- 1Biobank, Shenzhen Second People’s Hospital, First Affiliated Hospital of Shenzhen University, Shenzhen, China
- 2Guangdong Key Laboratory for Biomedical Measurements and Ultrasound Imaging, National-Regional Key Technology Engineering Laboratory for Medical Ultrasound, School of Biomedical Engineering, Shenzhen University Medical School, Shenzhen, China
- 3Food and Nutritional Sciences, School of Biological Sciences, The University of Hong Kong, Pokfulam, Hong Kong SAR, China
- 4Hengyang Medical School, University of South China, Hengyang, Hunan, China
- 5Plant Science, School of Biological Sciences, The University of Hong Kong, Pokfulam, Hong Kong SAR, China
- 6Eastern Institute for Advanced Study, Eastern Institute of Technology, Ningbo, Zhejiang, China
Breast cancer is the most frequently diagnosed and fatal cancer among women worldwide. Dairy protein-derived peptides and dairy products are important parts of the daily human diet and have shown promising activities in suppressing the proliferation, migration, and invasion of breast cancer cells, both in vitro and in vivo. Most of the review literature employs meta-analysis methods to explore the association between dairy intake and breast cancer risk. However, there is a lack of comprehensive summary regarding the anti-breast cancer properties of dairy protein-derived peptides, dairy products, and dairy protein-based nanoparticles as well as their underlying mechanisms of action. Therefore, the present study discussed the breast cancer inhibitory effects and mechanisms of active peptides derived from various dairy protein sources. Additionally, the characteristics, anti-breast cancer activities and active components of several types of dairy products, including fermented milk, yogurt and cheeses, were summarized. Furthermore, the preparation methods and therapeutic effects of various dairy protein-containing nanoparticle delivery systems for breast cancer therapy were briefly described. Lastly, this work also provided an overview of what is currently known about the anti-breast cancer effects of dairy products in clinical studies. Our review will be of interest to the development of natural anticancer drugs.
1 Introduction
Breast cancer represents a significant global health challenge, posing a major obstacle to the survival of patients and the augmentation of life expectancy worldwide (Chaudhari et al., 2024). In 2020, over 2.3 million women were diagnosed with breast cancer, resulting in 685,000 deaths (Arafat et al., 2023). Current treatments for breast cancer have considerable drawbacks. For instance, surgical methods may cause severe pain and compromise a woman’s physical integrity; radiation therapy may damage healthy cells and can thus lead to debilitation and shorter life expectancy; chemotherapy is not only cardiotoxic but also lead to drug resistance when administered for a long period, making breast cancer treatment particularly challenging (Anagnostopoulos and Myrgianni, 2009; Gärtner et al., 2009; Marquette and Nabell, 2012; Farhood et al., 2020; Wang and Tepper, 2021; Zhang T. Y. et al., 2023). Therefore, new approaches, especially those with minimal side effects, affordable costs, and high patient compliance, need to be developed urgently to cater to the expectations of patients undergoing breast cancer treatment and to reduce their economic and psychological burden.
The etiology of breast cancer is multifaceted, including a complex interplay of hereditary, dietary and environmental risk factors (Taleb et al., 2017). As suggested by several scholars, genetic determinants are responsible for 30%–40% of cancer cases, with the remaining 60%–70% ascribed to non-genetic environmental and lifestyle factors, including diet, exercise, obesity, geographic location, etc. (Keum and Giovannucci, 2019; Xiao et al., 2023). Among them, diet is one of the most modifiable aspects of lifestyle and is considered an effective strategy for breast cancer control (Arafat et al., 2023). There is substantial evidence suggesting that several food components, such as polysaccharides, milk proteins, polyphenols, and active peptides can markedly reduce the risk of breast cancer (Gu et al., 2020; Shin et al., 2020; Corso et al., 2021; Jabbari et al., 2022).
Dairy products are widely consumed across the globe and also an important part of dietary guidelines in various countries. The American Dietary Guidelines recommend that children and adolescents consume 2-3 cups of dairy products per day, the Chinese Dietary Guidelines suggest a milk intake of 300 g/day, and the French Dietary Guidelines recommend 2-3 servings of dairy products per day (Cámara et al., 2021). Furthermore, dairy products supply multiple nutrients for human metabolism, including macronutrients such as proteins and lipids, and micronutrients such as calcium, phosphorus, vitamin A, vitamin B, and riboflavin (Leischner et al., 2021). This comprehensive nutrient profile has attracted scholars to investigate the health benefits of dairy products.
Some studies have explored the association between dairy products and breast cancer risk. A case study involving 1,699 women showed that high consumption of dairy products among premenopausal women was linked to a reduced risk of breast cancer (Wajszczyk et al., 2021). Moreover, El-Din et al. reported that L. kefiri P-B1-fermented kefir significantly reduced tumor volume, enhanced breast cancer cell apoptosis and induced cell cycle arrest at G0/G1 phase in female Swiss albino mice injected with breast cancer cells (El-Din et al., 2020). These findings reveal a connection between dairy product intake and breast cancer progression. More than that, as shown in Figure 1A, research on breast cancer and dairy products has been on the rise over the past 25 years, according to the data retrieved from the Web of Science. In addition, the “Nutrition Dietetics,” “Oncology”, and “Food Science Technology” categories occupy a dominant role in the study of dairy products for breast cancer treatment (Figure 1B). The above evidence suggests that the importance of dairy products in the treatment of breast cancer is increasing, with numerous basic experimental and clinical studies demonstrating their positive effects. However, a review of recent progress in this field is still lacking.
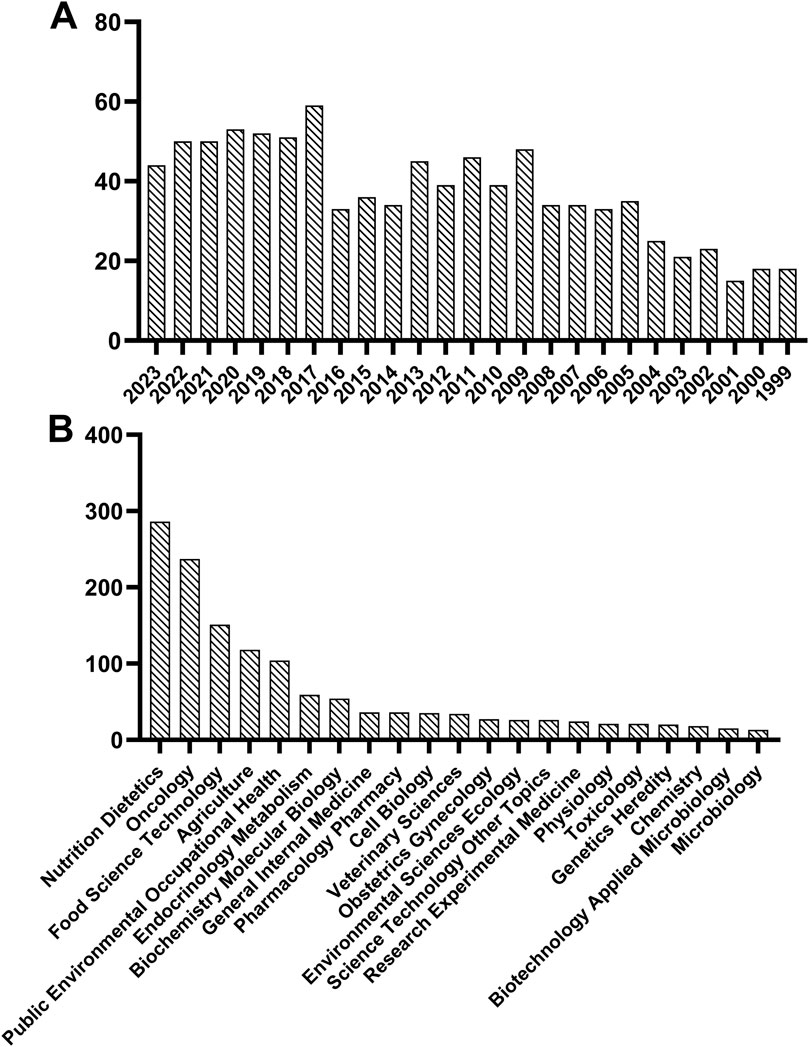
Figure 1. Statistics on dairy and breast cancer in web of science based on publication year (A) and research direction (B).
To clarify the great promise of dairy protein-related products for breast cancer treatment, the following work was undertaken (Figure 2). First, we provided an overview of the sources, processing methods, peptide sequences and anti-breast cancer effects of different dairy protein-derived peptides and introduced the underlying mechanisms by which they exhibited anticancer effects. Second, we summarized the characteristics and anti-breast cancer effects of milk proteins, fermented milk, yogurt and cheese. Third, various nanoparticle delivery systems containing dairy proteins or dairy peptides, which were designed for breast cancer administration, were included in this study. Fourth, clinical effects of dairy peptides, dairy proteins and dairy products on breast cancer treatment were also discussed in detail. Our study will be of great interest to the deep processing and clinical application of dairy proteins and dairy products.
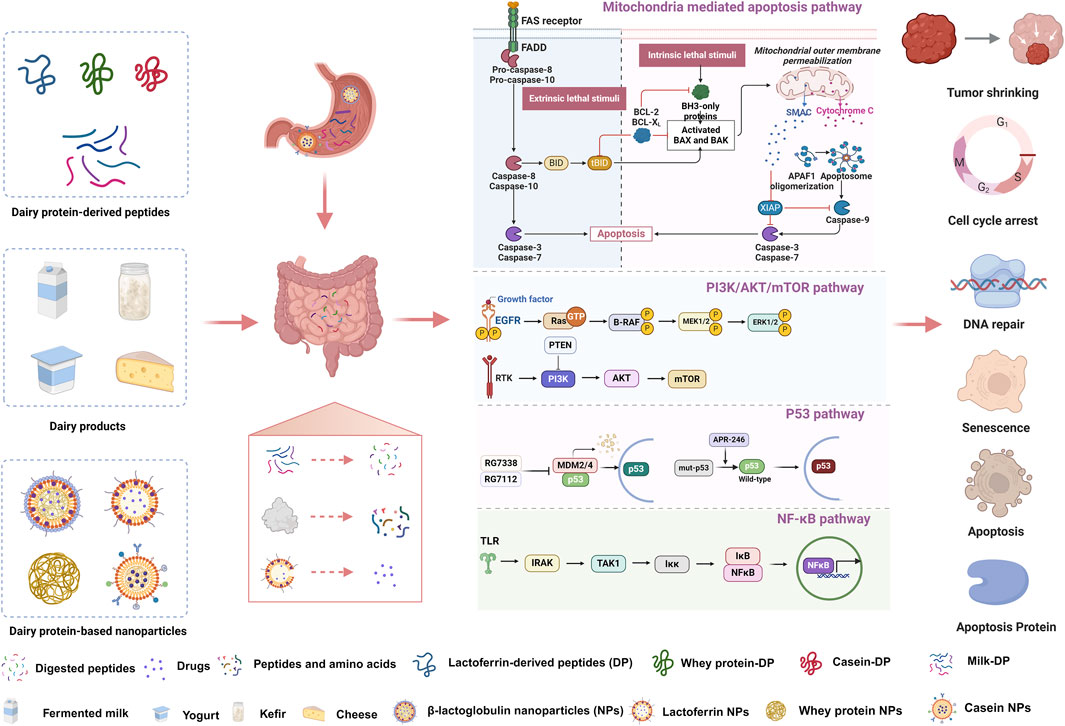
Figure 2. Anti-breast cancer effects and mechanisms of dairy peptides, dairy products and dairy protein-based nanoparticles.
2 Anti-breast cancer peptides derived from dairy protein
Milk is naturally a whole food, composed of 87% water, 4% lipids and 9% water-soluble compounds (proteins, lactose, calcium, vitamin D, etc.) (Auestad and Layman, 2021). More importantly, milk is easily digestible and rich in nine essential amino acids, making it an important source of highly bioactive peptides. Consequently, the major protein constituents of milk, α-S1-casein, α-S2-casein, β-casein, κ-casein, the whey proteins α-lactalbumin, lactoferrin (LF), and β-lactoglobulin, have been widely used for the extraction of bioactive peptides.
2.1 Lactoferrin-derived anti-breast cancer peptides
LF, which contains approximately 690 amino acid residues and has a molecular weight of approximately 80 kDa, is a functional glycoprotein first isolated from cow’s milk by Sorensen in 1939 and first separated from human breast milk by Sorensen and Sorensen (1939), Johanson et al. (1960), Zhang Y. Q. et al. (2021). Typically, LF is odorless, readily soluble in water, and exhibits a certain level of structural stability. It can withstand temperatures of 56°C for several hours but is rapidly inactivated at temperatures above 80°C (Kowalczyk et al., 2022). LF consists of two homologous globular lobes, where a carboxyl (C) and an amino (N) end are linked by an α-helix. Each lobe contains two structural domains, designated as C1, C2, N1, and N2, and they form a β-sheet (Gruden and Ulrih, 2021). The structure of LF makes it highly flexible and can form different variants after transcription, translation, and modification (phosphorylation, acetylation, lipidation, ubiquitination, or glycosylation, etc.). This enables it not only to be serve as a polymeric material, but also to exhibit multiple biological activities in vivo, such as tumor growth inhibition, and antibacterial, free radical scavenging, antiviral, and anti-inflammatory properties (Gruden and Ulrih, 2021). In addition, LF has a high affinity for trivalent iron (KD ∼ 10–20M), allowing it to regulate free iron in body fluids and perform multiple functions as an iron-binding glycoproteins (Zhang Y. Q. et al., 2021). LF with different iron saturation levels exhibits different biological functions. For instance, LF with higher iron content is thought to have antiviral capacity and bacteriostatic activity against both Gram-positive and Gram-negative bacteria, whereas LF with lower iron saturation could bind more iron, thereby reducing free radical damage caused by iron overload and exhibiting antioxidant capacity (Gruden and Ulrih, 2021; Zhang Y. Q. et al., 2021). Lactoferrin has a long history of dietary use and is classified as a Generally Recognized as Safe (GRAS) nutritional supplement by the U.S. Food and Drug Administration (Wakabayashi et al., 2006). Extensive studies in both animals and humans have shown that even in vulnerable populations with compromised immune systems or disease states, daily doses of lactoferrin ranging from 1.5 to 15 g for periods of 1 day–42 weeks have not demonstrated any significant toxicity-related outcomes in safety or tolerability assessments (Wakabayashi et al., 2006; Vishwanath-Deutsch et al., 2024). In recent years, as the relationship between dairy consumption and breast cancer risk reduction has become clearer, several scholars have also expressed interest in investigating the anti-breast cancer effects of LF-activated peptides.
Several possible anti-breast cancer peptides have been identified in LF such as FKCRRWQWRMKKLGAPSITCVRRAF, RRWQWRMKKLG, RRWQWR, RRWQWRMKKLG, FKARRWQWRMKKLGA, GEQELRKCNQWSGLSEGSVT, WSGLSEGSVTCSSASTTEDC, and RWQWRWQWR (Table 1). These LF-peptides inhibit the growth, adherence, and migration of breast cancer cells, promote cell death, induce cell cycle arrest, and lead to the overexpression of apoptosis-associated proteins, including caspase-3, -7, -8, and -9, thus hindering breast cancer progression. For instance, Fatemi et al. separated three peptides from human LF, namely GEQELRKCNQWSGLSEGSVT, WSGLSEGSVTCSSASTTEDC, and CSSASTTEDCIALVLKGEAD. The latter two peptides exhibited very strong cytotoxicity in MCF-7 and MDA-MB-231 cells, with 50% cytotoxic concentration values of 100 μg/mL and 100 μg/mL, respectively, in MCF-7 and 950 μg/mL and 1,000 μg/mL, respectively, in MDA-MB-231 cells (Fatemi et al., 2018). However, this study primarily employed computerized techniques to identify the active peptide fractions in human LF and preliminarily measured their anti-breast cancer activity, without investigating their anti-metastatic and invasive capabilities or the action mechanisms of these active peptides. Rahman et al. discovered that FKCRRWQWRMKKLGAPSITCVRRAF (derived from bovine LF (BLF)) exhibited a pro-apoptotic effect on breast cancer cells, leading to decreased viability in four breast cancer cells, MDA-MB-231, MDA-MB-468, SKBR3 and MCF7, as demonstrated in the MTT assay (Rahman et al., 2021). In addition, this study also found that FKCRRWQWRMKKLGAPSITCVRRAF reduced the invasion of breast cancer tumors and limited tumor growth in mice. Similar findings were obtained by Furlong et al. and Zhang et al., who stated that FKCRRWQWRMKKLGAPSITCVRRAF can promote apoptosis, increase DNA fragmentation, cause morphological changes and cause cell cycle arrest at the G2 phase in breast cancer cells. These effects were attributed to the downregulation of the mTOR signaling pathway and the upregulation of the AMPK signaling pathway (Furlong et al., 2006; Zhang et al., 2014). However, although the obtained peptides have excellent antitumor activity, their sequences are too long, and whether they can tolerate gastrointestinal digestion and be absorbed by the human body needs to be examined by in vitro gastrointestinal simulation or in vivo digestion experiments. Some short-chain anti-breast cancer peptides have also been obtained by researchers. Insuasty-Cepeda et al., Casanova et al. and Guerra et al. isolated RRWQWRMKKLG and RRWQWR from BLF, both of which led to decreased viability, increased apoptosis, and morphological changes in breast cancer cells (Casanova et al., 2017; Guerra et al., 2019; Insuasty-Cepeda et al., 2020). Additionally, the two peptides also caused mitochondrial membrane depolarization and cellular calcium overload in breast cancer cells, suggesting the activation of the mitochondria-mediated apoptosis pathway. These active peptides are relatively short in length and derived from the longer peptide FKCRRWQWRMKKLGAPSITCVRRAF, which implies that this sequence is critical for the biological activity of LF. Overall, current research on the anti-breast cancer mechanisms of LF-activated peptides is not deep enough, and more effort needs to be invested in this area in the future.
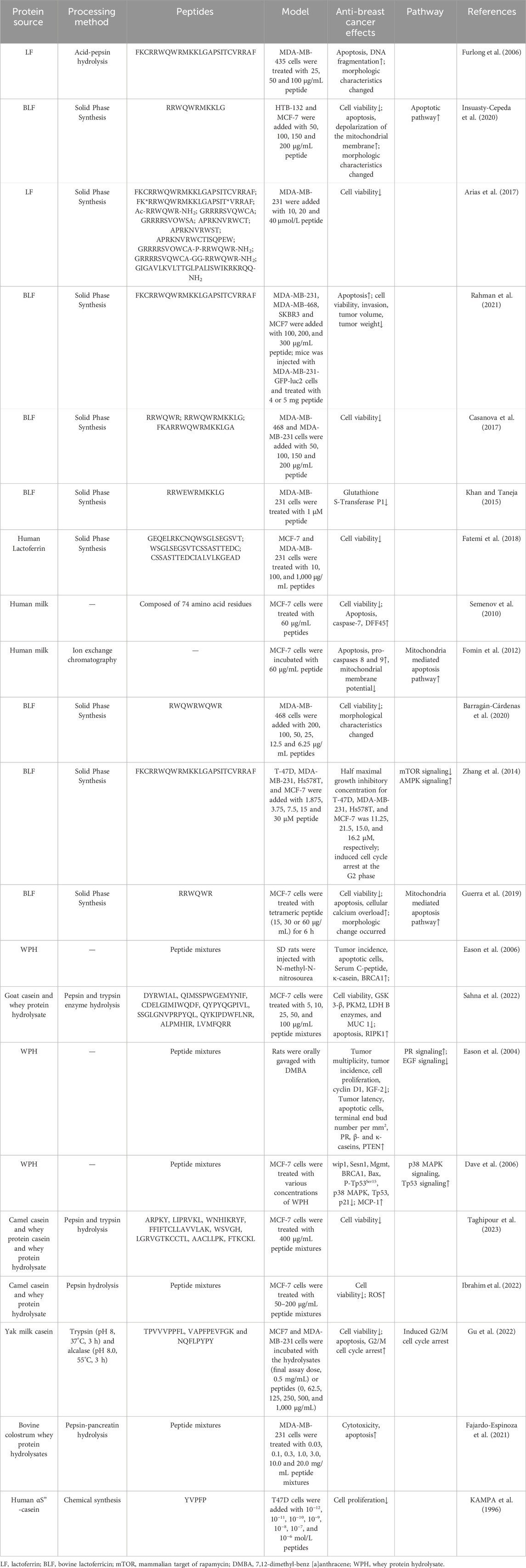
Table 1. Inhibitory effects and mechanisms of dairy-derived active peptides and peptide extracts on breast cancer progression.
2.2 Whey protein-derived anti-breast cancer peptides
Whey is a by-product of cheese manufacturing or other coagulated dairy product processing, containing 0.6% protein and 93% water, with a yellowish-green color (Zhang, 2022). Previously, whey separated from cheese production was considered a worthless by-product of the dairy industry and was difficult to process into other foods or sell as a food additive (Mehra et al., 2021). In recent years, liquid whey has gained attention for its mineral-rich, safe, easily digestible, and highly nutritious whey protein, resulting in increased effective utilization and conversion into various valuable human food supplements (Mehra et al., 2021). Whey can be converted into whey protein hydrolysate (WPH), whey protein concentrate (WPC), whey protein powder (WPP), whey protein isolate (WPI), and other metabolites using complex processing techniques including fermentation, membrane separation, chromatographic separation, and ultrafiltration (Zeng et al., 2024). These dry whey protein components consist of numerous subfractions such as β-lactoglobulin, α-lactalbumin, immunoglobulins, bovine serum albumin, proteose peptone, etc. (Zhang, 2022). In detail, WPP contains 63%–75% protein and 11.0%–14.5% lactose and its processing involves demineralization, acid, sweetening, and other reduced forms (Mehra et al., 2021). WPC has a protein content ranging from 25% to 80% and is a good source of lysine and sulfur-containing amino acids (Mehra et al., 2021; Zhang, 2022). WPI has the highest protein content (more than 90%), and generally undergoes an additional purification step to remove lactose and fat, making it popular among fitness enthusiasts (Chen et al., 2023). Interestingly, when WPP, WPC, and WPI are treated with acids, enzymes, or heat, the intact protein breaks down into polypeptides, smaller peptides, and amino acids, resulting in WPH (Mehra et al., 2021). Various whey proteins and WPH provide high-quality protein that can enhance athletic performance without toxic effects (Mangano et al., 2019; Zhao and Ashaolu, 2020). Specifically, whey protein, whey protein-derived peptides, and WPH are considered to have no adverse effects on body weight, food intake, behavior, organ weight, activity levels, or blood and urine parameters. They do not lead to abnormal changes in overall health, biochemical indicators, histopathological findings, or other observational metrics, nor do they exhibit acute or subacute toxicity (Zhao and Ashaolu, 2020). However, some studies suggest that excessive intake of certain bioactive peptides from whey protein may cause adverse reactions or allergies, though these anomalies can be mitigated through extensive hydrolysis (Cui et al., 2023). At present, scholars have isolated a large number of peptides from WPH, such as antioxidant peptides, anti-cancer peptides, ACE inhibitory peptides, anti-diabetic peptides, and immune-enhancing peptides (Zhao et al., 2022). These peptides typically exhibit stability to pH, ions, and heat, but their integrity may be compromised when exposed to the gastrointestinal environment (Adjonu et al., 2023; Wu et al., 2024). Importantly, research indicates that bioactive peptides maintain their biological activity, even when fully hydrolyzed into smaller peptide fragments (Tavares et al., 2011; Jiang et al., 2018).
Currently, the anti-breast cancer effects of whey protein-derived active peptides have received widespread attention. Various bioactive peptides, such as DYRWIAL, ARPKY, LIPRVKL, ALPMHIR, and LVMFQRR, have been isolated from fermented or enzymatically hydrolyzed whey proteins, demonstrating antitumor activity in breast cancer cells and/or animal models (Sahna et al., 2022; Taghipour et al., 2023). Except for individual active peptides, researchers have also investigated the anti-breast cancer effects of peptide mixtures from whey proteins of camel, goat, and cow (Dave et al., 2006; Sahna et al., 2022; Taghipour et al., 2023). Anti-breast carcinogenesis peptides and hybrid peptides identified from the aforementioned animal-derived whey proteins have shown effectiveness in reducing cancer cell proliferation, adhesion, and migration, promoting free radical accumulation, inducing apoptosis in cancer cells, and leading to the excessive expression of apoptosis-related proteins (Eason et al., 2004). Furthermore, investigations have reported that these peptides act mainly by activating several signaling pathways such as p38 MAPK and p53 signaling (Figure 2) (Dave et al., 2006).
Sahna et al. used pepsin and trypsin enzymes to hydrolysate goat casein and goat whey protein and obtained eight anti-cancer peptides consisting of 7–14 amino acids (Sahna et al., 2022). The research showed that the IC50 values (the concentration of the peptide required to achieve 50% viability in MCF-7 cells) of pepsin-treated whey protein hydrolysate, trypsin-treated whey protein hydrolysate, pepsin-treated casein hydrolysate, and trypsin-treated casein hydrolysate were 33.38, 23.49, 34.83 and 34.23 μg/mL, respectively. Besides, these peptide fragments also downregulated the expression of GSK 3-β, PKM2, LDH B, and MUC 1, while regulating RIPK1 levels, which may reveal the anticancer mechanism of these active peptides. Whey protein hydrolyzed peptides are the most studied active peptides, and anticancer peptides have also been isolated from them for research purposes. According to Dave et al. and Eason et al., the whey protein hydrolysate exhibited anti-tumor effects in MCF-7 cell and rat models, as evidenced by a decrease in tumor diversity, tumor incidence, and cell proliferation, as well as reduction in the expression of cyclin D1 and IGF-2 (Eason et al., 2004; Dave et al., 2006). There was also an upregulation of apoptotic cell proportions and terminal end bud number per mm2, along with the activation of some important anticancer factors (MCP-1, PTEN, PR) and inhibitory activation of oncogenic factors (p21, wip1, Sesn1, Mgmt, BRCA1, Bax), which may be attributed to the activation of multiple oncogenic signaling pathways such as p38 MAPK, Tp53, and PR signaling. However, neither study identified the specific anticancer peptide sequences contained in whey protein hydrolysates. Therefore, future studies could attempt to categorize the active whey protein-derived peptides into different fractions according to molecular weight and identify the most potent biopeptides with anti-breast cancer activity from these mixtures. In addition, probiotic fermentation presents a promising way to obtain active peptides from whey proteins, an area that has yet to be extensively explored.
2.3 Casein-derived anti-breast cancer peptides
Casein is a type of insoluble phosphoprotein and constitutes 80% of the milk proteins (Wusigale et al., 2020; Auestad and Layman, 2021). It is one of the predominant proteins in milk and can be isolated by precipitating milk at pH 4.6, as well as through techniques such as chromatography, electrophoresis, enzymatic treatment, and membrane filtration (Wusigale et al., 2020). Structurally, casein consists of four subfractions, αS1-, αS2-, β-, and κ-caseins, which account for approximately 38%, 10%, 36%, and 13% of the casein fraction, respectively (Auestad and Layman, 2021). These four casein subfractions are amphiphilic, with molecular weights ranging from 19 to 25 kDa and average isoelectric points between 4.1 and 5.3. This unique structure allows them to readily self-assemble into stable micellar structures in aqueous solutions, which can be destroyed in the presence of rennet (Wusigale et al., 2020). Casein is considered safe and non-toxic, with no mutagenic or clastogenic effects, and does not cause developmental, reproductive, or target organ toxicity (Dent et al., 2007; Phelan et al., 2009). Research by Dent et al., Doorten et al. and Phelan et al. robustly demonstrated that casein hydrolysates or their derived peptides, at doses not exceeding 2 g/kg body weight per day, did not induce any adverse reactions, nor did they cause abnormal changes in the eyes, nervous system, urine, blood, or kidneys (Dent et al., 2007; Doorten et al., 2009; Phelan et al., 2009). More importantly, casein provides the body with a well-balanced profile of essential amino acids, characterized by extremely high levels of proline (accounting for 16% of the total amino acids) and nearly complete absence of cysteine. This results in a structure that lacks the disulfide bridges and alpha-helices typically found in most proteins (Auestad and Layman, 2021). The biological activity of casein has also been widely reported by scholars. Some bioactive peptides have already been identified from casein by enzymatic hydrolysis methods, including alcalase, fermentation, and chemical and physical hydrolysis, and these peptides exhibit stability against heat, acidic conditions, and gastrointestinal enzymes (Quirós et al., 2009; Contreras et al., 2013; Wu et al., 2014; Kumar et al., 2016; Xue et al., 2021). Some of these bioactive peptides exhibit immunomodulatory, antioxidant, gut regulatory, and nutrient-absorption promoting effects locally in the intestinal cavity, while others need to be absorbed into the bloodstream to exhibit bioactivities. Therefore, studying both intact peptide absorption and peptide bioactivity is equally significant.
Anticancer peptides in casein proteins have been widely investigated, such as anti-ovarian cancer peptide PGPIPN, anti-melanoma peptide INKKI, and anti-leukemia peptide FFSDK (Sah et al., 2015). However, research on casein-derived peptides specifically targeting breast cancer has been limited, with only a few studies focusing on yak milk casein and human αS”-casein reporting the activities of anti-breast cancer peptides. For example, Gu et al. isolated three anti-breast cancer peptides, TPVVVPPFL, VAPFPEVFGK and NQFLPYPY, from yak milk casein and reported that these peptides exhibited excellent anticancer effects in both MCF-7 and MDA-MB-231 breast cancer cell models (Gu et al., 2022). These effects were characterized by an apparent increase in cancer cell death, a significant reduction in cell viability and cell cycle arrest at G2/M phase. Another study by KAMPA et al. also stated that YVPFP, an anti-breast cancer peptide derived from human αS”-casein, significantly (p < 0.05) decreased the proliferation of T47D cells (Kampa et al., 1996). Unfortunately, there is insufficient research on casein-derived anti-breast cancer peptides to draw definitive conclusions. Future efforts could focus on identifying active peptides from the four casein subfractions and comparing their anticancer activities in experimental models. In addition, while most of the identified active peptides or peptide mixtures have validated their anticancer activity in cancer cell models, animal transplantation tumor models are also very important for confirming experimental results or exploring anti-breast cancer mechanisms.
3 Anti-breast cancer activities of dairy products
Some scholars have also studied the anti-breast cancer activity of dairy products, with some popular examples including fermented milk, kefir, and cheese (Table 2). These fermented dairy products often contain a large number of anti-breast cancer active peptides and other anticancer bacterial metabolites. Identifying these anticancer active ingredients and investigating the anticancer effects and mechanisms of dairy products have become prominent areas of research in functional foods.
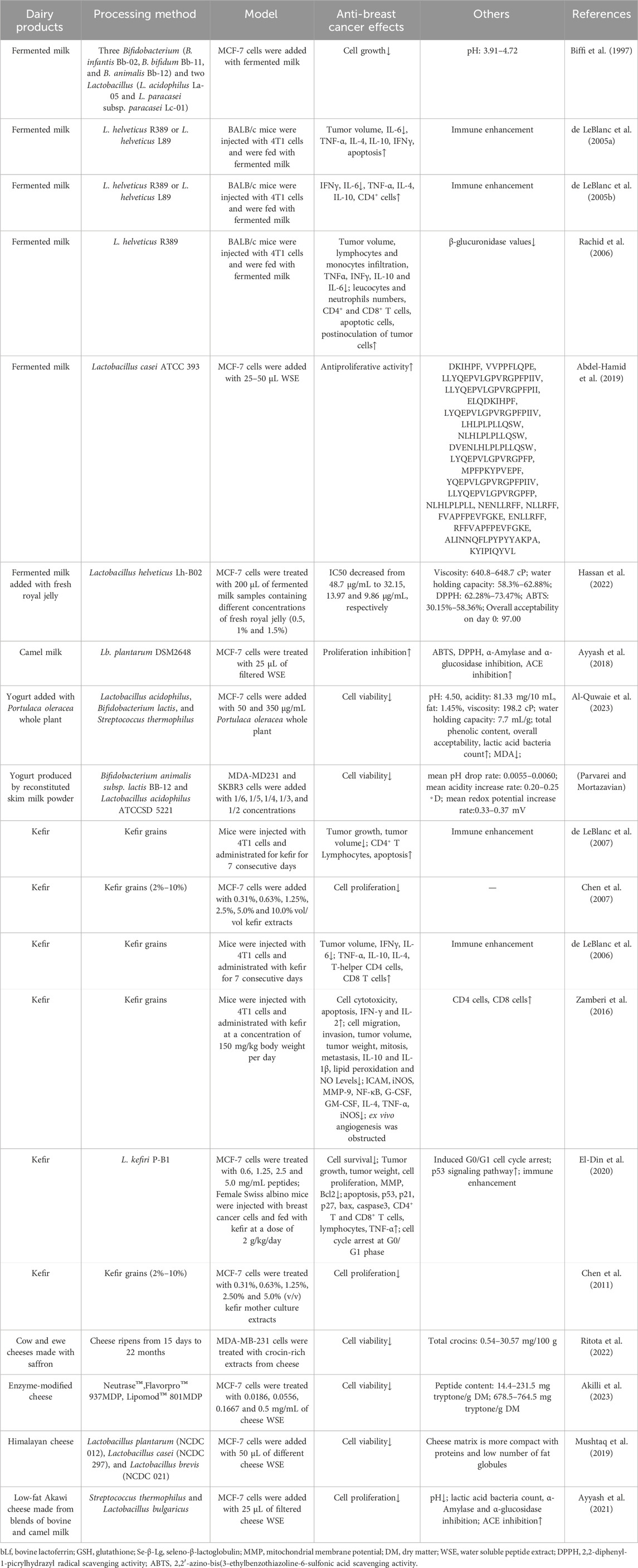
Table 2. Inhibitory effects and mechanisms of fermented or non-fermented dairy products on breast cancer progression.
3.1 Anti-breast cancer effects of fermented milk
The demand for fermented milk has been rapidly increasing due to the growing vegetarian population and the rising popularity of high-protein diets (Sakandar and Zhang, 2021). The global fermented milk market was valued at USD 320.60 billion in 2022 and is expected to grow at a CAGR of 4.9% from 2022 to 2031, potentially reaching nearly $500 billion by 2031 according to Straits Research. Fermented milk is produced through the fermentation and acidification of milk by live microorganisms, typically Lactobacillus bulgaricus and Streptococcus thermophilus, resulting in the breakdown and utilization of macromolecules in the milk, and further the production of peptides, bacteriocins, free fatty acids, and conjugated linoleic acid, finally enhancing bioavailability and thickness of the products, increasing the number of viable bacteria, and prolonging their shelf life (Alhaj and Kanekanian, 2014; Hadjimbei et al., 2022). Traditionally, milk from various mammalian species, including cows, sheep, goats, camels, mares, buffaloes, and yaks, can be used to prepare fermented milk products. However, the flavor, composition, and nutritional value of the final product are affected by factors such as geographic and climatic conditions, animal health, species, feeds, seasons and stages of lactation, strains of fermenting bacteria, and fermentation conditions (Bintsis and Papademas, 2022). Commonly used milk starters are Lactococcus lactis subsp. cremoris, Str. Thermophilus, Lc. lactis subsp. lactis, Lb. delbrueckii subsp. delbrueckii, Lb. delbrueckii subsp. lactis, Lb. helveticus, Leuconostoc spp., etc. (Bintsis and Papademas, 2022). They are safe and beneficial for enriching the natural flora of the human gut. Additionally, fermented milk is a good source of calcium, phosphorus, potassium, vitamin A, vitamin B2, vitamin B12, high biological value proteins and essential fatty acids (Ilesanmi-Oyelere and Kruger, 2020). They also offers unique flavors, smooth textures, appealing appearances, enhanced digestibility and specific benefits such as anti-fatigue, antioxidant, anti-inflammatory, anti-cancer, intestinal modulation, cardiovascular disease improvement, serum cholesterol-lowering effects, etc., as compared to regular milk, which makes them popular among consumers and researchers (HoSoNo et al., 2002; Szajnar et al., 2020; Abdelrazik and Elshaghabee, 2021; Zhang D. J. et al., 2023; Zhang Z. Q. et al., 2023).
Due to the existence of various anticancer components, such as active peptides, probiotics, short-chain fatty acids, and probiotic metabolites, the effect of fermented milk on cancers has been widely studied. Some scholars have focused on identifying anticancer peptides from fermented milk. Abdel-Hamid et al. used Lactobacillus casei ATCC 393 to prepare fermented milk and characterized the composition of the obtained products (Abdel-Hamid et al., 2019). They found that the fermented milk contained a bacterial population exceeding 9 log cfu/g, with a pH value of 4.52, titratable acid content of 0.73%, and proteolytic activity of 0.36. Additionally, both the water extract of fermented milk (WSE) and the fermented milk extract with a molecular weight of less than 2 kDa (F1) exhibited ACE-inhibitory, antioxidant, and anti-breast cancer activities. Notably, the antiproliferative activity of the F1 fraction was 64.25% on day 0 and this value increased to 78.90% on days 21 of storage. Finally, they also identified the sequences of active peptides in F1 and successfully obtained several peptides such as DKIHPF, VVPPFLQPE, ELQDKIHPF, LHLPLPLLQSW, NLHLPLPLLQSW, LYQEPVLGPVRGPFP. However, a limitation of this study is that no specific anti-breast cancer studies were conducted on the identified active peptides. Biffi et al., de LeBlanc et al., de LeBlanc et al. and Rachid et al. fermented cow’s milk with different starter bacteria and found that the extracts inhibited breast cancer progression in mouse and cellular models (Biffi et al., 1997; de LeBlanc et al., 2005a; de LeBlanc et al., 2005b; Rachid et al., 2006). Specifically, fermented milk inhibited breast cancer cell proliferation, reduced tumor size, and modulated cytokines (downregulated IL-6 levels and upregulated TNF-α, IL-4, and IL-10 levels). In addition, fermented milk enhanced the immune function of BALB/c mice, as evidenced by an increase in the number of CD4+ and CD8+ T cells. In recent years, the addition of other natural anticancer-active ingredients into fermented milk has become a hot topic. Hassan et al. discovered that incorporating fresh royal jelly into Lactobacillus helveticus Lh-B02 fermented milk significantly increased its anti-breast cancer activity, with the IC50 value of the fermented milk extract decreasing from 32.15 μg/mL to 9.89 μg/mL as the royal jelly addition concentration increased from 0.5% to 1.5% (Hassan et al., 2022). The addition of natural components, especially polyphenols, significantly improves the anticancer activities of fermented milk, although it may also alter other properties, such as color, viscosity, texture and sensory scores. Thus, these indicators should be taken into account when preparing fermented milk with added anticancer components. Some scholars have also explored the anti-breast cancer effects of fermented camel milk. On the first day of fermentation, fermented camel milk showed lower antiproliferative activity (41.5%) against MCF-7 cells compared to fermented cow’s milk (44.9%), however, after 21 days of post-ripening, the antiproliferative activity of fermented camel milk (47.9% (fermented camel milk) vs. 44.8% (fermented cow’s milk)) was significantly enhanced. Therefore, camel milk might be a better anticancer food ingredient than cow’s milk, and future research on fermented camel milk could focus on identifying anti-breast cancer peptides, and exploring the in vivo anticancer effects and anticancer mechanisms. In addition, the anti-breast cancer activity of dairy products such as fermented sheep, goats, mare, buffalo, and yak milk is also worth exploring.
Researchers have also explored the beneficial effects of fermented milk as an immunoadjuvant in breast cancer chemotherapy. In one study, milk fermented by Lactobacillus casei CRL431 not only enhanced the therapeutic efficacy of capecitabine but also effectively prevented cancer cell metastasis and alleviated the toxicity associated with capecitabine treatment (Utz et al., 2021). This was evidenced by reduced weight loss in mice, restored leukocyte counts and hematocrit levels, decreased diarrhea scores, improved inflammatory markers (IL-6, TNF-α, IL-10, IFN-γ, and MCP-1), and a higher villi length to crypt depth ratio. Overall, fermented dairy products are most likely to be initially applied as adjunctive agents in the clinical treatment of breast cancer.
3.2 Anti-breast cancer effects of yogurt
Yogurt is one of the most widely consumed and oldest fermented dairy products. It is prepared by fermenting and acidifying milk with bacterial culture (primarily Lactobacillus delbrueckii subsp. bulgaricus and Streptococcus thermophilus, but some products also use Bifidobacterium species, Lactobacillus helveticus, etc.), resulting in a thickened, more easily digestible product with an attractive flavor and a longer shelf life (Olson and Aryana, 2022; Hasegawa and Bolling, 2023). Yogurt is typically made from the milk of cows, buffalo, sheep, goats, and other livestock, and it can be categorized into various types, including plain yogurt, whipped yogurt, fruit-flavored yogurt, Greek yogurt, and frozen yogurt (Aryana and Olson, 2017; Oz et al., 2023). According to Statista, yogurt sales in the U.S. reached $7.24 billion in 2021, compared to $5.58 billion in 2011, implying a robust growth rate in the yogurt market over the past decade (Olson and Aryana, 2022). Nutritionally, yogurt provides highly digestible and bioavailable proteins, calcium, potassium, vitamin A and vitamin B12, and its rich components of live microorganisms and their metabolites also serves to nourish the intestinal tract (Webb et al., 2014). From a flavor perspective, more than 90 different volatile compounds have been detected in yogurt, including acids, alcohols, aldehydes, ketones, esters, etc. Key contributors to the desirable flavor of yogurt include lactic acid, acetoin, acetaldehyde, diacetyl, and 2-butanone (Chen et al., 2017).
Yogurt hydrolysates are rich in bioactive peptides and free amino acids, making them important resources for cancer prevention (Çakmakoglu et al., 2024). Kefir is one of the best-known branches of yogurt, with origins tracing back to the Balkans, Eastern Europe and the Caucasus, but nowadays its consumption has expanded to other parts of the world due to its excellent health-promoting effects (Azizi et al., 2021). Kefir is also an acidic and viscous fermented beverage prepared by fermentation carbonation of kefir grains with milk or water, typically composed of 89%–90% water, 6.0% sugar, 3.0% protein, 0.2% lipids, 0.7% ash, and 1.0% lactic acid and alcohol (Azizi et al., 2021). The main fermenting bacteria in kefir are lactic acid bacteria and yeasts, with the former mainly comprising Lactobacilli, Lactococci, Streptococci, etc., while the latter primarily containing Candida sp., Kluyveromyces sp., Saccharomyces sp., etc. (Arslan, 2015). The symbiotic metabolism of these complex mixtures contributes to protein hydrolysis and lipolytic degradation, thereby imparting a unique flavor to kefir (mainly derived from lactic-, acetic-, hippuric-, butyric-, pyruvic-, propionic-acid, diacetyl and acetaldehyde) (Arslan, 2015). However, due to the complexity of kefir colonies, the biological activity of the obtained kefir products varies largely, sparking interest among researchers in optimizing fermentation conditions and ingredients to enhance the functional properties of fermented dairy products.
The anti-breast cancer effects of kefir have been revealed in several studies. Scholars have stated that kefir reduces tumor cell viability and growth rates, regulates cytokine expression in tumor tissues (e.g., downregulates IL-6, IL-10 and IL-1β, upregulates TNF-α, IL-10, and IL-4), promotes apoptosis, and exerts immune-enhancing effects. As reported by Zamberi et al., kefir inhibited the growth and metastasis of breast cancer in both in vitro and in vivo experiments (Zamberi et al., 2016). In addition, kefir reduced lipid peroxidation and NO levels in vivo, attenuated the accumulation of inflammatory factors of IL-4, TNF-α, iNOS, IL-10, and IL-1β, enhanced the occurrence of blocked angiogenesis as well as induced a 5-fold increase in CD4+ T levels and a 7-fold increase in CD8+ T cells. These findings were corroborated by studies conducted by de LeBlanc et al. (de LeBlanc et al., 2006; de LeBlanc et al., 2007). The anticancer effects of kefir may stem from its ability to activate a series of apoptotic pathways (Bcl2↓, bax, caspase3↑) and tumor signaling inhibitory pathways (p53, p21, p27↑), as well as to induce immune enhancement, such as upregulation of CD4+ T and CD8+ T cells (El-Din et al., 2020). However, there are few studies that simultaneously focus on the quality, anticancer effects and anticancer components of kefir.
In addition to kefir, plain yogurt also exhibits anti-breast cancer activity in several studies. Parvarei and Mortazavian found that yogurt fermented with Bifidobacterium animalis subsp. lactis BB-12 and Lactobacillus acidophilus ATCCSD 5221 significantly reduced the activity of MDA-MD- 231 cells (Parvarei and Mortazavian, 2022). However, this study did not measure other anti-cancer indicators. A more popular research direction involves the addition of natural anticancer substances to yogurt. For example, Al-Quwaie et al. incorporated Portulaca oleracea extract into yogurt and evaluated its fat (1.45%), pH (4.50), titratable acid (81.33 mg/10 mL), viscosity (198.2 cP), and water holding capacity (7.7 mL/g) (Al-Quwaie et al., 2023). The addition of Portulaca oleracea not only improved the acceptability and Lactobacilli content of yogurt but also enhanced its anticancer potential and effectively inhibited the accumulation of malondialdehyde (MDA). Further investigations are needed to explore the effects of adding various probiotics or natural actives to yogurt to further enhance its anticancer efficacy.
3.3 Anti-breast cancer effects of cheese
Cheese is one of the most ancient dairy-based fermented products that was first produced in various Western Asia countries around 8,000 years ago. It is consumed by a huge amount of the world’s population, regardless of age or origin (Zheng et al., 2021; Zhang et al., 2024a). Recently, cheese consumption has been growing at an average annual rate of 3%, with global sales reaching $122.1 billion due to increased demand for healthier, more nutritious, and more convenient foods (Hutchins and Hurley, 2024; Zhang et al., 2024a). About 2,000 types of cheese are reported, with well-known varieties including Cheddar, Mozzarella, and Parmesan (Feeney et al., 2021). These cheeses are generally made from natural ingredients, such as fresh milk (cows, buffaloes, camels, goats, sheep and donkeys), starter culture, salt and enzymes, through a series of fermentation processes (Ozcan and Kurdal, 2012; Feeney et al., 2021; Bittante et al., 2022). Most cheeses are prepared by coagulating milk with rennet and then ripening for about 2 weeks to 2 years. Other cheeses are manufactured via acid coagulation or a combination of heat and acid and are typically consumed fresh (Feeney et al., 2021). From a nutritional standpoint, cheese can be recognized as a promising macronutrient-rich food, with a high fat and protein content, as well as a micronutrient-rich food, with considerable concentrations of vitamins A, B2 and B12 and calcium (Zhang et al., 2024a). The ultimate consumer acceptance of cheese largely depends on its sensory characteristics, such as flavor, texture and aroma, which are determined by the various compounds and molecules that constitute it, including fatty acids, ketones, amines, free amino acids, active peptides, alcohols and aldehydes (Zheng et al., 2021).
The frenzy of cheese consumption has also prompted researchers to investigate its potential health benefits. It is well established that cheese has anti-hypertensive, antifungal, antibacterial, antioxidant, and gut-regulating properties. Thus, isolating highly active substances from cheese, including anticancer peptides, is of high value and can promote the development of functional foods (Sprong et al., 2010; Bernabucci et al., 2014; Théolier et al., 2014; Chen et al., 2019). According to Akilli et al., the active peptide content in digested and undigested enzyme-modified cheese was found to be 678.5–764.5 mg tryptone/g DM (dry matter) and 14.4–231.5 mg tryptone/g DM, respectively (Akilli et al., 2023). These active peptides exhibited anti-breast proliferative effects against MCF-7 cells. In addition, the anticancer activity of cheese became higher with the extension of storage time, likely due to an increase in the proportion of small peptides resulting from protein hydrolysis during ripening, thus enhancing cancer cytotoxicity. The study of Ayyash et al. found that the anti-proliferation activity of cheese against MCF-7 cells (about 55%) reached the highest at camel milk to bovine milk ratio of 30:70 (Ayyash et al., 2021). After 30 days of storage, the anti-proliferation activity of cheese further increased to ∼90%. However, this study did not explore the effects of ripening time on the active components of cheeses, leaving the specific reasons for the enhanced anticancer activity unclear. The effect of different starter cultures on the anticancer activity of cheese was also investigated. Cheeses fermented with Lactobacillus plantarum NCDC 012, Lactobacillus casei NCDC 297, or Lactobacillus brevis NCDC 021 presented a significant increase in anticancer activity compared to cheeses without starter bacteria. However, no significant differences in proliferation inhibition were observed between different cheese extracts. The enhanced anticancer activity could be due to the massive release of bioactive peptides triggered by the starter culture, thus initiating a cascade of caspase cleavage, or it might be attributed to the competitive binding of peptides and cancer growth factors to cancer cell membrane receptors, leading to apoptosis of cancer cells.
Several active or functional ingredients can be added to cheese for a variety of reasons, such as enhancing flavor, increasing bioactivity and therapeutic effects, and improving texture and sensory scores. There are few studies focused on incorporating anticancer ingredients into cheese. In one notable study, saffron, a traditional Chinese medicine known for its antidiabetic, antithrombotic, anti-depression, anti-anxiety, and insomnia-relieving effects, was added to cheese curd. The obtained cheeses exhibited higher anti-breast cancer activity than cheeses made by adding saffron directly to cheese milk. In addition, stronger anticancer effect was observed after 19 months of cheese ripening, with the cheese extract reducing the activity of MDA-MB-231 cells to less than 60%. This study lays the foundation for supplementing other anticancer ingredients into cheese. Polysaccharides and protein hydrolysates have been reported to improve cheese quality as well as inhibit the growth of breast cancer cells making them potential candidates for blending into cheese milk in the future (Taniya et al., 2020; Wan et al., 2020; Zhang D. J. et al., 2021; Zhang et al., 2024b).
4 Anti-breast cancer activities of dairy protein-based nanoparticles
Nanotechnology is a crucial cutting-edge technology that facilitates contribution, development and sustainable impacts in all sectors of human activity, involving food, medicine and agriculture, and provides innovative applications and useful solutions for related industries (Wasilewska et al., 2023). Nanoparticles are synthetic particles with sizes ranging from 1 to 1,000 nm, serving as intermediate structures between atoms and microscopic objects (Wasilewska et al., 2023). Their appearance can be spherical, conical, tubular, cylindrical, hollow, helical, etc., or irregular, with significant variability in size, structures and crystalline morphology (Joudeh and Linke, 2022). In terms of material, nanoparticles can be categorized into three main categories: organic, carbon-based, and inorganic. Organic nanoparticles are further divided into carbohydrate-based, protein-based, lipid-based, and polymer-based nanoparticles, which have the advantage of being naturally nontoxic, biodegradable, and partially cavity-containing. Carbon-based nanoparticles includes fullerenes, carbon black nanoparticles, and carbon quantum dots, etc., which are characterized by high strength, electrical conductivity, special optical and thermal characteristics as well as excellent biocompatibility (Joudeh and Linke, 2022). Inorganic nanoparticles are mainly metal-based, ceramic-based and semiconductor-based nanoparticles, which demonstrate high stability and excellent loading capacity compared to other nanoparticles (Joudeh and Linke, 2022). In the cross fields of food and medicine, nanoparticles can be used to deliver bioactive compounds to the target organs or tissues, which has greatly advanced the application of nanoparticles in the food, nutraceutical, and pharmaceutical industries (Wen et al., 2022; Luo et al., 2023). These technologies not only protect active substances such as active peptides, polyphenols, vitamins, minerals, essential fatty acids, flavors, and antioxidants, but also control the release speed of drugs or bioactive compounds, ensuring their precise delivery and greatly improving therapeutic efficacy against various diseases (Luo et al., 2023; Zhang Z. Q. et al., 2023). Specifically, nanoparticles prevent damage to the active substance caused by the harsh environment of the gastrointestinal tract, improve drug absorption/permeation in the gastrointestinal tract, and target drugs or active substances to specific cells, tissues, or organs in the human body through passive or active transport pathways (Martins et al., 2023).
Dairy protein-based nanoparticles are considered safe, efficient, highly loaded and easy to prepare (Tavakoli et al., 2021). The main types of nanoparticles using dairy protein as wall material contain β-lactoglobulin nanoparticles, LF nanoparticles, whey protein nanoparticles and casein nanoparticles (Table 3). These nanoparticles offer advantages such as low cytotoxicity, excellent biocompatibility and biodegradability, and enhanced specific delivery (Tavakoli et al., 2021). In addition, several studies have utilized dairy protein hydrolysates or dairy protein-derived active peptides as encapsulated substances within nanoparticles, which effectively prevents the inactivation of active peptides in the hostile environment of moisture, gastric acid, and digestive enzymes, all of which ensures the precise delivery of the drug to the intestinal tract (Zhang Z. Q. et al., 2023). Both scenarios have been applied in the treatment of breast cancer, typically exhibiting more effective breast cancer cell-killing effects and significantly inhibiting the invasion, migration and metastasis of cancer cells, eventually leading to better therapeutic effects (Abu-Serie and El-Fakharany, 2017; Atallah et al., 2022). LF nanoparticles are currently one of the most studied nanoparticles, featuring unique advantages such as small particle size, injectability, excellent bioactivity and certain loading capacity (Tavakoli et al., 2021). The combination of LF and polysaccharides to prepare nanoparticles for the treatment of breast cancer is a hot research direction. Popular examples such as LF-chitosan, LF-pectin, LF-polysaccharide carboxy methyl cellulose, etc., have garnered substantial attention as prospective materials (Aval et al., 2021; Abu-Serie and El-Fakharany, 2017). The bovine lactoperoxidase (LPO), LF and chitosan nanoparticles (NPs) were crosslinked by Abu-Serie and El-Fakharany to obtain LF coat LPO-loaded NPs (Abu-Serie and El-Fakharany, 2017). The loading capacity, encapsulation efficiency (EE), particle size and zeta potential of these prepared NPs were 62.3%, 88.7%, 477.5 nm and 20.6 mV, respectively. Besides, LF coat LPO-loaded NPs inhibited cancer cell viability (IC50: 150.1 ± 4.8 μg/mL), induced apoptosis (apoptotic cells: 47%), and blocked the cell cycle, which was attributed to the regulation of NF-κB, Bcl-2 and p53 by the NPs. However, this study only explored the in vitro anticancer effects of the NPs, leaving unanswered whether they can be effectively released and exert similar efficacy in vivo. Considering LF is a protein with a high affinity for trivalent iron, some researchers and scholars have also explored the anticancer effect of LF nanoparticles saturated with trivalent iron ions. Kanwar et al. prepared an alginate-coated chitosan nanoparticle encapsulated Fe3O4-saturated BLF (FebLf NCs) and used it in MDA-MB-231 cells and a mouse tumor model (Kanwar et al., 2016). The results showed that FebLf NCs had a spherical appearance with a particle size of only 80 ± 5 nm. Furthermore, FebLf NCs exhibited antiproliferative effects against breast cancer cells, decreased cell viability, inhibited clone formation, reduced the diameter of 3D tumor spheroids, enhanced mitochondrial depolarization, reduced tumor volume in mice, and activated the pro-apoptotic protein of caspase-3, which was associated with the activation of the P13K signaling. However, this study did not compare the anticancer effects of LF nanoparticles with different trivalent iron ion saturation. This question was addressed by Roy et al., who successfully designed two LF nanoparticles with different iron saturations, one was apo-bovine lactoferrin (Apo-bLf) (∼ 2% iron saturated)-encapsulated Eri silk nanoparticles (NPs) (Apo-bLf-loaded silk NPs) and another was Fe-bLf (100% iron saturated)-encapsulated Eri silk nanoparticles (Fe-bLf-loaded silk NPs), and used them to treat breast cancer (Roy et al., 2016). The two nanoparticles did not differ significantly in terms of particle size, microscopic image, molecular weight, and fourier transform infrared (FT-IR) spectra. However, both nanoparticles were internalized by breast cancer cells, with Apo-bLf-loaded silk NPs showing greater internalization compared to void NPs, which resulted in higher cell toxicity, greater caspase-3 release, and better efficacy in reducing the tumor sphere size of Apo-bLf-loaded silk NPs than Fe-bLf-loaded silk NPs. Nevertheless, qPCR and western blot results showed that Fe-bLf-loaded silk NPs exhibited comparatively higher pro-apoptotic effect, evidenced by a more significant upregulation of Survivin, Fas, Fas-L, lipoprotein receptor-related protein (LRP)1, LRP2, caspase-8, caspase-9, and caspase-3 and a more significant downregulation of epidermal growth factor receptor (EGFR), transferrin receptor (TfR) 1, and TfR2. Viewed together, these two nanoparticles represent promising anti-cancer materials, implying that Eri silk NPs have broader medical uses.
Considering the need to enhance therapeutic effects and decrease the toxicity of chemotherapeutic agents, several studies have integrated these chemotherapeutic agents into LF-modified nanomaterials. Current research trends favor the use of dual or triple drug combinations to enhance the anti-breast cancer efficacy of LF nanoparticles. Atallah et al. developed crosslinked LF-carboxymethyl cellulose nanogels (Lf/CMC NGs) to encapsulate PMT and the herbal polyphenol honokiol (HK), achieving dual-drug therapy for breast cancer (Atallah et al., 2022). The nanoparticles showed an HK EE of 66.67% and a PMT conjugation efficiency of 80.1%, demonstrating excellent serum stability, low hemolytic activity, and can be effectively taken by breast cancer cells. This led to anti-breast cancer effect through the inhibition of angiogenesis, promotion of apoptosis in cancer cells, and increased expression of apoptotic proteins. However, the study did not explore the mechanisms behind the anti-breast cancer effects of LF-modified NPs. The triple drug combination represents an evolution of the dual drug strategy, aimed at maximizing anti-breast cancer efficacy through synergistic interactions among three different drugs. Salah et al. created triple drug-loaded nanoparticles by combining pemetrexed (PMT), rosuvastatin (RST), and HK within sodium alginate (ALG) and LF nanohybrids, achieving loading efficiencies of 7.86% RST, 5.24% PMT, and 6.11% HK (Salah et al., 2022). These nanoparticles showed prolonged drug release, stability in blood and serum, and significant cytotoxicity against breast cancer cells, with an IC50 of 0.94 µM for MCF-7 cells, a 5-fold reduction in tumor size after 3 weeks of treatment, and enhanced active caspase-3 levels alongside reduced VEGF and Ki67 expression. Interestingly, certain studies have introduced magnetic fields and photothermal therapy into LF nanoparticles loaded with chemotherapeutic drugs, not only improving anti-breast cancer efficacy and reducing treatment duration but also lowering tumor recurrence risk and mitigating chemotherapy resistance. Lf-Doxo-MMNPs (Size: 130 ± 1.48 nm), a therapeutic NP integrating chemotherapy, magnetic field, and photothermal tools, demonstrated prolonged blood circulation as well as notable cytotoxicity in breast cancer cells/tissues by activating Bax and caspase-3 and modulating CXCL12 and CXCR7, thereby causing necrosis and apoptosis, blocking cell cycle at the S1 and subG1 phases, and inducing ROS accumulation (Sharifi et al., 2020). Future investigations should focus on optimizing LF-modified nanoparticle formulations or combining diverse therapeutic strategies with nanoparticles to maximize efficacy while minimizing or avoiding the side effects of chemotherapy.
β-Lactoglobulin (BLG) is another commonly used material for preparing anticancer nanoparticles. It has a β-barrel structure consisting of eight anti-parallel β-sheets with high colloidal stability and net charge distribution, which facilitates the formation of a tight structure with other materials for delivering active substances to targeted sites (Salah et al., 2020; Gao et al., 2021). In one study, AuNP-BLG-CUR and AuNP-BLG-GEM were prepared by conjugating β-lactoglobulin (BLG) with gold nanoparticles (AuNP) and embedding curcumin (CUR) or gemcitabine (GEM) into it, which effectively prevented the degradation of curcumin due to light and oxidation. The particle size of AuNP-BLG ranged from 29 to 41 nm, with a zeta potential of −24.5 to −7.89 mV. The MTT results showed that the IC50 values of AuNP-BLG against MCF-7 cells were 1.445 nM at 24 h and 0.863 nM at 48 h, showing a very strong anticancer effect. However, the drawback of this study is that the anticancer effects and anticancer mechanisms of AuNP-BLG-CUR and AuNP-BLG-GEM were not further revealed in an in vivo model.
Whey protein, a by-product of cheese processing, is not only nutritionally valuable but also exhibits good availability, high resistance to pepsin digestion, and excellent protective film-forming abilities (Khan et al., 2019; Liu et al., 2022). In addition, whey protein is also thermoprotective compared to other materials as it resists heating-induced increase in flexible conformation and exposure of hydrophobic fragments on its exterior (Liu et al., 2022). In a study employing whey protein nanoparticles for the treatment of breast cancer, N-acetyl cysteine-modified doxorubicin hydrochloride-loaded whey protein nanoparticles (CyWD) were successfully synthesized and exhibited a particle size of 358.05–562.3 nm, a PDI of 0.1215–0.376, a zeta potential of −29.66–55.785 mV and an EE of 48.24%–84.58% (Singh et al., 2022). The optimally formulated nanoparticles were used in mice and results revealed that the tumor volume, areas of tumor damage and concentration of Dox significantly (p < 0.05) decreased after administration of CyWD, suggesting that CyWD greatly improved drug delivery efficiency and enhanced tumor suppression. However, this study lacks mechanistic exploration reagrding tumor inhibition by CyWD. Furthermore, although the formulation of CyWD is well-designed, its efficacy in vivo warrants further investigation.
Casein constitutes 80% of milk protein, with approximately 95% existing in the form of colloidal particles (Zimet et al., 2011). These particles are usually spherical, with diameters ranging from 50 to 500 nm (average 150 nm) and masses ranging from 106 to 3 × 109 Da (average 108 Da), rendering them extremely suitable for nanoparticles preparation due to their strong tendency to associate and very high loading capacity (Zimet et al., 2011). Moreover, another advantage of casein is that it lacks disulfide bonds, contains abundant content of proline, and has poor secondary and tertiary structure, making it an intrinsically unstructured protein. This characteristic allows for easy chemical modification, further enhancing the performance of the nanoparticles (Gandhi and Roy, 2019). Recently, casein nanoparticles have been used to enhance the DNA-damaging ability of 10-hydroxy camptothecin (CPT) on cancer cells. In a study of Khatun et al., CPT and Nile red-encapsulated casein nanoparticles (NR-CCS NPs) were synthesized, achieving an EE of 77.47%–80.79%, a hydrodynamic diameter of 16 ± 3 nm, a zeta potential of 24.08 ± 2 mV, and a spherical appearance (Khatun et al., 2023). NR-CCS NPs exhibited the highest CPT release efficiency under simulated tumor microenvironment conditions (pH 5.5°C and 37°C), with a mucin binding efficiency of 80% ± 2%. These NPs demonstrated excellent biocompatibility and intracellular uptake ability, which led to decreased 4T1 cell activity, increased apoptosis, inhibited clone formation, reduced cancer cell migration ability, elevated mitochondrial membrane potential, cell cycle arrest in the G2/M phase, aggravated DNA damage, and increased ROS accumulation. Further studies showed that these changes were related to the activation of the lysosome-mediated autophagy pathway. Based on previous studies, Khatun et al. improved the targeting ability of the nanoparticles for triple-negative breast cancer by creating glutathione (GSH)-IR 797 and CPT coupled casein nano-trojan (CCNG NPs), which can naturally bind to the overexpressed gamma-glutamyl transpeptidase (GGT) in cancer cells due to the presence of GSH, thus allowing for precise targeting of cancer cells (Khatun et al., 2024). The hydrodynamic diameter, PDI and zeta potential of the CCNG NPs were 160 nm, 0.130–0.177, and −2.53 ± 3 mV, respectively. The scanning electron microscopy experiments revealed that CCNG NPs had a larger volume than NR-CCS NPs, and accordingly, some parameters also changed. The killing effect of CCNG NPs against 4T1 cells was enhanced, reaching up to 85% ± 8.5%, alongside significant increases in ROS levels and mitochondrial membrane potential. Besides, this study also found that tumor volume and mice weight decreased significantly (p < 0.05) after 7 days of CCNG NPs injection, and a large number of cancer cell necrosis, and neutrophil infiltration were observed. Interestingly, an inhibitory effect on spleen enlargement was also observed in the CCNG NPs group, suggesting that CCNG NPs may be involved in immunomodulation, although this hypothesis was not confirmed in this study. Both two studies are well-established, in-depth studies exploring nanoparticle characterization, in vivo release and cancer inhibition. In the long term, there have been some basic research explorations on dairy protein-based nanoparticles in the field of breast cancer, but clinical studies on dairy protein-based nanoparticles are still lacking. Moreover, explorations such as optimizing the formulation of dairy protein nanoparticles, enhancing the encapsulation ability of milk protein nanoparticles, and improving the therapeutic effect of nanoparticles, are worthy of further development. Finally, the exploration of the anticancer mechanism of some dairy protein-based nanoparticles, such as β-LG nanoparticles and whey protein nanoparticles, is still incomplete, and these require the joint efforts of scholars. Addressing these scientific and practical issues will advance the field of milk protein nanoscience and significantly impact the progress of precision medicine, enabling the design of safe and efficient dosages of nanoparticles for anti-breast cancer drug delivery applications.
5 Anti-cancer effects of dairy proteins in clinical practice
Dairy products and their derivatives supply essential micronutrients, macronutrients, and probiotics, including proteins, lipids, vitamin D, calcium, potassium, and magnesium, all of which contribute to metabolic health (Marangoni et al., 2019; Hirahatake et al., 2020). Numerous studies summarize substantial evidence indicating that long-term consumption of dairy products is safe, with most associations between dairy intake and health being beneficial. These benefits extend to individuals across all age groups, expect for specific conditions such as lactose intolerance or milk protein allergy (Mihic et al., 2016; Marangoni et al., 2019). Furthermore, a study assessing the clinical safety of dairy products found that subjects consuming ½ cup of novel milk protein peptide and ½ cup of milk daily for 6 weeks did not exhibit any abnormal clinical blood markers or adverse effects; instead, they showed improvements in insulin sensitivity and the neutrophil-to-lymphocyte ratio (Kreider et al., 2011). These studies have encouraged researchers to investigate the potential health-promoting effects of dairy products and their derivatives in clinical settings, particularly regarding their impact on cancer.
Considering that dairy products have demonstrated breast cancer inhibitory effects in both in vitro and in vivo experiments, some researchers have also explored the breast cancer inhibitory ability of dairy products in clinical settings (Table 4). For instance, Bahadoran et al. investigated 100 breast cancer patients and 175 healthy individuals and found that dairy intake, including low-fat and fermented dairy products, was positively associated with a reduced risk of breast cancer (Bahadoran et al., 2013). Similar results were reported in studies by Yu et al., which involved 1,286 breast cancer patients and 1,461 controls, and Wu et al., which included more than 1 million women followed for 8–20 years (Yu et al., 2019; Wu et al., 2021). The breast cancer preventive effect of kefir, yogurt and cheese have also been revealed in the clinic. Vantveer et al. stated that compared to 289 population controls, 133 incident breast cancer cases, regardless of younger (25–44 years) or older (55–64 years), had lower consumption levels of yogurt, buttermilk, curds, Gouda cheese, and total fermented dairy products, resulting in an increased risk of breast cancer incidence (Vantveer et al., 1989). Clinical studies providing dairy-derived active peptides to patients with breast cancer are extremely rare. The only one providing dairy-derived active peptides to 134 women with breast cancer and 267 controls without breast cancer resulted in a reduced risk of ER/PR/HER2-negative breast cancer in Iranian women (Jabbari et al., 2022). However, more research evidence is needed to clarify the relationship between dairy-derived active peptides and the risk of breast cancer.
Recognizing the health benefits of dairy products, researchers have suggested providing dairy to breast cancer patients undergoing chemotherapy to promote their recovery. One clinical study offered kefir to breast cancer patients who had received chemotherapy and/or radiation within the past 2 years and found that the patients’ Beck depression inventory, total piper fatigue score, gastric distress score, intermediate monocytes, and IL-6 levels decreased significantly (p < 0.05), suggesting an improvement in the health status of patients (Smoak et al., 2021). Another study also supports the advantages of dairy consumption for breast cancer survivors. Research indicated that the intake of fermented dairy products reduced mortality and cancer recurrence risk among breast cancer survivors, and significantly improved disease-free survival (p = 0.001) and overall survival (p = 0.004). These studies collectively suggest that breast cancer patients receiving chemotherapy can greatly benefit from increased consumption of dairy products, particularly fermented dairy products.
Some scholars stated that the consumption of dairy products with differing fat contents may have varying effects on breast cancer. Low-fat dairy products are often associated with a reduced risk of breast cancer, while high-fat dairy products may negatively impact breast cancer development. Dashti et al., in a study involving 350 breast cancer patients and 700 healthy individuals, found that low-fat milk intake decreased breast cancer risk, whereas high-fat milk intake increased it (Dashti et al., 2022). Similarly, Shin et al. reported that high intake of low-fat dairy products, especially skim/low-fat milk, was associated with a reduced risk of breast cancer, while the consumption of high-fat dairy food and whole milk exhibited statistically nonsignificant inverse associations with premenopausal breast cancer risk (Shin et al., 2002). Further, the type of dairy product also affects the risk of breast cancer. Yogurt, fermented milk, and certain types of cheeses may be more effective than milk in reducing the risk of breast cancer. A study involving 64,904 Norwegian women, and 218 premenopausal and 1,189 postmenopausal breast cancer patients, showed that premenopausal women with the highest consumption of white cheese had a 50% lower risk of breast cancer compared to those with the lowest dairy consumption. However, other dairy intake did not show a positive or negative association with breast cancer risk in both premenopausal or postmenopausal women (Hjårtaker et al., 2010). It has also been suggested that increased yogurt intake reduces breast cancer risk, while higher consumption of American cheese, cheddar cheese, and cream cheese may increase it (McCann et al., 2017). Similarly, Maliou et al. also revealed that only the consumption of fresh cheese was associated with a reduced breast cancer risk in a study involving 184 breast cancer patients and an equal number of age-matched controls (Maliou et al., 2018). Generally, premenopausal intake of dairy products is more effective in intervening in breast cancer progression than postmenopausal intake of dairy products. For example, a case study involving 1,699 women (26–79 years of age, including 823 cancer cases and 876 randomly selected controls) indicated that higher consumption of dairy products significantly decreased breast cancer risk, but only among premenopausal women (Wajszczyk et al., 2021). This is further supported by Shin et al., who found that high low-fat dairy product intake in premenopausal women, especially skim/low-fat milk, was associated with a reduced risk of breast cancer (Shin et al., 2002).
However, several studies also claim that breast cancer risk is not associated with dairy product intake. Two of these studies involving large samples (a 15-year follow-up study of 351,041 women with 7,379 breast cancer patients, and an 8.8-year follow-up study of 319,826 women with 7,119 breast cancer patients) concluded that there was no significant association between dairy product intake and increased breast cancer risk (Missmer et al., 2002; Pala et al., 2009). Furthermore, other two studies, one involving 4,697 initially cancer-free women (88 women were eventually diagnosed with breast cancer), and another including 438 breast cancer cases and 438 controls, yielded similar results, both indicating no significant association between dairy intake and breast cancer risk. Interestingly, contrary results were reported in a study, which reported that high consumption of milk increased the risk of breast cancer by 7.2 times, but this study had a relatively small sample size, involving only 97 patients diagnosed with breast cancer and 104 controls (Galván-Salazar et al., 2015). Collectively, studies supporting the view that dairy intake has no negative effect on breast cancer are in the majority.
6 Conclusion, limitations, and future perspectives
Dairy products and dairy-derived peptides have exhibited notable advantages in the administration of breast cancer, such as high nutritional value, desirable efficacy, low side effects, and high compliance, which have garnered increasing scholarly attention. Different dairy product forms, containing dairy protein-derived peptides, dairy products, and dairy-protein based nanoparticles, have demonstrated anti-breast cancer proliferation, migration and invasion, pro-apoptotic and cell cycle blocking effects in both in vivo and in vitro models. Clinical trials further suggest that dairy intake is probably associated with reduced risk of breast cancer. This review provides ideas for future research and industrial application of dairy-derived peptides, dairy products and dairy-protein based nanoparticles in the fight against breast cancer. Specifically, from the perspective of the food industry, this study promotes the development of anticancer functional foods; from the perspective of nanomaterials field, this review points out problems that exist in the dairy protein-based nanomaterials field and guides new research directions for synthesizing high-performance materials; from the perspective of medical field, milk protein-derived peptides, fermented dairy products, and milk protein nanoparticles meet the patient’s needs for natural medicines that are highly efficacious, have fewer side effects, and are less expensive, presenting a promising avenue for cancer adjuvant therapy. However, due to a lack of relevant research, this review neglected to summarize the anti-breast cancer effects of less common dairy protein-derived peptides and dairy products (e.g., horse milk-derived peptides, buffalo milk yogurt, mozzarella cheese, and cheddar cheese), nor did it provide a deep discussion of the anticancer mechanisms of certain dairy products.
To address these gaps, we propose focusing on the following issues:
1. Exploration of alternative dairy sources: Deer milk, horse milk, and donkey milk may show greater anti-breast cancer effects and nutritional value. Isolating anticancer active peptides from these dairy products by enzymatic hydrolysis or fermentation is a promising research direction.
2. Active peptide identification: Fermented milk, yogurt and different types of cheese contain a large number of active peptides. Identifying highly anticancer active peptides and examining the relationship between their primary and secondary structures and anti-breast cancer activity will promote the development of small molecule peptide functional foods.
3. Mechanistic studies: The underlying mechanisms of certain dairy-derived peptides and dairy protein-based nanoparticles in combating breast cancer are not yet well understood and need further investigation. Addressing these issues will facilitate the production of functional dairy products with anticancer properties and promote their application in clinical settings.
Author contributions
DZ: Investigation, Software, Validation, Writing–original draft. YY: Resources, Writing–review and editing. JX: Investigation, Writing–review and editing. QZ: Writing–review and editing. YG: Writing–review and editing. KJ: Writing–review and editing. NX: Funding acquisition, Project administration, Supervision, Writing–review and editing.
Funding
The author(s) declare that financial support was received for the research, authorship, and/or publication of this article. This work was supported by National Natural Science Foundation of China (No. 82172356, No.82203559), Natural Science Foundation of Shenzhen, China (JCYJ20230807115112024) and Guangdong Basic and Applied Basic Research Foundation (2023A1515220238).
Conflict of interest
The authors declare that the research was conducted in the absence of any commercial or financial relationships that could be construed as a potential conflict of interest.
Publisher’s note
All claims expressed in this article are solely those of the authors and do not necessarily represent those of their affiliated organizations, or those of the publisher, the editors and the reviewers. Any product that may be evaluated in this article, or claim that may be made by its manufacturer, is not guaranteed or endorsed by the publisher.
References
Abdel-Hamid, M., Romeih, E., Gamba, R. R., Nagai, E., Suzuki, T., Koyanagi, T., et al. (2019). The biological activity of fermented milk produced by Lactobacillus casei ATCC 393 during cold storage. Int. Dairy J. 91, 1–8. doi:10.1016/j.idairyj.2018.12.007
Abdelrazik, T., and Elshaghabee, F. M. F. (2021). Enhancement of selected health benefits in fermented cow and soy milk supplemented with water soluble curcumin. Curr. Res. Nutr. Food Sci. 9 (3), 961–969. doi:10.12944/crnfsj.9.3.23
Abu-Serie, M. M., and El-Fakharany, E. M. (2017). Efficiency of novel nanocombinations of bovine milk proteins (lactoperoxidase and lactoferrin) for combating different human cancer cell lines. Sci. Rep. 7, 16769. doi:10.1038/s41598-017-16962-6
Adjonu, R., Doran, G., Torley, P., and Agboola, S. (2023). Stability of whey protein bioactive peptide-stabilised nanoemulsions: effect of pH, ions, heating and freeze-thawing. Int. J. Food Sci. Technol. 58 (4), 1787–1794. doi:10.1111/ijfs.16292
Akilli, A. A., Erkin, Ö., Aydemir, L. Y., and Erbay, Z. (2023). Variation of bioactive potentials during the production of enzyme-modified cheese. Int. Dairy J. 147, 105788. doi:10.1016/j.idairyj.2023.105788
Alhaj, O., and Kanekanian, A. D. (2014). “Milk-derived bioactive components from fermentation,” in Milk and dairy products as functional foods, 237–288.
Ali, O. M., Bekhit, A. A., Khattab, S. N., Helmy, M. W., Abdel-Ghany, Y. S., Teleb, M., et al. (2020). Synthesis of lactoferrin mesoporous silica nanoparticles for pemetrexed/ellagic acid synergistic breast cancer therapy. Colloids Surfaces B-Biointerfaces 188, 110824. doi:10.1016/j.colsurfb.2020.110824
Al-Quwaie, D. A., Allohibi, A., Aljadani, M., Alghamdi, A. M., Alharbi, A. A., Baty, R. S., et al. (2023). Characterization of Portulaca oleracea whole plant: evaluating antioxidant, anticancer, antibacterial, and antiviral activities and application as quality enhancer in yogurt. Molecules 28 (15), 5859. doi:10.3390/molecules28155859
Anagnostopoulos, F., and Myrgianni, S. (2009). Body image of Greek breast cancer patients treated with mastectomy or breast conserving surgery. J. Clin. Psychol. Med. Settings 16 (4), 311–321. doi:10.1007/s10880-009-9176-5
Arafat, H. M., Omar, J., Shafii, N., Naser, I. A., Al Laham, N. A., Muhamad, R., et al. (2023). The association between breast cancer and consumption of dairy products: a systematic review. Ann. Med. 55 (1), 2198256. doi:10.1080/07853890.2023.2198256
Arias, M., Hilchie, A. L., Haney, E. F., Bolscher, J. G. M., Hyndman, M. E., Hancock, R. E. W., et al. (2017). Anticancer activities of bovine and human lactoferricin-derived peptides. Biochem. Cell Biol. 95 (1), 91–98. doi:10.1139/bcb-2016-0175
Arslan, S. (2015). A review: chemical, microbiological and nutritional characteristics of kefir. Cyta-Journal Food 13 (3), 340–345. doi:10.1080/19476337.2014.981588
Aryana, K. J., and Olson, D. W. (2017). A 100-Year Review: yogurt and other cultured dairy products. J. Dairy Sci. 100 (12), 9987–10013. doi:10.3168/jds.2017-12981
Atallah, M. A., Sallam, M. A., Abdelmoneem, M. A., Teleb, M., Elkhodairy, K. A., Bekhit, A. A., et al. (2022). Green self-assembled lactoferrin carboxymethyl cellulose nanogels for synergistic chemo/herbal breast cancer therapy. Colloids Surfaces B-Biointerfaces 217, 112657. doi:10.1016/j.colsurfb.2022.112657
Auestad, N., and Layman, D. K. (2021). Dairy bioactive proteins and peptides: a narrative review. Nutr. Rev. 79, 36–47. doi:10.1093/nutrit/nuab097
Aval, S. D., Zibaei, S., Ramshini, H., Rahbarian, R., and Chamani, J. (2021). Loading lactoferrin drived from Camel milk on the pectin nanoparticles and studying the its effect on the MCF-7 cancer cell line. J. Res. Agric. Animal Sci. 8 (5), 36–43.
Ayyash, M., Abdalla, A., Alameri, M., Baig, M. A., Kizhakkayil, J., Chen, G., et al. (2021). Biological activities of the bioaccessible compounds after in vitro digestion of low-fat Akawi cheese made from blends of bovine and camel milk. J. Dairy Sci. 104 (9), 9450–9464. doi:10.3168/jds.2021-20438
Ayyash, M., Al-Nuaimi, A. K., Al-Mahadin, S., and Liu, S. Q. (2018). In vitro investigation of anticancer and ACE-inhibiting activity, α-amylase and α-glucosidase inhibition, and antioxidant activity of camel milk fermented with camel milk probiotic: a comparative study with fermented bovine milk. Food Chem. 239, 588–597. doi:10.1016/j.foodchem.2017.06.149
Azizi, N. F., Kumar, M. R., Yeap, S. K., Abdullah, J. O., Khalid, M., Omar, A. R., et al. (2021). Kefir and its biological activities. Foods 10 (6), 1210. doi:10.3390/foods10061210
Bahadoran, Z., Karimi, Z., Houshiar-rad, A., Mirzayi, H.-R., and Rashidkhani, B. (2013). Is dairy intake associated to breast cancer? A case control study of Iranian women. Nutr. Cancer. 65 (8), 1164–1170. doi:10.1080/01635581.2013.828083
Barick, K. C., Tripathi, A., Dutta, B., Shelar, S. B., and Hassan, P. A. (2021). Curcumin encapsulated casein nanoparticles: enhanced bioavailability and anticancer efficacy. J. Pharm. Sci. 110 (5), 2114–2120. doi:10.1016/j.xphs.2020.12.011
Barragán-Cárdenas, A., Urrea-Pelayo, M., Niño-Ramírez, V. A., Umaña-Pérez, A., Vernot, J. P., Parra-Giraldo, C. M., et al. (2020). Selective cytotoxic effect against the MDA-MB-468 breast cancer cell line of the antibacterial palindromic peptide derived from bovine lactoferricin. Rsc Adv. 10 (30), 17593–17601. doi:10.1039/d0ra02688c
Bernabucci, U., Catalani, E., Basiricò, L., Morera, P., and Nardone, A. (2014). In vitro ACE-inhibitory activity and in vivo antihypertensive effects of water-soluble extract by Parmigiano Reggiano and Grana Padano cheeses. Int. Dairy J. 37 (1), 16–19. doi:10.1016/j.idairyj.2014.02.009
Biffi, A., Coradini, D., Larsen, R., Riva, L., and DiFronzo, G. (1997). Antiproliferative effect of fermented milk on the growth of a human breast cancer cell line. Nutr. Cancer-an Int. J. 28 (1), 93–99. doi:10.1080/01635589709514558
Bintsis, T., and Papademas, P. (2022). The evolution of fermented milks, from artisanal to industrial products: a critical review. Fermentation-Basel 8 (12), 679. doi:10.3390/fermentation8120679
Bittante, G., Amalfitano, N., Bergamaschi, M., Patel, N., Haddi, M. L., Benabid, H., et al. (2022). Composition and aptitude for cheese-making of milk from cows, buffaloes, goats, sheep, dromedary camels, and donkeys. J. Dairy Sci. 105 (3), 2132–2152. doi:10.3168/jds.2021-20961
Borghei, Y. S., and Hosseinkhani, S. (2022). Bio-synthesis of a functionalized whey proteins theranostic nanoprobe with cancer-specific cytotoxicity and as a live/dead cell imaging probe. J. Photochem. Photobiol. a-Chemistry 431, 114025. doi:10.1016/j.jphotochem.2022.114025
Çakmakoglu, S. K., Dere, S., Bekiroglu, H., Bozkurt, F., Karasu, S., Dertli, E., et al. (2024). Production of bioactive peptides during yogurt fermentation, their extraction and functional characterization. Food Biosci. 61, 104805. doi:10.1016/j.fbio.2024.104805
Cámara, M., Giner, R. M., González-Fandos, E., López-García, E., Mañes, J., Portillo, M. P., et al. (2021). Food-based dietary guidelines around the world: a comparative analysis to update aesan scientific committee dietary recommendations. Nutrients 13 (9), 3131. doi:10.3390/nu13093131
Casanova, Y. V., Guerra, J. A. R., Pérez, Y. A. U., Castro, A. L. L., Reina, G. A., Castañeda, J. E. G., et al. (2017). Antibacterial synthetic peptides derived from bovine lactoferricin exhibit cytotoxic effect against MDA-MB-468 and MDA-MB-231 breast cancer cell lines. Molecules 22 (10), 1641. doi:10.3390/molecules22101641
Chaudhari, R., Patel, V., and Kumar, A. (2024). Cutting-edge approaches for targeted drug delivery in breast cancer: beyond conventional therapies. Nanoscale Adv. 6 (9), 2270–2286. doi:10.1039/d4na00086b
Chen, C., Chan, H. M., and Kubow, S. (2007). Kefir extracts suppress in vitro proliferation of estrogen-dependent human breast cancer cells but not normal mammary epithelial cells. J. Med. Food 10 (3), 416–422. doi:10.1089/jmf.2006.236
Chen, C., Chan, H. M., and Kubow, S. (2011). Fractionation and characterization of bioactive components in kefir mother culture that inhibit proliferation of cultured MCF-7. Human breast-cancer cells. Breast Cancer - Curr. Altern. Ther., 149–172. doi:10.5772/22474
Chen, C., Zhao, S. S., Hao, G. F., Yu, H. Y., Tian, H. X., and Zhao, G. Z. (2017). Role of lactic acid bacteria on the yogurt flavour: a review. Int. J. Food Prop. 20, S316–S330. doi:10.1080/10942912.2017.1295988
Chen, G. Q., Qu, Y. R., Gras, S. L., and Kentish, S. E. (2023). Separation technologies for whey protein fractionation. Food Eng. Rev. 15 (3), 438–465. doi:10.1007/s12393-022-09330-2
Chen, P., Liu, L., Zhang, X. X., Bora, A. F. M., Li, X. D., Zhao, M. Q., et al. (2019). Antioxidant activity of Cheddar cheese during its ripening time and after simulated gastrointestinal digestion as affected by probiotic bacteria. Int. J. Food Prop. 22 (1), 218–229. doi:10.1080/10942912.2019.1579836
Contreras, M. D., Sanchez, D., Sevilla, M. A., Recio, I., and Amigo, L. (2013). Resistance of casein-derived bioactive peptides to simulated gastrointestinal digestion. Int. Dairy J. 32 (2), 71–78. doi:10.1016/j.idairyj.2013.05.008
Corso, C. R., de Oliveira, N. M. T., Cordeiro, L. M., da Silva, K. S., Soczek, S. H. D., Rossato, V. F., et al. (2021). Polysaccharides with antitumor effect in breast cancer: a systematic review of non-clinical studies. Nutrients 13 (6), 2008. doi:10.3390/nu13062008
Cui, Q., Zhang, Z. Q., Li, M. L., Zhou, M., and Sun, X. M. (2023). Peptide profiles and allergy-reactivity of extensive hydrolysates of milk protein. Food Chem. 411, 135544. doi:10.1016/j.foodchem.2023.135544
Dashti, F., Soltani, S., Benisi-Kohansal, S., Azadbakht, L., and Esmaillzadeh, A. (2022). Consumption of dairy products and odds of breast cancer: an Iranian case-control study. Breast Cancer 29 (2), 352–360. doi:10.1007/s12282-021-01317-x
Dave, B., Eason, R. R., Geng, Y., Su, Y., Badger, T. M., and Simmen, R. C. M. (2006). Tp53-associated growth arrest and DNA damage repair gene expression is attenuated in mammary epithelial cells of rats fed whey proteins. J. Nutr. 136 (5), 1156–1160. doi:10.1093/jn/136.5.1156
de LeBlanc, A. D., Matar, C., Farnworth, E., and Perdigon, G. (2006). Study of cytokines involved in the prevention of a murine experimental breast cancer by kefir. Cytokine 34 (1-2), 1–8. doi:10.1016/j.cyto.2006.03.008
de LeBlanc, A. D., Matar, C., LeBlanc, N., and Perdigón, G. (2005a). Effects of milk fermented by Lactobacillus helveticus R389 on a murine breast cancer model. Breast Cancer Res. 7 (4), R477–R486. doi:10.1186/bcr1032
de LeBlanc, A. D., Matar, C., Thériault, C., and Perdigón, G. (2005b). Effects of milk fermented by Lactobacillus helveticus R389 on immune cells associated to mammary glands in normal and a breast cancer model. Immunobiology 210 (5), 349–358. doi:10.1016/j.imbio.2005.05.024
de LeBlanc, A. D. M., Matar, C., Farnworth, E., and Perdigón, G. (2007). Study of immune cells involved in the antitumor effect of kefir in a murine breast cancer model. J. Dairy Sci. 90 (4), 1920–1928. doi:10.3168/jds.2006-079
Dent, M. P., O'Hagan, S., Braun, W. H., Schaetti, P., Marburger, A., and Vogel, O. (2007). A 90-day subchronic toxicity study and reproductive toxicity studies on ACE-inhibiting lactotripeptide. Food Chem. Toxicol. 45 (8), 1468–1477. doi:10.1016/j.fct.2007.02.006
Doorten, A., vd Wiel, J. A. G., and Jonker, D. (2009). Safety evaluation of an IPP tripeptide-containing milk protein hydrolysate. Food Chem. Toxicol. 47 (1), 55–61. doi:10.1016/j.fct.2008.10.001
Eason, R. R., Till, S. R., Frank, J. A., Badger, T. M., Korourian, S., Simmen, F. A., et al. (2006). Tumor-protective and tumor-promoting actions of dietary whey proteins in an N-methyl-N-nitrosourea model of rat mammary carcinogenesis. Nutr. Cancer-an Int. J. 55 (2), 171–177. doi:10.1207/s15327914nc5502_8
Eason, R. R., Velarde, M. C., Chatman, L., Till, S. R., Geng, Y., Ferguson, M., et al. (2004). Dietary exposure to whey proteins alters rat mammary gland proliferation, apoptosis, and gene expression during postnatal development. J. Nutr. 134 (12), 3370–3377. doi:10.1093/jn/134.12.3370
El-Din, N. K. B., Shabana, S. M., Abdulmajeed, B. A., and Ghoneum, M. (2020). A novel kefir product (PFT) inhibits Ehrlich ascites carcinoma in mice via induction of apoptosis and immunomodulation. Bmc Complementary Med. Ther. 20 (1), 127. doi:10.1186/s12906-020-02901-y
Fajardo-Espinoza, F. S., Ordaz-Pichardo, C., Sankar, U., Romero-Rojas, A., Moreno-Eutimio, M. A., Hernández-Sánchez, H. J., et al. (2021). In vitro cytomodulatory and immunomodulatory effects of bovine colostrum whey protein hydrolysates. Int. J. Food Sci. and Technol. 56 (5), 2109–2121. doi:10.1111/ijfs.14767
Farhood, B., Khodamoradi, E., Hoseini-Ghahfarokhi, M., Motevaseli, E., Mirtavoos-Mahyari, H., Musa, A. E., et al. (2020). TGF-β in radiotherapy: mechanisms of tumor resistance and normal tissues injury. Pharmacol. Res. 155, 104745. doi:10.1016/j.phrs.2020.104745
Fatemi, M., Ghandehari, F., Bahrami, S., and Tajedin, N. J. (2018). In silico and in vitro studies of cytotoxic activity of different peptides derived from human lactoferrin protein. J. Kermanshah Univ. Med. Sci. 22 (1). doi:10.5812/jkums.69544
Feeney, E. L., Lamichhane, P., and Sheehan, J. J. (2021). The cheese matrix: understanding the impact of cheese structure on aspects of cardiovascular health - a food science and a human nutrition perspective. Int. J. Dairy Technol. 74 (4), 656–670. doi:10.1111/1471-0307.12755
Fomin, A. S., Koval, O. A., Semenov, D. V., Potapenko, M. O., Kuligina, E. V., Kit, Y. Y., et al. (2012). Analysis of biochemical markers of MCF-7 cell apoptosis induced by a recombinant analogue of lactaptin. Russ. J. Bioorg. Chem. 38 (1), 77–82. doi:10.1134/s1068162012010086
Fraser, G. E., Jaceldo-Siegl, K., Orlich, M., Mashchak, A., Sirirat, R., and Knutsen, S. (2020). Dairy, soy, and risk of breast cancer: those confounded milks. Int. J. Epidemiol. 49 (5), 1526–1537. doi:10.1093/ije/dyaa007
Furlong, S. J., Mader, J. S., and Hoskin, D. W. (2006). Lactoferricin-induced apoptosis in estrogen-nonresponsive MDA-MB-435 breast cancer cells is enhanced by C 6 ceramide or tamoxifen. Oncol. Rep. 15 (5), 1385–1390. doi:10.3892/or.15.5.1385
Galván-Salazar, H. R., Arreola-Cruz, A., Madrigal-Pérez, D., Soriano-Hernández, A. D., Guzman-Esquivel, J., Montes-Galindo, D. A., et al. (2015). Association of milk and meat consumption with the development of breast cancer in a western Mexican population. Breast Care 10 (6), 393–396. doi:10.1159/000442230
Gandhi, S., and Roy, I. (2019). Doxorubicin-loaded casein nanoparticles for drug delivery: preparation, characterization and in vitro evaluation. Int. J. Biol. Macromol. 121, 6–12. doi:10.1016/j.ijbiomac.2018.10.005
Gao, J., Mao, Y. Z., Xiang, C. Y., Cao, M. N., Ren, G. R., Wang, K. W., et al. (2021). Preparation of β-lactoglobulin/gum Arabic complex nanoparticles for encapsulation and controlled release of EGCG in simulated gastrointestinal digestion model. Food Chem. 354, 129516. doi:10.1016/j.foodchem.2021.129516
Gärtner, R., Jensen, M.-B., Nielsen, J., Ewertz, M., Kroman, N., and Kehlet, H. (2009). Prevalence of and factors associated with persistent pain following breast cancer surgery. JAMA. 302 (18), 1985–1992. doi:10.1001/jama.2009.1568
Gruden, S., and Ulrih, N. P. (2021). Diverse mechanisms of antimicrobial activities of lactoferrins, lactoferricins, and other lactoferrin-derived peptides. Int. J. Mol. Sci. 22 (20), 11264. doi:10.3390/ijms222011264
Gu, H. F., Liang, L., Zhu, Z. W., and Mao, X. Y. (2022). Preparation and identification of anti-breast cancer cells peptides released from yak milk casein. Front. Nutr. 9, 997514. doi:10.3389/fnut.2022.997514
Gu, H. F., Mao, X. Y., and Du, M. (2020). Prevention of breast cancer by dietary polyphenols-role of cancer stem cells. Crit. Rev. Food Sci. Nutr. 60 (5), 810–825. doi:10.1080/10408398.2018.1551778
Guerra, J. R., Cárdenas, A. B., Ochoa-Zarzosa, A., Meza, J. L., Perez, A., Fierro-Medina, R., et al. (2019). The tetrameric peptide LfcinB (20-25) 4 derived from bovine lactoferricin induces apoptosis in the MCF-7 breast cancer cell line. Rsc Adv. 9 (36), 20497–20504. doi:10.1039/c9ra04145a
Hadjimbei, E., Botsaris, G., and Chrysostomou, S. (2022). Beneficial effects of yoghurts and probiotic fermented milks and their functional food potential. Foods 11 (17), 2691. doi:10.3390/foods11172691
Hasegawa, Y., and Bolling, B. W. (2023). Yogurt consumption for improving immune health. Curr. Opin. Food Sci. 51, 101017. doi:10.1016/j.cofs.2023.101017
Hassan, A. A. M., Elenany, Y. E., Nassrallah, A., Cheng, W. W., and Abd El-Maksoud, A. A. (2022). Royal jelly improves the physicochemical properties and biological activities of fermented milk with enhanced probiotic viability. Lwt-Food Sci. Technol. 155, 112912. doi:10.1016/j.lwt.2021.112912
Hirahatake, K. M., Astrup, A., Hill, J. O., Slavin, J. L., Allison, D. B., and Maki, K. C. (2020). Potential cardiometabolic health benefits of full-fat dairy: the evidence base. Adv. Nutr. 11 (3), 533–547. doi:10.1093/advances/nmz132
Hjårtaker, A., Thoresen, M., Engeset, D., and Lund, E. (2010). Dairy consumption and calcium intake and risk of breast cancer in a prospective cohort: the Norwegian Women and Cancer study. Cancer Causes and Control 21 (11), 1875–1885. doi:10.1007/s10552-010-9615-5
HoSoNo, A., Otani, H., Yasui, H., and Watanuki, M. (2002). Impact of fermented milk on human health: cholesterol-lowering and immunomodulatory properties of fermented milk. Animal Sci. J. 73 (4), 241–256. doi:10.1046/j.1344-3941.2002.00034.x
Hutchins, D., and Hurley, R. A. (2024). A systematic review of articles influencing United States retail cheese packaging, labeling, and market trends related to cheese in the marketplace and cheese during consumption. J. Dairy Sci. S0022-0302 (24), 00724–730. doi:10.3168/jds.2023-23977
Ibrahim, H. R., Ahmed, A. S., Komeda, A., and Miyata, T. (2022). Lactophorin in camel milk undergoing specific proteolysis and exhibiting potent anticancer action against human colon and breast cancer cells through ROS generation. Avicenna J. Med. Biochem. 10 (1), 1–12. doi:10.34172/ajmb.2022.01
Ilesanmi-Oyelere, B. L., and Kruger, M. C. (2020). The role of milk components, pro-pre-and synbiotic foods in calcium absorption and bone health maintenance. Front. Nutr. 7, 578702. doi:10.3389/fnut.2020.578702
Insuasty-Cepeda, D. S., Barragán-Cárdenas, A. C., Ochoa-Zarzosa, A., López-Meza, J. E., Fierro-Medina, R., García-Castañeda, J. E., et al. (2020). Peptides derived from (RRWQWRMKKLG) 2-K-Ahx induce selective cellular death in breast cancer cell lines through apoptotic pathway. Int. J. Mol. Sci. 21 (12), 4550. doi:10.3390/ijms21124550
Jabbari, M., Barati, M., Shabani, M., Kazemian, E., Khalili-Moghadam, S., Javanmardi, F., et al. (2022). The association between consumption of dairy-originated digestion resistant and bioactive peptides and breast cancer risk: a case-control study. Nutr. Cancer-an Int. J. 74 (7), 2426–2435. doi:10.1080/01635581.2021.2009884
Jiang, C., Liu, L., Li, X. D., Ma, L. Y., Du, L. L., Zhao, Y. B., et al. (2018). Separation and purification of hypocholesterolaemic peptides from whey protein and their stability under simulated gastrointestinal digestion. Int. J. Dairy Technol. 71 (2), 460–468. doi:10.1111/1471-0307.12453
Johanson, B., Virtanen, A. I., Tweit, R. C., and Dodson, R. M. (1960). Isolation of an iron-containing red protein from human milk. Acta Chem. Scand. 14, 510–512. doi:10.3891/acta.chem.scand.14-0510
Joudeh, N., and Linke, D. (2022). Nanoparticle classification, physicochemical properties, characterization, and applications: a comprehensive review for biologists. J. Nanobiotechnology 20 (1), 262. doi:10.1186/s12951-022-01477-8
Kampa, M., Loukas, S., Hatzoglou, A., Martin, P., Martin, P.-M., and Castanas, E. (1996). Identification of a novel opioid peptide (Tyr-Val-Pro-Phe-Pro) derived from human αS1 casein (αS1-casomorphin, and αS1-casomorphin amide). Biochem. J. 319 (3), 903–908. doi:10.1042/bj3190903
Kanwar, J. R., Kamalapuram, S. K., Krishnakumar, S., and Kanwar, R. K. (2016). Multimodal iron oxide (Fe3O4)-saturated lactoferrin nanocapsules as nanotheranostics for real-time imaging and breast cancer therapy of claudin-low, triple-negative (ER-/PR-/HER2-). Nanomedicine 11 (3), 249–268. doi:10.2217/nnm.15.199
Keum, N., and Giovannucci, E. (2019). Global burden of colorectal cancer: emerging trends, risk factors and prevention strategies. Nat. Rev. Gastroenterology and Hepatology 16 (12), 713–732. doi:10.1038/s41575-019-0189-8
Khan, A., Wang, C. N., Sun, X. M., Killpartrick, A., and Guo, M. R. (2019). Preparation and characterization of whey protein isolate-DIM nanoparticles. Int. J. Mol. Sci. 20 (16), 3917. doi:10.3390/ijms20163917
Khan, A. R., and Taneja, P. (2015). Cationic peptide lactoferricin B inhibits glutathione s-transferase P1 from human placenta and breast cancer cell line MDA-MB-231 preventing anticancer drug metabolism. Int. J. Pharm. Pharm. Sci. 7, 238–241.
Khatun, S., Pebam, M., Putta, C. L., and Rengan, A. K. (2023). Camptothecin loaded casein nanosystem for tuning the therapeutic efficacy against highly metastatic triple-negative breast cancer cells. Biomaterials Sci. 11 (7), 2518–2530. doi:10.1039/d2bm01814d
Khatun, S., Pebam, M., Sankaranarayanan, S. A., Pogu, S. V., Bantal, V. S., and Rengan, A. K. (2024). Glutathione - IR 797 coupled Casein Nano-Trojan for augmenting the therapeutic efficacy of camptothecin in highly invasive triple negative breast cancer. Biomater. Adv. 159, 213802. doi:10.1016/j.bioadv.2024.213802
Knekt, P., Jarvinen, R., Seppanen, R., Pukkala, E., and Aromaa, A. (1996). Intake of dairy products and the risk of breast cancer. Br. J. Cancer 73 (5), 687–691. doi:10.1038/bjc.1996.119
Kowalczyk, P., Kaczynska, K., Kleczkowska, P., Bukowska-Osko, I., Kramkowski, K., and Sulejczak, D. (2022). The lactoferrin phenomenon-A miracle molecule. Molecules 27 (9), 2941. doi:10.3390/molecules27092941
Kreider, R. B., Iosia, M., Cooke, M., Hudson, G., Rasmussen, C., Chen, H., et al. (2011). Bioactive properties and clinical safety of a novel milk protein peptide. Nutr. J. 10, 99. doi:10.1186/1475-2891-10-99
Kumar, D., Chatli, M. K., Singh, R., Mehta, N., and Kumar, P. (2016). Enzymatic hydrolysis of camel milk casein and its antioxidant properties. Dairy Sci. and Technol. 96 (3), 391–404. doi:10.1007/s13594-015-0275-9
Leischner, C., Egert, S., Burkard, M., and Venturelli, S. (2021). Potential protective protein components of cow's milk against certain tumor entities. Nutrients 13 (6), 1974. doi:10.3390/nu13061974
Liu, Q. G., Sun, Y. X., Cheng, J. J., and Guo, M. R. (2022). Development of whey protein nanoparticles as carriers to deliver soy isoflavones. Lwt-Food Sci. Technol. 155, 112953. doi:10.1016/j.lwt.2021.112953
Luo, W., Bai, L. Y., Zhang, J., Li, Z. W., Liu, Y. N., Tang, X. Y., et al. (2023). Polysaccharides-based nanocarriers enhance the anti-inflammatory effect of curcumin. Carbohydr. Polym. 311, 120718. doi:10.1016/j.carbpol.2023.120718
Maliou, D., Belmadi, D., Saadi, W., Mahfouf, H., Benzidane, N., and Bitam, A. (2018). Effect of dairy products intake on breast cancer risk: a case-control study in Algeria. Nutr. Clinique Metabolisme 32 (3), 187–194. doi:10.1016/j.nupar.2018.04.001
Mangano, K. M., Bao, Y., and Zhao, C. J. (2019). Whey protein production, chemistry, functionality, and applications. Nutr. Prop. whey proteins, 103–140. doi:10.1002/9781119256052
Marangoni, F., Pellegrino, L., Verduci, E., Ghiselli, A., Bernabei, R., Calvani, R., et al. (2019). Cow's milk consumption and health: a health professional's guide. J. Am. Coll. Nutr. 38 (3), 197–208. doi:10.1080/07315724.2018.1491016
Marquette, C., and Nabell, L. (2012). Chemotherapy-Resistant metastatic breast cancer. Curr. Treat. Options Oncol. 13 (2), 263–275. doi:10.1007/s11864-012-0184-6
Martins, V. F. R., Pintado, M. E., Morais, R., and Morais, A. (2023). Valorisation of micro/nanoencapsulated bioactive compounds from plant sources for food applications towards sustainability. Foods 12 (1), 32. doi:10.3390/foods12010032
McCann, S. E., Hays, J., Baumgart, C. W., Weiss, E. H., Yao, S., and Ambrosone, C. B. (2017). Usual consumption of specific dairy foods is associated with breast cancer in the roswell park cancer institute data bank and BioRepository. Curr. Dev. Nutr. 1 (3), e000422. doi:10.3945/cdn.117.000422
McCullough, M. L., Rodriguez, C., Diver, W. R., Feigelson, H. S., Stevens, V. L., Thun, M. J., et al. (2005). Dairy, calcium, and vitamin D intake and postmenopausal breast cancer risk in the cancer prevention study II nutrition cohort. Cancer Epidemiol. Biomarkers and Prev. 14 (12), 2898–2904. doi:10.1158/1055-9965.Epi-05-0611
Mehra, R., Kumar, H., Kumar, N., Ranvir, S., Jana, A., Buttar, H. S., et al. (2021). Whey proteins processing and emergent derivatives: an insight perspective from constituents, bioactivities, functionalities to therapeutic applications. J. Funct. Foods 87, 104760. doi:10.1016/j.jff.2021.104760
Mihic, T., Rainkie, D., Wilby, K. J., and Pawluk, S. A. (2016). The therapeutic effects of camel milk: a systematic review of animal and human trials. J. Evidence-Based Integr. Med. 21 (4), NP110–NP126. doi:10.1177/2156587216658846
Missmer, S. A., Smith-Warner, S. A., Spiegelman, D., Yaun, S. S., Adami, H. O., Beeson, W. L., et al. (2002). Meat and dairy food consumption and breast cancer: a pooled analysis of cohort studies. Int. J. Epidemiol. 31 (1), 78–85. doi:10.1093/ije/31.1.78
Mushtaq, M., Gani, A., and Masoodi, F. A. (2019). Himalayan cheese (Kalari/Kradi) fermented with different probiotic strains: in vitro investigation of nutraceutical properties. Lwt-Food Sci. Technol. 104, 53–60. doi:10.1016/j.lwt.2019.01.024
Olson, D. W., and Aryana, K. J. (2022). Probiotic incorporation into yogurt and various novel yogurt-based products. Appl. Sciences-Basel 12 (24), 12607. doi:10.3390/app122412607
Oz, E., Brennan, C. S., Huppertz, T., Amarowicz, R., Khan, M. R., Elobeid, T., et al. (2023). Comparison of camel, buffalo, cow, goat, and sheep yoghurts in terms of various physicochemical, biochemical, textural and rheological properties. Int. Dairy J. 146, 105749. doi:10.1016/j.idairyj.2023.105749
Ozcan, T., and Kurdal, E. (2012). The effects of using a starter culture, lipase, and protease enzymes on ripening of Mihalic cheese. Int. J. Dairy Technol. 65 (4), 585–593. doi:10.1111/j.1471-0307.2012.00868.x
Pala, V., Krogh, V., Berrino, F., Sieri, S., Grioni, S., Tjonneland, A., et al. (2009). Meat, eggs, dairy products, and risk of breast cancer in the European Prospective Investigation into Cancer and Nutrition (EPIC) cohort. Am. J. Clin. Nutr. 90 (3), 602–612. doi:10.3945/ajcn.2008.27173
Parvarei, M. M., and Mortazavian, A. M. (2022). Cytotoxic effect of paraprobiotic yogurts on cancer cell lines. Indian J. Microbiol. Res. 9, 106–113. doi:10.18231/j.ijmr.2022.019
Phelan, M., Aherne, A., FitzGerald, R. J., and O'Brien, N. M. (2009). Casein-derived bioactive peptides: biological effects, industrial uses, safety aspects and regulatory status. Int. Dairy J. 19 (11), 643–654. doi:10.1016/j.idairyj.2009.06.001
Quirós, A., Contreras, M. D., Ramos, M., Amigo, L., and Recio, I. (2009). Stability to gastrointestinal enzymes and structure-activity relationship of β-casein-peptides with antihypertensive properties. Peptides 30 (10), 1848–1853. doi:10.1016/j.peptides.2009.06.031
Rachid, M., Matar, C., Duarte, J., and Perdigon, G. (2006). Effect of milk fermented with a Lactobacillus helveticus R389(+) proteolytic strain on the immune system and on the growth of 471 breast cancer cells in mice. Fems Immunol. Med. Microbiol. 47 (2), 242–253. doi:10.1111/j.1574-695X.2006.00088.x
Rahman, R., Fonseka, A. D., Sua, S. C., Ahmad, M., Rajendran, R., Ambu, S., et al. (2021). Inhibition of breast cancer xenografts in a mouse model and the induction of apoptosis in multiple breast cancer cell lines by lactoferricin B peptide. J. Cell. Mol. Med. 25 (15), 7181–7189. doi:10.1111/jcmm.16748
Ritota, M., Comitato, R., and Manzi, P. (2022). Cow and Ewe cheeses made with saffron: characterization of bioactive compounds and their antiproliferative effect in cervical adenocarcinoma (HeLa) and breast cancer (MDA-MB-231) cells. Molecules 27 (6), 1995. doi:10.3390/molecules27061995
Roy, K., Patel, Y. S., Kanwar, R. K., Rajkhowa, R., Wang, X. G., and Kanwar, J. R. (2016). Biodegradable Eri silk nanoparticles as a delivery vehicle for bovine lactoferrin against MDA-MB-231 and MCF-7 breast cancer cells. Int. J. Nanomedicine 11, 25–44. doi:10.2147/ijn.S91810
Sah, B. N. P., Vasiljevic, T., McKechnie, S., and Donkor, O. N. (2015). Identification of anticancer peptides from bovine milk proteins and their potential roles in management of cancer: a critical review. Compr. Rev. Food Sci. Food Saf. 14 (2), 123–138. doi:10.1111/1541-4337.12126
Sahna, K. O., Cakir, B., and Tunali-Akbay, T. (2022). Antiproliferative activity of whey and casein bioactive peptides on breast cancer: an in vitro and in silico study. Int. J. Peptide Res. Ther. 28 (4), 128. doi:10.1007/s10989-022-10436-8
Sakandar, H. A., and Zhang, H. P. (2021). Trends in Probiotic(s)-Fermented milks and their in vivo functionality: a review. Trends Food Sci. and Technol. 110, 55–65. doi:10.1016/j.tifs.2021.01.054
Salah, M., Mansour, M., Zogona, D., and Xu, X. Y. (2020). Nanoencapsulation of anthocyanins-loaded β-lactoglobulin nanoparticles: characterization, stability, and bioavailability in vitro</i>. Food Res. Int. 137, 109635. doi:10.1016/j.foodres.2020.109635
Salah, M., Sallam, M. A., Abdelmoneem, M. A., Teleb, M., Elkhodairy, K. A., Bekhit, A. A., et al. (2022). Sequential delivery of novel triple drug combination via crosslinked alginate/lactoferrin nanohybrids for enhanced breast cancer treatment. Pharmaceutics 14 (11), 2404. doi:10.3390/pharmaceutics14112404
Semenov, D. V., Fomin, A. S., Kuligina, E. V., Koval, O. A., Matveeva, V. A., Babkina, I. N., et al. (2010). Recombinant analogs of a novel milk pro-apoptotic peptide, lactaptin, and their effect on cultured human cells. Protein J. 29 (3), 174–180. doi:10.1007/s10930-010-9237-5
Sharifi, M., Jafari, S., Hasan, A., Paray, B. A., Gong, G. W., Zheng, Y. Z., et al. (2020). Antimetastatic activity of lactoferrin-coated mesoporous maghemite nanoparticles in breast cancer enabled by combination therapy. Acs Biomaterials Sci. and Eng. 6 (6), 3574–3584. doi:10.1021/acsbiomaterials.0c00086
Shin, M. H., Holmes, M. D., Hankinson, S. E., Wu, K., Colditz, G. A., and Willett, W. C. (2002). Intake of dairy products, calcium, and vitamin D and risk of breast cancer. Jnci-Journal Natl. Cancer Inst. 94 (17), 1301–1311. doi:10.1093/jnci/94.17.1301
Shin, W. K., Lee, H. W., Shin, A., Lee, J. K., and Kang, D. (2020). Milk consumption decreases risk for breast cancer in Korean women under 50 Years of age: results from the health examinees study. Nutrients 12 (1), 32. doi:10.3390/nu12010032
Singh, S., Maurya, P., Rani, S., Mishra, N., Nisha, R., Singh, P., et al. (2022). Development of doxorubicin hydrochloride-loaded whey protein nanoparticles and its surface modification with N-acetyl cysteine for triple-negative breast cancer. Drug Deliv. Transl. Res. 12 (12), 3047–3062. doi:10.1007/s13346-022-01169-8
Smoak, P., Harman, N., Flores, V., Kisiolek, J., Pullen, N. A., Lisano, J., et al. (2021). Kefir is a viable exercise recovery beverage for cancer survivors enrolled in a structured exercise program. Med. Sci. Sports Exerc. 53, 2045–2053. doi:10.1249/MSS.0000000000002690
Song, Y., Wang, Z. C., Ji, H., Jiang, Z. Y., Li, X. J., Du, Z. Y., et al. (2024). Fatty acid modification of casein bioactive peptides nano-assemblies, synthesis, characterization and anticarcinogenic effect. Int. J. Biol. Macromol. 254, 127718. doi:10.1016/j.ijbiomac.2023.127718
Sprong, R. C., Schonewille, A. J., and van der Meer, R. (2010). Dietary cheese whey protein protects rats against mild dextran sulfate sodium-induced colitis: role of mucin and microbiota. J. Dairy Sci. 93 (4), 1364–1371. doi:10.3168/jds.2009-2397
Szajnar, K., Znamirowska, A., and Kuzniar, P. (2020). Sensory and textural properties of fermented milk with viability of Lactobacillus rhamnosus and Bifidobacterium animalis ssp. lactis Bb-12 and increased calcium concentration. Int. J. Food Prop. 23 (1), 582–598. doi:10.1080/10942912.2020.1748050
Taghipour, M. J., Ezzatpanah, H., and Ghahderijani, M. (2023). In vitro and in silico studies for the identification of anti-cancer and antibacterial peptides from camel milk protein hydrolysates. Plos One 18 (7), e0288260. doi:10.1371/journal.pone.0288260
Taleb, S., Soualhia, M., and Rouabhia, S. (2017). “Breast cancer: hereditary, dietary and environmental risk factors,” in 1st euro-mediterranean conference for environmental integration (EMCEI), 1965–1969.
Taniya, M. S., Reshma, M. V., Shanimol, P. S., Krishnan, G., and Priya, S. (2020). Bioactive peptides from amaranth seed protein hydrolysates induced apoptosis and antimigratory effects in breast cancer cells. Food Biosci. 35, 100588. doi:10.1016/j.fbio.2020.100588
Tavakoli, N., Divsalar, A., Haertlé, T., Sawyer, L., Saboury, A. A., and Muronetz, V. (2021). Milk protein-based nanodelivery systems for the cancer treatment. J. Nanostructure Chem. 11 (4), 483–500. doi:10.1007/s40097-021-00399-5
Tavares, T., Contreras, M. D., Amorim, M., Pintado, M., Recio, I., and Malcata, F. X. (2011). Novel whey-derived peptides with inhibitory effect against angiotensin-converting enzyme: in vitro effect and stability to gastrointestinal enzymes. Peptides 32 (5), 1013–1019. doi:10.1016/j.peptides.2011.02.005
Théolier, J., Hammami, R., Fliss, I., and Jean, J. (2014). Antibacterial and antifungal activity of water-soluble extracts from Mozzarella, Gouda, Swiss, and Cheddar commercial cheeses produced in Canada. Dairy Sci. and Technol. 94 (5), 427–438. doi:10.1007/s13594-014-0170-9
Utz, V. E. M., Visñuk, D. P., Perdigón, G., and de LeBlanc, A. D. (2021). Milk fermented by Lactobacillus casei CRL431 administered as an immune adjuvant in models of breast cancer and metastasis under chemotherapy. Appl. Microbiol. Biotechnol. 105 (1), 327–340. doi:10.1007/s00253-020-11007-x
Vantveer, P., Dekker, J. M., Lamers, J. W. J., Kok, F. J., Schouten, E. G., Brants, H. A. M., et al. (1989). Consumption of fermented milk-products and breast-cancer-A case-control study in The Netherlands. Cancer Res. 49 (14), 4020–4023.
Vishwanath-Deutsch, R., Dallas, D. C., Besada-Lombana, P., Katz, L., Conze, D., Kruger, C., et al. (2024). A review of the safety evidence on recombinant human lactoferrin for use as a food ingredient. Food Chem. Toxicol. 189, 114727. doi:10.1016/j.fct.2024.114727
Waghmare, M. N., Qureshi, T. S., Krishna, C. M., Pansare, K., Gadewal, N., Hole, A., et al. (2022). β-Lactoglobulin-gold nanoparticles interface and its interaction with some anticancer drugs - an approach for targeted drug delivery. J. Biomol. Struct. and Dyn. 40 (13), 6193–6210. doi:10.1080/07391102.2021.1879270
Wajszczyk, B., Charzewska, J., Godlewski, D., Zemla, B., Nowakowska, E., Kozaczka, M., et al. (2021). Consumption of dairy products and the risk of developing breast cancer in polish women. Nutrients 13 (12), 4420. doi:10.3390/nu13124420
Wakabayashi, H., Yamauchi, K., and Takase, M. (2006). Lactoferrin research, technology and applications. Int. Dairy J. 16 (11), 1241–1251. doi:10.1016/j.idairyj.2006.06.013
Wan, X. L., Jin, X., Xie, M. L., Liu, J., Gontcharov, A. A., Wang, H., et al. (2020). Characterization of a polysaccharide from Sanghuangporus vaninii and its antitumor regulation via activation of the p53 signaling pathway in breast cancer MCF-7 cells. Int. J. Biol. Macromol. 163, 865–877. doi:10.1016/j.ijbiomac.2020.06.279
Wang, K., and Tepper, J. E. (2021). Radiation therapy-associated toxicity: etiology, management, and prevention. Ca-a Cancer J. Clin. 71 (5), 437–454. doi:10.3322/caac.21689
Wasilewska, A., Bielicka, M., Klekotka, U., and Kalska-Szostko, B. (2023). Nanoparticle applications in food - a review. Food and Funct. 14 (6), 2544–2567. doi:10.1039/d2fo02180c
Webb, D., Donovan, S. M., and Meydani, S. N. (2014). The role of Yogurt in improving the quality of the American diet and meeting dietary guidelines. Nutr. Rev. 72 (3), 180–189. doi:10.1111/nure.12098
Wen, H. D., Zhang, D. J., Liu, J. B., Shang, X. M., Liu, X. T., Du, Z. Y., et al. (2022). Application of γ-cyclodextrin-lysozyme as host materials for encapsulation of curcumin: characterization, stability, and controlled release properties. J. Sci. Food Agric. 102 (13), 5925–5934. doi:10.1002/jsfa.11943
Wu, W., Yu, P. P., Zhang, F. Y., Che, H. X., and Jiang, Z. M. (2014). Stability and cytotoxicity of angiotensin-I-converting enzyme inhibitory peptides derived from bovine casein. J. Zhejiang University-Science B 15 (2), 143–152. doi:10.1631/jzus.B1300239
Wu, Y., Huang, R. Y., Wang, M. L., Bernstein, L., Bethea, T. N., Chen, C., et al. (2021). Dairy foods, calcium, and risk of breast cancer overall and for subtypes defined by estrogen receptor status: a pooled analysis of 21 cohort studies. Am. J. Clin. Nutr. 114 (2), 450–461. doi:10.1093/ajcn/nqab097
Wu, Y. M., Wang, Y., Ma, Z. Z., Mu, G. Q., and Qian, F. (2024). Novel insights into whey protein peptide-iron chelating agents: structural characterization, in vitro stability and functional properties. Food Biosci. 60, 104317. doi:10.1016/j.fbio.2024.104317
Wusigale, , Liang, L., and Luo, Y. C. (2020). Casein and pectin: structures, interactions, and applications. Trends Food Sci. and Technol. 97, 391–403. doi:10.1016/j.tifs.2020.01.027
Xiao, J. X., Ma, J. Y., Khan, M. Z., Alugongo, G. M., Chen, T. Y., Liu, S. A., et al. (2023). Unlocking the potential of milk whey protein components in colorectal cancer prevention and therapy. Crit. Rev. Food Sci. Nutr., 1–38. doi:10.1080/10408398.2023.2258970
Xue, H. Y., Han, J. J., He, B. Y., Yi, M. X., Liu, X. F., Song, H. X., et al. (2021). Bioactive peptide release and the absorption tracking of casein in the gastrointestinal digestion of rats. Food and Funct. 12 (11), 5157–5170. doi:10.1039/d1fo00356a
Yu, L. X., Liu, L. Y., Wang, F., Zhou, F., Xiang, Y. J., Huang, S. Y., et al. (2019). Higher frequency of dairy intake is associated with a reduced risk of breast cancer: results from a case-control study in Northern and Eastern China. Oncol. Lett. 17 (3), 2737–2744. doi:10.3892/ol.2019.9898
Zamberi, N. R., Abu, N., Mohamed, N. E., Nordin, N., Keong, Y. S., Beh, B. K., et al. (2016). The antimetastatic and antiangiogenesis effects of kefir water on murine breast cancer cells. Integr. Cancer Ther. 15 (4), NP53–NP66. doi:10.1177/1534735416642862
Zeng, X. R., Wang, Y. J., Yang, S. D., Liu, Y. J., Li, X., and Liu, D. R. (2024). The functionalities and applications of whey/whey protein in fermented foods: a review. Food Sci. Biotechnol. 33 (4), 769–790. doi:10.1007/s10068-023-01460-5
Zhang, C. X., Ho, S. C., Fu, J. H., Cheng, S. Z., Chen, Y. M., and Lin, F. Y. (2011). Dairy products, calcium intake, and breast cancer risk: a case-control study in China. Nutr. Cancer-an Int. J. 63 (1), 12–20. doi:10.1080/01635581.2010.516478
Zhang, D. (2022). Improving quality and functionality of low-fat mozzarella cheese using whey proteins and plant proteins. Pokfulam, Hong Kong: The University of Hong Kong.
Zhang, D. J., Jiang, K., Luo, H., Zhao, X. R., Yu, P., and Gan, Y. M. (2024a). Replacing animal proteins with plant proteins: is this a way to improve quality and functional properties of hybrid cheeses and cheese analogs? Compr. Rev. Food Sci. Food Saf. 23 (1), 132622–e13336. doi:10.1111/1541-4337.13262
Zhang, D. J., Lillevang, S. K., and Shah, N. P. (2021a). Influence of pre-acidification, and addition of KGM and whey protein-based fat replacers CH-4560, and YO-8075 on texture characteristics and pizza bake properties of low-fat Mozzarella cheese. Lwt-Food Sci. Technol. 137, 110384. doi:10.1016/j.lwt.2020.110384
Zhang, D. J., Ling, X. L., Jiang, K., Zhao, X. R., and Gan, Y. M. (2024b). Development of low-fat Mozzarella cheeses enriched with soy or pea protein hydrolysates: composition, texture and functional properties during ageing. Int. J. Dairy Technol. 77 (1), 165–182. doi:10.1111/1471-0307.13021
Zhang, D. J., Xiong, J., Zhao, X. R., and Gan, Y. M. (2023a). Anti-fatigue activities of γ-aminobutyric acid-enriched soymilk in an acute exercise-treated mouse model via regulating AMPK/PGC-1a pathway. Food Biosci. 55, 103060. doi:10.1016/j.fbio.2023.103060
Zhang, T. Y., Li, N. N., Wang, R., Sun, Y. Y., He, X. Y., Lu, X. Y., et al. (2023b). Enhanced therapeutic efficacy of doxorubicin against multidrug-resistant breast cancer with reduced cardiotoxicity. Drug Deliv. 30 (1), 2189118. doi:10.1080/10717544.2023.2189118
Zhang, Y. L., Nicolau, A., Lima, C. F., and Rodrigues, L. R. (2014). Bovine lactoferrin induces cell cycle arrest and inhibits mtor signaling in breast cancer cells. Nutr. Cancer-an Int. J. 66 (8), 1371–1385. doi:10.1080/01635581.2014.956260
Zhang, Y. Q., Lu, C., and Zhang, J. (2021b). Lactoferrin and its detection methods: a review. Nutrients 13 (8), 2492. doi:10.3390/nu13082492
Zhang, Z. Q., Zhang, Y., Zhang, M. Y., Yu, C. F., Yang, P. P., Xu, M. X., et al. (2023c). Food-derived peptides as novel therapeutic strategies for NLRP3 inflammasome-related diseases: a systematic review. Crit. Rev. Food Sci. Nutr., 1–32. doi:10.1080/10408398.2023.2294164
Zhao, C. H., and Ashaolu, T. J. (2020). Bioactivity and safety of whey peptides. Lwt-Food Sci. Technol. 134, 109935. doi:10.1016/j.lwt.2020.109935
Zhao, C. H., Chen, N., and Ashaolu, T. J. (2022). Whey proteins and peptides in health-promoting functions – a review. Int. Dairy J. 126, 105269. doi:10.1016/j.idairyj.2021.105269
Zheng, X. C., Shi, X. W., and Wang, B. (2021). A review on the general cheese processing technology, flavor biochemical pathways and the influence of yeasts in cheese. Front. Microbiol. 12, 703284. doi:10.3389/fmicb.2021.703284
Keywords: milk-derived peptides, breast cancer, nanoparticles, apoptosis, antibreast cancer
Citation: Zhang D, Yuan Y, Xiong J, Zeng Q, Gan Y, Jiang K and Xie N (2024) Anti-breast cancer effects of dairy protein active peptides, dairy products, and dairy protein-based nanoparticles. Front. Pharmacol. 15:1486264. doi: 10.3389/fphar.2024.1486264
Received: 25 August 2024; Accepted: 29 October 2024;
Published: 13 November 2024.
Edited by:
Donato Cosco, University of Catanzaro “Magna Graecia”, ItalyReviewed by:
Afrasim Moin, University of Hail, Saudi ArabiaAgnese Gagliardi, University Magna Graecia of Catanzaro, Italy
Copyright © 2024 Zhang, Yuan, Xiong, Zeng, Gan, Jiang and Xie. This is an open-access article distributed under the terms of the Creative Commons Attribution License (CC BY). The use, distribution or reproduction in other forums is permitted, provided the original author(s) and the copyright owner(s) are credited and that the original publication in this journal is cited, in accordance with accepted academic practice. No use, distribution or reproduction is permitted which does not comply with these terms.
*Correspondence: Ni Xie, eG4xMDBAc3p1LmVkdS5jbg==
†These authors have contributed equally to this work and share first authorship.