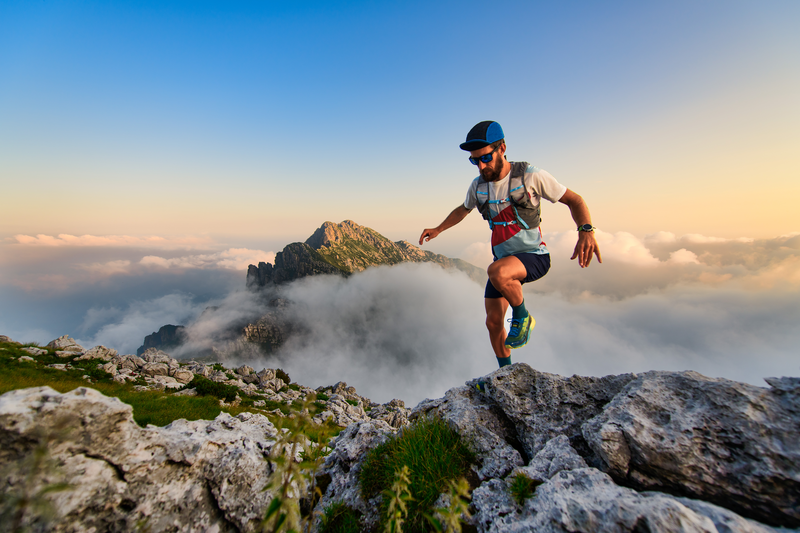
95% of researchers rate our articles as excellent or good
Learn more about the work of our research integrity team to safeguard the quality of each article we publish.
Find out more
ORIGINAL RESEARCH article
Front. Pharmacol. , 18 December 2024
Sec. Pharmacoepidemiology
Volume 15 - 2024 | https://doi.org/10.3389/fphar.2024.1486422
This article is part of the Research Topic Advances in Drug-induced Diseases Volume II View all 43 articles
Background: This study aims to investigate adverse drug reaction signals associated with coagulopathies through data mining using the Adverse Event Reporting System (FAERS) of the US Food and Drug Administration. Prompt identification of high-risk drugs provides a valuable basis for enhancing clinical drug safety.
Methods: The adverse event reports related to coagulopathies from Q1 2004 to Q2 2024 were extracted from the ASCII data packages in FAERS. The reporting odds ratio (ROR), proportional reporting ratio (PRR), and Bayesian confidence propagation neural network (BCPNN) were used to identify adverse drug reaction signals associated with coagulopathies.
Results: During the reporting period, 40,545 reports were retrieved, with a slightly higher proportion of females than males. Among the top 30 drugs associated with the occurrence of coagulopathies, 24 drugs exhibited positive signals in risk analysis. Based on the individual drug reporting odds ratio (95% confidence interval) as a measure of risk signal strength, the top five drugs are as follows: gemcitabine [ROR (95% CI):16.87 (15.83–17.98)], busulfan [ROR (95% CI):15.51 (13.69–17.58)], anti-thymocyte globulin [ROR (95% CI):15.49 (13.49–17.78)], tacrolimus [ROR (95% CI):12.7 (11.57–13.95)], etonogestrel and ethinylestradiol vaginal ring [ROR (95% CI):11.88 (10.95–12.89)]. After categorizing the drugs, the strongest risk signal is sex hormones and modulators of the genital system [ROR (95% CI):11.88 (10.95–12.89)], followed by analgesics [ROR (95%CI): 6.73 (6.38–7.1)], immunosuppressants [ROR (95% CI):3.91 (3.76–4.05)], antineoplastic agents [ROR (95% CI):3.33 (3.22–3.45)], corticosteroids for systemic use [ROR (95% CI): 2.94 (2.73–3.18)], antiepileptics [ROR (95% CI):1.93 (1.71–2.18)], drugs used in diabetes [ROR (95% CI):1.5 (1.34–1.67)], antibacterials for systemic use [ROR (95% CI):1.46 (1.28–1.68)].
Conclusion: Our findings indicate that multiple drugs are associated with an increased risk of coagulopathies. From the pharmacovigilance perspective, proactive analysis of these drugs aids in clinical monitoring and enhances risk identification of coagulopathies.
Coagulopathies are diseases characterized by reduced blood clotting capacity, resulting in a pathological tendency toward bleeding and thrombosis (Iba et al., 2019a; Iba and Levy, 2020). Coagulopathies arise from various conditions, such as severe trauma, sepsis, cancer, hematological malignancies, and pregnancy-related complications (Levi, 2014; Lockhart et al., 2016; Iba et al., 2019b; Kleinveld et al., 2022). In addition, drug-induced coagulopathies are also common in clinical practice (Novak et al., 2012; Cui et al., 2019; Kumar et al., 2019). Coagulopathies can stem from platelet dysfunction, impaired thrombin generation, hypofibrinogenemia, and hyperfibrinolysis (Gando et al., 2016; Bartoszko and Karkouti, 2021). Coagulopathies are severe complications in patients, which can lead to multiple organ dysfunction and are linked to poorer patient prognosis (Helms et al., 2023; Li et al., 2023). Studies have shown that up to 56% of trauma patients and over 40% of critically-ill patients develop coagulopathies (Stensballe et al., 2017; Petros, 2019). Severe coagulopathies are associated with a more than fourfold increase in adverse bleeding events, blood transfusion volume, and mortality, along with prolonged hospital and ICU stays. This heightened risk highlights the importance of early identification and prevention of coagulopathies (Zhao et al., 2021; Li et al., 2023).
Clinical manifestations of coagulopathies are diverse, primarily including bleeding tendency (such as skin ecchymosis, joint hematoma, and visceral hemorrhage), thrombosis (e.g., deep vein thrombosis and pulmonary embolism). They are usually accompanied by abnormal laboratory results, such as prothrombin time (PT), activated partial thromboplastin time (APTT), fibrinogen concentration, platelet count, function testing, and special coagulation factor testing (Moore et al., 2020; Giustozzi et al., 2021; Gómez-Mesa et al., 2021). Drugs can affect the coagulation system through multiple biomolecules and signal pathways. For example, some drugs inhibit the activity of catalases, while others cause bleeding by interfering with the interaction between platelets and blood vessel walls. These complex mechanisms of action complicate the diagnosis and treatment of drug-induced coagulopathies (Cassar et al., 2005; Xiao et al., 2013; Izzedine and Perazella, 2015; Bauer et al., 2022). Due to the complex pathogenesis and diagnostic challenges of coagulopathies, which often require multidisciplinary collaboration, research in this area is limited. Identifying drugs closely linked to coagulopathies is essential, as it enables medical institutions to develop precise monitoring and intervention strategies while providing critical safety information for clinicians and pharmacists to support safer drug use for patients.
Currently, information on adverse reactions related to coagulopathies is mainly recorded in drug labels. Although drug safety is evaluated in clinical trials, these clinical trials may not fully capture the real-world scenario due to sample limits, treatment duration, and co-morbid conditions (Javed and Kumar, 2024). Therefore, conducting real-world research offers a more comprehensive approach to understanding drug safety. Although identifying drugs related to coagulopathies is crucial, no comprehensive list of these drugs currently exists. While most existing research focuses on evaluating the coagulopathy risks of individual drugs, it is equally important to investigate coagulopathies associated with a broader range of medications (Chai and Babu, 2014; Cui et al., 2019; Peralta et al., 2019; Guo et al., 2022).
Compared to laboratory and clinical trial data, pharmacovigilance data reflects real-world drug use more accurately and is vital for post-market surveillance (Raschi et al., 2020b). The FAERS is the largest public drug alert database for spontaneous reports of adverse events, gathering data from medical personnel, consumers, manufacturers, etc. It plays an essential role in informing healthcare professionals and the public about the potential risks of drugs (Sakaeda et al., 2013; Raschi et al., 2019; Raschi et al., 2020a).
A signal is new or previously unknown information linking an adverse event to a drug (Javed and Kumar, 2024). Generating a signal requires more than one high-quality report (Subeesh et al., 2017; Sharma and Kumar, 2022). Data mining algorithms (DMAs) such as reporting odds ratio (ROR), proportional reporting ratio (PRR), and Bayesian confidence propagation neural network (BCPNN) are common analytical methods for detecting signals in pharmacovigilance databases (Kubota et al., 2004; Rothman et al., 2004). These methods identify patterns of associations or unexpected occurrences of events in large databases using statistical analysis.
The purpose of this study is to comprehensively investigate the risks of drug-induced coagulopathies through the FAERS and identify drugs with potential coagulopathy risks that are not listed in the package insert. This research aims to provide an overview of drugs that may induce coagulopathies from a pharmacovigilance perspective, offering valuable insights for clinical practice.
The FAERS database is the central system for post-marketing adverse drug reaction monitoring in the United States and is also one of the main approaches for current pharmacovigilance research (Zhang et al., 2024). In our study, ASCII data packages submitted from the first quarter of 2004 to the second quarter of 2024 were retrieved from the database. Each package contains demographic and administrative information (DEMO), drug information (DRUG), adverse events (REAC), patient outcomes (OUTC), report sources (RPSR), start and end dates for reported drugs (THER), indications for use (INDI) (Yang et al., 2022). Import all data analysis into R version 4.3.3 and Excel software for data cleaning and analysis. Delete duplicate data according to the FDA’s suggestion. If the case has the same case ID, it will retain the latest report with FDA_DT; if the case ID is the same as the FDA_DT, it will retain a large master ID. After the repeated data is eliminated, some primary ID repeated items are still found, so the auxiliary duplicate data is performed (Yu et al., 2021).
The symptoms of AEs are coded using the Medical Dictionary for Regulatory Activities (MedDRA). MedDRA is an internationally standardized and clinically validated terminology system (Kumar et al., 2019). In the FAERS database, encode each report using the preferred term (PT) from MedDRA terminology, which is categorized into High-Level Term (HLT), High-Level Group Term (HLGT), and System Organ Class (SOC) in MedDRA (Zhang et al., 2023; Li et al., 2024). According to the latest MedDRA 27.0 version, our study searched for “coagulopathies (MedDRA 10064477)” at the HLT level and identified 26 related PTs, mainly including coagulopathy, disseminated intravascular coagulation, thrombotic microangiopathy, hypercoagulation, antiphospholipid, etc. In line with MedDRA 27.0 standards, only “primary suspect (PS)” drugs coded by PT were included to focus on the highest level of suspicion for drug-related coagulopathies. The Anatomical Therapeutic Chemical (ATC) classification system was used to code the preliminary drug list, the final drug list for analysis was obtained after excluding ambiguous drug names and integrating drugs with the same ingredient (Fan et al., 2024; Li et al., 2024).
In this study, we employed ROR, PRR, and BCPNN to identify signals for potential increased risk of drug-related coagulopathies (Poluzzi et al., 2009; Sakaeda et al., 2013; Sharma and Kumar, 2022; Zou et al., 2023; Javed and Kumar, 2024). The ROR and PRR algorithms are frequentist (non-Bayesian) algorithms, which are simple to calculate and have high sensitivity. The advantage of ROR is that it corrects for bias due to the low number of reports of certain events compared to PRR, while the advantage of PRR is that it is less affected by the omission of adverse events (Evans et al., 2001; Rothman et al., 2004). BCPNN, a Bayesian algorithm, effectively integrates data from multiple sources and supports cross-validation (Kubota et al., 2004). It accounts for uncertainties in the disproportionate rate, especially with smaller adverse event samples, reduces false positives, and is used for pattern recognition in higher dimensions (Tang et al., 2022). This study combines multiple algorithms to leverage their respective strengths, expanding the detection range and cross-validating results to enhance sensitivity and specificity in signal detection (Noguchi et al., 2018; Zhou et al., 2023). Higher values of these parameters indicate stronger signal strength, representing the level of association between drugs and coagulopathies. The formulas and criteria for each algorithm are shown in Table 1 (Sharma et al., 2023; Liu et al., 2024). Positive signals are identified if any of the three methods’ criteria are met, indicating a possible association between the drugs and the event (Alenzi et al., 2024).
A total of 21, 433, 114 reports were retrieved from the FAERS database. After data cleaning and analysis, 40,545 reports on coagulopathies were collected. We found that 4,687 drugs are related to coagulopathies. After removing anticoagulant and antiplatelet drugs, we conducted a comprehensive analysis of the top 30 drugs, which is detailed in the flowchart (Figure 1).
Detailed information on the adverse event reports of the patient was introduced in Table 2. As for patients, males reported 17,893 (44.1%) adverse event reports, while females submitted 18,734 (46.2%), with a slightly higher number of reports from females than males. Regarding age composition, patients aged 18–64.9 submitted 16,547 (40.8%) adverse event reports, accounting for the most significant proportion. These data come from submissions from many countries. The United States is the most extensive reporting country, and it submitted 14,951 (36.9%) reports, followed by Japan, which submitted 7,310 (18%) adverse event reports. In terms of the ending of the patient, the number of “deaths” in patients is the largest, with a total of 13,183 (32.5%) adverse event reports, followed by “hospitalization or prolongation of hospitalization” with 12,511 (30.9%) adverse event reports. In terms of reporting year, 2014 had the highest reported cases (Figure 2). After searching the PTs contained in “coagulopathies”, a total of 26 related PTs were obtained. Among them, “coagulopathy” is the most reported PT (Table 3).
To evaluate the risk signals associated with various drugs that cause coagulopathies, analyze the top 30 drugs with representative reported quantities. These drugs can be divided into the following categories: antineoplastic agents, immunosuppressants, analgesics, corticosteroids for systemic use, sex hormones and modulators of the genital system, antiepileptics, drugs used in diabetes, antibacterials for systemic use. We analyzed the risk signal intensity of individual drugs, and the specific analysis results are shown in Figure 3 and Supplementary Table S1. At the same time, we also evaluated the strength of risk signals after classification, and a comprehensive summary of the detailed analysis is provided in Figure 4 and Supplementary Table S2. To provide a more comprehensive profile of these top 30 drugs, we compiled a list of drugs containing indications, dose, mode of administration, and adverse effects based on drug labels, which are summarized in Supplementary Table S3.
From Supplementary Table S1, it can be seen that 24 drugs exhibit positive signals. The top 5 drugs with positive signals are gemcitabine [ROR (95% CI):16.87 (15.83–17.98)], busulfan [ROR (95% CI):15.51 (13.69–17.58)], anti-thymocyte globulin [ROR (95% CI):15.49 (13.49–17.78)], tacrolimus [ROR (95% CI):12.7 (11.57–13.95)], etonogestrel and ethinylestradiol vaginal ring [ROR (95% CI):11.88 (10.95–12.89)]. Other positive signal drugs are arranged in order of risk signal strength: eculizumab, ciclosporin, mycophenolate mofetil, paracetamol, prednisolone, cyclophosphamide, bevacizumab, carboplatin, dexamethasone, sunitinib, cytarabine, nivolumab, lamotrigine, pembrolizumab, ibuprofen, capecitabine, metformin, ciprofloxacin, methotrexate. The larger the value of the ROR, the stronger the risk signal, indicating a greater risk of causing coagulopathies. The higher the ROR value, the greater the possibility of adverse events related to the use of specific drugs.
After the drug classification, the ranking is based on ROR: sex hormones and modulators of the genital system [ROR (95% CI):11.88 (10.95–12.89)], analgesics [ROR (95%CI): 6.73 (6.38–7.1)], immunosuppressants [ROR (95% CI):3.91 (3.76–4.05)], antineoplastic agents [ROR (95% CI):3.33 (3.22–3.45)], corticosteroids for systemic use [ROR (95% CI): 2.94 (2.73–3.18)], antiepileptics [ROR (95% CI):1.93 (1.71–2.18)], drugs used in diabetes [ROR (95% CI):1.5 (1.34–1.67)], antibacterials for systemic use [ROR (95% CI):1.46 (1.28–1.68)]. The strongest risk signal is sex hormones and modulators of the genital system, followed by analgesics, immunosuppressants, antineoplastic agents, corticosteroids for systemic use, antiepileptics, drugs used in diabetes, antibacterials for systemic use.
This study provides a comprehensive and systematic investigation of adverse events related to drug-induced coagulopathies using the FAERS database. We identified drugs significantly associated with coagulopathies based on case numbers and signal strength. We observed that some drugs do not list coagulopathy-related adverse reactions in their labeling, highlighting the need to further explore drugs closely associated with coagulopathies.
To minimize bias and reduce the occurrence of false positives and false negatives, we used ROR, PRR, and BCPNN methods for analysis. A total of 24 drugs exhibited positive signals in the risk analysis. In addition, standardized naming was used to ensure precise and reliable analysis of our findings. According to their pharmacological effects, the drugs with positive signals can be divided into the following categories: anti-tumor drugs, immunosuppressants, anti-inflammatory and analgesic drugs, hypoglycemic drugs, antiepileptic drugs, steroid hormone drugs, antibacterial drugs, and contraceptive drugs. Higher ROR values indicate a greater risk of coagulopathy-related adverse reactions. Our research provides a basis for clinicians to make informed prescribing decisions and serves as a reminder for healthcare professionals to be vigilant about potential coagulopathies of these drugs in clinical practice.
Anti-tumor drugs accounted for the largest proportion of coagulopathy cases in our study. The incidence of coagulopathies in cancer patients is approximately 6%–15%. With cancer increasingly managed as a chronic disease and the use of new drugs on the rise, their incidence rate is expected to rise (Lechner and Obermeier, 2012; Al-Nouri et al., 2015). Anti-tumor drug therapies appear to be more common contributors to coagulopathies than cancer itself, with associated conditions including thrombotic microangiopathy (TMA), thrombocytopenia, intravascular thrombosis, and ischemia-induced terminal organ damage (Izzedine and Perazella, 2015; Jodele et al., 2015). The chemotherapeutic drugs most frequently associated with coagulopathies are mitomycin-C and gemcitabine (Aklilu and Shirali, 2023). In our study, gemcitabine demonstrated the highest ROR value and risk intensity. Gemcitabine is a pyrimidine analog that promotes apoptosis in rapidly dividing cells by disrupting DNA synthesis (Pandit and Royzen, 2022). Numerous studies have reported gemcitabine-induced coagulopathies, and the use of gemcitabine may increase the incidence of cancer patients (Grall et al., 2021; Ishikawa et al., 2021; van der Heijden et al., 2023). While the mechanism of gemcitabine-induced coagulopathies remains unclear, it is hypothesized to involve microvascular endothelial damage and immune complex-mediated endothelial injury (Mini et al., 2006; Zupancic et al., 2007). Carboplatin, a second-generation platinum-based anticancer drug, is cell cycle non-specific and exerts anti-tumor effects by interfering with DNA synthesis and replication (Ettinger et al., 2022; Porter et al., 2023). It has been reported that carboplatin alone or in combination with other drugs can induce coagulopathies (Iams et al., 2013; Salhi et al., 2021). Given that many cancer patients are treated with multiple chemotherapy drugs, identifying the specific causative agent of coagulopathies can be challenging. Drug-induced coagulopathies may disrupt anti-tumor treatment in patients and increase the risk of cancer progression. Our study aims to discover drugs closely related to coagulopathies, which can help clinicians discover adverse drug reactions during treatment, support preventive measures and promote rational drug use in clinical practice.
Immunosuppressants-induced coagulopathies also accounted for a substantial proportion of cases in our study. Coagulopathies are a severe adverse reaction of immunosuppressants, mainly manifested as systemic platelet aggregation, thrombocytopenia, and mechanical damage to red blood cells (George and Nester, 2014). The mechanism behind immunosuppressant-induced coagulopathies may involve immune-mediated responses as well as dose- or duration-dependent toxicity (Bhavsar et al., 2016). Endothelial injury triggers the formation of microthrombi and platelet aggregation in the vascular system (Nwaba et al., 2013). Tacrolimus is a calcineurin inhibitor commonly used as an immunosuppressant post-solid organ transplants. There have been many reports on coagulopathies caused by tacrolimus (Nwaba et al., 2013; Cortina et al., 2015; Markan et al., 2021), and some have been fatal (Gill and Meghrajani, 2022). There are also some reports of coagulopathies caused by the combination of tacrolimus and ciclosporin; both drugs showed strong signals in our study (Pham et al., 2000). Recently, there have been reports that vascular endothelial growth factor (VEGF) inhibitors such as bevacizumab can lead to coagulopathies. VEGF stimulates signaling pathways and transcription by activating its receptor VEG-FR2, which is crucial for angiogenesis. Bevacizumab disrupts this pathway, leading to thrombotic microvascular disease (Apte et al., 2019; Estrada et al., 2019).
Our study reveals that sex hormones and reproductive system regulators have the highest risk of inducing coagulopathies. Compelling evidence suggests that female hormone replacement therapies and combination oral contraceptives can induce coagulopathies and increase the risk of venous thromboembolism (Watson, 2007). The formation of coagulopathies may be related to the increased plasma concentrations of procoagulant proteins, reduced anticoagulant proteins, and altered fibrinolysis (Gialeraki et al., 2017; Teal and Edelman, 2021). Studies have shown that patients with oral contraceptives may face a significantly increased risk of developing coagulopathies during the perioperative period (Watson, 2007). Especially for patients with compound oral contraceptives, the probability of occurrence of coagulopathies is 2–4 times higher compared to patients who do not use them (Haverinen et al., 2022). The risk of coagulopathies is dose-dependent on the dose of sex hormones used (Weill et al., 2016). Excessive use of antipyretic and analgesic drugs can also lead to coagulopathies, characterized by an increased prothrombin time and international normalized ratio (Larson et al., 2005; Habib et al., 2013). Some antibiotics can increase the risk of coagulopathies, which has been confirmed in multiple studies, such as cefoperazone sulbactam, tigecycline, and linezolid (Routsi et al., 2015; Hakeam et al., 2018; Cui et al., 2019; Treml et al., 2021; Wu et al., 2021). Our study found that ciprofloxacin is associated with coagulopathies, although few studies have been conducted.
In our study, we identified some (OTC) drugs, such as paracetamol and ibuprofen, as potentially associated with coagulopathies, even though they can be purchased without a prescription. Patients should carefully read instructions before using OTC drugs, strictly follow the recommended dosage and frequency, and avoid altering the dosage or administration method (Sánchez-Sánchez et al., 2021). If symptoms of coagulopathies appear, such as severe bleeding or uncontrollable bleeding, patients should discontinue use immediately and seek medical attention (Tan et al., 2020). Pharmacists should assess patients on their health status, allergy history, medication history, and any concurrent treatments that could influence coagulopathy risk when dispensing OTC drugs. Governments, medical institutions, and pharmaceutical manufacturers can collaborate to raise awareness about the adverse reactions associated with OTC drugs and help to enhance patients’ understanding of these potential risks (Gheorghe et al., 2019; Yousaf et al., 2020). Open communication between patients and healthcare providers is crucial to optimize patient care and ensure safety. Documenting the medical history of patient is a critical step in prevention, especially for those with a history of coagulopathies, who should exercise particular caution. For critically ill patients using these drugs, regular blood coagulation testing is particularly necessary (Song et al., 2020; Fan et al., 2024). In our study, monitoring cases of coagulopathies associated with any drug in the FAERS database was critical for helping clinicians properly diagnose and manage this condition. Comprehensive information enables healthcare providers to make informed treatment decisions and apply strategies to reduce the risk of drug-induced coagulopathies.
We found that many drugs with an elevated risk of coagulopathies, such as Mycophenolate Mofetil, Carboplatin, Cytarabine, Nivolumab, Metformin, Rituximab, and Infliximab, were not listed with coagulopathy risks in their instructions. Some drugs, such as Paracetamol, Prednisolone, Dexamethasone, and IbuProfen. In the instructions, only their use will affect the efficacy of oral anticoagulant drugs, but coagulopathies are not mentioned in adverse reactions. Although many drugs have been on the market for a long time, awareness of coagulopathy risks is limited, and data on the prevention and management of drug-induced coagulopathies remain sparse. Monitoring drug-induced coagulopathies is crucial, particularly for cancer and critically ill patients, as it may reduce mortality rates. With the advent of new drugs, especially anti-tumor drugs and immunosuppressants, continuous monitoring of drug-induced coagulopathies will better inform clinicians in making optimal treatment decisions.
This study has several limitations. First, the study established only the association between drugs and adverse events, while lacking definitive proof of the causal relationship between drug exposure and the reported event (Tobaiqy et al., 2010; Jain et al., 2023). Second, the FAERS data was based on spontaneous and voluntary reports, which may be influenced by recent research findings or media coverage (Fan et al., 2024; Jiang et al., 2024). Third, our study did not account for the impact of concomitant drugs and secondary suspect drugs (SS) on adverse reactions. Finally, this study did not assess whether coagulopathy-related adverse reactions are dose-dependent.
In summary, coagulopathies represent severe adverse reactions, with some cases leading to fatal outcomes, particularly among patients with severe illness and cancer. Despite the serious nature of coagulopathies, the risks have not been sufficiently assessed. In this study, we conducted a comprehensive evaluation of drugs related to coagulopathies using the FAERS database and systematically analyzed the top 30 drugs with strong risk signals. For clinical use, we recommend enhanced drug safety monitoring to reduce the risk of coagulopathies when administering these medications.
The original contributions presented in the study are included in the article/Supplementary Material, further inquiries can be directed to the corresponding author.
YL: Data curation, Formal Analysis, Investigation, Methodology, Writing–original draft, Writing–review and editing. QX: Data curation, Investigation, Writing–original draft. SZ: Data curation, Investigation, Writing–original draft.
The author(s) declare that no financial support was received for the research, authorship, and/or publication of this article.
The authors declare that the research was conducted without any commercial or financial relationships that could be construed as a potential conflict of interest.
All claims expressed in this article are solely those of the authors and do not necessarily represent those of their affiliated organizations, or those of the publisher, the editors and the reviewers. Any product that may be evaluated in this article, or claim that may be made by its manufacturer, is not guaranteed or endorsed by the publisher.
The Supplementary Material for this article can be found online at: https://www.frontiersin.org/articles/10.3389/fphar.2024.1486422/full#supplementary-material
Aklilu, A. M., and Shirali, A. C. (2023). Chemotherapy-associated thrombotic microangiopathy. Kidney360 4 (3), 409–422. doi:10.34067/kid.0000000000000061
Alenzi, K. A., Alsuhaibani, D., Batarfi, B., and Alshammari, T. M. (2024). Pancreatitis with use of new diabetic medications: a real-world data study using the post-marketing FDA adverse event reporting system (FAERS) database. Front. Pharmacol. 15, 1364110. doi:10.3389/fphar.2024.1364110
Al-Nouri, Z. L., Reese, J. A., Terrell, D. R., Vesely, S. K., and George, J. N. (2015). Drug-induced thrombotic microangiopathy: a systematic review of published reports. Blood 125 (4), 616–618. doi:10.1182/blood-2014-11-611335
Apte, R. S., Chen, D. S., and Ferrara, N. (2019). VEGF in signaling and disease: beyond discovery and development. Cell 176 (6), 1248–1264. doi:10.1016/j.cell.2019.01.021
Bartoszko, J., and Karkouti, K. (2021). Managing the coagulopathy associated with cardiopulmonary bypass. J. Thrombosis Haemostasis 19 (3), 617–632. doi:10.1111/jth.15195
Bauer, A. T., Gorzelanny, C., Gebhardt, C., Pantel, K., and Schneider, S. W. (2022). Interplay between coagulation and inflammation in cancer: limitations and therapeutic opportunities. Cancer Treat. Rev. 102, 102322. doi:10.1016/j.ctrv.2021.102322
Bhavsar, K. V., Lin, S., Rahimy, E., Joseph, A., Freund, K. B., Sarraf, D., et al. (2016). Acute macular neuroretinopathy: a comprehensive review of the literature. Surv. Ophthalmol. 61 (5), 538–565. doi:10.1016/j.survophthal.2016.03.003
Cassar, K., Bachoo, P., Ford, I., Greaves, M., and Brittenden, J. (2005). Clopidogrel has no effect on D-dimer and thrombin-antithrombin III levels in patients with peripheral arterial disease undergoing peripheral percutaneous transluminal angioplasty. J. Vasc. Surg. 42 (2), 252–258. doi:10.1016/j.jvs.2005.04.027
Chai, P., and Babu, K. (2014). Toxin-induced coagulopathy. Emerg. Med. Clin. N. Am. 32 (1), 53–78. doi:10.1016/j.emc.2013.10.001
Cortina, G., Trojer, R., Waldegger, S., Schneeberger, S., Gut, N., and Hofer, J. (2015). De novo tacrolimus-induced thrombotic microangiopathy in the early stage after renal transplantation successfully treated with conversion to everolimus. Pediatr. Nephrol. 30 (4), 693–697. doi:10.1007/s00467-014-3036-8
Cui, N., Cai, H., Li, Z., Lu, Y., Wang, G., and Lu, A. (2019). Tigecycline-induced coagulopathy: a literature review. Int. J. Clin. Pharm. 41 (6), 1408–1413. doi:10.1007/s11096-019-00912-5
Estrada, C. C., Maldonado, A., and Mallipattu, S. K. (2019). Therapeutic inhibition of VEGF signaling and associated nephrotoxicities. J. Am. Soc. Nephrol. 30 (2), 187–200. doi:10.1681/asn.2018080853
Ettinger, D. S., Wood, D. E., Aisner, D. L., Akerley, W., Bauman, J. R., Bharat, A., et al. (2022). Non–small cell lung cancer, version 3.2022, NCCN clinical practice guidelines in oncology. J. Natl. Compr. Cancer Netw. 20 (5), 497–530. doi:10.6004/jnccn.2022.0025
Evans, S. J. W., Waller, P. C., and Davis, S. (2001). Use of proportional reporting ratios (PRRs) for signal generation from spontaneous adverse drug reaction reports. Pharmacoepidemiol. Drug Saf. 10 (6), 483–486. doi:10.1002/pds.677
Fan, M., Niu, K., Wu, X., and Shi, H. (2024). Risk of drug-induced angioedema: a pharmacovigilance study of FDA adverse event reporting system database. Front. Pharmacol. 15, 1417596. doi:10.3389/fphar.2024.1417596
Gando, S., Levi, M., and Toh, C.-H. (2016). Disseminated intravascular coagulation. Nat. Rev. Dis. Prim. 2 (1), 16037. doi:10.1038/nrdp.2016.37
George, J. N., and Nester, C. M. (2014). Syndromes of thrombotic microangiopathy. N. Engl. J. Med. 371 (7), 654–666. doi:10.1056/NEJMra1312353
Gheorghe, C.-M., Purcărea, V. L., and Gheorghe, I. R. (2019). Assessing the effectiveness of OTC Advertising on artificial tear drops from an experiential marketing perspective. romanian J. Ophthalmol. 63 (3), 297–305. doi:10.22336/rjo.2019.46
Gialeraki, A., Valsami, S., Pittaras, T., Panayiotakopoulos, G., and Politou, M. (2017). Oral contraceptives and HRT risk of thrombosis. Clin. Appl. Thrombosis/Hemostasis 24 (2), 217–225. doi:10.1177/1076029616683802
Gill, R., and Meghrajani, V. (2022). Tacrolimus-induced thrombotic microangiopathy after orthotopic heart transplant: a case report. Cureus 14, e25874. doi:10.7759/cureus.25874
Giustozzi, M., Ehrlinder, H., Bongiovanni, D., Borovac, J. A., Guerreiro, R. A., Gąsecka, A., et al. (2021). Coagulopathy and sepsis: pathophysiology, clinical manifestations and treatment. Blood Rev. 50, 100864. doi:10.1016/j.blre.2021.100864
Gómez-Mesa, J. E., Galindo-Coral, S., Montes, M. C., and Muñoz Martin, A. J. (2021). Thrombosis and coagulopathy in COVID-19. Curr. Problems Cardiol. 46 (3), 100742. doi:10.1016/j.cpcardiol.2020.100742
Grall, M., Daviet, F., Chiche, N. J., Provot, F., Presne, C., Coindre, J.-P., et al. (2021). Eculizumab in gemcitabine-induced thrombotic microangiopathy: experience of the French thrombotic microangiopathies reference centre. BMC Nephrol. 22 (1), 267. doi:10.1186/s12882-021-02470-3
Guo, M., Liang, J., Li, D., Zhao, Y., Xu, W., Wang, L., et al. (2022). Coagulation dysfunction events associated with tigecycline: a real-world study from FDA adverse event reporting system (FAERS) database. Thrombosis J. 20 (1), 12. doi:10.1186/s12959-022-00369-z
Habib, M., Roberts, L. N., Patel, R. K., Wendon, J., Bernal, W., and Arya, R. (2013). Evidence of rebalanced coagulation in acute liver injury and acute liver failure as measured by thrombin generation. Liver Int. 34 (5), 672–678. doi:10.1111/liv.12369
Hakeam, H. A., Al Duhailib, Z., Salahuddin, N., and Amin, T. (2018). Impact of tigecycline versus imipenem–cilastatin on fibrinogen levels following cytoreductive surgery (CRS) and hyperthermic intraperitoneal chemotherapy (HIPEC): a randomized-controlled study. J. Chemother. 30 (4), 224–232. doi:10.1080/1120009x.2018.1452333
Haverinen, A. H., Luiro, K. M., Szanto, T., Kangasniemi, M. H., Hiltunen, L., Sainio, S., et al. (2022). Combined oral contraceptives containing estradiol valerate vs ethinylestradiol on coagulation: a randomized clinical trial. Acta Obstetricia Gynecol. Scand. 101 (10), 1102–1111. doi:10.1111/aogs.14428
Helms, J., Iba, T., Connors, J. M., Gando, S., Levi, M., Meziani, F., et al. (2023). How to manage coagulopathies in critically ill patients. Intensive Care Med. 49 (3), 273–290. doi:10.1007/s00134-023-06980-6
Iams, W., Beckermann, K. E., Neff, A. T., Mayer, I. A., and Abramson, V. G. (2013). Thrombotic microangiopathy during docetaxel, trastuzumab, and carboplatin chemotherapy for early-stage HER2+ breast cancer: a case report. Med. Oncol. 30 (2), 568. doi:10.1007/s12032-013-0568-x
Iba, T., and Levy, J. H. (2020). Sepsis-induced coagulopathy and disseminated intravascular coagulation. Anesthesiology 132 (5), 1238–1245. doi:10.1097/aln.0000000000003122
Iba, T., Levy, J. H., Thachil, J., Wada, H., and Levi, M.Scientific and Standardization Committee on DIC of the International Society on Thrombosis and Haemostasis (2019a). The progression from coagulopathy to disseminated intravascular coagulation in representative underlying diseases. Thrombosis Res. 179, 11–14. doi:10.1016/j.thromres.2019.04.030
Iba, T., Levy, J. H., Warkentin, T. E., Thachil, J., van der Poll, T., Levi, M., et al. (2019b). Diagnosis and management of sepsis-induced coagulopathy and disseminated intravascular coagulation. J. Thrombosis Haemostasis 17 (11), 1989–1994. doi:10.1111/jth.14578
Ishikawa, M., Nakayama, K., Razia, S., Ishida, A., Yamashita, H., Ishibashi, T., et al. (2021). Neutropenic enterocolitis-induced sepsis and disseminated intravascular coagulation after chemotherapy: a case report. BMC Women's Health 21 (1), 187. doi:10.1186/s12905-021-01302-8
Izzedine, H., and Perazella, M. A. (2015). Thrombotic microangiopathy, cancer, and cancer drugs. Am. J. Kidney Dis. 66 (5), 857–868. doi:10.1053/j.ajkd.2015.02.340
Jain, D., Sharma, G., and Kumar, A. (2023). Adverse effects of proton pump inhibitors (PPIs) on the renal system using data mining algorithms (DMAs). Expert Opin. Drug Saf. 22 (8), 741–752. doi:10.1080/14740338.2023.2189698
Javed, F., and Kumar, A. (2024). Identification of signal of clindamycin associated renal failure acute: a disproportionality analysis. Curr. Drug Saf. 19 (1), 123–128. doi:10.2174/1574886318666230228142856
Jiang, T., Su, H., Xu, J., Li, C., Zhang, N., Li, Y., et al. (2024). Drug-induced interstitial lung disease: a real-world pharmacovigilance study of the FDA Adverse Event Reporting System from 2004 to 2021. Ther. Adv. Drug Saf. 15, 20420986231224227. doi:10.1177/20420986231224227
Jodele, S., Laskin, B. L., Dandoy, C. E., Myers, K. C., El-Bietar, J., Davies, S. M., et al. (2015). A new paradigm: diagnosis and management of HSCT-associated thrombotic microangiopathy as multi-system endothelial injury. Blood Rev. 29 (3), 191–204. doi:10.1016/j.blre.2014.11.001
Kleinveld, D. J. B., Hamada, S. R., and Sandroni, C. (2022). Trauma-induced coagulopathy. Intensive Care Med. 48 (11), 1642–1645. doi:10.1007/s00134-022-06834-7
Kubota, K., Koide, D., and Hirai, T. (2004). Comparison of data mining methodologies using Japanese spontaneous reports. Pharmacoepidemiol. Drug Saf. 13 (6), 387–394. doi:10.1002/pds.964
Kumar, R., Vidaurre, J., and Gedela, S. (2019). Valproic acid–induced coagulopathy. Pediatr. Neurol. 98, 25–30. doi:10.1016/j.pediatrneurol.2019.04.019
Larson, A. M., Polson, J., Fontana, R. J., Davern, T. J., Lalani, E., Hynan, L. S., et al. (2005). Acetaminophen-induced acute liver failure: results of a United States multicenter, prospective study. Hepatology 42 (6), 1364–1372. doi:10.1002/hep.20948
Lechner, K., and Obermeier, H. L. (2012). Cancer-related microangiopathic hemolytic anemia: clinical and laboratory features in 168 reported cases. Medicine 91 (4), 195–205. doi:10.1097/MD.0b013e3182603598
Levi, M. (2014). Cancer-related coagulopathies. Thrombosis Res. 133, S70–S75. doi:10.1016/s0049-3848(14)50012-6
Li, D., Wang, H., Qin, C., Du, D., Wang, Y., Du, Q., et al. (2024). Drug-induced acute pancreatitis: a real-world pharmacovigilance study using the FDA adverse event reporting system database. Clin. Pharmacol. and Ther. 115 (3), 535–544. doi:10.1002/cpt.3139
Li, Y., Li, H., Wang, Y., Guo, J., and Zhang, D. (2023). Potential biomarkers for early diagnosis, evaluation, and prognosis of sepsis-induced coagulopathy. Clin. Appl. Thrombosis/Hemostasis 29, 10760296231195089. doi:10.1177/10760296231195089
Liu, M., Gu, L., Zhang, Y., Zhou, H., Wang, Y., and Xu, Z.-X. (2024). A real-world disproportionality analysis of mesalazine data mining of the public version of FDA adverse event reporting system. Front. Pharmacol. 15, 1290975. doi:10.3389/fphar.2024.1290975
Lockhart, E., Peterson-Layne, C., Ahmadzia, H., Grotegut, C., and James, A. (2016). Management of coagulopathy in postpartum hemorrhage. Seminars Thrombosis Hemostasis 42 (07), 724–731. doi:10.1055/s-0036-1593417
Markan, A., Ayyadurai, N., and Singh, R. (2021). Tacrolimus induced thrombotic microangiopathy (TMA) presenting as acute macular neuroretinopathy. Ocular Immunol. Inflamm. 31 (1), 231–232. doi:10.1080/09273948.2021.1998549
Mini, E., Nobili, S., Caciagli, B., Landini, I., and Mazzei, T. (2006). Cellular pharmacology of gemcitabine. Ann. Oncol. 17, v7–v12. doi:10.1093/annonc/mdj941
Moore, H. B., Gando, S., Iba, T., Kim, P. Y., Yeh, C. H., Brohi, K., et al. (2020). Defining trauma-induced coagulopathy with respect to future implications for patient management: communication from the SSC of the ISTH. J. Thrombosis Haemostasis 18 (3), 740–747. doi:10.1111/jth.14690
Noguchi, Y., Katsuno, H., Ueno, A., Otsubo, M., Yoshida, A., Kanematsu, Y., et al. (2018). Signals of gastroesophageal reflux disease caused by incretin-based drugs: a disproportionality analysis using the Japanese adverse drug event report database. J. Pharm. Health Care Sci. 4 (1), 15. doi:10.1186/s40780-018-0109-z
Novak, J., Mocikova, H., Pavlicek, P., Gaherova, L., and Kozak, T. (2012). Rituximab-induced coagulopathy. Leukemia and Lymphoma 53 (11), 2299–2301. doi:10.3109/10428194.2012.682313
Nwaba, A., MacQuillan, G., Adams, L. A., Garas, G., Delriviere, L., Augustson, B., et al. (2013). Tacrolimus-induced thrombotic microangiopathy in orthotopic liver transplant patients: case series of four patients. Intern. Med. J. 43 (3), 328–333. doi:10.1111/imj.12048
Pandit, B., and Royzen, M. (2022). Recent development of prodrugs of gemcitabine. Genes 13 (3), 466. doi:10.3390/genes13030466
Peralta, R., Thani, H. A., and Rizoli, S. (2019). Coagulopathy in the surgical patient: trauma-induced and drug-induced coagulopathies. Curr. Opin. Crit. Care 25 (6), 668–674. doi:10.1097/mcc.0000000000000676
Petros, S. (2019). Trauma-induced coagulopathy. Hämostaseologie 39 (01), 020–027. doi:10.1055/s-0039-1677853
Pham, P.-T. T., Peng, A., Wilkinson, A. H., Gritsch, H. A., Lassman, C., Pham, P.-C. T., et al. (2000). Cyclosporine and tacrolimus–associated thrombotic microangiopathy. Am. J. Kidney Dis. 36 (4), 844–850. doi:10.1053/ajkd.2000.17690
Poluzzi, E., Raschi, E., Moretti, U., and De Ponti, F. (2009). Drug-induced torsades de pointes: data mining of the public version of the FDA Adverse Event Reporting System (AERS). Pharmacoepidemiol. Drug Saf. 18 (6), 512–518. doi:10.1002/pds.1746
Porter, L. H., Zhu, J. J., Lister, N. L., Harrison, S. G., Keerthikumar, S., Goode, D. L., et al. (2023). Low-dose carboplatin modifies the tumor microenvironment to augment CAR T cell efficacy in human prostate cancer models. Nat. Commun. 14 (1), 5346. doi:10.1038/s41467-023-40852-3
Raschi, E., Gatti, M., Gelsomino, F., Ardizzoni, A., Poluzzi, E., and De Ponti, F. (2020a). Lessons to be learnt from real-world studies on immune-related adverse events with checkpoint inhibitors: a clinical perspective from pharmacovigilance. Target. Oncol. 15 (4), 449–466. doi:10.1007/s11523-020-00738-6
Raschi, E., La Placa, M., Poluzzi, E., and De Ponti, F. (2020b). The value of case reports and spontaneous reporting systems for pharmacovigilance and clinical practice. Br. J. Dermatology 184 (3), 581–583. doi:10.1111/bjd.19677
Raschi, E., Mazzarella, A., Antonazzo, I. C., Bendinelli, N., Forcesi, E., Tuccori, M., et al. (2019). Toxicities with immune checkpoint inhibitors: emerging priorities from disproportionality analysis of the FDA adverse event reporting system. Target. Oncol. 14 (2), 205–221. doi:10.1007/s11523-019-00632-w
Rothman, K. J., Lanes, S., and Sacks, S. T. (2004). The reporting odds ratio and its advantages over the proportional reporting ratio. Pharmacoepidemiol. Drug Saf. 13 (8), 519–523. doi:10.1002/pds.1001
Routsi, C., Kokkoris, S., Douka, E., Ekonomidou, F., Karaiskos, I., and Giamarellou, H. (2015). High-dose tigecycline-associated alterations in coagulation parameters in critically ill patients with severe infections. Int. J. Antimicrob. Agents 45 (1), 90–93. doi:10.1016/j.ijantimicag.2014.07.014
Sakaeda, T., Tamon, A., Kadoyama, K., and Okuno, Y. (2013). Data mining of the public version of the FDA adverse event reporting system. Int. J. Med. Sci. 10 (7), 796–803. doi:10.7150/ijms.6048
Salhi, S., Ribes, D., and Faguer, S. (2021). Complement C5 inhibition reverses bleomycin-induced thrombotic microangiopathy. Clin. Kidney J. 14 (4), 1275–1276. doi:10.1093/ckj/sfaa101
Sánchez-Sánchez, E., Fernández-Cerezo, F. L., Díaz-Jimenez, J., Rosety-Rodriguez, M., Díaz, A. J., Ordonez, F. J., et al. (2021). Consumption of over-the-Counter drugs: prevalence and type of drugs. Int. J. Environ. Res. Public Health 18 (11), 5530. doi:10.3390/ijerph18115530
Sharma, A., and Kumar, A. (2022). Identification of novel signal of clobazam-associated drug reaction with eosinophilia and systemic symptoms syndrome: a disproportionality analysis. Acta Neurol. Scand. 146 (5), 623–627. doi:10.1111/ane.13690
Sharma, A., Roy, S., Sharma, R., and Kumar, A. (2023). Association of antiviral drugs and their possible mechanisms with DRESS syndrome using data mining algorithms. J. Med. Virology 95 (3), e28671. doi:10.1002/jmv.28671
Song, J.-C., Wang, G., Zhang, W., Zhang, Y., Li, W.-Q., Zhou, Z., et al. (2020). Chinese expert consensus on diagnosis and treatment of coagulation dysfunction in COVID-19. Mil. Med. Res. 7 (1), 19. doi:10.1186/s40779-020-00247-7
Stensballe, J., Henriksen, H. H., and Johansson, P. I. (2017). Early haemorrhage control and management of trauma-induced coagulopathy: the importance of goal-directed therapy. Curr. Opin. Crit. Care 23 (6), 503–510. doi:10.1097/mcc.0000000000000466
Subeesh, V., Singh, H., Maheswari, E., and Beulah, E. (2017). Novel adverse events of vortioxetine: a disproportionality analysis in USFDA adverse event reporting system database. Asian J. Psychiatry 30, 152–156. doi:10.1016/j.ajp.2017.09.005
Tan, E., Braithwaite, I., McKinlay, C. J. D., and Dalziel, S. R. (2020). Comparison of acetaminophen (paracetamol) with ibuprofen for treatment of fever or pain in children younger than 2 Years: a systematic review and meta-analysis. JAMA Netw. Open 3 (10), e2022398. doi:10.1001/jamanetworkopen.2020.22398
Tang, S., Wu, Z., Xu, L., Wen, Q., and Zhang, X. (2022). Adverse reaction signals mining and hemorrhagic signals comparison of ticagrelor and clopidogrel: a pharmacovigilance study based on FAERS. Front. Pharmacol. 13, 970066. doi:10.3389/fphar.2022.970066
Teal, S., and Edelman, A. (2021). Contraception selection, effectiveness, and adverse effects: a review. Jama 326 (24), 2507–2518. doi:10.1001/jama.2021.21392
Tobaiqy, M., Stewart, D., Helms, P. J., Bond, C., Lee, A. J., Bateman, N., et al. (2010). A pilot study to evaluate a community pharmacy–based monitoring system to identify adverse drug reactions associated with paediatric medicines use. Eur. J. Clin. Pharmacol. 66 (6), 627–632. doi:10.1007/s00228-010-0790-9
Treml, B., Rajsic, S., Hell, T., Fries, D., and Bachler, M. (2021). Progression of fibrinogen decrease during high dose tigecycline therapy in critically ill patients: a retrospective analysis. J. Clin. Med. 10 (20), 4702. doi:10.3390/jcm10204702
van der Heijden, M. S., Sonpavde, G., Powles, T., Necchi, A., Burotto, M., Schenker, M., et al. (2023). Nivolumab plus gemcitabine–cisplatin in advanced urothelial carcinoma. N. Engl. J. Med. 389 (19), 1778–1789. doi:10.1056/NEJMoa2309863
Watson, H. G. (2007). Sex hormones and thrombosis. Seminars Hematol. 44 (2), 98–105. doi:10.1053/j.seminhematol.2007.01.004
Weill, A., Dalichampt, M., Raguideau, F., Ricordeau, P., Blotière, P.-O., Rudant, J., et al. (2016). Low dose oestrogen combined oral contraception and risk of pulmonary embolism, stroke, and myocardial infarction in five million French women: cohort study. Bmj 353, i2002. doi:10.1136/bmj.i2002
Wu, S., Wu, G., and Wu, H. (2021). A comparison of coagulation function in patients receiving aspirin and cefoperazone-sulbactam with and without vitamin K1: a retrospective, observational study. Clin. Ther. 43 (12), e335–e345. doi:10.1016/j.clinthera.2021.10.005
Xiao, Q., Ungerer, M., Li, Z., Baumgartner, C., Goebel, S., Vogelmann, J., et al. (2013). The GPVI – Fc fusion protein revacept reduces thrombus formation and improves vascular dysfunction in atherosclerosis without any impact on bleeding times. PLoS ONE 8 (8), e71193. doi:10.1371/journal.pone.0071193
Yang, Z., Lv, Y., Yu, M., Mei, M., Xiang, L., Zhao, S., et al. (2022). GLP-1 receptor agonist-associated tumor adverse events: a real-world study from 2004 to 2021 based on FAERS. Front. Pharmacol. 13, 925377. doi:10.3389/fphar.2022.925377
Yousaf, A., Hagen, R., Delaney, E., Davis, S., and Zinn, Z. (2020). The influence of social media on acne treatment: a cross-sectional survey. Pediatr. Dermatol. 37 (2), 301–304. doi:10.1111/pde.14091
Yu, R. J., Krantz, M. S., Phillips, E. J., and Stone, C. A. (2021). Emerging causes of drug-induced anaphylaxis: a review of anaphylaxis-associated reports in the FDA adverse event reporting system (FAERS). J. Allergy Clin. Immunol. Pract. 9 (2), 819–829.e2. doi:10.1016/j.jaip.2020.09.021
Zhang, L., Mao, W., Liu, D., Hu, B., Lin, X., Ran, J., et al. (2023). Risk factors for drug-related acute pancreatitis: an analysis of the FDA adverse event reporting system (FAERS). Front. Pharmacol. 14, 1231320. doi:10.3389/fphar.2023.1231320
Zhang, X., Ren, X., Zhu, T., Zheng, W., Shen, C., and Lu, C. (2024). A real-world pharmacovigilance study of FDA adverse event reporting system (FAERS) events for sunitinib. Front. Pharmacol. 15, 1407709. doi:10.3389/fphar.2024.1407709
Zhao, Q.-Y., Liu, L.-P., Luo, J.-C., Luo, Y.-W., Wang, H., Zhang, Y.-J., et al. (2021). A machine-learning approach for dynamic prediction of sepsis-induced coagulopathy in critically ill patients with sepsis. Front. Med. 7, 637434. doi:10.3389/fmed.2020.637434
Zhou, Q., Du, Z., Qu, K., Shen, Y., Jiang, Y., Zhu, H., et al. (2023). Adverse events of epidiolex: a real-world drug safety surveillance study based on the FDA adverse event reporting system (FAERS) database. Asian J. Psychiatry 90, 103828. doi:10.1016/j.ajp.2023.103828
Zou, F., Zhu, C., Lou, S., Cui, Z., Wang, D., Ou, Y., et al. (2023). A real-world pharmacovigilance study of mepolizumab in the FDA adverse event reporting system (FAERS) database. Front. Pharmacol. 14, 1320458. doi:10.3389/fphar.2023.1320458
Keywords: coagulopathies, FAERS database, adverse event, data mining, disproportionality analyses, pharmacovigilance
Citation: Lu Y, Xu Q and Zhu S (2024) Drug-induced coagulopathies: a real-world pharmacovigilance study using the FDA adverse event reporting system. Front. Pharmacol. 15:1486422. doi: 10.3389/fphar.2024.1486422
Received: 26 August 2024; Accepted: 05 December 2024;
Published: 18 December 2024.
Edited by:
Linan Zeng, McMaster University, CanadaReviewed by:
Mamunur Rashid, University of Nebraska Medical Center, United StatesCopyright © 2024 Lu, Xu and Zhu. This is an open-access article distributed under the terms of the Creative Commons Attribution License (CC BY). The use, distribution or reproduction in other forums is permitted, provided the original author(s) and the copyright owner(s) are credited and that the original publication in this journal is cited, in accordance with accepted academic practice. No use, distribution or reproduction is permitted which does not comply with these terms.
*Correspondence: Yanjun Lu, MTM3OTI4MzA5MTZAMTYzLmNvbQ==
Disclaimer: All claims expressed in this article are solely those of the authors and do not necessarily represent those of their affiliated organizations, or those of the publisher, the editors and the reviewers. Any product that may be evaluated in this article or claim that may be made by its manufacturer is not guaranteed or endorsed by the publisher.
Research integrity at Frontiers
Learn more about the work of our research integrity team to safeguard the quality of each article we publish.