharmacother. Biomedecine Pharmacother. 90, 548–554. doi:10.1016/j.biopha.2017.03.073
Mx, Z., H, W., and Gp, S. (2020). Tumor-suppressor Fbxw7 targets SIK2 for degradation to interfere with TORC2-AKT signaling in pancreatic cancer. Cell Biol. Int. 44 (9), 1900–1910. doi:10.1002/cbin.11396
N, K., A, S., and I, A. (2015). Emerging roles for the FBXW7 ubiquitin ligase in leukemia and beyond. Curr. Opin. Cell Biol. 37, 28–34. doi:10.1016/j.ceb.2015.09.003
Nault, J. C., and Villanueva, A. (2021). Biomarkers for hepatobiliary cancers. Hepatol. Balt. Md 73 (Suppl. 1), 115–127. doi:10.1002/hep.31175
Nemecek, R., Berkovcova, J., Radova, L., Kazda, T., Mlcochova, J., Vychytilova-Faltejskova, P., et al. (2016). Mutational analysis of primary and metastatic colorectal cancer samples underlying the resistance to cetuximab-based therapy. OncoTargets Ther. 9, 4695–4703. doi:10.2147/OTT.S102891
Nie, J., Lu, L., Du, C., and Gao, X. (2022). FAM83D promotes the proliferation and migration of hepatocellular carcinoma cells by inhibiting the FBXW7/MCL1 pathway. Transl. Cancer Res. 11 (10), 3790–3802. doi:10.21037/tcr-22-2069
Notch signaling pathway in cancer: from mechanistic insights to targeted therapies. 2024. Available at: https://www.ncbi.nlm.nih.gov/pmc/articles/PMC11128457/
Notch1 in Cancer Therapy (2024). Possible clinical implications and challenges. Available at: https://pubmed.ncbi.nlm.nih.gov/32913140/.
Okazaki, H., Matsunaga, N., Fujioka, T., Okazaki, F., Akagawa, Y., Tsurudome, Y., et al. (2014). Circadian regulation of mTOR by the ubiquitin pathway in renal cell carcinoma. Cancer Res. 74 (2), 543–551. doi:10.1158/0008-5472.CAN-12-3241
Ou, B., Zhao, J., Guan, S., Wangpu, X., Zhu, C., Zong, Y., et al. (2016). Plk2 promotes tumor growth and inhibits apoptosis by targeting Fbxw7/Cyclin E in colorectal cancer. Cancer Lett. 380 (2), 457–466. doi:10.1016/j.canlet.2016.07.004
Pai, S. G., Carneiro, B. A., Mota, J. M., Costa, R., Leite, C. A., Barroso-Sousa, R., et al. (2017). Wnt/beta-catenin pathway: modulating anticancer immune response. J. Hematol. OncolJ Hematol. Oncol. 10, 101. doi:10.1186/s13045-017-0471-6
Pan, Y., Liu, J., Gao, Y., Guo, Y., Wang, C., Liang, Z., et al. (2023). FBXW7 loss of function promotes esophageal squamous cell carcinoma progression via elevating MAP4 and ERK phosphorylation. J. Exp. Clin. Cancer Res. CR 42 (1), 75. doi:10.1186/s13046-023-02630-3
Pancreatic CancerPancreatic cancer: changing epidemiology and new approaches to risk assessment, early detection, and prevention - PubMed Available at: https://pubmed.ncbi.nlm.nih.gov/36804602/. doi:10.1053/j.gastro.2023.02.012
Park, J., Cho, J., and Song, E. J. (2020). Ubiquitin-proteasome system (UPS) as a target for anticancer treatment. Arch. Pharm. Res. 43 (11), 1144–1161. doi:10.1007/s12272-020-01281-8
Pavicevic, S., Reichelt, S., Uluk, D., Lurje, I., Engelmann, C., Modest, D. P., et al. (2022). Prognostic and predictive molecular markers in cholangiocarcinoma. Cancers 14 (4), 1026. doi:10.3390/cancers14041026
Perez-Losada, J., Mao, J. H., and Balmain, A. (2005). Control of genomic instability and epithelial tumor development by the p53-Fbxw7/Cdc4 pathway. Cancer Res. 65 (15), 6488–6492. doi:10.1158/0008-5472.CAN-05-1294
Pervin, S., Tran, A., Tran, L., Urman, R., Braga, M., Chaudhuri, G., et al. (2011). Reduced association of anti-apoptotic protein Mcl-1 with E3 ligase Mule increases the stability of Mcl-1 in breast cancer cells. Br. J. Cancer 105 (3), 428–437. doi:10.1038/bjc.2011.242
Pickart, C. M. (2001). Mechanisms underlying ubiquitination. Annu. Rev. Biochem. 70, 503–533. doi:10.1146/annurev.biochem.70.1.503
Q, H., Y, Q., B, Z., C, L., S, J., S, S., et al. (2017). FBW7 increases the chemosensitivity of pancreatic cancer cells to gemcitabine through upregulation of ENT1. Oncol. Rep. 38 (4), 2069–2077. doi:10.3892/or.2017.5856
Q, Z., L, H., Y, G., Z, X., Q, X., and X, T. (2020). FBW7 in hematological tumors. Oncol. Lett. 19 (3), 1657–1664. doi:10.3892/ol.2020.11264
Qi, Y., Rezaeian, A. H., Wang, J., Huang, D., Chen, H., Inuzuka, H., et al. (2024). Molecular insights and clinical implications for the tumor suppressor role of SCFFBXW7 E3 ubiquitin ligase. Biochim. Biophys. Acta Rev. Cancer 1879 (5), 189140. doi:10.1016/j.bbcan.2024.189140
Qin, Y., Hu, Q., Xu, J., Ji, S., Dai, W., Liu, W., et al. (2019). PRMT5 enhances tumorigenicity and glycolysis in pancreatic cancer via the FBW7/cMyc axis. Cell Commun. Signal CCS 17 (1), 30. doi:10.1186/s12964-019-0344-4
R, S., S, M., J, X., X, R., P, G., H, L., et al. (2023). A novel polypeptide encoded by the circular RNA ZKSCAN1 suppresses HCC via degradation of mTOR. Mol. Cancer 22 (1), 16. doi:10.1186/s12943-023-01719-9
Rodrigues, P. M., Vogel, A., Arrese, M., Balderramo, D. C., Valle, J. W., and Banales, J. M. (2021). Next-generation biomarkers for cholangiocarcinoma. Cancers 13 (13), 3222. doi:10.3390/cancers13133222
Roling, L., Flammersfeld, A., Pradel, G., and Bennink, S. (2022). The WD40-protein PfWLP1 ensures stability of the PfCCp-based adhesion protein complex in plasmodium falciparum gametocytes. Front. Cell Infect. Microbiol. 12, 942364. doi:10.3389/fcimb.2022.942364
Ruan, F., Ruan, Y., Gu, H., Sun, J., and Chen, Q. (2024). Clitocine enhances the drug sensitivity of colon cancer cells by promoting FBXW7-mediated MCL-1 degradation via inhibiting the A2B/cAMP/ERK axis. Am. J. Physiol-Cell Physiol. 327, C884–C900. doi:10.1152/ajpcell.00310.2024
S, I., Lo, T., T, U., Y, M., T, I., Y, A., et al. (2014). Role of Fbxw7 expression in hepatocellular carcinoma and adjacent non-tumor liver tissue. J. Gastroenterol. Hepatol. 29 (10), 1822–1829. doi:10.1111/jgh.12623
Sailo, B. L., Banik, K., Girisa, S., Bordoloi, D., Fan, L., Halim, C. E., et al. (2019). FBXW7 in cancer: what has been unraveled thus far? Cancers 11 (2), 246. doi:10.3390/cancers11020246
Sancho, R., Blake, S. M., Tendeng, C., Clurman, B. E., Lewis, J., and Behrens, A. (2013). Fbw7 repression by hes5 creates a feedback loop that modulates Notch-mediated intestinal and neural stem cell fate decisions. PLoS Biol. 11 (6), e1001586. doi:10.1371/journal.pbio.1001586
Sancho, R., Gruber, R., Gu, G., and Behrens, A. (2014). Loss of Fbw7 reprograms adult pancreatic ductal cells into α, δ, and β cells. Cell Stem Cell 15 (2), 139–153. doi:10.1016/j.stem.2014.06.019
Schapira, M., Tyers, M., Torrent, M., and Arrowsmith, C. H. (2017). WD-repeat domain proteins: a novel target class? Nat. Rev. Drug Discov. 16 (11), 773–786. doi:10.1038/nrd.2017.179
Schell, M. J., Yang, M., Teer, J. K., Lo, F. Y., Madan, A., Coppola, D., et al. (2016). A multigene mutation classification of 468 colorectal cancers reveals a prognostic role for APC. Nat. Commun. 7, 11743. doi:10.1038/ncomms11743
Schlick, K., Kiem, D., and Greil, R. (2021). Recent advances in pancreatic cancer: novel prognostic biomarkers and targeted therapy—a review of the literature. Biomolecules 11 (10), 1469. doi:10.3390/biom11101469
Schülein, C., Eilers, M., and Popov, N. (2011). PI3K-dependent phosphorylation of Fbw7 modulates substrate degradation and activity. FEBS Lett. 585 (14), 2151–2157. doi:10.1016/j.febslet.2011.05.036
Senichkin, V. V., Streletskaia, A. Y., Gorbunova, A. S., Zhivotovsky, B., and Kopeina, G. S. (2020). Saga of Mcl-1: regulation from transcription to degradation. Cell Death Differ. 27 (2), 405–419. doi:10.1038/s41418-019-0486-3
Sentani, K., Oue, N., Kondo, H., Kuraoka, K., Motoshita, J., Ito, R., et al. (2001). Increased expression but not genetic alteration of BRG1, a component of the SWI/SNF complex, is associated with the advanced stage of human gastric carcinomas. Pathobiol. J. Immunopathol. Mol. Cell Biol. 69 (6), 315–320. doi:10.1159/000064638
Sepulveda, A. R., Hamilton, S. R., Allegra, C. J., Grody, W., Cushman-Vokoun, A. M., Funkhouser, W. K., et al. (2017). Molecular biomarkers for the evaluation of colorectal cancer: guideline from the American society for clinical pathology, college of American pathologists, association for molecular pathology, and the American society of clinical oncology. J. Clin. Oncol. Off. J. Am. Soc. Clin. Oncol. 35 (13), 1453–1486. doi:10.1200/JCO.2016.71.9807
Shang, W., Yan, C., Liu, R., Chen, L., Cheng, D., Hao, L., et al. (2021). Clinical significance of FBXW7 tumor suppressor gene mutations and expression in human colorectal cancer: a systemic review and meta-analysis. BMC Cancer 21 (1), 770. doi:10.1186/s12885-021-08535-8
Shenoy, A. R., Kirschnek, S., and Häcker, G. (2014). IL-15 regulates Bcl-2 family members Bim and Mcl-1 through JAK/STAT and PI3K/AKT pathways in T cells. Eur. J. Immunol. 44 (8), 2500–2507. doi:10.1002/eji.201344238
Shima, T., Taniguchi, K., Inomata, Y., Arima, J., and Lee, S. W. (2024). Glycolysis in gastrointestinal stromal tumor: a brief overview. Neoplasia N. Y. 55, 101022. doi:10.1016/j.neo.2024.101022
Siegel, R. L., Giaquinto, A. N., and Jemal, A. (2024). Cancer statistics, 2024. CA Cancer J. Clin. 74 (1), 12–49. doi:10.3322/caac.21820
Skaar, J. R., Pagan, J. K., and Pagano, M. (2013). Mechanisms and function of substrate recruitment by F-box proteins. Nat. Rev. Mol. Cell Biol. 14 (6), 369–381. doi:10.1038/nrm3582
Song, X., Shen, L., Tong, J., Kuang, C., Zeng, S., Schoen, R. E., et al. (2020). Mcl-1 inhibition overcomes intrinsic and acquired regorafenib resistance in colorectal cancer. Theranostics 10 (18), 8098–8110. doi:10.7150/thno.45363
Song, P., Gao, Z., Bao, Y., Chen, L., Huang, Y., Liu, Y., et al. (2024). Wnt/β-catenin signaling pathway in carcinogenesis and cancer therapy. J. Hematol. OncolJ Hematol. Oncol. 17 (1), 46. doi:10.1186/s13045-024-01563-4
Sun, X. F., Sun, J. P., Hou, H. T., Li, K., Liu, X., and Ge, Q. X. (2016). MicroRNA-27b exerts an oncogenic function by targeting Fbxw7 in human hepatocellular carcinoma. Tumour Biol. J. Int. Soc. Oncodevelopmental Biol. Med. 37 (11), 15325–15332. doi:10.1007/s13277-016-5444-9
Sun, Y., Nie, W., Qiu, B., Yang, Q., and Zhao, H. (2023). FBXW7 affects autophagy through MCL1 in oral squamous cell carcinoma. Oral Dis. 29, 3259–3267. doi:10.1111/odi.14325
Sun, D., Mülder, D. T., Li, Y., Nieboer, D., Park, J. Y., Suh, M., et al. (2024). The effect of nationwide organized cancer screening programs on gastric cancer mortality: a synthetic control study. Gastroenterology 166 (3), 503–514. doi:10.1053/j.gastro.2023.11.286
T, M., C, G., and Cyclin, E. (2004). Int. J. Biochem. Cell Biol. 36 (8). doi:10.1016/j.biocel.2003.12.005
Taieb, J., Jung, A., Sartore-Bianchi, A., Peeters, M., Seligmann, J., Zaanan, A., et al. (2019). The evolving biomarker landscape for treatment selection in metastatic colorectal cancer. Drugs 79 (13), 1375–1394. doi:10.1007/s40265-019-01165-2
Takada, M., Zhang, W., Suzuki, A., Kuroda, T. S., Yu, Z., Inuzuka, H., et al. (2017). FBW7 loss promotes chromosomal instability and tumorigenesis via cyclin E1/CDK2-mediated phosphorylation of CENP-A. Cancer Res. 77 (18), 4881–4893. doi:10.1158/0008-5472.CAN-17-1240
Takeishi, S., and Nakayama, K. I. (2014). Role of Fbxw7 in the maintenance of normal stem cells and cancer-initiating cells. Br. J. Cancer 111 (6), 1054–1059. doi:10.1038/bjc.2014.259
Takeishi, S., and Nakayama, K. I. (2016). To wake up cancer stem cells, or to let them sleep, that is the question. Cancer Sci. 107 (7), 875–881. doi:10.1111/cas.12958
Takeishi, S., Matsumoto, A., Onoyama, I., Naka, K., Hirao, A., and Nakayama, K. I. (2013). Ablation of Fbxw7 eliminates leukemia-initiating cells by preventing quiescence. Cancer Cell 23 (3), 347–361. doi:10.1016/j.ccr.2013.01.026
Tang, B., Lei, B., Qi, G., Liang, X., Tang, F., Yuan, S., et al. (2016). MicroRNA-155-3p promotes hepatocellular carcinoma formation by suppressing FBXW7 expression. J. Exp. Clin. Cancer Res. CR 35 (1), 93. doi:10.1186/s13046-016-0371-6
Tang, X., Yang, W., Shu, Z., Shen, X., Zhang, W., Cen, C., et al. (2019). MicroRNA-223 promotes hepatocellular carcinoma cell resistance to sorafenib by targeting FBW7. Oncol. Rep. 41 (2), 1231–1237. doi:10.3892/or.2018.6908
Tang, G. L. Q., Lai, J. X. H., and Pervaiz, S. (2023). Ubiquitin-proteasome pathway-mediated regulation of the Bcl-2 family: effects and therapeutic approaches. Haematologica 109 (1), 33–43. doi:10.3324/haematol.2023.283730
Targeting cell-cycle machinery in cancer - PubMed (2024). Available at: http://pubmed6.0089.lunwenlib.com/33891890/
Tekcham, D. S., Chen, D., Liu, Y., Ling, T., Zhang, Y., Chen, H., et al. (2020). F-box proteins and cancer: an update from functional and regulatory mechanism to therapeutic clinical prospects. Theranostics 10 (9), 4150–4167. doi:10.7150/thno.42735
Thirimanne, H. N., Wu, F., Janssens, D. H., Swanger, J., Diab, A., Feldman, H. M., et al. (2022). Global and context-specific transcriptional consequences of oncogenic Fbw7 mutations. eLife 11, e74338. doi:10.7554/eLife.74338
Tong, J., Tan, S., Zou, F., Yu, J., and Zhang, L. (2017). FBW7 mutations mediate resistance of colorectal cancer to targeted therapies by blocking Mcl-1 degradation. Oncogene 36 (6), 787–796. doi:10.1038/onc.2016.247
Tu, K., Yang, W., Li, C., Zheng, X., Lu, Z., Guo, C., et al. (2014). Fbxw7 is an independent prognostic marker and induces apoptosis and growth arrest by regulating YAP abundance in hepatocellular carcinoma. Mol. Cancer 13 (1), 110. doi:10.1186/1476-4598-13-110
Venizelos, A., Elvebakken, H., Perren, A., Nikolaienko, O., Deng, W., Lothe, I. M. B., et al. (2021). The molecular characteristics of high-grade gastroenteropancreatic neuroendocrine neoplasms. Endocr. Relat. Cancer 29 (1), 1–14. doi:10.1530/ERC-21-0152
Wang, Y., Liu, Y., Lu, J., Zhang, P., Wang, Y., Xu, Y., et al. (2013). Rapamycin inhibits FBXW7 loss-induced epithelial-mesenchymal transition and cancer stem cell-like characteristics in colorectal cancer cells. Biochem. Biophys. Res. Commun. 434 (2), 352–356. doi:10.1016/j.bbrc.2013.03.077
Wang, X., Zhang, J., Zhou, L., Sun, W., Zheng, Z. G., Lu, P., et al. (2015). Fbxw7 regulates hepatocellular carcinoma migration and invasion via Notch1 signaling pathway. Int. J. Oncol. 47 (1), 231–243. doi:10.3892/ijo.2015.2981
Wang, J., Wang, H., Peters, M., Ding, N., Ribback, S., Utpatel, K., et al. (2019). Loss of Fbxw7 synergizes with activated Akt signaling to promote c-Myc dependent cholangiocarcinogenesis. J. Hepatol. 71 (4), 742–752. doi:10.1016/j.jhep.2019.05.027
Wang, S., Zheng, Y., Yang, F., Zhu, L., Zhu, X. Q., Wang, Z. F., et al. (2021a). The molecular biology of pancreatic adenocarcinoma: translational challenges and clinical perspectives. Signal Transduct. Target Ther. 6 (1), 249. doi:10.1038/s41392-021-00659-4
Wang, H., Guo, M., Wei, H., and Chen, Y. (2021b). Targeting MCL-1 in cancer: current status and perspectives. J. Hematol. OncolJ Hematol. Oncol. 14, 67. doi:10.1186/s13045-021-01079-1
Wang, H. P., Chen, W. J., Shen, J. M., Ye, T., and Xie, H. W. (2021c). Attenuating glucose metabolism by Fbxw7 promotes Taxol sensitivity of colon cancer cells through downregulating NADPH oxidase 1 (Nox1). Ann. Transl. Med. 9 (10), 886. doi:10.21037/atm-21-2076
Wang, W., Ye, L., Li, H., Chen, W., Hong, W., Mao, W., et al. (2025). A narrative review on advances in neoadjuvant immunotherapy for esophageal cancer: molecular biomarkers and future directions. Int. J. Cancer 156, 20–33. doi:10.1002/ijc.35153
Wei, W., Qin, B., Wen, W., Zhang, B., Luo, H., Wang, Y., et al. (2023). FBXW7β loss-of-function enhances FASN-mediated lipogenesis and promotes colorectal cancer growth. Signal Transduct. Target Ther. 8 (1), 187. doi:10.1038/s41392-023-01405-8
Welcker, M., and Clurman, B. E. (2007). Fbw7/hCDC4 dimerization regulates its substrate interactions. Cell Div. 2, 7. doi:10.1186/1747-1028-2-7
Welcker, M., and Clurman, B. E. (2008). FBW7 ubiquitin ligase: a tumour suppressor at the crossroads of cell division, growth and differentiation. Nat. Rev. Cancer 8 (2), 83–93. doi:10.1038/nrc2290
Welcker, M., Larimore, E. A., Swanger, J., Bengoechea-Alonso, M. T., Grim, J. E., Ericsson, J., et al. (2013). Fbw7 dimerization determines the specificity and robustness of substrate degradation. Genes Dev. 27 (23), 2531–2536. doi:10.1101/gad.229195.113
Willson, J. (2022). DUBTACs for targeted protein stabilization. Nat. Rev. Drug Discov. 21 (4), 258. doi:10.1038/d41573-022-00039-9
Wood, K. C. (2020). Overcoming MCL-1-driven adaptive resistance to targeted therapies. Nat. Commun. 11 (1), 531. doi:10.1038/s41467-020-14392-z
Wu, X. Z., Wang, K. P., Song, H. J., Xia, J. H., Jiang, Y., and Wang, Y. L. (2015). MiR-27a-3p promotes esophageal cancer cell proliferation via F-box and WD repeat domain-containing 7 (FBXW7) suppression. Int. J. Clin. Exp. Med. 8 (9), 15556–15562.
Wu, W. J., Shi, J., Hu, G., Yu, X., Lu, H., Yang, M. L., et al. (2016). Wnt/β-catenin signaling inhibits FBXW7 expression by upregulation of microRNA-770 in hepatocellular carcinoma. Tumor Biol. 37 (5), 6045–6051. doi:10.1007/s13277-015-4452-5
Wu, X., Iwatsuki, M., Takaki, M., Saito, T., Hayashi, T., Kondo, M., et al. (2024). FBXW7 regulates the sensitivity of imatinib in gastrointestinal stromal tumors by targeting MCL1. Gastric Cancer 27 (2), 235–247. doi:10.1007/s10120-023-01454-6
X, C., X, X., D, C., F, Z., and W, W. (2019a). Therapeutic potential of targeting the Wnt/β-catenin signaling pathway in colorectal cancer. Biomed. Pharmacother. Biomedecine Pharmacother. 110, 473–481. doi:10.1016/j.biopha.2018.11.082
X, K., L, L., R, C., K, W., M, C., B, C., et al. (2019b). SCFFBXW7/GSK3β-Mediated GFI1 degradation suppresses proliferation of gastric cancer cells. Cancer Res. 79 (17), 4387–4398. doi:10.1158/0008-5472.CAN-18-4032
X, W., Y, L., P, L., S, L., Y, P., and Pan, Y. (2022). FBXW7 reduces the cancer stem cell-like properties of hepatocellular carcinoma by regulating the ubiquitination and degradation of ACTL6A. Stem Cells Int. 2022, 3242482. doi:10.1155/2022/3242482
Xia, W., Zhou, J., Luo, H., Liu, Y., Peng, C., Zheng, W., et al. (2017). MicroRNA-32 promotes cell proliferation, migration and suppresses apoptosis in breast cancer cells by targeting FBXW7. Cancer Cell Int. 17, 14. doi:10.1186/s12935-017-0383-0
Xiang, J., Hang, J. B., Che, J. M., and Li, H. C. (2015). miR-25 is up-regulated in non-small cell lung cancer and promotes cell proliferation and motility by targeting FBXW7. Int. J. Clin. Exp. Pathol. 8 (8), 9147–9153.
Xie, C. M., and Sun, Y. (2019). The MTORC1-mediated autophagy is regulated by the FBXW7-SHOC2-RPTOR axis. Autophagy 15 (8), 1470–1472. doi:10.1080/15548627.2019.1609864
Xie, X., Li, H., Gao, C., Lai, Y., Liang, J., Chen, Z., et al. (2022). Downregulation of circular RNA circPSD3 promotes metastasis by modulating FBXW7 expression in clear cell renal cell carcinoma. J. Oncol. 2022 (1), 5084631. doi:10.1155/2022/5084631
Xu, Y., Sengupta, T., Kukreja, L., and Minella, A. C. (2010). MicroRNA-223 regulates cyclin E activity by modulating expression of F-box and WD-40 domain protein 7. J. Biol. Chem. 285 (45), 34439–34446. doi:10.1074/jbc.M110.152306
Xu, D., Shan, B., Lee, B. H., Zhu, K., Zhang, T., Sun, H., et al. (2015). Phosphorylation and activation of ubiquitin-specific protease-14 by Akt regulates the ubiquitin-proteasome system. eLife 4, e10510. doi:10.7554/eLife.10510
Xu, W., Taranets, L., and Popov, N. (2016). Regulating Fbw7 on the road to cancer. Semin. Cancer Biol. 36, 62–70. doi:10.1016/j.semcancer.2015.09.005
Y, I., I, O., Ki, N., and K, N. (2008). Notch-dependent cell cycle arrest and apoptosis in mouse embryonic fibroblasts lacking Fbxw7. Oncogene 27 (47), 6164–6174. doi:10.1038/onc.2008.216
Y, N., H, I., Y, K., M, K., A, M., T, K., et al. (2010). Decreased expression of FBXW7 is correlated with poor prognosis in patients with esophageal squamous cell carcinoma. Exp. Ther. Med. 1 (5), 841–846. doi:10.3892/etm.2010.115
Y, L., Y, S., Y, Z., X, H., G, Z., J, H., et al. (2021). Demethylzeylasteral inhibits proliferation, migration, and invasion through FBXW7/c-Myc axis in gastric cancer. MedComm 2 (3), 467–480. doi:10.1002/mco2.73
Y, L., H, C., H, B., J, Z., R, W., and L, Z. (2023). Comprehensive characterization of FBXW7 mutational and clinicopathological profiles in human colorectal cancers. Front. Oncol. 13, 1154432. doi:10.3389/fonc.2023.1154432
Yang, W., Dou, C., Wang, Y., Jia, Y., Li, C., Zheng, X., et al. (2015). MicroRNA-92a contributes to tumor growth of human hepatocellular carcinoma by targeting FBXW7. Oncol. Rep. 34 (5), 2576–2584. doi:10.3892/or.2015.4210
Yang, Q., Sun, Y., Qiu, B., and Zhao, H. (2022). FBXW7 enhances cisplatin-induced apoptosis in oral cancer cell lines. Int. Dent. J. 73 (5), 620–627. doi:10.1016/j.identj.2022.11.008
Ye, Z., Zhuo, Q., Hu, Q., Xu, X., liu, M., Zhang, Z., et al. (2020). FBW7-NRA41-SCD1 axis synchronously regulates apoptosis and ferroptosis in pancreatic cancer cells. Redox Biol. 38, 101807. doi:10.1016/j.redox.2020.101807
Yeh, C. H., Bellon, M., and Nicot, C. (2018). FBXW7: a critical tumor suppressor of human cancers. Mol. Cancer 17 (1), 115. doi:10.1186/s12943-018-0857-2
Yokobori, T., Mimori, K., Iwatsuki, M., Ishii, H., Onoyama, I., Fukagawa, T., et al. (2009). p53-Altered FBXW7 expression determines poor prognosis in gastric cancer cases. Cancer Res. 69 (9), 3788–3794. doi:10.1158/0008-5472.CAN-08-2846
Yokobori, T., Mimori, K., Iwatsuki, M., Ishii, H., Tanaka, F., Sato, T., et al. (2012). Copy number loss of FBXW7 is related to gene expression and poor prognosis in esophageal squamous cell carcinoma. Int. J. Oncol. 41 (1), 253–259. doi:10.3892/ijo.2012.1436
Yu, J., Zhang, W., Gao, F., Liu, Y. X., Chen, Z. Y., Cheng, L. Y., et al. (2014). FBW7 increases chemosensitivity in hepatocellular carcinoma cells through suppression of epithelial-mesenchymal transition. Hepatobiliary Pancreat. Dis. Int. HBPD Int. 13 (2), 184–191. doi:10.1016/s1499-3872(14)60029-1
Yu, H., Ling, T., Shi, R., Shu, Q., Li, Y., and Tan, Z. (2015). Expression of FBXW7 in esophageal squamous cell carcinoma and its clinical significance. Zhonghua Zhong Liu Za Zhi 37 (5), 347–351. doi:10.3760/cma.j.issn.0253-3766.2015.05.006
Yu, F., Yu, C., Li, F., Zuo, Y., Wang, Y., Yao, L., et al. (2021). Wnt/β-catenin signaling in cancers and targeted therapies. Signal Transduct. Target Ther. 6, 307. doi:10.1038/s41392-021-00701-5
Yumimoto, K., and Nakayama, K. I. (2020). Recent insight into the role of FBXW7 as a tumor suppressor. Semin. Cancer Biol. 67 (Pt 2), 1–15. doi:10.1016/j.semcancer.2020.02.017
Zeng, J., Chen, Z., He, Y., Jiang, Z., Zhang, Y., Dong, Q., et al. (2024). A patent review of SCF E3 ligases inhibitors for cancer: structural design, pharmacological activities and structure–activity relationship. Eur. J. Med. Chem. 278, 116821. doi:10.1016/j.ejmech.2024.116821
Zhan, P., Wang, Y., Zhao, S., Liu, C., Wang, Y., Wen, M., et al. (2015). FBXW7 negatively regulates ENO1 expression and function in colorectal cancer. Lab. Investig. J. Tech. Methods Pathol. 95 (9), 995–1004. doi:10.1038/labinvest.2015.71
Zhang, Y., and Wang, X. (2020). Targeting the Wnt/β-catenin signaling pathway in cancer. J. Hematol. OncolJ Hematol. Oncol. 13 (1), 165. doi:10.1186/s13045-020-00990-3
Zhang, P., Cao, L., Fan, P., Mei, Y., and Wu, M. (2016a). LncRNA-MIF, a c-Myc-activated long non-coding RNA, suppresses glycolysis by promoting Fbxw7-mediated c-Myc degradation. EMBO Rep. 17 (8), 1204–1220. doi:10.15252/embr.201642067
Zhang, Q., Zhang, Y., Parsels, J. D., Lohse, I., Lawrence, T. S., Pasca di Magliano, M., et al. (2016b). Fbxw7 deletion accelerates KrasG12D-driven pancreatic tumorigenesis via Yap accumulation. Neoplasia N. Y. N. 18 (11), 666–673. doi:10.1016/j.neo.2016.08.009
Zhang, Q., Mady, A. S. A., Ma, Y., Ryan, C., Lawrence, T. S., Nikolovska-Coleska, Z., et al. (2019). The WD40 domain of FBXW7 is a poly(ADP-ribose)-binding domain that mediates the early DNA damage response. Nucleic Acids Res. 47 (8), 4039–4053. doi:10.1093/nar/gkz058
Zhang, Q., Li, X., Cui, K., Liu, C., Wu, M., Prochownik, E. V., et al. (2020). The MAP3K13-TRIM25-FBXW7α axis affects c-Myc protein stability and tumor development. Cell Death Differ. 27 (2), 420–433. doi:10.1038/s41418-019-0363-0
Zhou, N., and Hofstetter, W. L. (2020). Prognostic and therapeutic molecular markers in the clinical management of esophageal cancer. Expert Rev. Mol. Diagn 20 (4), 401–411. doi:10.1080/14737159.2020.1731307
Zhou, Z. yu, sheng, Tu K., Zhang, J., Zheng, X., Gao, J., Yao, Y. min, et al. (2012). [Expression of Fbxw7 and its correlation with cell proliferation in human hepatocellular carcinoma]. Xi bao yu fen zi mian yi xue za zhi. Chin. J. Cell Mol. Immunol. 28 (12), 1303–1306.
Zhou, X., Jin, W., Jia, H., Yan, J., and Zhang, G. (2015). MiR-223 promotes the cisplatin resistance of human gastric cancer cells via regulating cell cycle by targeting FBXW7. J. Exp. Clin. Cancer Res. CR 34 (1), 28. doi:10.1186/s13046-015-0145-6
Zhong, Z., and Virshup, D. M. (2024). Recurrent mutations in tumor suppressor FBXW7 bypass Wnt/β-catenin addiction in cancer. Sci. Adv. 10 (14), eadk1031. doi:10.1126/sciadv.adk1031
C/EBPδCCAAT enhancer-binding protein-delta
CHD4 Chromodomain helicase DNA-binding protein 4
CLM Colorectal cancer liver metastases
CoA Coactivator
CPD Cdc4 phosphodegron
CRC Colorectal cancer
CSCs Cancer stem cells
CSL CBF-1/suppressor of hairless/Lag1
CSN6 COP9 signalosome subunit 6
ctDNA Circulating tumor DNA
E1 Ubiquitin-activating enzymes
E2 Ubiquitin-conjugating enzymes
E3 Ubiquitin ligase
EBV Epstein-Barr virus
EGFR Epidermal Growth Factor Receptor
EMT Epithelial-mesenchymal transition
EMT Epithelial-mesenchymal transition
ENO1 Enolase 1
ERK1/2 Extracellular signal-regulated kinases 1 and 2
ESCC Esophageal squamous cell carcinoma
FASN Fatty acid synthase
FBXW7 F-Box and WD Repeat Domain Containing 7
FGFR Fibroblast growth factor receptor 2
GBM Glioblastoma
GC Gastric cancer
GIST Gastrointestinal stromal tumors
GSK3β Glycogen synthase kinase-3β
GSK3β Glycogen synthase kinase-3β
HCC Hepatocellular carcinoma
HER-2 Human epidermal growth factor receptor 2
HES5 HES family bHLH transcription factor 5
Hsp90 Heat shock protein 90
iCCA Intrahepatic Cholangiocarcinoma
IDH1/2 Isocitrate dehydrogenase 1 or 2
KRAS Kirsten rat sarcoma viral oncogene
KRAS Kirsten rat sarcoma viral oncogene
LEF lymphocyte enhancer factor-1
LSD1 Lysine-specific demethylase 1
MAMLs Mastermind-like proteins
MAP4 Microtubule-associated proteins 4
MAPK Mitogen-activated protein kinase
MCL-1 Myeloid leukemia 1
MiRNA micro-RNA
MMR Mismatch Repair
MSI Microsatellite instability
mTOR Mammalian target of rapamycin
N/A Not assessable
ncRNA Noncoding RNAs
NICD NOTCH intracellular domain
Nox1 NADPH oxidase 1
NTRK Neurotrophic tyrosine receptor kinase
NTRK Neurotrophic tyrosine receptor kinase
PC Pancratic cancer
PD-L1 Programmed cell death ligand 1
PI3K Phosphoinositide 3-kinase
Plk2 Polo-like kinase 2
PTEN Phosphatase and tensin homolog
SCF Skp1-Cullin1-F-box
SKP1 S-phase kinase-associated protein 1
STYX Serine/threonine/tyrosine interacting protein
TCF T cell factor
TERT Telomerase reverse transcriptase
TGF-β Transforming growth factor beta
TMB Tumor mutation burden
TNBC Triple negative breast cancer
UPS Ubiquitin-proteasome system
USP28 Ubiquitin-specific proteases 28
Usp9x Ubiquitin-specific peptidase 9X
VEGFR Vascular endothelial growth factor
VEGFR Vascular endothelial growth factor
YAP Yes-associated protein
Keywords: FBXW7, gastrointestinal cancers, molecular mechanism, therapeutic strategies, biomarker
Citation: Wang W, Liu X, Zhao L, Jiang K, Yu Z, Yang R, Zhou W, Cui J and Liang T (2024) FBXW7 in gastrointestinal cancers: from molecular mechanisms to therapeutic prospects. Front. Pharmacol. 15:1505027. doi: 10.3389/fphar.2024.1505027
Received: 01 October 2024; Accepted: 28 November 2024;
Published: 18 December 2024.
Edited by:
Ralf Weiskirchen, RWTH Aachen University, GermanyCopyright © 2024 Wang, Liu, Zhao, Jiang, Yu, Yang, Zhou, Cui and Liang. This is an open-access article distributed under the terms of the Creative Commons Attribution License (CC BY). The use, distribution or reproduction in other forums is permitted, provided the original author(s) and the copyright owner(s) are credited and that the original publication in this journal is cited, in accordance with accepted academic practice. No use, distribution or reproduction is permitted which does not comply with these terms.
*Correspondence: Tingting Liang, bGlhbmd0dEBqbHUuZWR1LmNu
Disclaimer: All claims expressed in this article are solely those of the authors and do not necessarily represent those of their affiliated organizations, or those of the publisher, the editors and the reviewers. Any product that may be evaluated in this article or claim that may be made by its manufacturer is not guaranteed or endorsed by the publisher.
Research integrity at Frontiers
Learn more about the work of our research integrity team to safeguard the quality of each article we publish.