- Department of Pharmacy, Shanghai Chest Hospital, School of Medicine, Shanghai Jiao Tong University, Shanghai, China
Crush syndrome often occurs after severe crush injury caused by disasters or accidents, and is associated with high mortality and poor prognosis. Cardiovascular complications, such as cardiac arrest, hypovolemic shock, and hyperkalemia-related cardiac dysfunction, are the primary causes of on-site death in crush syndrome. Prehospital evaluation, together with timely and correct treatment, is of great benefit to crush syndrome patients, which is difficult in most cases due to limited conditions. Based on current data and studies, early fluid resuscitation remains the most important on-site treatment for crush syndrome. Novel solutions and drugs used in fluid resuscitation have been investigated for their effectiveness and benefits. Several drugs have proven effective for the prevention or treatment of cardiovascular complications in crush syndrome, such as hypovolemic shock, hyperkalemia-induced cardiac complications, myocardial ischemia/reperfusion injury, ventricular dysfunction, and coagulation disorder experimentally. Moreover, these drugs are beneficial for other complications of crush syndrome, such as renal dysfunction. In this review, we will summarize the existing on-site treatments for crush syndrome and discuss the potential pharmacological interventions for cardiovascular complications to provide clues for clinical therapy of crush syndrome.
Introduction
Crush syndrome is usually caused by severe natural or human-made disasters, such as earthquakes, landslides, terrorist attacks, and air or railway crashes, and is characterized by systemic manifestations of traumatic muscle injury and subsequent multi-organ dysfunction or failure (Long et al., 2023; Usuda et al., 2023). Data based on current disaster investigations indicate that almost half of the survivors have been subjected to severe crush injuries, among which as high as 40%–70% suffered from crush syndrome (Long et al., 2023; Sever et al., 2012; Sever and Vanholder, 2013; Gibney et al., 2014). Crush syndrome can be complicated with hypovolemic shock, hyperkalemia, cardiac arrest, acute kidney injury, systemic inflammation, and sepsis, resulting in high mortality up to 48% (Sahjian and Frakes, 2007; Long et al., 2023; Usuda et al., 2023; Genthon and Wilcox, 2014; Metzger et al., 2010; McCullough et al., 2014; Sever et al., 2003). It is often difficult to accurately diagnose crush syndrome and initiate appropriate treatment in on-site situations (Usuda et al., 2023). As reported, approximately 20% of crush victims die of cardiac arrest induced by hyperkalemia or hypovolemic shock within a short time after decompression (Ashkenazi et al., 2005). A previous study also indicated that the survival rate of traumatic cardiac arrest patients with crush injuries was only 10.6% (Yang et al., 2022). Therefore, new therapeutic methods and effective drugs, especially those targeting cardiovascular symptoms in crush syndrome, are urgently needed. Recently, there have been some advances in the treatment and pathological mechanisms of crush syndrome, including novel therapeutic drug for crush-induced hyperkalemia and the role of ferroptosis in crush syndrome-related acute kidney injury (Li et al., 2024; Qiao et al., 2023). In this review, we make efforts to comprehensively summarize and illuminate current on-site therapies and pharmacological investigations of cardiovascular complications in crush syndrome. We also indicate the limitations and advantages of these potential drugs. For example, only a few drugs could be beneficial for multiple symptoms related to crush syndrome, whereas some drugs could treat hyperkalemia without the risk of hypokalemia in crush syndrome. Our findings provide insights for the future clinical treatment of crush syndrome.
On-site therapeutic strategies
Routine on-site treatments for crush syndrome
Immediate and proper on-site treatments are crucial for victims exposed to severe crush injuries to prevent potential crush syndrome or reduce the mortality of crush syndrome that already existed. As reported, even simple on-site therapies could reduce 13%–40% of early deaths (Long et al., 2023). With the exception of extrication as soon as possible, other effective treatments at the scene of the incident include right triage, airway support management with portable ventilators (especially for those chest crush victims), hemorrhage control with tourniquet, fracture fixation, fluid resuscitation, hypothermia prevention, pain control, and efficient transport (Zhang et al., 2014; Long et al., 2023; Sever et al., 2012; Ashkenazi et al., 2005). On-site care providers should especially notice the occurrence of hyperkalemia and inducible cardiac dysrhythmias by monitoring serum potassium levels and electrocardiographic parameters as early as possible and throughout the entire prehospital care period of crush injury patients to prevent sudden cardiac arrest or heart failure (Usuda et al., 2023; Genthon and Wilcox, 2014; Gonzalez, 2005; Burns et al., 2010).
Severe crush injury with serious muscular damage and large amounts of bleeding often leads to crush syndrome with multiple complications, such as traumatic rhabdomyolysis, acute compartment syndrome, acute kidney injury, and many cardiovascular-related symptoms, including cardiac or hypovolemic shock, metabolic acidosis, hyperkalemia, and disseminated intravascular coagulation (Sahjian and Frakes, 2007; Long et al., 2023; Usuda et al., 2023; Abu-Zidan et al., 2023). Hemorrhage-induced volume loss and fast transfer of extracellular fluids into injured tissues lead to hypovolemia during severe crush injury. Hypoperfusion of organs induces toxicity aggregation, resulting in severe complications of multiple systems in crush syndrome. For example, the release of myoglobin and toxic metabolites from damaged tissues along with renal hypoperfusion may facilitate renal tubular cast formation and finally cause acute renal failure. Early aggressive fluid resuscitation has been confirmed as the most essential on-site treatment to restore circulating blood volume and reduce complications from crush syndrome or even prevent rapid death after decompression (Sahjian and Frakes, 2007; Long et al., 2023; Usuda et al., 2023; Li et al., 2009). Sufficient fluid resuscitation could correct hypotension and acidosis, alleviate hypovolemia and hyperkalemia, increase urine flow to excrete toxins, relieve limb swelling, restore end-organ perfusion, and prevent heart or renal failure (Sahjian and Frakes, 2007; Long et al., 2023; Li et al., 2009).
On-site fluid resuscitation in crush syndrome
Initiation of fluid resuscitation should be as early as possible even before extrication, best within 6 h of crush injury to reduce the risk of hypovolemic shock and acute kidney injury, and throughout the rescue process (Long et al., 2023; Usuda et al., 2023; Sever et al., 2012; Better and Stein, 1990; Better, 1990). The vascular access choice and volume of fluid resuscitation depend on victim’s status and environmental conditions, such as age, comorbidities, injury pattern, and crush duration (Abu-Zidan et al., 2023). Intravenous access is preferred at an earliest rate of 1–1.5 L/h for adults and 10–20 mL/kg/h for children if the extremity vein can be found, and the volume can be up to 6–12 L/d (Long et al., 2023; Genthon and Wilcox, 2014; Ashkenazi et al., 2005; Gonzalez, 2005; Sever et al., 2012). If not available, intraosseous or subcutaneous access is recommended for fluid resuscitation (Sahjian and Frakes, 2007; Sever et al., 2012; Sever and Vanholder, 2013; Gibney et al., 2014; Abu-Zidan et al., 2023). A urinary catheter could be needed to track urine output and a target urine output of more than 300 mL/h helps to prevent crush-induced acute kidney injury (Malinoski et al., 2004; Long et al., 2023; Scharman and Troutman, 2013; Sever et al., 2006).
Multiple fluids or drugs can be useful in fluid resuscitation processes, such as isotonic sodium chloride solution, 5% glucose, blood or artificial plasma, sodium bicarbonate, 20% mannitol, human serum albumin, 5% dextran, and diuretics, when circulation is steady (Sahjian and Frakes, 2007; Long et al., 2023; Genthon and Wilcox, 2014; Li et al., 2009). Potassium-containing solutions such as Ringer’s lactate should be avoided for crush patients during fluid resuscitation in cases of hyperkalemia which could cause cardiac dysrhythmias (Sever and Vanholder, 2013). Doctors should properly make decisions about the single, combined, or sequential utilization of these fluids according to the patient’s situation. A variety of studies have proven that the first-line recommendation of fluid resuscitation for crushed patients is isotonic saline, which is most effective for volume replacement, to correct hypotension or tachycardia, prevent hypovolemic shock, reduce acute kidney injury and the use of renal replacement therapy, and avoid death (Long et al., 2023; Walters et al., 2016; Sahjian and Frakes, 2007; Gunal et al., 2004; Genthon and Wilcox, 2014). In some cases, blood products can be used to treat hypotension or coagulopathy by transfusing plasma or platelets to supplement essential blood components in time (Sahjian and Frakes, 2007; Li et al., 2009; Sever et al., 2012; Walters et al., 2016).
It is also recommended that sodium bicarbonate be added to fluids to reduce metabolic acidosis, treat hyperkalemia, and alkalinize the urine (Sahjian and Frakes, 2007; Better, 1999; Long et al., 2023; Usuda et al., 2023). In crush syndrome, severe acidosis damages cardiac function by decreasing myocardial contractility and cardiac output and causing arrhythmias or hypotension (Sabatini and Kurtzman, 2009; Kraut and Kurtz, 2006; Kraut and Madias, 2010). Breakdown of myoglobin from injured muscles may cause tubular epithelium slough and cast formation in the kidney, obstruction of renal blood flow, and finally, renal failure. Alkalinization of the blood and urine by sodium bicarbonate could reduce the harmful effects on the cardiovascular system and myoglobin breakdown by promoting the precipitation of myoglobin (Sahjian and Frakes, 2007; Criddle, 2003; Long et al., 2023; Genthon and Wilcox, 2014). The therapeutic goal of urine alkalinization is to achieve a pH of 6–7 (Sahjian and Frakes, 2007; Genthon and Wilcox, 2014). Sodium bicarbonate also has a temporary effect on treating hyperkalemia, although this impact has been doubtful in the absence of acidemia (Long et al., 2018; Weisberg, 2008; Allon and Shanklin, 1996).
A 20% mannitol solution is recommended after adequate resuscitation and urine output (Smith and Greaves, 2003; Sever et al., 2006; Sahjian and Frakes, 2007; Gonzalez, 2005). Mannitol is mainly used to force diuresis, clear myoglobin, scavenge free radicals to avoid oxidative injury, and reduce tissue swelling or alleviate compartment pressures to avoid compartment syndrome (Sahjian and Frakes, 2007; Malinoski et al., 2004; Genthon and Wilcox, 2014). With a urine flow of more than 20 mL/h, mannitol can be added at a total dose of 1–2 g/kg/d at an infusion rate of 5 g/h (Sever et al., 2006). It is worth noting that anuric patients or those who have been volume-depleted should not use mannitol. Other contraindications for mannitol include congestive heart failure and electrolyte abnormalities (Smith and Greaves, 2003; Sever et al., 2012; Gibney et al., 2014). Monitoring electrolyte disturbances and serum osmolality when mannitol is infused is necessary, in order to avoid hypokalemia which may cause cardiac dysrhythmias or arrest and even death, and to avoid an increase in osmolality which might indicate a dehydrated situation (Sahjian and Frakes, 2007). Although mannitol and bicarbonate (even the cocktail administration of mannitol-bicarbonate, with a mix of bicarbonate, mannitol, sodium chloride, and dextrose) are believed to protect against acute renal failure in crush syndrome, reliable data from randomized controlled trials are still needed to confirm the exact effects of bicarbonate and/or mannitol for fluid resuscitation in crush syndrome (Altintepe et al., 2007; Chavez et al., 2016; Schwartz et al., 2015; Brown et al., 2004).
Pharmacological investigations of cardiovascular complications
Hypovolemic shock in crush syndrome
Hypovolemic shock is one of the most common complications in crush syndrome. Lower cardiac output, sharply decreased mean arterial blood pressure, and increased heart rate usually appear in crush syndrome patients with hypovolemic shock (Usuda et al., 2023; Emig et al., 2003; Xu et al., 2018). Hypovolemic shock in crush syndrome is mainly caused by excessive hemorrhage-induced severe circulatory volume inadequacy and tissue hypoperfusion as well as consequent microcirculation disturbances and other metabolic alterations. Myoglobin released from damaged skeletal muscle into the circulation can induce vasoconstriction in multiple organs, leading to increased vascular resistance and an obstacle for sufficient blood supply to tissues in crush syndrome-related hypovolemic shock (Emig et al., 2003). Currently, sufficient fluid resuscitation and suitable liquid selection are still the most important methods for preventing and treating hypovolemic shock in crush syndrome, and more helpful candidate drugs are being researched.
Potential resuscitation solutions have been studied for their effectiveness and benefits compared with conventional fluid resuscitation (Song et al., 2013; Katz et al., 2002; Philbin et al., 2005; Kaplan et al., 2006; Malkevich et al., 2008; Mohri et al., 2006). Researchers found that in comparison with hypertonic or normal saline, bolus infusion of hypertonic hydroxyethyl starches (HHESs) increased the survival rate of crush-injured rats 72 h after decompression and their mean arterial blood pressures, and also improved the acid-base balance and renal function, proving that HHES combined with normal saline might be a better choice for fluid resuscitation to treat hypovolemic shock in crush syndrome (Song et al., 2013). Even so, investigations on hemoglobin-based oxygen carrier (HBOC-201), a bovine hemoglobin solution that improves oxygen delivery to tissues without obvious vasoconstriction, have demonstrated that HBOC-201 could be superior to HHES in hypovolemic shock in a crush-induced hemorrhagic shock model. Compared with HHES, resuscitation with HBOC-201 resulted in significantly higher survival rates, mean arterial blood pressures, mean pulmonary artery pressures, and pulmonary capillary wedge pressures, lower heart rates and lactate levels, and better cardiac index in crush-injured models. Animals treated with HBOC-201 also had higher tissue oxygenation and strong ion differences, as well as a lower need for resuscitated fluids or blood transfusion (Katz et al., 2002; Philbin et al., 2005; Kaplan et al., 2006; York et al., 2003; McNeil et al., 2001; Sampson et al., 2003; Knudson et al., 2003; Fitzpatrick et al., 2004; Lee et al., 2002). In addition, the combined use of HBOC-201 and recombinant factor VIIa during fluid resuscitation helped reduce immune activation in pigs with crush-induced hemorrhagic shock (Malkevich et al., 2008). Administration of recombinant human soluble thrombomodulin with fluid resuscitation increased the survival rate of crush-injured rats by attenuating hemoconcentration, manifested as increased hemoglobin and hematocrit levels, mitigating hyperkalemia, decreasing serum interleukin-6 and lactate levels, and improving acid-base balance and renal function (Mohri et al., 2006). These potential solutions or additive drugs for fluid resuscitation exert better anti-shock effects by elevating mean arterial blood pressures, regulating pH or lactate levels of blood, improving cardiac function, inhibiting immune responses, reducing blood creatinine and K+ levels, and mitigating hemoconcentration in crush syndrome.
In addition to the components used for fluid resuscitation, other drugs have been tested for their anti-shock effects in crush syndrome. Administration of salvianolic acid B or dexamethasone before fluid resuscitation could improve the survival rates in crush syndrome models through its anti-shock and anti-inflammatory effects, manifested as mean arterial blood pressure elevation, hematocrit level decrease, endothelial inflammation-induced coagulation disorder alleviation, acid-base balance enhancement, hyperkalemia control, renal function improvement, decrease in serum lactate and interleukin-6 levels, and inhibition of myeloperoxidase activity (Murata et al., 2022; Murata et al., 2016; Murata et al., 2013). Our previous studies also demonstrated the effects of anisodamine on crush syndrome-related shock by increasing blood pressure in crush syndrome rats, which relied on the activation of α7 nicotinic acetylcholine receptor (α7nAChR) (Yu et al., 2019; Fan et al., 2016) (Figure 1: Anti-shock drugs). Moreover, the anti-shock effect of anisodamine was strengthened by the co-administration of neostigmine in crush syndrome animals (Xu et al., 2016).
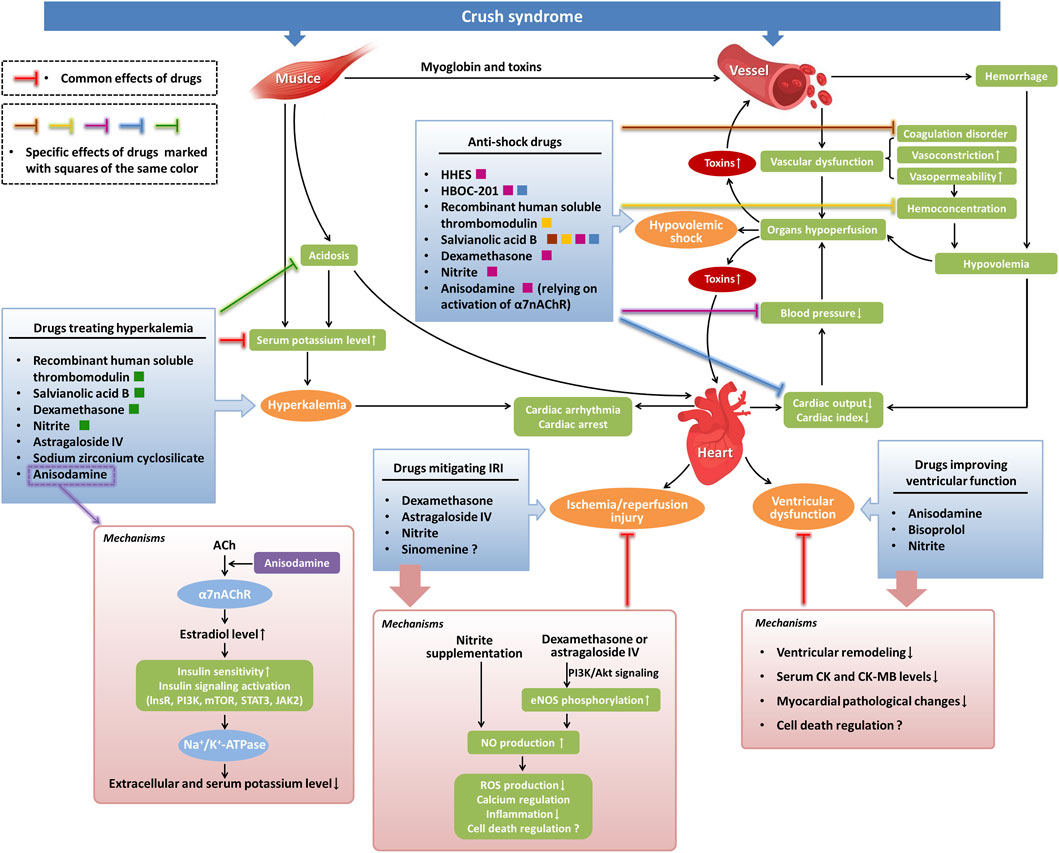
Figure 1. Potential pharmacological investigations of cardiovascular complications in crush syndrome. Severe crush injuries of muscle lead to the release of myoglobin and toxic cellular components from damaged cells to the blood in crush syndrome, resulting in vascular dysfunction, such as the occurrence of endothelial inflammation-induced coagulation disorder and an increase in vasoconstriction and vasopermeability. Large amounts of bleeding, combined with high vasopermeability-induced hemoconcentration, cause hypovolemia in crush syndrome, which leads to hypotension by reducing cardiac output. Hypoperfusion of vital organs induced by hypovolemia, hypotension, and vascular dysfunction in crush syndrome causes hypovolemic shock and increases toxic effects in tissues. Muscle damage-induced and acidosis-induced hyperkalemia in crush syndrome can lead to cardiac arrhythmia or even cardiac arrest. Crush syndrome can also cause myocardial IRI and ventricular dysfunction. In our findings, several anti-shock drugs exert their effects by alleviating coagulation disorders and decreasing hemoconcentration. Other anti-shock drugs treat hypovolemic shock by directly increasing blood pressure or by improving cardiac output. Drugs treating hyperkalemia function by reducing serum potassium levels directly or relying on the alleviation of acidosis. Drugs that mitigate myocardial IRI mainly depend on NO-mediated signaling pathways. The mechanisms involved in drugs improving ventricular function include attenuation of ventricular remodeling, decrease in serum CK and CK-MB levels, inhibition of myocardial pathological changes, and regulation of cell death possibly. ACh, acetylcholine; lnsR, insulin receptor; PI3K, phosphoinositide 3-kinase; mTOR, mammalian target of rapamycin; STAT3, signal transducer and activator of transcription 3; JAK2, Janus kinase 2; NO, nitric oxide; eNOS, endothelial nitric oxide synthase; ROS, reactive oxygen species.
Hyperkalemia-induced cardiac complications in crush syndrome
Several studies have reported that crush-injured patients or models can be complicated with hyperkalemia, cardiac arrest, arrhythmia, and heart failure (Yang et al., 2022; Whiffin et al., 2019; Guo et al., 2013; Murata et al., 2011; Safari et al., 2017; He et al., 2011; Fernandes et al., 2022; Yu et al., 2022; Murata et al., 2022). Hyperkalemia, with triggered cardiac or renal complications, is a major cause of death in crush syndrome. Severely increased potassium in the blood can lead to cardiac dysrhythmia, such as ventricular fibrillation, and even cardiac arrest without proper treatment in time, resulting in high mortality in crush syndrome (Metzger et al., 2010; McCullough et al., 2014). Potassium levels are also believed to be closely associated with mortality in acute myocardial infarction (McCullough et al., 2014; Goyal et al., 2012). Clinically, 70% of patients with hyperkalemia manifest typical electrocardiographic changes, such as elevated ST segments, tall-peaked T waves, PR interval lengthening, and P wave loss, as well as QRS complex widening. Acute treatments for hyperkalemia traditionally include intravenous calcium gluconate, correction of acidosis with sodium bicarbonate, and use of nebulized albuterol in some conditions (McCullough et al., 2014; Yu et al., 2022).
Currently, several novel drugs have been proven effective for hyperkalemia in crush syndrome and crush injury, consequently decreasing the on-site mortality. Co-administration of recombinant human soluble thrombomodulin or astragaloside IV with resuscitating fluids could decrease plasma potassium levels in crush-injured rats (Mohri et al., 2006; Murata et al., 2017). Dexamethasone treatment in the prehospital period also attenuated hyperkalemia in crush syndrome (Murata et al., 2016; Murata et al., 2013). Salvianolic acid B reduced plasma potassium levels, and improved the survival rate by alleviating hyperkalemia-induced cardiac failure, regulating acid-base balance, improving mitochondrial function, and decreasing the inflammatory response (Murata et al., 2022). Early intervention with sodium zirconium cyclosilicate reduced blood potassium levels in a rat crush syndrome model with no adverse effect on renal function (Li et al., 2024). Our studies demonstrated that anisodamine could alleviate hyperkalemia in crush syndrome animals with no risk of hypokalemia. Although the mechanisms involved in the downregulation of blood potassium levels for some drugs are still unclear, we found some clues regarding the effects of anisodamine. Anisodamine could decrease serum potassium levels in crush syndrome animals through activation of α7nAChR and downstream estradiol-induced enhancement of insulin sensitivity, manifested as an increase in serum estradiol which leads to the activation of tyrosine kinase on the insulin receptor, phosphoinositide 3-kinase, mammalian target of rapamycin, signal transducer and activator of transcription 3, and Na+/K+-ATPase (Yu et al., 2019; Fan et al., 2016) (Figure 1: Drugs treating hyperkalemia). Both the anti-hyperkalemia and anti-shock effects of anisodamine contribute to the reduction in the on-site mortality of crush syndrome animals. Another investigation on the combined use of anisodamine and neostigmine also demonstrated their effect on serum potassium decrease in crush syndrome, which depended on the role of α7nAChR in increasing the phosphorylation of Janus kinase two and signal transducer and activator of transcription 3 (Xu et al., 2016).
Other cardiovascular complications in crush syndrome
In addition to shock- and hyperkalemia-induced cardiac complications, crush syndrome can also induce other cardiovascular injuries and impairment of cardiac function, such as myocardial ischemia/reperfusion injury (IRI), ventricular dysfunction, aortic injury, and venous thromboembolic disease (Adachi et al., 1996; Simpson et al., 2023; Mosquera et al., 2013; Liu et al., 2013; Lopez et al., 2021; Rawlins et al., 1999; Hoofnagle et al., 2023; Yu et al., 2022; Murata et al., 2022; Odeh, 1991; Kobayashi and Murata, 2018; Murata et al., 2012). Bisoprolol, a selective β1-blocker, used in combination with saline resuscitation alleviated the changes in the myocardial enzymes creatine kinase (CK) and creatine kinase-MB (CK-MB) in serum and the pathological changes in myocardial tissues in crush-injured rats. It also relieved ventricular remodeling, exhibiting a reduction in left ventricular mass, left ventricular volume at end-systole, and left ventricular internal diameter. The protective role of bisoprolol against heart injury in crush syndrome might result from its effects on decreasing the demand for oxygen and nutrients in the heart and reducing the toxicity of excessive catecholamines on cardiac myocytes, depending on selective and competitive blockade of β1 adrenergic receptors. These effects of bisoprolol led to an increase in the survival rate of crush-injured rats (Yu et al., 2022). Interestingly, our previous study found that anisodamine could also reduce CK and CK-MB levels in the serum of rats with crush syndrome (Fan et al., 2016) (Figure 1: Drugs improving ventricular function). Moreover, it has been reported that in crush injury, oscillatory shear stress-induced gene expression of FOXC2 and PROX1 could regulate the crush-induced procoagulant phenotype and the deep venous thrombosis-related valvular phenotype, guiding us in a novel direction towards crush syndrome therapy (Hoofnagle et al., 2023).
Crush syndrome is usually characterized by IRI (Odeh, 1991; Kobayashi and Murata, 2018). Throughout compression and subsequent rescue and therapy, multiple vital organs, such as the heart, can be damaged during both ischemia and reperfusion periods (Odeh, 1991; Kobayashi and Murata, 2018; Jennische, 1984; Korthuis et al., 1989; Sexton et al., 1990). The mechanisms underlying myocardial IRI in crush syndrome may involve oxidative stress, calcium regulation, and leukocyte activation (Odeh, 1991; Watts et al., 1980; Allen and Orchard, 1987). Specific therapeutic methods for myocardial IRI in crush syndrome are lacking, although there has been a report mentioning tourniquet application to attenuate IRI in crush injury (Schwartz et al., 2015).
Nitrite has been thought beneficial for the treatment of IRI experimentally (Kobayashi and Murata, 2018; Murata et al., 2012). Nitrite deficiency may cause severe myocardial dysfunction such as IRI-induced myocardial infarction, which can be relieved by nitrite supplementation (Bryan et al., 2007). Administration of nitrite before reperfusion in crush-injured rats covered the deficit of cardiac and systemic nitrite and restored nitric oxide bioavailability, leading to the alleviation of increased potassium, lactate dehydrogenase, and CK, inhibition of systemic inflammation, and reduction of crush-injured rat mortality (Murata et al., 2012). The underlying mechanisms by which nitrite alleviates myocardial IRI in crush syndrome might be related to nitric oxide-mediated cytoprotection, such as the reduction of reactive oxygen species production, regulation of Ca2+ channels, and attenuation of systemic inflammation (Chouchani et al., 2013; Sun et al., 2007; Sun and Murphy, 2010). In addition, the therapeutic effects of dexamethasone and astragaloside IV on crush syndrome include the prevention of IRI by elevating nitrite levels, increasing nitric oxide production via the phosphoinositide 3-kinase/Akt signaling pathway, and enhancing endothelial nitric oxide synthase phosphorylation (Murata et al., 2013; Murata et al., 2017; Kobayashi and Murata, 2018) (Figure 1: Drugs mitigating IRI). Studies have reported that sinomenine can attenuate cerebral, renal and hepatic IRI, as well as myocardial IRI (Yang et al., 2016; Zhao et al., 2013; Song et al., 2010; Lu et al., 2022; Xia et al., 2022). Sinomenine may be a promising drug for myocardial IRI therapy in crush syndrome, which requires further research (Zhang et al., 2021). In addition, several regulated cell death patterns, such as apoptosis and ferroptosis, are involved in crush syndrome and its complications. Cardiovascular complications in crush syndrome may be related to the death of cardiovascular cells (Liu et al., 2013; Qiao et al., 2023; Usuda et al., 2023). Studies focusing on death regulation in cardiomyocytes and vascular cells may also be beneficial for therapeutic strategies of crush syndrome in the future.
Early and proper pharmacological therapy is important for the treatment of crush syndrome. Besides making efforts to reduce on-site mortality by modulating cardiovascular symptoms, treatment of other systemic complications is also necessary. For example, anisodamine and salvianolic acid B can protect both the cardiovascular system and kidney function, manifested as a decrease in serum creatinine and blood urea nitrogen (Yu et al., 2019; Fan et al., 2016; Murata et al., 2022). Drugs with multiple therapeutic effects appear to be a promising strategy for treating crush syndrome. However, most of the potential drugs for crush syndrome included in our review are focused on the treatment of a single symptom or simplex systemic complications involved in crush syndrome, such as cardiovascular systemic symptoms. In addition, the cellular or molecular regulatory pathways of these therapeutic drugs have not been fully illustrated because the specific underlying mechanisms are not entirely clear. These limitations lied in our review call for further studies on these drugs.
Conclusion
Current prehospital treatments for crush syndrome have focused on prompt fluid resuscitation and precautions against complications associated with crush syndrome, such as hyperkalemia, cardiac dysrhythmias, and acute kidney injury. Crush syndrome-related cardiovascular complications lead to a low survival rate and poor prognosis in crush syndrome patients, while safe and effective therapeutic methods are still lacking. Several drug candidates have proven promising for the treatment of hypovolemic shock, hyperkalemia-induced cardiac complications, myocardial IRI, ventricular dysfunction, and coagulation disorders, as summarized in Table 1. Further clinical investigations in patients with crush syndrome are required to promote the clinical application of these drugs in crush syndrome therapy.
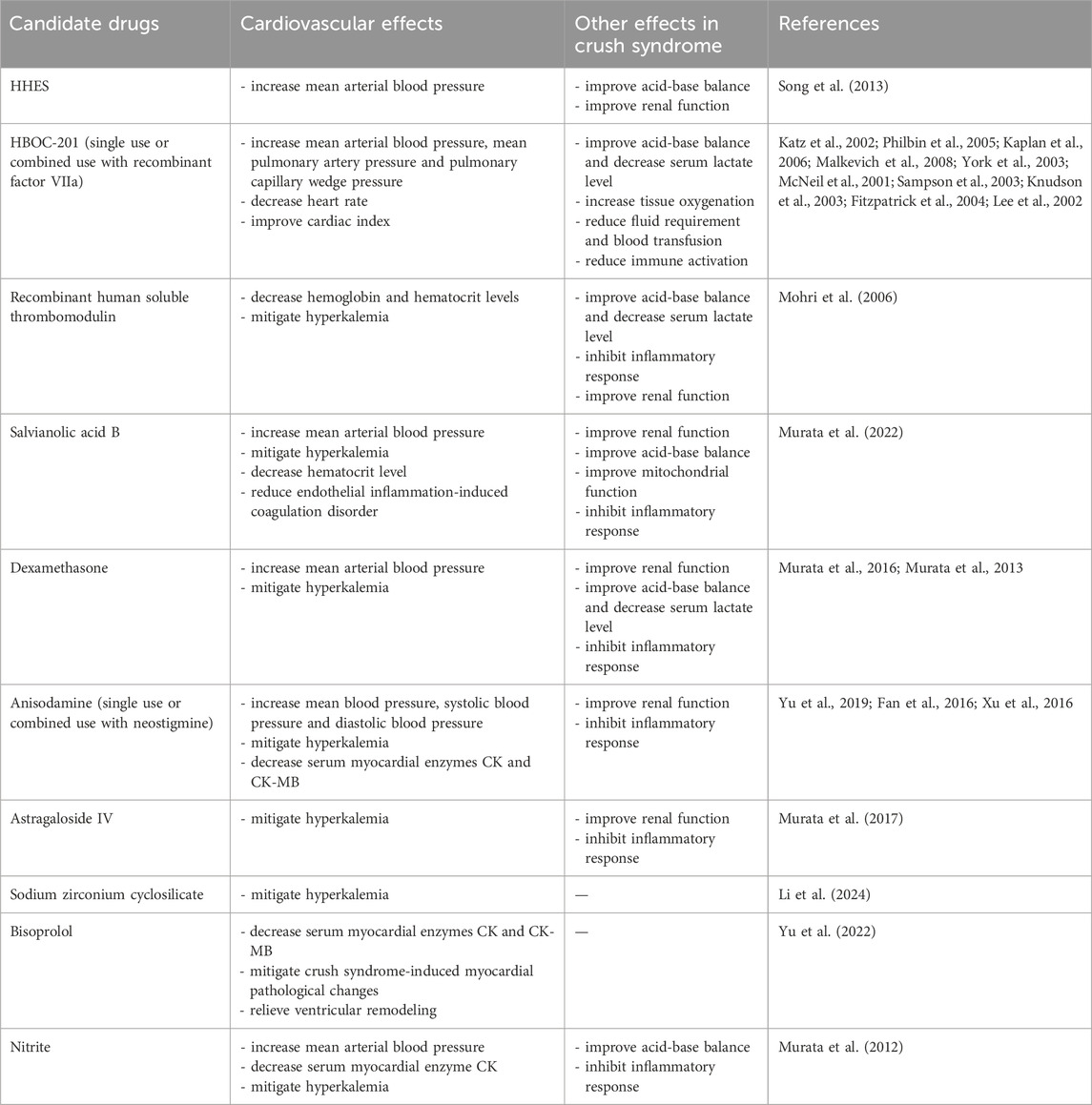
Table 1. Drug candidates besides fluid resuscitation for cardiovascular complications in crush syndrome.
Author contributions
M-WZ: Conceptualization, Writing–original draft, Funding acquisition. F-QT: Conceptualization, Writing–original draft. J-RY: Methodology, Writing–original draft. J-GY: Conceptualization, Writing–review and editing, Funding acquisition.
Funding
The author(s) declare that financial support was received for the research, authorship, and/or publication of this article. This work was supported by grants from the National Natural Science Foundation of China (82173812 and 82104161) and the Nurture projects for basic research of Shanghai Chest Hospital (2020YNJCQ01 and 2020YNJCM08).
Conflict of interest
The authors declare that the research was conducted in the absence of any commercial or financial relationships that could be construed as a potential conflict of interest.
Publisher’s note
All claims expressed in this article are solely those of the authors and do not necessarily represent those of their affiliated organizations, or those of the publisher, the editors and the reviewers. Any product that may be evaluated in this article, or claim that may be made by its manufacturer, is not guaranteed or endorsed by the publisher.
References
Abu-Zidan, F. M., Idris, K., and Cevik, A. A. (2023). Prehospital management of earthquake crush injuries: a collective review. Turk J. Emerg. Med. 23, 199–210. doi:10.4103/tjem.tjem_201_23
Adachi, K., Kawata, M., Araki, S., Matsumoto, A., Mukai, T., and Ikoma, T. (1996). A case of Crush syndrome with giant negative T waves and reversible left ventricular dysfunction. Jpn. Circ. J. 60, 809–814. doi:10.1253/jcj.60.809
Allen, D. G., and Orchard, C. H. (1987). Myocardial contractile function during ischemia and hypoxia. Circ. Res. 60, 153–168. doi:10.1161/01.res.60.2.153
Allon, M., and Shanklin, N. (1996). Effect of bicarbonate administration on plasma potassium in dialysis patients: interactions with insulin and albuterol. Am. J. Kidney Dis. 28, 508–514. doi:10.1016/s0272-6386(96)90460-6
Altintepe, L., Guney, I., Tonbul, Z., Turk, S., Mazi, M., Agca, E., et al. (2007). Early and intensive fluid replacement prevents acute renal failure in the crush cases associated with spontaneous collapse of an apartment in Konya. Ren. Fail 29, 737–741. doi:10.1080/08860220701460095
Ashkenazi, I., Isakovich, B., Kluger, Y., Alfici, R., Kessel, B., and Better, O. S. (2005). Prehospital management of earthquake casualties buried under rubble. Prehosp Disaster Med. 20, 122–133. doi:10.1017/s1049023x00002302
Better, O. S. (1990). The crush syndrome revisited (1940-1990). Nephron 55, 97–103. doi:10.1159/000185934
Better, O. S. (1999). Rescue and salvage of casualties suffering from the crush syndrome after mass disasters. Mil. Med. 164, 366–369. doi:10.1093/milmed/164.5.366
Better, O. S., and Stein, J. H. (1990). Early management of shock and prophylaxis of acute renal failure in traumatic rhabdomyolysis. N. Engl. J. Med. 322, 825–829. doi:10.1056/NEJM199003223221207
Brown, C. V., Rhee, P., Chan, L., Evans, K., Demetriades, D., and Velmahos, G. C. (2004). Preventing renal failure in patients with rhabdomyolysis: do bicarbonate and mannitol make a difference? J. Trauma 56, 1191–1196. doi:10.1097/01.ta.0000130761.78627.10
Bryan, N. S., Calvert, J. W., Elrod, J. W., Gundewar, S., Ji, S. Y., and Lefer, D. J. (2007). Dietary nitrite supplementation protects against myocardial ischemia-reperfusion injury. Proc. Natl. Acad. Sci. U. S. A. 104, 19144–19149. doi:10.1073/pnas.0706579104
Burns, K., Cone, D. C., and Portereiko, J. V. (2010). Complex extrication and crush injury. Prehosp Emerg. Care 14, 240–244. doi:10.3109/10903120903564498
Chavez, L. O., Leon, M., Einav, S., and Varon, J. (2016). Beyond muscle destruction: a systematic review of rhabdomyolysis for clinical practice. Crit. Care 20, 135. doi:10.1186/s13054-016-1314-5
Chouchani, E. T., Methner, C., Nadtochiy, S. M., Logan, A., Pell, V. R., Ding, S., et al. (2013). Cardioprotection by S-nitrosation of a cysteine switch on mitochondrial complex I. Nat. Med. 19, 753–759. doi:10.1038/nm.3212
Criddle, L. M. (2003). Rhabdomyolysis. Pathophysiology, recognition, and management. Crit. Care Nurse 23 (14-22), 14–32. 24-16, 28 passim; quiz 31-12.
Emig, U., Schmidt, G., Hellige, G., and Vetterlein, F. (2003). Contribution of myoglobin-induced increases in vascular resistance to shock decompensation in experimental Crush-syndrome in anesthetized rats. Shock 19, 79–84. doi:10.1097/00024382-200301000-00015
Fan, B. S., Zhang, E. H., Wu, M., Guo, J. M., Su, D. F., Liu, X., et al. (2016). Activation of α7 nicotinic acetylcholine receptor decreases on-site mortality in crush syndrome through insulin signaling-Na/K-ATPase pathway. Front. Pharmacol. 7, 79. doi:10.3389/fphar.2016.00079
Fernandes, S., Oatman, E., Weinberger, J., Dixon, A., Osei-Owusu, P., and Hou, S. (2022). The susceptibility of cardiac arrhythmias after spinal cord crush injury in rats. Exp. Neurol. 357, 114200. doi:10.1016/j.expneurol.2022.114200
Fitzpatrick, C. M., Savage, S. A., Kerby, J. D., Clouse, W. D., and Kashyap, V. S. (2004). Resuscitation with a blood substitute causes vasoconstriction without nitric oxide scavenging in a model of arterial hemorrhage. J. Am. Coll. Surg. 199, 693–701. doi:10.1016/j.jamcollsurg.2004.07.025
Genthon, A., and Wilcox, S. R. (2014). Crush syndrome: a case report and review of the literature. J. Emerg. Med. 46, 313–319. doi:10.1016/j.jemermed.2013.08.052
Gibney, R. T., Sever, M. S., and Vanholder, R. C. (2014). Disaster nephrology: crush injury and beyond. Kidney Int. 85, 1049–1057. doi:10.1038/ki.2013.392
Gonzalez, D. (2005). Crush syndrome. Crit. Care Med. 33, S34–S41. doi:10.1097/01.ccm.0000151065.13564.6f
Goyal, A., Spertus, J. A., Gosch, K., Venkitachalam, L., Jones, P. G., Van Den Berghe, G., et al. (2012). Serum potassium levels and mortality in acute myocardial infarction. JAMA 307, 157–164. doi:10.1001/jama.2011.1967
Gunal, A. I., Celiker, H., Dogukan, A., Ozalp, G., Kirciman, E., Simsekli, H., et al. (2004). Early and vigorous fluid resuscitation prevents acute renal failure in the crush victims of catastrophic earthquakes. J. Am. Soc. Nephrol. 15, 1862–1867. doi:10.1097/01.asn.0000129336.09976.73
Guo, X., Wang, D., and Liu, Z. (2013). Electrocardiographic changes after injury in a rat model of combined crush injury. Am. J. Emerg. Med. 31, 1661–1665. doi:10.1016/j.ajem.2013.08.054
He, Q., Wang, F., Li, G., Chen, X., Liao, C., Zou, Y., et al. (2011). Crush syndrome and acute kidney injury in the Wenchuan Earthquake. J. Trauma 70, 1213–1217. ; discussion 1217-1218. doi:10.1097/TA.0b013e3182117b57
Hoofnagle, M. H., Hess, A., Nalugo, M., Ghosh, S., Hughes, S. W., Fuchs, A., et al. (2023). Defects in vein valve PROX1/FOXC2 antithrombotic pathway in endothelial cells drive the hypercoagulable state induced by trauma and critical illness. J. Trauma Acute Care Surg. 95, 197–204. doi:10.1097/TA.0000000000003945
Jennische, E. (1984). Post-ischemic calcification in skeletal muscle. A light microscopic study in the rat. Acta Pathol. Microbiol. Immunol. Scand. A 92, 139–145. doi:10.1111/j.1699-0463.1984.tb04388.x
Kaplan, L. J., Philbin, N., Arnaud, F., Rice, J., Dong, F., and Freilich, D. (2006). Resuscitation from hemorrhagic shock: fluid selection and infusion strategy drives unmeasured ion genesis. J. Trauma 61, 90–97. ; discussion 97-98. doi:10.1097/01.ta.0000222578.85413.4e
Katz, L. M., Manning, J. E., Mccurdy, S., Pearce, L. B., Gawryl, M. S., Wang, Y., et al. (2002). HBOC-201 improves survival in a swine model of hemorrhagic shock and liver injury. Resuscitation 54, 77–87. doi:10.1016/s0300-9572(02)00053-9
Knudson, M. M., Lee, S., Erickson, V., Morabito, D., Derugin, N., and Manley, G. T. (2003). Tissue oxygen monitoring during hemorrhagic shock and resuscitation: a comparison of lactated Ringer's solution, hypertonic saline dextran, and HBOC-201. J. Trauma 54, 242–252. doi:10.1097/01.TA.0000037776.28201.75
Kobayashi, J., and Murata, I. (2018). Nitrite as a pharmacological intervention for the successful treatment of crush syndrome. Physiol. Rep. 6, e13633. doi:10.14814/phy2.13633
Korthuis, R. J., Smith, J. K., and Carden, D. L. (1989). Hypoxic reperfusion attenuates postischemic microvascular injury. Am. J. Physiol. 256, H315–H319. doi:10.1152/ajpheart.1989.256.1.H315
Kraut, J. A., and Kurtz, I. (2006). Use of base in the treatment of acute severe organic acidosis by nephrologists and critical care physicians: results of an online survey. Clin. Exp. Nephrol. 10, 111–117. doi:10.1007/s10157-006-0408-9
Kraut, J. A., and Madias, N. E. (2010). Metabolic acidosis: pathophysiology, diagnosis and management. Nat. Rev. Nephrol. 6, 274–285. doi:10.1038/nrneph.2010.33
Lee, S. K., Morabito, D., Hemphill, J. C., Erickson, V., Holcroft, J. J., Derugin, N., et al. (2002). Small-volume resuscitation with HBOC-201: effects on cardiovascular parameters and brain tissue oxygen tension in an out-of-hospital model of hemorrhage in swine. Acad. Emerg. Med. 9, 969–976. doi:10.1111/j.1553-2712.2002.tb02127.x
Li, D., Zhang, Y., Chen, Y., Yang, B., Chen, J., Shi, J., et al. (2024). Advancing crush syndrome management: the potent role of Sodium zirconium cyclosilicate in early hyperkalemia intervention and survival enhancement in a rat model. Front. Pharmacol. 15, 1381954. doi:10.3389/fphar.2024.1381954
Li, W., Qian, J., Liu, X., Zhang, Q., Wang, L., Chen, D., et al. (2009). Management of severe crush injury in a front-line tent ICU after 2008 Wenchuan earthquake in China: an experience with 32 cases. Crit. Care 13, R178. doi:10.1186/cc8160
Liu, S., Yu, Y., Luo, B., Liao, X., and Tan, Z. (2013). Impact of traumatic muscle crush injury as a cause of cardiomyocyte-specific injury: an experimental study. Heart Lung Circ. 22, 284–290. doi:10.1016/j.hlc.2012.11.008
Long, B., Liang, S. Y., and Gottlieb, M. (2023). Crush injury and syndrome: a review for emergency clinicians. Am. J. Emerg. Med. 69, 180–187. doi:10.1016/j.ajem.2023.04.029
Long, B., Warix, J. R., and Koyfman, A. (2018). Controversies in management of hyperkalemia. J. Emerg. Med. 55, 192–205. doi:10.1016/j.jemermed.2018.04.004
Lopez, K., Suen, A., Yang, Y., Wang, S., Williams, B., Zhu, J., et al. (2021). Hypobaria exposure worsens cardiac function and endothelial injury in an animal model of polytrauma: implications for aeromedical evacuation. Shock 56, 601–610. doi:10.1097/SHK.0000000000001716
Lu, C., Guo, X., He, X., Chang, Y., Zheng, F., Xu, C., et al. (2022). Cardioprotective effects of sinomenine in myocardial ischemia/reperfusion injury in a rat model. Saudi Pharm. J. 30, 669–678. doi:10.1016/j.jsps.2022.04.005
Malinoski, D. J., Slater, M. S., and Mullins, R. J. (2004). Crush injury and rhabdomyolysis. Crit. Care Clin. 20, 171–192. doi:10.1016/s0749-0704(03)00091-5
Malkevich, N. V., Dong, F., Vandermolen, C. A., Philbin, N. B., Rice, J. P., Scultetus, A., et al. (2008). Innate immune response after resuscitation with hemoglobin-based oxygen carrier and recombinant factor VIIA in uncontrolled hemorrhagic shock in a swine model. J. Trauma 64, 1498–1510. doi:10.1097/TA.0b013e3181454a05
Mccullough, P. A., Beaver, T. M., Bennett-Guerrero, E., Emmett, M., Fonarow, G. C., Goyal, A., et al. (2014). Acute and chronic cardiovascular effects of hyperkalemia: new insights into prevention and clinical management. Rev. Cardiovasc Med. 15, 11–23. doi:10.3909/ricm0727
Mcneil, C. J., Smith, L. D., Jenkins, L. D., York, M. G., and Josephs, M. J. (2001). Hypotensive resuscitation using a polymerized bovine hemoglobin-based oxygen-carrying solution (HBOC-201) leads to reversal of anaerobic metabolism. J. Trauma 50, 1063–1075. doi:10.1097/00005373-200106000-00015
Metzger, J. C., Eastman, A. L., and Pepe, P. E. (2010). Year in review 2009: critical Care--cardiac arrest, trauma and disasters. Crit. Care 14, 242. doi:10.1186/cc9302
Mohri, T., Tanaka, H., Tajima, G., Kajino, K., Sonoi, H., Hosotsubo, H., et al. (2006). Synergistic effects of recombinant human soluble thrombomodulin and fluid-volume resuscitation in a rat lethal crush injury model. Shock 26, 581–586. doi:10.1097/01.shk.0000233198.48612.6b
Mosquera, V. X., Marini, M., Muniz, J., Lopez-Perez, J. M., Gulias, D., and Cuenca, J. J. (2013). Aortic injuries in crush trauma patients: different mechanism, different management. Injury 44, 60–65. doi:10.1016/j.injury.2011.09.022
Murata, I., Abe, Y., Yaginuma, Y., Yodo, K., Kamakari, Y., Miyazaki, Y., et al. (2017). Astragaloside-IV prevents acute kidney injury and inflammation by normalizing muscular mitochondrial function associated with a nitric oxide protective mechanism in crush syndrome rats. Ann. Intensive Care 7, 90. doi:10.1186/s13613-017-0313-2
Murata, I., Goto, M., Komiya, M., Motohashi, R., Hirata, M., Inoue, Y., et al. (2016). Early therapeutic intervention for crush syndrome: characterization of intramuscular administration of dexamethasone by pharmacokinetic and biochemical parameters in rats. Biol. Pharm. Bull. 39, 1424–1431. doi:10.1248/bpb.b15-01034
Murata, I., Nozaki, R., Ooi, K., Ohtake, K., Kimura, S., Ueda, H., et al. (2012). Nitrite reduces ischemia/reperfusion-induced muscle damage and improves survival rates in rat crush injury model. J. Trauma Acute Care Surg. 72, 1548–1554. doi:10.1097/TA.0b013e31824a76b5
Murata, I., Ooi, K., Sasaki, H., Kimura, S., Ohtake, K., Ueda, H., et al. (2011). Characterization of systemic and histologic injury after crush syndrome and intervals of reperfusion in a small animal model. J. Trauma 70, 1453–1463. doi:10.1097/TA.0b013e31820ca00a
Murata, I., Ooi, K., Shoji, S., Motohashi, Y., Kan, M., Ohtake, K., et al. (2013). Acute lethal crush-injured rats can be successfully rescued by a single injection of high-dose dexamethasone through a pathway involving PI3K-Akt-eNOS signaling. J. Trauma Acute Care Surg. 75, 241–249. doi:10.1097/TA.0b013e3182905f11
Murata, I., Sugai, T., Murakawa, Y., Miyamoto, Y., Kobayashi, J., Inoue, Y., et al. (2022). Salvianolic acid B improves the survival rate, acute kidney dysfunction, inflammation and NETosis-mediated antibacterial action in a crush syndrome rat model. Exp. Ther. Med. 23, 320. doi:10.3892/etm.2022.11249
Odeh, M. (1991). The role of reperfusion-induced injury in the pathogenesis of the crush syndrome. N. Engl. J. Med. 324, 1417–1422. doi:10.1056/NEJM199105163242007
Philbin, N., Rice, J., Gurney, J., Mcgwin, G., Arnaud, F., Dong, F., et al. (2005). A hemoglobin-based oxygen carrier, bovine polymerized hemoglobin (HBOC-201) versus hetastarch (HEX) in a moderate severity hemorrhagic shock swine model with delayed evacuation. Resuscitation 66, 367–378. doi:10.1016/j.resuscitation.2005.03.019
Qiao, O., Wang, X., Wang, Y., Li, N., and Gong, Y. (2023). Ferroptosis in acute kidney injury following crush syndrome: a novel target for treatment. J. Adv. Res. 54, 211–222. doi:10.1016/j.jare.2023.01.016
Rawlins, M., Gullichsen, E., Kuttila, K., Peltola, O., and Niinikoski, J. (1999). Central hemodynamic changes in experimental muscle crush injury in pigs. Eur. Surg. Res. 31, 9–18. doi:10.1159/000008616
Sabatini, S., and Kurtzman, N. A. (2009). Bicarbonate therapy in severe metabolic acidosis. J. Am. Soc. Nephrol. 20, 692–695. doi:10.1681/ASN.2007121329
Safari, S., Najafi, I., Hosseini, M., Baratloo, A., Yousefifard, M., and Mohammadi, H. (2017). 20-Day trend of serum potassium changes in bam earthquake victims with crush syndrome; a cross-sectional study. Emerg. (Tehran) 5, e5.
Sahjian, M., and Frakes, M. (2007). Crush injuries: pathophysiology and current treatment. Nurse Pract. 32, 13–18. doi:10.1097/01.NPR.0000287464.81259.8b
Sampson, J. B., Davis, M. R., Mueller, D. L., Kashyap, V. S., Jenkins, D. H., and Kerby, J. D. (2003). A comparison of the hemoglobin-based oxygen carrier HBOC-201 to other low-volume resuscitation fluids in a model of controlled hemorrhagic shock. J. Trauma 55, 747–754. doi:10.1097/01.TA.0000084519.47163.77
Scharman, E. J., and Troutman, W. G. (2013). Prevention of kidney injury following rhabdomyolysis: a systematic review. Ann. Pharmacother. 47, 90–105. doi:10.1345/aph.1R215
Schwartz, D. S., Weisner, Z., and Badar, J. (2015). Immediate lower extremity tourniquet application to delay onset of reperfusion injury after prolonged crush injury. Prehosp Emerg. Care 19, 544–547. doi:10.3109/10903127.2015.1005264
Sever, M. S., Erek, E., Vanholder, R., Kantarci, G., Yavuz, M., Turkmen, A., et al. (2003). Serum potassium in the crush syndrome victims of the Marmara disaster. Clin. Nephrol. 59, 326–333. doi:10.5414/cnp59326
Sever, M. S., and Vanholder, R. (2013). Management of crush victims in mass disasters: highlights from recently published recommendations. Clin. J. Am. Soc. Nephrol. 8, 328–335. doi:10.2215/CJN.07340712
Sever, M. S., Vanholder, R., and Disasters, R. O. I. W. G. O. R. F. T. M. O. C. V. I. M. (2012). Recommendation for the management of crush victims in mass disasters. Nephrol. Dial. Transpl. 27 (Suppl. 1), i1–i67. doi:10.1093/ndt/gfs156
Sever, M. S., Vanholder, R., and Lameire, N. (2006). Management of crush-related injuries after disasters. N. Engl. J. Med. 354, 1052–1063. doi:10.1056/NEJMra054329
Sexton, W. L., Korthuis, R. J., and Laughlin, M. H. (1990). Ischemia-reperfusion injury in isolated rat hindquarters. J. Appl. Physiol. 68, 387–392. doi:10.1152/jappl.1990.68.1.387
Simpson, R., Praditsuktavorn, B., Wall, J., Morales, V., Thiemermann, C., Tremoleda, J. L., et al. (2023). Myocardial alterations following traumatic hemorrhagic injury. J. Trauma Acute Care Surg. 95, 481–489. doi:10.1097/TA.0000000000003987
Smith, J., and Greaves, I. (2003). Crush injury and crush syndrome: a review. J. Trauma 54, S226–S230. doi:10.1097/01.TA.0000047203.00084.94
Song, S., Shen, X., Tang, Y., Wang, Z., Guo, W., Ding, G., et al. (2010). Sinomenine pretreatment attenuates cold ischemia/reperfusion injury in rats: the role of heme oxygenase-1. Int. Immunopharmacol. 10, 679–684. doi:10.1016/j.intimp.2010.03.011
Song, X. B., Zhou, X. L., Ni, S. Z., Yang, G. F., Tian, S. F., Shan, J., et al. (2013). Early fluid resuscitation with hypertonic solution in a rat crush injury model. Shock 39, 453–459. doi:10.1097/SHK.0b013e31828e674a
Sun, J., Morgan, M., Shen, R. F., Steenbergen, C., and Murphy, E. (2007). Preconditioning results in S-nitrosylation of proteins involved in regulation of mitochondrial energetics and calcium transport. Circ. Res. 101, 1155–1163. doi:10.1161/CIRCRESAHA.107.155879
Sun, J., and Murphy, E. (2010). Protein S-nitrosylation and cardioprotection. Circ. Res. 106, 285–296. doi:10.1161/CIRCRESAHA.109.209452
Usuda, D., Shimozawa, S., Takami, H., Kako, Y., Sakamoto, T., Shimazaki, J., et al. (2023). Crush syndrome: a review for prehospital providers and emergency clinicians. J. Transl. Med. 21, 584. doi:10.1186/s12967-023-04416-9
Walters, T. J., Powell, D., Penny, A., Stewart, I., Chung, K. K., Keenan, S., et al. (2016). Management of crush syndrome under prolonged field care. J. Spec. Oper. Med. 16, 79–85. doi:10.55460/AW4P-UO9F
Watts, J. A., Koch, C. D., and Lanoue, K. F. (1980). Effects of Ca2+ antagonism on energy metabolism: Ca2+ and heart function after ischemia. Am. J. Physiol. 238, H909–H916. doi:10.1152/ajpheart.1980.238.6.H909
Weisberg, L. S. (2008). Management of severe hyperkalemia. Crit. Care Med. 36, 3246–3251. doi:10.1097/CCM.0b013e31818f222b
Whiffin, A. N. H., Spangler, J. D., Dhir, K., Zhang, R., and Ferguson, J. D. (2019). Bathroom entrapment leading to cardiac arrest from crush syndrome. Prehosp Emerg. Care 23, 90–93. doi:10.1080/10903127.2018.1471558
Xia, B., Li, Q., Wu, J., Yuan, X., Wang, F., Lu, X., et al. (2022). Sinomenine confers protection against myocardial ischemia reperfusion injury by preventing oxidative stress, cellular apoptosis, and inflammation. Front. Pharmacol. 13, 922484. doi:10.3389/fphar.2022.922484
Xu, P., Wang, F., Zhou, X. L., Li, L., Xiong, D., Yong, Y. Q., et al. (2018). Systemic inflammatory response and multiple organ dysfunctions following crush injury: a new experimental model in rabbits. Inflammation 41, 240–248. doi:10.1007/s10753-017-0683-5
Xu, Z. Q., Shao, B. Z., Ke, P., Liu, J. G., Liu, G. K., Chen, X. W., et al. (2016). Combined administration of anisodamine and neostigmine rescued acute lethal crush syndrome through α7nAChR-dependent JAK2-STAT3 signaling. Sci. Rep. 6, 37709. doi:10.1038/srep37709
Yang, S., Ning, F., Li, J., Guo, D., Zhang, L., Cui, R., et al. (2016). Therapeutic effect analysis of sinomenine on rat cerebral ischemia-reperfusion injury. J. Stroke Cerebrovasc. Dis. 25, 1263–1269. doi:10.1016/j.jstrokecerebrovasdis.2016.02.023
Yang, X., Tang, N., Li, L., Xu, G., Dai, J., Tao, K., et al. (2022). Management of a patient with cardiac arrest, intestinal ischemia necrosis, multiple fractures, hemorrhagic shock, renal failure, disseminated intravascular coagulation, and thrombosis after severe abdominal crush injury: a case report. Exp. Ther. Med. 23, 386. doi:10.3892/etm.2022.11313
York, G. B., Eggers, J. S., Smith, D. L., Jenkins, D. H., Mcneil, J. D., Mueller, D., et al. (2003). Low-volume resuscitation with a polymerized bovine hemoglobin-based oxygen-carrying solution (HBOC-201) provides adequate tissue oxygenation for survival in a porcine model of controlled hemorrhage. J. Trauma 55, 873–885. doi:10.1097/01.TA.0000092681.17874.6F
Yu, J. G., Fan, B. S., Guo, J. M., Shen, Y. J., Hu, Y. Y., and Liu, X. (2019). Anisodamine ameliorates hyperkalemia during crush syndrome through estradiol-induced enhancement of insulin sensitivity. Front. Pharmacol. 10, 1444. doi:10.3389/fphar.2019.01444
Yu, M., Lv, Q., Shi, J., Liu, Y., Fan, H., Ding, H., et al. (2022). β1-Blocker improves survival and ventricular remodelling in rats with lethal crush injury. Eur. J. Trauma Emerg. Surg. 48, 455–470. doi:10.1007/s00068-020-01408-y
Zhang, M. W., Wang, X. H., Shi, J., and Yu, J. G. (2021). Sinomenine in cardio-cerebrovascular diseases: potential therapeutic effects and pharmacological evidences. Front. Cardiovasc Med. 8, 749113. doi:10.3389/fcvm.2021.749113
Zhang, X., Bai, X., and Zhou, Q. (2014). First-aid treatments of crush injuries after earthquake: 2 special cases. Am. J. Emerg. Med. 32, 817 e813–e4. doi:10.1016/j.ajem.2013.12.062
Keywords: crush syndrome, fluid resuscitation, hypovolemic shock, hyperkalemia, ischemia/reperfusion injury
Citation: Zhang M-W, Tan F-Q, Yang J-R and Yu J-G (2024) Cardiovascular events in crush syndrome: on-site therapeutic strategies and pharmacological investigations. Front. Pharmacol. 15:1472971. doi: 10.3389/fphar.2024.1472971
Received: 30 July 2024; Accepted: 11 September 2024;
Published: 20 September 2024.
Edited by:
Jun Kobayashi, Josai University, JapanReviewed by:
Mohammad Keykhaei, Johns Hopkins Medicine, United StatesCopyright © 2024 Zhang, Tan, Yang and Yu. This is an open-access article distributed under the terms of the Creative Commons Attribution License (CC BY). The use, distribution or reproduction in other forums is permitted, provided the original author(s) and the copyright owner(s) are credited and that the original publication in this journal is cited, in accordance with accepted academic practice. No use, distribution or reproduction is permitted which does not comply with these terms.
*Correspondence: Jian-Guang Yu, yueqingqiu@163.com
†ORCID: Jian-Guang Yu, orcid.org/0000-0001-5596-5406
‡These authors have contributed equally to this work and share first authorship