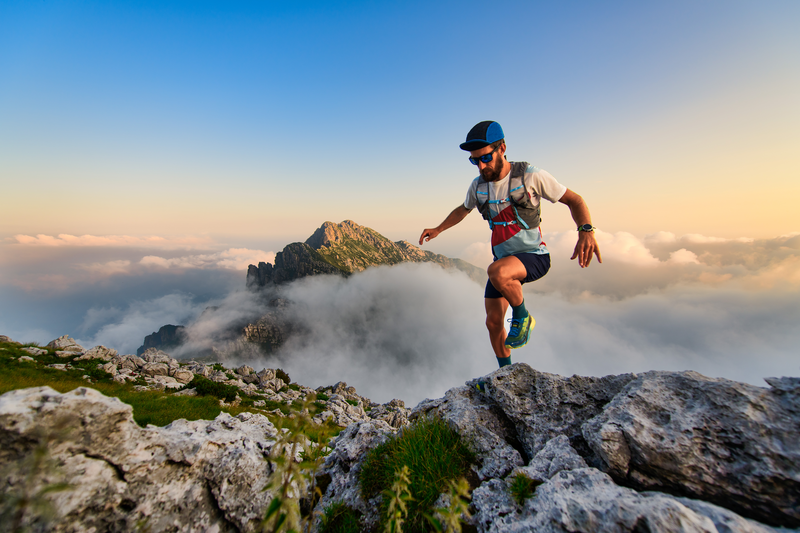
94% of researchers rate our articles as excellent or good
Learn more about the work of our research integrity team to safeguard the quality of each article we publish.
Find out more
ORIGINAL RESEARCH article
Front. Pharmacol. , 27 September 2024
Sec. Pharmacogenetics and Pharmacogenomics
Volume 15 - 2024 | https://doi.org/10.3389/fphar.2024.1467036
Introduction: The Cuban population is genetically diverse, and information on the prevalence of genetic variants is still limited. As complex admixture processes have occurred, we hypothesized that the frequency of pharmacogenetic variants and drug responses may vary within the country. The aims of the study were to describe the frequency distribution of 43 single-nucleotide variants (SNVs) from 25 genes of pharmacogenetic interest within the Cuba population and in relation to other populations, while taking into consideration some descriptive variables such as place of birth and skin color.
Materials and Methods: SNVs were analyzed in 357 unrelated healthy Cuban volunteers. Genotype, allele frequencies, and ancestry proportions were determined, and the pairwise fixation index (FST ) was evaluated.
Results: Hardy–Weinberg equilibrium (HWE) deviations in six loci (rs11572103, rs2740574, rs776746, rs3025039, rs861539, and rs1762429) were identified. Minor allele frequencies (MAFs) ranged from 0.00 to 0.15 for variants in genes encoding xenobiotic metabolizing enzymes. They also ranged from 0.01 to 0.21 for variants in DNA repair, growth factors, methyltransferase, and methyl-binding proteins, while they ranged from 0.04 to 0.27 for variants in the O-6-methylguanine-DNA methyltransferase enzyme. Moderate genetic divergence was observed upon comparison to Africans (FST = 0.071 and SD 0.079), with 19 markers exhibiting moderate-to-large genetic differentiation. The average European, African, and Amerindian ancestry proportions were 67.8%, 27.2%, and 5.3%, respectively. Ancestry proportions differed by skin color and birthplace for both African and European components, with the exception of the European component, which showed no significant difference between individuals from Western and Eastern regions. Meanwhile, the statistical significance varied in comparisons by skin color and birthplace within the Amerindian component. Low genetic divergence was observed across geographical regions. We identified 12 variants showing moderate-to-large differentiation between White/Black individuals.
Conclusion: Altogether, our results may support national strategies for the introduction of pharmacogenetic tools in clinical practice, contributing to the development of precision medicine in Cuba.
Single-nucleotide variants (SNVs) occur throughout the genome and constitute most of the human genetic diversity (Robert and Pelletier, 2018). These genomic variants can alter all steps of gene expression and protein functionality, ultimately modifying the effects of environmental exposures. In the context of drug responses and the safety of commonly prescribed drugs, SNVs are key factors accounting for interindividual variability. Therefore, characterization of populations in terms of the frequency distribution of SNVs relevant to pharmacogenetics provides a powerful approach for evaluating the suitability of its clinical applications in drug selection and dosage optimization, improving therapeutic efficacy while reducing adverse effects.
The American continent is highly heterogeneous and presents the second-greatest number of abundant region-specific alleles (i.e., that are common in one continent but absent in the rest of the world), reflecting their unique evolution (Fedorova et al., 2022). A study of the Consortium of the Ibero-American Network of Pharmacogenetics and Pharmacogenomics (CEIBA-RIBEF) showed that interethnic variability in clinically relevant drug metabolizing enzymes (CYP2D6, CYP2C9, and CYP2C19) in Latin America leads to a lack of correlation between the ‘‘predicted’’ enzyme metabolic capacity and the genotype (Naranjo et al., 2018). Differences in admixture history in Latin American populations have important implications for the frequency distribution of variants associated with drug absorption, distribution, metabolism, and excretion (ADME) responses between and within populations (Céspedes-Garro et al., 2016), making extrapolation of data not suitable. By characterizing the admixture proportions in Latin American countries and the distribution of ADME variants of pharmacogenetic relevance, it should be possible to share health policies and logistic solutions between similar populations. At the individual level, individual ancestry proportions may determine the probability of having a pharmacogenetic relevant genotype (Bonifaz-Pena et al., 2014). Thus, collectively studying SNVs across human populations is of great interest to develop precision medicine.
Cuba is the most populated country in the Caribbean and has a rich genetic heritage. The population is essentially the result of admixture between Spaniards, West Africans, and, to a lesser degree, native Amerindians who inhabited the island. Evidence from historical and anthropological studies indicates that native populations were drastically reduced to a few thousand people within 50 years after 1492 (Guanche, 2020). Studies on the Cuban population, using ancestry informative marker (AIM) panels purposely designed to reveal differences in allele frequencies between Native American, West African, and European populations, have confirmed the small contribution of the Amerindian component to the current Cuban genetic background (Cintado et al., 2009; Diaz-Horta et al., 2010; Marcheco-Teruel et al., 2014; Fortes-Lima et al., 2018). Spanish immigration took place during more than four centuries and consisted mainly of male individuals, who intensely mixed with other ethnic components of the Cuba population (i.e., native and African women). During the XX century, a marked tendency was observed among the Spanish immigrants to establish themselves in the Western–Central areas of the country compared to the eastern region (Guanche, 2020). As a result, insights into Cuban contemporary population and admixture dynamics show different ancestry proportions across provinces and regions of the country (Marcheco-Teruel et al., 2014; Fortes-Lima et al., 2018).
Comparisons to other populations have identified interethnic variability in CYP2D6 and CYP2C9 allele frequencies and metabolic phenotypes among Cubans, Spaniards (Llerena et al., 2014), and Nicaraguans (Llerena et al., 2012). In addition, there is evidence of geographic variation within the Cuban population in allele and genotype frequency of rs1045642 MDR1 when skin color categories are considered (Rodeiro et al., 2022). As complex admixture processes have occurred, we hypothesized that the frequency of pharmacogenetic variants and drug responses may vary within the country. Understanding the genetic heterogeneity and admixture of Cubans between geographical regions should have important implications for the design and interpretation of clinical trials, the implementation of pharmacogenetic tools for drug prescription and dosage adjustment, and the extrapolation of data from other, more homogeneous populations. Despite the increasing number of studies describing genetic biomarkers in Cubans (Sotomayor-Lugo et al., 2022; Camacho et al., 2011; García et al., 2010), information about population allele frequencies from a representative sample of the Cuban population is still limited. In accordance, this study aimed to describe the distribution of 43 SNVs in 25 target pharmacogenes for the Cuban population and in relation to other populations. It was also intended to characterize the distribution of the SNVs by considering the descriptive variables such as place of birth and skin color. This knowledge should provide valuable data for (Robert and Pelletier, 2018) understanding the allele and genotype distribution of variants in clinically relevant pharmacogenes in the country (Fedorova et al., 2022), implementing personalized medicine approaches and pharmacogenetic testing in public health policies, and (Naranjo et al., 2018) establishing public health priorities across the country.
The study included 357 Cuban healthy volunteers older than 18 years; female volunteers accounted for 52.7% (n = 188) of the sample. Demographic variables such as skin color and place of birth were recorded. The ethnic classification was based on skin color self-perception, as defined in the last national census (Oficina Nacional de Estadísticas e Información. Centro de Estudios de Población y Desarrollo, 2016). According to this classification, the Cuban population may be clustered into three categories based on skin color: ‘Blanco’’ (white), ‘‘Mestizo’’ (admixed), and ‘‘Negro’’ (black) individuals. From the whole sample, 190 volunteers self-identified as White individuals (53.2%), 101 as admixed individuals (28.3%), and 66 as Black individuals (18.5%), and according to the place of birth, participants were grouped into Western (48.7%, n = 174), Center (13.7%. n = 49), and Eastern (37.5%, n = 134) regions.
Whole blood samples were obtained by venipuncture. Genomic DNA extraction was performed using the QIAGEN DNeasy® Blood and Tissue Kit, following the manufacturer’s recommendations. A total of 41 SNVs (see Supplementary Table 1) and 34 AIMs (Phillips et al., 2012) (AIM panel details provided in Supplementary Table 2) were determined by targeted sequencing (amplicon sequencing method) on a HiSeq X Ten sequencer (Illumina platform) according to Illumina protocols. The GSTM1 null and GSTT1 null variants were analyzed using one-step multiplex real-time RT-PCR followed by high-resolution melting (HRM) curve analysis. The primers used were GSTT1-forward TTCCTTACTGGTCCTCACATCTC, GSTT1-reverse GGAAAAGGGTACAGACTGGGGA, GSTM1-forward AACTCCCTGAAAAGCTAAAGC, and GSTM1-reverse GTTGGGCTCAAATATACGGTGG. The DNA amplification protocol included an initial denaturation at 95°C for 10 min, followed by 35 cycles of denaturation at 95°C for 10 s, annealing at 62°C for 30 s, and extension at 72°C for 25 s for DNA polymerization. The program of the melting curve analysis consisted of 95°C for 10 s, 65°C for 1 min, and then ramping from 65°C to 95°C at a rate of 0.1°C/s.
Global ancestry proportions were determined using the program STRUCTURE v 2.3.4 (Pritchard et al., 2000). The runs consisted of 100,000 Markov Chain Monte Carlo steps after a burn-in period of length 50,000 with 20 replicates for a K-value of 3. The admixture and correlated allele frequencies models were applied, and we used prior specification of the population of origin of reference samples. The results were combined using the online version of CLUMPAK (available at http://clumpak.tau.ac.il.) (Kopelman et al., 2015). Individuals from the Human Genome Diversity Project (HGDP) (Bergström et al., 2020) and 1000 Genomes Project (Fairley et al., 2019) datasets were used as reference ancestral populations. Based on Cuban admixture, reference genotypes included 107 Europeans (Iberians IBS) and 405 Africans (YRI Yoruba, ESN Nigeria, MSL Sierra Leona, and GWD Gambia). HGDP populations consisted of 60 Spanish, 35 Mexicans (Pima and Maya), 22 Brazil (Karitiana and Suri), and 7 Colombia (Colombians) genotypes. These were obtained using the online tool SPSmart SNPforID 34-plex variability browser (http://spsmart.cesga.es/snpforid.php) (Amigo et al., 2008).
Allele and genotype frequencies were calculated. The chi square (χ2) test was used to check the Hardy–Weinberg equilibrium (HWE) and Fisher exact test (Fisher–Freeman–Halton format) (Freeman and Halton, 1951) to compare the genotype distribution among subgroups. Statistical differences in ancestry proportions were evaluated using Kruskal–Wallis (skin color and place of birth) analysis of variance, followed by post hoc Dunn’s test using a Bonferroni corrected alpha of 0.017 for multiple comparisons. Statistical significance was set at p-value < 0.05 in the two-sided test. Statistical analyses were carried out using the RStudio programming environment for data analysis and “stats” package version 4.1.2 (R Core Team, 2023).
Wright’s FST statistic was used as a metric to quantify genetic differentiation at SNVs across populations (Martin et al., 2023; Phan et al., 2020) and within the Cuban population. Pairwise variant-specific FST values were calculated as
The rs35742686 (CYP2D6*3) and rs4986893 (CYP2C19*3) were not detected. Conversely, null variants in the glutathione S-transferase (GST) system, mu 1 (GSTM1*0) and theta 1 (GSTT1*0), were identified. The frequency of the GSTM1 null genotype was 0.39, while that of the GSTT1 null genotype was 0.18. The frequency of individuals with the concomitant deletion of GSTM1 and GSTT1 was 0.07. Genotype and allele frequencies of the remaining 39 SNVs are shown in Table 1.
All SNVs, except for six variants (CYP2C8 rs11572103, CYP3A4 rs2740574, CYP3A5 rs776746, VEGF rs3025039, XRCC3 rs861539, and MGMT rs1762429), were in HWE. Population heterozygosity exhibited a wide range (6.0%–51.0%) among Cubans. Heterozygous genotypes were the most common genotype for ABCB1 rs1045642, EZH1 rs7359598, and SNVs in MGMT. In general terms, alternative homozygous genotypes in xenobiotic biotransformation enzymes showed low frequency (≤10.0%), with only three SNVs exhibiting frequencies higher than 10.0% (CYP3A4 rs2242480, CYP3A5 rs776746, and ABCB1 rs1045642). Meanwhile, alternative homozygous genotypes were more frequent than the reference genotypes in CYP3A5 rs776746, MBD4 rs140695, MBD2 rs603097, MTHFR rs11121832, EZH2 rs2072408, and XRCC1 rs25487. Alternative genotypes were usually higher than 10.0% in SNVs from the MGMT gene, with exceptions of 4.0% for rs12259379 and 2.0% for rs12763287. Afterward, the level of genetic differentiation between Cubans and other related populations was explored (Figure 1) (extended information is presented in Supplementary Table 3).
Figure 1. Allele-specific FST values in pairwise comparisons between Cuba and populations related by ancestry or geographical area. (A) Targeted SNVs in xenobiotic biotransformation enzymes and transporters. (B) Targeted SNVs in DNA repair, growth factors, methyltransferase, and methyl-binding proteins. (C) Targeted SNVs in the MGMT gene. The dashed lines show the FST threshold value at 0.05 for moderate divergence and 0.15 for large divergence. Frequency data from Latin America and Africa (Yoruba) were collected from the 1000 Genome Project database. Colored dots highlight loci that displayed small, moderate, and large genetic differentiation in pairwise comparisons with the Cuban population.
Pairwise FST analysis revealed that Cubans display high genetic similarity to Spanish and Latin American populations (Figure 1). Average pairwise FST values were 0.014 (SD 0.021) in Cuba vs. Spain and 0.011 (SD 0.019) for Cuba vs. Latin America comparisons. FST values were less than 0.05 for the majority of SNVs, indicating minimal genetic differentiation between these populations. However, few markers exceeded the FST threshold of moderate genetic differentiation (FST = 0.05), specifically CYP3A4 and CYP3A5 in Cuba vs. Spain and CYP1A1 rs1048943 and MBD5 in Cuba vs. Latin America.
Pairwise analysis for Cuba vs. Africa showed moderate divergence (mean FST = 0.071, SD 0.079). Variants in CYP3A4, CYP3A5, and MGMT (rs1762429 and rs11016798) presented large divergence (FST > 0.15), while other 14 SNVs in epigenetic proteins (MBD2, MBD4, MBD5, and EZH1/2), metabolic enzymes (AHR, CYP2D6, CYP2C8, CYP2C9, and EPHX1), and transport (ABCB1) and DNA repair-related proteins (TP53 and MGMT) displayed moderate differentiation (Figure 1). Other variants with small differentiation nearly reached the moderate divergence threshold, namely, CYP1A1 (rs1048943, FST = 0.042), XRCC1 (FST = 0.042), GSTT1 null (FST = 0.044), CYP2C8 (rs11572103, FST = 0.042), and two SNVs in MGMT (rs12763287, FST = 0.044 and rs11016879, FST = 0.049).
The extent of admixture in the Cuban sample is illustrated in Figure 2 along with data from reference ancestral populations. There were clear differences in individual admixture estimates among these four population samples. The Cuban population was much more diverse in its ancestry proportions, and on average, individuals had large degrees of European (67.8%) and African ancestry (27.2%), while the contribution of Amerindian ancestry was small (5.0%). Average proportions of European ancestry decreased progressively among self-reported groups: White 85.9% (98.2–41.3), admixed 57.7% (95.5–13.6), and Black individuals 31.2% (73.6–5.4) (Kruskal–Wallis, KW: European, p < 0.001, followed by post hoc Dunn’s test). The opposite trend was observed in the African ancestry, which, respectively, averaged 9.3% (55.4–1.2), 36.3% (85.9–3.7), and 64.7% (94.1–18.7) in self-identified White, admixed, and Black individuals (KW: African, p < 0.001). Amerindian ancestry contribution was small across the three groups (KW, p = 0.004). Post hoc analysis indicated that Amerindian ancestry in admixed individuals (6.0%) was significantly different from that in self-identified White (4.8%) or Black individuals (4.1%).
Figure 2. Estimated population structure using a genetic model-based clustering algorithm (STRUCTURE program). Each individual is represented by a vertical line, which is partitioned into colored segments that represent the individual’s estimated membership fractions in each cluster. Individuals can have membership in multiple clusters, with membership coefficients summing up to 1 in each cluster. Populations are labeled below each panel. The clusters are in different colors: blue for Europeans (EUR, Iberians), orange for Amerindians (AMR, Native Americans), and purple for Africans (AFR). Population data were collected from the databases HGDP-CEPH and 1000 Genome Project (n = 993): Cubans (n = 357), White Cubans (n = 190), Admixed Cubans (n = 101), and Black Cubans (n = 66).
The ancestry composition by place of birth showed distinctive geographic trends in admixture proportions. The Western and Central regions had higher European ancestry (70.4% and 83.3%, respectively) than the Eastern region (58.9%) (KW, p < 0.001). For African ancestry, the Eastern region showed larger proportions (34.1%), followed by the Western (26.9%) and the Central regions (8.9%), with significant differences among groups (KW, p < 0.001). Similar proportions of the Amerindian component were found in Central and Eastern regions (7.7% and 7.0%, respectively), but these were significantly different from the Western region (2.7%, KW, p < 0.001).
Heterogeneous admixture levels were observed in individuals when skin color and place of birth were taken into consideration. In order to evaluate whether these variables could be useful to expose the distinctive distribution of the SNVs, we compared the genotype and allele frequencies and characterized the genetic differentiation using the FST statistic among subgroups. No significant differences were detected in allele frequencies across skin color subgroups or birthplace. However, genotype frequency significantly differed (Fisher exact test, p < 0.05) at 24 loci among skin color subgroups (Figure 3) and 11 loci among birthplaces. Extended information on the minor allele frequency (MAF) of the SNVs in this study compared to other populations is presented in Supplementary Table 4.bj
Figure 3. Distribution of 24 SNV genotype frequencies significantly different in the stratification analysis by individuals’ skin color (Fisher exact test), ref: reference homozygous genotype, het: heterozygous genotype, and alt: alternative homozygous genotype (N = 357, white = 190, admixed = 101, and black = 66).
It can be noted that self-identified admixed individuals showed intermediate frequencies of the alternative genotypes compared to White/Black individuals. Admixed resembled Black individuals in the frequencies of five SNVs: CYP3A4 rs2740574, EPHX1, MBD2, MBD4, and MGMT rs11016885, and they resembled White subjects in the frequencies of just two SNVs: XRCC1 and MGMT rs12259379. Comparable alternative genotype frequencies between groups were observed for five SNVs: CYP2D6 rs28371706, CYP2C9, CYP2C8 rs11572103, rs10509681, and CYP1A1 (Figure 3).
On the other hand, the frequencies of alternative genotypes were generally higher, either in the Central or Eastern regions, whereas the Western region showed intermediate frequencies. Particularly, alternative genotypes of the SNVs in MGMT rs1762429, rs11016798, XRCC3, TP53, and CYP3A5 were predominant in the Central region, whereas SNVs in EZH1, MBD4, and CYP3A4 rs2242480 had higher frequencies in the Eastern region. Only, the EGFR SNV showed the highest frequency in Western provinces (0.19), followed the Central provinces (0.08), and was not detected in the Eastern region.
Genetic heterogeneity within the Cuban sample was explored using the FST statistic. Allele-specific pairwise FST analysis was conducted among skin color categories and geographical place of birth (Figure 4; Supplementary Table 3). Low genetic divergence was found when comparing self-identified admixed vs. Black and admixed vs. White individuals, with mean FST values of 0.013 (SD 0.013) and 0.015 (SD 0.022), respectively. The mean FST value of 0.045 (SD 0.051) indicated greater differentiation between self-identified White vs. Black individuals (Figure 4A). The variants in CYP3A4 and CYP3A5 genes showed moderate differentiation in White vs. admixed individuals but large differentiation in White vs. Black Cubans. Only MGMT rs1762429 (FST = 0.052) and CYP3A5 (FST = 0.044) approached the FST threshold value for moderate genetic divergence in admixed vs. Black individuals. These results agreed with the admixture proportions (according to skin color) reported here. Variants in AHR, TP53, EZH1, and CYP2D6 rs28371706 and four variants in MGMT displayed moderate divergence in White vs. Black Cubans, while MBD4 (FST = 0.044), CYP2C8 rs11572103 (FST = 0.049), and XRCC3 (FST = 0.046) approached the moderate threshold.
Figure 4. Allele-specific FST values for 41 variants in pairwise comparisons. (A) Stratified by skin color of individuals. (B) Stratified by region of origin. The dashed lines show the FST threshold at 0.05 for moderate divergence and 0.15 for large divergence. Color points specify SNVs with small, moderate, and large divergence in pairwise comparisons. N = 357, self-reported White n = 190, admixed n = 101, Black n = 66, Western n = 174, Central n = 49, and Eastern n = 134.
Low genetic divergence was found between the Central vs. Eastern (FST = 0.017; SD = 0.019), Western vs. Central (FST = 0.010; SD = 0.011), and Western vs. Eastern (FST = 0.005; SD = 0.011) geographical regions (Figure 4B). Three SNVs, namely, CYP3A4 rs2242480, rs2740574, and CYP3A5 rs776746, exhibited moderate differentiation when Center vs. Eastern were compared and in CYP1A1 rs1048943 for Western vs. Eastern regions.
Other SNVs were close to the moderate threshold: in MBD4 (FST = 0.049) and two variants in MGMT when the Central vs. Eastern regions were compared. Meanwhile, TP53 (FST = 0.043) in Western vs. Central and EGFR (FST = 0.044) in Western vs. Eastern regions were also close to the moderate threshold. In short, 12 SNVs surpassed the moderate threshold FST value when White vs. Black individuals were compared, while low divergence was observed in the rest of the comparisons.
In this section, we studied the frequencies of 43 variants in genes affecting drug metabolism, transport, and pharmacological efficacy in healthy Cuban volunteers. Five out of the six SNVs that showed HWE deviations (Table 1) displayed statistical differences by skin color and four by birthplace. Except for VEGF rs3025039, the markers presented moderate-to-large genetic differentiation in pairwise comparisons within Cubans and with other populations. Hence, population stratification seems to be the most plausible explanation for the observed HWE deviations.
Ancestry analyses in this study revealed admixture diversity among individuals and within group of individuals from the same birthplace and skin color. In general, admixture estimations detected here ranged closely to a previous report on Cubans (Fortes-Lima et al., 2018). An element derived from the population structure described may have pharmacogenetic implications. The European and African components together account for 95% of the genetic diversity in this sample, as compared to the 5% for Amerindian ancestry. Consequently, European and African ancestry will have considerably greater impact on the frequency distribution of variants with pharmacological relevance.
There is a strong relationship between admixture proportions and census categories in the Cuban population, as suggested by Marcheco-Teruel et al. (2014). In agreement with these data, the estimations of European ancestry detected here decreased from self-reported White to Black individuals, and the opposite trend was observed in regard to the African ancestry, while admixed individuals displayed intermediate values for both ancestry components. By place of birth, European ancestry was predominant in the Western and Central regions, while the Eastern region had the highest African contribution, followed by the Western and Central regions.
Genetic differentiation, as described by FST pairwise comparisons within the Cuban population, suggested that drug responses may vary individually by skin color categories and regions of the country, with admixture proportions being a key factor. In this line, geographic differences observed in 11 genotype frequencies could also be associated, at least in part, with admixture patterns geographically structured across the island. Estimates of African and European ancestry by birthplace were comparable to reported proportions (Fortes-Lima et al., 2018). The central region showed a genotype distribution similar to that of the Spanish population, while individuals from the Eastern and Western provinces showed intermediate frequencies relative to the parental populations. Eastern provinces had relatively higher African contributions (average 34%) than Western and Central provinces. Data were readily extended to the greater genetic differentiation found in pairwise FST comparisons of the Central vs. Eastern regions, showing six markers with moderate divergence.
FST pairwise comparisons with other populations indicated genetic similarity to Spanish and Latin American populations. However, interethnic variability was observed. CYP1A1 rs1048943 (CYP1A1*2C) is greatly represented in Latin American populations (Amerindian ancestry), whereas in Africans and Europeans, its frequency is low (Pérez-Morales et al., 2008). Given the small Amerindian component estimated in Cubans, moderate divergence was expected in comparison to Latin American populations (Figure 1). The global allele distribution of rs776746 (CYP3A5*3) increased from 18% to 94% in African to European populations (Fairley et al., 2019), respectively. On average, the frequency of CYP3A5*3 is approximately 80% in Latin Americans, but it was found to be lower in this study (69%), compared to other reports on Chileans (76%) (Roco et al., 2012), Brazilians (73%) (Suarez-Kurtz et al., 2014), and admixed Mexicans (74%) (Fricke-Galindo et al., 2016). On the contrary, rs2740574 (CYP3A4*1B) is found in African populations due to a suggested selection factor against non-African populations involving vitamin D metabolism (Schirmer et al., 2006). The CYP3A4*1B frequency in Cubans was higher than in Ecuadorians (Sinués et al., 2008), admixed Mexicans (Gonzalez-Covarrubias et al., 2019), and Chileans (Roco et al., 2012), but similar to that in Brazilians (Rodrigues-Soares et al., 2018).
Previous studies have reported about CYP allele frequencies in Cubans (Llerena et al., 2014; Rodrigues-Soares et al., 2020). In individuals with European ancestry, rs35742686 (CYP2D6*3) is found at a low frequency (Zhou et al., 2017), as well in Latin Americans (Naranjo et al., 2018), and it is virtually absent in Chileans (Roco et al., 2012). In agreement with these data, CYP2D6*3 was not detected in the present sample cohort. CYP2D6*10 is related to Asian ancestry but is also distinctive of African populations together with CYP2D6*17. However, a higher CYP2D6 *10 allele frequency was reported in this study (18%) and Brazilians (Suarez-Kurtz et al., 2012a). The six variant alleles studied in the CYP2C subfamily ranged closely to reports on Brazilians (Suarez-Kurtz et al., 2012b) and CYP2C9*2 and *3 to Venezuelans (Flores-Gutiérrez et al., 2017). CYP2C19*3 is less frequent than CYP2C19*2 worldwide and has its highest frequency in Asians (Zhou et al., 2017). It is almost non-existent in Latin America (Suarez-Kurtz et al., 2012b), it is not present in Chileans (Roco et al., 2012), and it was also not detected in this Cuban cohort. One of the highest frequencies of CYP2C19*2 and *3 is found in Venezuela, associated with their Amerindian component (Castro de Guerra et al., 2013).
Frequency estimates of EPHX1 rs2234922 and AHR rs2066853 are 8% and 15% in Mexicans (Pérez-Morales et al., 2011), respectively, and 8% for AHR rs2066853 in Brazilians (Abnet et al., 2007), whereas we observed lower frequencies for both SNVs. The estimated frequency of rs1045642 (ABCB1) in this study (37%, A allele) was intermediate to the frequencies reported for Spaniards (48%) and African Americans (16%) but similar to those observed in Brazilians (39%) (Scheiner et al., 2010) and in a previous Cuban study (36.5%), with comparable demographical variables (Rodeiro et al., 2022).
The frequency of the GSTT1 null genotype in Cubans was closer to that observed in Spanish than to Africans (Kasthurinaidu et al., 2015), with an FST value in Cuba vs. Africa comparison near the moderate differentiation threshold (FST = 0.045). Results are consistent with those of other studies stating that the GSTM1 null genotype is more frequent than GSTT1 worldwide (Nakanishi et al., 2022). The prevalence of both deletions was similar to admixed Mexicans (Palma-Cano et al., 2017) and Chileans (Roco et al., 2012) but lower than in Brazilians (Magno et al., 2009) and Colombians (Ramírez et al., 2019).
Variants and genotypes (germline DNA) do not depend on disease status or evolution, in contrast to somatic mutations; thus, its clinical use can be considered with strong confidence as predictive biomarkers. Several SNVs in genes related to DNA repair processes and growth factors have clinical annotations on PharmGKB with different levels of evidence (Whirl-Carrillo et al., 2021). Therefore, validating the usefulness of the recommended markers would promote a safe and cost-effective use of drugs in the clinical management of patients.
To illustrate this statement, 23% of the global population carries the EGFR rs712829 T allele. Similarly, the frequency for Hispanics is 21%, with notable ethnic variations. This variant is less common in Peruvians, but reaches 28% in Puerto Ricans (Fairley et al., 2019) and 23% in Mexicans (Torres-Jasso et al., 2015). A higher frequency was observed in Cubans (32%), closer to the frequencies found in Europeans and Iberians. Regarding VEGF rs3025039, Hispanics have the highest frequency for the T variant allele, particularly among Mexicans and Peruvians. However, these variants were less common in the Cuban sample, and a low frequency is also observed in Iberians, Colombians (Fairley et al., 2019), and Brazilians (Carvalho et al., 2021). This is important in the context of cancer susceptibility, side effects, and response rate to EGFR and VEGF-targeting drugs. The variant allele of EGFR correlates with increased receptor expression (Heist and Christiani, 2009), whereas the VEGF variant is associated with significantly lower VEGF plasma levels (Sibertin-Blanc et al., 2015), which would make carriers suitable for EGFR-target therapies and less appropriate for VEGF-target therapies. Meanwhile, moderate differentiation to Africans was observed for rs1042522 (TP53), which had a 63% prevalence in Cubans. Studies in Latin America agree on the predominance of the TP53 Arg72 allele (Rodrigues-Soares et al., 2018; Olloquequi et al., 2022). Few variants in DNA repair enzymes have been characterized in Latin American populations. The frequency of rs13181 (ERCC2), rs861539 (XRCC3), and rs25487 (XRCC1) in Cubans resembled those of Mexicans (Pérez-Morales et al., 2011) and Brazilians (Duarte et al., 2005).
Attention has been drawn to variants in methylation enzymes or methyl recognition binding proteins (Majchrzak-Celińska et al., 2019). SNVs in epigenetic writer–reader–eraser enzymes had noticeable differences in prevalence among Africans, Europeans (Iberians), and Latin Americans. Hispanics have the highest frequency of MBD5 rs16828708 (Fairley et al., 2019). Its frequency in Cubans matched that of the Spanish, displaying moderate divergence from Latin Americans (Figure 1). Allele variants in MBD2/4 and EZH1/2 enzymes showed moderate differentiation in FST pairwise comparison to Africa. According to the 1000 Genomes Project database, the frequencies observed here are closer to Spaniards than to Africans. The MGMT enzyme is of special interest; its expression is ubiquitous in normal cells, with considerable variation in the activity in the same tissue of different individuals, and it is frequently overexpressed in tumors vs. non-neoplastic tissues (Christmann et al., 2011). Genome-wide association studies have revealed that non-coding SNVs of MGMT affect gene expression (Huang et al., 2018). The FST values indicated low divergence from Spain and Latin America, but several markers showed large-to-moderate genetic differentiation to Africans. The FST statistic provides an approach to estimating the genetic divergence of pharmacogenetic variants and its potential impact on clinical response to drugs in different populations or strata within a population (Suarez-Kurtz and de Araújo, 2022). It also supports that the distribution of genetic variants among Cubans is influenced by the large variability in ancestry proportions.
The cost-effectiveness of pharmacogenetic testing has been demonstrated, supporting its integration into clinical practice (Morris et al., 2022; Plumpton et al., 2019). The pharmacogenes described here have been widely studied for their usefulness in predicting and tailoring efficient therapeutic responses (Zhao et al., 2021) and have clinical annotations on the PharmGKB Knowledge Base (Altman, 2007), with variable levels of evidence for current clinical guidelines. The prevalence of variants in these pharmacogenes in the Cuban population justifies the implementation of pharmacogenetic tools. While the use of AIM panels to infer ancestry in pharmacogenetic studies is recommended, the use of descriptive variables like skin color categories and region of birth may also be informative in different contexts. For instance, it may explain how admixture diversity may be translated into different prevalence of genetic variants among skin color categories and country regions. This information should be taken into account when designing clinical trials and public health policies related to pharmacogenetic testing. As an illustrative example, important pharmacogenetic variants, such as CYP3A4*1B and CYP3A5*3, related to immunosuppressive drug responses, were found to have high divergence between self-identified White/Black individuals and between Central and Eastern provinces. Among the human CYP3A enzymes, CYP3A4 and CYP3A5 are considered the most important in drug metabolism. Approximately half of the medications that are metabolized by P450 are CYP3A substrates (Saiz-Rodríguez et al., 2020). Thus, the present results provided evidence for its applications in public health priorities across the island. A study limitation is that recruitment was most successful in Western provinces, mainly in Havana. Future comprehensive studies should promote the participation of individuals from all provinces in the Central and Eastern regions.
In summary, this data was a contribution to pharmacogenetic characterization of Cuban population and to the development of precision medicine in the country.
The datasets presented in this article are not readily available because the nature of this research contains information that could compromise the participants’ privacy, and they did not agree to share their data publicly. Requests to access the datasets should be directed to the corresponding author IR-G.
The studies involving humans were approved by the Institutional Review Board and Ethics Committee of Institute of Oncology and Radiobiology, Havana, Cuba. The studies were conducted in accordance with the local legislation and institutional requirements. The participants provided their written informed consent to participate in this study.
ER-R: conceptualization, data curation, visualization, writing–original draft, and writing–review and editing. JH-I: conceptualization, funding acquisition, visualization, and writing–review and editing. EC-L: investigation, supervision, and writing–review and editing. ZS: supervision and writing–review and editing. DV: supervision and writing–review and editing. CP-N: data curation, investigation, and writing–review and editing. GF-P: data curation, investigation, and writing–review and editing. IG-P: data curation, investigation, and writing–review and editing. MF-P: supervision and writing–review and editing. WV-B: conceptualization, funding acquisition, project administration, supervision, and writing–review and editing. IR-G: conceptualization, funding acquisition, project administration, supervision, and writing–review and editing.
The author(s) declare that financial support was received for the research, authorship, and/or publication of this article. This research was funded by Project: VLIR TEAM CU2022TEA501A102 “Building in vitro plant biotechnology capacities for ecological sustainable production of marine phytochemical formulations against skin-cancer in Cuba” and the Project UNPD/GEF 11037 “Developing the potential of Thalassia testudinum in the health sector in Cuba in accordance with the Nagoya Protocol and Biodiversity Conservation.” VLIR TEAM ZEIN2016PR420–75155 Implementation of personalized medicine and pharmaco(epi)genetic biomarkers for cost effective improvement of the therapeutic outcome of lung cancer treatment in Cuba.
The authors thank the volunteers, administrators, and staff members from the recruitment sites for contributing with their time and expertise to our research and sharing their experiences with us.
The authors declare that the research was conducted in the absence of any commercial or financial relationships that could be construed as a potential conflict of interest.
The author(s) declared that they were an editorial board member of Frontiers, at the time of submission. This had no impact on the peer review process and the final decision.
All claims expressed in this article are solely those of the authors and do not necessarily represent those of their affiliated organizations, or those of the publisher, the editors, and the reviewers. Any product that may be evaluated in this article, or claim that may be made by its manufacturer, is not guaranteed or endorsed by the publisher.
The Supplementary Material for this article can be found online at: https://www.frontiersin.org/articles/10.3389/fphar.2024.1467036/full#supplementary-material
Abnet, C. C., Fagundes, R. B., Strickland, P. T., Kamangar, F., Roth, M. J., Taylor, P. R., et al. (2007). The influence of genetic polymorphisms in Ahr, CYP1A1, CYP1A2, CYP1B1, GST M1, GST T1 and UGT1A1 on urine 1-hydroxypyrene glucuronide concentrations in healthy subjects from Rio Grande do Sul, Brazil. Carcinogenesis 28, 112–117. doi:10.1093/carcin/bgl131
Altman, R. B. (2007). PharmGKB: a logical home for knowledge relating genotype to drug response phenotype. Nat. Genet. 39, 426. doi:10.1038/ng0407-426
Amigo, J., Phillips, C., Lareu, M., and Carracedo, Á. (2008). The SNP for ID browser: an online tool for query and display of frequency data from the SNP for ID project. Int. J. Leg. Med. 122, 435–440. doi:10.1007/s00414-008-0233-7
Bergström, A., McCarthy, S. A., Hui, R., Almarri, M. A., Ayub, Q., Danecek, P., et al. (2020). Insights into human genetic variation and population history from 929 diverse genomes. Science. 367, eaay5012. doi:10.1126/science.aay5012
Bonifaz-Pena, V., Contreras, A. V., Struchiner, C. J., Roela, R. A., Furuya-Mazzotti, T. K., Chammas, R., et al. (2014). Exploring the distribution of genetic markers of pharmacogenomics relevance in Brazilian and Mexican populations. PloS one 9, e112640. doi:10.1371/journal.pone.0112640
Camacho, H., Nazabal, M., Domínguez, M., Mendoza, R., Leal, L., Roca, J., et al. (2011). Análisis de asociación de polimorfismos del gen COMT y la esquizofrenia en una muestra familiar cubana. Result. Prelim. Biotecnol. Apl. 28, 97–105.
Carvalho, S. C., Vasconcelos, L. R., Fonseca, Ld, Carmo, R. F., Tomitão, M. T., Aroucha, D. C., et al. (2021). Methylene tetrahydrofolate reductase (MTHFR) and vascular endothelial growth factor (VEGF) polymorphisms in Brazilian patients with Hepatitis C virus (HCV)-related hepatocellular carcinoma (HCC). Clinics 76, e2881. doi:10.6061/clinics/2021/e2881
Castro de Guerra, D., Flores, S., and Izaguirre, M. H. (2013). Distribution of CYP2C19* 2 and CYP2C19* 3 polymorphisms in Venezuelan populations with different admixture. Ann. Hum. Biol. 40, 197–200. doi:10.3109/03014460.2012.749946
Céspedes-Garro, C., Naranjo, M.-E. G., Rodrigues-Soares, F., LLerena, A., Duconge, J., Montané-Jaime, L. K., et al. (2016). Pharmacogenetic research activity in Central America and the Caribbean: a systematic review. Pharmacogenomics 17, 1707–1724. doi:10.2217/pgs-2016-0053
Chen, J., Teo, Y. Y., Toh, D. S., and Sung, C. (2010). Interethnic comparisons of important pharmacology genes using SNP databases: potential application to drug regulatory assessments. Pharmacogenomics 11, 1077–1094. doi:10.2217/pgs.10.79
Christmann, M., Verbeek, B., Roos, W. P., and Kaina, B. (2011). O6-Methylguanine-DNA methyltransferase (MGMT) in normal tissues and tumors: enzyme activity, promoter methylation and immunohistochemistry. Biochimica Biophysica Acta 1816, 179–190. doi:10.1016/j.bbcan.2011.06.002
Cintado, A., Companioni, O., Nazabal, M., Camacho, H., Ferrer, A., De Cossio, M. F., et al. (2009). Admixture estimates for the population of Havana City. Ann. Hum. Biol. 36, 350–360. doi:10.1080/03014460902817984
Diaz-Horta, O., Cintado, A., Fernandez-De-Cossio, M. E., Nazabal, M., Ferrer, A., Roca, J., et al. (2010). Relationship of type 1 diabetes to ancestral proportions and HLA DR/DQ alleles in a sample of the admixed Cuban population. Ann. Hum. Biol. 37, 778–788. doi:10.3109/03014461003766984
Duarte, M. C., Colombo, J., Rossit, A. R. B., and Silva, A. E. (2005). Polymorphisms of the DNA repair genes XRCC1 and XRCC3 in a Brazilian population. Genet. Mol. Biol. 28, 397–401. doi:10.1590/S1415-47572005000300011
Fairley, S., Lowy-Gallego, E., Perry, E., and Flicek, P. (2019). The International Genome Sample Resource (IGSR) collection of open human genomic variation resources. Nucleic Acids Res. 48, D941–D7. doi:10.1093/nar/gkz836
Fedorova, L., Khrunin, A., Khvorykh, G., Lim, J., Thornton, N., Mulyar, O. A., et al. (2022). Analysis of common SNPs across continents reveals major genomic differences between human populations. Genes 13, 1472. doi:10.3390/genes13081472
Flores-Gutiérrez, S., Rodríguez-Larralde, Á., Vivenes de Lugo, M., and Castro de Guerra, D. (2017). Distribution of polymorphisms in the CYP2C9 gene and CYP2C19/CYP2C9 haplotypes among Venezuelan populations. Ann. Hum. Biol. 44, 191–198. doi:10.1080/03014460.2016.1192218
Fortes-Lima, C., Bybjerg-Grauholm, J., Marin-Padrón, L. C., Gomez-Cabezas, E. J., Bækvad-Hansen, M., Hansen, C. S., et al. (2018). Exploring Cuba’s population structure and demographic history using genome-wide data. Sci. Rep. 8, 11422. doi:10.1038/s41598-018-29851-3
Freeman, G., and Halton, J. H. (1951). Note on an exact treatment of contingency, goodness of fit and other problems of significance. Biometrika 38, 141–149. doi:10.2307/2332323
Fricke-Galindo, I., Ortega-Vázquez, A., Monroy-Jaramillo, N., Dorado, P., Jung-Cook, H., Peñas-Lledó, E., et al. (2016). Allele and genotype frequencies of genes relevant to anti-epileptic drug therapy in Mexican-Mestizo healthy volunteers. Pharmacogenomics 17, 1913–1930. doi:10.2217/pgs-2016-0078
García, G., Sierra, B., Pérez, A. B., Aguirre, E., Rosado, I., Gonzalez, N., et al. (2010). Asymptomatic dengue infection in a Cuban population confirms the protective role of the RR variant of the FcgammaRIIa polymorphism. Am. J. Trop. Med. Hyg. 82, 1153–1156. doi:10.4269/ajtmh.2010.09-0353
Gonzalez-Covarrubias, V., Morales-Franco, M., Cruz-Correa, O. F., Martínez-Hernández, A., García-Ortíz, H., Barajas-Olmos, F., et al. (2019). Variation in actionable pharmacogenetic markers in natives and mestizos from Mexico. Front. Pharmacol. 10, 1169. doi:10.3389/fphar.2019.01169
Guanche, J. (2020) “Componentes étnicos de la nación cubana,” in República Popular China: Universidad de Estudios Internacionales de Hebei. 4th ed, 255.
Heist, R. S., and Christiani, D. (2009). EGFR-targeted therapies in lung cancer: predictors of response and toxicity. pharmacogenomics 10, 59–68. doi:10.2217/14622416.10.1.59
Huang, L., Xu, W., Dai, L., Yan, D., Zhang, S., and Shi, X. (2018). An intronic genetic variation of MGMT affects enhancer activity and is associated with glioma susceptibility. Cancer Manag. Res. 10, 3995–4003. doi:10.2147/CMAR.S176622
Kasthurinaidu, S. P., Ramasamy, T., Ayyavoo, J., Dave, D. K., and Adroja, D. A. (2015). GST M1-T1 null allele frequency patterns in geographically assorted human populations: a phylogenetic approach. PLOS one 10, e0118660. doi:10.1371/journal.pone.0118660
Kopelman, N. M., Mayzel, J., Jakobsson, M., Rosenberg, N. A., and Mayrose, I. (2015). Clumpak: a program for identifying clustering modes and packaging population structure inferences across K. Mol. Ecol. Resour. 15, 1179–1191. doi:10.1111/1755-0998.12387
Llerena, A., Alvarez, M., Dorado, P., González, I., Peñas-LLedó, E., Pérez, B., et al. (2014). Interethnic differences in the relevance of CYP2C9 genotype and environmental factors for diclofenac metabolism in Hispanics from Cuba and Spain. pharmacogenomics J. 14, 229–234. doi:10.1038/tpj.2013.28
Llerena, A., Dorado, P., Ramírez, R., González, I., Alvarez, M., Peñas-Lledó, E., et al. (2012). CYP2D6 genotype and debrisoquine hydroxylation phenotype in Cubans and Nicaraguans. pharmacogenomics J. 12, 176–183. doi:10.1038/tpj.2010.85
Magno, L. A. V., Talbot, J., Talbot, T., Borges Santos, A. M., Souza, R. P., Marin, L. J., et al. (2009). Glutathione s-transferase variants in a brazilian population. Pharmacology 83, 231–236. doi:10.1159/000205823
Majchrzak-Celińska, A., and Baer-Dubowska, W. (2019). “Pharmacoepigenetics: basic principles for personalized medicine,” in Traslational epigenetics pharmacoepigenetics. Editor R. Cacabelos (Academic Press), 10, 101–112. doi:10.1016/B978-0-12-813939-4.00002-4
Marcheco-Teruel, B., Parra, E. J., Fuentes-Smith, E., Salas, A., Buttenschøn, H. N., Demontis, D., et al. (2014). Cuba: exploring the history of admixture and the genetic basis of pigmentation using autosomal and uniparental markers. PLoS Genet. 10, e1004488. doi:10.1371/journal.pgen.1004488
Martin, F. J., Amode, M. R., Aneja, A., Austine-Orimoloye, O., Azov Andrey, G., Barnes, I., et al. (2023). Ensembl 2023. Nucleic Acids Res. 51, D933–D941. doi:10.1093/nar/gkac958
Morris, S. A., Alsaidi, A. T., Verbyla, A., Cruz, A., Macfarlane, C., Bauer, J., et al. (2022). Cost effectiveness of pharmacogenetic testing for drugs with Clinical Pharmacogenetics Implementation Consortium (CPIC) guidelines: a systematic review. Clin. Pharmacol. and Ther. 112, 1318–1328. doi:10.1002/cpt.2754
Nakanishi, G., Pita-Oliveira, M., Bertagnolli, L. S., Torres-Loureiro, S., Scudeler, M. M., Cirino, H. S., et al. (2022). Worldwide systematic review of GSTM1 and GSTT1 null genotypes by continent, ethnicity, and therapeutic area. OMICS A J. Integr. Biol. 26, 528–541. doi:10.1089/omi.2022.0090
Naranjo, M.-E. G., Rodrigues-Soares, F., Penas-Lledo, E. M., Tarazona-Santos, E., Farinas, H., Rodeiro, I., et al. (2018). Interethnic variability in CYP2D6, CYP2C9, and CYP2C19 genes and predicted drug metabolism phenotypes among 6060 Ibero-and native Americans: RIBEF-CEIBA consortium report on population pharmacogenomics. Omics a J. Integr. Biol. 22, 575–588. doi:10.1089/omi.2018.0114
Oficina Nacional de Estadísticas e Información. Centro de Estudios de Población y Desarrollo (2016). El Color de la Piel según el Censo de Población y Viviendas 2012. La Habana 1, 1–418.
Olloquequi, J., Castro-Santos, P., and Díaz-Peña, R. (2022). Pharmacogenetic variation and its clinical relevance in a Latin American rural population. Int. J. Mol. Sci. 23, 11758. doi:10.3390/ijms231911758
Palma-Cano, L. E., Córdova, E. J., Orozco, L., Martínez-Hernández, A., Cid, M., Leal-Berumen, I., et al. (2017). GSTT1 and GSTM1 null variants in Mestizo and Amerindian populations from northwestern Mexico and a literature review. Genet. Mol. Biol. 40, 727–735. doi:10.1590/1678-4685-GMB-2016-0142
Pérez-Morales, R., Castro-Hernández, C., Gonsebatt, M. E., and Rubio, J. (2008). Polymorphism of CYP1A1* 2C, GSTM1* 0, and GSTT1* 0 in a Mexican Mestizo population: a similitude analysis. Hum. Biol. 80, 457–465. doi:10.3378/1534-6617-80.4.457
Pérez-Morales, R., Méndez-Ramírez, I., Castro-Hernández, C., Martínez-Ramírez, O. C., Gonsebatt, M. E., and Rubio, J. (2011). Polymorphisms associated with the risk of lung cancer in a healthy Mexican Mestizo population: application of the additive model for cancer. Genet. Mol. Biol. 34, 546–552. doi:10.1590/S1415-47572011005000053
Phan, L., Jin, Y., Zhang, H., Qiang, W., Shekhtman, E., Shao, D., et al. (2020). ALFA: allele frequency aggregator. Bethesda, Maryland: National Center for Biotechnology Information, and US National Library of Medicine.
Phillips, C., Fondevila, M., and Lareau, M. V. (2012). A 34-plex autosomal SNP single base extension assay for ancestry investigations. Methods Mol. Biol. 830, 109–126. doi:10.1007/978-1-61779-461-2_8
Plumpton, C. O., Pirmohamed, M., and Hughes, D. A. (2019). Cost-effectiveness of panel tests for multiple pharmacogenes associated with adverse drug reactions: an evaluation framework. Clin. Pharmacol. and Ther. 105, 1429–1438. doi:10.1002/cpt.1312
Pritchard, J. K., Stephens, M., and Donnelly, P. (2000). Inference of population structure using multilocus genotype data. Genetics 155, 945–959. doi:10.1093/genetics/155.2.945
Ramírez, B., Niño-Orrego, M. J., Cárdenas, D., Ariza, K. E., Quintero, K., Contreras Bravo, N. C., et al. (2019). Copy number variation profiling in pharmacogenetics CYP-450 and GST genes in Colombian population. BMC Med. genomics 12, 110–119. doi:10.1186/s12920-019-0556-x
R Core Team (2023). R: a language and environment for statistical computing. Vienna, Austria: R Foundation for Statistical Computing.
Robert, F., and Pelletier, J. (2018). Exploring the impact of single-nucleotide polymorphisms on translation. Front. Genet. 9, 507. doi:10.3389/fgene.2018.00507
Roco, Á., Quiñones, L., Agúndez, J. A., García-Martín, E., Squicciarini, V., Miranda, C., et al. (2012). Frequencies of 23 functionally significant variant alleles related with metabolism of antineoplastic drugs in the Chilean population: comparison with Caucasian and Asian populations. Front. Genet. 3, 229. doi:10.3389/fgene.2012.00229
Rodeiro, I., Herrea, J., Cuétara, E., Garrido, G., Reyes, E., Martínez, I., et al. (2022). Prevalence of ABCB1 3435C> T polymorphism in the Cuban population. Drug Metabolism Personalized Ther. 37, 141–148. doi:10.1515/dmpt-2020-0156
Rodrigues-Soares, F., Kehdy, F. S., Sampaio-Coelho, J., Andrade, P. X., Céspedes-Garro, C., Zolini, C., et al. (2018). Genetic structure of pharmacogenetic biomarkers in Brazil inferred from a systematic review and population-based cohorts: a RIBEF/EPIGEN-Brazil initiative. Pharmacogenomics J. 18, 749–759. doi:10.1038/s41397-018-0015-7
Rodrigues-Soares, F., Peñas-Lledó, E. M., Tarazona-Santos, E., Sosa-Macías, M., Terán, E., López-López, M., et al. (2020). Genomic ancestry, CYP 2D6, CYP 2C9, and CYP 2C19 among Latin Americans. Clin. Pharmacol. and Ther. 107, 257–268. doi:10.1002/cpt.1598
Saiz-Rodríguez, M., Almenara, S., Navares-Gómez, M., Ochoa, D., Román, M., Zubiaur, P., et al. (2020). Effect of the most relevant CYP3A4 and CYP3A5 polymorphisms on the pharmacokinetic parameters of 10 CYP3A substrates. Biomedicines 8, 94. doi:10.3390/biomedicines8040094
Scheiner, M. A. M., Damasceno, A. M., and Maia, R. C. (2010). ABCB1 single nucleotide polymorphisms in the Brazilian population. Mol. Biol. Rep. 37, 111–118. doi:10.1007/s11033-009-9547-x
Schirmer, M., Toliat, M. R., Haberl, M., Suk, A., Kamdem, L. K., Klein, K., et al. (2006). Genetic signature consistent with selection against the CYP3A4* 1B allele in non-African populations. Pharmacogenetics genomics 16, 59–71. doi:10.1097/01.fpc.0000182779.03180.ba
Sibertin-Blanc, C., Mancini, J., Fabre, A., Lagarde, A., Del Grande, J., Levy, N., et al. (2015). Vascular Endothelial Growth Factor A c.* 237C> T polymorphism is associated with bevacizumab efficacy and related hypertension in metastatic colorectal cancer. Dig. Liver Dis. 47, 331–337. doi:10.1016/j.dld.2014.12.013
Sinués, B., Vicente, J., Fanlo, A., Mayayo-Sinués, E., González-Andrade, F., Sánchez-Q, D., et al. (2008). CYP3A5* 3, CYP3A4* 1B and MDR1 C3435T genotype distributions in Ecuadorians. Dis. markers 24, 325–331. doi:10.1155/2008/750804
Sotomayor-Lugo, F., Alemañy-Díaz Perera, C., Roblejo-Balbuena, H., Zúñiga-Rosales, Y., Monzón-Benítez, G., Suárez-Besil, B., et al. (2022). The role of tumor necrosis factor alpha− 308A> G polymorphism on the clinical states of SARS-CoV-2 infection. Egypt. J. Med. Hum. Genet., 23. doi:10.1186/s43042-022-00274-0
Suarez-Kurtz, G., and de Araújo, G. S. (2022). Pharmacogenetic differentiation across Latin America. Pharmacogenomics 23, 225–233. doi:10.2217/pgs-2021-0152
Suarez-Kurtz, G., Genro, J., De Moraes, M., Ojopi, E., Pena, S., Perini, J., et al. (2012b). Global pharmacogenomics: impact of population diversity on the distribution of polymorphisms in the CYP2C cluster among Brazilians. pharmacogenomics J. 12, 267–276. doi:10.1038/tpj.2010.89
Suarez-Kurtz, G., Pena, S. D. J., Struchiner, C. J., and Hutz, M. H. (2012a). Pharmacogenomic diversity among Brazilians: influence of ancestry, self-reported color, and geographical origin. Front. Pharmacol. 3, 191. doi:10.3389/fphar.2012.00191
Suarez-Kurtz, G., Vargens, D. D., Santoro, A. B., Hutz, M. H., de Moraes, M. E., Pena, S. D., et al. (2014). Global pharmacogenomics: distribution of CYP3A5 polymorphisms and phenotypes in the Brazilian population. PloS one 9, e83472. doi:10.1371/journal.pone.0083472
Torres-Jasso, J., Marín, M., Santiago-Luna, E., Leoner, J., Torres, J., Magaña-Torres, M., et al. (2015). EGFR gene polymorphisms-216G> T and-191C> A are risk markers for gastric cancer in Mexican population. Genet. Mol. Res. 14, 1802–1807. doi:10.4238/2015.March.13.8
Whirl-Carrillo, M., Huddart, R., Gong, L., Sangkuhl, K., Thorn, C. F., Whaley, R., et al. (2021). An evidence-based framework for evaluating pharmacogenomics knowledge for personalized medicine. Clin. Pharmacol. and Ther. 110, 563–572. doi:10.1002/cpt.2350
Zhao, M., Ma, J., Li, M., Zhang, Y., Jiang, B., Zhao, X., et al. (2021). Cytochrome P450 enzymes and drug metabolism in humans. Int. J. Mol. Sci. 22, 12808. doi:10.3390/ijms222312808
Keywords: genetic variants, single-nucleotide variants, pharmacogenetic, Cuban population, admixed population, precision medicine
Citation: Reyes-Reyes E, Herrera-Isidrón JA, Cuétara-Lugo E, Shkedy Z, Valkenborg D, Pérez-Novo CA, Fernández-Peña G, González-Pérez I, Fernández-Pérez MD, Vanden-Berghe W and Rodeiro-Guerra I (2024) Prevalence of single-nucleotide variants in twenty-five pharmacogenes from a Cuban sample cohort. Front. Pharmacol. 15:1467036. doi: 10.3389/fphar.2024.1467036
Received: 19 July 2024; Accepted: 11 September 2024;
Published: 27 September 2024.
Edited by:
Luis Andrés López-Fernández, Gregorio Marañón Hospital, SpainReviewed by:
Juan Carlos Fernandez-Lopez, National Institute of Genomic Medicine (INMEGEN), MexicoCopyright © 2024 Reyes-Reyes, Herrera-Isidrón, Cuétara-Lugo, Shkedy, Valkenborg, Pérez-Novo, Fernández-Peña, González-Pérez, Fernández-Pérez, Vanden-Berghe and Rodeiro-Guerra. This is an open-access article distributed under the terms of the Creative Commons Attribution License (CC BY). The use, distribution or reproduction in other forums is permitted, provided the original author(s) and the copyright owner(s) are credited and that the original publication in this journal is cited, in accordance with accepted academic practice. No use, distribution or reproduction is permitted which does not comply with these terms.
*Correspondence: Idania Rodeiro-Guerra, aWRhbmlhQGljaW1hci5jdQ==; Wim Vanden-Berghe, d2ltLnZhbmRlbmJlcmdoZUB1YW50d2VycGVuLmJl
Disclaimer: All claims expressed in this article are solely those of the authors and do not necessarily represent those of their affiliated organizations, or those of the publisher, the editors and the reviewers. Any product that may be evaluated in this article or claim that may be made by its manufacturer is not guaranteed or endorsed by the publisher.
Research integrity at Frontiers
Learn more about the work of our research integrity team to safeguard the quality of each article we publish.