- 1Gansu University of Chinese Medicine, Lanzhou, China
- 2Affiliated Hospital of Gansu University of Chinese Medicine, Lanzhou, China
- 3College of Pharmacy and Health Sciences, St. John’s University, Queens, NY, United States
Regulatory immune cells regulate immune responses through various mechanisms, affecting the occurrence, development, and therapeutic effects of tumors. In this article, we reviewed the important roles of regulatory immune cells, such as regulatory T cells (Tregs), regulatory B cells (Bregs), myeloid-derived suppressor cells (MDSCs), regulatory dendritic cells (DCregs), and tumor-associated macrophages (TAMs), in the tumor microenvironment (TME). The immunomodulatory effects of natural products, such as polysaccharides, polyphenols, glycosides, alkaloids, terpenoids, quinones, and other compounds, which affect the functions of regulatory immune cells through molecular signaling pathways, thereby enhancing the potential of the antitumor immune response, are discussed. These findings provide new ideas and possibilities for the application of natural products in tumor treatment, which can help enhance the effectiveness of tumor treatment and improve patient prognosis.
Highlights
• The role of regulatory immune cells in the tumor microenvironment was comprehensively discussed.
• A comprehensive overview of the classification and sources of natural products was provided.
• The mechanisms by which natural products act on regulatory immune cells are discussed.
1 Introduction
The tumor microenvironment (TME), which is composed of cancer cells, stromal cells, and immune cells, plays an important role in tumorigenesis (Ceglie et al., 2024). Regulatory immunological cells, including regulatory T cells (Tregs), regulatory B cells (Bregs), regulatory dendritic cells (DCregs), myeloid-derived suppressor cells (MDSCs), mesenchymal stem cells (MSCs), and tumor-associated macrophages (TAMs), abundantly infiltrate tumor tissues and suppress immunity, which is often associated with poor progression in cancer patients (Farbod et al., 2022). Studies have shown that regulatory immune cells can be selectively depleted or functionally attenuated to evoke an effective antitumor immune response. Furthermore, regulatory immune cells are characterized by high levels of immune checkpoints (María et al., 2023). Thus, immune checkpoint inhibitors that block related molecules enhance the immune response and attenuate the inhibitory activity of regulatory cells (María et al., 2023). An increasing number of studies indicate that regulatory immune cells are potential targets for immunotherapy in cancer patients. In this review, we discuss the relationships among different regulatory immune cells and the molecular basis of their behavior in tumor tissues to evoke effective antitumor immunity (Figure 1).
Cancer is one of the leading causes of death worldwide. While battling this disease in China and other East Asian countries, many traditional Chinese medicines (TCMs) are remarkably effective in treating patients with cancer (Xiang et al., 2019). TCM drugs are traditionally composed of many different components, such as polysaccharides, polyphenols, glycosides, alkaloids, terpenoids, and quinones, that can target various pathways to directly inhibit the growth of cancer and change the host immune system (Li et al., 2021). We summarize the latest progress in TCM-related natural components in cancer from theory to clinical practice, which could lead to the development of sophisticated TCM and promote the development of modern medicine.
2 The classification and function of regulatory immune cells
2.1 Regulatory T cells
Tregs, a subset of CD4+ T cells, are closely related to the pathogenesis of cancer and express the transcription factor FoxP3. FoxP3 plays a major role in maintaining immunological balance and is a vital factor in the immunosuppressive TME (Liu et al., 2024). Tregs have significant phenotypic and functional heterogeneity and are classified into three categories on the basis of the expression of FoxP3 and its inhibitory effects. Stable Tregs, which express sustained FoxP3, Nrp1, and Helios, have immunosuppressive functions and constitute the main subpopulation of Tregs. Unstable Tregs, which have low expression of FoxP3 and IFN-γ, support the antitumor immune response. Fragile Tregs are functionally less suppressive in the TME, which is defined as the retention of FoxP3 expression and the secretion of IFN-γ (Abigail and Vignali Dario, 2018). Tregs can inhibit antitumor immune responses and promote tumor growth and cross-talk with type 2 macrophages (M2), MDSCs, Bregs, and natural killer (NK) cells. Tregs inhibit NK cells by releasing TGF-β, IL-10, STAT3, and VEGF to mediate the differentiation of M2 macrophages. Tregs express PD-1 receptors on their surface and release STAT3 into the TME to inhibit the immune system, which leads to immune escape from cancer cells and a reduction in antitumor immune function (Najafi et al., 2019).
2.2 Regulatory B cells
Bregs are defined as all B cells that suppress immune responses and mediate the negative regulation of immune responses; these Bregs secrete cytokines to affect antitumor immune function and cancer cell growth directly and indirectly. Based on the cytokines that they secrete, Bregs are classified into four types. CD19+ CD25hi Bregs can enhance the function of Tregs. CD24+ CD38+ Bregs express IL-10. CD19+ CD38+ CD1d+ IgM+ CD147+ Bregs express granzyme B (GrB), which is induced by IL-21. CD19+ CD24+ CD38+ Bregs exist in patients with invasive breast cancer (IBCa) (Tanja et al., 2019). Bregs exert immunomodulatory effects through cell-to-cell contact and cytokines. In the TME, Bregs inhibit effector T cells and target other tumor-infiltrating immune cells, such as Tregs, MDSCs, NK cells, and macrophages, to hinder antitumor immunity. Additionally, the cross-regulation of Bregs and tumor cells promotes cancer progression and helps tumors evade immune surveillance (Shang et al., 2020). Studies have shown that Bregs produce TGF-β to increase the expression of FoxP3 in Tregs and secrete IL-10 and IL-35 to inhibit the function of CD8+ T cells in vitro. In addition to directly inhibiting the function of effector T cells, Bregs indirectly convert CD4+ T cells into FoxP3+ Tregs through TGF-β, which induces and promotes the production of an immune-suppressive microenvironment (Chen et al., 2019). At the same time, PD-L1 is constitutively expressed on B cells, called PD-L1 Bregs, which interact with PD-1 CD4+ T cells and PD-1 follicular helper T cells, leading to an inhibition of humoral immune responses. Of note, PD-L1 Bregs also decrease the production of pro-inflammatory cytokines and increase IL-10 to suppress the antitumor response (Catalán et al., 2021; Michaud et al., 2021).
2.3 Dendritic cells
DCregs, which are subsets of dendritic cells (DCs) with immunosuppressive activity in the TME, have strong immunosuppressive activity and are generally found in the microenvironment of advanced solid tumors (José et al., 2016). DCs include myeloid, lymphoid, conventional (cDCs), and plasma cell subsets (pDCs), which have specific immunostimulatory and immunosuppressive functions (Badillo et al., 2024). DCregs directly inhibit the activity of antitumor T cells by producing IL-10 and TGF-β and produce strong antigen-specific tolerance by inducing the differentiation of CD4+ Tregs. Additionally, IL-10 and TGF-β secreted by DCregs promote the transformation of CD4+ T cells into Tregs and enhance the immunosuppressive activity of natural Tregs. In conclusion, DCregs directly and indirectly induce regulatory responses by cross-talking with B cells, NK cells, and CD8+ T cells, thus inhibiting antitumor immunity (Verena et al., 2015).
2.4 Myeloid-derived suppressor cells
MDSCs are immature cells composed of myeloid progenitor cells, immature macrophages, immature granulocytes, and immature dendritic cells that affect tumor immunosuppression, angiogenesis, drug resistance, and metastasis (Chen H. et al., 2022). Based on their different surface markers, MDSCs can be divided into two subtypes: granulocytic MDSCs (PMN-MDSCs, also known as multinucleated MDSCs) and monocytic MDSCs (M-MDSCs). PMN-MDSCs constitute the main population of MDSCs (approximately 80%), which primarily inhibit antigen-specific CD8+ T cells by producing reactive oxygen species (ROS). M-MDSCs are rapidly transformed into tumor-associated macrophages (TAMs) in the hypoxic region of tumors to increase their immunosuppressive effect (Gabrilovich et al., 2012). PMN- and M-MDSCs not only produce Arg1, iNOS, and IDO1 to inhibit the functions of T cells but also secrete IFN-γ, IL-10, and TGF-β to convert CD4+ T cells into Tregs and impair the function of DCs by producing IL-10. MDSCs also exert immunosuppressive effects by impairing other immune cell functions, including those of B cells and NK cells (Wang et al., 2022).
2.5 Mesenchymal stem cells
MSCs are self-renewing pluripotent stem cells that were initially identified in the bone marrow; these cells regulate innate and adaptive immune responses and affect the antigen-presenting properties of DCs, B cells, and macrophages. Studies have shown that MSCs have the ability to regulate the phagocytic capacity of neutrophils and monocytes; change the polarization of macrophages and the cytotoxicity of NK cells; and regulate the proliferation, activation, and effector functions of CD4+ and CD8+ T cells (Harrell et al., 2021). MSCs also induce the generation of immunosuppressive Tregs and MDSCs in a paracrine manner and exert suppressive effects on CD8+ Cytotoxic T-lymphocytes (CTLs). Thus weakening antitumor immunity and enabling tumor growth and progression (Sarhan et al., 2020; Song et al., 2020).
2.6 Tumor-associated macrophages
TAMs, another type of tumor-infiltrating cell, originate from monocytes in the bone marrow and are among the key regulators of the immune response in the TME. After being exposed to the TME, macrophages undergo M1-like or M2-like polarization to promote or inhibit tumors (Zhang, et al., 2022). M1 macrophages promote a pro-inflammatory response, damage tissue integrity, dampen tumor progression, and are induced by T-helper type-1 cytokines, including IFN-γ, IL-1β, and lipopolysaccharides (LPSs). However, M2 macrophages, also called TAMs, are anti-inflammatory cells induced by IL-4 and IL-13 secreted by Th2 cells, which promote cancer cell proliferation, invasion, tumor metastasis, and angiogenesis and participate in immune suppression (Basak et al., 2023). TAMs inhibit the antitumor activity of lymphocytes through various mechanisms. On one hand, TAMs release TGF-β to directly inhibit the effector functions of T cells and NK cells. On the other hand, TAMs hinder the maturation of dendritic cells, promote the expansion of Tregs, and impair the functions of T cells by releasing the immunomodulatory enzymes arginase-1 (Arg–1), indole-2, and 3-dioxygenase (IDO) (MdNabiul et al., 2022).
3 Natural products that act on regulatory immune cells
3.1 Polysaccharides
Most TCM substances are derived from herbal plants, and polysaccharides are major components of these plants. In recent decades, polysaccharides isolated from different types of TCMs have received much attention in the treatment of cancer because of their multiple working pathways and minimal adverse reactions. The natural sources and comprehensive effects of polysaccharides on immune cells are listed in Table 1.
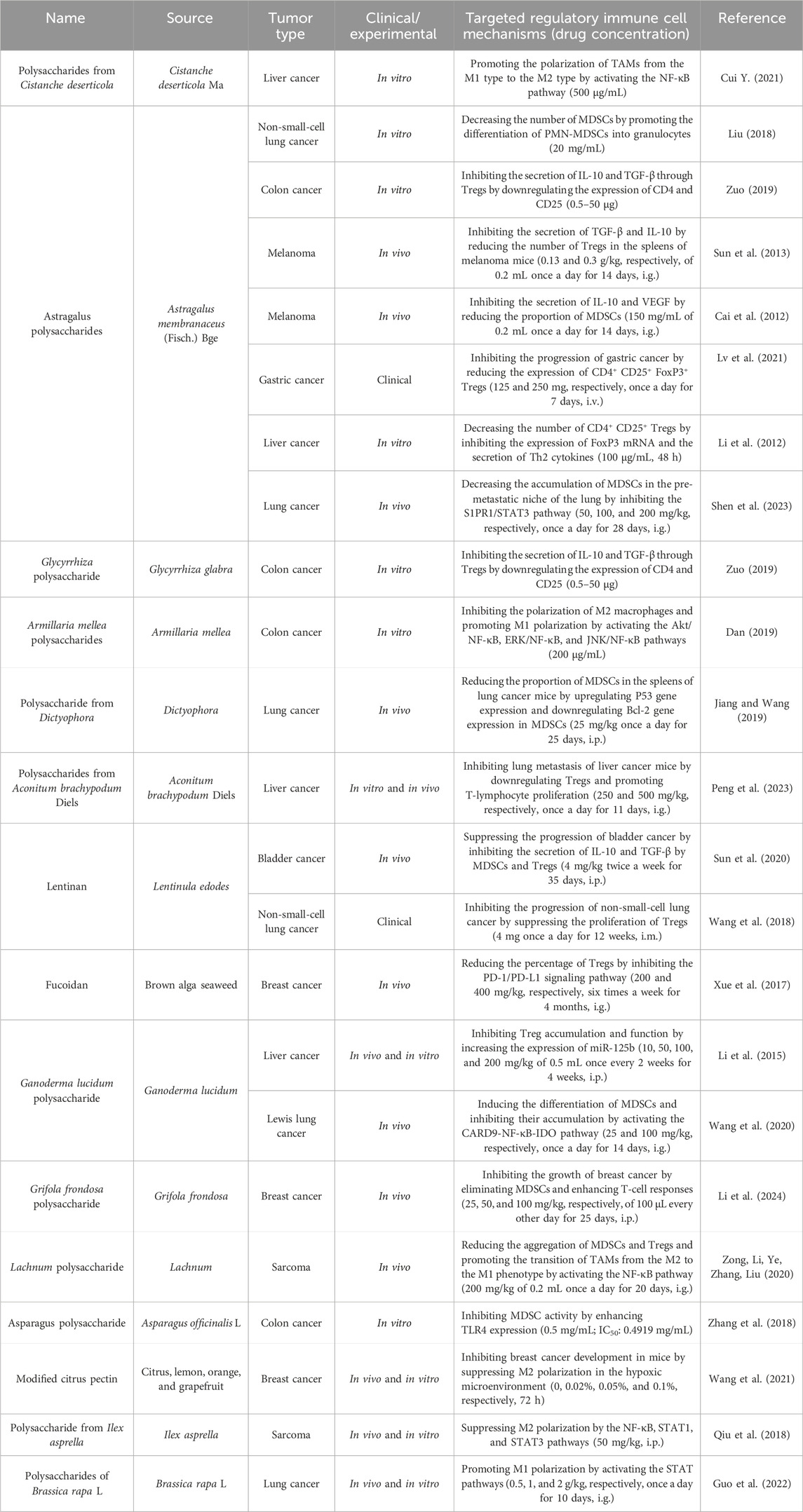
Table 1. Natural antitumor polysaccharides and their sources, experimental models, and mechanisms of targeting regulatory immune cells.
Astragalus polysaccharides are monomeric components extracted from Huangqi that have been widely studied for the treatment of non-small-cell lung cancer by inhibiting the S1PR1/STAT3 pathway and further promoting the differentiation of MDSCs in the peripheral blood (Liu, 2018; Shen et al., 2023). Additionally, Astragalus polysaccharides inhibit the recruitment of MDSCs and Tregs, which reduces the expression of IL-10, TGF-β, and VEGF (Cai et al., 2012). Some immunosuppressive cytokines expressed in tumors, such as IL-10 and TGF-β, are considered to be the main cause of failed antitumor immune responses (Sun et al., 2013; Wiguna and Walden, 2015; Zuo, 2019). Lentinan suppresses the progression of bladder cancer, accompanied by a significant reduction in IL-10 and TGF-β levels in MDSCs and Tregs (Sun et al., 2020). In addition, Glycyrrhiza polysaccharides also have the ability to inhibit the secretion of IL-10 and TGF-β in Tregs and downregulate the expression of CD4 and CD25 (Zuo, 2019). Ganoderma lucidum polysaccharides have recently been exploited as potential components in the treatment of cancer, which induce the differentiation of MDSCs and inhibit their accumulation by activating the CARD9-NF-κB-IDO pathway in Lewis lung cancer, thereby preventing cancer progression (Wang et al., 2020). G. lucidum polysaccharides also significantly suppress tumor growth in hepatoma-bearing mice, which is associated with an increase in the ratio of Teffs to Tregs through increased miR-125b expression (Li et al., 2015). The NF-κB and STAT pathways are central coordinators in innate and adaptive immune responses and play important roles in the control of malignant cells to resist apoptosis-based tumor surveillance (Fan et al., 2013).
Most polysaccharides have been reported to inhibit tumor growth and reduce the aggregation of MDSCs, Tregs, and M2-TAMs through the NF-κB and STAT pathways. Examples include polysaccharides from Armillaria mellea, Cistanche deserticola, Lachnum, Ilex asprella, and Brassica rapa L (Cui Y. 2021; Dan, 2019; Guo et al., 2022; Qiu et al., 2018). Dictyophora indusiata, an edible mushroom, has demonstrated significant anticancer activity by enhancing immune function. It was found to significantly lower the proportion of MDSCs in the spleen of tumor-bearing mice by upregulating the expression of the pro-apoptotic P53 gene and downregulating the expression of the anti-apoptotic Bcl-2 gene (Jiang and Wang, 2019). Tregs play a crucial role in maintaining the balance between autoimmunity and immune suppression and are key in suppressing T-cell activation (Shan et al., 2022). Polysaccharides from Aconitum brachiopod Diels and fucoidan have been shown to reduce the percentage of CD3+ FoxP3+ Tregs while increasing the proportions of CD4+ and CD8+ T cells, providing experimental evidence of the immune enhancement effects induced by polysaccharides (Peng et al., 2023; Xue et al., 2017). The structural formulas of these polysaccharide products are given in Figure 2.
3.2 Polyphenols
Numerous studies have reported that natural polyphenols present in foods and beverages of plant origin have promising anticancer effects through the targeting of regulatory immune cells (Table 2). Based on their chemical structure, natural polyphenols can be divided into six classes: stilbenes, flavanols, flavonols, flavones, chalcones, and isoflavones. Resveratrol, a polyphenol found in grapes and blueberries, is widely recognized as an antitumor compound that modulates inflammation (Inoue and Nakata, 2015) and can inhibit tumor growth by increasing the percentages of CD8+ T cells, NK cells, and NK T cells and decreasing the percentages of Tregs, tBregs, and MDSCs (Catalina et al., 2013; Li et al., 2014; Tong et al., 2021; Yang et al., 2008; Zhou, et al., 2020). As discussed above, MDSCs are important factors in the TME. Resveratrol can promote not only the apoptosis of G-MDSCs but also the differentiation of M-MDSCs (Zhao, 2018; Zhao et al., 2019; Zhao et al., 2018). Recent research has shown that resveratrol exerts anticancer action by regulating the polarization state of TAMs and further inhibiting the migration and invasion of CAL27 cells by inhibiting the phosphorylation of the Syk protein (Li, 2022). A growing number of epidemiologic studies have shown an inverse relationship between cancer incidence and flavonoid intake. Epigallocatechin-3-gallate originates from green tea extract, which has the ability to downregulate canonical pathways in MDSCs, mainly through the Arg–1/iNOS/Nox2/NF-κB/STAT3 signaling pathway, and upregulate CD4+ and CD8+ T cells to ameliorate immunosuppression (Xu et al., 2020). Quercetin, silymarin, and Radix Tetrastigma hemsleyani flavones are flavonoid compounds that inhibit MDSCs (Hu, 2016; Hu et al., 2021; Ma et al., 2020; Wu et al., 2018). Notably, some flavanones, including wogonin, baicalein, and apigenin, can induce M1 macrophage polarization by activating the JAK2-STAT1 pathway and increasing the expression of SHIP-1 (Kazim et al., 2022; Xiao, 2021). Formononetin is a type of isoflavanone obtained from Astragalus membranaceus, whose therapeutic role in cancer involves blocking the PD-1/PD-L1 pathway to activate CD8+ T cells and reduce the release of immunosuppressive factors in Tregs (Li et al., 2023). Cardamonin is a dihydrochalcone isolated from Elettaria cardamomum with anticancer activity. Mechanistically, cardamonin suppresses the M2 polarization of TAMs and downregulates IL-10 and VEGF secreted by TAMs by inhibiting the phosphorylation of mTOR and STAT3 (Chen Z. et al., 2022). The anticancer potential of curcumin is observed in multiple cancer types, such as breast cancer and OSCC, and is associated with the suppression of the activity of Tregs and TAMs, as well as the downregulation of TGF-β and IL-10 by the MAO-A/STAT6 signaling pathway (Bhattacharyya et al., 2010; Jiang, 2023). The structural formulas of the polyphenolic products are shown in Figure 3.
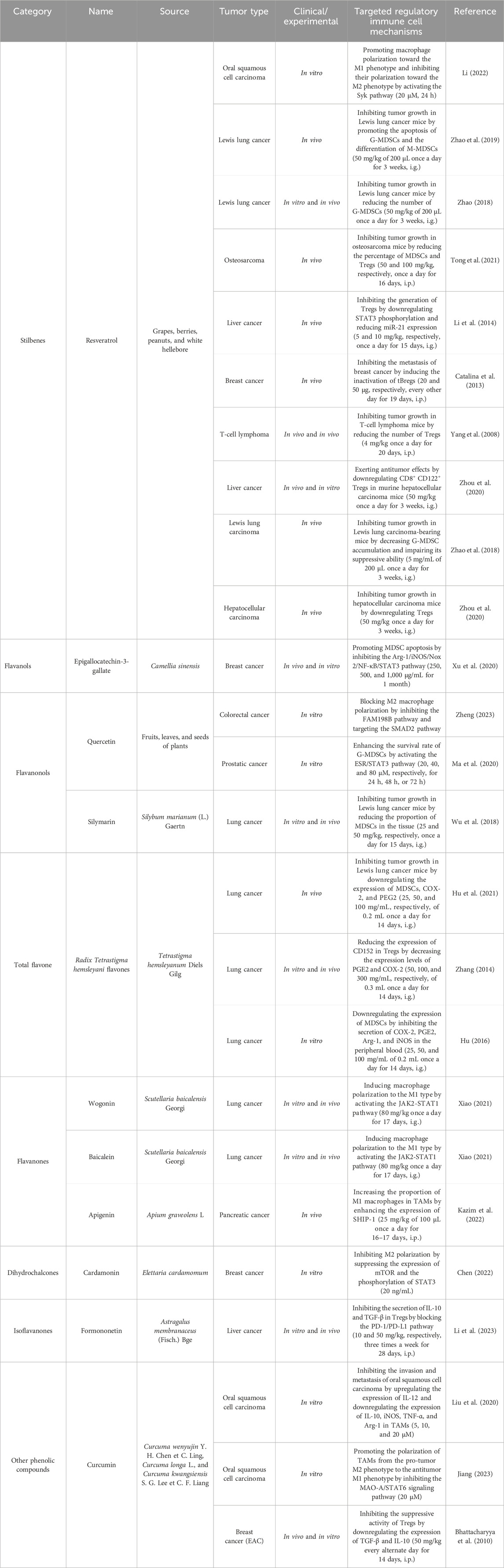
Table 2. Natural antitumor polyphenols and their sources, experimental models, and mechanisms of targeting regulatory immune cells.
3.3 Saponins
Saponins consist of sapogenin and sugar molecules. Sapogenins are triterpenes or spirostanes, and the make-up sugars are commonly glucose, arabinose, rhamnose, galactose, and xylose. Many traditional Chinese herbs, such as Paeonia lactiflora, Panax ginseng, A. membranaceus, Epimedium Tourn, and Rhodiola crenulata, contain glycosides as their primary active ingredients. Owing to the structures of sapogenins, saponins exhibit biological activities such as antitumor, immune-regulatory, and anti-inflammatory effects. The natural sources and comprehensive influence of saponins on immune cells are listed in Table 3. B-cell activating factor (BAFF), a cytokine belonging to the TNF family, plays a crucial role in regulating the survival and differentiation of B cells. The total glucosides of Paeonia can reduce the proportion of Bregs by inhibiting the BAFF-BAFF-R signaling pathway (Yuan, 2015). Dioscin is a glycoside saponin isolated from Dioscorea zingiberensis and Dioscorea nipponica that can inhibit the polarization of M2 macrophages in TAMs by activating the Cx43/STAT1/IFN-γ pathway and inhibiting the IL-4/JAK2/STAT3 pathway (Cui et al., 2020; Sun, 2022). Ginsenoside Rg3 and ginsenoside Rh2, which originate from P. ginseng C. A. Mey, can inhibit tumor growth and metastasis in Lewis lung cancer mice by polarizing TAMs from M2 to M1 (He et al., 2012; Jiang, 2018; Li et al., 2018). Moreover, an increasing number of studies have reported that ginsenoside Rg3 exerts anticancer effects by inhibiting MDSCs, which is related to the suppression of the STAT3 and NOTCH signaling pathways (Song et al., 2020). Vitexin, a flavonoid-like compound derived from Vitex negundo L. var., promotes the polarization of M1 macrophages and inhibits M2 polarization by increasing the expression of STAT1/p-STAT1, thereby inhibiting tumor growth in colon and breast cancer mice (X. Yuan, 2019; Yuan et al., 2022). Tregs are crucial for inducing acquired tolerance to tumors and suppressing various immune responses. Astragaloside IV and salidroside can reduce tumor growth in Lewis lung cancer mice by weakening the immune function of Tregs (Li B. et al., 2022; Zhang et al., 2014). The structural formulas of saponin products are shown in Figure 4.
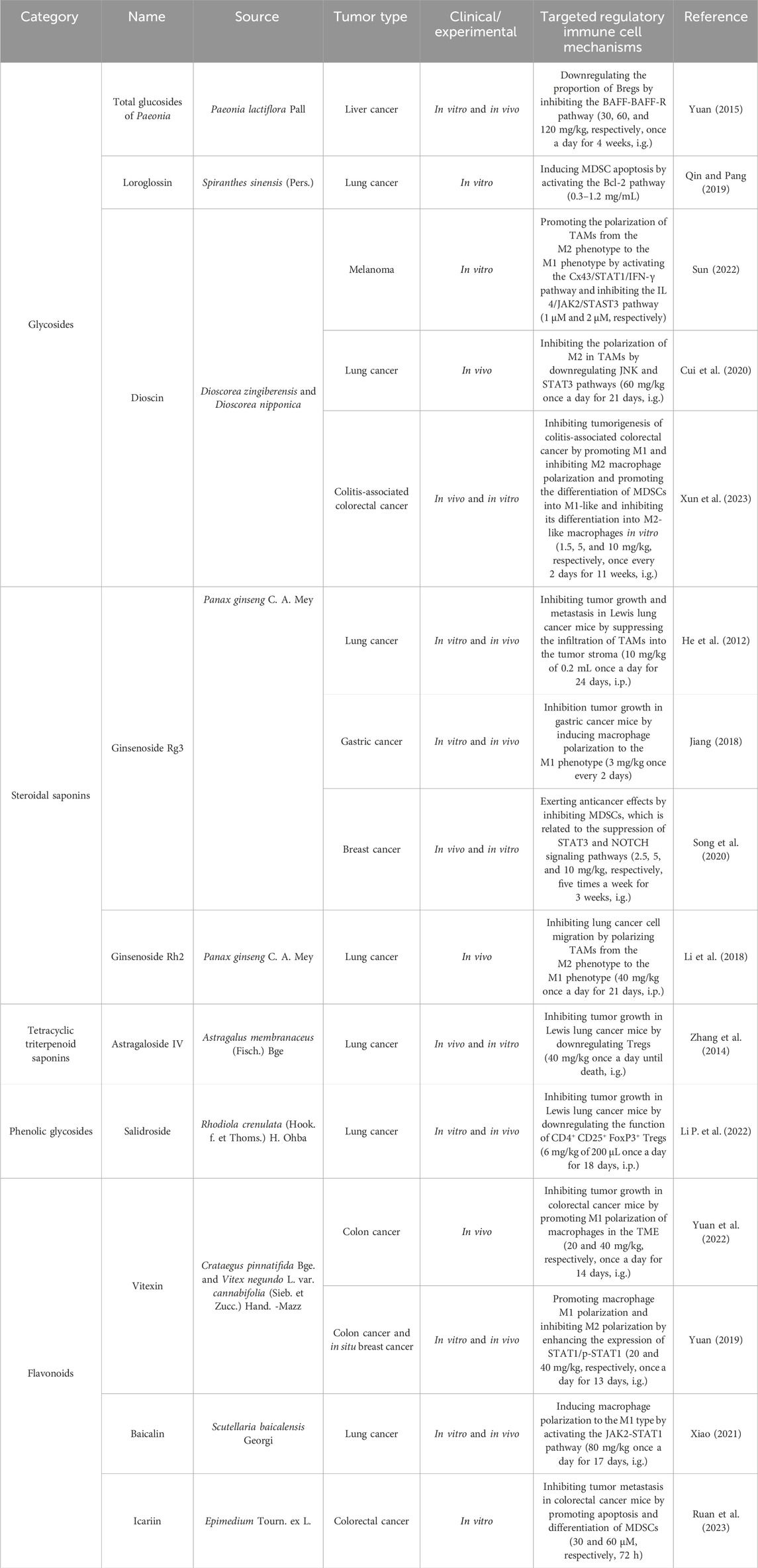
Table 3. Natural antitumor saponins and their sources, experimental models, and mechanisms of targeting regulatory immune cells.
3.4 Alkaloids
Numerous studies have reported that natural alkaloids, whose heterocyclic moieties often exhibit improved solubility and can facilitate salt formation, can be used for the treatment and prevention of cancer (Rampogu et al., 2022). Therefore, heterocyclic moieties are important for oral absorption and bioavailability (Xiong et al., 2015). On the basis of their chemical structure, alkaloids can be categorized into three groups: isoquinolines, piperidines, and steroid alkaloids (Table 4). Berberine is an eminent isoquinoline quaternary alkaloid of TCM. Recently, berberine has gained much attention for its pharmacological action in treating cancer (Ortiz et al., 2014), which not only reduces the formation of TAMs but also downregulates IL-10 and TGF-β (J. Li et al., 2011). Among plant derivatives with biological properties, sanguinarine has a broad range of therapeutic uses against lung cancer. Specifically, sanguinarine inhibits angiogenesis in lung cancer by preventing M2 macrophage polarization via the Wnt/β-catenin pathway, while it also induces the differentiation of MDSCs into macrophages and DCs through the NF-κB pathway (Li P. et al., 2022; Cui Y. J. 2021). Solanine, oxymatrine, and sophoridine, important and active components of piperidines, have been found to have anticancer effects on multiple cancer types through the inhibition of immunosuppression. Solanine, extracted from Solanum tuberosum L., may activate the antitumor immune response by downregulating Tregs via the TGF-β/SMAD pathway (Gao et al., 2020). Oxymatrine can also mediate the differentiation of T cells into Tregs (Liu et al., 2019). Sophoridine induces TAM polarization into the M1 type through the TLR4/IRF3 axis and exerts stronger pro-inflammatory effects through the upregulation of the expression of INOS, IFN-β, and IL-12α (Zhuang et al., 2020). Cyclovirobuxine D, a natural alkaloid derived from Buxus sinica var. parvifolia, regulates the TME in the macrophage phenotype by regulating the immune checkpoint Siglec-10 (Gu, 2022). Solamargine also alleviates the expression of IL-4, IL-6, IL-10, and IL-13 and promotes the expression of IL-12 and TNF-α by inhibiting the polarization of TAMs (Liang, 2018). The related structural formulas of the natural alkaloid products are shown in Figure 5.
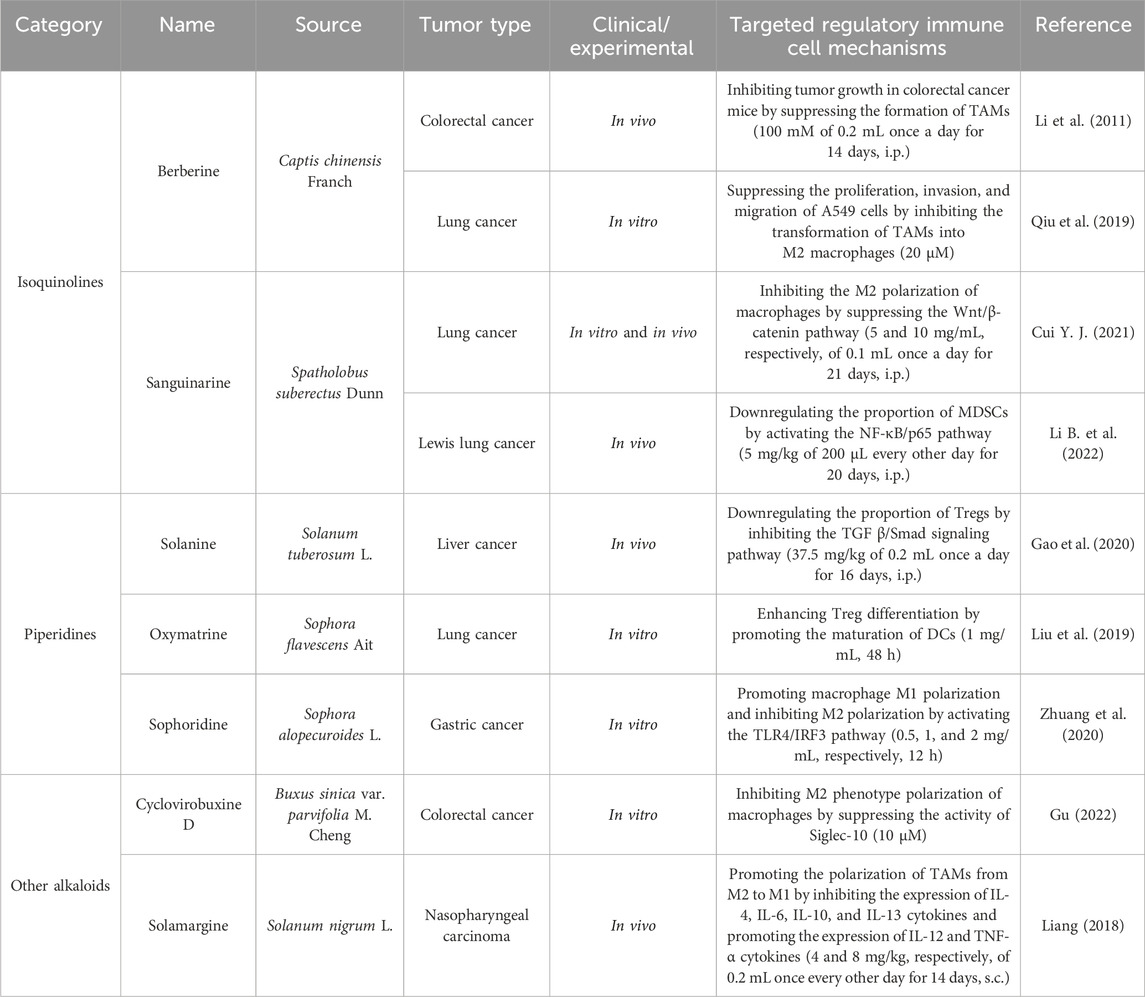
Table 4. Natural antitumor alkaloids and their sources, experimental models, and mechanisms of targeting regulatory immune cells.
3.5 Terpenoids
Terpenoids are natural products derived from mevalonic acid and composed of multiple isoprene (C5) units with a general formula of (C5H8)n. Terpenoids are divided into three groups, namely, triterpenoids, diterpenoids, and sesquiterpenoids, based on their different molecular structures. The natural sources and comprehensive influences of terpenoids on immune cells are listed in Table 5. A triterpenoid compound, 23-hydroxybetulinic acid, isolated from Pulsatilla chinensis (Bge.) Regel, inhibits tumor growth in colon cancer mice by reducing the proportion of MDSCs and decreasing the expression of the immunosuppressive factor Arg1 in MDSCs (He et al., 2024). In a mouse model of colorectal cancer, 23-hydroxybetulinic acid can also promote the polarization of TAMs from the M1 phenotype to the M2 phenotype by activating the JAK/STAT3/NF-κB/STAT1 pathway (Liu, 2023). Madecassic acid alleviates colitis-associated colorectal cancer by blocking the recruitment of MDSCs via the inhibition of the expression of IL-17 in γδT17 cells (Yun et al., 2022). Moreover, oleanolic acid and ursolic acid are triterpenoid compounds that decrease the proportion of Tregs by inhibiting STAT5 phosphorylation and IL-10 secretion and promoting the expression of miR-98–5 (Xu et al., 2021; Zhang et al., 2020). Obacunone can also suppress tumor growth by promoting M1 macrophage polarization (Zou, et al., 2023). Notably, triptolide is the key diterpenoid extracted from Tripterygium wilfordii Hook. f., which reduces the proportion of Tregs and inhibits the secretion of IL-10 and TGF-β (Liu, 2011; Liu et al., 2013). Triptolide can also promote the apoptosis of MDSCs and the polarization of TAMs from M2 to M1 by inhibiting the PI3K/AKT/NF-κB pathway (Hu, 2020). Oridonin, paclitaxel, and carnosol inhibit the generation of Tregs by decreasing the expression of TGF-β and increasing the expression of CD95 and IFN-γ (Guo et al., 2020; Maryam, et al., 2015; Zhang et al., 2008). By promoting the differentiation of MDSCs into DCs, paclitaxel suppresses tumor growth in melanoma mice (Tillmann et al., 2012). The compounds of the sesquiterpenoids artemisinin and tehranolide, which are extracted from Artemisia annua L., inhibit tumor growth by reducing the number of Tregs (Cao et al., 2019; Noori, et al., 2009; Noori et al., 2010). The structural formulas of the terpenoid products are shown in Figure 6.
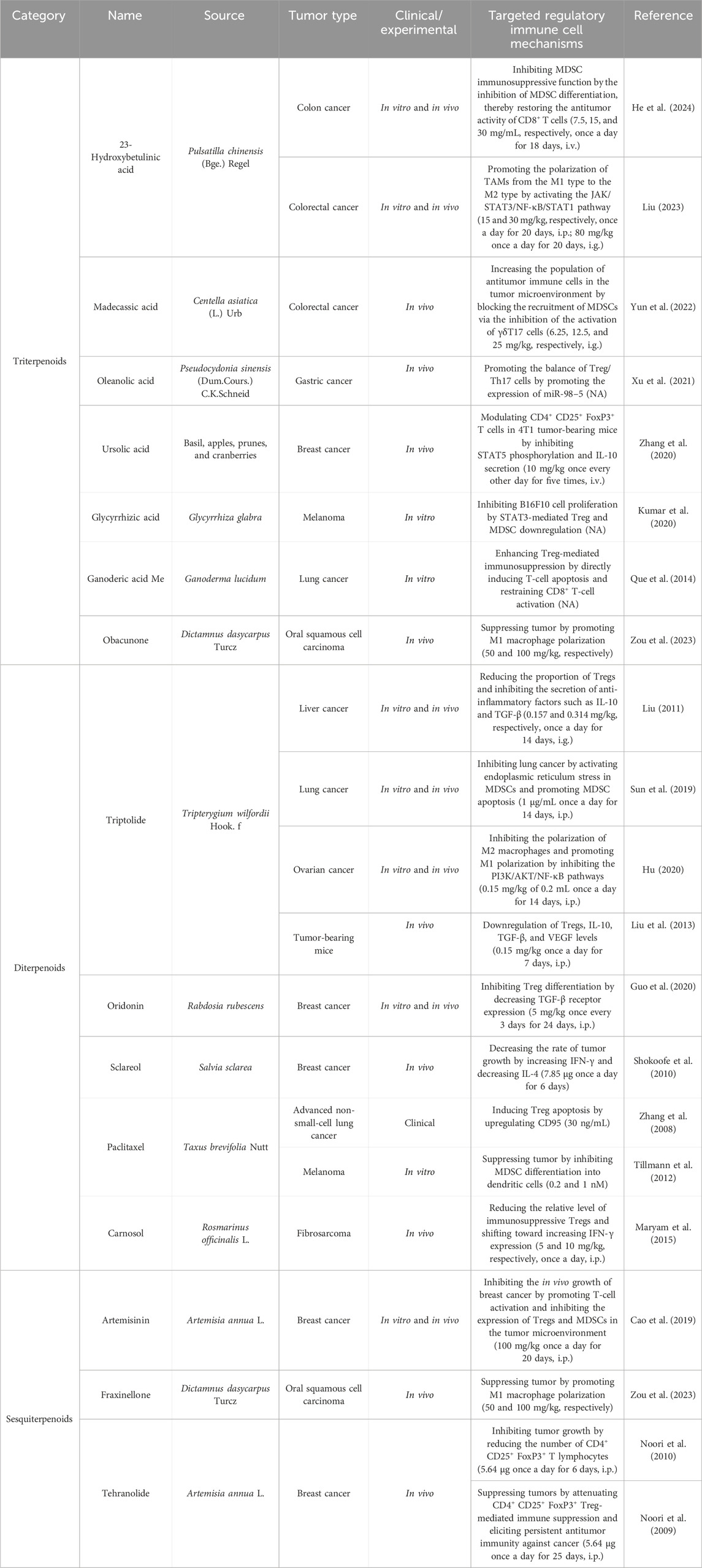
Table 5. Natural antitumor terpenoids and their sources, experimental models, and mechanisms of targeting regulatory immune cells.
3.6 Quinones and other compounds
Quinones that target the regulation of immune cells can be classified into three categories: anthraquinone, naphthoquinone, and benzoquinone. Emodin inhibits Treg migration by inhibiting the expression of CCR4, VEGF-C, and MMP-9 in tumor tissue (Ruan, et al., 2014; Ruan et al., 2013; Wang, 2018). Plumbagin can reduce the inhibition of T cells by inhibiting the differentiation of MDSCs and the expression of CCL2 and IL-6, ultimately exerting an antitumor effect (Chen, 2018). Sulforaphane (SFN) induces MDSC apoptosis by promoting the expression of IL12RB2 and enhancing the infiltration of CD8+ T cells (Chen, 2022). Zeylenone promotes the polarization of M1 macrophages and inhibits M2 polarization by inhibiting the PI3K/AKT signaling pathway, thereby reducing the activity of colorectal cancer cells and promoting their apoptosis (Li D. et al., 2024). Detailed information is presented in Table 6, and the structural formula is shown in Figure 7.
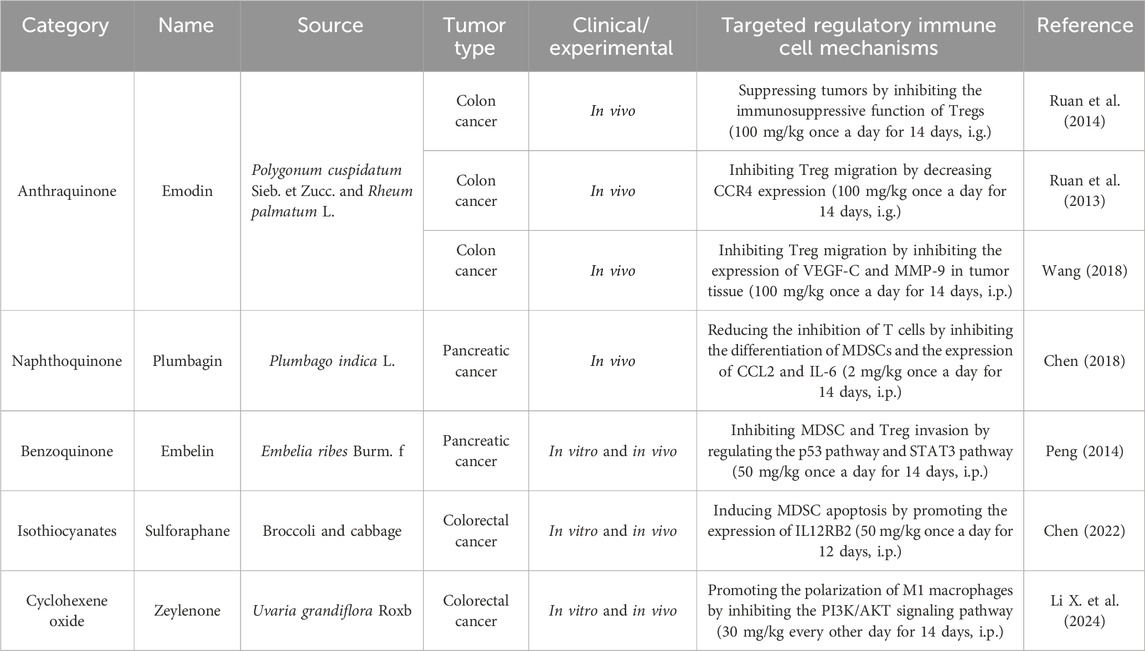
Table 6. Natural antitumor quinones and other compounds, their sources, experimental models, and mechanisms of targeting regulatory immune cells.
4 Conclusion and prospects
Regulatory immune cells strongly influence the tumor microenvironment. Tregs, Bregs, MDSCs, DCregs, and TAMs regulate immune responses through various mechanisms, affecting the occurrence, development, and therapeutic effects of tumors. In this article, we reviewed the classification, function, and mechanism of action of these regulatory immune cells in the tumor microenvironment and investigated the potential of natural products in regulating these immune cells. Natural products exhibit significant immunomodulatory effects, influencing the function of regulatory immune cells and promoting antitumor immune responses (Figure 8).
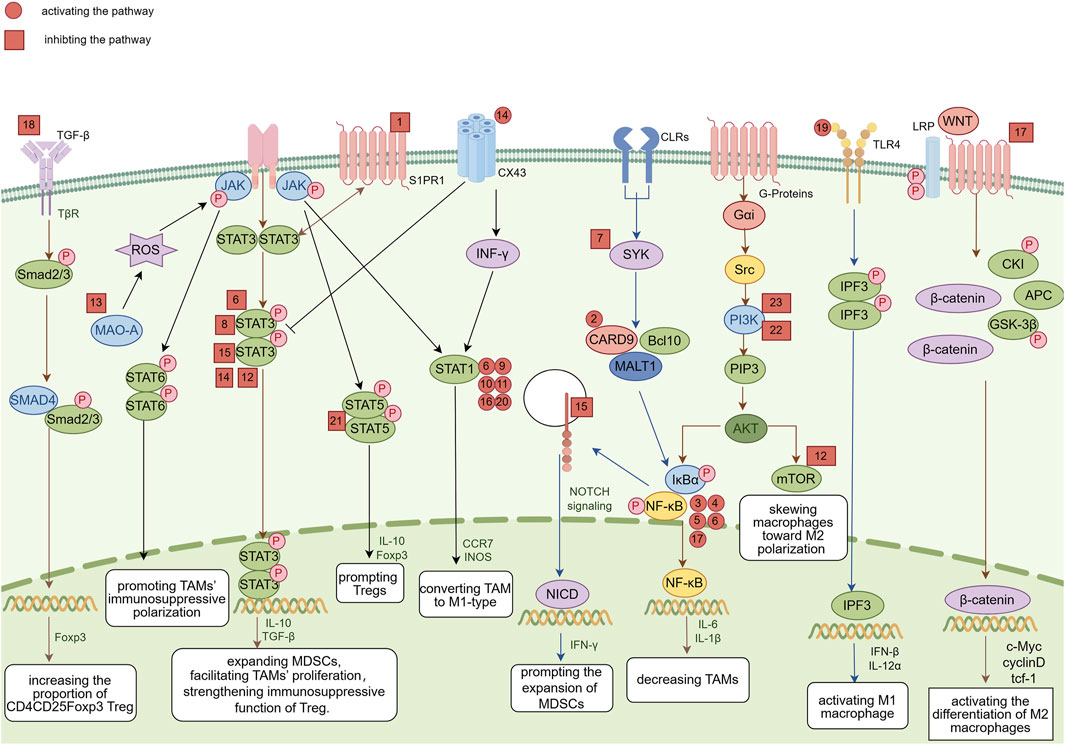
Figure 8. Overview of the molecular mechanisms of representative natural products, which are indicated by corresponding numbers. 1: Astragalus. 2: Ganoderma lucidum polysaccharide. 3: Armillaria mellea polysaccharides. 4: Polysaccharides from Cistanche deserticola. 5: Lachnum polysaccharide. 6: Polysaccharide from Ilex asprella. 7: Resveratrol. 8: Epigallocatechin-3-gallate. 9: Wogonin. 10: Baicalein. 11: Apigenin. 12: Cardamonin. 13: Curcumin. 14: Dioscin. 15: Ginsenoside Rg3. 16: Vitexin. 17: Sanguinarine. 18: Solanine. 19: Sophoridine. 20: 23-Hydroxybetulinic acid. 21: Ursolic acid. 22: Triptolide. 23: Zeylenone. Image was created using Figdraw.com.
In addition, the antineoplastic agent paclitaxel has gained importance as a promising agent for reducing the number of Tregs in the peripheral blood of non-small cell lung cancer patients at a dose of 30 ng/mL (Zhang et al., 2008). Furthermore, additional treatment with 4 mg of lentinan once daily, administered through intramuscular injection for 12 weeks to non-small-cell lung cancer patients who received NP (combination of vinorelbine and cisplatin) first-line chemotherapy, can prolong the survival benefits of progression-free survival (PFS) or even OS by downregulating the percentage of Tregs in the peripheral blood and leading to a shift in the inflammatory status from Th2 to Th1 (Wang et al., 2018). By treating with different doses of APS (10, 50, 100, 150, and 200 μg/mL) for 24, 48, and 72 h, the number of Tregs decreased in a dose-dependent manner in the peripheral blood of hepatocellular carcinoma (HCC) patients. It indicated that the application of APS in the tumor microenvironment could enhance the antitumor effects of immunotherapy approaches, thereby potentially increasing the survival rate in patients with HCC (Li, et al., 2012).
Despite the substantial amount of literature exploring natural products, most studies continue to focus on the mechanisms of drug action, with relatively few studies addressing the bioavailability and toxicity of these natural compounds. Furthermore, research on the clinical development of natural products is also limited. In the future, more systematic clinical trials should be conducted to verify the efficacy and safety of natural products and investigate the combination of natural products and existing tumor treatment methods, such as immune checkpoint inhibitors, chemotherapy, and radiotherapy, which may synergistically affect and improve treatment effectiveness. Researchers should also identify and develop more natural products with immunomodulatory functions and evaluate their ability to treat tumors, thereby promoting their application in cancer treatment.
To summarize, research on regulatory immune cells and natural products can improve the efficacy of cancer treatment. In-depth research on the mechanism of action, clinical applications, combination therapy, and the development of new natural products can further enhance the effectiveness of tumor treatment and improve patient prognosis.
Author contributions
PS: writing–review and editing and writing–original draft. FS: writing–original draft. TS: writing–review and editing. PW: writing–review and editing. RL: writing–original draft. Z-SC: writing–review and editing. ZZ: writing–review and editing. GX: writing–review and editing.
Funding
The author(s) declare that financial support was received for the research, authorship, and/or publication of this article. This work was supported by the National Natural Science Foundation of China (82260859 and 81803779), the Gansu Province Science Foundation for Distinguished Young Scholars (20JR10RA348), Longyuan Innovation and Entrepreneurship Team Project of Gansu Province, Longyuan Talent Project in Gansu Province, Natural Science Foundation of Gansu Province (22JR5RA611 and 22JR11RA125), and Traditional Chinese Medicine Scientific Research Project in Gansu Province in 2020 (GZKP-2020-32).
Conflict of interest
The authors declare that the research was conducted in the absence of any commercial or financial relationships that could be construed as a potential conflict of interest.
Publisher’s note
All claims expressed in this article are solely those of the authors and do not necessarily represent those of their affiliated organizations, or those of the publisher, the editors, and the reviewers. Any product that may be evaluated in this article, or claim that may be made by its manufacturer, is not guaranteed or endorsed by the publisher.
References
Abigail, O. D., and Vignali Dario, A. A. (2018). Treg fragility: a prerequisite for effective antitumor immunity? Cancer Immunol. Res. 6 (8), 882–887. doi:10.1158/2326-6066.cir-18-0066
Badillo, O., Helfridsson, L., Niemi, J., and Hellström, M. (2024). Exploring dendritic cell subtypes in cancer immunotherapy: unraveling the role of mature regulatory dendritic cells. Ups. J. Med. Sci. 129, e10627. doi:10.48101/ujms.v129.10627
Basak, U., Sarkar, T., Mukherjee, S., Chakraborty, S., Dutta, A., Dutta, S., et al. (2023). Tumor-associated macrophages: an effective player of the tumor microenvironment. Front. Immunol. 14, 1295257. doi:10.3389/fimmu.2023.1295257
Bhattacharyya, S., Hossain, D., Mohanty, S., Sen, G., Chattopadhyay, S., Banerjee, S., et al. (2010). Curcumin reverses T cell-mediated adaptive immune dysfunctions in tumor-bearing hosts. Cell. Mol. Immunol. 7 (4), 306–315. doi:10.1038/cmi.2010.11
Cai, W., He, X., Zhu, J., Lv, C., Zhao, H., Lv, A., et al. (2012). Immunoregulatory effects of astragalus polysaccharides on myeloid derived suppressor cells in B16-F10 tumor bearing mice. J. Basic Chin. Med. 18 (01), 63–65. doi:10.19945/j.cnki.issn.1006-3250.2012.01.030
Cao, Y., Feng, Y., Gao, L., Li, X., Jin, Q., Wang, Y., et al. (2019). Artemisinin enhances the anti-tumor immune response in 4T1 breast cancer cells in vitro and in vivo. Int. Immunopharmacol. 70, 110–116. doi:10.1016/j.intimp.2019.01.041
Catalán, D., Mansilla, M., Ferrier, A., Soto, L., Oleinika, K., Aguillón, J. C., et al. (2021). Immunosuppressive mechanisms of regulatory B cells. Front. Immunol. 12, 611795. doi:10.3389/fimmu.2021.611795
Catalina, L., Monica, B., Aléjandro, M., Katarzyna, W., Sanghvi, M., Moaddel, R., et al. (2013). Inhibition of breast cancer metastasis by resveratrol-mediated inactivation of tumor-evoked regulatory B cells. J. Immunol. 191 (8), 4141–4151. doi:10.4049/jimmunol.1300606
Ceglie, I., Carnevale, S., Rigatelli, A., Grieco, G., Molisso, P., and Jaillon, S. (2024). Immune cell networking in solid tumors: focus on macrophages and neutrophils. Front. Immunol. 15, 1341390. doi:10.3389/fimmu.2024.1341390
Chen, H. (2022). The mechanisms of sulforaphane on apoptosis and function ofMyeloid-derived suppressor cells. doi:10.27466/d.cnki.gzzdu.2022.005825
Chen, H., Huang, S., Niu, P., Zhu, Y., Zhou, J., Jiang, L., et al. (2022). Cardamonin suppresses pro-tumor function of macrophages by decreasing M2 polarization on ovarian cancer cells via mTOR inhibition. Mol. Ther. - Oncolytics 26, 175–188. doi:10.1016/j.omto.2022.06.009
Chen, Y. (2018). The role and mechanism of Plumbagin in pancreatic cancer and differentiation myeloid-derived suppressor cells.
Chen, Z., Yuan, R., Hu, S., Yuan, W., and Sun, Z. (2022). Roles of the exosomes derived from myeloid-derived suppressor cells in tumor immunity and cancer progression. Front. Immunol. 13, 817942. doi:10.3389/fimmu.2022.817942
Chen, Z., Zhu, Y., Du, R., Pang, N., Zhang, F., Dong, D., et al. (2019). Role of regulatory B cells in the progression of cervical cancer. Mediat. Inflamm. 2019, 6519427. doi:10.1155/2019/6519427
Cui, L., Yang, G., Ye, J., Yao, Y., Lu, G., Chen, J., et al. (2020). Dioscin elicits anti-tumour immunity by inhibiting macrophage M2 polarization via JNK and STAT3 pathways in lung cancer. J. Cell. Mol. Med. 24 (16), 9217–9230. doi:10.1111/jcmm.15563
Cui, Y, Y. (2021). Structural characteristics of polysaccharides from Cistanche deserticola and Cistanche tubulosa and their antitumor effects based on tumor-associated macrophage. doi:10.27366/d.cnki.gtyku.2021.001169
Cui, Y. J. (2021). Molecular Mechanism Research on Sanguinarine targeting Wnt/β-catenin pathway in the regulation of tumor-associated macrophages against angiogenesis in the lung cancer. doi:10.27320/d.cnki.gszyu.2021.000101
Dan, Y. (2019). Study on the role of Amillariella Mellea polysaccharides in modulating antitumor response by promoting the transformation of M2-type macrophages to M1-type. doi:10.27011/d.cnki.gdbsu.2019.000287
Fan, Y., Mao, R., and Yang, J. (2013). NF-κB and STAT3 signaling pathways collaboratively link inflammation to cancer. Protein Cell 4 (3), 176–185. doi:10.1007/s13238-013-2084-3
Farbod, G., Nader, E., Fatemeh, M., Negin, M., Beiranvand, S., Pournazari, M., et al. (2022). The emerging role of regulatory cell-based therapy in autoimmune disease. Front. Immunol. 13, 1075813. doi:10.3389/fimmu.2022.1075813
Gabrilovich, D. I., Rosenberg, S. O., and Bronte, V. (2012). Coordinated regulation of myeloid cells by tumours. Nat. Rev. Immunol. 12 (4), 253–268. doi:10.1038/nri3175
Gao, J., Ying, Y., Wang, J., and Cui, Y. (2020). Solanine inhibits immune escape mediated by hepatoma Treg cells via the tgfβ/smad signaling pathway. BioMed Res. Int. 2020, 9749631. doi:10.1155/2020/9749631
Gu, G. (2022). Molecular mechanisms of cyclovirobuxine D (CVB-D)Regulating tumor associated macrophages against colorectal cancer metastasis.
Guo, J. F., Chen, T., Ma, Z. Y., Chenxiao, Q., Yuan, F., Guo, X., et al. (2020). Oridonin inhibits 4T1 tumor growth by suppressing Treg differentiation via TGF-β receptor. Int. Immunopharmacol. 88, 106831. doi:10.1016/j.intimp.2020.106831
Guo, W., Liu, X., Guo, J., Gao, R., Xiang, X., An, X., et al. (2022). Polysaccharides of Brassica rapa L. attenuate tumor growth via shifting macrophages to M1-like phenotype. Phytotherapy Res. 36 (10), 3957–3968. doi:10.1002/ptr.7545
Harrell, C., Volarević, A., Djonov, V., Jovičić, N., and Volarević, V. (2021). Mesenchymal stem cell: a friend or foe in anti-tumor immunity. Int. J. Mol. Sci. 22 (22), 12429. doi:10.3390/ijms222212429
He, J., Liao, H., Yang, K., Yi, G., and Xie, M. (2012). Mice lung adenocarcinoma inhibitory effeet of ginsenoside Rg3 on growth, metastasis and expression of TAMs in mice lung adenocarcinoma. Cancer Res. Prev. Treat. 39 (12), 1411–1415. doi:10.3971/i.issn.1000-8578.2012.12.002
He, T., Chen, L., Cui, Y., and Luo, Y. (2024). Effects of 23-hydroxybetulinic acid on the growth of mouse colon cancer by inhibiting myeloid-derived suppressor cells. Chin. Tradit. Pat. Med. 46 (04), 1126–1134. doi:10.3969/j.issn.1001-1528.2024.04.010
Hu, H. (2020). Study on the mechanism of triptolide inhibition on Cisplatin resistant epithelial ovarian cancer based on the polarization of TAMs. doi:10.27232/d.cnki.gnchu.2020.000064
Hu, T. (2016). Study on the influence of the flavonoids from Tetrastigma hemsleyanum dielset gilg on the expression of myeloid derived suppressive cells (MDSCs) and related cytokines in mice with. Lung Cancer. doi:10.27465/d.cnki.gzzyc.2016.000234
Hu, T., Zhong, L., and Feng, Z. (2021). Study on Tetrastigma Hemsleyani Radix flavones for MDSCs production and expression of related factors in mice with lung cancer. New J. Tradit. Chin. Med. 53 (08), 118–121. doi:10.13457/j.cnki.jncm.2021.08.031
Inoue, H., and Nakata, R. (2015). Resveratrol targets in inflammation. Endocr. Metab. Immune Disord. Drug Targets 15 (3), 186–195. doi:10.2174/1871530315666150316120316
Jiang, H., and Wang, X. (2019). Antitumor mechanism of polysaccharide from dictyophora targetedon myeloid derived suppressor sell. Chin. Med. Sci. 9 (24), 21–26.
Jiang, M. (2023). Regulation of curcumin on polarization of tumor-associated macrophages and its mechanism in oral squamous cell carcinoma. doi:10.27652/d.cnki.gzyku.2023.001205
José, R. C., Melanie, R. R., and Juan, R. C.-R. (2016). State-of-the-art of regulatory dendritic cells in cancer. Pharmacol. Ther. 164, 97–104. doi:10.1016/j.pharmthera.2016.04.003
Kazim, H., Krystal, V., Valentina, L., Oscar, A. V., Bradley, M., Kazim, S., et al. (2022). Apigenin targets MicroRNA-155, enhances SHIP-1 expression, and augments anti-tumor responses in pancreatic cancer. Cancers 14 (15), 3613. doi:10.3390/cancers14153613
Kumar, J. S., Sweta, G., and Subrata, M. (2020). Glycyrrhizic acid facilitates anti-tumor immunity by attenuating Tregs and MDSCs: an immunotherapeutic approach. Int. Immunopharmacol. 88, 106932. doi:10.1016/j.intimp.2020.106932
Li, A., Shuai, X., Jia, Z., Li, H., Liang, X., Su, D., et al. (2015). Ganoderma lucidum polysaccharide extract inhibits hepatocellular carcinoma growth by downregulating regulatory T cells accumulation and function by inducing microRNA-125b. J. Transl. Med. 13, 100. doi:10.1186/s12967-015-0465-5
Li, B., Luo, Y., Zhou, Y., Wu, J., Fang, Z., and Li, Y. (2022). Role of sanguinarine in regulating immunosuppression in a Lewis lung cancer mouse model. Int. Immunopharmacol. 110, 108964. doi:10.1016/j.intimp.2022.108964
Li, D., Wang, T., Yue, L., Zhao, X., and Wang, S. (2024). Zeylenone affects colorectal cancer progression by regulating PI3K/AKTmediated polarization of tumor-associated macrophages. Chin. J. Immunol. 40 (05), 970–976. doi:10.3969/i.issn.1000-484X.2024.05.011
Li, H., Huang, N., Zhu, W., Wu, J., Yang, X., Teng, W., et al. (2018). Modulation the crosstalk between tumor-associated macrophages and non-small cell lung cancer to inhibit tumor migration and invasion by ginsenoside Rh2. BMC Cancer 18 (1), 579. doi:10.1186/s12885-018-4299-4
Li, J., Hao, Y., Zhang, Y., Xie, W., li, J., and Xue, X. (2011). Berberine inhibits tumor-associated macrophages in subcutaneous tumor of mice. Chin. J. Histochem. Cytochem. 20 (03), 203–206. doi:10.3870/zgzzhx.2011.03.001
Li, M., Jiang, C., Chen, J., and Wang, J. (2023). Formononetin enhances the antitumor effect of H22 hepatoma transplanted mice. Chin. J. Cell. Mol. Immunol. 39 (12), 1063–1068. doi:10.13423/j.cnki.cjcmi.009768
Li, P., Lin, W., He, Y., and Wen, Z. (2022). Inhibition of salidroside on regulatory T cells in Lewis tumor-bearing mice. Mod. Immunol. 42 (05), 395–400+420.
Li, Q., Bao, J., Li, X., Zhang, T., and Shen, X. h. (2012). Inhibiting effect of Astragalus polysaccharides on the functions of CD4+CD25 highTreg cells in the tumor microenvironment of human hepatocellular carcinoma. Chin. Med. J. Engl. 125 (5), 786–793. doi:10.3760/cma.j.issn.0366-6999.2012.05.012
Li, R., Song, X., Guo, J., Song, P., Duan, D., and Chen, Z. (2021). Natural products: a promising therapeutics for targeting tumor angiogenesis. Front. Oncol. 11, 772915. doi:10.3389/fonc.2021.772915
Li, T., Wang, W., Zhang, S., Xing, J., and Wang, Z. (2014). Preliminary study on the effect of resveratrol on regulating the expression of STAT3 and miR-21 in anti-liver cancer. Chin. Pharmacol. Bull. 30 (02), 186–191. doi:10.3969/i.issn.1001-1978.2014.02.010
Li, W. (2022). Effect of resveratrol on oral squamous cell carcinoma invasion and metastasis by regulating the polarization state of TAMs. doi:10.27162/d.cnki.gjlin.2022.003364
Li, X., Ruan, Q., Yang, W., Tian, H., Wu, N., Qadir, J., et al. (2024). Polysaccharide isolated from Grifola frondosa eliminates myeloid-derived suppressor cells and inhibits tumor growth by enhancing T cells responses. Int. J. Biol. Sci. 20 (2), 664–679. doi:10.7150/ijbs.85276
Liang, Y. (2018). Mechanism of solanidine inhibiting proliferation of transplanted CNE-1 tumor in nude mice by reversing TAMs phenotype. Chiang-hsi Chung I Yao 49 (09), 35–38.
Liu, B. (2011). Mechanism study of anti-tumor effect of triptolide in B16-F10 tumor bearing mice based on Treg.
Liu, B., Zhang, H., Li, J., Lü, C., Gao, C., Zhang, G., et al. (2013). Triptolide downregulates Treg cells and the level of IL-10, TGF-β, and VEGF in melanoma-bearing mice. Planta Medica 79 (15), 1401–1407. doi:10.1055/s-0033-1350708
Liu, H., Zou, M., Li, P., Wang, H., Lin, X., and Ye, J. (2019). Oxymatrine-mediated maturation of dendritic cells leads to activation of FOXP3+/CD4+ Treg cells and reversal of cisplatin-resistance in lung cancer cells. Mol. Med. Rep. 19 (5), 4081–4090. doi:10.3892/mmr.2019.10064
Liu, L., Zhang, W., Cao, J., Li, W., Sun, H., and Li, B. (2020). Effect of curcumin on gene expressions of cytokines secreted by tumor-associated macrophages. J. Jilin Univ. Med. 46 (05), 930–936. doi:10.13481/j.1671-587x.20200506
Liu, P. (2023). Effective components of Pulsatilla regulate M1/M2 phenotype transformation of TAMs against colorectal cancer. doi:10.27180/d.cnki.gjxzc.2023.000022
Liu, S. (2018). Effect of Astragalus polysaccharides on myeloid derived suppressor cells in patients with non-small cell lung cancer.
Liu, Y., Zhang, C., Jiang, A., Lin, A., Han, X., Cheng, X., et al. (2024). Potential anti-tumor effects of regulatory T cells in the tumor microenvironment: a review. J. Transl. Med. 22 (1), 293. doi:10.1186/s12967-024-05104-y
Lv, C., Fan, X., Shao, W., and Li, H. (2021). Effects of astragalus polysaccharide on expression and function of regulatory T cells in elderly patients with advanced gastric cancer. Pract. Geriatr. 35 (06), 564–567. doi:10.3969/i.issn.1003-9198.2021.06.007
Ma, Z., Xia, Y., Hu, C., Yu, M., and Yi, H. (2020). Quercetin promotes the survival of granulocytic myeloid-derived suppressor cells via the ESR2/STAT3 signaling pathway. Biomed. Pharmacother. 125, 109922. doi:10.1016/j.biopha.2020.109922
María, I., Noelia, A.-G., and Eva, M. n. (2023). Regulatory cells and the effect of cancer immunotherapy. Mol. Cancer 22 (1), 26. doi:10.1186/s12943-023-01714-0
Maryam, R., Mahmoud, M., ShahrzadZamani, T., Mahdi, R., Karimi, G., Tabasi, N., et al. (2015). Evaluation of anti-cancer and immunomodulatory effects of carnosol in a Balb/c WEHI-164 fibrosarcoma model. J. Immunotoxicol. 12 (3), 231–238. doi:10.3109/1547691x.2014.934975
MdNabiul, H., Okan, C., Shivani, M. P., and Sun, D. (2022). The role of metabolic plasticity of tumor-associated macrophages in shaping the tumor microenvironment immunity. Cancers 14 (14), 3331. doi:10.3390/cancers14143331
Michaud, D., Steward, C. R., Mirlekar, B., and Pylayeva-Gupta, Y. (2021). Regulatory B cells in cancer. Immunol. Rev. 299 (1), 74–92. doi:10.1111/imr.12939
Najafi, M., Farhood, B., and Mortezaee, K. (2019). Contribution of regulatory T cells to cancer: a review. J. Cell. Physiology 234 (6), 7983–7993. doi:10.1002/jcp.27553
Noori, S., Taghikhani, M., Hassan, Z., Allameh, A., and Mostafaei, A. (2009). Tehranolide could shift the immune response towards Th1 and modulate the intra-tumor infiltrated T regulatory cells. Iran. J. Immunol. 6 (4), 216–224.
Noori, S., Taghikhani, M., Hassan, Z. M., Allameha, A., and Mostafaei, A. (2010). Tehranolide molecule modulates the immune response, reduce regulatory T cell and inhibits tumor growth in vivo. Mol. Immunol. 47 (7-8), 1579–1584. doi:10.1016/j.molimm.2010.01.007
Ortiz, L. M., Lombardi, P., Tillhon, M., and Scovassi, A. I. (2014). Berberine, an epiphany against cancer. Molecules 19 (8), 12349–12367. doi:10.3390/molecules190812349
Peng, L., Zhang, X., Zuo, A., Zhang, J., Li, S., Fang, Z., et al. (2023). Polysaccharides from Aconiturn brachypodum Diels ameliorate liver metastasis via regulating Treg cells. J. Yunnan Univ. Chin. Med. 46 (04), 75–80. doi:10.19288/j.cnki.issn.1000-2723.2023.04.015
Qin, Y., and Pang, Z. (2019). Study on myeloid inhibitory cell apoptosis induced by activation of Bcl-2 pathway by loroside. Tianjin J. Tradit. Chin. Med. 36 (03), 288–291. doi:10.11656/j.issn.1672-1519.2019.03.20
Qiu, L., Hao, Z., Hong, Y., He, W., and Zhao, W. (2018). Reprogramming tumor associated macrophage phenotype by a polysaccharide from Ilex asprella for sarcoma immunotherapy. Int. J. Mol. Sci. 19 (12), 3816. doi:10.3390/ijms19123816
Qiu, S., Wang, B., and Cai, N. (2019). Effects of berberine on the proliferation migration and invasion of A549 cells. Chin. Tradit. Pat. Med. 41 (07), 1521–1526. doi:10.3969/i.issn.1001-528.2019.07.009
Que, Z., FangYuan, Z., Zhang, A., Zheng, Y., Bi, L., Zhong, J., et al. (2014). Ganoderic acid Me induces the apoptosis of competent T cells and increases the proportion of Treg cells through enhancing the expression and activation of indoleamine 2,3-dioxygenase in mouse lewis lung cancer cells. Int. Immunopharmacol. 23 (1), 192–204. doi:10.1016/j.intimp.2014.08.001
Rampogu, S., Balasubramaniyam, T., and Lee, J. H. (2022). Phytotherapeutic applications of alkaloids in treating breast cancer. Biomed. Pharmacother. 155, 113760. doi:10.1016/j.biopha.2022.113760
Ruan, Y., Chen, W., and Feng, Y. (2023). Study of icariin’s inhibitory effects on colorectal cancer metastasis via regulations on myeloid suppressor cells function. Chin. Tradit. Pat. Med. 45 (03), 768–774. doi:10.3969/i.issn.1001-528.2023.03.013
Ruan, Z., Shang, L., Deng, X., and Cai, L. (2014). Effect of emodin on Treg cells function in a mouse model of CT26 colon cancer. J. Pract. Med. 30 (15), 2377–2380. doi:10.3969/i.issn.1006-5725.2014.15.008
Ruan, Z., Wang, P., Deng, X., Wang, L., and Zou, L. (2013). Study on the correlation between Emodin effect on Tregs migration and inhibition of colon cancer development in mice. J. Guangzhou Univ. Tradit. Chin. Med. 30 (04), 529–532+604. doi:10.13359/j.cnki.gzxbtcm.2013.04.030
Sarhan, D., Wang, J., Arvindam, U., Hallstrom, C., Verneris, M. R., Grzywacz, B., et al. (2020). Mesenchymal stromal cells shape the MDS microenvironment by inducing suppressive monocytes that dampen NK cell function. JCI insight 5 (5), e130155. doi:10.1172/jci.insight.130155
Shan, F., Somasundaram, A., Bruno, T. C., Workman, C. J., and Vignali, D. A. A. (2022). Therapeutic targeting of regulatory T cells in cancer. Trends Cancer 8 (11), 944–961. doi:10.1016/j.trecan.2022.06.008
Shang, J., Zha, H., and Sun, Y. (2020). Phenotypes, functions, and clinical relevance of regulatory B cells in cancer. Front. Immunol. 11, 582657. doi:10.3389/fimmu.2020.582657
Shen, M., Wang, Y., Liu, Z., Chen, Y., Liang, Q. K., Li, Y., et al. (2023). Inhibitory effect of Astragalus polysaccharide on premetastatic niche of lung cancer through the S1PR1-STAT3 signaling pathway. Evidence-based Complementary Altern. Med. 2023, 4010797. doi:10.1155/2023/4010797
Shokoofe, N., Zuhair, M., Mehdi, M., Zohre, H., Sohrabi, N., and Bayanolhagh, S. (2010). Sclareol modulates the Treg intra-tumoral infiltrated cell and inhibits tumor growth in vivo. Cell. Immunol. 263 (2), 148–153. doi:10.1016/j.cellimm.2010.02.009
Song, J., Eum, D., Park, S., Jin, Y., Shim, J., Park, S., et al. (2020). Inhibitory effect of ginsenoside Rg3 on cancer stemness and mesenchymal transition in breast cancer via regulation of myeloid-derived suppressor cells. Plos One 15 (10), e0240533. doi:10.1371/journal.pone.0240533
Sun, L., Zhao, J., Lan, X., and Lv, Z. (2019). Effects and mechanism of triptolide on myeloid derived suppressor cells in lung cancer-bearing mice. Zhejiang Med. J. 41 (22), 2379–2383. doi:10.12056/i.issn.1006-2785.2019.41.22.2018-1622
Sun, M., Bu, R., Zhang, B., Cao, Y., Liu, C., and Zhao, W. (2020). Lentinan inhibits tumor progression by immunomodulation in a mouse model of bladder cancer. Integr. Cancer Ther. 19 (1-7), 1534735420946823. doi:10.1177/1534735420946823
Sun, Q. (2022). Dioscin induced tumor-associated-macrophages from M2 type to M1 type and its mechanism via Cx43. doi:10.27441/d.cnki.gyzdu.2022.001177
Sun, S., He, X., Cai, W., Lv, C., and Wang, P. (2013). Effects of Astragalus polysaccharide on Treg cells in tumor-bearing mice. Chin. J. Exp. Tradit. Med. Formulae 19 (12), 176–178. doi:10.11653/syj2013120176
Tanja, D., BožićNedeljković, B., and Vladimir, J. (2019). Function of innate lymphoid cells in the immune-related disorders. Hum. Cell 32 (3), 231–239. doi:10.1007/s13577-019-00257-1
Tillmann, M., Galina, V. S., Hiam, N., Alexandra, S., Viktor, U., and Michael, R. S. (2012). Paclitaxel promotes differentiation of myeloid-derived suppressor cells into dendritic cellsin vitroin a TLR4-independent manner. J. Immunotoxicol. 9 (3), 292–300. doi:10.3109/1547691x.2011.642418
Tong, C., Yang, X., Zhou, E., and Zheng, Y. (2021). Resveratrol inhibits growth of osteosarcoma transplanted in mice. Chin. J. Immunol. 37 (05), 557–563. doi:10.3969/j.issn.1000-484X.2021.05.009
Verena, R., Matthias, P. D., and Kerstin, S. (2015). Tolerogenic dendritic cells for regulatory T cell induction in man. Front. Immunol. 6, 569. doi:10.3389/fimmu.2015.00569
Wang, H. (2018). Effect of emodin CT26 oncolon cancer mice. Chin. J. Clin. Harmacol. 34 (12), 1463–1465+1469. doi:10.13699/j.cnki.1001-6821.2018.12.020
Wang, L., Zhao, L., Gong, F., Sun, C., Du, D., Yang, X., et al. (2021). Modified citrus pectin inhibits breast cancer development in mice by targeting tumor-associated macrophage survival and polarization in hypoxic microenvironment. Acta Pharmacol. Sin. 43 (6), 1556–1567. doi:10.1038/s41401-021-00748-8
Wang, X., Wang, Y., Zhou, Q., Peng, M., Zhang, J., Chen, M., et al. (2018). Immunomodulatory effect of lentinan on aberrant T subsets and cytokines profile in non-small cell lung cancer patients. Pathology Oncol. Res. 26 (1), 499–505. doi:10.1007/s12253-018-0545-y
Wang, Y., Fan, X., and Wu, X. (2020). Ganoderma lucidum polysaccharide (GLP) enhances antitumor immune response by regulating differentiation and inhibition of MDSCs via a CARD9-NF-κB-Ido pathway. Biosci. Rep. 40 (6), BSR20201170. doi:10.1042/bsr20201170
Wang, Y., Johnson, K., GattiMays Margaret, E., and Li, Z. (2022). Emerging strategies in targeting tumor-resident myeloid cells for cancer immunotherapy. J. Hematol. Oncol. 15 (1), 118. doi:10.1186/s13045-022-01335-y
Wiguna, A. P., and Walden, P. (2015). Role of IL-10 and TGF-β in melanoma. Exp. Dermatol 24 (3), 209–214. doi:10.1111/exd.12629
Wu, T., Wang, X., and Zhu, X. (2018). The anti-lung cancer mechanisms of Silymarin via inhibiting myeloid-derived suppressor cells. J. Mod. Oncol. 26 (06), 822–827. doi:10.3969/i.issn.1672-4992.2018.06.003
Xiang, Y., Guo, Z., Zhu, P., Jia, C., and Huang, Y. (2019). Traditional Chinese medicine as a cancer treatment: modern perspectives of ancient but advanced science. Cancer Med. 8 (5), 1958–1975. doi:10.1002/cam4.2108
Xiao, Y. (2021). Study of Scutellariae Radix active components on macrophage-mediated innate anti-tumor immunity. doi:10.27405/d.cnki.gxbdu.2021.001779
Xiong, Y. X., Huang, Z. S., and Tan, J. H. (2015). Targeting G-quadruplex nucleic acids with heterocyclic alkaloids and their derivatives. Eur. J. Med. Chem. 97, 538–551. doi:10.1016/j.ejmech.2014.11.021
Xu, P., Yan, F., Zhao, Y., Chen, X., Sun, S., Wang, Y., et al. (2020). Green tea polyphenol EGCG attenuates MDSCs-mediated immunosuppression through canonical and non-canonical pathways in a 4T1 murine breast cancer model. Nutrients 12 (4), 1042. doi:10.3390/nu12041042
Xu, Q., Peng, H., Hu, Y., Xu, Z., and Xu, J. (2021). Oleanolic acid regulates the Treg/Th17 imbalance in gastric cancer by targeting IL-6 with miR-98-5p. Cytokine 148, 155656. doi:10.1016/j.cyto.2021.155656
Xue, M., Liang, H., Tang, Q., Xue, C., He, X., Zhang, L., et al. (2017). The protective and immunomodulatory effects of fucoidan against 7,12-dimethyl benz a anthracene-induced experimental mammary carcinogenesis through the PD1/PDL1 signaling pathway in rats. Nutr. Cancer 69 (8), 1234–1244. doi:10.1080/01635581.2017.1362446
Xun, J., Zhou, S., ZongJing, L., Wang, B., Luo, H., Zhang, L., et al. (2023). Dioscin modulates macrophages polarization and MDSCs differentiation to inhibit tumorigenesis of colitis-associated colorectal cancer. Int. Immunopharmacol. 117, 109839. doi:10.1016/j.intimp.2023.109839
Yang, Y., Paik, J., Cho, D., Cho, J., and Kim, C. (2008). Resveratrol induces the suppression of tumor-derived CD4+CD25+ regulatory T cells. Int. Immunopharmacol. 8 (4), 542–547. doi:10.1016/j.intimp.2007.12.006
Yuan, P. (2015). Effects of total glucosides of paeony on diethylnitrosamine-induced Hepatocellular Carcinoma and its mechanisms.
Yuan, X. (2019). Vitexin reduces tumor growth by reprogramming tumor-associated macrophages to an M1 profile. doi:10.27044/d.cnki.ggzzu.2019.001259
Yuan, X., Liu, Y., Liang, J., Liu, H., and Hou, s. (2022). Regulation of vitexin on TAMs/cd8+T cell activity in colon cancer transplanted tumor-bearing mice. Tradit. Chin. Drug Res. Clin. Pharmacol. 33 (06), 754–761. doi:10.19378/j.issn.1003-9783.2022.06.005
Yun, X., Zhang, Q., Fang, Y., Lv, C., Chen, Q., Chu, Y., et al. (2022). Madecassic acid alleviates colitis-associated colorectal cancer by blocking the recruitment of myeloid-derived suppressor cells via the inhibition of IL-17 expression in γδT17 cells. Biochem. Pharmacol. 202, 115138. doi:10.1016/j.bcp.2022.115138
Zhang, A., Zheng, Y., Que, Z., Zhang, L., Lin, S., Le, V., et al. (2014). Astragaloside IV inhibits progression of lung cancer by mediating immune function of Tregs and CTLs by interfering with Ido. J. Cancer Res. Clin. Oncol. 140 (11), 1883–1890. doi:10.1007/s00432-014-1744-x
Zhang, J., Gao, J., Cui, J., Wang, Y., Jin, Y., Zhang, D., et al. (2022). Tumor-associated macrophages in tumor progression and the role of traditional Chinese medicine in regulating TAMs to enhance antitumor effects. Front. Immunol. 13, 1026898. doi:10.3389/fimmu.2022.1026898
Zhang, L., Dermawan, K., Jin, M., Liu, R., Zheng, H., Xu, L., et al. (2008). Differential impairment of regulatory T cells rather than effector T cells by paclitaxel-based chemotherapy. Clin. Immunol. 129 (2), 219–229. doi:10.1016/j.clim.2008.07.013
Zhang, N., Liu, S., Shi, S., Chen, Y., Xu, F., Wei, X., et al. (2020). Solubilization and delivery of Ursolic-acid for modulating tumor microenvironment and regulatory T cell activities in cancer immunotherapy. J. Control. Release 320, 168–178. doi:10.1016/j.jconrel.2020.01.015
Zhang, Q. (2014). Experimental Study on Influence of the Flavonoids from Tetrastigma hemsleyanumDiels et Gilg on the Expression of Regulatory T Cells(Treg) and Related Cytokines of Lewis Lung Cancer Mice. doi:10.27465/d.cnki.gzzyc.2014.000008
Zhang, W., He, W., Shi, X., Li, X., Wang, Y., Hu, M., et al. (2018). An Asparagus polysaccharide fraction inhibits MDSCs by inducing apoptosis through toll-like receptor 4. Phytotherapy Res. 32 (7), 1297–1303. doi:10.1002/ptr.6058
Zhao, Y. (2018). The effect of Resveratrol on the number and function of G-MDSCs andits role in anti-tumor immunity.
Zhao, Y., Liu, Y., Zhou, T., Zhou, L., Su, Z., and Xu, H. (2019). Resveratrol delays tumor progression in Lewis lung cancer bearing mice by inducing apoptosis of G-MDSCs and differentiation of M-MDSCs. J. Jiangsu Univ. Med. Ed. 29 (01), 26–30. doi:10.13312/j.issn.1671-7783.y180111
Zhao, Y., Shao, Q., Zhu, H., Xu, H., Long, W., Yu, B., et al. (2018). Resveratrol ameliorates Lewis lung carcinoma-bearing mice development, decreases granulocytic myeloid-derived suppressor cell accumulation and impairs its suppressive ability. Cancer Sci. 109 (9), 2677–2686. doi:10.1111/cas.13720
Zheng, X. (2023). Quercetin inhibits colorectal cancer progression by regulating the polarization of tumor-associated macrophages via the FAM198B signaling pathway. doi:10.27465/d.cnki.gzzyc.2023.000112
Zhou, Q., Huang, H., Zheng, F., Liu, H., Qiu, F., Chen, Y., et al. (2020). Resveratrol exerts antitumor effects by downregulating CD8+CD122+ Tregs in murine hepatocellular carcinoma. OncoImmunology 9 (1), 1829346. doi:10.1080/2162402x.2020.1829346
Zhuang, H., Dai, X., Zhang, X., Mao, Z., and Huang, H. (2020). Sophoridine suppresses macrophage-mediated immunosuppression through TLR4/IRF3 pathway and subsequently upregulates CD8+ T cytotoxic function against gastric cancer. Biomed. Pharmacother. 121, 109636. doi:10.1016/j.biopha.2019.109636
Zong, S., Li, J., Ye, Z., Zhang, X., Liu, Y., Chen, X., et al. (2020). Lachnum polysaccharide suppresses S180 sarcoma by boosting anti-tumor immune responses and skewing tumor-associated macrophages toward M1 phenotype. Int. J. Biol. Macromol. 144, 1022–1033. doi:10.1016/j.ijbiomac.2019.09.179
Zou, Y., Zhang, J., and Guan, X. (2023) “Study on the anti-OSCC effect and mechanism of ASH and buccarone on macrophages,” in Paper presented at the the 15th national conference on oral mucosa and the 13th national conference on the integration of traditional Chinese and western medicine of Chinese stomatological association. doi:10.26914/c.cnkihy.2023.090177
Keywords: regulatory immune cells, natural products, polysaccharides, polyphenols, tumor therapy
Citation: Song P, Song F, Shao T, Wang P, Li R, Chen Z-S, Zhang Z and Xue G (2024) Natural products: promising therapeutics for targeting regulatory immune cells in the tumor microenvironment. Front. Pharmacol. 15:1481850. doi: 10.3389/fphar.2024.1481850
Received: 16 August 2024; Accepted: 23 October 2024;
Published: 13 November 2024.
Edited by:
Daotai Nie, Southern Illinois University Carbondale, United StatesReviewed by:
Jin Zhang, University of Mississippi Medical Center, United StatesTomasz M. Karpiński, Poznan University of Medical Sciences, Poland
Copyright © 2024 Song, Song, Shao, Wang, Li, Chen, Zhang and Xue. This is an open-access article distributed under the terms of the Creative Commons Attribution License (CC BY). The use, distribution or reproduction in other forums is permitted, provided the original author(s) and the copyright owner(s) are credited and that the original publication in this journal is cited, in accordance with accepted academic practice. No use, distribution or reproduction is permitted which does not comply with these terms.
*Correspondence: Guozhong Xue, Z3VveHVlLmx6QDE2My5jb20=; Zhaofang Zhang, ODQxMzIwMTQzQHFxLmNvbQ==; Rongkun Li, ODczMTE3MDcxQHFxLmNvbQ==
†These authors share first authorship