- 1Department of Pharmacy, Shanghai Children’s Hospital, School of Medicine, Shanghai Jiao Tong University, Shanghai, China
- 2Institute of Chinese Materia Medica, Shanghai University of Traditional Chinese Medicine, Shanghai, China
- 3Department of Pharmacy, Clinical Medical College, Affiliated Hospital of Chengdu University, Chengdu, China
- 4Department of Nephrology and Rheumatology, Shanghai Children’s Hospital, School of Medicine, Shanghai Jiao Tong University, Shanghai, China
Background: Tacrolimus is widely used to treat pediatric nephrotic range proteinuria (NRP). Diltiazem, a CYP3A4/5 inhibitor, is often administered with tacrolimus, affecting its pharmacokinetic profile. The impact of this combination on tacrolimus exposure, particularly in CYP3A5*3 genetic polymorphism, remains unclear in pediatric NRP patients. This study aimed to evaluate the effects of diltiazem on tacrolimus pharmacokinetics, focusing on the CYP3A5*3 polymorphism.
Methods: We conducted a retrospective clinical study involving pediatric NRP patients, divided into two groups: those receiving tacrolimus with diltiazem and those receiving tacrolimus alone. Propensity score matching (PSM) was used to balance the baseline characteristics between the groups. We compared daily dose-adjusted trough concentrations (C0/D) of tacrolimus in both the original and PSM cohorts. The influence of diltiazem on tacrolimus C0/D, stratified by CYP3A5*3 genetic polymorphism, was assessed in a self-controlled case series study.
Results: Before PSM, the tacrolimus C0/D in patients taking diltiazem was significantly higher compared to those with tacrolimus alone (75.84 vs. 56.86 ng/mL per mg/kg, P = 0.034). This finding persisted after PSM (75.84 vs. 46.93 ng/mL per mg/kg, P= 0.028). In the self-controlled case study, tacrolimus C0/D elevated about twofold (75.84 vs. 34.76 ng/mL per mg/kg, P < 0.001) after diltiazem administration. CYP3A5 expressers (CYP3A5*1/*1 and *1/*3) and CYP3A5 non-expressers (CYP3A5*3/*3) experienced a 1.8-fold and 1.3-fold increase in tacrolimus C0/D when combined with diltiazem, respectively.
Conclusion: Diltiazem significantly increased tacrolimus C0/D, with CYP3A5*3 expressers showing higher elevations than non-expressers among pediatric NRP patients. These findings highlight the importance of personalized tacrolimus therapy based on CYP3A5*3 genotypes in pediatric patients taking diltiazem.
1 Introduction
Nephrotic syndrome (NS) is clinically characterized by massive proteinuria, hypoalbuminemia, edema, and hyperlipidemia (Downie et al., 2017; Mattoo and Sanjad, 2022). Tacrolimus, a widely used immunosuppressive drug, is recommended by the Kidney Disease Improving Global Outcomes (KDIGO) guidelines for treating NS in children and adults (Radhakrishnan and Cattran, 2012). Previous studies have shown that tacrolimus can reduce proteinuria by inhibiting the MAPK signaling pathway (Shen et al., 2016). However, the narrow therapeutic window of tacrolimus coupled with the potential for related toxicities present significant challenges and limitations for its clinical use (Brunet et al., 2019). Therefore, close therapeutic drug monitoring (TDM) and individualized dosing strategies are essential to ensure safe and effective administration of tacrolimus in patients.
Tacrolimus is primarily metabolized by the CYP3A enzyme in humans, leading to a high propensity for drug-drug interactions, particularly when taking CYP3A4 and CYP3A5 inhibitors or inducers (Niwa et al., 2007). Diltiazem, a CYP3A4/5 inhibitor, is often used in combination with tacrolimus to elevate the tacrolimus concentration in kidney transplant recipients and refractory nephrotic syndrome (RNS) based on its repressive roles on the metabolic elimination of tacrolimus and its protective roles from nephrotoxicity (Becker et al., 1996; Kidney Disease: Improving Global Outcomes KDIGO Transplant Work Group, 2009; Shen et al., 2016; Larpparisuth et al., 2019; Sun et al., 2019; Susomboon et al., 2022) Diltiazem has been reported to influence tacrolimus concentration by sharing the same CYP3A4/5 metabolic pathway (Sun et al., 2018; Larpparisuth et al., 2019). Initial studies in kidney transplant recipients have explored this interaction. A retrospective analysis from Canada found that diltiazem had a minimal effect on tacrolimus exposure in 64 adult kidney transplant recipients (Kothari et al., 2004). In contrast, a nonrandomized seven-period stepwise pharmacokinetic study conducted in Australia demonstrated that diltiazem significantly increased tacrolimus exposure by 26%–177% in renal transplant recipients, suggesting a substantial impact (Jones and Morris, 2002). Similarly, a Malaysian study reported a significant increase in tacrolimus exposure from 50% to 100% in kidney transplant recipients aged 18 years and older (Choong et al., 2018). Although these studies had limitations, such as only including adult recipients and not considering the effects of the CYP3A4 and CYP3A5 polymorphisms, they highlight the variability of the pharmacokinetic interaction between tacrolimus and diltiazem between different populations. Studies on the diltiazem-tacrolimus interaction in pediatric NS, particularly in China, are limited. Sun et al. initially reported a 43%–126% increase in whole blood tacrolimus trough levels (C0) in 7 pediatric patients with NS without analyzing the influences of the CYP3A genetic polymorphism (Sun et al., 2019). Subsequent studies confirmed the effects of CYP3A5*3 and diltiazem on tacrolimus pharmacokinetics (Guo et al., 2020; Li et al., 2021; Wang et al., 2021). The combination of CYP3A5*3 polymorphisms, concomitant use of diltiazem, body weight, and age significantly influenced daily dose-adjusted tacrolimus C0 (C0/D) (Guo et al., 2020; Li et al., 2021). In addition to CYP3A5*3, polymorphisms in CYP3A4, ABCB1, and SLCO1B3 could be related to the moderate effect of diltiazem on tacrolimus sparing (Wang et al., 2021). Several studies have shown that other factors, such as drugs (e.g., voriconazole, omeprazole, wuzhi capsules, phenobarbital), dietary supplements, herbs, and food, can also lead to individual differences in tacrolimus metabolism, distribution, and responsiveness (Bosó et al., 2013; Imamura et al., 2016; Wang et al., 2021; Zhao et al., 2021; Miedziaszczyk et al., 2022; Zhang et al., 2024).
Previous studies have demonstrated that the co-administration of diltiazem and tacrolimus leads to a significant drug-drug interaction, resulting in elevated tacrolimus trough levels (C0) in adult renal transplant recipients. Tacrolimus C0 levels increased significantly in CYP3A5 expressers compared to CYP3A5 non-expressers (Jones and Morris, 2002; Kothari et al., 2004; Li et al., 2011; Choong et al., 2018) However, the extent of the interaction between diltiazem and tacrolimus, influenced by the CYP3A5*3 polymorphism, remains unclear and insufficiently explored in pediatric NS patients. Addressing this gap is crucial for advancing clinical practice. To this end, we conducted a retrospective single-center study to investigate the effect of diltiazem on tacrolimus exposure and the impact of the CYP3A5*3 polymorphism in pediatric patients with nephrotic range proteinuria (NRP). This study provides insights that may inform clinical decision-making before routine co-prescription of diltiazem and tacrolimus.
2 Methods
2.1 Study design and subjects
This study followed the Declaration of Helsinki and received approval from the Ethics Committee of Shanghai Children’s Hospital (approval number: 2024R029-E01). Given that the results of this retrospective study did not influence further therapeutic decision-making, the Ethics Committee determined that formal consent was not required. The study comprises a cohort study and a self-controlled case series study.
2.1.1 Part 1: cohort study
A retrospective cohort study was conducted on patients with NRP (urinary protein excretion >50 mg/kg/day (The Subspecialty Group of Renal Diseases, the Society of Pediatrics, Chinese Medical Association, 2017) who received tacrolimus between 1 January 2015 and 1 January 2022 at Shanghai Children’s Hospital. Patients who had taken other drugs that could affect tacrolimus blood levels, such as ketoconazole, itraconazole, verapamil, erythromycin, clarithromycin, voriconazole, omeprazole, wuzhi capsules, or phenobarbital were excluded. The patients were divided into two groups: those receiving tacrolimus with diltiazem and those receiving only tacrolimus. Clinical information including age, sex, body weight, height, pathological pattern, tacrolimus dosage, diltiazem dosage, blood concentrations, serum creatinine (Scr), serum albumin (ALB), hematocrit (HCT), alanine aminotransferase (ALT), aspartate aminotransferase (AST), and evaluate glomerular filtration rate (eGFR) were collected from the hospital information system and follow-up records.
2.1.2 Part 2: self-controlled case series study
This part of the study used a self-controlled case series design, where each participant served as their control, thus automatically controlling for all fixed confounding variables that could alter the true association between exposure and outcome (Whitaker, 2008). Patients diagnosed with NRP who received tacrolimus and diltiazem between January 2015 and January 2022 were included following the same criteria as the retrospective study. This study examined the changes in dose-adjusted trough concentrations (C0/D) of tacrolimus before and after diltiazem administration.
2.2 Drug therapy regimen and determination of tacrolimus concentrations
All children received a combination treatment with tacrolimus and glucocorticoids. Tacrolimus was administered orally twice daily, with dosages tailored to trough concentrations (C0) obtained through TDM. The C0 of tacrolimus was measured before the sixth or seventh dose after achieving a steady-state concentration, with target levels set at 5–10 ng/mL. For patients who did not reach the target C0, diltiazem was administered to increase the blood concentration of tacrolimus, with a subsequent measurement of C0 before the sixth or seventh dose of tacrolimus after the initiation of diltiazem. For the patient who did not receive diltiazem, the tacrolimus concentrations collected for this study were those measured for the first C0 in patients at Shanghai Children’s Hospital. For the patient who received diltiazem, the tacrolimus concentrations collected for this study were those measured for first tacrolimus C0 after the initiation of diltiazem at Shanghai Children’s Hospital. Glucocorticoids, either prednisone or methylprednisolone, were administered once daily in conjunction with tacrolimus. The initial dose of prednisone was set at 1 mg/kg per day, with subsequent dose reductions. The methylprednisolone dose was converted to the equivalent prednisone dose, with 4 mg of methylprednisolone equal to 5 mg of prednisone.
Blood samples were collected from all patients 30 min before the morning dose. Tacrolimus C0 was determined according to the previous study (Yu et al., 2022). Briefly, whole blood samples were individually added into 300 μL of an internal standard extracting solution, which contained 3.0 ng/mL of ASC, 50 mmol/L of ZnSO4, and a methanol/water mixture at 50% concentration. Each sample within a centrifuge tube was subjected to vortex mixing for a duration exceeding 30 s, followed by a thorough blending for 5 min using a Vortex mixer V3 (Essenscien). Subsequent to a 5-minute centrifugation at 14,000 rpm while maintained at 4 °C, a minimum of 100 μL of the supernatant was carefully pipetted into a sample vial in preparation for LC-MS/MS analysis. The weight-adjusted daily dose and the tacrolimus daily C0/D were calculated.
2.3 DNA extraction and genotyping
Total DNA was extracted from whole blood samples obtained from subjects using a TIANamp Blood DNA Kit (Tiangen Biotech, Beijing). Genotyping of the SNP CYP3A5*3 (rs776746) was carried out by direct sequencing, as previously described (Liu et al., 2019). The primers used for CYP3A5*3 were:
Forward primer: 5′ACTGCCCTTGCAGCATTTA 3′
Reverse primer: 5′CCAGGAAGCCAGACTTTGA 3′
2.4 Statistical analysis
Data analysis was conducted using SPSS software (version 24.0, IBM, Armonk, NY, United States) and GraphPad Prism (version 7.0, GraphPad Software, CA, United States). Continuous variables are presented as mean ± standard deviation (SD) for normally distributed data or median (interquartile range, IQR) for data that are not normally distributed. Categorical data are described by frequencies and proportions. Propensity score matching (PSM) was used to reduce confounding biases. To minimize confounding biases between the group receiving tacrolimus comedicated with diltiazem and the group receiving tacrolimus alone, PSM was conducted to balance baseline characteristics (age, sex, body mass index, tacrolimus dosage, genotype, ALB, HCT, ALT, AST, and eGFR) between the group receiving tacrolimus with diltiazem and the group receiving tacrolimus alone. This was achieved using a 1:1 nearest-neighbor matching algorithm with a caliper set at 0.02. Differences in tacrolimus C0/D and other changes between groups were analyzed using the Mann-Whitney U test. The comparison of tacrolimus blood exposure before and after diltiazem co-administration was performed using a Wilcoxon signed-rank sum test. Statistical significance was defined as p < 0.05.
3 Results
3.1 Patient characteristics
Between 1 January 2015 and 1 January 2022, a total of 232 pediatric patients who received tacrolimus were initially identified. Of these, 93 patients were excluded because of the low level of urinary protein excretion (lower than 50 mg/kg/day). The following patients were further excluded: patients who had taken other drugs that could affect tacrolimus blood levels, such as ketoconazole, itraconazole, verapamil, erythromycin, clarithromycin, voriconazole, omeprazole, wuzhi capsules, or phenobarbital were excluded. Finally, a total of 117 patients (76 males and 41 females) were included. Among them, 96 patients were treated with tacrolimus, while 21 were taking diltiazem at a median dose of 1.82 mg/kg/day (range 1.56–2.67 mg/kg/day). A summary of the baseline characteristics of the patients is presented in Table 1. The groups differed primarily in terms of tacrolimus dosage and AST. After PSM, all baseline characteristics were well-balanced between the two groups. Ultimately, 21 patients were enrolled in each group: one group received tacrolimus and diltiazem, and the other received only tacrolimus.
3.2 Genotype frequencies
The frequencies of the CYP3A5 genotype in each study group are presented in Table 2. Genotype frequencies were expected to adhere to the Hardy-Weinberg equilibrium proportions. The observed frequencies did not deviate significantly from these expectations (p > 0.05), indicating no significant differences in genotype distribution between the group receiving tacrolimus with diltiazem and the group receiving tacrolimus alone (p > 0.05).
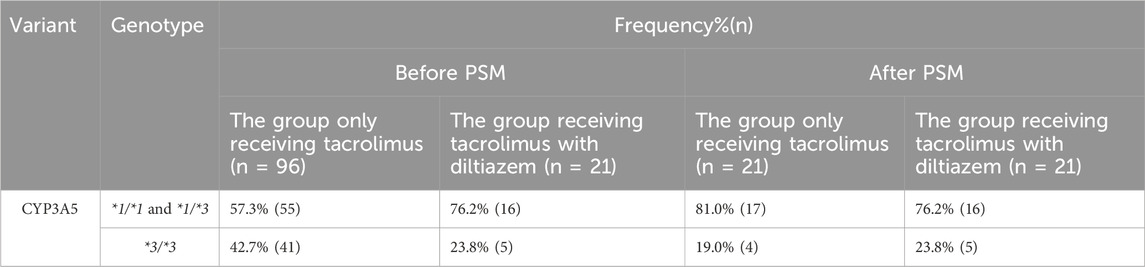
Table 2. Distribution of CYP3A5 genotype in the study population of each group. *1/*1 and *1/*3: CYP3A5 expressers; *3/*3: CYP3A5 nonexpressers
3.3 Diltiazem significantly increased tacrolimus C0/D
As shown in Figure 1 and Supplementary Table S1, the dose-adjusted C0 of tacrolimus in diltiazem patients was significantly higher than in those without diltiazem (75.84 vs. 56.86 ng/mL per mg/kg, p = 0.034). After matching, the post-PSM results were consistent with the initial findings, showing a significant difference (75.84 vs. 46.93 ng/mL per mg/kg, p = 0.028). In the 21 patients analyzed before and after adding diltiazem, tacrolimus C0/D increased nearly two-fold (75.84 vs. 34.76 ng/mL per mg/kg, p < 0.001), as shown in Figure 2 and Supplementary Table S2. These results demonstrate that diltiazem significantly increases tacrolimus C0/D.
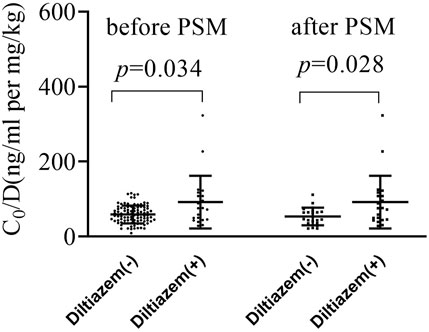
Figure 1. The change of tacrolimus C0/D by diltiazem in both the original and propensity score matching (PSM) cohort study. PSM, propensity score matching; C0/D, dose-adjusted trough concentrations; Diltiazem -: without diltiazem; Diltiazem +: with diltiazem; The horizontal lines represent the medium value and the interquartile range.
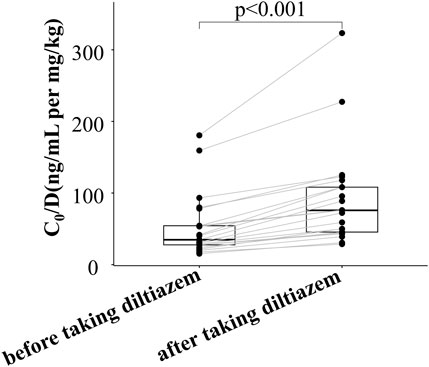
Figure 2. Change in tacrolimus C0/D of 21 patients before and after taking diltiazem in the self-controlled case series study. C0/D: tacrolimus dose-adjusted trough concentration; The horizontal lines represent the medium value and the interquartile range.
3.4 Effect of the CYP3A5*3 genotype on tacrolimus C0/D and the extent of diltiazem–tacrolimus interaction in pediatric patients with NRP
As shown in Figure 3 and detailed in Supplementary Table S2, the current study highlights a significant drug-drug interaction between tacrolimus and diltiazem, particularly influenced by CYP3A5*3 polymorphisms. The results of our self-controlled case series study (n = 21) revealed that CYP3A5 expressers (CYP3A5*1/*1 and *1/*3) who received diltiazem exhibited a nearly 1.8-fold higher tacrolimus C0/D compared to CYP3A5 expressers who did not receive diltiazem (Figure 3, p < 0.001). Similarly, CYP3A5 nonexpressers (CYP3A5*3/*3) treated with diltiazem showed an approximately a 1.3-fold increase in tacrolimus C0/D compared to CYP3A5 nonexpressers without diltiazem (Figure 3, p = 0.043). A significant difference in the degree of increase in tacrolimus exposure due to diltiazem was observed between pediatric patients with different CYP3A5 genotype (Figure 4, p = 0.028), with CYP3A5 expressers experiencing a more pronounced increase in tacrolimus exposure when taking with diltiazem. These findings suggest that CYP3A5 expressers are more susceptible to the inhibitory effects of diltiazem on tacrolimus metabolism.
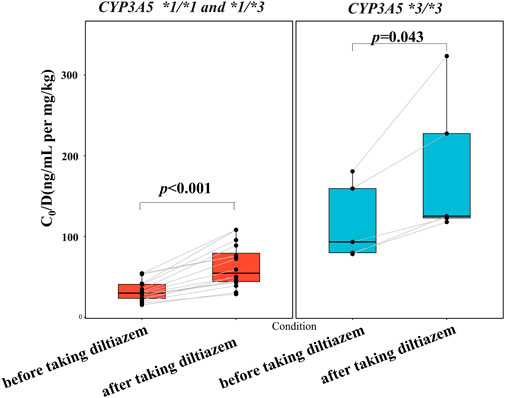
Figure 3. Impact of the CYP3A5 genotype on diltiazem-tacrolimus interaction in pediatric patients with NRP enrolled in a self-controlled case series study.
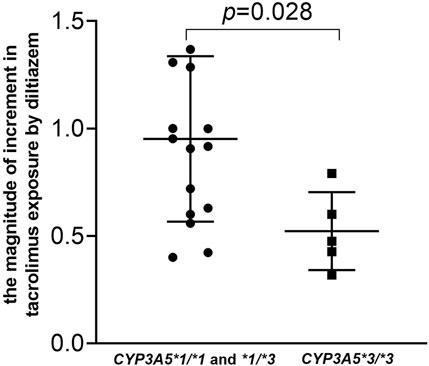
Figure 4. Impact of the CYP3A5 genotype on the magnitude of increase in tacrolimus by diltiazem in pediatric patients with NRP enrolled in a self-controlled case series study. The magnitude of increase were calculated using the following equation: The magnitude of increase = (Tacrolimus concentration of patients who received tiltiazem-Tacrolimus concentration of patients who did not receive diltiazem)/Tacrolimus concentration of patients who did not receive diltiazem.
4 Discussion
The combination of tacrolimus with diltiazem, although recommended by the KDIGO clinical practice guidelines (Kidney Disease: Improving Global Outcomes KDIGO Transplant Work Group, 2009), is not commonly used in Chinese pediatric patients with NS. We explored the effect of diltiazem on tacrolimus C0/D in pediatric RNS patients and examined its relationship with CYP3A5*3 SNPs. Our findings indicate a significant increase in tacrolimus C0/D in pediatric patients when taking diltiazem, particularly among CYP3A5 expressers who showed significantly higher increases in tacrolimus exposure. The results offer valuable information for clinical management and will inform future prospective clinical trials in this patient group, potentially improving treatment outcomes and quality of life.
Previous studies have demomstrated the impact of diltiazem on systemic tacrolimus exposure (Jones and Morris, 2002; Choong et al., 2018; Larpparisuth et al., 2019; Lodha et al., 2020). Our observations are consistent with these reports, showing a significant increase in the tacrolimus C0/D ratio, irrespective of the baseline characteristics being balanced across the study population. We conducted an additional assessment through a self-controlled case series study to validate our findings. The consistency between this additional analysis and our primary results further corroborates the drug-drug interaction between diltiazem and tacrolimus, enhancing confidence in the validity and generalizability of our findings. Although the observed trend of increased tacrolimus exposure induced by diltiazem mirrors prior study, the variability in the magnitude of this increase underscores the complexity of predicting drug interactions. The magnitude of the increase in tacrolimus exposure caused by diltiazem varies and may be influenced by several factors. First, racial ancestry plays a significant role. Previous research has shown that the increase in tacrolimus exposure due to diltiazem ranged from 60% to 100% in Malaysian patients (Choong et al., 2018), compared to 26%–177% in Australian patients (Jones and Morris, 2002). These differences could be attributed to variations in genetic makeup or other factors that affect the extent of the diltiazem-tacrolimus interaction between Malaysian populations and Australian populations. Second, the dosage of diltiazem also impacts tacrolimus C0/D. Previous studies noted an increase in tacrolimus exposure in pediatric patients receiving diltiazem at 2.1 mg/kg/day (range 1.2–3.4 mg/kg/day) (Sun et al., 2019). Our study used a diltiazem dosage of 1.82 mg/kg/day (range 1.56–2.67 mg/kg/day), which could lead to varying effects on the tacrolimus C0/D. This suggests that the diltiazem dosage is critical in determining its influence on tacrolimus pharmacokinetics. To fully understand the impact of diltiazem dosage on tacrolimus exposure, further research involving various dosing regimens is necessary. Conducting extensive and well-designed studies across larger and more varied patient cohorts would provide deeper insight into the dynamics of this drug-drug interaction.
Previous studies have demonstrated that diltiazem competes with tacrolimus for the CYP3A5 binding site, reducing tacrolimus metabolism and increasing its exposure (Kuehl et al., 2001; Dai et al., 2006; Uesugi et al., 2006; Wang et al., 2021). This interaction is significantly affected by the CYP3A5*3 polymorphism. Higher sensitivity to the inhibitory effects of diltiazem on tacrolimus metabolism in CYP3A5 expressers was observed (Li et al., 2011). Similarly, our study observed that pediatric patients with CYP3A5 expressers exhibited significantly increased tacrolimus exposure when co-treated with diltiazem. These findings are consistent with previous research, confirming the crucial role of genetic variations in CYP3A5 in modulating the diltiazem-tacrolimus interaction (Kuehl et al., 2001; Dai et al., 2006; Uesugi et al., 2006; Li et al., 2011). The presence of the CYP3A5*1 allele, associated with increased enzymatic activity, leads to more potent CYP3A inhibition and increased tacrolimus C0/D. Wang et al. (2021) observed a 12.2% increase in tacrolimus C0/D in pediatric RNS patients taking diltiazem compared to those without (94.0 vs 83.8 ng/mL per mg/kg, P < 0.05), subjects with CYP3A5*1/*1 and *1/*3 experiencing a 54.5% increase compared to subjects with CYP3A5*3/*3. Our study found a significant increase in tacrolimus C0/D, with patients taking diltiazem experiencing a 61.6% increase. In the self-controlled case study, tacrolimus C0/D in CYP3A5 expressers increased 1.8-fold after combining with diltiazem, while CYP3A5 non-expressers increased 1.3-fold. These results are consistent with previous findings (Wang et al., 2021), highlighting the influence of CYP3A5 polymorphism on CYP3A enzyme activity, which is critical for pharmacotherapy decisions, particularly when managing drug interactions involving tacrolimus and diltiazem.
In addition, some clinical studies shown that diltiazem significantly affects tacrolimus pharmacokinetics by inhibiting CYP3A4 or P-glycoprotein (P-gp) (Jones and Morris, 2002; Guan et al., 2018). Among them, P-gp is encoded by ABCB1. CYP3A4*1G polymorphism and the ABCB1-C3435T polymorphism has been shown to affect tacrolimus exposure and the extent of its interaction with diltiazem in Chinese pediatric RNS patients (Wang et al., 2021). Research also indicates a linkage disequilibrium between CYP3A5 and CYP3A4 alleles in the Asian population, affecting drug interactions (Park et al., 2008). Interactions between CYP3A4 and CYP3A5 could be observed in Chinese kidney transplant recipients (Zuo et al., 2013). Diltiazem acts as a substrate for CYP3A4 and a potent inhibitor of CYP3A activity, could reduce tacrolimus (a CYP3A4/5 substrate) metabolism and increase tacrolimus exposure (Bosó et al., 2013; Imamura et al., 2016). The mechanism by which diltiazem affects blood exposure to tacrolimus may be attributed to its inhibition of CYP3A4. This inhibition induced by diltiazem leads to a decrease in overall CYP3A enzyme activity. As a result, the expression and metabolic activity of CYP3A5 are reduced, leading to increased exposure to tacrolimus. Patients carrying ABCB1-3435TT experienced greater increases in tacrolimus exposure due to reduced intestinal P-gp expression induced by ABCB1-3435TT mutation, which results in enhanced absorption (Wang et al., 2021). Interestingly, Diltiazem also competitively inhibits P-gp, enhancing the absorption of tacrolimus (Sakaeda et al., 2003). However, our study did not assess the CYP3A4*1G and ABCB1-C3435T polymorphisms and their involvement remains unexplored in this retrospective analysis. More research is necessary to fully understand these genetic influences on the interaction between diltiazem and tacrolimus.
This study has several limitations. First, the findings may lack generalizability since the data were obtained retrospectively from a single center. Conducting a multi-country and multi-center clinical investigation would enhance the precision and validity of our results. Second, the retrospective nature of the data collection means that the duration of tacrolimus treatment and other confounding factors such as diet could have varied between patients, potentially introducing confounding factors and bias. Third, the small sample size may limit statistical power to detect differences among smaller subgroups within our study, particularly the limited number of CYP3A5 non-expressers in the tacrolimus combined with the diltiazem group. A larger sample size would increase the reliability and robustness of the analysis. Forth, the interaction between corticosteroids and tacrolimus was not investigated, which is a limitation of our research. We will explore and clarify it in the future study. Lastly, although this retrospective study provides valuable preliminary information, validating the findings with prospective studies is imperative. Future prospective randomized controlled trials are needed to confirm these results and strengthen the evidence base, thus contributing to a more comprehensive understanding of the diltiazem-tacrolimus interaction in pediatric patients.
5 Conclusion
Our study demonstrates that diltiazem significantly increases the dose-adjusted trough concentration (C0/D) of tacrolimus in both cohort and self-controlled case series studies. CYP3A5 expressers are more susceptible to the inhibitory effects of diltiazem on tacrolimus metabolism compared to CYP3A5 non-expressers. These results underscore the need for personalized therapy approaches that incorporate the detection of the CYP3A5 genotype with TDM.
Data availability statement
The original contributions presented in the study are included in the article/Supplementary Material, further inquiries can be directed to the corresponding authors.
Ethics statement
The studies involving humans were approved by the Ethics Committee of Shanghai Children’s Hospital. The studies were conducted in accordance with the local legislation and institutional requirements. The ethics committee/institutional review board waived the requirement of written informed consent for participation from the participants or the participants’ legal guardians/next of kin because Given that the results of this retrospective study did not influence further therapeutic decision-making, the requirement of informed consent was waived by the Ethics Committee of the Shanghai Children’s Hospital.
Author contributions
QY: Conceptualization, Data curation, Formal Analysis, Funding acquisition, Investigation, Supervision, Validation, Visualization, Writing–original draft, Writing–review and editing. YW: Data curation, Formal Analysis, Methodology, Project administration, Resources, Validation, Writing–review and editing. XW: Conceptualization, Formal Analysis, Investigation, Supervision, Validation, Visualization, Writing–original draft, Writing–review and editing. PW: Methodology, Resources, Writing–review and editing. BT: Formal Analysis, Methodology, Writing–review and editing. YL: Data curation, Formal Analysis, Methodology, Resources, Writing–review and editing. HS: Conceptualization, Formal Analysis, Investigation, Supervision, Writing–review and editing. WH: Conceptualization, Formal Analysis, Investigation, Project administration, Supervision, Visualization, Writing–review and editing. HL: Conceptualization, Data curation, Formal Analysis, Funding acquisition, Investigation, Project administration, Supervision, Writing–review and editing, Writing–original draft.
Funding
The author(s) declare that financial support was received for the research, authorship, and/or publication of this article. This work is financially sponsored by the Shanghai Sailing Program (No. 21YF1437900), the Interdisciplinary Program of Shanghai Jiao Tong University (No. YG2019QNA03), and the Special Cultivation Project of the National Natural Science Foundation of Shanghai Children’s Hospital (No. 2020YGZQ08) to QY. It is also financially sponsored by Shanghai “Rising Stars of Medical Talents” Youth Development Program (SHWSRS(2020)-087) to HL.
Acknowledgments
The authors thank Xiaoyan Qiu for her support in editing this manuscript, and Zhiling Li for her contribution to the discussions.
Conflict of interest
The authors declare that the research was conducted in the absence of any commercial or financial relationships that could be construed as a potential conflict of interest.
Publisher’s note
All claims expressed in this article are solely those of the authors and do not necessarily represent those of their affiliated organizations, or those of the publisher, the editors and the reviewers. Any product that may be evaluated in this article, or claim that may be made by its manufacturer, is not guaranteed or endorsed by the publisher.
Supplementary material
The Supplementary Material for this article can be found online at: https://www.frontiersin.org/articles/10.3389/fphar.2024.1463595/full#supplementary-material
Abbreviations
AST, Aspartate aminotransferase; ALT, Alanine aminotransferase; AUC, Area under curve; ALB, Albumin; BMI, Body mass index; BUN, Blood urea nitrogen; ETDA, Ethylene diamine tetraacetic acid; HCT, Hematocrit; PSM, Propensity score matching; Scr, Serum creatinine; TDM, Therapeutic drug monitoring; SNPs, Single nucleotide polymorphisms.
References
Becker, G., Witzke, O., Baltes, A., Hamar, P., Philipp, T., and Heemann, U. (1996). Diltiazem minimizes tubular damage due to FK506-mediated nephrotoxicity following ischemia and reperfusion in rats. Transpl. Immunol. 4, 68–71. doi:10.1016/s0966-3274(96)80040-2
Bosó, V., Herrero, M. J., Bea, S., Galiana, M., Marrero, P., Marqués, M. R., et al. (2013). Increased hospital stay and allograft dysfunction in renal transplant recipients with Cyp2c19 AA variant in SNP rs4244285. Drug Metab. Dispos. 41, 480–487. doi:10.1124/dmd.112.047977
Brunet, M., van Gelder, T., Åsberg, A., Haufroid, V., Hesselink, D. A., Langman, L., et al. (2019). Therapeutic drug monitoring of tacrolimus-personalized therapy: second consensus report. Ther. Drug Monit. 41, 261–307. doi:10.1097/FTD.0000000000000640
Choong, C. L., Wong, H. S., Lee, F. Y., Lee, C. K., Kho, J. V., Lai, Y. X., et al. (2018). Dose-Response relationship between diltiazem and tacrolimus and its safety in renal transplant recipients. Transpl. Proc. 50, 2515–2520. doi:10.1016/j.transproceed.2018.04.024
Dai, Y., Hebert, M. F., Isoherranen, N., Davis, C. L., Marsh, C., Shen, D. D., et al. (2006). Effect of CYP3A5 polymorphism on tacrolimus metabolic clearance in vitro. Drug Metab. Dispos. 34, 836–847. doi:10.1124/dmd.105.008680
Downie, M. L., Gallibois, C., Parekh, R. S., and Noone, D. G. (2017). Nephrotic syndrome in infants and children: pathophysiology and management. Paediatr. Int. Child. Health 37, 248–258. doi:10.1080/20469047.2017.1374003
Guan, X.-F., Li, D.-Y., Yin, W.-J., Ding, J.-J., Zhou, L.-Y., Wang, J.-L., et al. (2018). Population pharmacokinetic modeling of diltiazem in Chinese renal transplant recipients. Eur. J. Drug Metab. Pharmacokinet. 43, 55–62. doi:10.1007/s13318-017-0425-y
Guo, H.-L., Xu, J., Sun, J.-Y., Li, L., Guo, H.-L., Jing, X., et al. (2020). Tacrolimus treatment in childhood refractory nephrotic syndrome: a retrospective study on efficacy, therapeutic drug monitoring, and contributing factors to variable blood tacrolimus levels. Int. Immunopharmacol. 81, 106290. doi:10.1016/j.intimp.2020.106290
Imamura, C. K., Furihata, K., Okamoto, S., and Tanigawara, Y. (2016). Impact of cytochrome P450 2C19 polymorphisms on the pharmacokinetics of tacrolimus when coadministered with voriconazole. J. Clin. Pharmacol. 56, 408–413. doi:10.1002/jcph.605
Jones, T. E., and Morris, R. G. (2002). Pharmacokinetic interaction between tacrolimus and diltiazem: dose-response relationship in kidney and liver transplant recipients. Clin. Pharmacokinet. 41, 381–388. doi:10.2165/00003088-200241050-00005
Kidney Disease: Improving Global Outcomes (KDIGO) Transplant Work Group (2009). KDIGO clinical practice guideline for the care of kidney transplant recipients. Am. J. Transpl. 9 (Suppl. 3), S1–S155. doi:10.1111/j.1600-6143.2009.02834.x
Kothari, J., Nash, M., Zaltzman, J., and Ramesh Prasad, G. V. (2004). Diltiazem use in tacrolimus-treated renal transplant recipients. J. Clin. Pharm. Ther. 29, 425–430. doi:10.1111/j.1365-2710.2004.00578.x
Kuehl, P., Zhang, J., Lin, Y., Lamba, J., Assem, M., Schuetz, J., et al. (2001). Sequence diversity in CYP3A promoters and characterization of the genetic basis of polymorphic CYP3A5 expression. Nat. Genet. 27, 383–391. doi:10.1038/86882
Larpparisuth, N., Skulratanasak, P., Vongwiwatana, A., and Premasathian, N. (2019). Effect on dosage change and intrapatient variability after conversion from twice-daily to once-daily tacrolimus among Thai kidney transplant patients with and without CYP3A4/5 inhibitors. Transpl. Proc. 51, 2620–2623. doi:10.1016/j.transproceed.2019.02.065
Li, J.-L., Wang, X.-D., Chen, S.-Y., Liu, L.-S., Fu, Q., Chen, X., et al. (2011). Effects of diltiazem on pharmacokinetics of tacrolimus in relation to CYP3A5 genotype status in renal recipients: from retrospective to prospective. Pharmacogenomics J. 11, 300–306. doi:10.1038/tpj.2010.42
Li, L., Zhu, M., Li, D.-Y., Guo, H.-L., Hu, Y.-H., Xu, Z.-Y., et al. (2021). Dose tailoring of tacrolimus based on a non-linear pharmacokinetic model in children with refractory nephrotic syndrome. Int. Immunopharmacol. 98, 107827. doi:10.1016/j.intimp.2021.107827
Liu, H., Xu, Q., Huang, W., Zhao, Q., Jiang, Z., Kuang, X., et al. (2019). CYP3A5 and CYP3A7 genetic polymorphisms affect tacrolimus concentration in pediatric patients with nephrotic range proteinuria. Eur. J. Clin. Pharmacol. 75, 1533–1540. doi:10.1007/s00228-019-02726-w
Lodha, A. R., Pillai, A., Sheth, K., and Hiremath, J. (2020). A retrospective cohort study exploring diltiazem as a pharmaco-enhancer for tacrolimus, in a post-heart transplant setting. Clin. Transpl. 34, e14100. doi:10.1111/ctr.14100
Mattoo, T. K., and Sanjad, S. (2022). Current understanding of nephrotic syndrome in children. Pediatr. Clin. North Am. 69, 1079–1098. doi:10.1016/j.pcl.2022.08.002
Miedziaszczyk, M., Bajon, A., Jakielska, E., Primke, M., Sikora, J., Skowrońska, D., et al. (2022). Controversial interactions of tacrolimus with dietary supplements, herbs and food. Pharmaceutics 14, 2154. doi:10.3390/pharmaceutics14102154
Niwa, T., Yamamoto, S., Saito, M., Shiraga, T., and Takagi, A. (2007). Effect of cyclosporine and tacrolimus on cytochrome p450 activities in human liver microsomes. Yakugaku Zasshi 127, 209–216. doi:10.1248/yakushi.127.209
Park, S. Y., Kang, Y. S., Jeong, M. S., Yoon, H. K., and Han, K. O. (2008). Frequencies of CYP3A5 genotypes and haplotypes in a Korean population. J. Clin. Pharm. Ther. 33, 61–65. doi:10.1111/j.1365-2710.2008.00879.x
Radhakrishnan, J., and Cattran, D. C. (2012). The KDIGO practice guideline on glomerulonephritis: reading between the (guide) lines-application to the individual patient. Kidney Int. 82, 840–856. doi:10.1038/ki.2012.280
Sakaeda, T., Nakamura, T., and Okumura, K. (2003). Pharmacogenetics of MDR1 and its impact on the pharmacokinetics and pharmacodynamics of drugs. Pharmacogenomics 4, 397–410. doi:10.1517/phgs.4.4.397.22747
Shen, X., Jiang, H., Ying, M., Xie, Z., Li, X., Wang, H., et al. (2016). Calcineurin inhibitors cyclosporin A and tacrolimus protect against podocyte injury induced by puromycin aminonucleoside in rodent models. Sci. Rep. 6, 32087. doi:10.1038/srep32087
Sun, J.-Y., Hu, Y.-H., Guo, H.-L., Jing, X., Xu, Z.-J., Sun, F., et al. (2019). Diltiazem used as a tacrolimus-sparing agent for treatment of pediatric patients with refractory nephrotic syndrome: a case report and retrospective analysis. Eur. J. Clin. Pharmacol. 75, 591–593. doi:10.1007/s00228-018-2604-4
Sun, J.-Y., Xu, Z.-J., Sun, F., Guo, H.-L., Ding, X.-S., Chen, F., et al. (2018). Individualized tacrolimus therapy for pediatric nephrotic syndrome: considerations for ontogeny and pharmacogenetics of CYP3A. Curr. Pharm. Des. 24, 2765–2773. doi:10.2174/1381612824666180829101836
Susomboon, T., Kunlamas, Y., Vadcharavivad, S., and Vongwiwatana, A. (2022). The effect of the very low dosage diltiazem on tacrolimus exposure very early after kidney transplantation: a randomized controlled trial. Sci. Rep. 12, 14247. doi:10.1038/s41598-022-18552-7
The Subspecialty Group of Renal Diseases, the Society of Pediatrics, Chinese Medical Association (2017). Evidence-based guideline on diagnosis and treatment of steroid-sensitive, relapsing/steroid-dependent nephrotic syndrome in children (2016).55, 729–734. doi:10.3760/cma.j.issn.0578-1310.2017.10.003
Uesugi, M., Masuda, S., Katsura, T., Oike, F., Takada, Y., and Inui, K. (2006). Effect of intestinal CYP3A5 on postoperative tacrolimus trough levels in living-donor liver transplant recipients. Pharmacogenet Genomics 16, 119–127. doi:10.1097/01.fpc.0000184953.31324.e4
Wang, J., Huang, L., Gao, P., Hu, Y., Ni, Y., Zhu, Z., et al. (2021). Diltiazem on tacrolimus exposure and dose sparing in Chinese pediatric primary nephrotic syndrome: impact of CYP3A4, CYP3A5, ABCB1, and SLCO1B3 polymorphisms. Eur. J. Clin. Pharmacol. 77, 71–77. doi:10.1007/s00228-020-02977-y
Yu, K.-W., Li, B.-L., Yuan, Y.-S., Liao, J.-M., Li, W.-K., Dong, H., et al. (2022). A modified LC-MS/MS method for the detection of whole blood tacrolimus and its clinical value in Chinese kidney transplant patients. Heliyon 8, e10214. doi:10.1016/j.heliyon.2022.e10214
Zhang, C., Ren, X., Liu, Y., Huang, L., Feng, Y., and Zhang, X. (2024). Effects of wuzhi capsule on whole-blood tacrolimus concentration levels: a systematic review and meta-analysis. Ther. Drug Monit. 46, 33–41. doi:10.1097/FTD.0000000000001155
Zhao, X., Lu, X., Zuo, M., Wang, N., Zhang, Y., Chen, J., et al. (2021). Drug-drug interaction comparison between tacrolimus and phenobarbital in different formulations for paediatrics and adults. Xenobiotica 51, 877–884. doi:10.1080/00498254.2021.1943564
Keywords: tacrolimus, diltiazem, drug-drug interaction, CYP3A5, pediatric patients, nephrotic range proteinuria
Citation: Yang Q, Wang Y, Wang X, Wang P, Tan B, Li Y, Sun H, Huang W and Liu H (2024) Drug-drug interaction between diltiazem and tacrolimus in relation to CYP3A5 genotype status in Chinese pediatric patients with nephrotic range proteinuria: a retrospective study. Front. Pharmacol. 15:1463595. doi: 10.3389/fphar.2024.1463595
Received: 12 July 2024; Accepted: 20 August 2024;
Published: 03 September 2024.
Edited by:
Shusen Sun, Western New England University, United StatesReviewed by:
Huaijun Zhu, Nanjing Drum Tower Hospital, ChinaChangcheng Shi, Hangzhou First People’s Hospital, China
Copyright © 2024 Yang, Wang, Wang, Wang, Tan, Li, Sun, Huang and Liu. This is an open-access article distributed under the terms of the Creative Commons Attribution License (CC BY). The use, distribution or reproduction in other forums is permitted, provided the original author(s) and the copyright owner(s) are credited and that the original publication in this journal is cited, in accordance with accepted academic practice. No use, distribution or reproduction is permitted which does not comply with these terms.
*Correspondence: Wenyan Huang, aHVhbmd3eUBzaGNoaWxkcmVuLmNvbS5jbg==; Hongxia Liu, MTYwOUBzaGNoaWxkcmVuLmNvbS5jbg==
†These authors have contributed equally to this work