- 1Department of Thoracic Surgery, Organ Transplantation Center, The First Hospital of Jilin University, Changchun, Jilin, China
- 2Department of Respiratory and Critical Care Medicine, The Second Hospital of Hebei Medical University, Shijiazhuang, Hebei, China
- 3Department of Vaccination Clinic, Xiangyang Center for Disease Control and Prevention, Xiangyang, Hubei, China
Esophageal cancer is a common and lethal digestive system malignancy, and both treatment efficacy and patient survival rates face significant challenges. In recent years, exosomes have emerged as crucial mediators of intercellular communication, demonstrating tremendous clinical potential, particularly in the diagnosis, treatment, and prognostic evaluation of esophageal cancer. These exosomes not only serve as biomarkers for early diagnosis and prognosis but also modulate tumor growth, metastasis, and drug resistance by delivering bioactive molecules. Importantly, exosomes can act as carriers for esophageal cancer-related therapeutic agents, optimizing gene therapy strategies to enhance efficacy while reducing toxicity and side effects. Despite facing challenges in clinical applications such as purification, enrichment, and standardization of analytical methods, exosomes maintain broad prospects for application in esophageal cancer treatment, with the potential to significantly improve patient outcomes and quality of life. This review focuses on the innovative role of exosomes in the early diagnosis of esophageal cancer, exploring their application value and safety in disease monitoring and assessment of treatment response. Furthermore, this study outlines the challenges and limitations of transitioning exosome research from basic studies to clinical applications, as well as potential solutions and future research directions to address these obstacles.
1 Introduction
According to statistics from the International Agency for Research on Cancer of the World Health Organization (Sung et al., 2021), there were approximately 604,100 new cases of esophageal cancer (EC) worldwide in 2020, accounting for 3.1% of all cancer cases and ranking 7th for all cancers. The estimated number of deaths from EC in 2020 was approximately 544,076, accounting for 5.5% of all cancer-related deaths and ranking 6th sixth among malignant tumors. More concerning is that over half of the global EC cases occur in China, accounting for 53.7% of new cases and 55.3% of deaths (Zhou et al., 2019). Although in the latest global cancer statistics report (Bray et al., 2024), the incidence rate of EC had dropped to 11th place, it remains the 7th highest mortality cause of mortality worldwide. Globally, esophageal squamous cell carcinoma (ESCC) is the most common subtype, accounting for approximately 85% of all cases. Esophageal adenocarcinoma (EAC) accounts for approximately 14% (Morgan et al., 2022). There are significant differences in the incidence rates of EC among different populations and regions. Generally, the incidence rate in men is higher than that in women (Chen et al., 2023a). In terms of geographical distribution, the incidence rate of EAC is the highest in Northern Europe and North America, whereas that of ESCC is higher in South Africa, Central and South Asia, and East Asia (Dawsey and Duits, 2023; GBD 2017 Oesophageal Cancer Collaborators, 2020). The main pathological type of EC in China is ESCC, with a 5-year survival rate of approximately 30% and a poor prognosis (Li et al., 2017).
Precancerous EC lesions exhibit obvious pathological features, progressing slowly from early precancerous lesions to invasive cancers (Gao et al., 2023). A follow-up study of 1,183 patients with precancerous lesions of EC found that only 88 patients (7.44%) progressed to advanced tumors (Li et al., 2023a). This characteristic provides an opportunity for the early diagnosis and treatment of EC. Unfortunately, the onset of EC is often insidious. Patients with precancerous lesions in the early stages (stage 0, I, and II) may not exhibit obvious symptoms. However, by the time a diagnosis is established, the disease often progresses to an advanced stage, resulting in missed opportunities for optimal surgical treatment. Therefore, it is crucial to develop a highly sensitive and specific diagnostic tool for the screening and early diagnosis of EC in high-risk populations.
Liquid biopsy is an innovate approach for the diagnosis of diseases including cancer by examining the biomarkers in blood or other body fluids. Liquid biopsy has garnered significant attention in global cancer research (Markou et al., 2022). The technology was recognized as one of the top ten breakthrough technologies by the Massachusetts Institute of Technology Review magazine in 2015 because of its speed, low invasiveness, and good patient compliance. The significance of liquid biopsy in the early diagnosis, drug monitoring, and prognostic assessment of solid tumors has become increasingly evident.
Traditional tumor liquid biopsy is primarily divided into two categories: circulating tumor cells and circulating tumor nucleic acids. However, both approaches have drawbacks that include low detection rate, low specificity, and significant individual variability (Zhu et al., 2023a). The importance of extracellular vesicle, which encompass different subtypes such as exosomes (EVs), was highlighted in 2013 with the awarding of the Nobel Prize in Physiology or Medicine, thereby propelling research in this field. Among extracellular vesicle, EVs are a specific subtype, typically ranging from 30 to 150 nm in size, with a protective phospholipid bilayer membrane rich in membrane proteins that enhances the stability of the bioactive molecules they carry, such as DNA, RNA, proteins, and metabolites (Kimiz-Gebologlu and Oncel, 2022) (Arya et al., 2024). Due to these characteristics, EVs are particularly well-suited for biomarker research and hold significant promise in liquid biopsy assays.
Physiologically, exosomes are nanoscale vesicles secreted by various cells. Their payloads of various biological macromolecules that include proteins, nucleic acids, and lipids confer critical roles of EVs in the development and progression of tumors, particularly in the formation of the tumor microenvironment, facilitating communication among tumor cells and interactions between tumors and host cells (Preethi et al., 2022). Notably, EVs can circulate throughout the body via blood and other bodily fluids, and thus are a potential source of non-invasive tumor markers (Li et al., 2021a).
This comprehensive review addresses the diagnosis and treatment of EC using exosomes based on liquid biopsy (Figure 1). The review delves into the relationship between liquid biopsy and exosomes, details the primary isolation and detection techniques for exosomes, and discusses the latest research on exosomes in the early diagnosis and treatment of EC in recent years. Future clinical applications of exosomes are discussed and research directions, limitations, and technical challenges are analyzed.
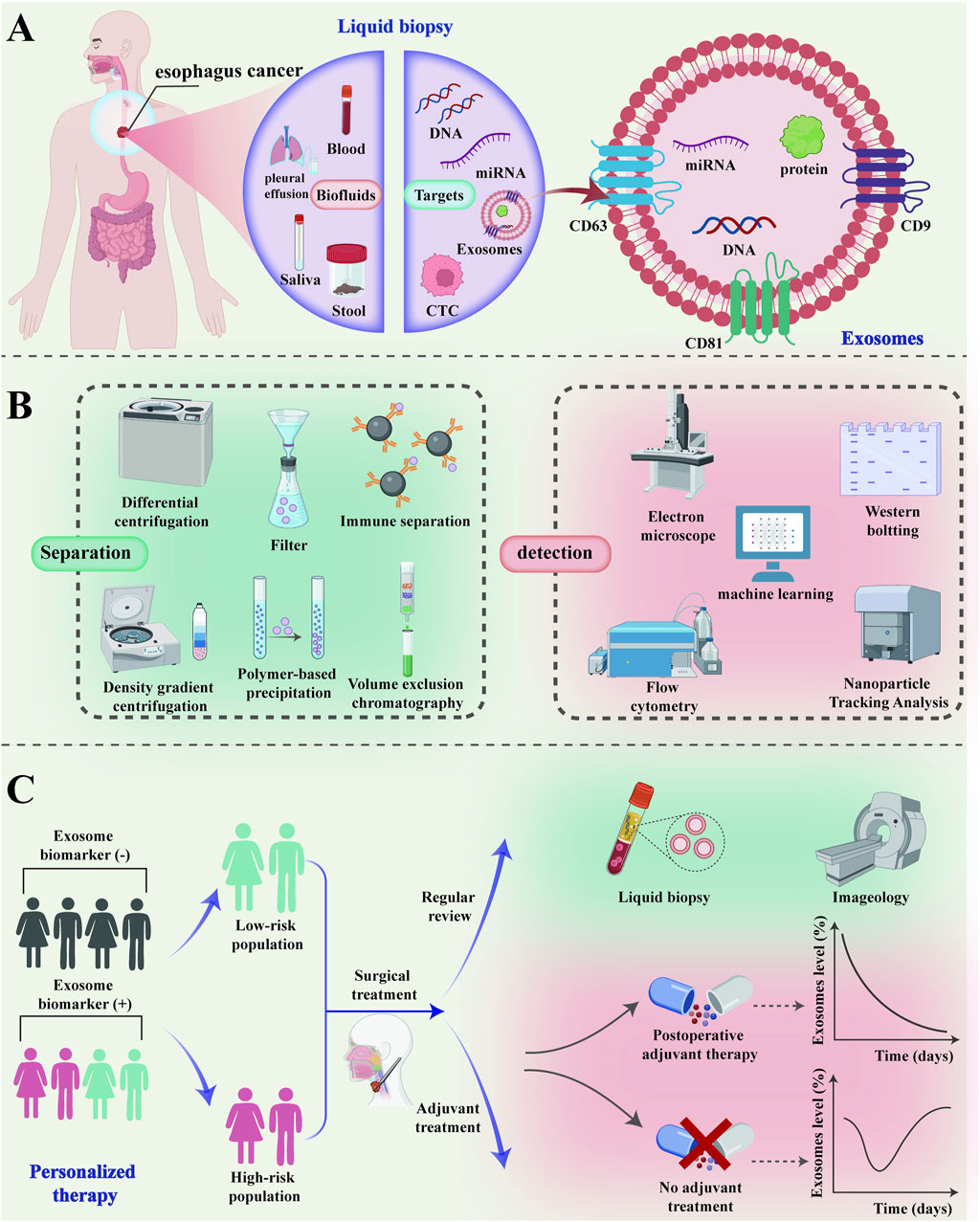
Figure 1. The clinical application of exosomes in the diagnosis and treatment of esophageal cancer. (A) As a “new star” of liquid biopsy, exosomes can be used in the diagnosis and treatment of esophageal cancer through a variety of body fluids; (B) The common isolation and detection techniques of exosomes; (C) Exosomes can be used for screening and risk stratification of high-risk groups of esophageal cancer for personalized precision treatment. The low-risk population of esophageal cancer can be monitored for recurrence by imaging examination and liquid biopsy after surgical treatment. Exosomes can be quantitatively analyzed to monitor the efficacy and prognosis of adjuvant therapy in high-risk patients with esophageal cancer after surgical treatment. CTC, Circulating Tumor Cell; miRNA, microRNA; CT, Computed Tomography.
2 Relationship between exosomes and liquid biopsy
The liquid biopsy technology primarily involves the detection of free circulating tumor cells, circulating tumor DNA, and exosomes, which are used for early screening, personalized treatment, efficacy monitoring, and recurrence monitoring in patients with cancer (Amato et al., 2023). Compared to traditional tissue biopsy, the unique advantages of liquid biopsy include non-invasive, real-time dynamic monitoring, means of overcoming tumor heterogeneity, and ability of comprehensive detection information (Wu et al., 2023; McLaren and Aitman, 2023; Takahashi et al., 2023). Exosomes (Zhao et al., 2021a) are extracellular vesicles released by cells into the extracellular space. They vary between 30 and 150 nm in size, have a double-layer membrane structure, are saucer shaped, and contain a variety of components including nucleic acids, proteins, and lipids. Serving as crucial signaling molecules, EVs contribute to a novel cell-cell information transmission system involved in processes such as cell communication, cell migration, angiogenesis, and tumor cell growth (Figure 2) (Szwedowicz et al., 2022). In this review, we specifically address the use of EVs for the diagnosis and treatment of EC.
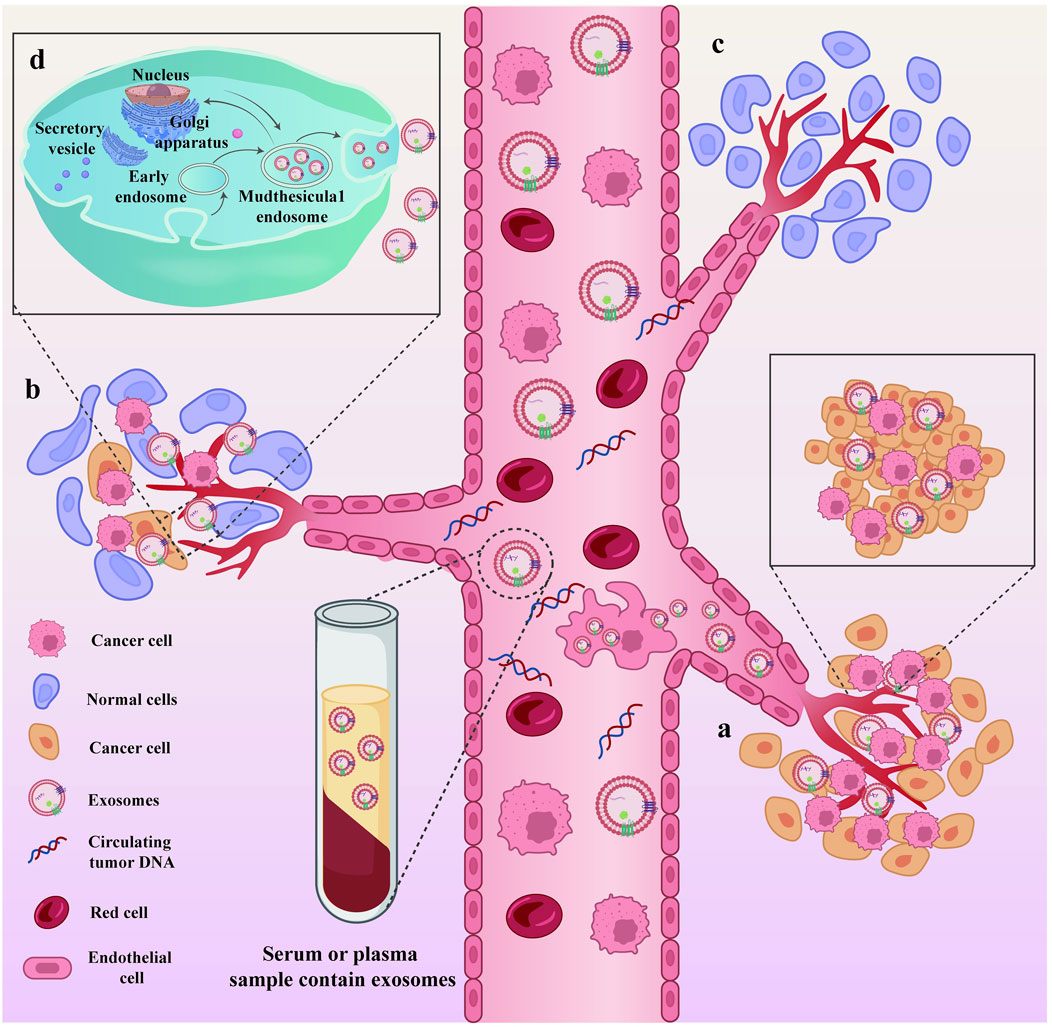
Figure 2. Exosomes, as cell-cell messaging systems, are involved in tumor cell growth and metastasis. Exosomes, as cell-cell messaging systems, are involved in tumor cell growth and metastasis. (A) During the progression of the disease, the primary tumor site continuously secretes exosomes and sheds necrotic tumor cells into the peripheral circulation; (B) Exosomes act as mediators of information transfer, facilitating the metastasis of tumors from the primary site to other organs; (C) Sites within tumor patients where metastasis has not occurred; (D) The process of exosome secretion within cells.
Specifically, the available data support the view that EVs could be leveraged as new targets for liquid biopsy in clinical practice can be understood through three aspects. First, as part of the tumor microenvironment, EVs mirror the biological essence of tumors. EVs released by cancer cells harbor tumor-specific markers that include tumor-specific proteins and mutated RNA sequences (Huang et al., 2019). Analysis of these biomarkers allows tumor evaluation without the need for direct tumor tissue acquisition, enabling early diagnosis. Second, EVs have huge potential in the transmission of information, permitting the study of DNA, RNA, and protein expression, which is more diverse than the research scope of single biomarkers, such as circulating tumor DNA. Third, as tumors progresses, the composition of EVs changes. For patients with mid-to late-stage EC, these alterations can be used to predict therapeutic biomarkers and aid clinical treatment.
Compared to other liquid biopsy biomarkers, EVs have many advantages as novel biomarkers. First, the concentration of EVs in the peripheral blood circulation of tumor patients is higher, with good accuracy and specificity (Wang et al., 2022). Second, the lipid bilayer structure of exosomes protects the contents carried within them from degradation. The abundance of microRNA in plasma exosomes remains stable even when stored at 4°C for 96 h or −80°C for an extended period (Kalluri and LeBleu, 2020). Third, patients harboring tumors accumulate proteins and nucleic acids in their body fluids through exosomes; most of the free DNA in their plasma is enriched in exosomes. These exosomes have been significantly correlated with the occurrence, development, metastasis, and drug resistance of tumors (Han et al., 2022). Fourth, EVs reflect the mutations and metabolic status of the source cells. For example, Exosomes loaded with CRISPR/Cas9 can target the mutant KrasG12D oncogenic allele in pancreatic cancer cells, thereby suppressing proliferation and inhibiting tumor growth in syngeneic subcutaneous and orthotopic models of pancreatic cancer (McAndrews et al., 2021). Additionally, EVs mediated by cytosolic phospholipase A2 small interfering RNA and metformin can target and regulate the metabolism of glioblastoma. Thus, EVs are suitable for precise personalized treatment of tumors (Zhan et al., 2022).
3 Separation of exosomes and detection technology
The separation and detection of EVs are crucial aspects of related research. As the research deepens, new technological advancements continue to emerge. This section aims to discuss commonly used separation and detection techniques for EVs, and new techniques that have emerged in the past 3 years.
Ultracentrifugation is commonly used to recover EVs in high purity and yield (Martins et al., 2023). Ultrahigh-speed centrifugation methods include differential centrifugation and density gradient centrifugation (Roux et al., 2023). This method is suitable for the analysis of high-concentration biological samples. However, the pronounced heterogeneity of extracellular fluid may lead to non-ideal EV aggregation. Density gradient ultracentrifugation, as proposed by Zhao et al. (2022a), is considered the best method for separating many high-quality EVs. Size exclusion chromatography is based on EV size separation and is recommended in the latest (2023) release of the Minimal Information for Studies of Extracellular Vesicles (Welsh et al., 2024). This chromatography method is repeatable, cost-effective, and does not require specialized equipment, making it suitable for isolating EV (Sidhom et al., 2020). Ultrafiltration method utilizes ultrafine nanomembranes with different molecular weight cut off values to separate EVs from clinical samples or cell culture media. EVs and co-vesicles are based on large cell sizes. For example, Ko et al. (2023) introduced electrophoretic aspiration into tangent flow-driven ultrafiltration to solve the problems of clogging and contamination of ultrafiltration membrane pores. The approach separated exosomes with high concentration, purity, and intact morphology using electrophoretic aspiration assisted tangential flow. This report indicates that it may be possible to separate highly pure EVs using ultrafiltration.
Polymer-based precipitation methods have the advantages of simplicity, scalability, and high yield, making them suitable for the rapid diagnosis of diseases. Recently, many commercial reagent kits utilizing precipitation technology have been developed for the separation and enrichment of EVs. Examples of these kits include ExoQuick TC exosome precision solution, Total Exosome Isolation from cell culture media, and the ExoEasy Maxi Kit (Xiong et al., 2022). Gao et al. (2022) compared the ultrafiltration method with the ExoQuick TC precision method and found that size exclusion ultrafiltration resulted in higher yields, acceptable purity, and comparable biophysical properties and biological functions to the more expensive commercial precipitation method.
When traditional separation techniques cannot meet the requirements of exosome detection, the 2023 minimal information for studies guidelines of EVs suggest that combining detection techniques can enhance the detection of EVs. Combining immunoaffinity technology with magnetic separation efficiently enriches the recovery of EVs. For example, Pei et al. (2024) discovered that when titanium ions and antibodies were used in conjunction with magnetic nanoparticles, the captured EV protein content could reach 97 μg/mL. Additionally, 1,060 proteomes originating from exosomes were identified in the final isolated proteins. The MEIS microfluidic chip-based magnetically labeled exosome isolation system also greatly enhanced the enrichment of exosomes (Mun et al., 2023). The MEIS chip utilizes immunoaffinity antibodies to separate her2 overexpressing exosomes from normal exosomes that lack disease-related information, making these isolated exosomes valuable for cancer diagnosis and monitoring (Mun et al., 2023). Microfluidic chips can be combined with immunoaffinity separation technology, as well as physical separation and enrichment methods. Liu et al. (2021) introduced the filter deterministic lateral displacement method to achieve high filtration flux and precise dimensional separation. By integrating a cascaded chip with a filter-deterministic lateral displacement structure, Liu et al. (2021) achieved outstanding separation efficiency (>96%), high cell purity (white blood cell removal rate of 99.995%), high cell viability (>98%), and a processing rate of 1 mL/min.
Finally, it is important to note that EV separation techniques are continuously evolving, and we may not be well versed in all their characteristics. Often, the method of separation selected may not meet the criteria for the target samples, leading to a lower purity of EVs (Yu et al., 2022). Therefore, we compiled and compared the operational mechanisms, advantages, and disadvantages of the EV separation technologies that have emerged in recent years (Table 1).
After isolation, exosomes must be identified to analyze their roles in tumor growth, disease progression, and metastasis. Currently, exosome identification primarily depends on morphological characteristics, nanoparticle tracing analysis for particle size detection, protein blotting technology (Pan et al., 2023a), and flow cytometry to analyze exosome marker proteins such as CD9, CD63, CD81, and Heat Shock Protein 90 (HSP90) (Barranco et al., 2024; Huang et al., 2023). To provide a more comprehensive understanding of the current possibilities for identifying EVs, Table 2 compares and summarizes the commonly used methods. Additionally, we include examples that provide clear insights into the specific applications of EV identification analysis. Qi et al. (2023) used protein imprinting technology, nanoparticle tracking analysis and transmission electron microscopy to characterize exosomes. The authors suggested that exosomes containing Acyl-CoA synthetase long chain family member 4 play a role in the gemcitabine resistance of pancreatic cancer cells. Han et al. (2023) integrated flow cytometry analysis to demonstrate that tumor-derived exosomes carrying mir-1258-3p and miR-17-5p decreased T cell infiltration and activation, while increasing macrophage infiltration and M2 polarization, resulting in an immunosuppressive microenvironment.
Considering the heterogeneity and subtle differences in the performance of EVs, many exciting new separation and detection technologies are being explored. Xia et al. (2023) studied the DNA probe adsorbent manganese ion-modified black phosphorus (BP@Mn2+). The DNA hybrid nanosensor quickly distinguished exosomes derived from colorectal cancer cells from those derived from intestinal epithelial cells by sensing microRNAs. Jiang et al. (2024) developed a tetrahedral DNA (Apt TDNA) microelectrode sensor loaded with an adapter, which assisted the use of a polydopamine (PDA) coating with semiconductor properties to achieve single-particle-level sensitivity for cancer-derived exosome detection. Jang et al. (2021) developed recombinant exosomes loaded with photosensitizers.
4 Applications of exosomes for early diagnosis of EC
Tumor growth, invasion, and metastasis are regulated by bidirectional communication between cells and complex tissue environments. Tumor cells release EVs that shape the tumor microenvironment (Wang et al., 2023a), leading to changes and expansion that promote tumor development (Jin et al., 2022; Zhao et al., 2023). Because EVs can travel through body fluids such as blood and urine, they carry molecular information that reflects the biological status of various cell types, including tumor cells (Mishra et al., 2023; Zhou et al., 2023). Consequently, EVs have emerged as promising biomarkers with significant clinical relevance for early cancer detection, especially in cases where symptoms are not yet apparent (Chen et al., 2023b; Liang et al., 2023). For instance, Kim et al. (2023) demonstrated that using a combination of three miRNAs (miR-200b-3p, miR-3124-5p, and miR-92b-5p) significantly enhanced the diagnostic accuracy of small-cell lung cancer [area under the receiver operating characteristic curve (AUC, also abbreviated AUROC) = 0.93]. Additionally, Hinestrosa developed a classification system based on exocrine body markers that could detect stage I and II pancreatic, ovarian, and bladder cancers with high accuracy (95.5% for stage I pancreatic cancer, 74.4% for ovarian cancer, and 43.8% for bladder cancer, with a specificity >99% for all tumors screened) (Hinestrosa et al., 2022). Here, we focus primarily on the clinical application of EVs in the early diagnosis of EC.
Firstly, this study summarizes the specificity and sensitivity of various exosomal biomarkers for the early diagnosis of EC in recent years (Li et al., 2022a; Li et al., 2023b; Xing et al., 2017; Tong et al., 2015; Miyoshi et al., 2022) (Table 3). EVs can be utilized for the early diagnosis of EC owing to their involvement in malignant processes such as proliferation, angiogenesis, and metastasis. For instance, (Feng et al., 2023) discovered through protein mass spectrometry analysis that the interaction between p53 mutation G245S (p53-G245S) and heterogeneous nuclear ribonucleoprotein A2B1 (hnRNPA2B1) affects the formation of exosomes. Additionally, Liu et al. (Li et al., 2023b) revealed that p53 G245S mutation enhances ESCC proliferation and metastasis through hnRNPA2B1-ADP-ribosylation factor GTPase-activating protein 1 (AGAP1) mediated exosome formation. This study presented novel evidence of the molecular mechanism of EVs in ESCC progression, with AGAP1 mRNA potentially serving as a non-invasive biomarker for early ESCC detection. Furthermore, Zhang et al. (2022) identified that Poly(A) binding protein cytoplasmic 1 (PABPC1) is highly expressed in ESCC compared to normal esophageal epithelial tissue, suggesting its potential as a target for early EC diagnosis. Specifically, the expression of PABPC1 in ESCC positively correlates with tumor differentiation, and high PABPC1 expression in ESCC patients is associated with shorter median survival time post-surgery compared to low PABPC1 expression (p = 0.043) (Zhang et al., 2022). In addition, PABPC1 can enhance angiogenesis by mediating miR-21-5p expression and promoting the release and targeting of extracellular miR-21-5p to endothelial cells. Moreover, Rao et al. (2023) confirmed that the EV protein CD54 can be used as a biomarker for early EAC diagnosis. The available data indicate the promise of the use of tumor cell-secreted EVs as biomarkers for early EC detection as a novel clinical diagnostic approach.
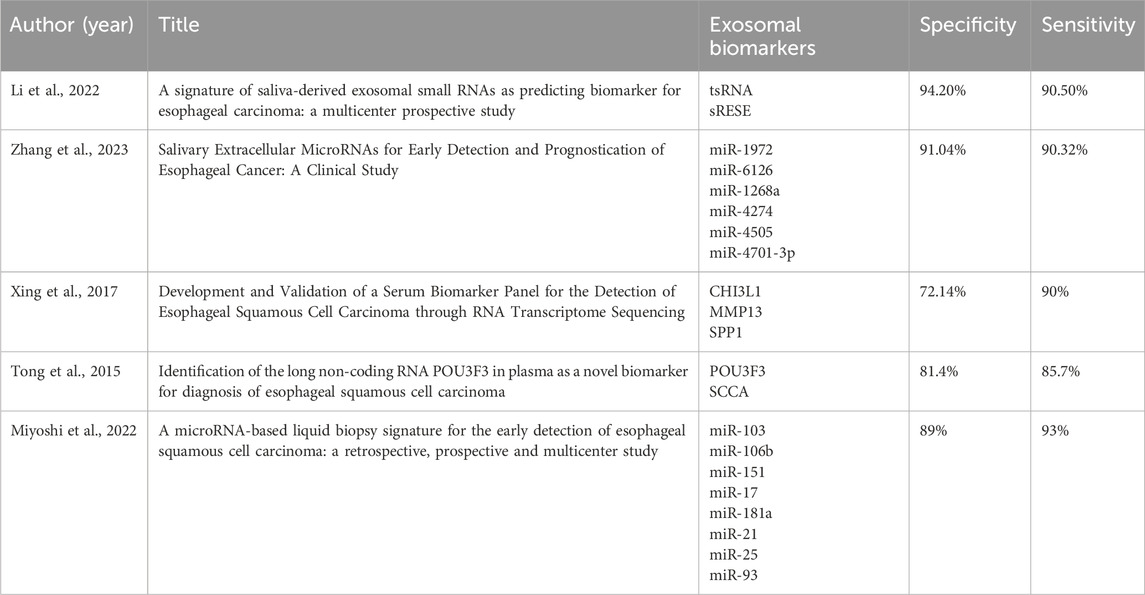
Table 3. The application of different exosomal biomarkers in the early diagnosis of esophageal cancer.
EVs are found in nearly all bodily fluids including blood (Wang et al., 2023b), saliva (Xia et al., 2023b), and urine (Zhang et al., 2023), broadening the potential sources of liquid biopsy samples. In 2019, al.(Lin et al., 2019) revealed that salivary exosome GOLM1-NAA35 chimeric RNA (seG-NchiRNA) differentiates between tumor patients and healthy individuals, but also identify early stage tumor patients. A study published in 2022 reported that dual characteristic biomarkers (identified through small RNA Seq screening) from saliva-derived exosomes, tRNA GlyGCC-5 and sRESE, could distinguish patients with ESCC from the control group with high sensitivity (90.50%) and specificity (94.20%) (Li et al., 2022a). Additionally, the prognostic dual-feature risk score (RSP) proposed by Hao (Li et al., 2023b) could preoperatively predict that patients with high RSPs would benefit from postoperative adjuvant therapy. By continuing their research efforts, the team achieved significant breakthroughs. In July 2023, Hao et al. (Li et al., 2023b) published a clinical study on the early detection of salivary extracellular miRNAs for ESCC, with the goal of establishing miRNA profiles from salivary EVs and granules (EVPs) for early ESCC detection (Li et al., 2023b). In the study, we developed an early diagnostic model comprising six miRNAs. The model accurately identified all patients with ESCC in the training set (AUROC = 0.968) and successfully validated the results in two independent cohorts. Importantly, the model could differentiate patients with early stage (I/II) ESCC from the control group in the training (AUROC = 0.969, sensitivity = 92.00%, specificity = 89.17%), internal (sensitivity = 90.32%, specificity = 91.04%), and external (sensitivity = 91.07%, specificity = 88.06%) cohorts. Furthermore, the reproducibility of the six identified miRNAs ensured consistency in sample analysis and verification, which is critical for further validation in larger cohorts and potential integration into clinical practice.
Zhu et al. (2023b) initially conducted a proteomic analysis of plasma EVs and identified 419 proteins. Subsequently, they utilized bioinformatics techniques such as Gene Ontology and Kyoto Encyclopedia of Genes and Genomes enrichment analyses to identify differentially expressed proteins. The researchers have ultimately pinpointed a biomarker EV-CD14 that can be leveraged for the early detection of ESCC. In the test set comprising 30 cases, EV-CD14 demonstrated a high AUC value of 96% and an accuracy rate of 90% in predicting ESCC. Building on this research, Xing et al. (2017) investigated the RNA transcriptome of six pairs of ESCC tissues and identified three potential biomarkers: chitinase-3-like protein 1 (CHI3L1), matrix metallopeptidase 13 (MMP13), and osteopontin (SPP1). The AUC values for serum CHI3L1, MMP13, and SPP1 levels for differentiating patients with ESCC from controls were 0.732, 0.881, and 0.661, respectively. Combining these three proteins into a joint diagnostic model significantly enhanced the performance compared to individual markers, resulting in an AUC value of 0.928 (95% confidence interval CI 0.900–0.956), sensitivity of 90%, and specificity of 72.14% (Xing et al. 2017). The improved diagnostic efficacy of the combined model was subsequently validated. However, the use of a single exosome target for early EC detection presents limitations. Therefore, the association between solitary exosome targets and cancer assessment may not be sufficiently robust. Additionally, exosome protein mass spectrometry has inherent constraints and may not fully capture disease onset, making it susceptible to sample variations and methodological differences. Consequently, the primary objective at present is to identify multiple EV markers linked to cancer characterization and establish a comprehensive, multi-quantitative early diagnostic model. Tong et al. (2015) highlighted the diagnostic potential of plasma POU class 3 homeobox 3 (POU3F3) in ESCC (AUC 0.842; p < 0.001; sensitivity 72.8%; specificity 89.4%). When combined with Squamous Cell Carcinoma Antigen (SCCA), the diagnostic performance significantly improved, yielding an AUC of 0.926, sensitivity of 85.7%, and specificity of 81.4%. Notably, the combined detection of POU3F3 and SCCA effectively identified 80.8% of early ESCC cases (Tong et al., 2015).
Miyoshi et al. (2022) screened eight miRNAs (miR-103, miR-106b, miR-151, miR-17, miR-181a, miR-21, miR-25, and miR-93) that were overexpressed in ESCC serum samples from three miRNA expression datasets and constructed a circulating miRNA signature. Subsequently, the diagnostic performance of the ESCC model was validated in two independent cohorts [n = 126, AUC: 0.80 (95% CI: 0.69–0.91)]; and n = 165, AUC: 0.89 [95% CI: 0.83–0.94]. Importantly, the eight-miRNA model outperformed current clinical serological tumor markers (p < 0.001) in distinguishing early ESCC patients from healthy controls. Furthermore, Miyoshi et al. performed two prospective verifications confirming that the eight-miRNA combined diagnostic model can achieve good results in early screening of EC, with ideal diagnostic performance [n = 185, AUC: 0.92 (95% CI: 0.87–0.96)] and [n = 188, AUC: 0.93 (95% CI: 0.88–0.97)]. Larger scale studies with retrospective designs have been performed (Shin et al., 2023) and the prospective validation has been validated following retrospective studies. The data to date indicates the reliability and reproducibility of the foregoing research findings and will expedite the translation of research results into clinical applications.
Liquid biopsy has shown significant potential for EC. There have been numerous advancements in research on EVs in tumor diagnosis, with many scholars identifying EV markers for the diagnosis of EC. For example, the report that plasma EVs harboring miR-205-5p, miR-429, and miR-375-3p can be utilized as non-invasive biomarkers for early ESCC diagnosis (Kim et al., 2022) offers new perspectives and strategies for detecting esophageal tumors at an early stage. Looking ahead, the application of exosomes in clinical practice requires a shift towards prospective studies focused on high-risk EC populations. These studies should aim to differentiate patients who may develop EC in the future and guide precise personalized treatment strategies. For instance, Exosome Diagnostics, a leading industry in exosome diagnosis since 2008, released the midterm results of a prospective randomized study in May 2023 (Tutrone et al., 2023). This study reported that the ExoDx™ prostate test successfully stratified patients into low- and high-grade prostate cancer risk categories, assisting physicians in making informed decisions regarding the necessity of biopsies for patients with uncertain cancer risk based on prostate specific antigen results. Moreover, for men identified as having either extremely low or high prostate cancer risk as indicated by ExoDx, standard treatment protocols have proven vital in providing necessary information and care to ensure optimal patient outcomes (Tutrone et al., 2023).
5 Applications of exosomes in adjuvant therapy for EC
Treatment of EC has always posed a clinical challenge. Previous clinical data have revealed that patients with advanced EC have a 5-year survival rate of 30% (Wang et al., 2023c) with a median survival time of 25.9 months; tumor progression occurs in the majority (85.2%). Traditional treatment methods such as surgery, radiation therapy, and chemotherapy can partially control the condition. However, they have limitations and challenges (Triantafyllou and Wijnhoven, 2020), especially for advanced patients. Therefore, the discovery of new treatment strategies is imperative to enhance the survival rate of patients with EC. In recent years, EVs have garnered attention as potential therapeutic agents. These vesicles regulate inflammatory responses, angiogenesis, and fibroblast activation (Xie et al., 2021; Jin et al., 2021). They also create a conducive growth environment for tumor cells (Li et al., 2021b) and aid in evading immune system attacks (Zhao et al., 2022b), thereby fostering tumor cell proliferation, invasion, metastasis, and drug resistance (Lin et al., 2022; Akoto and Saini, 2021). This section delves into the clinical applications and potential mechanisms of EVs in the treatment of EC.
EVs are primarily responsible for transmitting information between tumor cells and can be likened to “couriers.” As new targets for cancer-targeted therapy, tumor exosomes can be suppressed in several ways: One method is to directly prevent the couriers from leaving, which involves disrupting the synthesis and secretion of EVs. For example, Zhao et al. (2021b) discovered that interleukin-6 (IL-6) secreted by cancer-associated fibroblasts (CAF) and miRNA-21 (miR-21) packaged in exosomes activate IL-6 signaling and transcription activating factor 3 (STAT3), leading to cisplatin resistance in patients with ESCC. Therefore, inhibiting the paracrine and autocrine secretion of CAF-related IL-6 is a potential target for reversing drug resistance. Secondly, if the exosomes cannot be intercepted during their formation, obstacles can be placed on their delivery route. For instance, Jin et al. (2021) found that blocking the phosphoinositide 3-kinase/AKT and β-catenin signaling pathways derived from exosomal miR-3656 can inhibit the growth and metastasis of ESCC cells. Additionally, Qiao et al. (2023) described the significant reduction of the tumor-promoting effect of LINC01592 tumor-associated macrophages by disrupting the E2F6/NBR1/MHC-I signaling axis derived from exosomes of LINC01592. Interestingly, the authors also discovered that inhibiting LINC01592 can also lead to increased major histocompatibility complex class I (MHC-I) expression on the surface of tumor cells, thereby enhancing the effectiveness of CD8+ T cell reinfusion.
Owing to the protection of the phospholipid bilayer, EVs have good stability (Arya et al., 2024; Liu et al., 2024a) and can protect their internal payload. Therefore, EV testing can be used to guide the prognosis of patients with EC. Zhu et al. (2021) analyzed the metabolomic patterns of EVs in recurrent and non-recurrent patients after esophagectomy using machine learning and identified a panel of extracellular vesicle metabolic biomarkers (3′-UMP, palmitic acid, palmitol, and isobutyl decanoate) for predicting the recurrence of ESCC with 98% accuracy. These metabolomic features exhibit significant differential changes throughout all ESCC stages and are likely associated with cancer metabolism, potentially serving as novel biomarkers for ESCC prognosis. Li et al. (2022) suggested that miR-200a promotes the proliferation, migration, and invasion of EC cells by altering the Keap1/Nrf2 signaling pathway. The authors reported that miR-200a transported via EVs can be a valuable biomarker for predicting the prognosis of patients with ESCC. Additionally, Luo et al. (2016) found that long noncoding RNAs (lncRNAs) AFAP1-AS1 promotes the in vitro proliferation of esophageal cancer cells, with expression observed in both the nucleus and cytoplasm, indicating that AFAP1-AS1 is predominantly localized within the nucleus, thereby enhancing its potential role in epigenetic or transcriptional regulation. The authors suggest that AFAP1-AS1 may influence the proliferation of ESCC cells through epigenetic regulation of Homeobox B7 (HOXB7) and by interacting with Polycomb Repressive Complex 2 (PRC2). Consequently, Luo et al. (2016) concluded that the upregulation of exosomal lncRNA AFAP1-AS1 in ESCC is closely associated with shorter progression-free survival and unfavorable overall survival.
The exosome miRNA model established by Liu et al. (2020) can effectively identify patients with lymph node metastasis in ESCC and is significantly superior to preoperative X-ray computed tomography. This miRNA model has the potential to predict preoperative lymph node metastasis in ESCC patients, assist thoracic surgeons in determining appropriate treatment plans, and enhance patient prognosis. Zhang et al. (2024) quantified the plasma exosome concentrations of patients with locally advanced ESCC at two time points: before radiotherapy and chemotherapy, and 2 months after treatment. A significant decrease in average exosome concentration from 7.3 × 1011 particles/mL before treatment to 5.4 × 1011 particles/mL after treatment was evident (p < 0.001). Using multivariate Cox regression analysis, the authors further demonstrated that dynamic changes in exosome levels could serve as independent predictors of progression-free survival. Liu et al. (2020b) proposed that serum exosome miR-766-3p could act as a prognostic indicator for evaluating ESCC. The findings validated the correlation between Tumor, Node, Metastasis (TNM) staging and elevated levels of exosomal miR-766-3p in ESCC patients (p < 0.05), with higher levels of miR-766-3p expression in serum exosomes indicating a poorer prognosis, with hazard ratios [95% CI] for overall survival of 2.21 [1.00, 4.87] and disease-free survival of 2.15 [1.01, 4.59].
Exosomes have a dual effect on tumors. In addition to inhibiting the immune system and promoting tumor development, tumor exosomes can improve the efficacy of adjuvant therapy. Chen et al. (2020) found that overexpression of miR-450a-5p can increase radiation sensitivity in patients with ESCC and could be a potential new therapeutic target to reduce radiation therapy resistance. Similarly, Du et al. (2022) found that inhibiting the high expression of plasma exosomes harboring High mobility group box 1 protein can reduce radiation-related adverse reactions in patients with ESCC. Sun et al. (2021) found that miR-199a-5p enhances the radiosensitivity of radiation-resistant cells by targeting Endonuclease/exonuclease/phosphatase family domain-containing protein 1 and inhibiting the Ataxia-telangiectasia and Rad3-related protein/Checkpoint kinase 1 signaling pathway. However, lncRNA-NORAD inhibits miR-199a-5p expression by competitively binding to PUM1 from pri-miR-199a1, thereby rendering ESCC patients resistant to radiotherapy. Finally, they confirmed that lncRNA activated by deoxyribonucleic acid damage (lncRNA-NORAD) is a potential therapeutic target for improving the efficacy of ESCC immunotherapy combined with radiation therapy. Luo et al. (2019) found that high concentrations of miR-339-5p in serum are positively correlated with high sensitivity to radiotherapy and favorable survival rates. Additionally, they observed that miR-339-5p can predict the pathological response to preoperative radiotherapy in locally advanced ESCC, as miR-339-5p expression was significantly downregulated in the T3/T4 stage compared to the T1/T2 stage in ESCC patients (p < 0.05). Mechanistically, miR-339-5p enhances radiosensitivity by targeting Cdc25A and is transcriptionally regulated by Runx3. Therefore, miR-339-5p may serve as a promising non-invasive biomarker for facilitating personalized treatment.
Recently, EV drug delivery has become a major research focus. The main advantages of using EVs as drug delivery carriers are their ability to lower drug concentration, accurately target the site of injury, enhance efficiency, and reduce drug toxicity and side effects (Tian et al., 2023; Chen et al., 2023c). Accordingly, this review also discusses the potential applications of EV drug delivery in EC (Figure 3). Ma et al. (2022) engineered exosomes produced by M1 macrophages (M1Exos) to address the triple challenges of the tumor immune suppression microenvironment, hypoxic microenvironment, and rapid DNA damage repair occurring following radiotherapy. Additionally, the authors described that the outer membrane of M1Exos contains nanoantibodies targeting programmed death-ligand 1, which can alleviate CD8+ T cell immunosuppression and reshape the immunosuppressive microenvironment of tumors. Moreover, exosomes carrying miR-375 from human umbilical cord mesenchymal stem cells can inhibit the expression of ENAH actin regulator 1, thus hindering the progression of ESCC (He et al., 2020). To identify differentially expressed miRNAs in cancerous versus adjacent tissues from various public miRNA databases, the authors additionally aimed to validate their functionality through experiments involving exosomes and critical biological pathways. The encapsulation of artificially designed miR-375 molecules in exosomes could open new avenues for ESCC drug development. Similarly, as suggested by He et al. (2020) and Chen et al. (2023d), miR-100-5p inhibits the proliferation, migration, invasion, and tube formation of tumor-associated lymphatic endothelial cells (TLECs). Furthermore, miR-100-5p also suppresses lymphangiogenesis in ESCC xenografts. Mechanistically, this inhibitory effect is mediated by the miR-100-5p-induced suppression of the IGF1R/PI3K/AKT signaling axis. Therefore, taken together, CAF-derived exosomal miR-100-5p can target the IGF1R/PI3K/AKT axis to inhibit lymphatic metastasis in ESCC. Consequently, exosomal miR-100-5p also holds potential as a drug delivery vehicle in the development of therapies aimed at suppressing ESCC progression. Furthermore, this study provides an overview of current extracellular vesicle drug delivery methods, encompassing small molecule drug delivery, including ultrasound (Wang et al., 2019), mixed drug delivery (Kim et al., 2016), extracellular vesicle peptide modifications (Shen et al., 2022), EV delivery of nucleic acids that include small interfering RNA, miRNA, and plasmids (Geng et al., 2023), and EV delivery of macromolecule such as chitosan oligosaccharide (Li et al., 2021c).
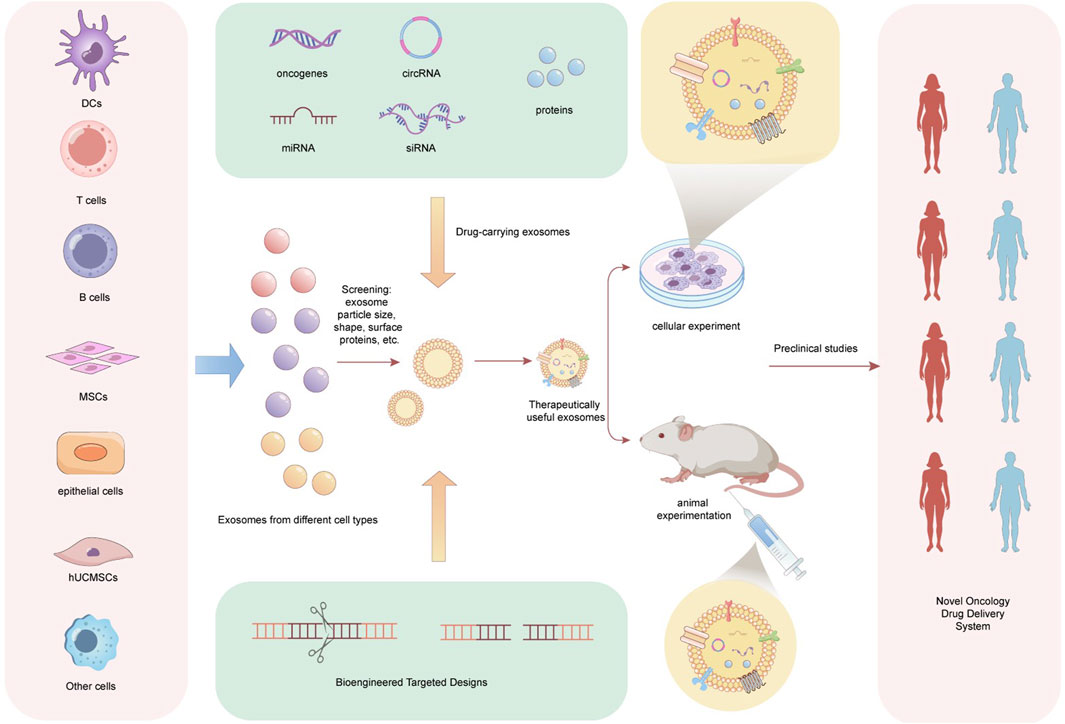
Figure 3. The clinical application prospect of exosomes as a new generation of drug delivery vehicles. Exosomes are collected from various cell types, including dendritic cells (DCs), T cells, B cells, mesenchymal stem cells (MSCs), and epithelial cells. These exosomes are screened based on characteristics such as size, dimensions, and surface proteins to identify suitable candidates. Utilizing bioengineering targeting technologies, different drug-loaded exosomes are designed, incorporating agents such as oncogenes, circRNA, miRNA, siRNA, and proteins. Upon obtaining exosomes with therapeutic potential, in vitro experiments (cell-based assays) and in vivo studies (mouse models) are conducted to evaluate the safety and efficacy of the drug-loaded exosomes. Furthermore, prior to the comprehensive clinical application of these drug-loaded exosomes, preclinical studies must be performed in relevant populations. Ultimately, the resulting drug-loaded exosomes are poised to serve as a novel tumor drug delivery system. DCs, Dendritic Cells, T cells, Thymus Dependent Lymphocytes, B cells, Bursa Dependent Lymphocytes, MSCs, Mesenchymal Stem Cells, hUCMSCs, human Umbilical cord Mesenchymal Stem Cells.
6 Future prospects
As one of the three categories of liquid biopsy, EVs are abundant in body fluids and carry various biological information molecules from secreted cells. The double phospholipid layer membrane structure provides better protection for the contents, making them potential biomarkers. An advantage of exosomes is their abundance (Li et al., 2023c; Ten et al., 2024). Tumor cells can continuously secrete exosomes and enter the circulatory system during their growth process, and their high metabolic rate leads to a much higher production of exosomes than that of normal cells (Vahabi et al., 2023; Liu et al., 2024b). Additionally, compared to the dependence of circulating tumor DNA generation on tumor cell death, exosomes secreted by living cells represent a continuous event that can reflect the real-time progression of tumor development (Claridge et al., 2021; Liu et al., 2022). However, it is important to note that current research on the mechanism of action of EVs has mainly involved in vitro experimental systems and animal models (Xu et al., 2023; Pan et al., 2023b). There is no consensus on experimental methods for extracting and quantifying EVs from cell culture supernatants. As a result, research on EV-related functions only simulates an almost in vivo environment, and individual differences can lead to heterogeneity in the functions of extracellular vesicles. Therefore, further in-depth studies are required to elucidate the mechanisms of EV formation and secretion.
Furthermore, although exosomes have demonstrated clinical value in the diagnosis and treatment of esophageal cancer, their safety issues warrant attention. Exosomes may elicit potential immune responses, as their components could be recognized by the host immune system as foreign substances, activating immune reactions that may lead to adverse effects (Xia et al., 2024). Additionally, the non-target effects of exosomes should not be overlooked; their widespread distribution could impact various cell types and tissues, inadvertently delivering therapeutics to non-target cells and thereby causing side effects (Fusco et al., 2024). Current data on long-term safety remain limited, and the metabolic and clearance mechanisms of exosomes within the body are not yet fully understood, posing potential risks for their application in the long-term treatment of esophageal cancer. Therefore, establishing rigorous standardized protocols and regulatory frameworks to ensure the safety and efficacy of exosome extraction, characterization, and application is of significant importance.
To facilitate the transition of exosomes from basic research to clinical application, this study suggests focusing on the following three aspects. First, given the heterogeneity of exosomes and their close relationship with tumor heterogeneity, subtypes, and therapeutic detection, it is essential to develop more single-exosome detection technologies. Second, future research should stratify patients (for example, based on cancer risk stratification, pathological staging, etc.) using established standardized exosome research methods to ensure the reproducibility and comparability of experiments, which will aid in advancing the application of exosome-related molecules in esophageal cancer. Third, to enable the large-scale clinical application of exosomes in the future, subsequent studies could place greater emphasis on the diagnostic value of multi-molecule combined detection models involving exosomes in esophageal cancer, while conducting more extensive clinical trials to validate the actual efficacy of exosomes in this context.
7 Conclusion
In recent years, exosomes have been extensively studied and have demonstrated clinical value in various cancers. For instance, in prostate cancer, exosomes have been found to carry specific proteins and small RNAs that are closely associated with the onset and progression of the disease (Canovai et al., 2023; Atri et al., 2024). Similarly, in esophageal cancer, some studies have identified small RNAs such as LncRNA RP11-465B22.8 within exosomes as potential biomarkers (Hu et al., 2021). In lung cancer, exosomes promote tumor cell evasion and metastasis by delivering immune regulatory factors (Wang et al., 2024). Preliminary data also suggest that esophageal cancer cells may utilize exosomes to alter the surrounding microenvironment, thereby facilitating aggressive behavior (Ning et al., 2023). However, further exploration of the role of exosomes in the microenvironment of esophageal cancer is necessary to reveal new therapeutic targets.
In the competitive landscape of exosome diagnosis, there have been some breakthrough achievements and technological developments in industrial research on exosome diagnosis internationally. Exosome Diagnostics has approved multiple products for market launch, such as the ExoDxLung (ALK) lung cancer diagnostic kit and the ExoDx Prostate (IntelliScore) blood/urine detection kit. These products can be used to analyze RNA, proteins, and other components in exosomes obtained from the blood or urine samples of patients to accurately diagnose cancer. Furthermore, tumor-related exosomes can exert various biological functions through biological barriers, making them a promising new route for drug delivery and gene therapy vectors (Peswani Sajnani et al., 2021). Owing to their highly complex and variable composition, the cellular specificity and biological effects of EVs are unpredictable. When used as drug carriers, it is essential to improve cell specificity and circulation time, increase accumulation in targeted tissues, and enhance carrier delivery. This is a new trend for EVs as targeted drugs for disease treatment, providing compelling advantages and prospects for treating radiation damage.
Author contributions
RZ: Conceptualization, Data curation, Writing–original draft. CJ: Investigation, Writing–review and editing. DL: Investigation, Writing–original draft. LX: Investigation, Resources, Writing–original draft. ZY: Conceptualization, Funding acquisition, Writing–original draft, Writing–review and editing.
Funding
The author(s) declare that financial support was received for the research, authorship, and/or publication of this article. This study was funded by the Jilin Provincial Department of Science and Technology Project (No. YDZJ202402027CXJD) and Beijing Medical Award Foundation Project (YXJL-2022-0080-0433).
Acknowledgments
We would like to thank Editage (www.editage.cn) for English language editing.
Conflict of interest
The authors declare that the research was conducted in the absence of any commercial or financial relationships that could be construed as a potential conflict of interest.
Publisher’s note
All claims expressed in this article are solely those of the authors and do not necessarily represent those of their affiliated organizations, or those of the publisher, the editors and the reviewers. Any product that may be evaluated in this article, or claim that may be made by its manufacturer, is not guaranteed or endorsed by the publisher.
References
Akoto, T., and Saini, S. (2021). Role of exosomes in prostate cancer metastasis. Int. J. Mol. Sci. 22 (7), 3528. doi:10.3390/ijms22073528
Amato, O., Aftimos, P., and Ignatiadis, M. (2023). Liquid biopsy accelerates precision medicine. Ann. Oncol. 34 (4), 333–335. doi:10.1016/j.annonc.2023.02.004
Arya, S. B., Collie, S. P., and Parent, C. A. (2024). The ins-and-outs of exosome biogenesis, secretion, and internalization. Trends Cell Biol. 34 (2), 90–108. doi:10.1016/j.tcb.2023.06.006
Atri, R. G., Kokal-Ribaudo, M., Heidari Horestani, M., Pungsrinont, T., and Baniahmad, A. (2024). The protein composition of exosomes released by prostate cancer cells is distinctly regulated by androgen receptor-antagonists and -agonist to stimulate growth of target cells. Cell Commun. Signal 22 (1), 219. doi:10.1186/s12964-024-01584-z
Barranco, I., Alvarez-Barrientos, A., Parra, A., Martínez-Díaz, P., Lucas, X., and Roca, J. (2024). Immunophenotype profile by flow cytometry reveals different subtypes of extracellular vesicles in porcine seminal plasma. Cell Commun. Signal 22 (1), 63. doi:10.1186/s12964-024-01485-1
Bray, F., Laversanne, M., Sung, H., Ferlay, J., Siegel, R. L., Soerjomataram, I., et al. (2024). Global cancer statistics 2022: GLOBOCAN estimates of incidence and mortality worldwide for 36 cancers in 185 countries. CA Cancer J. Clin. 74 (3), 229–263. doi:10.3322/caac.21834
Canovai, M., Evangelista, M., Mercatanti, A., D'Aurizio, R., Pitto, L., Marrocolo, F., et al. (2023). Secreted miR-210-3p, miR-183-5p and miR-96-5p reduce sensitivity to docetaxel in prostate cancer cells. Cell Death Discov. 9 (1), 445. doi:10.1038/s41420-023-01696-4
Chen, C., Yang, C., Tian, X., Liang, Y., Wang, S., Wang, X., et al. (2023d). Downregulation of miR-100-5p in cancer-associated fibroblast-derived exosomes facilitates lymphangiogenesis in esophageal squamous cell carcinoma. Cancer Med. 12 (13), 14468–14483. doi:10.1002/cam4.6078
Chen, H., Yao, H., Chi, J., Li, C., Liu, Y., Yang, J., et al. (2023c). Engineered exosomes as drug and RNA co-delivery system: new hope for enhanced therapeutics? Front. Bioeng. Biotechnol. 11, 1254356. doi:10.3389/fbioe.2023.1254356
Chen, H., Yao, X., Di, X., Zhang, Y., Zhu, H., Liu, S., et al. (2020). MiR-450a-5p inhibits autophagy and enhances radiosensitivity by targeting dual-specificity phosphatase 10 in esophageal squamous cell carcinoma. Cancer Lett. 483, 114–126. doi:10.1016/j.canlet.2020.01.037
Chen, Q., Shi, J., Ruan, D., and Bian, C. (2023b). The diagnostic and therapeutic prospects of exosomes in ovarian cancer. Bjog 130 (9), 999–1006. doi:10.1111/1471-0528.17446
Chen, R., Zheng, R. S., Zhang, S. W., Wang, S. M., Sun, K. X., Zeng, H. M., et al. (2023a). Patterns and trends in esophageal cancer incidence and mortality in China: an analysis based on cancer registry data. J. Natl. Cancer Cent. 3 (2), 21–27. doi:10.1016/j.jncc.2023.01.002
Claridge, B., Lozano, J., Poh, Q. H., and Greening, D. W. (2021). Development of extracellular vesicle therapeutics: challenges, considerations, and opportunities. Front. Cell Dev. Biol. 9, 734720. doi:10.3389/fcell.2021.734720
Dawsey, S. M., and Duits, L. C. (2023). A substantial advance for screening of oesophageal cancer. Lancet Gastroenterol. Hepatol. 8 (5), 393–395. doi:10.1016/S2468-1253(23)00065-1
Du, X., Zhang, X., Dong, J., Zou, N., Guo, D., Yao, W., et al. (2022). Irradiation-induced exosomal HMGB1 to confer radioresistance via the PI3K/AKT/FOXO3A signaling pathway in ESCC. J. Transl. Med. 20 (1), 507. doi:10.1186/s12967-022-03720-0
Feng, R., Yin, Y., Wei, Y., Li, Y., Li, L., Zhu, R., et al. (2023). Mutant p53 activates hnRNPA2B1-AGAP1-mediated exosome formation to promote esophageal squamous cell carcinoma progression. Cancer Lett. 562, 216154. doi:10.1016/j.canlet.2023.216154
Fusco, C., De Rosa, G., Spatocco, I., Vitiello, E., Procaccini, C., Frigè, C., et al. (2024). Extracellular vesicles as human therapeutics: a scoping review of the literature. J. Extracell. Vesicles 13 (5), e12433. doi:10.1002/jev2.12433
Gao, D., Lu, P., Zhang, N., Zhao, L., Liu, J., Yang, J., et al. (2023). Progression of precancerous lesions of esophageal squamous cell carcinomas in a high-risk, rural Chinese population. Cancer Med. 12 (2), 1791–1800. doi:10.1002/cam4.4965
Gao, M., Cai, J., Zitkovsky, H. S., Chen, B., and Guo, L. (2022). Comparison of yield, purity, and functional properties of large-volume exosome isolation using ultrafiltration and polymer-based precipitation. Plast. Reconstr. Surg. 149 (3), 638–649. doi:10.1097/PRS.0000000000008830
Geng, Y., Long, X., Zhang, Y., Wang, Y., You, G., Guo, W., et al. (2023). FTO-targeted siRNA delivery by MSC-derived exosomes synergistically alleviates dopaminergic neuronal death in Parkinson's disease via m6A-dependent regulation of ATM mRNA. J. Transl. Med. 21 (1), 652. doi:10.1186/s12967-023-04461-4
Han, Q. F., Li, W. J., Hu, K. S., Gao, J., Zhai, W. L., Yang, J. H., et al. (2022). Exosome biogenesis: machinery, regulation, and therapeutic implications in cancer. Mol. Cancer 21 (1), 207. doi:10.1186/s12943-022-01671-0
Han, R., Guo, H., Shi, J., Wang, H., Zhao, S., Jia, Y., et al. (2023). Tumour microenvironment changes after osimertinib treatment resistance in non-small cell lung cancer. Eur. J. Cancer 189, 112919. doi:10.1016/j.ejca.2023.05.007
He, Z., Li, W., Zheng, T., Liu, D., and Zhao, S. (2020). Human umbilical cord mesenchymal stem cells-derived exosomes deliver microRNA-375 to downregulate ENAH and thus retard esophageal squamous cell carcinoma progression. J. Exp. Clin. Cancer Res. 39 (1), 140. doi:10.1186/s13046-020-01631-w
Hinestrosa, J. P., Kurzrock, R., Lewis, J. M., Schork, N. J., Schroeder, G., Kamat, A. M., et al. (2022). Early-stage multi-cancer detection using an extracellular vesicle protein-based blood test. Commun. Med. (Lond). 2, 29. doi:10.1038/s43856-022-00088-6
Hu, R., Bi, R., Jiang, L., Xiao, H., Xie, X., Liu, H., et al. (2021). LncRNA RP11-465B22.8 triggers esophageal cancer progression by targeting miR-765/KLK4 axis. Cell Death Discov. 7 (1), 262. doi:10.1038/s41420-021-00631-9
Huang, C., Zhou, Y., Feng, X., Wang, J., Li, Y., and Yao, X. (2023). Delivery of engineered primary tumor-derived exosomes effectively suppressed the colorectal cancer chemoresistance and liver metastasis. ACS Nano 17 (11), 10313–10326. doi:10.1021/acsnano.3c00668
Huang, L., Wang, Y., Chen, J., Wang, Y., Zhao, Y., Wang, Y., et al. (2019). Long noncoding RNA PCAT1, a novel serum-based biomarker, enhances cell growth by sponging miR-326 in oesophageal squamous cell carcinoma. Cell Death Dis. 10 (7), 513. doi:10.1038/s41419-019-1745-4
Jang, Y., Kim, H., Yoon, S., Lee, H., Hwang, J., Jung, J., et al. (2021). Exosome-based photoacoustic imaging guided photodynamic and immunotherapy for the treatment of pancreatic cancer. J. Control Release 330, 293–304. doi:10.1016/j.jconrel.2020.12.039
Jiang, B., Zhang, T., Liu, S., Sheng, Y., and Hu, J. (2024). Polydopamine-assisted aptamer-carrying tetrahedral DNA microelectrode sensor for ultrasensitive electrochemical detection of exosomes. J. Nanobiotechnology 22 (1), 55. doi:10.1186/s12951-024-02318-6
Jin, Y., Meng, Q., Zhang, B., Xie, C., Chen, X., Tian, B., et al. (2021). Cancer-associated fibroblasts-derived exosomal miR-3656 promotes the development and progression of esophageal squamous cell carcinoma via the ACAP2/PI3K-AKT signaling pathway. Int. J. Biol. Sci. 17 (14), 3689–3701. doi:10.7150/ijbs.62571
Jin, Y., Xing, J., Xu, K., Liu, D., and Zhuo, Y. (2022). Exosomes in the tumor microenvironment: promoting cancer progression. Front. Immunol. 13, 1025218. doi:10.3389/fimmu.2022.1025218
Kalluri, R., and LeBleu, V. S. (2020). The biology, function, and biomedical applications of exosomes. Science 367 (6478), eaau6977. doi:10.1126/science.aau6977
Kim, D. H., Park, H., Choi, Y. J., Im, K., Lee, C. W., Kim, D. S., et al. (2023). Identification of exosomal microRNA panel as diagnostic and prognostic biomarker for small cell lung cancer. Biomark. Res. 11 (1), 80. doi:10.1186/s40364-023-00517-1
Kim, M. S., Haney, M. J., Zhao, Y., Mahajan, V., Deygen, I., Klyachko, N. L., et al. (2016). Development of exosome-encapsulated paclitaxel to overcome MDR in cancer cells. Nanomedicine 12 (3), 655–664. doi:10.1016/j.nano.2015.10.012
Kim, S., Kim, G. H., Park, S. J., Kwon, C. H., I, H., Lee, M. W., et al. (2022). Exosomal MicroRNA analyses in esophageal squamous cell carcinoma cell lines. J. Clin. Med. 11 (15), 4426. doi:10.3390/jcm11154426
Kimiz-Gebologlu, I., and Oncel, S. S. (2022). Exosomes: large-scale production, isolation, drug loading efficiency, and biodistribution and uptake. J. Control Release 347, 533–543. doi:10.1016/j.jconrel.2022.05.027
Ko, M., Kim, H. J., Park, J., Lee, H., Lee, K. N., Kim, K., et al. (2023). Isolation of bovine milk exosome using electrophoretic oscillation assisted tangential flow filtration with antifouling of micro-ultrafiltration membrane filters. ACS Appl. Mater Interfaces 15 (21), 26069–26080. doi:10.1021/acsami.3c00446
Li, C., Teixeira, A. F., Zhu, H. J., and Ten Dijke, P. (2021b). Cancer associated-fibroblast-derived exosomes in cancer progression. Mol. Cancer 20 (1), 154. doi:10.1186/s12943-021-01463-y
Li, H., Zhang, S., Zhou, J., Tong, F., Gong, J., Zha, Z., et al. (2023a). Endoscopic surveillance for premalignant esophageal lesions: a community-based multicenter, prospective cohort study. Clin. Gastroenterol. Hepatol. 21 (3), 653–662.e8. doi:10.1016/j.cgh.2022.04.039
Li, J., Zhang, Y., Dong, P. Y., Yang, G. M., and Gurunathan, S. (2023c). A comprehensive review on the composition, biogenesis, purification, and multifunctional role of exosome as delivery vehicles for cancer therapy. Biomed. Pharmacother. 165, 115087. doi:10.1016/j.biopha.2023.115087
Li, K., Lin, Y., Luo, Y., Xiong, X., Wang, L., Durante, K., et al. (2022a). A signature of saliva-derived exosomal small RNAs as predicting biomarker for esophageal carcinoma: a multicenter prospective study. Mol. Cancer 21 (1), 21. doi:10.1186/s12943-022-01499-8
Li, K., Lin, Y., Zhou, Y., Xiong, X., Wang, L., Li, J., et al. (2023b). Salivary extracellular MicroRNAs for early detection and prognostication of esophageal cancer: a clinical study. Gastroenterology 165 (4), 932–945.e9. doi:10.1053/j.gastro.2023.06.021
Li, M., Wan, X., Wang, Y., Sun, Y., Yang, G., and Wang, L. (2017). Time trends of esophageal and gastric cancer mortality in China, 1991-2009: an age-period-cohort analysis. Sci. Rep. 7 (1), 6797. doi:10.1038/s41598-017-07071-5
Li, P., Liu, X., Xing, W., Qiu, H., Li, R., Liu, S., et al. (2022b). Exosome-derived miR-200a promotes esophageal cancer cell proliferation and migration via the mediating Keap1 expression. Mol. Cell Biochem. 477 (4), 1295–1308. doi:10.1007/s11010-022-04353-z
Li, S., Liu, J., Liu, S., Jiao, W., and Wang, X. (2021c). Chitosan oligosaccharides packaged into rat adipose mesenchymal stem cells-derived extracellular vesicles facilitating cartilage injury repair and alleviating osteoarthritis. J. Nanobiotechnology 19 (1), 343. doi:10.1186/s12951-021-01086-x
Li, S., Yi, M., Dong, B., Tan, X., Luo, S., and Wu, K. (2021a). The role of exosomes in liquid biopsy for cancer diagnosis and prognosis prediction. Int. J. Cancer 148 (11), 2640–2651. doi:10.1002/ijc.33386
Liang, H., Wang, X., Li, F., Xie, Y., Shen, J., Wang, X., et al. (2023). Label-free plasmonic metasensing of PSA and exosomes in serum for rapid high-sensitivity diagnosis of early prostate cancer. Biosens. Bioelectron. 235, 115380. doi:10.1016/j.bios.2023.115380
Lin, Y., Dong, H., Deng, W., Lin, W., Li, K., Xiong, X., et al. (2019). Evaluation of salivary exosomal chimeric GOLM1-NAA35 RNA as a potential biomarker in esophageal carcinoma. Clin. Cancer Res. 25 (10), 3035–3045. doi:10.1158/1078-0432.CCR-18-3169
Lin, Z., Wu, Y., Xu, Y., Li, G., Li, Z., and Liu, T. (2022). Mesenchymal stem cell-derived exosomes in cancer therapy resistance: recent advances and therapeutic potential. Mol. Cancer 21 (1), 179. doi:10.1186/s12943-022-01650-5
Liu, L., Ye, Y., Lin, R., Liu, T., Wang, S., Feng, Z., et al. (2024b). Ferroptosis: a promising candidate for exosome-mediated regulation in different diseases. Cell Commun. Signal 22 (1), 6. doi:10.1186/s12964-023-01369-w
Liu, S., Lin, Z., Zheng, Z., Rao, W., Lin, Y., Chen, H., et al. (2020b). Serum exosomal microRNA-766-3p expression is associated with poor prognosis of esophageal squamous cell carcinoma. Cancer Sci. 111 (10), 3881–3892. doi:10.1111/cas.14550
Liu, T., Du, L. T., Wang, Y. S., Gao, S. Y., Li, J., Li, P. L., et al. (2020a). Development of a novel serum exosomal MicroRNA nomogram for the preoperative prediction of lymph node metastasis in esophageal squamous cell carcinoma. Front. Oncol. 10, 573501. doi:10.3389/fonc.2020.573501
Liu, Y., Li, M., Liu, H., Kang, C., and Wang, C. (2024a). Cancer diagnosis using label-free SERS-based exosome analysis. Theranostics 14 (5), 1966–1981. doi:10.7150/thno.92621
Liu, Z., Han, Y., Dang, Q., Xu, H., Zhang, Y., Duo, M., et al. (2022). Roles of circulating tumor DNA in PD-1/PD-L1 immune checkpoint Inhibitors: current evidence and future directions. Int. Immunopharmacol. 111, 109173. doi:10.1016/j.intimp.2022.109173
Liu, Z., Huang, Y., Liang, W., Bai, J., Feng, H., Fang, Z., et al. (2021). Cascaded filter deterministic lateral displacement microchips for isolation and molecular analysis of circulating tumor cells and fusion cells. Lab. Chip 21 (15), 2881–2891. doi:10.1039/d1lc00360g
Luo, A., Zhou, X., Shi, X., Zhao, Y., Men, Y., Chang, X., et al. (2019). Exosome-derived miR-339-5p mediates radiosensitivity by targeting Cdc25A in locally advanced esophageal squamous cell carcinoma. Oncogene 38 (25), 4990–5006. doi:10.1038/s41388-019-0771-0
Luo, H. L., Huang, M. D., Guo, J. N., Fan, R. H., Xia, X. T., He, J. D., et al. (2016). AFAP1-AS1 is upregulated and promotes esophageal squamous cell carcinoma cell proliferation and inhibits cell apoptosis. Cancer Med. 5 (10), 2879–2885. doi:10.1002/cam4.848
Ma, X., Yao, M., Gao, Y., Yue, Y., Li, Y., Zhang, T., et al. (2022). Functional immune cell-derived exosomes engineered for the trilogy of radiotherapy sensitization. Adv. Sci. (Weinh) 9 (23), e2106031. doi:10.1002/advs.202106031
Markou, A., Tzanikou, E., and Lianidou, E. (2022). The potential of liquid biopsy in the management of cancer patients. Semin. Cancer Biol. 84, 69–79. doi:10.1016/j.semcancer.2022.03.013
Martins, T. S., Vaz, M., and Henriques, A. G. (2023). A review on comparative studies addressing exosome isolation methods from body fluids. Anal. Bioanal. Chem. 415 (7), 1239–1263. doi:10.1007/s00216-022-04174-5
McAndrews, K. M., Xiao, F., Chronopoulos, A., LeBleu, V. S., Kugeratski, F. G., and Kalluri, R. (2021). Exosome-mediated delivery of CRISPR/Cas9 for targeting of oncogenic Kras(G12D) in pancreatic cancer. Life Sci. Alliance 4 (9), e202000875. doi:10.26508/lsa.202000875
McLaren, D. B., and Aitman, T. J. (2023). Redefining precision radiotherapy through liquid biopsy. Br. J. Cancer 129 (6), 900–903. doi:10.1038/s41416-023-02398-5
Mishra, A., Bharti, P. S., Rani, N., Nikolajeff, F., and Kumar, S. (2023). A tale of exosomes and their implication in cancer. Biochim. Biophys. Acta Rev. Cancer 1878 (4), 188908. doi:10.1016/j.bbcan.2023.188908
Miyoshi, J., Zhu, Z., Luo, A., Toden, S., Zhou, X., Izumi, D., et al. (2022). A microRNA-based liquid biopsy signature for the early detection of esophageal squamous cell carcinoma: a retrospective, prospective and multicenter study. Mol. Cancer 21 (1), 44. doi:10.1186/s12943-022-01507-x
Morgan, E., Soerjomataram, I., Rumgay, H., Coleman, H. G., Thrift, A. P., Vignat, J., et al. (2022). The global landscape of esophageal squamous cell carcinoma and esophageal adenocarcinoma incidence and mortality in 2020 and projections to 2040: new estimates from GLOBOCAN 2020. Gastroenterology 163 (3), 649–658.e2. doi:10.1053/j.gastro.2022.05.054
Mun, B., Kim, R., Jeong, H., Kang, B., Kim, J., Son, H. Y., et al. (2023). An immuno-magnetophoresis-based microfluidic chip to isolate and detect HER2-Positive cancer-derived exosomes via multiple separation. Biosens. Bioelectron. 239, 115592. doi:10.1016/j.bios.2023.115592
Ning, X. Y., Ma, J. H., He, W., and Ma, J. T. (2023). Role of exosomes in metastasis and therapeutic resistance in esophageal cancer. World J. Gastroenterol. 29 (42), 5699–5715. doi:10.3748/wjg.v29.i42.5699
Pan, L., Zhang, C., Zhang, H., Ke, T., Bian, M., Yang, Y., et al. (2023a). Osteoclast-derived exosomal miR-5134-5p interferes with alveolar bone homeostasis by targeting the JAK2/STAT3 Axis. Int. J. Nanomedicine 18, 3727–3744. doi:10.2147/IJN.S413692
Pan, T., Wu, Y., Zhang, X., Wang, J., Wang, X., Gu, Q., et al. (2023b). Lens epithelial cell-derived exosome inhibits angiogenesis in ocular pathological neovascularization through its delivery of miR-146a-5p. Faseb J. 37 (10), e23192. doi:10.1096/fj.202301020RR
Pei, S., Sun, W., Han, Q., Wang, H., and Liang, Q. (2024). Bifunctional immunoaffinity magnetic nanoparticles for high-efficiency separation of exosomes based on host-guest interaction. Talanta 272, 125790. doi:10.1016/j.talanta.2024.125790
Peswani Sajnani, S. L., Zhang, Y., and Vllasaliu, D. (2021). Exosome-based therapies for mucosal delivery. Int. J. Pharm. 608, 121087. doi:10.1016/j.ijpharm.2021.121087
Preethi, K. A., Selvakumar, S. C., Ross, K., Jayaraman, S., Tusubira, D., and Sekar, D. (2022). Liquid biopsy: exosomal microRNAs as novel diagnostic and prognostic biomarkers in cancer. Mol. Cancer 21 (1), 54. doi:10.1186/s12943-022-01525-9
Qi, R., Bai, Y., Li, K., Liu, N., Xu, Y., Dal, E., et al. (2023). Cancer-associated fibroblasts suppress ferroptosis and induce gemcitabine resistance in pancreatic cancer cells by secreting exosome-derived ACSL4-targeting miRNAs. Drug Resist Updat 68, 100960. doi:10.1016/j.drup.2023.100960
Qiao, X, Cheng, Z, Xue, K, Xiong, C, Zheng, Z, Jin, X, et al. (2023). Tumor-associated macrophage-derived exosomes LINC01592 induce the immune escape of esophageal cancer by decreasing MHC-I surface expression. J Exp Clin Cancer Res 42 (1), 289. doi:10.1186/s13046-023-02871-2
Rao, D., Lu, H., Wang, X., Lai, Z., Zhang, J., and Tang, Z. (2023). Tissue-derived exosome proteomics identifies promising diagnostic biomarkers for esophageal cancer. Elife 12, e86209. doi:10.7554/eLife.86209
Roux, Q., Boiy, R., De Vuyst, F., Tkach, M., Pinheiro, C., de Geyter, S., et al. (2023). Depletion of soluble cytokines unlocks the immunomodulatory bioactivity of extracellular vesicles. J. Extracell Vesicles 12 (8), e12339. doi:10.1002/jev2.12339
Shen, A. R., Jin, X. X., Tang, T. T., Ding, Y., Liu, X. T., Zhong, X., et al. (2022). Exosomal vaccine loading T cell epitope peptides of SARS-CoV-2 induces robust CD8(+) T cell response in HLA-A transgenic mice. Int. J. Nanomedicine 17, 3325–3341. doi:10.2147/IJN.S367494
Shin, H., Choi, B. H., Shim, O., Kim, J., Park, Y., Cho, S. K., et al. (2023). Single test-based diagnosis of multiple cancer types using Exosome-SERS-AI for early stage cancers. Nat. Commun. 14 (1), 1644. doi:10.1038/s41467-023-37403-1
Sidhom, K., Obi, P. O., and Saleem, A. (2020). A review of exosomal isolation methods: is size exclusion chromatography the best option? Int. J. Mol. Sci. 21 (18), 6466. doi:10.3390/ijms21186466
Sun, Y., Wang, J., Ma, Y., Li, J., Sun, X., Zhao, X., et al. (2021). Radiation induces NORAD expression to promote ESCC radiotherapy resistance via EEPD1/ATR/Chk1 signalling and by inhibiting pri-miR-199a1 processing and the exosomal transfer of miR-199a-5p. J. Exp. Clin. Cancer Res. 40 (1), 306. doi:10.1186/s13046-021-02084-5
Sung, H., Ferlay, J., Siegel, R. L., Laversanne, M., Soerjomataram, I., Jemal, A., et al. (2021). Global cancer statistics 2020: GLOBOCAN estimates of incidence and mortality worldwide for 36 cancers in 185 countries. CA Cancer J. Clin. 71 (3), 209–249. doi:10.3322/caac.21660
Szwedowicz, U., Łapińska, Z., Gajewska-Naryniecka, A., and Choromańska, A. (2022). Exosomes and other extracellular vesicles with high therapeutic potential: their applications in oncology, neurology, and dermatology. Molecules 27 (4), 1303. doi:10.3390/molecules27041303
Takahashi, K., Takeda, Y., Ono, Y., Isomoto, H., and Mizukami, Y. (2023). Current status of molecular diagnostic approaches using liquid biopsy. J. Gastroenterol. 58 (9), 834–847. doi:10.1007/s00535-023-02024-4
Ten, A., Kumeiko, V., Farniev, V., Gao, H., and Shevtsov, M. (2024). Tumor microenvironment modulation by cancer-derived extracellular vesicles. Cells 13 (8), 682. doi:10.3390/cells13080682
GBD 2017 Oesophageal Cancer Collaborators (2020). The global, regional, and national burden of oesophageal cancer and its attributable risk factors in 195 countries and territories, 1990-2017: a systematic analysis for the Global Burden of Disease Study 2017. Lancet Gastroenterol. Hepatol. 5 (6), 582–597. doi:10.1016/S2468-1253(20)30007-8
Tian, J., Han, Z., Song, D., Peng, Y., Xiong, M., Chen, Z., et al. (2023). Engineered exosome for drug delivery: recent development and clinical applications. Int. J. Nanomedicine 18, 7923–7940. doi:10.2147/IJN.S444582
Tong, Y. S., Wang, X. W., Zhou, X. L., Liu, Z. H., Yang, T. X., Shi, W. H., et al. (2015). Identification of the long non-coding RNA POU3F3 in plasma as a novel biomarker for diagnosis of esophageal squamous cell carcinoma. Mol. Cancer 14, 3. doi:10.1186/1476-4598-14-3
Triantafyllou, T., and Wijnhoven, B. P. L. (2020). Current status of esophageal cancer treatment. Chin. J. Cancer Res. 32 (3), 271–286. doi:10.21147/j.issn.1000-9604.2020.03.01
Tutrone, R., Lowentritt, B., Neuman, B., Donovan, M. J., Hallmark, E., Cole, T. J., et al. (2023). ExoDx prostate test as a predictor of outcomes of high-grade prostate cancer - an interim analysis. Prostate Cancer Prostatic Dis. 26 (3), 596–601. doi:10.1038/s41391-023-00675-1
Vahabi, M., Comandatore, A., Franczak, M. A., Smolenski, R. T., Peters, G. J., Morelli, L., et al. (2023). Role of exosomes in transferring chemoresistance through modulation of cancer glycolytic cell metabolism. Cytokine Growth Factor Rev. 73, 163–172. doi:10.1016/j.cytogfr.2023.07.004
Wang, D., Wang, S., Jin, M., Zuo, Y., Wang, J., Niu, Y., et al. (2024). Hypoxic exosomal circPLEKHM1-mediated crosstalk between tumor cells and macrophages drives lung cancer metastasis. Adv. Sci. (Weinh) 11 (22), e2309857. doi:10.1002/advs.202309857
Wang, N., Yao, C., Luo, C., Liu, S., Wu, L., Hu, W., et al. (2023b). Integrated plasma and exosome long noncoding RNA profiling is promising for diagnosing non-small cell lung cancer. Clin. Chem. Lab. Med. 61 (12), 2216–2228. doi:10.1515/cclm-2023-0291
Wang, P., Wang, H., Huang, Q., Peng, C., Yao, L., Chen, H., et al. (2019). Exosomes from M1-polarized macrophages enhance paclitaxel antitumor activity by activating macrophages-mediated inflammation. Theranostics 9 (6), 1714–1727. doi:10.7150/thno.30716
Wang, X., Huang, J., Chen, W., Li, G., Li, Z., and Lei, J. (2022). The updated role of exosomal proteins in the diagnosis, prognosis, and treatment of cancer. Exp. Mol. Med. 54 (9), 1390–1400. doi:10.1038/s12276-022-00855-4
Wang, X., Liang, F., Wang, X., Wu, Y., Wang, D., Cheng, Y., et al. (2023c). Quality of life and survival outcomes of patients with inoperable esophageal squamous cell carcinoma after definitive radiation therapy: a multicenter retrospective observational study in China from 2015 to 2016. J. Natl. Cancer Cent. 3 (No.2), 150–158. doi:10.1016/j.jncc.2023.05.001
Wang, Z., Kim, S. Y., Tu, W., Kim, J., Xu, A., Yang, Y. M., et al. (2023a). Extracellular vesicles in fatty liver promote a metastatic tumor microenvironment. Cell Metab. 35 (7), 1209–1226.e13. doi:10.1016/j.cmet.2023.04.013
Welsh, J. A., Goberdhan, D. C. I., O'Driscoll, L., Buzas, E. I., Blenkiron, C., Bussolati, B., et al. (2024). Minimal information for studies of extracellular vesicles (MISEV2023): from basic to advanced approaches. J. Extracell. Vesicles 13 (2), e12404. doi:10.1002/jev2.12404
Wu, X., Shi, M., Lian, Y., and Zhang, H. (2023). Exosomal circRNAs as promising liquid biopsy biomarkers for glioma. Front. Immunol. 14, 1039084. doi:10.3389/fimmu.2023.1039084
Xia, B., Liu, Y., Wang, J., Lu, Q., Lv, X., Deng, K., et al. (2023b). Emerging role of exosome-shuttled noncoding RNAs in gastrointestinal cancers: from intercellular crosstalk to clinical utility. Pharmacol. Res. 195, 106880. doi:10.1016/j.phrs.2023.106880
Xia, Q., Zheng, J., Bu, J., Li, R., Li, X., Fan, S., et al. (2023a). Mn(2+)-modified black phosphorus nanosensor for detection of exosomal microRNAs and exosomes. Mikrochim. Acta 190 (8), 295. doi:10.1007/s00604-023-05880-2
Xia, Y., Zhang, J., Liu, G., and Wolfram, J. (2024). Immunogenicity of extracellular vesicles. Adv. Mater 36 (33), e2403199. doi:10.1002/adma.202403199
Xie, X., Lian, S., Zhou, Y., Li, B., Lu, Y., Yeung, I., et al. (2021). Tumor-derived exosomes can specifically prevent cancer metastatic organotropism. J. Control Release 331, 404–415. doi:10.1016/j.jconrel.2021.01.030
Xing, S., Zheng, X., Wei, L. Q., Song, S. J., Liu, D., Xue, N., et al. (2017). Development and validation of a serum biomarker panel for the detection of esophageal squamous cell carcinoma through RNA transcriptome sequencing. J. Cancer 8 (12), 2346–2355. doi:10.7150/jca.19465
Xiong, Y. H., Fan, X. G., Chen, Y. Y., Huang, Y., Quan, J., Yi, P. P., et al. (2022). Comparison of methods of isolating extracellular vesicle microRNA from HepG2 cells for High-throughput sequencing. Front. Mol. Biosci. 9, 976528. doi:10.3389/fmolb.2022.976528
Xu, Y., Zhang, C., Cai, D., Zhu, R., and Cao, Y. (2023). Exosomal miR-155-5p drives widespread macrophage M1 polarization in hypervirulent Klebsiella pneumoniae-induced acute lung injury via the MSK1/p38-MAPK axis. Cell Mol. Biol. Lett. 28 (1), 92. doi:10.1186/s11658-023-00505-1
Yu, D., Li, Y., Wang, M., Gu, J., Xu, W., Cai, X., et al. (2022). Exosomes as a new frontier of cancer liquid biopsy. Mol Cancer 21 (1), 56. doi:10.1186/s12943-022-01509-9
Zhan, Q., Yi, K., Cui, X., Li, X., Yang, S., Wang, Q., et al. (2022). Blood exosomes-based targeted delivery of cPLA2 siRNA and metformin to modulate glioblastoma energy metabolism for tailoring personalized therapy. Neuro Oncol. 24 (11), 1871–1883. doi:10.1093/neuonc/noac071
Zhang, C., Guo, Z., and Jing, Z. (2024). Prediction of response to chemoradiotherapy by dynamic changes of circulating exosome levels in patients with esophageal squamous cell carcinoma. Int. J. Nanomedicine 19, 1351–1362. doi:10.2147/IJN.S440684
Zhang, L. S., Chen, Q. C., Zong, H. T., and Xia, Q. (2023). Exosome miRNA-203 promotes M1 macrophage polarization and inhibits prostate cancer tumor progression. Mol. Cell Biochem. 479, 2459–2470. doi:10.1007/s11010-023-04854-5
Zhang, Y., Chen, C., Liu, Z., Guo, H., Lu, W., Hu, W., et al. (2022). PABPC1-induced stabilization of IFI27 mRNA promotes angiogenesis and malignant progression in esophageal squamous cell carcinoma through exosomal miRNA-21-5p. J. Exp. Clin. Cancer Res. 41 (1), 111. doi:10.1186/s13046-022-02339-9
Zhao, A. G., Shah, K., Cromer, B., and Sumer, H. (2022a). Comparative analysis of extracellular vesicles isolated from human mesenchymal stem cells by different isolation methods and visualisation of their uptake. Exp. Cell Res. 414 (2), 113097. doi:10.1016/j.yexcr.2022.113097
Zhao, Q., Huang, L., Qin, G., Qiao, Y., Ren, F., Shen, C., et al. (2021b). Cancer-associated fibroblasts induce monocytic myeloid-derived suppressor cell generation via IL-6/exosomal miR-21-activated STAT3 signaling to promote cisplatin resistance in esophageal squamous cell carcinoma. Cancer Lett. 518, 35–48. doi:10.1016/j.canlet.2021.06.009
Zhao, Y., Li, X., Zhang, W., Yu, L., Wang, Y., Deng, Z., et al. (2021a). Trends in the biological functions and medical applications of extracellular vesicles and analogues. Acta Pharm. Sin. B 11 (8), 2114–2135. doi:10.1016/j.apsb.2021.03.012
Zhao, Y., Liu, L., Sun, R., Cui, G., Guo, S., Han, S., et al. (2022b). Exosomes in cancer immunoediting and immunotherapy. Asian J. Pharm. Sci. 17 (2), 193–205. doi:10.1016/j.ajps.2021.12.001
Zhao, Y., Shen, M., Wu, L., Yang, H., Yao, Y., Yang, Q., et al. (2023). Stromal cells in the tumor microenvironment: accomplices of tumor progression? Cell Death Dis. 14 (9), 587. doi:10.1038/s41419-023-06110-6
Zhou, M., He, X., Mei, C., and Ou, C. (2023). Exosome derived from tumor-associated macrophages: biogenesis, functions, and therapeutic implications in human cancers. Biomark. Res. 11 (1), 100. doi:10.1186/s40364-023-00538-w
Zhou, M., Wang, H., Zeng, X., Yin, P., Zhu, J., Chen, W., et al. (2019). Mortality, morbidity, and risk factors in China and its provinces, 1990-2017: a systematic analysis for the Global Burden of Disease Study 2017. Lancet 394 (10204), 1145–1158. doi:10.1016/S0140-6736(19)30427-1
Zhu, Q., Huang, L., Yang, Q., Ao, Z., Yang, R., Krzesniak, J., et al. (2021). Metabolomic analysis of exosomal-markers in esophageal squamous cell carcinoma. Nanoscale 13 (39), 16457–16464. doi:10.1039/d1nr04015d
Zhu, Q., Xu, H., Huang, L., Luo, J., Li, H., Yang, R., et al. (2023b). Identification and detection of plasma extracellular vesicles-derived biomarkers for esophageal squamous cell carcinoma diagnosis. Biosens. Bioelectron. 225, 115088. doi:10.1016/j.bios.2023.115088
Keywords: exosomes, liquid biopsy, esophageal cancer, early diagnosis, adjuvant therapy
Citation: Zihan R, Jingsi C, Lingwen D, Xin L and Yan Z (2024) Exosomes in esophageal cancer: a promising frontier for liquid biopsy in diagnosis and therapeutic monitoring. Front. Pharmacol. 15:1459938. doi: 10.3389/fphar.2024.1459938
Received: 05 July 2024; Accepted: 28 November 2024;
Published: 17 December 2024.
Edited by:
Lei Huang, University of Massachusetts Medical School, United StatesReviewed by:
Zhenyu Dai, Stanford University, United StatesLili Sun, The University of Utah, United States
Hui Xing, Houston Methodist Research Institute, United States
Copyright © 2024 Zihan, Jingsi, Lingwen, Xin and Yan. This is an open-access article distributed under the terms of the Creative Commons Attribution License (CC BY). The use, distribution or reproduction in other forums is permitted, provided the original author(s) and the copyright owner(s) are credited and that the original publication in this journal is cited, in accordance with accepted academic practice. No use, distribution or reproduction is permitted which does not comply with these terms.
*Correspondence: Zhang Yan, emhhbmd5YW4yMDE2QGpsdS5lZHUuY24=