- 1School of Pharmaceutical Sciences, Hunan University of Medicine, Huaihua, China
- 2School of Pharmacy, Shandong Second Medical University, Weifang, China
- 3School of Pharmacy, Baotou Medicai College, Baotou, China
The botanical drugs of genus Duhaldea DC. have been traditionally utilized in folk medicine for the treatment of a wide array of illnesses, encompassing fractures, bone wounds, carbuncles and poisoning, bronchitis, bruises, giddy with hypertension of qi, and lung deficiency cough. The genus Duhaldea DC. comprises 15 species widespread in Central, East, and Southeast Asia and 7 species (2 endemic) in China. The review aims to provide a systematic overview of ethnopharmacology, phytochemistry, and pharmacology of Duhaldea DC. and to explore the future therapeutic potential and scientific potential of this genus. The data were systematically collected from books and scientific databases such as PubMed, Web of Science, Google Scholar, CNKI, and doctoral and master’s theses. To date, a total of 352 metabolites have been isolated from this genus, and terpenoids, flavonoids, phenylpropanoids, and inositol angelates are the primary contributors to the pharmacological activities of Duhaldea DC. The crude extracts and isolated phytochemical metabolites from this genus have been shown to exhibit various pharmacological activities, including anti-inflammatory, antimicrobial, anti-osteoporotic, anticancer, and antioxidant activities. Despite notable advancements in our understanding of the chemical constituents and pharmacological properties of Duhaldea DC., it is absolutely crucial to conduct additional research into the pharmacology and toxicology of these species to definitively ascertain their safety, efficacy, and quality.
1 Introduction
Previously, plants of the genus Duhaldea DC. were considered to belong to the genus Inula L. (Cheng et al., 2014a; Li, 2017). However, in the last decade or so, plant taxonomists have concluded that these plants should become a separate genus under the family Asteraceae, and the scientific name of the genus has been revised to Duhaldea DC (Wu and Peter, 2011). Duhaldea DC consists of 15 species, mainly shrubs or perennial botanical drugs. The genus is widely distributed in Asia, with seven species in China, of which two are endemic (Wu and Peter, 2011). The stem is erect. The leaves are alternate and densely hairy. The capitulum is often solitary or found in dense terminal corymbs. It has multiple total bracts, with white edge flowers. The disk florets are bisexual, either yellow or whitish. The achenes are ellipsoid and hairy, with an epidermis featuring elongated crystals (Ding and Jin, 2017).
Species in the genus Duhaldea DC. are traditionally used for the treatment of stomachache, relieving rheumatism, fractures, bone wounds, asthma, headache, rheumatism, peptic ulcers, loose motions, indigestion, bronchitis, angeitides, vasculitis, and dizziness (Long, 2004; Yan et al., 2011; Jyoti et al., 2017; Huang et al., 2021a). Duhaldea DC. species are rich in sesquiterpene lactones, sesquiterpenes, phenylpropanoids, inositol derivatives, triterpenes, and flavonoids, which authenticate their medicinal importance (Baruah et al., 1982; Yan et al., 2010; Cheng et al., 2014b; Wu et al., 2015; Zheng et al., 2015). Anti-inflammatory, piscicidal, anti-osteoporotic, and anticancer activities exhibited by various extracts and metabolites isolated from the genus Duhaldea DC. showed its pharmacological importance (Yoshida et al., 1995; Xie et al., 2007; Wang et al., 2013; Tai et al., 2014). D. nervosa and D. cappa are the most popular, widely utilized, and highly investigated species in the genus despite the relatively low number of other species within the genus, which are more or less underexplored. This species is an important medicinal species extensively used in Asian countries to treat stomachache and rheumatism (Yan et al., 2011).
This review aims to establish a relationship between traditional uses and scientific studies by critically assessing the available literature on ethnopharmacology, phytochemistry, and pharmacology, possible mechanisms of action, and toxicology of the plant species from the genus Duhaldea DC. Furthermore, this review also highlights the various research gaps for the better exploitation of this genus and provides a baseline for future research studies. Related scientific literature studies up to March 2024 were collected from the following databases: PubMed, Elsevier, Web of Science, Springer, ScienceDirect, Wiley, ACS, CNKI, and doctoral and master’s theses. The search terms included “Duhaldea,” “rubricaulis,” “wissmanniana,” “lachnocephala,” “forrestii,” “nervosa,” “cappa,” “pterocaula,” “eupatorioides,” “cuspidata,” “griffithii,” “latifolia,” “revoluta,” “simonsii,” “xiaoheiyao,” “caoweiling,” and “baimianfeng.” The collected data from different sources were comprehensively summarized for botany, ethnopharmacology, phytochemistry, pharmacology, and toxicology of the genus Duhaldea DC. ChemDraw 20.0 was used to extract the chemical metabolites. The PubChem database (https://pubchem.ncbi.nlm.nih.gov) was used to confirm the chemical classifications and structures, and iPlant (https://www.iplant.cn/) was used to verify the names of the plants.
2 Traditional uses and ethnopharmacology
Botanical drugs have been used to treat an array of conditions by humans since ancient days (Schulz et al., 2001). Duhaldea DC species are widely distributed in Asia. The botanical drug uses of the genus Duhaldea DC are summarized in Table 1.
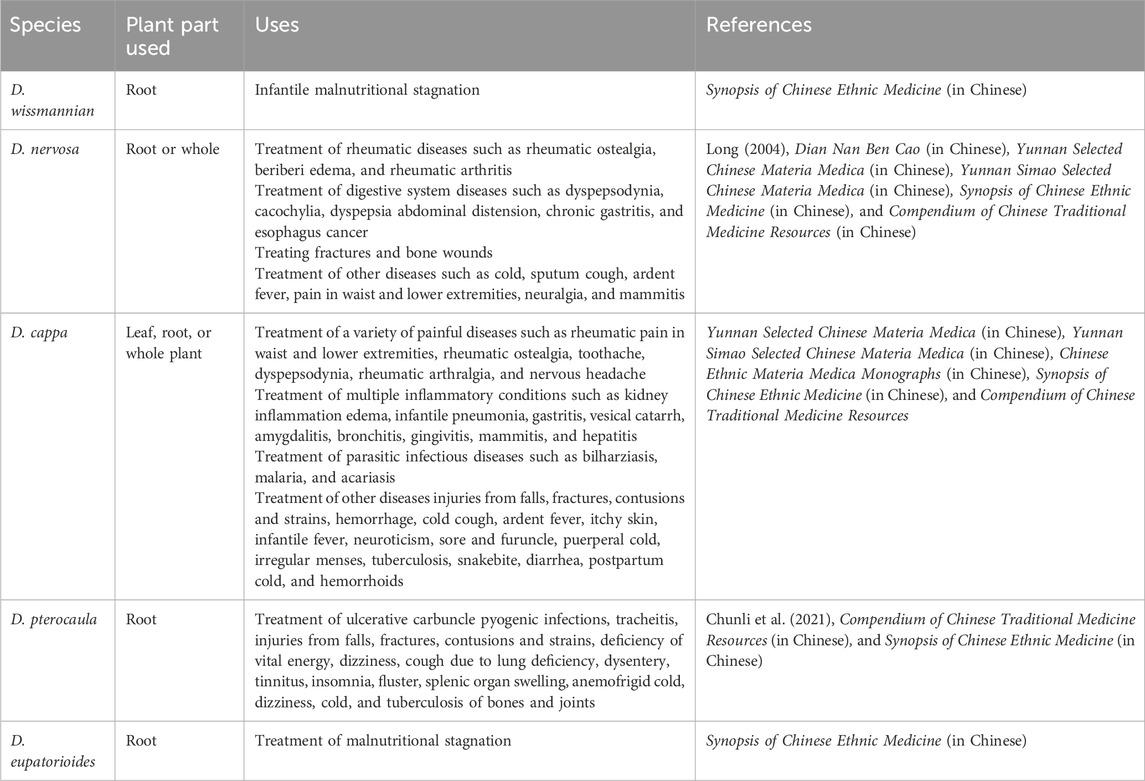
Table 1. Summary of the ethnobotanical uses of the genus Duhaldea DC (species name, plant part, country, uses, mode of administration, and associated references).
3 Phytochemistry
3.1 Preliminary phytochemical screening
Early phytochemical studies of Duhaldea DC. were conducted in the 1990s and revealed the presence of saponins in the sesquiterpenoids of this species (Baruah et al., 1980; Goswami et al., 1984). Large sesquiterpenoids were also recently identified in the alcohol extract of D. wissmanniana (Cheng et al., 2013; Cheng et al., 2014b). Other studies in subsequent years have led to the isolation and identification of phenylpropanoids, flavonoids, inositol angelates, phenolic metabolites, phenolic, monoterpenes, diterpenes, and triterpenes from acetone, chloroform, methanol, and ethanol extracts of D. cappa (Wang et al., 2012; Zhou, 2017). Moreover, a study on the chemical metabolites of the root of D. cappa resulted in the extraction and characterization of a mixture of five ceramide metabolites from this species (Guo et al., 2007). In the past few decades, liquid chromatography–mass spectrometry (LC-MS), especially ultra-high-performance liquid chromatography–high-resolution mass spectrometry (UHPLC-HRMS), has become the most powerful and reliable analytical instrument in the detection and characterization of metabolites from traditional Chinese medicine, drug, or biological samples (Wei et al., 2020). UHPLC-HRMS is an advanced form of an analytical technique used to separate and identify the complex mixture of metabolites found in botanical drugs. It is important to make generalization about the fragmentation pathways of reference metabolites using the HRMS technique to speculate the identity of potential metabolites in genus Duhaldea DC. (Ma et al., 2022). In the study of the chemical composition of the whole botanical drug and inflorescence of D. nervosa, UHPLC-Q-Exactive Orbitrap mass spectrometer and UHPLC-QTOF-MS/MS were used, and 149 chlorogenic acid derivatives and 34 metabolites were finally identified, respectively (Wei et al., 2020; Wei et al., 2022). UHPLC-Q-Exactive Orbitrap mass spectrometer and UHPLC-QTOF-MS/MS were used in the study of the chemical composition of D. cappa, and 68 chlorogenic acid derivatives and 12 metabolites were finally identified, respectively (Peng et al., 2017; Peng et al., 2021).
3.2 Chemical composition
Detailed phytochemical studies on Duhaldea DC. have revealed in an array of secondary metabolites. Many researchers, especially in the past 40 years, have discovered new metabolite structures from Duhaldea DC. To date, a total of 393 chemical metabolites have been reported from Duhaldea DC. species, including sesquiterpenoids, monoterpenes, diterpene, triterpenes, inositol angelates, phenylpropanoids, flavonoids, phenolic metabolites, and ceramide metabolites (Figure 1). The detailed information on these metabolites is summarized in Supplementary Table S1.
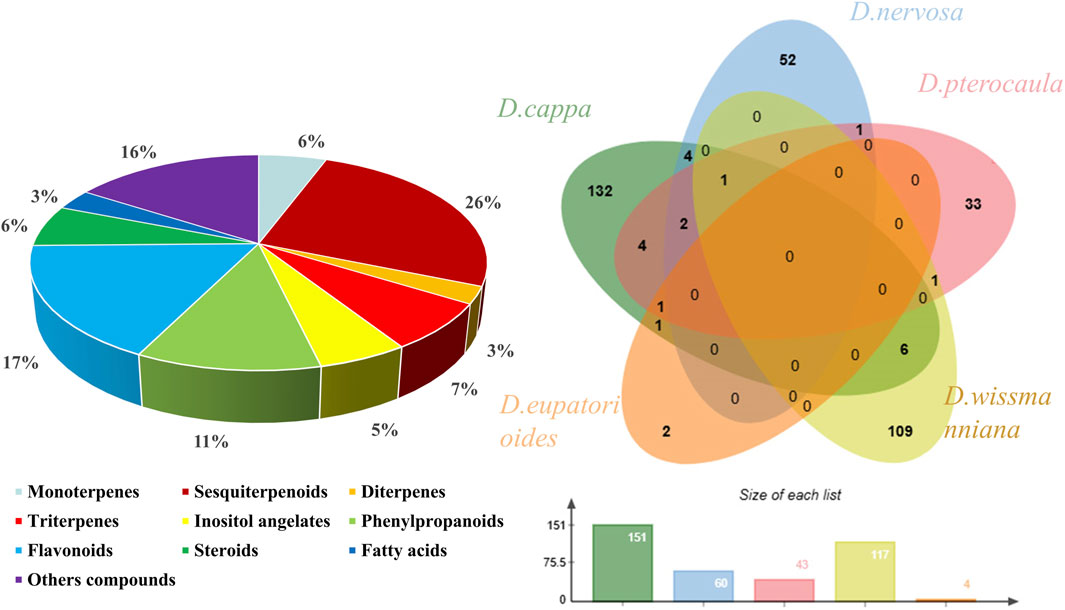
Figure 1. Chemical classes and proportions of phytochemical metabolites isolated and characterized from the genus Duhaldea DC.
3.2.1 Monoterpenes
Monoterpenes belong to a large and diverse group of naturally occurring metabolites. The basic structure of monoterpenes, or monoterpenoids, consists of two linked isoprene units. They might be cyclized and oxidized in a variety of ways. Due to their low molecular weight, many of them exist in the form of essential oils. Many monoterpenes and their derivatives have anti-inflammatory, antimicrobial, anticonvulsant, analgesic, antiviral, anticancer, antituberculosis, and antioxidant biological activities (Zielińska Błajet and Feder Kubis, 2020). A total of 20 monoterpenes (1–20) have been reported from the genus Duhaldea DC., and Figure 2 shows their chemical structures.
3.2.2 Sesquiterpenoids
In the study of chemotaxonomy based on secondary metabolites from the Asteraceae family, sesquiterpene lactones, which are used as taxonomic markers, are a more studied class of secondary metabolites. More than 4,000 sesquiterpene lactones with around 30 different skeletons have been reported from several tribes of Asteraceae (Wu et al., 2006). A total of 90 sesquiterpenoids (21–110) have been reported from the genus Duhaldea DC., and Figure 3 shows their chemical structures.
3.2.3 Diterpenes
Diterpenes are the product of the mevalonic acid biosynthesis pathway. Some diterpenes have antiviral activity, such as kirkinine, Excoecaria toxin (anti-HIV), jiadifenoic acids JP (anti-Coxsackie virus), briaexcavatolide U, briaexcavatin L (anti-HCMV), genkwanin P, laurifolioside A (anti-HBV), linearol, isosidol (anti-HPIV-2), and debromoaplysiatoxin (anti-CHIKV) (Wardana et al., 2021). A total of nine diterpenes (111–119) have been reported from the genus Duhaldea DC., and their chemical structures are shown in Figure 4.
3.2.4 Triterpenes
Triterpenoids are a class of terpenoids with a basic skeleton of 30 carbon atoms consisting of six isoprene units, which exist in the plant body in free form or in the form of glycosides or esters combined with sugars, and have a wide range of biochemical activities, such as anti-inflammatory and antitumorigenic activities (Gill et al., 2016; Miranda et al., 2022). At the same time, triterpenes play a vital role in the formation of structures in plant membranes, which stabilize the phospholipid bilayers in the cell membranes (Liby et al., 2007). A total of 25 triterpenoids (120–144) have been reported from the genus Duhaldea DC., and their chemical structures are shown in Figure 5.
3.2.5 Inositol angelates
Inositol, also known as cyclohexane hexanol, is widely distributed in animals and plants and is a growth factor for animals and microorganisms. It was first isolated from the heart muscle and liver. Muscle inositol is an essential nutrient source for birds and mammals, and a deficiency of muscle inositol can, for example, cause symptoms such as hair loss in mice and periocular abnormalities in rats. Furthermore, inositol deficiency may be involved in the pathogenesis of diseases, such as metabolic syndrome, spina bifida (a neural tube defect), polycystic ovary syndrome, and diabetes (Marine and Christophe, 2013; Kiani et al., 2021; Dorina et al., 2023). A total of 19 inositol angelates (145–163) have been reported from the genus Duhaldea DC., and their chemical structures are shown in Figure 6.
3.2.6 Phenylpropanoids
Phenylpropanoids form a class of metabolites with a core structure consisting of a phenyl group attached to a three-carbon chain. This C6–C3 carbon skeleton is usually obtained from the enzymatic deamination of the aromatic amino acid phenylalanine. Phenylpropanoids, owing to their antimicrobial and antioxidant activities, are applied in pharmaceutical products as a preservative. Currently, in many countries, phenylpropanoid derivatives have been approved as food additives and active metabolites in skincare products (Neelam et al., 2020). A total of 39 phenylpropanoids (164–202) have been reported from the genus Duhaldea DC., and their chemical structures are shown in Figure 7.
3.2.7 Flavonoids
Flavonoids are a group of polyphenolic metabolites produced in plants as secondary metabolites. Flavonoids are a class of yellow pigments derived from 2-phenylchromanone as the mother nucleus, including the isomers of flavonoids and their hydrogenation and reduction products, i.e., a series of metabolites with C6-C3-C6 as the basic carbon framework. Flavonoids are widely found in fruits, vegetables, and other food crops. They have favorable biochemical effects on multiple diseases (e.g., cardiovascular disease and atherosclerosis), as well as other bioactivities (e.g., anti-inflammatory, antiviral, and antioxidant activities) (Serafini et al., 2010; Fardoun et al., 2020; Badshah et al., 2021; Shen et al., 2022). A total of 61 flavonoids (203–263) have been reported from the genus Duhaldea DC., and their chemical structures are shown in Figure 8.
3.2.8 Steroids
Steroid is a general term for a large group of cyclopentane-thickened fully hydrogenated phenanthrene derivatives that are widely distributed in living organisms. Steroids are widely known for their potent anti-inflammatory and immune-modulating activities (Neilsen et al., 2014). A total of 22 steroids (264–285) have been isolated from the genus Duhaldea DC., and their chemical structures are shown in Figure 9.
3.2.9 Fatty acids
Fatty acids are carboxylic acids with a typical RCOOH structure, containing a methyl end, a hydrocarbon chain (R), and a carboxylic terminus. Fatty acids have both a systematic and a common name (e.g., octadecanoic and stearic). Fatty acids play multiple roles in humans and other organisms. At the same time, fatty acids are a substantial part of lipids, one of the three major metabolites of biological matter (along with proteins and carbohydrates) (Tvrzicka et al., 2011). A total of 11 fatty acids (286–296) have been reported from the genus Duhaldea DC., and their chemical structures are shown in Figure 10.
3.2.10 Other metabolites
In addition to the above metabolites, Duhaldea DC. also contains phenolic acids, chlorogenic acid, organic acids, and polyols. These metabolites are categorized as other because they rarely exert similar pharmacological effects as a class of metabolites or they occur less frequently in the genus. For example, Wang et al. isolated and purified a novel water-soluble polysaccharide (DNP-1) from the root of D. nervosa via column chromatography (Wang et al., 2023). This is the only isolation of polysaccharides that we have found in our research searches of this genus. A total of 56 other metabolites (297–352) have been reported from the genus Duhaldea DC., and their chemical structures are shown in Figure 11.
4 Pharmacology
Present-day research and the study of historical texts have shown that whole plants or their respective plant parts have been used to treat or alleviate different illnesses. Currently, botanical drugs are also used in the conventional system of medicine, where plants and associated phytochemicals are being actively explored for their direct use as pharmaceutical agents. At the same time, it can be noted that the plant is used as a medicine and food, is usually used as a spice and seasoning in food, and also prevents and controls some diseases in people.
The genus Duhaldea DC. has long been used therapeutically in different countries in Asia due to its wide range of biological and pharmacological activities. The broad-spectrum ethnomedicinal uses of the different species of Duhaldea DC. have led to the initiation of several pharmacological investigations, such as anti-inflammatory, antioxidant, antitumor, and antibacterial activities. A brief summary of these pharmacological effects is given in Figure 12. An overview of the modern pharmacological studies on these species is detailed in the following sections.
4.1 Anti-inflammatory activity
Inflammation is a defensive response of the body to stimulation, manifested by redness, swelling, heat, pain, and dysfunction. In immune cells, macrophages are the main cell type involved in the inflammatory process. RAW 264.7 macrophages are a good model for screening anti-inflammatory drugs. The modern pharmacology of Duhaldea DC has proven to have significant anti-inflammatory effects, and its main material basis of anti-inflammation is sesquiterpenoids. A total of 21 eudesmane and germacrane derivatives were isolated from D. wissmannian. These isolates exhibited significant inhibitory effects on lipopolysaccharide (LPS)-induced nitric oxide (NO) production in RAW 264.7 macrophages (20, 5, 0.5, and 0.1 µM), with aminoguanidine as a positive control (Cheng et al., 2013; Cheng et al., 2014b). Wang et al. screened a total of 35 monomers isolated from D. wissmannian, including sesquiterpenoids, monoterpenes, and flavonoid lignans, for their inhibitory effects on NO metabolism in LPS-induced RAW 264.7 macrophages. Four concentration gradients of 50, 10, 2, and 0.4 µM were used to determine the metabolic inhibition rate and cytotoxicity of each monomer metabolite on NO, and 1β-hydroxy-alantolactone and inulicin were used as a positive control. The results showed that the sesquiterpenoids containing α-methylene-γ-lactone fragments exhibited strong NO metabolism inhibition, and some flavonoid lignans and myo-inositol derivatives also showed some anti-inflammatory activities (Wang, 2013). The D. wissmannian eudesmane-derivatized sesquiterpene, Chengwissmanolide A (IC50 = 0.38 μM), showed stronger or similar NO inhibitory activity to the positive drug aminoguanidine (0.20 or 0.37 μM) (Cheng, 2012). Four new sesquiterpenes, pterocaullins A−D (1–4), along with 10 known metabolites isolated from the whole plants of D. pterocaula, were tested for their inhibitory effects against LPS-induced NO production in RAW 264.7 macrophages using parthenolide (10 μM) as a positive control. Pterocaullin B exhibited promising anti-inflammatory activity, with an IC50 value of 7.03 µM (Zhu et al., 2019).
Other types of metabolites in the genus also have good anti-inflammatory activity. A new polysaccharide, DNP-1, was isolated from D. nervosa and inhibited the concentrations of NO, TNF-α, MCP-1, IL-2, and IL-6 pro-inflammatory factors in LPS-induced RAW 264.7 cells at concentrations of 50, 100, and 150 μg/mL (Wang et al., 2023). Wang et al. isolated 17 metabolites from D. wissmannian, and the anti-inflammatory activity of RAW 264.7 macrophages against LPS-induced NO production was also evaluated. Metabolites 3-acetate-1,2,4,5-tetrakis(2-methyl-2-butenoate) inositol, 2,3-dehydrosilychristin, hydnocarpin, 8-hydroxy-7,9-di-isobutyryloxythymol, and 7-hydroxy-8,9-bis(isobutyryloxy)thymol showed moderate activities, with IC50 values of 13.3, 19.6, 23.3, 10.8, and 10.1 μM, respectively. On the other hand, 4-acetate-1,2,3,5-tetrakis (2-methyl-2-butenoate) inositol, 23-o-acetylsilychristin A, isohydnocarpin, silybin B, and isosilybin A exhibited weak activities, with IC50 values of 32.6, 36.7, 48.2, 50.5, and 50.5 μM, respectively (Wang et al., 2013).
The metabolite (4R,5R,6S,7S,9S,10R)-9-angeloyloxy-4,5-epoxygermacra-11 (13)-en-12,6-olide, isolated from D. wissmannian, demonstrated potent inhibition of NO with an IC50 value of 1.04 ± 0.07 μM. Aminoguanidine served as the positive control and exhibited its inhibition with an IC50 value of 0.79 ± 0.05 μM (Cheng et al., 2014b). Maoxiucaioside A obtained from D. nervosa. has a significant inhibitory effect on the release of TNF-α, IL-6, and IL-1β, with IC50 values of 17.90 ± 2.29 μM, 19.45 ± 2.16 μM, and 18.54 ± 1.30 μM, respectively, slightly lower than those of dexamethasone (Li et al., 2023). The metabolites derived from D. nervosa, i.e., nervolan A, nervolan B, nervolan C, coniferyl diangelate, and sinapyl diangelate, were evaluated for their inhibitory effects on LPS-induced NO production in RAW 264.7 cells. The results demonstrated that these metabolites exhibited mild inhibitory activities against NO production, with IC50 values of 33.31, 15.43, 21.32, 16.19, and 33.56 μM, respectively (Yan et al., 2010). The five known analogs, isolated from D. cappa, exhibited anti-inflammatory activities against the production of NO in RAW 264.7 cells stimulated by LPS. Their IC50 values ranged from 7 to 23 μM, with celecoxib (IC50 1.6 μM) serving as the positive control (Wu et al., 2015).
The D. cappa methanolic extract (75 and 150 mg/kg) showed reduction in the rat paw edema, significant inhibition of the cotton pellet-induced granulomas in rats, and potential immunomodulatory activity in all the assays performed (Jyoti et al., 2017). The D. cappa root ethanol extract (5 g/kg) significantly inhibited xylene-induced ear swelling and acetic acid-induced capillary permeability in mice and has certain anti-inflammatory effects (Mo et al., 2012), and 60% ethanol fraction of D. cappa is the main anti-inflammatory constituent (Gong et al., 2018; Wang et al., 2018). The D. cappa aqueous extract (24 g/kg) also improved the inflammatory response of severe pneumonia rats, which may be related to the inhibition of the TLR2/MyD88/NF-κB signaling pathway (Aizaizi et al., 2018). The D. cappa aqueous extract (24 g/kg) can improve inflammation of severe pneumonia induced by Klebsiella pneumoniae by blocking p38 MAPK and NF-κBp65 signaling pathways (Li, 2017).
4.2 Antitumor activity
Tumors are formed by the proliferation of local tissue cells under the action of various tumorigenic factors. According to the cell characteristics of tumors and the degree of harm to the body, tumors are divided into benign tumors and malignant tumors. Cancer, a malignant tumor, has become the second leading fatal disease after cardiovascular and cerebrovascular diseases and seriously threatens human health and life. Modern pharmacological studies have shown that Duhaldea DC. has certain anticancer effects, and its main anticancer substance is sesquiterpenoids. Cheng et al. evaluated all 21 isolates; these isolates showed no significant toxicity in RAW 264.7 macrophages at concentrations up to 20 μM. Metabolites (5R,7R,10S)-4,5-epoxy-4,5-secoeudesma-1,3-dien-12,5-olide, 3-oxoeudesma-1,4,11 (13)-trien-12,6β-olide, haageanolide, and 11,13-dehydroisohyposantonin had strong inhibitory effects on NO, with IC50 values of 0.68, 0.68, 0.97, and 0.65 μM, respectively. Moreover, the cytotoxicity assay in four human tumor cell lines suggested that new metabolites (5R,7R,10S)-4,5-epoxy-4,5-secoeudesma-1,3-dien-12,5-olide, (2R,4E,6R,7S,9S,10R)-2-acetoxy-9-angeloyloxygermacra-4,11(13)-dien-12,6-olide, 4E-9β-angeloyloxy-2α-hydroxy-7α,10αH-germacra-4,11(13)-dien-12,6α-olide (4E,6R,7S,9S,10R)-9-angeloyloxygermacra-4,11 (13)-dien-12,6-olide, 4E-9β-methacryloxy-7α,10αH-germacra-4,11 (13)-dien-12,6α-olide, 4E-9β-hydroxy-7α,10αH-germacra-4,11 (13)-dien-12,6α-olide, and 3-oxoeudesma-1,4,11 (13)-trien-12,6β-olide showed strong toxicity against HepG2, PC-3, and MGC-803 cells (Cheng et al., 2013). Five germacrane-type sesquiterpene lactones (ineupatolide D, ineupatolide E, dvaricin B, nepalolide C, and inculacappolide) were isolated from D. cappa showed moderate inhibitory effects on A431, A549, BGC-823, HL-60, HT-29, and MCF-7 cancer cell lines with IC50 values ranging from 2.1 to 36.3 µM, and doxorubicin was used as the positive control (Wu et al., 2017). The cytotoxicity assay showed that inulacappolide has anti-proliferative effects against human cervical cancer (HeLa), human leukemia (K562), and human nasopharyngeal carcinoma (KB) cell lines, with IC50 values of 1.2 mM, 3.8 mM, and 5.3 µM, respectively (Xie et al., 2007). The sesquiterpene metabolite ineupatorolide B, isolated from D. cappa, exhibited potent growth-inhibitory activity against HeLa cells, whereas its activity against MM1-CB melanoma cells was weaker. The mechanism by which ineupatorolide B exerts its growth-inhibitory effects may involve the activation of PKCα, leading to an enhancement of the retrotranscriptional activation capacity of NFAT (Mei et al., 2015).
As a medicinal plant, this genus not only serves as a flavorful seasoning but also inhibits the production of carcinogens in food. Cheng et al. discovered that the electrophilic metabolites act as creatinine inhibitors to reduce the generation of heterocyclic aromatic amines. Their research reveals that the rhizome of D. nervosa, when used as a spice, can inhibit the production of carcinogens in food (Cheng et al., 2023). D. nervosa shows greater potential as a functional food for cancer prevention and anticancer effects. Under the condition of simulated gastric fluid in vitro, the stem and leaf extracts of D. nervosa can effectively remove nitrite and block the production of nitrosamines (Yuan et al., 2021).
4.3 Antioxidant activity
An increasing body of research indicates that antioxidants play a crucial role in mitigating the effects of aging as free radicals and oxidants contribute to cellular and tissue degradation, impair metabolic functions, and are associated with various health issues. Cheng et al. compared the chemical space and antioxidant activities of ethanol extracts from different parts of D. nervosa. Findings support the traditional use of its roots and indicate thymol di-isobutyrate as a major functional factor. The results showed that significant correlations and extracts upregulated the mRNA expression of antioxidant response actors in H2O2-challenged HepG2 cells, hence also cueing the potential antioxidant activity of other parts (Cheng et al., 2020). He et al. compared the antioxidant effects of ultrasonic alcoholic extracts from different parts of the plant through in vivo and ex vivo experiments. In vitro, the extract amount was positively correlated with the clearing effect of free radicals at certain drug concentrations, with VC as the positive drug. In vivo, control and aging model groups had different treatments, while the experimental group had low, medium, and high (300, 600, and 1,200 μg g−1·d−1)-dose intragastric administrations. The results showed good free radical scavenging and antioxidant capacity, with the underground part having a significantly higher antioxidant effect than the aboveground part (He et al., 2016). Yang et al. investigated the antioxidant activity of polysaccharides from above-ground and below-ground parts of D. nervosa and total flavonoid of D. nervosa and found that the reducing ability of polysaccharide and flavonoid, the inhibiting ability of superoxide anion free radical, and the scavenging ability of the DPPH radical were greater than that of para-rutin at high concentration, and the antioxidant ability was higher than that of rutin with the increase in concentration. They show a high scavenging rate of hydroxyl radicals (Tao et al., 2015; Tao et al., 2016). Kalola et al. used the DPPH radical scavenging assay, superoxide radical scavenging assay, measurement of reducing power, and measurement of the effect on lipid peroxidation in rat liver homogenates, and butylated hydroxytoluene, gallic acid, and ascorbic acid were used as the positive control. The results showed that the D. cappa methanolic extract and the ethyl acetate-soluble fraction exhibited higher antioxidant activity (Kalola and Shah, 2006). The volatile oil (310–520 mg/L) of D. cappa has some scavenging ability for both hydroxyl radicals (positive control: mannitol and thiourea) and superoxide anion radicals (positive control: VC), but the scavenging activity of hydroxyl radicals is stronger (Liu et al., 2009a). D. nervosa extracts (0.05 g/g) can also effectively inhibit the oxidation of oils (Liu et al., 2011).
4.4 Antibacterial activity
Antimicrobial activity is an important indicator for screening natural metabolites for their potential to become antibiotics. Ethanol extracts (10 μg/mL) and petroleum ether extracts (10 μg/mL) of D. nervosa significantly inhibited Staphylococcus aureus and Bacillus subtilis (Liu et al., 2011). The essential oil derived from D. cappa comprises approximately 50% sesquiterpene hydrocarbons. Streptomycin (30 μg/disk) and erythromycin (15 μg/disk) were used as positive controls, while hexane was taken as a negative control, and at the concentration of 1,000 μL/mL, it has a significant inhibitory effect on Enterococcus faecalis, Klebsiella pneumoniae, Xanthomonas viripennis, and B. subtilis (Priydarshi et al., 2016). The antimicrobial activity of the flavonoid extracts from D. cappa was studied against nine microorganisms. The results showed that the minimal inhibitory concentration (MIC) of the D. cappa flavonoids to Sarcina lutea was the best with a ratio of 0.0039 g/mL; the MIC of E. faecalis, B. subtilis, and S. aureus was 0.1250 g/mL; the MIC of Escherichia coli, Salmonella typhimurium, and Salmonella paratyphi A was 0.5000 g/mL but no obvious inhibitory effect on Proteus vulgaris and Candida albicans (Liu W. et al., 2010). Liu et al. showed that the MIC values of roots, stems, and leaves against S. aureus were 15.63, 62.5, and 62.5 mg/mL, respectively, while the MIC values of roots, stems, and leaves against Pseudomonas aeruginosa were 15.63, 31.25, and 62.5 mg/mL, respectively (Liu et al., 2009b). The antimicrobial effects of different extracts from different parts of D. cappa on different bacteria were compared, among which the glacial acetic acid extract had the best antimicrobial effect, the root and leaf extracts had better antimicrobial effects than the stem extract, and the extracts had better inhibitory effects on Gram-positive than Gram-negative bacteria (Liu S. et al., 2010). The growth of 15 plant pathogenic fungi and 4 bacteria could be inhibited by 100 mg/mL of thymol isolated from D. cappa (Xie et al., 2012). By comparing the various extraction and separation fractions of the ethanol extract of D. cappa, the chloroform:acetone 10:0 fraction of the ethyl acetate extract exhibited the most significant antibacterial activity at a concentration of 2.0 mg/mL, followed by the ethyl acetate extract and the chloroform:acetone 9:1 fraction (Li et al., 2020).
4.5 Others’ activity
The electrophilic metabolites (0.5, 2.0, and 8.0 μg/mL) of D. nervosa attenuated hepatic steatosis in FFA-treated HepG2 cells. Studies suggest that it improved hepatic lipid metabolism disorder, Krebs cycle activity, oxidative phosphorylation, and the cellular and mitochondrial redox status. Additionally, 10-isobutyryloxy-8,9-epoxythymol isobutyrate activates the Nrf2-ARE signaling pathway by upregulating Nrf2 expression and promoting Nrf2 nuclear translocation (Cheng et al., 2022). Dong et al. found that the ethyl acetate fraction of D. nervosa (0.5–2.0 μg/mL) effectively reduced hepatic lipid accumulation and ROS production, lowered TG and TC levels, and enhanced antioxidant enzyme activity. The depletion of electrophilic components reduced its efficacy and regulatory effects on lipid metabolism and redox-related gene expression (Dong et al., 2020).
The ethyl acetate fraction of D. pterocaula (40 mg/kg, 100 μL) demonstrated potent analgesic effects in inflammatory pain mouse models and caused no anti-nociceptive tolerance (Huang et al., 2021b). The alcoholic extract of the D. cappa root (5 g/kg) significantly inhibited the pain response induced by the hot plate method and the twisting method in mice and had some analgesic effects (Mo et al., 2012). Kiran et al. and Yupei et al. developed a hepatotoxicity model through the intraperitoneal injection of carbon tetrachloride suspended in sterile oil or D-GalN. Silymarin at a dosage of 100 mg/kg and bifendate at 0.2 g/kg were identified as positive control drugs. The dosages for the water extract of D. cappa were administered at 9.8 g/kg, 19.5 g/kg, and 39.0 g/kg and 200 mg/kg and 400 mg/kg, and the effects on hepatotoxicity were assessed after a 10-day treatment period. The aqueous extract of D. cappa chrysanthemum showed a hepatoprotective effect against carbon CCl4 and D-GalN-induced hepatotoxicity in rats (Kiran et al., 2017; He et al., 2019). Yang et al. screened a PXR-HepG2 cell model with high expression of the pregnane X receptor. A negative control group (DMSO group, 0.1%), a drug treatment group (25, 50, and 100 mg/L), and a positive control group were treated with the pregnane X receptor agonist rifampicin (10 μmol/L) for 24, 48, and 72 h, respectively. Yang et al. showed that D. cappa can affect the expression of P450 enzymes in primary rat hepatocytes and HepG2 cells with high expression of the pregnane X receptor (Chang et al., 2020).
The methanol and dichloromethane extracts of D. cappa are potent inhibitors of herpes simplex virus infection in vitro. The D. cappa methanol extract exhibited significantly higher anti-herpes simplex virus (HSV) activity than the D. cappa dichloromethane extract as it inhibited more than 50% of the virus. The 50% effective doses of the D. cappa methanol extract against HSV-1 and HSV-2 were determined to be 720.1 ± 32.7 μg/mL and 529.2 ± 5.2 μg/mL, respectively (Nikomtat et al., 2011). The 12 isolated triterpenoids were screened for their anti-osteoporotic activities, and the metabolites oleanoic acid 3-O-(β-D-glucopyranosyl)-28-O-β-D-glucopyranosyl ester and 2β-hydroxyolean-3-O-(β-D-glucopyranosyloxy)-12-en-23, 28-dioic acid showed good anti-osteoporotic activities with IC50 values of 86.3 and 51.6 μg/mL, respectively, and their inhibitory effects were slightly lower than those of the positive control, teriparatide (45.6 μg/mL) (Tai et al., 2014). Fujita et al. studied the mechanism of action of 5–15 µM inulavosin isolated from D. nervosa (Compositae) in inhibiting melanogenesis, reducing the melanin content without affecting the enzymatic activities or the transcription of tyrosinase or Tyrp1 in B16 melanoma cells (Hideaki et al., 2009). Wen et al. evaluated the anti-complementary effects of blossoms of D. nervosa extracts based on the classical pathway. Their results showed that chlorogenic acid, 3,5-dicaffeoylquinic acid, 1,5-dicaffeoylquinic acid, and thymol were the major anti-complementary metabolites in the blossoms of D. nervosa (Wen et al., 2019).
5 Conclusion and future perspectives
The genus Duhaldea DC. has been used in traditional medicine to treat fractures, bone wounds, carbuncle and poison, bronchitis, bruises, giddy with hypertension of qi, and lung deficiency cough. Five species of the genus Duhaldea DC. have been reported to have various applications in traditional systems of medicine in several Asian countries. However, ethnobotanical data are sometimes hard to find, and it is difficult to access sources. Therefore, although the authors have made great efforts to cover the available literature as rigorously as possible, there may be possibilities that some publications, reports, or books on traditional medicinal uses of the genus Duhaldea DC. escaped our exploration. Available data indicate that over 352 metabolites have been identified from this genus, including terpenoids, flavonoids, phenylpropanoids, inositol angelates, chalcones, phenolics, and ceramide metabolites. The genus Duhaldea DC. is associated with various pharmacological activities, including anti-inflammatory, antimicrobial, anti-osteoporotic, anticancer, and antioxidant activities. Despite the fruitful phytochemical and pharmacological studies on genus Duhaldea DC., there are still several key issues to be resolved regarding the need for further development of genus Duhaldea DC.
At present, the genus Duhaldea DC. comprises 15 species widespread in Central, East, and Southeast Asia. However, only three (D. wissmannian, D. nervosa, and D. cappa) of the plants in the genus have been more extensively studied. In the future, more attention needs to be paid to underexplored plants in the study of this genus; these underexplored plant species could be promising candidates for further research.
Regrettably, aside from the systematic characterization and separation of plant metabolites conducted by Wang and Cheng et al. on D. wissmannian (Cheng, 2012; Wang, 2013), most other phytochemical investigations related to this species remain relatively fragmented. Phytochemistry seeks to identify valuable new plant metabolites. There exist considerable gaps in the study of the chemistry within this genus, making the systematic characterization and separation of its metabolites an urgent priority. Simultaneously, plant metabolites encompass not only various small-molecule metabolites but also macromolecular metabolites such as polysaccharides and peptides, which exhibit remarkable pharmacological activities. However, it is disheartening to note that among the extensive studies conducted on this genus, only Wang et al. realized this aspect (Wang et al., 2023).
Although advancements have been achieved in understanding the chemical and pharmacological characteristics of the genus Duhaldea DC., several concerns remain. Notably, the majority of pharmacological investigations on plant metabolites have been conducted exclusively in vitro, leading to a low confidence level regarding the validity of such simplistic in vitro studies demonstrating activity. Likewise, certain pharmacological assessments of extracts from this genus have only confirmed their efficacy in vitro. The current study mainly focused on the pharmacological activity profile of the extracts and isolated metabolites of the genus Duhaldea DC, while the mechanism of action was less studied, and targets and channels of action of the active metabolites corresponding to diseases were not scientifically elucidated. Modern scholars can consider using network pharmacology, data mining, and virtual screening to predict the targets, receptors, and pathways of their chemical metabolites related to pharmacological activities. At the same time, the molecular mechanisms and relationships between the active metabolites of the genus and the potential pharmacological activities are validated through high-quality and well-designed in vivo and in vitro and clinical studies.
In terms of pharmacological effects, most of them have focused on the anti-inflammatory, antitumor, antioxidant, and other effects, but at present, there are few modern pharmacological studies of the traditional effects of FI in the treatment of diseases. These ignore the modern pharmacological interpretation of traditional applications. In the future, pharmacologic studies of the genus Duhaldea DC can focus on indications in its traditional applications and should also provide a modern pharmacological explanation for the traditional application of the genus Duhaldea DC. At the same time, it should be noted that the plants of the genus Duhaldea DC are used as both medicine and food, and the pharmacological effects of the application in food and the effect on the inhibition of some disease-causing factors in food, as well as the prevention and control of some diseases.
Finally, Duhaldea DC species possess various biological activities, which can be applied to clinical medicine with further research. In addition, with the advancements observed recently in analytical techniques and quality control methods, among which the improvement and update in chromatography techniques and molecular identification methods, it is inevitable that new quality markers and quality control measures may be adopted for the better quality assessment of traditional botanical drug medicine in the future.
Plants of the genus Duhaldea DC as traditional plant medicines have made outstanding contributions in treating diseases and maintaining physical health. In this study, the ethnopharmacology, phytochemistry, and pharmacological effects of the genus Duhaldea DC were systematically elaborated to obtain a comprehensive understanding of the genus Duhaldea DC and reveal the shortcomings of the current research studies. In response to these shortcomings, this study provides guidance for future research on the genus Duhaldea DC, providing an effective scientific basis for expanding the pharmacological effect of Duhaldea DC, explaining the traditional application of Duhaldea DC, developing new drugs rationally, ensuring drug safety, controlling drug quality, and adapting Duhaldea DC to clinical application.
Author contributions
LS: conceptualization, data curation, formal analysis, investigation, methodology, resources, software, validation, and writing–original draft. KL: conceptualization, visualization, and writing–original draft. SY: formal analysis, investigation, and writing–original draft. MH: investigation, resources, and writing–original draft. XM: conceptualization, software, and writing–original draft. BY: software and writing–original draft. LG: data curation, project administration, and writing–review and editing. SX: data curation, funding acquisition, project administration, supervision, and writing–review and editing.
Funding
The author(s) declare that financial support was received for the research, authorship, and/or publication of this article. This study was supported by the Science and Technology Innovation Program of Hunan Province (No. 2022RC1228).
Conflict of interest
The authors declare that the research was conducted in the absence of any commercial or financial relationships that could be construed as a potential conflict of interest.
Publisher’s note
All claims expressed in this article are solely those of the authors and do not necessarily represent those of their affiliated organizations, or those of the publisher, the editors, and the reviewers. Any product that may be evaluated in this article, or claim that may be made by its manufacturer, is not guaranteed or endorsed by the publisher.
Supplementary material
The Supplementary Material for this article can be found online at: https://www.frontiersin.org/articles/10.3389/fphar.2024.1479963/full#supplementary-material
References
Aizaizi, A., Zhu, W. C., and Patihan, K. (2018). Effect and mechanism of aqueous Inula Cappa aqueous extract on serum inflammatory factors in rats with severe pneumonia. J. Clin. Exp. Med. 17 (16), 1708–1711. doi:10.3969/j.issn.1671-4695.2018.16.008
Badshah, S. L., Faisal, S., Muhammad, A., Poulson, B. G., Emwas, A. H., and Jaremko, M. (2021). Antiviral activities of flavonoids. Biomed. Pharmacother. 140, 111596. doi:10.1016/j.biopha.2021.111596
Baruah, R. N., Sharma, R. P., Baruah, J. N., Mondeshka, D., Hertz, W., and Watanabe, K. (1982). Ineupatoriol, a thiophene analogue of ichthyothereol, from Inula eupatorioides. Phytochemistry 21 (3), 665–667. doi:10.1016/0031-9422(82)83161-0
Baruah, R. N., Sharma, R. P., Thyagarajan, G., Herz, W., Govindan, S. V., and Blount, J. F. (1980). Unusual germacranolides from Inula eupatorioides. J. Org. Chem. 45 (24), 4838–4843. doi:10.1021/jo01312a007
Chang, Y., Fei, S., Junqi, H., Yonglin, W., Yongjun, L., Yanyu, L., et al. (2020). Effect of active constituents from inula cappa on the protein expression of CYP3A4 in HepG2 cells with high PXR expression. Guizhou Yike Daxue Xuebao 45 (10), 1137–1142. doi:10.19367/j.cnki.2096-8388.2020.10.004
Cheng, X. (2012). Studies on bioactive secondary metabolites from four plants of inula genus. Doctor: Shanghai Jiao Tong University.
Cheng, X., Ma, J., Amadou, I., Zhao, W., Chen, Y., Zhang, C., et al. (2023). Electrophilic components from Xiaoheiyao (rhizomes of Inula nervosa Wall.) alleviate the production of heterocyclic aromatic amines via creatinine inhibition. Food Chem. 404, 134561. doi:10.1016/j.foodchem.2022.134561
Cheng, X., Shao, W., Zhang, S., Wang, G., Shan, L., Shen, Y., et al. (2014a). Winolides A–C, bioactive sesquiterpene lactones with unusual 5, 6-secoeudesmane frameworks from Inula wissmanniana. RSC Adv. 4 (64), 33815–33818. doi:10.1039/c4ra05131a
Cheng, X., Shoude, Z., Chunhui, W., Jie, R., Jiangjiang, Q., Xue, T., et al. (2013). Bioactive eudesmane and germacrane derivatives from Inula wissmanniana Hand.-Mazz. Phytochemistry 96, 214–222. doi:10.1016/j.phytochem.2013.10.006
Cheng, X., Tu, P., Dong, W., Yu, B., Xia, S., Muskat, M. N., et al. (2022). Electrophilic thymol isobutyrate from Inula nervosa Wall.(Xiaoheiyao) ameliorates steatosis in HepG2 cells via Nrf2 activation. J. Funct. Foods 88, 104895. doi:10.1016/j.jff.2021.104895
Cheng, X., Wang, C., Wei, P., Zhang, X., Zeng, Q., Yan, S., et al. (2014b). New sesquiterpenic acids from Inula wissmanniana. Fitoterapia 95, 139–146. doi:10.1016/j.fitote.2014.03.013
Cheng, X., Zhao, W., Dong, W., and Le, G. (2020). Chemical space charting of different parts of inula nervosa wall.: upregulation of expression of Nrf2 and correlated antioxidants enzymes. Molecules 25 (20), 4789. doi:10.3390/molecules25204789
Chunli, H., Lehao, w., Yang, Y., Huizi, J., and Yan, Z. (2021). The anti-inflammatory activity and mechanisms of Inula pterocaula extract in LPS-induced inflammatory responses in RAW 264.7 macrophages. Dalian Gongye Daxue Xuebao 40 (04), 253–259. doi:10.19670/j.cnki.dlgydxxb.2021.0404
Dong, W., Yuge, J., Shufang, X., Congcong, C., Wei, Z., and Xiang-rong, C. (2020). Study on intervention effects of electrophilic components from Xiaoheiyao against free fatty acid-induced steatosis in HepG2 cells. Packag. Food Mach. 36 (3), 24–33. doi:10.13652/j.issn.1003-5788.2020.03.005
Dorina, G., E, J. A., Szilárd, V., Alex, V., Zoltán, S., Julia, S., et al. (2023). Inositol is an effective and safe treatment in polycystic ovary syndrome: a systematic review and meta-analysis of randomized controlled trials. Reprod. Biol. Endocrin 21 (1), 10. doi:10.1186/s12958-023-01055-z
Fardoun, M. M., Maaliki, D., Halabi, N., Iratni, R., Bitto, A., Baydoun, E., et al. (2020). Flavonoids in adipose tissue inflammation and atherosclerosis: one arrow, two targets. Clin. Sci. 134 (12), 1403–1432. doi:10.1042/CS20200356
Gill, B. S., Kumar, S., and Navgeet, M. (2016). Triterpenes in cancer: significance and their influence. Mol. Biol. Rep. 43, 881–896. doi:10.1007/s11033-016-4032-9
Gong, Z., Li, M., Xiong, D., Wu, L., Liu, T., Lan, Y., et al. (2018). Evaluation of anti-inflammatory activity of inula cappa extract based on inflammation model in vitro and in vivo. Nat. Prod. Res. 29 (12), 2050. doi:10.16333/j.1001-6880.2017.12.009
Goswami, A. C., Baruah, R. N., Sharma, R. P., Baruah, J. N., Kulanthaivel, P., and Herz, W. (1984). Germacranolides from inula cappa. Phytochemistry 23 (2), 367–372. doi:10.1016/S0031-9422(00)80334-9
Guo, Q., Yang, J., and Liu, J. (2007). Studies on the chemical constituents from Inula cappa (II). J. Chin. Med. Mat. 30 (1), 35–37. doi:10.13863/j.issn1001-4454.2007.01.015
He, A., She, C., Zeng, J., and Peng, S. (2016). Comparison study on in vitro and in vivo antioxidant activities of Inula nervosa Wall. extracts from different parts. Chin. Pharmacol. Bull., 79–83. doi:10.3969/j.issn.1001-1978.2016.01.017
He, Y., Wu, Q., Huang, L., Su, Y., Yao, X., Wu, D., et al. (2019). Protective effect of InuIa cappa (buch.-ham) DC. Water extract on CCl4 and D-GalN induced acute liver injury in mice. Sci. Technol. Eng. 19 (23), 42–46. doi:10.3969/j.issn.1671-1815.2019.23.007
Hideaki, F., Tomonori, M., Takayuki, K., Sadaki, Y., Akitsugu, Y., Masaru, H., et al. (2009). Inulavosin, a melanogenesis inhibitor, leads to mistargeting of tyrosinase to lysosomes and accelerates its degradation. J. Invest. Dermatol 129 (6), 1489–1499. doi:10.1038/jid.2008.376
Huang, C., Changsheng, D., Yanan, Z., Yang, Y., Huizi, J., and Yan, Z. (2021a). Duhaldea pterocaula (franch.) anderb. Attenuates nociception and inflammation via GABAA receptors. Front. Pharmacol. 12, 753128. doi:10.3389/fphar.2021.753128
Huang, C., Dong, C., Zhu, Y., Yu, Y., Jin, H., and Zhang, Y. (2021b). Duhaldea pterocaula (franch.) anderb. Attenuates nociception and inflammation via GABAA receptors. Front. Pharmacol. 12, 753128. doi:10.3389/fphar.2021.753128
Jyoti, K., Rahul, S., Arti, P., K, L. S., and B, S. M. (2017). Anti-inflammatory and immunomodulatory activities of Inula cappa roots (Compositae). J. Complement. Integr. Med. 14 (3), 20160083. doi:10.1515/jcim-2016-0083
Kalola, J., and Shah, M. (2006). Actividad de barrido de los radicales libres de Inula cappa. Ars Pharm. 47 (4), 385–401. doi:10.30827/ars
Kiani, A., Paolacci, S., Calogero, A., Cannarella, R., Di Renzo, G., Gerli, S., et al. (2021). From Myo-inositol to D-chiro-inositol molecular pathways. Eur. Rev. Med. Pharm. 25 (5), 2390–2402. doi:10.26355/eurrev_202103_25279
Kiran, K. A., Kumar, S., and Sharma, P. K. (2017). Hepatoprotective activity of Inula cappa DC. Aqueous extract against carbon tetrachloride induced hepatotoxicity in Wistar rats. Int. Res. J. Pharm. 8, 14–19. doi:10.7897/2230-8407.08013
Li, F. (2017). Anti-inflammatory effect of inula cappa aqueous extract on Klebsiella pneumoniae-induced severe pneumonia in rats. World Chin. Med. 12 (10), 2438–2442. doi:10.3969/j.issn.1673-7202.2017.10.041
Li, W., Yang, Y., Wu, J., Jiang, S., Yang, Y., Guo, T., et al. (2023). A new labdane diterpenoid glycoside and other constituents from Inula nervosa (Asteraceae) and their chemotaxonomic importance. Biochem. Syst. Ecol. 109, 104662. doi:10.1016/j.bse.2023.104662
Li, Y., Li, T., Chen, Z., Wang, R., Li, B., Dong, F., et al. (2020). Study on antibacterial activity of extracts from roots of inula cappa. Chin. Arch. Tradit. Chin. Med. 38 (01), 24–27. doi:10.13193/j.issn.1673-7717.2020.01.006
Liby, K. T., Yore, M. M., and Sporn, M. B. (2007). Triterpenoids and rexinoids as multifunctional agents for the prevention and treatment of cancer. Nat. Rev. Cancer 7 (5), 357–369. doi:10.1038/nrc2129
Liu, P., Yang, G., Tian, X., Zhang, Y., Li, F., and Wang, F. (2011). Antioxidating effects of Inula nervosa Wall. ex DC. on the edible oils and its antimicrobial activity. Sci. Technol. Food Ind. 32 (10), 187–189. doi:10.13386/j.issn1002-0306.2011.10.026
Liu, S., Chen, J. J., Li, J., Chen, F., and Deng, L. (2010). Antmi icrobial effects of inula cappa O rganic SolventExtrac ts. Liaoning J. Tradit. Chin. Med. 37 (03), 398–400. doi:10.13192/j.ljtcm.2010.03.19.liushg.087
Liu, S., Hu, X., Liu, X., and Deng, L. (2009a). Chemical campositin of the essental oil fram inula cappa and their effects on free radicals scavenging. J. Anhui Agric. Univ. 37 (26), 12536–12537+12666. doi:10.13989/j.cnki.0517-6611.2009.26.148
Liu, S., Li, J., Chen, F., Liu, X., and Deng, L. (2009b). Studies on the antinicrobial activities of aqueous fram inula cappa DC in vitro. Lishizhen Med. Mat. Med. Res. 20 (12), 3072–3074. doi:10.3969/j.issn.1008-0805.2009.12-3072-02
Liu, W., Ge, T., Liu, S., and Liu, X. (2010). Extraction of flavonoids from inula cappa and its antibacterial activity. Hubei. Agric. Sci. 49 (02), 426–429. doi:10.14088/j.cnki.issn0439-8114.2010.02.072
Long, S. (2004). Clinical experience of “maoshoucai” for treating traumatic injury. JMPCM 12, 231–232.
Ma, J., Li, K., Shi, S., Li, J., Tang, S., and Liu, L. (2022). The application of UHPLC-HRMS for quality control of traditional Chinese medicine. Front. Pharmacol. 13, 922488. doi:10.3389/fphar.2022.922488
Marine, L. C., and Christophe, O. S. (2013). Potential role and therapeutic interests of myo-inositol in metabolic diseases. Biochimie 95 (10), 1811–1827. doi:10.1016/j.biochi.2013.05.011
Mei, D., Takaki, H., Bin, C., Qingwen, S., Siming, W., Kazuko, K., et al. (2015). Protein kinase Cα-mediated cytotoxic activity of ineupatorolide B from Inula cappa DC. in HeLa cells. Int. J. Oncol. 47 (5), 1839–1844. doi:10.3892/ijo.2015.3172
Miranda, R. d.S., de Jesus, B. d.S. M., da Silva Luiz, S. R., Viana, C. B., Adao Malafaia, C. R., Figueiredo, F. d.S., et al. (2022). Antiinflammatory activity of natural triterpenes—an overview from 2006 to 2021. Phytother. Res. 36 (4), 1459–1506. doi:10.1002/ptr.7359
Mo, J., Xu, M., Yang, D., and Huang, Z. (2012). Experimental study on anti-inflammatory and analgesic effects of ethanol extracts of Dong-medicine Inula cappa. Chin. J. Exp. Tradit. Med. Form. 18, 258–260. doi:10.13422/j.cnki.syfjx.2012.21.021
Neelam, E., Khatkar, A., and Sharma, K. K. (2020). Phenylpropanoids and its derivatives: biological activities and its role in food, pharmaceutical and cosmetic industries. Crit. Rev. Food Sci. Nutr. 60 (16), 2655–2675. doi:10.1080/10408398.2019.1653822
Neilsen, E., William, K., and David, A. (2014). Steroids: pharmacology, complications, and practice delivery issues. Ochsner J. 14 (2), 203–207.
Nikomtat, J., Meepowpan, P., and Tragoolpua, Y. (2011). Inhibition of Inula cappa (Ham. ex D. Don) DC. extracts on herpes simplex virus infection in vitro. Afr. J. Microbiol. 5 (24), 4049–4058. doi:10.5897/AJMR10.387
Peng, G., Xiong, D., Li, M., Wu, L., Chen, T., Li, Y., et al. (2017). Analysis of chemical constituents of effective components of Inula cappa. J. Anhui. Agri. Sci. 45 (29), 131–133. doi:10.13989/j.cnki.0517-6611.2017.29.041
Peng, J., Xie, J., Shi, S., Luo, L., Li, K., Xiong, P., et al. (2021). Diagnostic fragment-ion-based for rapid identification of chlorogenic acids derivatives in Inula cappa Using UHPLC-Q-Exactive orbitrap mass spectrometry. J. Anal. Methods Chem. 2021, 6393246. doi:10.1155/2021/6393246
Priydarshi, R., Melkani, A. B., Mohan, L., and Pant, C. C. (2016). Terpenoid composition and antibacterial activity of the essential oil from Inula cappa (Buch-Ham. ex. D. Don) DC. J. Essent. 28 (2), 172–176. doi:10.1080/10412905.2015.1090935
Schulz, V., Hänsel, R., and Tyler, V. E. (2001). Rational phytotherapy: a physician's guide to herbal medicine. Psychology Press, 1–39.
Serafini, M., Peluso, I., and Raguzzini, A. (2010). Flavonoids as anti-inflammatory agents. Proc. Nutr. Soc. 69 (3), 273–278. doi:10.1017/S002966511000162X
Shen, N., Wang, T., Gan, Q., Liu, S., Wang, L., and Jin, B. (2022). Plant flavonoids: classification, distribution, biosynthesis, and antioxidant activity. Food Chem. 383, 132531. doi:10.1016/j.foodchem.2022.132531
Tai, Z., Chen, C., Qin, B., Cai, L., and Xu, Y. (2014). Studies on triterpenoid saponins from inula pterocaula. J. Kunming Univ. Sci. Technol. Nat. Sci. 39 (05), 70–75. doi:10.3969/j.issn.1007-855x.2014.05.014
Tao, Y., Lei, H., Guoqiang, F., Peipei, S., and Pinhua, L. (2015). Antioxidant activity of total flavonoids from sanicula astrantiifolia wolff. Mod. Agric. Sci. Technol. (17), 311–313+316. doi:10.3969/j.issn.1007-5739.2015.17-0311-03
Tao, Y., Tingting, L., Fen, Y., Fang, W., and Pinhua, L. (2016). Polysaccharides contents and antioxidant activity of Sanicula astrantiifolia Wolff. Sci. Technol. Cereal. Oil. Food 24 (03), 55–58. doi:10.16210/j.cnki.1007-7561.2016.03.012
Tvrzicka, E., Kremmyda, L.-S., Stankova, B., and Zak, A. (2011). Fatty acids as biocompounds: their role in human metabolism, health and disease-a review. part 1: classification, dietary sources and biological functions. Biomed. Pap. Med. Fac. Palacky. Univ. Olomouc 155 (2), 117–130. doi:10.5507/bp.2011.038
Wang, A., Li, M., Sun, J., Xiong, F., Chen, T. T., Wu, L., et al. (2018). Study on the antibacterial and anti-inflammatory activities of extracts from inula cappa (DC.). Shizhen Guo Yi Guo Yao 29 (07), 1580–1584. doi:10.3969/j.issn.1008-0805.2018.07.014
Wang, C., Zhang, X., Wei, P., Cheng, X., Ren, J., Yan, S., et al. (2013). Chemical constituents from Inula wissmanniana and their anti-inflammatory activities. Arch. Pharmacal Res. 36, 1516–1524. doi:10.1007/s12272-013-0143-1
Wang, C. H. (2013). Studies on the constituents from inula wissmanniana and quallty control of inula cappa. master. Shanghai Jiao Tong University.
Wang, F., Li, X., Sun, Q., Yao, S., Ke, C., Tang, C., et al. (2012). Sesquiterpene lactones from Inula cappa. Phytochem. Lett. 5 (3), 639–642. doi:10.1016/j.phytol.2012.06.012
Wang, Z., Ma, X., Shi, S., He, S., Li, J., Wilson, G., et al. (2023). Structural characterization and anti-inflammatory activity of a novel polysaccharide from Duhaldea nervosa. Polymers 15 (9), 2081. doi:10.3390/polym15092081
Wardana, A. P., Aminah, N. S., Rosyda, M., Abdjan, M. I., Kristanti, A. N., Tun, K. N. W., et al. (2021). Potential of diterpene compounds as antivirals, a review. Heliyon 7 (8), e07777. doi:10.1016/j.heliyon.2021.e07777
Wei, C., Kailin, L., Pei, X., Kaiyan, G., Lian, Z., Jianbo, Y., et al. (2020). A systematic strategy for rapid identification of chlorogenic acids derivatives in Duhaldea nervosa using UHPLC-Q-Exactive Orbitrap mass spectrometry. Arab. J. Chem. 13 (2), 3751–3761. doi:10.1016/j.arabjc.2020.01.007
Wei, L., Zhiqi, W., Dong, W., Yulin, H., Mengchu, L., Jianping, G., et al. (2022). Analysis of chemical constituents and study on anti-complement activity in the inflorescences of inula nervosa. Chin. Tradit. Pat. Med. 44 (07), 2401–2405. doi:10.3969/j.issn.1001-1528.2022.07.061
Wen, Z., Huang, W., Long, W., Gong, J., and Wu, D. (2019). In vitro anticomplementary activity and quality evaluation of dried blossoms of Inula nervosa Wall. from different geographical origins. Biomed. Chromatogr. 33 (12), e4682. doi:10.1002/bmc.4682
Wu, J., Tang, C., Cai, Y., Ke, C., Lin, L., Yao, S., et al. (2017). Cytotoxic germacrane-type sesquiterpene lactones from the whole plant of Inula cappa. Chin. Chem. Lett. 28 (5), 927–930. doi:10.1016/j.cclet.2016.11.011
Wu, J., Tang, C., Yao, S., Zhang, L., Ke, C., Feng, L., et al. (2015). Anti-inflammatory inositol derivatives from the whole plant of Inula cappa. J. Nat. Prod. 78 (10), 2332–2338. doi:10.1021/acs.jnatprod.5b00135
Wu, Q., Shi, Y., and Jia, Z. (2006). Eudesmane sesquiterpenoids from the Asteraceae family. Nat. Prod. Rep. 23 (5), 699–734. doi:10.1039/B606168K
Xie, H., Chen, H., Cao, B., Zhang, H., and Zou, Z. (2007). Cytotoxic germacranolide sesquiterpene from Inula cappa. Chem. Pharm. Bull. 55 (8), 1258–1260. doi:10.1248/cpb.55.1258
Xie, H., Wang, J., Chen, Z., Yuan, G., Li, Q., and Qin, L. (2012). Antimicrobial compound isolated from inula cappa and its inhibitory characteristics. Nat. Prod. Res. Dev. 24 (11), 1534–1537. doi:10.16333/j.1001-6880.2012.11.021
Yan, L., Cheng, X., Zeng, Q., Qin, J., Zhang, W., and Jin, H. (2011). Phytane and neoclerodane diterpenes from the aerial parts of Inula nervosa Wall. Biochem. Syst. Ecol. 39 (4-6), 700–703. doi:10.1016/j.bse.2011.06.001
Yan, L., Huang, Y., Fu, J., Qin, J., Zeng, Q., Zhu, Y., et al. (2010). Three new phenylpropanoids from inula nervosa wall. Helv. Chim. Acta 93 (7), 1418–1421. doi:10.1002/hlca.200900432
Yoshida, T., Mori, K., and Guangxin, H. (1995). Inulavosin, a new thymol dimer with piscicidal activity from Inula nervosa. Heterocycles 9 (41), 1923–1926. doi:10.1002/CHIN.199552231
Yuan, Z., Hongyan, Z., Ling, X., and Mengli, L. (2021). Scavenging and disconnecting effects on nitrosation reaction by extracts of stem and leaf of inula nervosa wall. Southwest China J. Agric. Sci. 34 (10), 2145–2150. doi:10.16213/j.cnki.scjas.2021.10.012
Zheng, L., Hao, X., Yuan, C., Huang, L., Zhang, J., Dong, F., et al. (2015). Study on chemical constituents of Inula cappa. China J. Chin. Mater Med. 40 (4), 672–678. doi:10.4268/cjcmm20150419
Zhou, W. (2017). Chemical constituents of Inula cappa. J. Chin. Pharm. Sci., 25–30. doi:10.11669/cpj.2017.01.005
Zhu, S., Chunli, H., Yongzhen, X., Yangguo, X., Guojing, W., Weidong, Z., et al. (2019). Pterocaullins A− D, four sesquiterpene lactones from Inula pterocaula. Phytochem. Lett. 33, 70–76. doi:10.1016/j.phytol.2019.07.010
Keywords: Duhaldea DC., ethnobotany, pharmacology, phytochemistry, traditional uses
Citation: Shu L, Li K, Yang S, Hu M, Ming X, Yan B, Guan L and Xiao S (2024) A review on ethnobotany, phytochemistry, and pharmacology of the genus Duhaldea DC. Front. Pharmacol. 15:1479963. doi: 10.3389/fphar.2024.1479963
Received: 13 August 2024; Accepted: 31 October 2024;
Published: 15 November 2024.
Edited by:
Zhichao Xu, Northeast Forestry University, ChinaReviewed by:
Le-Le Zhang, Chengdu University, ChinaZhi Chai, Shanxi University of Chinese medicine, China
Copyright © 2024 Shu, Li, Yang, Hu, Ming, Yan, Guan and Xiao. This is an open-access article distributed under the terms of the Creative Commons Attribution License (CC BY). The use, distribution or reproduction in other forums is permitted, provided the original author(s) and the copyright owner(s) are credited and that the original publication in this journal is cited, in accordance with accepted academic practice. No use, distribution or reproduction is permitted which does not comply with these terms.
*Correspondence: Liangjun Guan, Z3VhbmxqM0AxNjMuY29t; Shunli Xiao, eGlhb3NsM0AxNjMuY29t