- 1Department of Zoology and Anthropology, Faculty of Natural Sciences and Informatics, Constantine the Philosopher University in Nitra, Nitra, Slovakia
- 2Department of Botany and Genetics, Faculty of Natural Sciences and Informatics, Constantine the Philosopher University in Nitra, Nitra, Slovakia
- 3Department of Genetics, Cancer Research Institute, Biomedical Research Center of Slovak Academy of Sciences, Bratislava, Slovakia
Bone tissue and breast tissue are interrelated, as demonstrated by breast microcalcifications, breast cancer bone metastases, bone morphogenetic proteins, and Wnt signaling. In addition, osteoblasts and osteoclasts represent an important switch of tumor cell dormancy during bone metastasis. Damage to both types of tissues mentioned above is highly prevalent, especially in postmenopausal women, and manifests itself in osteoporosis and breast cancer. Sea buckthorn (Elaeagnus rhamnoides L.), a botanical drug with high antioxidant, antitumor, anti-inflammatory, immunomodulatory, and regenerative properties, has great therapeutic potential due to the unique composition of its bioactive metabolites. This review aimed to summarize the current knowledge from in vitro and in vivo studies on the effect of sea buckthorn, as well as its most widespread flavonoids isorhamnetin, quercetin, and kaempferol, on bone and breast tissue health. In vitro studies have revealed the beneficial impacts of sea buckthorn and aforementioned flavonoids on both bone health (bone remodeling, mineralization, and oxidative stress) and breast tissue health (cancer cell proliferation, apoptosis, tumor growth, and metastatic behavior). In vivo studies have documented their protective effects against disturbed bone microarchitecture and reduced bone strength in animal models of osteoporosis, as well as against tumor expansion and metastatic properties in animal xenograft models. In any case, further research and clinical trials are needed to carefully evaluate the potential therapeutic benefits of sea buckthorn and its flavonoids. Based on the available information, however, it can be concluded that these bioactive metabolites favorably affect both bone and breast tissue health.
1 Introduction
Sea buckthorn (Elaeagnus rhamnoides L.) is a nitrogen-fixing thorny deciduous shrub that is naturally distributed in Asia and Europe. It can grow in difficult conditions such as frost, drought, and polluted air. This botanical drug has been widely used for its nutritional and medicinal purposes (Patel et al., 2012; Jaśniewska and Diowksz, 2021). Raw fruits, various products made from them (e.g., juices, jams, tinctures), alcoholic extracts from different parts of this botanical drug and oil from the seeds have shown to possess antioxidant, antitumor, anti-inflammatory, immunomodulatory, and regenerative properties, which are related to a unique composition of bioactive metabolites, rich in phenolic metabolites (mainly phenolic acids and flavonoids), essential fatty acids (palmitic acid, palmitoleic acid, stearic acids), vitamins (A, B, C, E, K), phytosterols (cycloartenol, campesterol, sitosterol), and carotenoids (lycopene, lutein, zeaxantin) (Olas et al., 2018; Dudau et al., 2021; Stochmal et al., 2022). In general, more than 60 flavonoids and 10 phenolic acids have been identified in sea buckthorn. The most abundant flavonoids in fruits, leaves, and seeds are isorhamnetin and quercetin, followed by kaempferol, luteolin, myricetin, syringetin, naringenin, and epicatechin (Ren et al., 2020; He et al., 2023). Considering phenolic acids, gallic acid, caffeic acid, and ferulic acid are present in leaves and fruits (Jaśniewska and Diowksz, 2021; Danielski and Shahidi, 2024). This broad spectrum of bioactive metabolites can help prevent or treat a variety of conditions, such as cardiovascular diseases, diabetes mellitus, liver damage, gastrointestinal disorders, neuronal damage, skin lesions, retina damage, osteoporosis, and tumors (Ren et al., 2020).
In this review, we summarize the current knowledge from in vitro and in vivo studies regarding the effect of sea buckthorn, as well as its most widespread flavonoids isorhamnetin, quercetin, and kaempferol, on bone and breast tissue health. In individual studies, where it was relevant, we assessed the fulfillment of the requirements for the phytochemical characterization of the analysed extracts according to the ConPhyMP (Heinrich et al., 2022). Selected flavonoids exhibit both protective effects on bone tissue and antitumor impacts on breast tissue, potentially ameliorating bone loss in osteoporosis and inhibiting breast cancer progression. Therefore, we hypothesized that all of these bioactive metabolites should simultaneously attenuate bone damage and breast malignancy. These profitable effects of aforementioned bioactive metabolites on the health status of bone and breast tissues are still not sufficiently presented, as well as the interplay between both tissues.
2 The link between bone tissue and breast tissue
The relationships between bone and breast tissues are generally based on a constant dependence on the same regulatory and signaling molecules, as well as on mutual interactions through tissue-specific molecules that are mainly exposed at specific periods of life. Both bone tissue and breast tissue are dependent on estrogen, a key hormone that regulates bone mineral density (BMD), thereby maintaining the balance between bone formation and bone resorption. Estrogen is also an important mediator of mammary gland morphogenesis (Stingl, 2011). Furthermore, receptor activator of nuclear factor kappa β (RANK) and its ligand RANKL were discovered as key regulators of osteoclast development and activation. RANK and RANKL, however, also play an important role in the development of a functional lactating mammary gland during pregnancy (Sigl and Penninger, 2014). The mammary gland and bones are closely linked during lactation, when increased calcium requirements for milk production change bone and mineral metabolism (Athonvarangkul and Wysolmerski, 2023). In addition, with increasing age, cells and tissues, including breast and bone, become more susceptible to oxidative stress, which could modify the activity of key proteins and pathways needed to protect against bone and breast tissue damage (Figure 1). Disruption of the aforementioned important regulatory mechanisms and age-related changes in the organism, including oxidative stress, inflammation, and lipid accumulation contribute to simultaneous occurrence of osteoporosis and breast cancer in postmenopausal women (Cho et al., 2015; Muhammad et al., 2018; Martiniakova et al., 2023a). Overall, osteoporosis is the most common type of bone disease and its prevalence was reported at 18.3%. By 2050, more than 30 million people in Europe are expected to be affected by osteoporosis (Salari et al., 2021; Martiniakova et al., 2024). Globally, breast cancer is the second most common cause of cancer death in women, representing 12.5% of all new annual cancer cases worldwide (Muhammad et al., 2018; World Health Organization International Agency for Research on Cancer, 2024). There is a close clinically significant relationship between osteoporosis and breast cancer. Estrogen deficiency is considered the main cause of postmenopausal osteoporosis. Conversely, elevated estrogen levels during life (e.g., late menopause, obesity, estrogen replacement therapy) are associated with increased incidence of breast malignancy. Deregulation of the RANK/RANKL system also contributes to the development of postmenopausal osteoporosis. The RANK/RANKL pathway has also been found to be involved in hormone-induced breast cancer development and metastatic spread to bone (Muhammad et al., 2018; Martiniakova et al., 2023a).
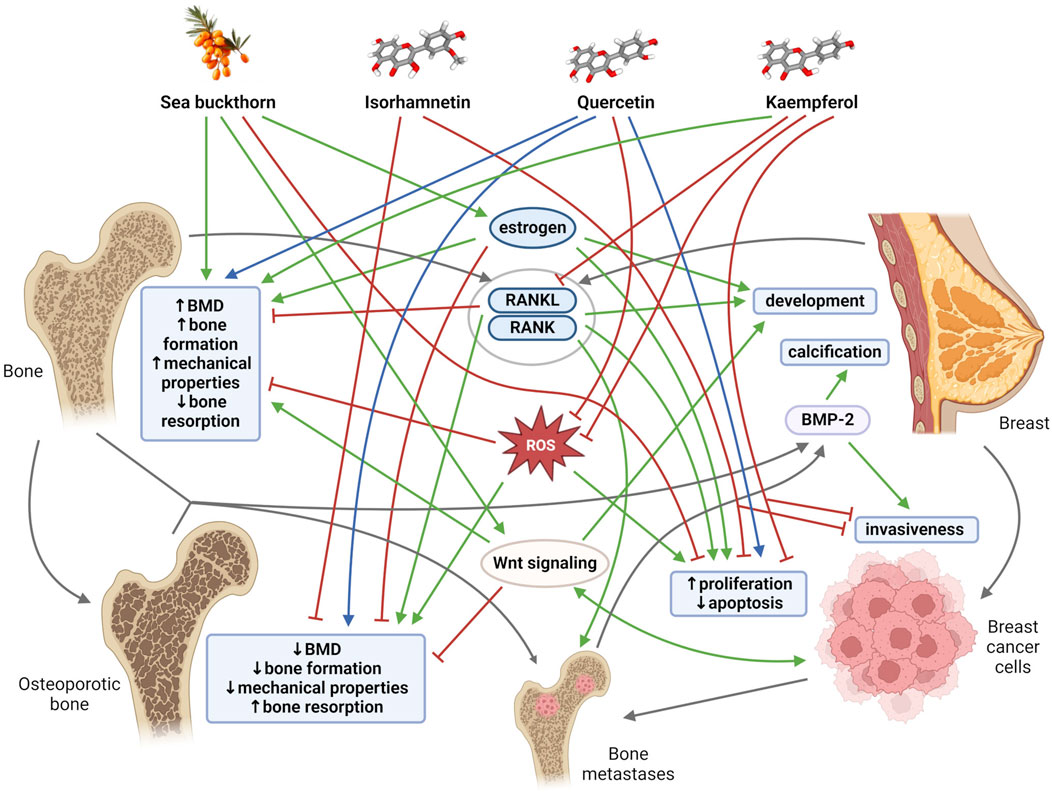
Figure 1. General mechanisms and links among bone tissue, breast tissue, sea buckthorn, its flavonoids (isorhamnetin, quercetin, kaempferol). Blunt red arrows indicate an inhibitory effect, sharp green arrows designate a stimulatory effect. Blue arrows show a dose-different effect. Gray arrows indicate the connection of individual mechanisms, molecules and structures. BMD, bone mineral density; BMP-2, bone morphogenetic protein-2; RANK, receptor activator of nuclear factor kappa β; RANKL, receptor activator of nuclear factor kappa β ligand; ROS, reactive oxygen species. Created with BioRender.com using chemical structures from PubChem (Kim et al., 2023); CIDs: 5281654, 5280343, 5280863, 122228.
Another clinical interrelationship between bone tissue and breast tissue is demonstrated by breast microcalcifications and breast cancer bone metastases (Antonacci et al., 2018). The presence of breast microcalcifications and localized deposits of hydroxyapatite in the breast tissue is actually considered an early mammographic sign of breast cancer (Johnson et al., 1999). Approximately 70%–80% of patients with advanced breast cancer experienced bone metastases (Chen et al., 2010), which seriously affect their quality of life and can lead to death. Moreover, osteoblasts and osteoclasts represent an important switch in tumor cell dormancy during bone metastasis (Haider et al., 2020; Dai et al., 2022). Several researchers have also demonstrated the expression of typical bone markers in breast cells, including predominantly bone morphogenetic proteins (BMPs) and Wnt signaling (Liu et al., 2008; Bramwell et al., 2014). In general, BMPs are cytokines belonging to the transforming growth factor (TGF)-β superfamily that play multiple functions during development and tissue homeostasis, including the regulation of bone homeostasis (Sánchez-Duffhues et al., 2015). Current studies have shown that BMPs may also be involved in breast tissue. They can support oncogenic behavior by affecting apoptosis, migration, invasion, and angiogenesis (Alarmo and Kallioniemi, 2010), initiate microcalcification (Liu et al., 2008), and promote the phenomenon of epithelial-mesenchymal transition (EMT) (Gonzalez and Medici, 2014). Canonical Wnt signaling activity supports bone formation (Turashvili et al., 2006), and it is also involved in several stages of mammary gland growth and differentiation and human breast cancer development (Howe and Brown, 2004). Additionally, it is also important to mention that the implication of other bone-derived factors (e.g., osteocalcin, fibroblast growth factor 23, sclerostin, lipocalin 2) in cancer biology has attracted research interest in recent years (Mansinho et al., 2019; Santiago-Sánchez et al., 2020; Martiniakova et al., 2023b), as they may be used as promising tumor biomarkers. Conversely, breast cancer cells are able to secrete various cytokines, including parathyroid hormone (PTH)-related protein, vascular endothelial growth factor (VEGF), RANKL, various interleukins (ILs) that serve as crucial bone modulators (Shemanko et al., 2016). These facts also contribute to a profound interplay between bone tissue and breast tissue.
Current pharmacological treatments for both osteoporosis and breast cancer often cause undesirable side effects; therefore, various natural metabolites, including those found in sea buckthorn, are being intensively researched to discover an alternative and effective treatment method with less harmful impacts (Li Y. et al., 2017; Martiniakova et al., 2020).
3 The impact of sea buckthorn and its flavonoids isorhamnetin, quercetin, and kaempferol on bone tissue health
Several studies have shown that sea buckthorn fatty acids significantly elevated levels of serum estrogen, TGF, insulin-like growth factor (IGF), BMD, cortical bone thickness, trabecular number, and bone mechanical properties in aged female rats (Liu et al., 2006b; Liu et al., 2006a). In this context, Yuan et al. (2022) found that sea buckthorn was able not only to increase BMD and estrogen levels, but also to raise levels of bone turnover markers (e.g., procollagen type 1N propeptide: P1NP, C-terminal telopeptide: CTX) and improve trabecular bone microstructure (relative bone volume, trabecular thickness) in ovariectomized (OVX) rats. Furthermore, it enhanced the efficacy of a traditional Chinese medicine QiangGuYin (used to treat osteoporosis) by inhibiting Casein kinase 2-interacting protein-1 (CKIP-1) and Notum expression through the Wnt/β-catenin pathway. Molecular docking analysis revealed that seven active components, including isorhamnetin, quercetin, and kaempferol, were able to potentially influence CKIP-1 and Notum (Yuan et al., 2022). However, this study did not provide important details about the material used (Supplementary Table 1). Moreover, the discovery of the ‘active’ metabolites of sea buckthorn was based only on the calculated results of molecular docking and was not followed by experimental studies. According to Park et al. (2022), an alcoholic extracts of sea buckthorn fruits and their fractions increased BMD and exerted the protective effects against cartilage damage and disturbed trabecular bone microarchitecture. Moreover, the extracts and their fractions stimulated the differentiation of murine mesenchymal stem cells into osteoblasts and elevated gene expression of osteogenic factors and markers, e.g., alkaline phosphatase (ALP), runt-related transcription factor 2 (RUNX2), osteopontin (OPN), osterix (OSX). Lee et al. (2023) pointed out the anti-osteoporotic impacts of triterpenoids from sea buckthorn fruit by promoting osteoblast differentiation from mesenchymal stem cells. However, the extracts used in the studies mentioned above would require better characterization. Relevant information related to this issue is presented in Supplementary Table 1; Figure 1.
There is a limited number of studies examining the effect of isorhamnetin in relation to bone damage. According to Yamaguchi et al. (2007), isorhamnetin inhibited PTH-stimulated osteoclastogenesis in mouse bone marrow cells and elevated PTH-decreased calcium content in femoral-diaphyseal tissue cultures. Moreover, isorhamnetin reduced osteoclast formation in bone marrow macrophages through inhibition of mitogen-activated protein kinase (MAPK), nuclear factor-kappa B (NF-κB), and protein kinase B (AKT) signaling (Zhou et al., 2019).
In contrast to isorhamnetin, more in vitro studies have shown the ability of quercetin to reduce osteoblast apoptosis, osteoclastogenesis, and oxidative stress (Woo et al., 2004; Yamaguchi et al., 2007; Tsuji et al., 2009; Yamaguchi and Weitzmann, 2011; Tripathi et al., 2015; Niu et al., 2020; Wong et al., 2020). On the contrary, quercetin significantly increased osteoblastogenesis, ALP activity, calcium content, expression of bone formation-associated proteins in mouse preosteoblastic MC3T3-E1 cells (Tripathi et al., 2015), rat osteoblast-like ROS 17/2.8 cells (Kim et al., 2007), human osteoblast-like MG-63 cells (Prouillet et al., 2004), and bone marrow mesenchymal stem cells (BMSCs) (Yuan et al., 2018; Feng et al., 2023). In some researches mentioned above, the effect of quercetin on NF-κB and Wnt/β-catenin signaling was also reported. Notably, higher doses of quercetin had either suppressive or decreased activity on osteoblast-specific gene expression, osteoblast growth and mineralization in several studies (Yamaguchi and Weitzmann, 2011; Wong et al., 2020). Furthermore, quercetin has an ability to bind to the estrogen receptor (ER) (Ross and Kasum, 2002) and influence osteoblast and osteoclast activity, as well as the expression and activity of various inflammatory cytokines, participating in bone remodeling (Wang et al., 2017). Numerous in vivo studies have established the protective effects of quercetin against bone loss through increased BMD, improved bone microarchitecture and bone strength, elevated bone growth, decreased bone resorption markers, and increased bone formation markers (Tsuji et al., 2009; Oršolić et al., 2018; Yuan et al., 2018; Niu et al., 2020; Sun et al., 2022; Feng et al., 2023). Yurteri et al. (2023) revealed that quercetin was also able to strengthen bone in both the early and late stages of fracture healing.
Several in vitro studies have shown that inhibitory effects of kaempferol on osteoclastogenesis may be linked to the downregulation of osteoclastogenic factors, such as RANKL, nuclear factor of activated T-cells cytoplasmic 1 (NFAT-c1), tumor necrosis factor receptor-associated factor 6 (TRAF6), c-Fos proto-oncogene, NF-κB signaling (Pang et al., 2006; Lee et al., 2014; Kim et al., 2018; Wong et al., 2019). On the other hand, conducted experiments have reported that kaempferol increased ALP activity and promoted osteogenic differentiation and mineralization in rat bone marrow mesenchymal stem cells (rBMSCs) via mediation of SOX2/miR-124-3p/PI3K/AKT/mTOR axis as well as in osteoblastic MC3T3-E1 cells by inducing autophagy and activating osteoblast differentiation markers such as RUNX2, OSX, collagen 1 (Kim I. R. et al., 2016; Xie et al., 2021; Gan et al., 2022). In addition, kaempferol could affect bone through the regulation of AKT serine/threonine kinase 1 (AKT1) and matrix metalloproteinase (MMP)-9 gene expressions, which are closely related to the pathogenesis of bone loss (Dong et al., 2024). Furthermore, kaempferol can modulate bone metabolism also through the ER (Jia et al., 2012). Tang et al. (2008) revealed that kaempferol activated ERβ-mediated estrogen response element (ERE) transcription in MG-63 cell line. In vivo studies have demonstrated that kaempferol can increase BMD and ALP activity, reduce bone turnover, and improve trabecular bone microarchitecture and bone strength in OVX rats (Trivedi et al., 2008; Nowak et al., 2017; Liu et al., 2021). Moreover, kaempferol was found to be able to ameliorate osteoporosis by raising C-X-C motif ligand 12 (CXCL12) expression and decreasing miR-10a-3p (Liu et al., 2021).
4 The impact of sea buckthorn and its flavonoids isorhamnetin, quercetin, and kaempferol on breast tissue health
Several in vitro studies have demonstrated the antitumor activity of sea buckthorn. Zhang et al. (2005) examined changes in apoptosis-related gene expression profiles in human breast carcinoma Bcap-37 cells induced by flavonoids from sea buckthorn seeds. These authors found that the expression of 32 apoptosis-related genes (e.g., IGFBP4, CTNNB1, CASP3, GADD34) was affected by flavonoid treatment. According to Wang et al. (2014), sea buckthorn procyanidins isolated from the seeds could induce apoptosis of human breast cancer MDA-MB-231 cells through fatty acid synthase inhibition. Indeed, high levels of this enzyme have been identified in cancer cells. Olsson et al. (2004) reported that sea buckthorn fruit extracts reduced the proliferation of breast cancer MCF-7 cells in a concentration-dependent manner. Similarly, Boivin et al. (2007) determined the inhibition of breast cancer (MCF-7 and MDA-MB-231) cell proliferation by sea buckthorn berry juice, as well as the suppression of tumor necrosis factor (TNF)-induced activation of the nuclear transcription factor NF-κB. A limitation of the studies mentioned above is the lack of characterization of the experimental material. In addition, many studies have determined a positive role of sea buckthorn oil in cancer treatment, including chemotherapy and radiotherapy (Olas, 2018; Olas et al., 2018). Table 1; Figure 1 summarize relevant information related to this issue.
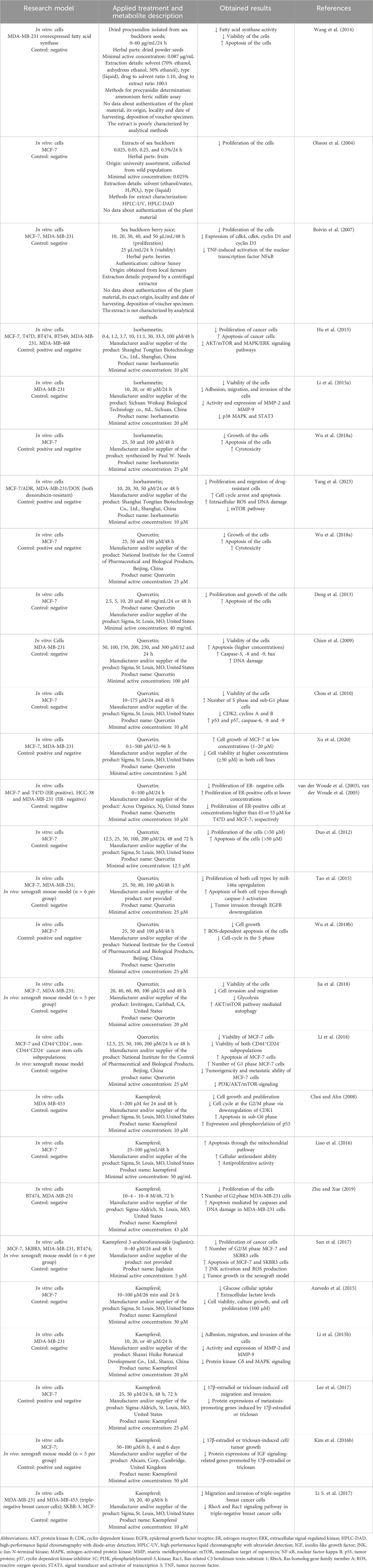
Table 1. In vitro and in vivo studies reflecting the potential of sea buckthorn and its flavonoids isorhamnetin, quercetin, and kaempferol against breast cancer.
Hu et al. (2015) revealed the anti-proliferative and pro-apoptotic effects of isorhamnetin in breast cancer mediated through inhibition of AKT/mTOR and MEK/ERK signaling pathways. Li et al. (2015a) discovered an inhibitory impact of isorhamnetin on the invasion of human breast carcinoma MDA-MB-231 cells by reducing the expression and activity of MMP-2 and MMP-9. This inhibition can be potentially linked to p38 MAPK and STAT3 suppression. Wu et al. (2018a) found that isorhamnetin dose-dependently inhibited the growth of human breast cancer MCF-7 cells, and exerted a strong cytotoxic effect through the reactive oxygen species (ROS)-dependent apoptosis pathway. According to Yang et al. (2023), isorhamnetin significantly reduced cell proliferation and migration and enhanced antitumor competence of doxorubicin (DOX) against resistant breast cancer cells both in vitro and in vivo, indicating its anti-breast tumor action as a DOX sensitizer.
Numerous in vitro studies have shown that quercetin at high concentrations exerts anti-proliferative impacts on breast cancer cells by arresting the cell cycle and inducing apoptosis (Chien et al., 2009; Chou et al., 2010; Deng et al., 2013). Conversely, lower doses of quercetin resulted in strong pro-proliferative effects (van der Woude et al., 2003; Xu et al., 2020). van der Woude et al. (2005) found that quercetin-induced stimulation of breast cancer cell proliferation was mediated by the ER. In ER+ (e.g., MCF-7) cells, lower concentrations of quercetin led to proliferative effects, while higher concentrations decreased cell viability. In ER− (e.g., MDA-MB-231) cells, reduced cell proliferation was observed even at low doses of quercetin. According to Duo et al. (2012), quercetin at higher concentrations was able to induce apoptosis through induction of BAX with concomitant inhibition of BCL-2 in human breast cancer MCF-7 cells and also through mitochondria- and caspase-3-dependent pathways in human breast carcinoma MDA-MB-231 cells (Chien et al., 2009). Tao et al. (2015) found that quercetin strongly inhibited cell proliferation in human breast cancer cells in a time- and dose-dependent fashion, which was associated with upregulation of miR-146a expression and induction of apoptosis through activation of caspase-3 and mitochondrial-dependent pathways. Wu et al. (2018b) pointed out the antitumor effects of quercetin through the induction of ROS-dependent apoptosis in MCF-7 cells. Jia et al. (2018) revealed that quercetin suppressed breast cancer progression by inhibiting cell motility and glycolysis via the induction of autophagy mediated by the AKT/mTOR pathway. Animal studies using tumor xenografts revealed that quercetin administration led to a reduction in tumor volume and decreased the markers associated with tumor growth and metastatic properties (Tao et al., 2015; Jia et al., 2018; Li et al., 2018).
In breast cancer, kaempferol can inhibit cell growth by destroying the cell cycle and induce apoptosis through p53 phosphorylation (Choi and Ahn, 2008), mitochondria-dependent pathway (Liao et al., 2016; Zhu and Xue, 2019), ROS/c-Jun N-terminal kinase (JNK) signaling pathway (Sun et al., 2017). The primary intracellular antioxidant mechanism of kaempferol involves scavenging the ROS accumulation and maintaining the activity of antioxidant enzymes at a physiological level. According to Azevedo et al. (2015), kaempferol at high concentrations strongly inhibited glucose uptake by breast carcinoma MCF-7 cells, leading to a significant decline in cell viability and proliferative capability. Li et al. (2015b) reported that kaempferol suppressed the invasion of human breast cancer MDA-MB-231 cells by downregulating the activity and expression of MMP-9. Kaempferol was also able to inhibit triclosan-induced EMT and metastatic behavior in breast cancer MCF-7 cells (Lee et al., 2017). Zhu and Xue (2019) demonstrated that the inhibitory effects of kaempferol on cell proliferation are greater in ER− (MDA-MB-231) cells compared to ER+ breast carcinoma (BT474) cells. According to Kim S. H. et al. (2016), kaempferol exerted anti-proliferative activity against breast cancer by suppressing triclosan- and estrogen-induced cancer progression by acting as an antagonist of ER and IGF-1R signaling in both cellular and xenograft breast cancer models. Li S. et al. (2017) revealed that a low dose of kaempferol inhibited the migration and invasion of triple-negative breast cancer (TNBC) cells by targeting the Rac1 or RhoA signaling pathway. In a mouse xenograft model, kaempferol inhibited the growth of breast cancer in vivo (Sun et al., 2017).
5 Methodological aspects and limitations of the reviewed studies
From the point of view of the interpretation and validity of the studies listed in Supplementary Table 1; Table 1, it is necessary to focus attention on several methodological aspects that should be fulfilled during pharmacological research (Heinrich et al., 2020). An important limitation of the studies analysing sea buckthorn extracts is the lack of characterization of the experimental material. Moreover, although relevant animal and cell models, negative and in several cases positive controls were used in those researches, no comparable healthy controls were available for cultured tumor cells to monitor metabolite selectivity. On the contrary, it is positive that studies showing antioxidant effects of metabolites used cell-based antioxidant assays, which, unlike chemical ones, are pharmacologically relevant. Other potential methodological risks arise from the structure and nature of flavonoids investigated. In general, polyphenols are categorized as Pan-Assay INterference compounds (PAINs or promiscuous inhibitors) and can interfere with the results of various assays. They also bind broadly to the protein targets of the assays themselves (Sheridan and Spelman, 2022). It has been revealed that polyphenols can self-associate to form colloids, which can affect their affinity for proteins. Flavonoids are more prone to aggregation than other phenolic metabolites under the conditions of the biochemical assays, with quercetin confirmed as promiscuous inhibitor (Pohjala and Tammela, 2012). Consequently, flavonoids interfere with colorimetric protein assays in a concentration- and structure-dependent manner (Singh et al., 2020) and affect other commonly used assays, such as MTT, by altering succinate dehydrogenase activity or directly interacting with MTT (Wang et al., 2010). In addition, such properties of flavonoids can be a potential source of misleading results in molecular docking analysis, therefore its findings should be verified in experimental studies. Thus, all circumstances, specifics, and risks of using individual methods should always be considered when analysing flavonoids and the failure to provide details on dealing with these methodological aspects can be considered as another limitation.
6 Conclusion
Botanical drugs have recently achieved remarkable success in promoting the treatment of various diseases. Sea buckthorn shows great medicinal and therapeutic potential due to its high content of bioactive metabolites with anti-proliferative, antioxidant, and anti-inflammatory activities. This review described the contemporary knowledge from in vitro and in vivo studies on the effect of sea buckthorn and its flavonoids isorhamnetin, quercetin, and kaempferol on bone and breast tissue health with an emphasis on osteoporosis and breast cancer, given their raising incidence in postmenopausal women. Conducted studies related to bone damage have demonstrated favorable impacts of all aforementioned bioactive metabolites on bone remodeling and mineralization, oxidative stress, bone microarchitecture and strength. In relation to breast cancer, sea buckthorn and its flavonoids inhibited cancer cell proliferation while inducing apoptosis, reduced tumor expansion and metastatic properties. In any case, it should be noted that several studies using extracts did not provide a sufficiently detailed definition of the study material or reports on the phytochemical analysis of the extracts as recommended by the best practice guidelines, indicating limitations and lower reliability of these studies. In addition, the known interference of flavonoids with commonly used assays (such as protein or MTT assays) should be always considered and may be another source of limitation. On the contrary, all these investigations used standard research models or cell lines and were published in peer-reviewed journals. By evaluating the available studies that analysed extracts and flavonoids mentioned in our manuscript, we can state that our hypothesis was confirmed, as all bioactive metabolites improved the impaired health status of both bone and breast tissues. In addition, some research has investigated the role of sea buckthorn extracts in reducing chemotherapy- and radiotherapy-related side effects, suggesting their potential benefits to improve overall treatment outcomes. Further in vitro studies and animal model studies that provide enough detailed information on the investigated material are needed, as well as clinical trials involving osteoporotic/non-osteoporotic and breast cancer/non-breast cancer patients, which may provide the key findings for identifying more effective therapies against bone and breast tissue damage. In this regard, the appropriate selection of the optimal dose and type of bioactive agent for inducing protective effects on bone and breast tissues in humans requires careful consideration and further validation in clinical trials.
Author contributions
MM: Conceptualization, Funding acquisition, Methodology, Supervision, Writing–original draft, Writing–review and editing. NP: Formal Analysis, Visualization, Writing–original draft. RB: Formal Analysis, Visualization, Writing–review and editing. AS: Formal Analysis, Visualization, Writing–review and editing. VK: Formal Analysis, Visualization, Writing–review and editing. VM: Formal Analysis, Visualization, Writing–review and editing. SC: Supervision, Writing–review and editing. RO: Funding acquisition, Supervision, Visualization, Writing–original draft, Writing–review and editing.
Funding
The author(s) declare that financial support was received for the research, authorship, and/or publication of this article. This work was supported by the Ministry of Education, Research, Development and Youth of the Slovak Republic, grant numbers VEGA 1/0416/22 and VEGA 1/0328/24.
Conflict of interest
The authors declare that the research was conducted in the absence of any commercial or financial relationships that could be construed as a potential conflict of interest.
Publisher’s note
All claims expressed in this article are solely those of the authors and do not necessarily represent those of their affiliated organizations, or those of the publisher, the editors and the reviewers. Any product that may be evaluated in this article, or claim that may be made by its manufacturer, is not guaranteed or endorsed by the publisher.
Supplementary material
The Supplementary Material for this article can be found online at: https://www.frontiersin.org/articles/10.3389/fphar.2024.1462823/full#supplementary-material
References
Alarmo, E.-L., and Kallioniemi, A. (2010). Bone morphogenetic proteins in breast cancer: dual role in tumourigenesis? Endocr. Relat. Cancer 17, R123–R139. doi:10.1677/ERC-09-0273
Antonacci, C., Scimeca, M., Bonfiglio, R., Fazi, S., and Bonanno, E. (2018). Breast and bone: a story of hidden similarities. Chronicles Oncol. 1. doi:10.33582/2638-4248/1001
Athonvarangkul, D., and Wysolmerski, J. J. (2023). Crosstalk within a brain-breast-bone axis regulates mineral and skeletal metabolism during lactation. Front. Physiol. 14, 1121579. doi:10.3389/fphys.2023.1121579
Azevedo, C., Correia-Branco, A., Araújo, J. R., Guimarães, J. T., Keating, E., and Martel, F. (2015). The chemopreventive effect of the dietary compound kaempferol on the MCF-7 human breast cancer cell line is dependent on inhibition of glucose cellular uptake. Nutr. Cancer 67, 504–513. doi:10.1080/01635581.2015.1002625
Boivin, D., Blanchette, M., Barrette, S., Moghrabi, A., and Béliveau, R. (2007). Inhibition of cancer cell proliferation and suppression of TNF-induced activation of NFkappaB by edible berry juice. Anticancer Res. 27, 937–948.
Bramwell, V. H., Tuck, A. B., Chapman, J.-A. W., Anborgh, P. H., Postenka, C. O., Al-Katib, W., et al. (2014). Assessment of osteopontin in early breast cancer: correlative study in a randomised clinical trial. Breast Cancer Res. 16, R8. doi:10.1186/bcr3600
Chen, Y.-C., Sosnoski, D. M., and Mastro, A. M. (2010). Breast cancer metastasis to the bone: mechanisms of bone loss. Breast Cancer Res. 12, 215. doi:10.1186/bcr2781
Chien, S.-Y., Wu, Y.-C., Chung, J.-G., Yang, J.-S., Lu, H.-F., Tsou, M.-F., et al. (2009). Quercetin-induced apoptosis acts through mitochondrial- and caspase-3-dependent pathways in human breast cancer MDA-MB-231 cells. Hum. Exp. Toxicol. 28, 493–503. doi:10.1177/0960327109107002
Cho, J.-H., Kim, J.-H., and Lee, H.-K. (2015). The relationship between breast density and bone mineral density after menopause. J. Phys. Ther. Sci. 27, 1243–1246. doi:10.1589/jpts.27.1243
Choi, E. J., and Ahn, W. S. (2008). Kaempferol induced the apoptosis via cell cycle arrest in human breast cancer MDA-MB-453 cells. Nutr. Res. Pract. 2, 322–325. doi:10.4162/nrp.2008.2.4.322
Chou, C.-C., Yang, J.-S., Lu, H.-F., Ip, S.-W., Lo, C., Wu, C.-C., et al. (2010). Quercetin-mediated cell cycle arrest and apoptosis involving activation of a caspase cascade through the mitochondrial pathway in human breast cancer MCF-7 cells. Arch. Pharm. Res. 33, 1181–1191. doi:10.1007/s12272-010-0808-y
Dai, R., Liu, M., Xiang, X., Xi, Z., and Xu, H. (2022). Osteoblasts and osteoclasts: an important switch of tumour cell dormancy during bone metastasis. J. Exp. Clin. Cancer Res. 41, 316. doi:10.1186/s13046-022-02520-0
Danielski, R., and Shahidi, F. (2024). Phenolic composition and bioactivities of sea buckthorn (Hippophae rhamnoides L.) fruit and seeds: an unconventional source of natural antioxidants in North America. J. Sci. Food Agric. 104, 5553–5564. doi:10.1002/jsfa.13386
Deng, X.-H., Song, H.-Y., Zhou, Y.-F., Yuan, G.-Y., and Zheng, F.-J. (2013). Effects of quercetin on the proliferation of breast cancer cells and expression of survivin in vitro. Exp. Ther. Med. 6, 1155–1158. doi:10.3892/etm.2013.1285
Dong, Q., Ren, G., Li, Y., and Hao, D. (2024). Network pharmacology analysis and experimental validation to explore the mechanism of kaempferol in the treatment of osteoporosis. Sci. Rep. 14, 7088. doi:10.1038/s41598-024-57796-3
Dudau, M., Vilceanu, A. C., Codrici, E., Mihai, S., Popescu, I. D., Albulescu, L., et al. (2021). Sea-buckthorn seed oil induces proliferation of both normal and dysplastic keratinocytes in basal conditions and under UVA irradiation. J. Pers. Med. 11, 278. doi:10.3390/jpm11040278
Duo, J., Ying, G.-G., Wang, G.-W., and Zhang, L. (2012). Quercetin inhibits human breast cancer cell proliferation and induces apoptosis via Bcl-2 and Bax regulation. Mol. Med. Rep. 5, 1453–1456. doi:10.3892/mmr.2012.845
Feng, L., Yang, Z., Hou, N., Wang, M., Lu, X., Li, Y., et al. (2023). Long non-coding RNA Malat1 increases the rescuing effect of quercetin on tnfα-impaired bone marrow stem cell osteogenesis and ovariectomy-induced osteoporosis. Int. J. Mol. Sci. 24, 5965. doi:10.3390/ijms24065965
Gan, L., Leng, Y., Min, J., Luo, X.-M., Wang, F., and Zhao, J. (2022). Kaempferol promotes the osteogenesis in rBMSCs via mediation of SOX2/miR-124-3p/PI3K/Akt/mTOR axis. Eur. J. Pharmacol. 927, 174954. doi:10.1016/j.ejphar.2022.174954
Gonzalez, D. M., and Medici, D. (2014). Signaling mechanisms of the epithelial-mesenchymal transition. Sci. Signal 7, re8. doi:10.1126/scisignal.2005189
Haider, M.-T., Smit, D. J., and Taipaleenmäki, H. (2020). The endosteal niche in breast cancer bone metastasis. Front. Oncol. 10, 335. doi:10.3389/fonc.2020.00335
He, N., Wang, Q., Huang, H., Chen, J., Wu, G., Zhu, M., et al. (2023). A comprehensive review on extraction, structure, detection, bioactivity, and metabolism of flavonoids from sea buckthorn (hippophae rhamnoides L.). J. Food Biochem. 2023, 1–27. doi:10.1155/2023/4839124
Heinrich, M., Appendino, G., Efferth, T., Fürst, R., Izzo, A. A., Kayser, O., et al. (2020). Best practice in research - overcoming common challenges in phytopharmacological research. J. Ethnopharmacol. 246, 112230. doi:10.1016/j.jep.2019.112230
Heinrich, M., Jalil, B., Abdel-Tawab, M., Echeverria, J., Kulić, Ž., McGaw, L. J., et al. (2022). Best Practice in the chemical characterisation of extracts used in pharmacological and toxicological research-The ConPhyMP-Guidelines. Front. Pharmacol. 13, 953205. doi:10.3389/fphar.2022.953205
Howe, L. R., and Brown, A. M. C. (2004). Wnt signaling and breast cancer. Cancer Biol. Ther. 3, 36–41. doi:10.4161/cbt.3.1.561
Hu, S., Huang, L., Meng, L., Sun, H., Zhang, W., and Xu, Y. (2015). Isorhamnetin inhibits cell proliferation and induces apoptosis in breast cancer via Akt and mitogen-activated protein kinase kinase signaling pathways. Mol. Med. Rep. 12, 6745–6751. doi:10.3892/mmr.2015.4269
Jaśniewska, A., and Diowksz, A. (2021). Wide spectrum of active compounds in sea buckthorn (hippophae rhamnoides) for disease prevention and food production. Antioxidants (Basel) 10, 1279. doi:10.3390/antiox10081279
Jia, L., Huang, S., Yin, X., Zan, Y., Guo, Y., and Han, L. (2018). Quercetin suppresses the mobility of breast cancer by suppressing glycolysis through Akt-mTOR pathway mediated autophagy induction. Life Sci. 208, 123–130. doi:10.1016/j.lfs.2018.07.027
Jia, M., Nie, Y., Cao, D.-P., Xue, Y.-Y., Wang, J.-S., Zhao, L., et al. (2012). Potential antiosteoporotic agents from plants: a comprehensive review. Evid. Based Complement. Altern. Med. 2012, 364604. doi:10.1155/2012/364604
Johnson, J. M., Dalton, R. R., Wester, S. M., Landercasper, J., and Lambert, P. J. (1999). Histological correlation of microcalcifications in breast biopsy specimens. Arch. Surg. 134, 712–715. doi:10.1001/archsurg.134.7.712
Kim, C.-J., Shin, S.-H., Kim, B.-J., Kim, C.-H., Kim, J.-H., Kang, H.-M., et al. (2018). The effects of kaempferol-inhibited autophagy on osteoclast formation. Int. J. Mol. Sci. 19, 125. doi:10.3390/ijms19010125
Kim, D.-S., Takai, H., Arai, M., Araki, S., Mezawa, M., Kawai, Y., et al. (2007). Effects of quercetin and quercetin 3-glucuronide on the expression of bone sialoprotein gene. J. Cell Biochem. 101, 790–800. doi:10.1002/jcb.21233
Kim, I. R., Kim, S.-E., Baek, H.-S., Kim, B.-J., Kim, C.-H., Chung, I.-K., et al. (2016). The role of kaempferol-induced autophagy on differentiation and mineralization of osteoblastic MC3T3-E1 cells. BMC Complementary Altern. Med. 16, 333. doi:10.1186/s12906-016-1320-9
Kim, S., Chen, J., Cheng, T., Gindulyte, A., He, J., He, S., et al. (2023). PubChem 2023 update. Nucleic Acids Res. 51, D1373–D1380. doi:10.1093/nar/gkac956
Kim, S. H., Hwang, K.-A., and Choi, K.-C. (2016). Treatment with kaempferol suppresses breast cancer cell growth caused by estrogen and triclosan in cellular and xenograft breast cancer models. J. Nutr. Biochem. 28, 70–82. doi:10.1016/j.jnutbio.2015.09.027
Lee, D., Park, K., Hong, J.-H., Kim, S., Park, K.-M., and Kim, K. H. (2023). Anti-osteoporosis effects of triterpenoids from the fruit of sea buckthorn (Hippophae rhamnoides) through the promotion of osteoblast differentiation in mesenchymal stem cells, C3H10T1/2. Archives Pharmacal Res. 46, 771–781. doi:10.1007/s12272-023-01468-9
Lee, G.-A., Choi, K.-C., and Hwang, K.-A. (2017). Kaempferol, a phytoestrogen, suppressed triclosan-induced epithelial-mesenchymal transition and metastatic-related behaviors of MCF-7 breast cancer cells. Environ. Toxicol. Pharmacol. 49, 48–57. doi:10.1016/j.etap.2016.11.016
Lee, W.-S., Lee, E.-G., Sung, M.-S., and Yoo, W.-H. (2014). Kaempferol inhibits IL-1β-stimulated, RANKL-mediated osteoclastogenesis via downregulation of MAPKs, c-Fos, and NFATc1. Inflammation 37, 1221–1230. doi:10.1007/s10753-014-9849-6
Li, C., Yang, D., Zhao, Y., Qiu, Y., Cao, X., Yu, Y., et al. (2015a). Inhibitory effects of isorhamnetin on the invasion of human breast carcinoma cells by downregulating the expression and activity of matrix metalloproteinase-2/9. Nutr. Cancer 67, 1191–1200. doi:10.1080/01635581.2015.1073763
Li, C., Zhao, Y., Yang, D., Yu, Y., Guo, H., Zhao, Z., et al. (2015b). Inhibitory effects of kaempferol on the invasion of human breast carcinoma cells by downregulating the expression and activity of matrix metalloproteinase-9. Biochem. Cell Biol. 93, 16–27. doi:10.1139/bcb-2014-0067
Li, S., Yan, T., Deng, R., Jiang, X., Xiong, H., Wang, Y., et al. (2017). Low dose of kaempferol suppresses the migration and invasion of triple-negative breast cancer cells by downregulating the activities of RhoA and Rac1. OncoTargets Ther. 10, 4809–4819. doi:10.2147/OTT.S140886
Li, X., Zhou, N., Wang, J., Liu, Z., Wang, X., Zhang, Q., et al. (2018). Quercetin suppresses breast cancer stem cells (CD44+/CD24−) by inhibiting the PI3K/Akt/mTOR-signaling pathway. Life Sci. 196, 56–62. doi:10.1016/j.lfs.2018.01.014
Li, Y., Li, S., Meng, X., Gan, R.-Y., Zhang, J.-J., and Li, H.-B. (2017). Dietary natural products for prevention and treatment of breast cancer. Nutrients 9, 728. doi:10.3390/nu9070728
Liao, W., Chen, L., Ma, X., Jiao, R., Li, X., and Wang, Y. (2016). Protective effects of kaempferol against reactive oxygen species-induced hemolysis and its antiproliferative activity on human cancer cells. Eur. J. Med. Chem. 114, 24–32. doi:10.1016/j.ejmech.2016.02.045
Liu, B., Yuan, B., and Guo, X. (2006a). Experimental study on effect of tiangui gengnian soft capsule on aged female rats with osteoporosis. Zhongguo Zhong Xi Yi Jie He Za Zhi 26, 135–139.
Liu, B., Yuan, B.-X., Guo, X., Wei, X.-Z., Zhao, L.-M., Chen, D., et al. (2006b). Experimental study on the effect of tiangui gengnian capsule on the aged female rats ostepoprosis. Zhong Yao Cai 29, 803–806.
Liu, F., Bloch, N., Bhushan, K. R., De Grand, A. M., Tanaka, E., Solazzo, S., et al. (2008). Humoral bone morphogenetic protein 2 is sufficient for inducing breast cancer microcalcification. Mol. Imaging 7, 7290.2008.00018–186. doi:10.2310/7290.2008.00018a
Liu, H., Yi, X., Tu, S., Cheng, C., and Luo, J. (2021). Kaempferol promotes BMSC osteogenic differentiation and improves osteoporosis by downregulating miR-10a-3p and upregulating CXCL12. Mol. Cell Endocrinol. 520, 111074. doi:10.1016/j.mce.2020.111074
Mansinho, A., Ferreira, A. R., Casimiro, S., Alho, I., Vendrell, I., Costa, A. L., et al. (2019). Levels of circulating fibroblast growth factor 23 (FGF23) and prognosis in cancer patients with bone metastases. Int. J. Mol. Sci. 20, E695. doi:10.3390/ijms20030695
Martiniakova, M., Babikova, M., and Omelka, R. (2020). Pharmacological agents and natural compounds: available treatments for osteoporosis. J. Physiol. Pharmacol. 71. doi:10.26402/jpp.2020.3.01
Martiniakova, M., Biro, R., Penzes, N., Sarocka, A., Kovacova, V., Mondockova, V., et al. (2024). Links among obesity, type 2 diabetes mellitus, and osteoporosis: bone as a target. Int. J. Mol. Sci. 25, 4827. doi:10.3390/ijms25094827
Martiniakova, M., Kovacova, V., Mondockova, V., Zemanova, N., Babikova, M., Biro, R., et al. (2023a). Honey: a promising therapeutic supplement for the prevention and management of osteoporosis and breast cancer. Antioxidants (Basel) 12, 567. doi:10.3390/antiox12030567
Martiniakova, M., Mondockova, V., Biro, R., Kovacova, V., Babikova, M., Zemanova, N., et al. (2023b). The link between bone-derived factors osteocalcin, fibroblast growth factor 23, sclerostin, lipocalin 2 and tumor bone metastasis. Front. Endocrinol. (Lausanne) 14, 1113547. doi:10.3389/fendo.2023.1113547
Muhammad, A., Mada, S. B., Malami, I., Forcados, G. E., Erukainure, O. L., Sani, H., et al. (2018). Postmenopausal osteoporosis and breast cancer: the biochemical links and beneficial effects of functional foods. Biomed. Pharmacother. 107, 571–582. doi:10.1016/j.biopha.2018.08.018
Niu, Y., Yang, Y., Xiao, X., Sun, Y., Zhou, Y., Zhang, Y., et al. (2020). Quercetin prevents bone loss in hindlimb suspension mice via stanniocalcin 1-mediated inhibition of osteoclastogenesis. Acta Pharmacol. Sin. 41, 1476–1486. doi:10.1038/s41401-020-00509-z
Nowak, B., Matuszewska, A., Nikodem, A., Filipiak, J., Landwójtowicz, M., Sadanowicz, E., et al. (2017). Oral administration of kaempferol inhibits bone loss in rat model of ovariectomy-induced osteopenia. Pharmacol. Rep. 69, 1113–1119. doi:10.1016/j.pharep.2017.05.002
Olas, B. (2018). The beneficial health aspects of sea buckthorn (Elaeagnus rhamnoides (L.) A.Nelson) oil. J. Ethnopharmacol. 213, 183–190. doi:10.1016/j.jep.2017.11.022
Olas, B., Skalski, B., and Ulanowska, K. (2018). The anticancer activity of sea buckthorn [elaeagnus rhamnoides (L.) A. Nelson]. Front. Pharmacol. 9, 232. doi:10.3389/fphar.2018.00232
Olsson, M. E., Gustavsson, K.-E., Andersson, S., Nilsson, A., and Duan, R.-D. (2004). Inhibition of cancer cell proliferation in vitro by fruit and berry extracts and correlations with antioxidant levels. J. Agric. Food Chem. 52, 7264–7271. doi:10.1021/jf030479p
Oršolić, N., Jeleč, Ž., Nemrava, J., Balta, V., Gregorović, G., and Jeleč, D. (2018). Effect of quercetin on bone mineral status and markers of bone turnover in retinoic acid-induced osteoporosis. Pol. J. Food Nutr. Sci. 68, 149–162. doi:10.1515/pjfns-2017-0023
Pang, J. L., Ricupero, D. A., Huang, S., Fatma, N., Singh, D. P., Romero, J. R., et al. (2006). Differential activity of kaempferol and quercetin in attenuating tumor necrosis factor receptor family signaling in bone cells. Biochem. Pharmacol. 71, 818–826. doi:10.1016/j.bcp.2005.12.023
Park, K. H., Hong, J.-H., Kim, S.-H., Kim, J.-C., Kim, K. H., and Park, K.-M. (2022). Anti-osteoporosis effects of the fruit of sea buckthorn (hippophae rhamnoides) through promotion of osteogenic differentiation in ovariectomized mice. Nutrients 14, 3604. doi:10.3390/nu14173604
Patel, C. A., Divakar, K., Santani, D., Solanki, H. K., and Thakkar, J. H. (2012). Remedial prospective of hippophae rhamnoides linn. (sea buckthorn). ISRN Pharmacol. 2012, 436857. doi:10.5402/2012/436857
Pohjala, L., and Tammela, P. (2012). Aggregating behavior of phenolic compounds--a source of false bioassay results? Molecules 17, 10774–10790. doi:10.3390/molecules170910774
Prouillet, C., Mazière, J.-C., Mazière, C., Wattel, A., Brazier, M., and Kamel, S. (2004). Stimulatory effect of naturally occurring flavonols quercetin and kaempferol on alkaline phosphatase activity in MG-63 human osteoblasts through ERK and estrogen receptor pathway. Biochem. Pharmacol. 67, 1307–1313. doi:10.1016/j.bcp.2003.11.009
Ren, R., Li, N., Su, C., Wang, Y., Zhao, X., Yang, L., et al. (2020). The bioactive components as well as the nutritional and health effects of sea buckthorn. RSC Adv. 10, 44654–44671. doi:10.1039/D0RA06488B
Ross, J. A., and Kasum, C. M. (2002). DIETARY FLAVONOIDS: bioavailability, metabolic effects, and safety. Annu. Rev. Nutr. 22, 19–34. doi:10.1146/annurev.nutr.22.111401.144957
Salari, N., Ghasemi, H., Mohammadi, L., Behzadi, M. H., Rabieenia, E., Shohaimi, S., et al. (2021). The global prevalence of osteoporosis in the world: a comprehensive systematic review and meta-analysis. J. Orthop. Surg. Res. 16, 609. doi:10.1186/s13018-021-02772-0
Sánchez-Duffhues, G., Hiepen, C., Knaus, P., and Ten Dijke, P. (2015). Bone morphogenetic protein signaling in bone homeostasis. Bone 80, 43–59. doi:10.1016/j.bone.2015.05.025
Santiago-Sánchez, G. S., Pita-Grisanti, V., Quiñones-Díaz, B., Gumpper, K., Cruz-Monserrate, Z., and Vivas-Mejía, P. E. (2020). Biological functions and therapeutic potential of lipocalin 2 in cancer. Int. J. Mol. Sci. 21, 4365. doi:10.3390/ijms21124365
Shemanko, C. S., Cong, Y., and Forsyth, A. (2016). What is breast in the bone? Int. J. Mol. Sci. 17, 1764. doi:10.3390/ijms17101764
Sheridan, R., and Spelman, K. (2022). Polyphenolic promiscuity, inflammation-coupled selectivity: whether PAINs filters mask an antiviral asset. Front. Pharmacol. 13, 909945. doi:10.3389/fphar.2022.909945
Sigl, V., and Penninger, J. M. (2014). RANKL/RANK - from bone physiology to breast cancer. Cytokine Growth Factor Rev. 25, 205–214. doi:10.1016/j.cytogfr.2014.01.002
Singh, R., Lu, R., and Hu, M. (2020). Flavonoids interference in common protein assays: effect of position and degree of hydroxyl substitution. Anal. Biochem. 597, 113644. doi:10.1016/j.ab.2020.113644
Stingl, J. (2011). Estrogen and progesterone in normal mammary gland development and in cancer. Horm. Cancer 2, 85–90. doi:10.1007/s12672-010-0055-1
Stochmal, A., Rolnik, A., Skalski, B., Zuchowski, J., and Olas, B. (2022). Antiplatelet and anticoagulant activity of isorhamnetin and its derivatives isolated from sea buckthorn berries, measured in whole blood. Molecules 27, 4429. doi:10.3390/molecules27144429
Sun, J., Pan, Y., Li, X., Wang, L., Liu, M., Tu, P., et al. (2022). Quercetin attenuates osteoporosis in orchiectomy mice by regulating glucose and lipid metabolism via the GPRC6A/AMPK/mTOR signaling pathway. Front. Endocrinol. 13, 849544. doi:10.3389/fendo.2022.849544
Sun, Z.-L., Dong, J.-L., and Wu, J. (2017). Juglanin induces apoptosis and autophagy in human breast cancer progression via ROS/JNK promotion. Biomed. Pharmacother. 85, 303–312. doi:10.1016/j.biopha.2016.11.030
Tang, X., Zhu, X., Liu, S., Nicholson, R. C., and Ni, X. (2008). Phytoestrogens induce differential estrogen receptor beta-mediated responses in transfected MG-63 cells. Endocr 34, 29–35. doi:10.1007/s12020-008-9099-1
Tao, S., He, H., and Chen, Q. (2015). Quercetin inhibits proliferation and invasion acts by up-regulating miR-146a in human breast cancer cells. Mol. Cell Biochem. 402, 93–100. doi:10.1007/s11010-014-2317-7
Tripathi, G., Raja, N., and Yun, H. S. (2015). Effect of direct loading of phytoestrogens into the calcium phosphate scaffold on osteoporotic bone tissue regeneration. J. Mat. Chem. B 3, 8694–8703. doi:10.1039/C5TB01574J
Trivedi, R., Kumar, S., Kumar, A., Siddiqui, J. A., Swarnkar, G., Gupta, V., et al. (2008). Kaempferol has osteogenic effect in ovariectomized adult Sprague-Dawley rats. Mol. Cell Endocrinol. 289, 85–93. doi:10.1016/j.mce.2008.02.027
Tsuji, M., Yamamoto, H., Sato, T., Mizuha, Y., Kawai, Y., Taketani, Y., et al. (2009). Dietary quercetin inhibits bone loss without effect on the uterus in ovariectomized mice. J. Bone Min. Metab. 27, 673–681. doi:10.1007/s00774-009-0088-0
Turashvili, G., Bouchal, J., Burkadze, G., and Kolar, Z. (2006). Wnt signaling pathway in mammary gland development and carcinogenesis. Pathobiology 73, 213–223. doi:10.1159/000098207
van der Woude, H., Gliszczyńska-Swigło, A., Struijs, K., Smeets, A., Alink, G. M., and Rietjens, I. M. C. M. (2003). Biphasic modulation of cell proliferation by quercetin at concentrations physiologically relevant in humans. Cancer Lett. 200, 41–47. doi:10.1016/s0304-3835(03)00412-9
van der Woude, H., Ter Veld, M. G. R., Jacobs, N., van der Saag, P. T., Murk, A. J., and Rietjens, I. M. C. M. (2005). The stimulation of cell proliferation by quercetin is mediated by the estrogen receptor. Mol. Nutr. Food Res. 49, 763–771. doi:10.1002/mnfr.200500036
Wang, P., Henning, S. M., and Heber, D. (2010). Limitations of MTT and MTS-based assays for measurement of antiproliferative activity of green tea polyphenols. PLoS One 5, e10202. doi:10.1371/journal.pone.0010202
Wang, T., Liu, Q., Tjhioe, W., Zhao, J., Lu, A., Zhang, G., et al. (2017). Therapeutic potential and outlook of alternative medicine for osteoporosis. Curr. Drug Targets 18, 1051–1068. doi:10.2174/1389450118666170321105425
Wang, Y., Nie, F., Ouyang, J., Wang, X., and Ma, X. (2014). Inhibitory effects of sea buckthorn procyanidins on fatty acid synthase and MDA-MB-231 cells. Tumour Biol. 35, 9563–9569. doi:10.1007/s13277-014-2233-1
Wong, S. K., Chin, K.-Y., and Ima-Nirwana, S. (2019). The osteoprotective effects of kaempferol: the evidence from in vivo and in vitro studies. Drug Des. Devel Ther. 13, 3497–3514. doi:10.2147/DDDT.S227738
Wong, S. K., Chin, K.-Y., and Ima-Nirwana, S. (2020). Quercetin as an agent for protecting the bone: a review of the current evidence. Int. J. Mol. Sci. 21, 6448. doi:10.3390/ijms21176448
Woo, J.-T., Nakagawa, H., Notoya, M., Yonezawa, T., Udagawa, N., Lee, I.-S., et al. (2004). Quercetin suppresses bone resorption by inhibiting the differentiation and activation of osteoclasts. Biol. Pharm. Bull. 27, 504–509. doi:10.1248/bpb.27.504
World Health Organization International Agency for Research on Cancer (2024). Cancer today. Available at: https://gco.iarc.who.int/today/(Accessed July 10, 2024).
Wu, Q., Kroon, P. A., Shao, H., Needs, P. W., and Yang, X. (2018a). Differential effects of quercetin and two of its derivatives, isorhamnetin and isorhamnetin-3-glucuronide, in inhibiting the proliferation of human breast-cancer MCF-7 cells. J. Agric. Food Chem. 66, 7181–7189. doi:10.1021/acs.jafc.8b02420
Wu, Q., Needs, P. W., Lu, Y., Kroon, P. A., Ren, D., and Yang, X. (2018b). Different antitumor effects of quercetin, quercetin-3′-sulfate and quercetin-3-glucuronide in human breast cancer MCF-7 cells. Food Funct. 9, 1736–1746. doi:10.1039/C7FO01964E
Xie, B., Zeng, Z., Liao, S., Zhou, C., Wu, L., and Xu, D. (2021). Kaempferol ameliorates the inhibitory activity of dexamethasone in the osteogenesis of mc3t3-E1 cells by JNK and p38-MAPK pathways. Front. Pharmacol. 12, 739326. doi:10.3389/fphar.2021.739326
Xu, Z., Zhao, D., Zheng, X., Huang, B., Xia, X., and Pan, X. (2020). Quercetin exerts bidirectional regulation effects on the efficacy of tamoxifen in estrogen receptor-positive breast cancer therapy: an in vitro study. Environ. Toxicol. 35, 1179–1193. doi:10.1002/tox.22983
Yamaguchi, M., Hamamoto, R., Uchiyama, S., and Ishiyama, K. (2007). Effects of flavonoid on calcium content in femoral tissue culture and parathyroid hormone-stimulated osteoclastogenesis in bone marrow culture in vitro. Mol. Cell Biochem. 303, 83–88. doi:10.1007/s11010-007-9458-x
Yamaguchi, M., and Weitzmann, M. N. (2011). Quercetin, a potent suppressor of NF-κB and Smad activation in osteoblasts. Int. J. Mol. Med. 28, 521–525. doi:10.3892/ijmm.2011.749
Yang, T., Xiao, Y., Liu, S., Luo, F., Tang, D., Yu, Y., et al. (2023). Isorhamnetin induces cell cycle arrest and apoptosis by triggering DNA damage and regulating the AMPK/mTOR/p70S6K signaling pathway in doxorubicin-resistant breast cancer. Phytomedicine 114, 154780. doi:10.1016/j.phymed.2023.154780
Yuan, Y.-F., Wang, S., Zhou, H., Tang, B.-B., Liu, Y., Huang, H., et al. (2022). Exploratory study of sea buckthorn enhancing QiangGuYin efficacy by inhibiting CKIP-1 and Notum activating the Wnt/β-catenin signaling pathway and analysis of active ingredients by molecular docking. Front. Pharmacol. 13, 994995. doi:10.3389/fphar.2022.994995
Yuan, Z., Min, J., Zhao, Y., Cheng, Q., Wang, K., Lin, S., et al. (2018). Quercetin rescued TNF-alpha-induced impairments in bone marrow-derived mesenchymal stem cell osteogenesis and improved osteoporosis in rats. Am. J. Transl. Res. 10, 4313–4321. Available at: https://www.ncbi.nlm.nih.gov/pmc/articles/PMC6325508/(Accessed June 20, 2024).
Yurteri, A., Yildirim, A., Çelik, Z. E., Vatansev, H., and Durmaz, M. S. (2023). The effect of quercetin on bone healing in an experimental rat model. Jt. Dis. Relat. Surg. 34, 365–373. doi:10.52312/jdrs.2023.870
Zhang, P., Mao, Y.-C., Sun, B., Qian, M., and Qu, W.-J. (2005). Changes in apoptosis-related genes expression profile in human breast carcinoma cell line Bcap-37 induced by flavonoids from seed residues of Hippophae Rhamnoides L. Ai Zheng 24, 454–460.
Zhou, F., Mei, J., Yuan, K., Han, X., Qiao, H., and Tang, T. (2019). Isorhamnetin attenuates osteoarthritis by inhibiting osteoclastogenesis and protecting chondrocytes through modulating reactive oxygen species homeostasis. J. Cell Mol. Med. 23, 4395–4407. doi:10.1111/jcmm.14333
Keywords: sea buckthorn, isorhamnetin, quercetin, kaempferol, bone tissue, breast tissue, osteoporosis, breast cancer
Citation: Martiniakova M, Penzes N, Biro R, Sarocka A, Kovacova V, Mondockova V, Ciernikova S and Omelka R (2024) Sea buckthorn and its flavonoids isorhamnetin, quercetin, and kaempferol favorably influence bone and breast tissue health. Front. Pharmacol. 15:1462823. doi: 10.3389/fphar.2024.1462823
Received: 10 July 2024; Accepted: 24 September 2024;
Published: 09 October 2024.
Edited by:
Michael Heinrich, University College London, United KingdomReviewed by:
Maria Dudau, Victor Babes National Institute of Pathology (INCDVB), RomaniaCristiana Tanase, Victor Babes National Institute of Pathology (INCDVB), Romania
Copyright © 2024 Martiniakova, Penzes, Biro, Sarocka, Kovacova, Mondockova, Ciernikova and Omelka. This is an open-access article distributed under the terms of the Creative Commons Attribution License (CC BY). The use, distribution or reproduction in other forums is permitted, provided the original author(s) and the copyright owner(s) are credited and that the original publication in this journal is cited, in accordance with accepted academic practice. No use, distribution or reproduction is permitted which does not comply with these terms.
*Correspondence: Monika Martiniakova, bW1hcnRpbmlha292YUB1a2Yuc2s=; Radoslav Omelka, cm9tZWxrYUB1a2Yuc2s=
†These authors have contributed equally to this work