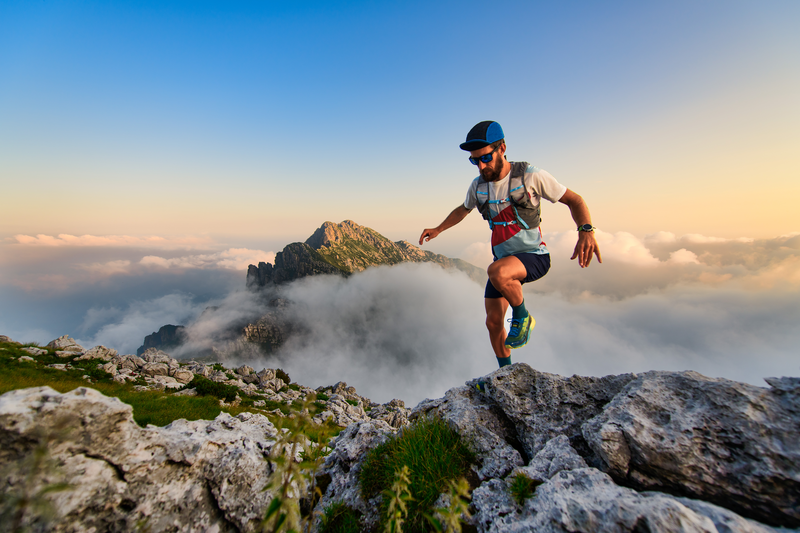
95% of researchers rate our articles as excellent or good
Learn more about the work of our research integrity team to safeguard the quality of each article we publish.
Find out more
ORIGINAL RESEARCH article
Front. Pharmacol. , 25 October 2024
Sec. Pharmacology of Infectious Diseases
Volume 15 - 2024 | https://doi.org/10.3389/fphar.2024.1456741
Purpose: Lefamulin is the first pleuromutilin antibiotic approved for the treatment of community-acquired bacterial pneumonia (CABP). However, the pharmacokinetic/pharmacodynamic (PK/PD) characteristics in Chinese CABP patients are not fully understood. This study aimed to evaluate its microbiological efficacy against Streptococcus pneumoniae and Staphylococcus aureus via PK/PD analysis.
Methods: The population PK (PopPK) model, established with foreign data was validated using data from Chinese CABP patients. PK/PD analysis was conducted for the intravenous administration of 150 mg q12 h for 1-h, 1.5-h and extended 2-h infusion. Oral administrations of 600 mg q12 h were assessed, considering original and higher plasma protein binding.
Results: Lefamulin displayed similar PK characteristics in both Chinese and Western populations. The PopPK model effectively predicted lefamulin concentrations in Chinese CABP patients. Under the dosage regimen of 150 mg q12 h via intravenous infusion for 1/1.5/2 h, the probability of target attainments reached 98% at the minimum inhibitory concentration for both 90% S. pneumoniae and S. aureus, considering both original and higher protein binding rates. It is advisable to extend the infusion duration from 1/1.5 h–2 h to minimize the risk of adverse effects at the infusion site. Regardless of fasted or fed conditions, the PTAs for 600 mg q12 h lefamulin via oral administration proved comparable to those for intravenous administration.
Conclusion: This study proved that intravenous and oral administrations of lefamulin can reach preclinical PK/PD targets of S. pneumoniae and S. aureus. These findings support the optimal use of lefamulin for the safe and effective treatment of Chinese CABP patients.
Lefamulin, the first systemic pleuromutilin antibiotic for treating community-acquired bacterial pneumonia (CABP) (Chahine and Sucher, 2020; Zhanel et al., 2021), has been approved by the U.S. Food and Drug Administration (FDA) in 2019 (3), the European Medicines Agency (EMA) in 2020, and the China National Medical Products Administration (NMPA) in 2023, respectively. It exhibits a broad spectrum of antimicrobial activity against respiratory pathogens, including Staphylococcus aureus and Streptococcus pneumoniae regardless of resistance phenotype or genotype (Zhanel et al., 2021; Wu et al., 2020). Recommended dosing regimens involve either a 1/1.5-h intravenous infusion of 150 mg q12 h, oral administration of 600 mg q12 h, or a sequential combination of intravenous and oral administration (Xenleta, 2019; Covvey and Guarascio, 2022; Lefamulin Acetate Concentrated Solution for Injection, 2023). Clinical development of lefamulin in China commenced in 2019, with completed studies including a clinical trial in healthy subjects (Hu et al., 2023) and an efficacy comparison study with moxifloxacin in CABP patients. The pharmacokinetic similarities between Chinese and Western populations, as well as the pharmacokinetic/pharmacodynamic (PK/PD) relationships in Chinese patients, remain unclear. Bhavnani et al. (2019) evaluated lefamulin PK/PD based on pharmacokinetic data from healthy volunteers. However, the exposure of lefamulin in patients with CABP was higher than that in healthy volunteers which will affect the PK/PD evaluation. From the clinical pharmacology report of FDA, the mean AUC0-24 and Cmax in CABP patients was approximately 1.73- and 1.3-fold greater compared to adults without pneumonia following the therapeutic IV and PO dosing regimens on Day 1 (9). This study compared PK similarities of lefamulin in Chinese and Western populations and conducted PK/PD analysis to assess its microbiological efficacy against S. pneumoniae (S. pneumoniae) and S. aureus (S. aureus) with both intravenous and oral administrations in patients with CABP. Additionally, the lefamulin package insert notes adverse reactions related to intravenous formulations, specifically administration site reactions, with an incidence of 7% (19/273) (Xenleta, 2019). To address above situation, this study proposed extending the infusion time from 1/1.5 h–2 h and evaluating the microbiological efficacy under extended infusion through PK/PD analysis.
The population pharmacokinetic (PopPK) data from Chinese CABP patients was used as an external validation dataset to assess the applicability of the PopPK model established based on foreign data in predicting lefamulin concentrations in Chinese CABP patients. Considering that the drug exposure at the infection site is more relevant to microbiological efficacy (Levison and Levison, 2009), this study simultaneously simulated the lefamulin exposure in plasma and epithelial lining fluid (ELF) with intravenous and oral dosage (fasted vs. fed) based on the validated PopPK model (Zhang et al., 2019). PK/PD assessment was conducted using pharmacodynamic data of S. pneumoniae (S. pneumoniae) and S. aureus (S. aureus) from China to evaluate the impact of infusion time and food on exposure, probability of target attainment (PTA), and cumulative fraction of response (CFR).
Plasma protein binding plays a key role in drug therapy, affecting PK and PD. The plasma protein binding parameters of lefamulin used in the established PopPK model were derived from an in vitro study, and the binding rate was 73%–88% (Nabriva Therapeutics, 2019) (original plasma protein binding rate). During the review process, the FDA noted that another in vitro plasma protein binding study resulted in a higher binding rate, with a binding rate of 86%–97% (Nabriva Therapeutics, 2019) (higher plasma protein binding rate). When the FDA conducted a PK/PD compliance probability analysis, it chose to re-estimate the parameters of the existing PopPK model (without changing the structure of the PopPK model) using a higher binding rate (Nabriva Therapeutics, 2019). This study assessed the effects of original and higher plasma protein binding rates on PTA, and anticipated microbial efficacy of lefamulin in Chinese CABP patients. The findings could offer guidance for the clinical application of lefamulin upon its introduction in China, serving as a foundation for practical use.
The PopPK dataset comprises subjects with at least one assessable drug concentration datum. The dataset encompasses a range of information, including plasma drug concentrations, dosing, PK sampling, demographic characteristics, clinical laboratory results, and study phase. This study incorporated data from the Chinese Phase III clinical trial (BC-3781-S301), with a total of 217 plasma drug concentration entries from 33 subjects. Table 1 summarizes the number of subjects, concentrations stratified by administration route, and data below the lower limit of quantitation. The concentrations of lefamulin were determined via liquid chromatography-tandem mass spectrometry after protein precipitation treatment.
Figure 1 illustrates the established PopPK model (Nabriva Therapeutics, 2019). The model consists of one central compartment and two peripheral compartments with parallel rapid and delayed absorption pathways. Additionally, it assumes linear elimination of the drug from the central compartment. The model employs an exponential model to characterize inter-individual variability and utilizes a mixed-effects model incorporating additive and proportional components to describe residuals. Covariates integrated into the model encompass albumin levels, body weight, and study phase.
CL, clearance, CLD1, distributional clearance to the first peripheral compartment, CLD2, distributional clearance to the second peripheral compartment, Vc, volume of distribution of the central compartment, Vp1, volume of distribution for the first peripheral compartment, Vp2, volume of distribution for the second peripheral compartment, Ka, absorption rate constant through the immediate process, Ka2, absorption rate constant through the delayed process, Ftot, total oral bioavailability.
The fixed-effect parameters in the model encompass clearance (CL), distributional clearance to the first peripheral compartment (CLD1), distributional clearance to the second peripheral compartment (CLD2), volume of distribution of the central compartment (Vc), volume of distribution for the first peripheral compartment (Vp1), volume of distribution for the second peripheral compartment (Vp2), absorption rate constant through the immediate process (Ka), absorption rate constant through the delayed process (Ka2), total oral bioavailability (Ftot), fraction of dose absorbed through slow absorption (FS), minimum fraction unbound of the free drug (fu, min), maximum fraction unbound of the free drug (fu, max), concentration at which the fraction unbound is half-maximal (fu50), and lag time (ALAG). These fixed parameters (θx) and their corresponding random-effect parameters (ηx) are described by the following formula (Equation 1).
When PHASE = 3, CLPHASE = 1, when PHASE = 2, CLPHASE=(1+θ21), when PHASE = 1, CLPHASE=(1+θ22). ALB represents the individual plasma albumin level (g/dL), serving as a covariate influencing CL.
When PHASE = 3, Q1PHASE = 1, when PHASE = 2, Q1PHASE=(1+θ23), when PHASE = 1, Q1PHASE=(1+θ24) (Equations 2, 3).
When PHASE = 3, Vp1PHASE = 1, when PHASE = 2, Vp1PHASE=(1+θ25), when PHASE = 1, Vp1PHASE = (1+θ26). WTKG is the individual body weight, serving as a covariate influencing Vp1 (Equation 4). The CLD2 and Vp2 were described as Equations 5, 6, respectively.
In the fasted state, FAST = 1, FEDD = 0. In the fed state, FAST = 0, FEDD = 1 (applicable to Equations 7–9).
When BIO>0,
The fraction of lefamulin in the delayed absorption part, FS, can be used to calculate the bioavailability of immediate absorption (F1) and the bioavailability of delayed absorption (F2) (Equations 11, 12). The calculation formulas are as follows (Equations 13, 14).
When administered intravenously, the default bioavailability is set to 1.
Regarding the lag time, the lag time for immediate absorption and the lag time for delayed absorption are assumed to be equal in duration (Equation 15).
The calculation formulas for the free fraction of lefamulin, which follows a Sigmoid Emax model, are as follows (Equations 16–19).
The concentrations of lefamulin in plasma or ELF were calculated according to the following formulas (Equation 20).
In central compartment, PLASMA = 1, ELF = 0 while in ELF compartment, PLASMA = 0, ELF = 1. IPRED refers to the individual predicted concentrations.
External validation was performed using the Bayesian posterior method of the Nonlinear Mixed Effects Model (NONMEM) to estimate individual predicted concentrations. Model bias and precision were assessed by comparing the model-predicted values with observed values. This assessment included the calculation of mean prediction error (MPE), mean absolute prediction error (MAPE), and root mean square prediction error (RMSE) based on the predicted and observed concentrations of lefamulin. Additionally, MPE%, MAPE%, and RMSE% were utilized to evaluate the accuracy and precision of the model. The predictive performance was further evaluated by goodness of fit (GOF), visual predictive check (VPC), and normalized prediction distribution errors (NPDE).
Based on the lefamulin concentrations in plasma and ELF obtained from foreign clinical studies, a PopPK model incorporating an ELF distribution compartment has been established.
When the original protein binding rate was used, ELF, as an effect compartment, was linked to the central compartment of the PopPK model. The fixed-effect parameters, including the distribution rate constant from the central compartment to the ELF compartment (KELF,in) and the elimination rate constant from the ELF compartment (KEFL,out) for lefamulin, are derived from the previously established model. The model diagram and the parameter values are shown in Figure 2; Supplementary Table S1.
Figure 2. Population pharmacokinetic model of lefamulin integrating epithelial lining fluid compartment.
ELF distribution model from the FDA review report was adopted when a higher protein binding rate was utilized.
LPR (1 mg/L) represents the penetration rate from plasma (lefamulin concentration of 1 mg/L) to ELF, Cp(t) is plasma drug concentration. The exponent power induces changes in permeability as the plasma drug concentration varies. The model parameters are shown in Supplementary Table S2.
The simulation dataset was from a phase III study involving 125 Chinese CABP patients (baseline body weight and albumin levels), a covariate dataset for 5000 CABP patients was generated through a resampling approach. Simulations were conducted for lefamulin 150 mg q12 h intravenous infusion over 1 h, 1.5 h and 2 h, as well as 600 mg q12 h oral administration under fasted or fed conditions. During simulations, numerical integration was employed to calculate the plasma free drug exposure from 0 to 24 h (fAUC0–24h, plasma) and the ELF drug exposure (AUC0–24h, ELF) at steady state (Day 3).
From the Chinese lefamulin pharmacodynamic study, the minimum inhibitory concentration (MIC) distributions of S. pneumoniae and S. aureus isolated between 2017 and 2019 are shown in Supplementary Table S3, (Wu et al., 2020).
The PK/PD indice of lefamulin is fAUC0–24h/MIC (Bhavnani et al., 2019; Rodvold, 2019). In the mouse in vivo pneumonia model, the median targets of free drug in plasma and total drug in ELF were demonstrated in Supplementary Table S4 (Wicha et al., 2019a). Monte Carlo simulation was conducted to estimate the probability target attainment (PTA) and cumulative fraction of response (CFR) of lefamulin against S. pneumoniae and S. aureus. Dosage regimens with PTA and CFR >90% were considered as microbiologically effective.
The descriptive statistics for continuous and categorical covariates of subjects in the modeling dataset and the validation dataset are presented in Supplementary Table S5. In the Chinese Phase III trial, the values of continuous covariates for subjects (including age, height, weight, body surface area, creatinine clearance and albumin) fall within the range observed in foreign subjects, showing similarity with the values observed in the foreign Phase III trial. For categorical covariates (including gender and race), in the Chinese Phase III study, the proportion of male subjects is higher than in the foreign study, with percentages of 81.82% for males in the Chinese study, compared to 57.9% males in the foreign Phase III study. The foreign Phase III study includes subjects from different racial groups, such as White, Black, and Asian populations, with Asians comprising 11.3%. In contrast, the Chinese Phase III study only includes Asian subjects (all Chinese). The final covariates included in the foreign PopPK model are albumin, weight, and study phase.
The distribution characteristics of the concentration-time curves for intravenous (150 mg) and oral (600 mg) administration in the Chinese Phase III study closely resemble the features of corresponding curves observed in foreign studies (Figure 3). The concentration distribution ranges are similar across different administration routes.
Figure 3. Plasma concentration vs. time curves at steady state in foreign and Chinese studies. (A) presents semi-logarithmic drug concentration-time curves of lefamulin from foreign clinical studies stratified by administration route and study phase, with different colors representing distinct study phases. (B) illustrates semi-logarithmic drug concentration-time curves of lefamulin from the Chinese Phase III clinical study, stratified by administration route. IV, intravenous administration, PO, oral administration.
Based on the predictions and observed values of lefamulin concentrations in Chinese patients with CABP, the model prediction errors are summarized in Table 2. The overall MPE is −0.01, with a 95% confidence interval (CI) of −0.08 to 0.06. The MPE% is 10.90%. When administered intravenously and orally, the MPE is 0.02 and −0.07, and the MPE% is 15.60% and −1.70%, respectively. These results indicate that the overall model predictions are close to the observed values, with small relative errors (MPE% ≤ 20%).
The overall MAPE and its relative error MAPE% are 0.27% and 25.90%, respectively. The overall RMSE and RMSE% are 0.45% and 62.70%, respectively. For intravenous and oral administration, the MAPE is 0.30 and 0.19, the MAPE% is 30.0% and 15.30%, the RMSE is 0.50 and 0.24, and the RMSE% is 72.70% and 18.90%, respectively. For composite indicators F20 and F30, which simultaneously represent prediction accuracy and precision, the overall F20 and F30 are 63.93% and 80.33%, respectively. For intravenous administration, F20 and F30 are 61.65% and 76.69%, while for oral administration, F20 and F30 are 70.00% and 90.00%, respectively. These findings suggest that the model adequately describes the validation dataset. (MAPE% ≤ 30%, F20 ≥ 35%, F30 ≥ 50%).
The goodness of fit and conditional weighted residuals (CWRES) distribution for the final lefamulin PopPK model are presented in Figures 4, 5, respectively. As shown in Figure 4, the overall GOF indicates good consistency between individual predicted and observed values, with the trend line coinciding with the diagonal line. This suggests that the lefamulin PopPK model fits well with the concentration observations of Chinese CABP patients. The majority of the overall CWRES are distributed within ±2, and they are relatively evenly distributed around 0. The less accurate predictions at later timepoints may due to the sparse concentration datapoints.
Figure 4. Goodness of fit of lefamulin final PopPK model. The blue dots are observed values, the black lines are diagonal lines, the red lines are loess regression curves.
Figure 5. Histogram of CWRES distribution of lefamulin final PopPK model. (A) CWRES distribution for intravenous and oral administrations. (B) CWRES distribution for intravenous administrations. (C) CWRES distribution for oral administrations. IV, intravenous administration, Oral, oral administration, All, intravenous administration + oral administration.
Similarly, as illustrated in Supplementary Figure S1, the GOF indicates a good fit of the model for both the intravenous and oral administrations. In the histogram of CWRES distribution (Figure 5), the overall CWRES is distributed relatively evenly around 0 (Figure 5A), resembling a normal distribution. This suggests that the model has a minimal bias in predicting lefamulin plasma concentrations, indicating a well-fitted model. The CWRES distribution histograms for different administration routes exhibit a similar pattern to the overall distribution (Figures 5B, C).
Plasma concentrations of lefamulin from 1,000 simulations show that the 95th, 50th, and fifth percentiles of observed plasma concentrations are generally within the 90% prediction intervals for the corresponding percentiles (Figure 6). The VPC results for different administration routes are similar to the overall VPC results (Supplementary Figure S2), indicating that the model exhibits good predictive performance.
The solid red line represents the 50th percentile of observed values, with the dashed red lines from top to bottom indicating the 95th and fifth percentiles of observed values. The solid black line represents the 50th percentile of predicted values, with dashed black lines from top to bottom indicating the 95th and fifth percentiles of predicted values. The blue and red shaded areas represent the 90% confidence intervals for the corresponding predicted percentiles.
The following statistical tests were conducted based on the NPDE: t-test, Fisher test, and Shapiro-Wilks (SW) test. These tests aimed to assess whether the distribution of NPDE significantly differs from a standard normal distribution. As shown in Table 3, the P-values for the overall and intravenous administration in the t-test, Fisher test, and SW test are all less than 0.05. This suggests a significant difference between the distribution of NPDE for the overall dataset and intravenous administration compared to a standard normal distribution. However, for oral administration, the P-values for the t-test and SW test are greater than 0.05, indicating relatively better predictive performance compared to intravenous administration.
The QQ plot and histogram of the overall NPDE show that the distribution is close to a standard normal distribution. In the NPDE vs. time and predicted concentration plot, NPDE is evenly distributed around the X-axis without exhibiting trend-related shifts (Figure 7). However, there are slight differences in the QQ plot and histogram of NPDE for different administration routes. The QQ plot for intravenous administration suggests some deviation in the distribution of NPDE from a standard normal distribution, and the histogram shows a leftward skew (Supplementary Figure S3A). In contrast, the QQ plot for oral administration indicates a distribution that closely follows a standard normal distribution (Supplementary Figure S3B).
Figure 7. Normalized prediction distribution errors of the lefamulin final PopPK model. From the upper left to the lower right are the NPDE-standard normal distribution QQ plot, NPDE histogram, NPDE versus time plot, and NPDE versus predicted concentration plot.
The plasma-free drug and ELF drug exposure achieved with each dosage regimen are shown in Supplementary Figure S4; Supplementary Table S6. The varying infusion times did not impact lefamulin exposure in plasma and ELF. ELF drug exposure levels were comparable under original and higher plasma protein binding rates. While plasma-free drug exposure levels were relatively lower under high protein binding rates. Lefamulin exposure in plasma and ELF achieved via 600 mg q12 h oral administration were similar to those achieved under 150 mg q12 h intravenous administration. In the fed state, the drug exposures decreased by 9%–42% compared to the fasted state.
Based on original and higher plasma protein binding rates, PK/PD analysis was performed based on the lefamulin exposure at steady state with the dosing regimen of 150 mg q12 h infused over 1/1.5/2 h, as well as the oral administration of 600 mg under fasted or fed conditions.
The PTA achieved with oral dose of 600 mg under fasted condition (not shown in Figure 8) was highly similar to those with 150 mg q12 h via a 1/1.5/2 h infusion.When the pharmacodynamic tartet achieving 1-log10 CFU/mL was utilized in PK/PD analysis, all the intravenous and oral administrations can reach PTA of 98% at MIC90 of S. pneumonia and S. aureus with both original and higher protein binding rates. When the pharmacodynamic tartet of 2-log10 CFU/mL was utilized, all the intravenous and oral administrations under fasted condition can reach PTA of 94% at MIC90 of S. pneumonia and S. aureus with both original and higher protein binding rates (Supplementary Table S7; Figure 8).
Figure 8. Probability of target attainment and MIC distribution for Streptococcus pneumoniae and Staphylococcus aureus in plasma and epithelial lining fluid with original and higher plasma protein binding after lefamulin intravenous and oral administration. Lefamulin was administered at 150 mg q12 h via a 1/1.5/2 h infusion or as an oral dose of 600 mg under fasted or fed conditions. PK/PD targets corresponding to 1-log10/2-log10 CFU/mL bacteria reductions were utilized. Original ppb refers to the diluted plasma protein binding, higher ppb refers to the non-diluted plasma protein binding rate. PTA, probability of target attainment, elf, epithelial lining fluid, MIC, minimum inhibitory concentration, S. pneumoniae, Streptococcus pneumoniae, S. aureus, Staphylococcus aureus.
The PK/PD breakpoints of lefamulin with the recommended dosage regimens were almost all higher than MIC50/MIC90 of S. pneumoniae and S. aureus indicating that these two bacteria species are generally susceptible to lefamulin (Supplementary Table S8).
The cumulative response percentages of different bacteria to lefamulin at different protein binding rates are shown in Table 4. Under both lower and higher protein binding rates, the dosing regimen of lefamulin 150 mg q12 h administered via intravenous infusion over 1/1.5/2 h resulted in cumulative response percentages exceeding 95%. This suggests that the proposed regimen achieves a favorable microbiological efficacy in the treatment of community-acquired pneumonia caused by S. pneumoniae and S. aureus.
Table 4. Cumulative fraction of response for Streptococcus pneumoniae and Staphylococcus aureus in plasma and epithelial lining fluid with original and higher plasma protein binding after lefamulin intravenous and oral administration.
Lefamulin is a potential antimicrobial agent for CABP caused by S. pneumoniae and S. aureus (Veve and Wagner, 2018; Eraikhuemen et al., 2021). This study evaluated the PTA and CFR for different administration regimens of intravenous infusion of lefamulin 150 mg q12 h over 1 h, 1.5 h, extending the infusion to 2 h, and oral administration of lefamulin 600 mg q12 h under fasted or fed conditions in the treatment of Chinese patients with CABP. The study also assessed the impact of original and higher plasma protein binding rates on microbiological efficacy.
This study included 183 blood concentration data points from 33 Chinese CABP patients as a validation dataset, validating the lefamulin PopPK model published by the FDA (9). The results indicated that the distribution characteristics of drug concentration-time curves in Chinese patients with CABP were similar to those observed in foreign clinical studies. The validation of the established foreign lefamulin PopPK model using clinical study data from Chinese CABP patients demonstrated acceptable accuracy and precision. Therefore, this study employed the PopPK model to simulate the exposure levels in the plasma and epithelial lining fluid (ELF) of Chinese CABP patients under different dosing regimens and conducted PK/PD analysis.
Foreign pharmacodynamic data indicate that lefamulin has MIC90 values of 0.12 mg/L for both S. pneumoniae and S. aureus (Paukner et al., 2019). The corresponding MIC90 values for Chinese clinically isolated strains are 0.125 and 0.06 mg/L, respectively, similar to those observed in foreign studies (Wu et al., 2020). Foreign PK/PD analysis demonstrates that, under the regimen of lefamulin 150 mg q12 h infused over 1 h, the probability of achieving the pharmacodynamic target of a 1-log10 CFU/mL reduction for S. pneumoniae and S. aureus is above 99% at the MIC90 of 0.12 mg/L in both plasma and epithelial lining fluid (ELF) (Bhavnani et al., 2019; Bhavnani and Rubino, 1976).
Our results indicate that extending the infusion time has no significant impact on the exposure levels and PTA for lefamulin in both plasma and ELF. It is recommended to use an extended infusion method to mitigate adverse reactions at the infusion site. From clinical aspect, in the Phase III Lefamulin Evaluation Against Pneumonia (LEAP 1) Trial, lefamulin (150 mg q12 h IV followed by 600 mg q12 h orally) was noninferior to moxifloxacin (400 mg qd IV followed by 400 mg qd orally) for early clinical response (87.3% vs. 90.2%, respectively) and investigator assessment of clinical response (mITT, 81.7% vs. 84.2%, respectively; CE, 86.9% vs. 89.4%, respectively). In microbiological ITT population, lefamulin produced 88.2% and 100% early clinical response agaisnt S. pneumoniae and S. aureus. In the Chinese bridging trial, the investigator assessment of clinical response was 76.8% in the lefamulin group and 71.4% in the moxifloxacin group. The above clinical efficacy results demonstrated the effectiveness of the recommended regimen based on PK/PD analysis. There was a slight decrease of drug exposure in plasma and ELF in the fed state, which was in accordance with the slightly reduced bioavailability in subjects who consumed high-fat meals (Wicha et al., 2019b). It was reported that the absolute oral bioavailability is 25.8% in the fasted state and 21.0% in the fed state (Wicha et al., 2017). However, regardless of fasted or fed conditions, the PTA for oral administration of lefamulin 600 mg q12 h is comparable to the intravenous infusion of 150 mg q12 h, supporting the sequential intravenous and oral treatment of CABP patients caused by S. pneumoniae and S. aureus (Xenleta, 2019).
This study conducted PK/PD analysis based on the exposure levels of plasma-free drug and ELF drug at steady state under different protein binding rates. At the MIC90 values for S. pneumoniae and S. aureus, the probability of achieving the pharmacodynamic target of a 1-log10 CFU/mL reduction in plasma remains above 98%. High protein binding had no significant impact on ELF drug exposure levels and the probability of reaching the target, with the highest MIC covered by PTA at 90% being essentially the same for S. pneumoniae and S. aureus using original and higher protein binding. The above results indicate that PK/PD assessment should be conducted according to drug exposure at the infection site. More attention should be given to the drug concentrations at the site of infection, considering the influence of protein binding.
This study provides data support for the selection of lefamulin 150 mg q12 h with extended infusion from 1 h to 2 h and oral administration of 600 mg q12 h with or without food for the treatment of Chinese CABP. It is anticipated that the aforementioned dosing regimens will exhibit good microbiological efficacy against CABP caused by S. pneumoniae and S. aureus.
Several limitations of this study should be mentioned here. In the PK prediction for intravenous administration, MAPE and RMSE are slightly higher. Further model refinement should be done to improve the predictive accuracy. Additionally, future studies incorporating real-world data are necessary to further validate these conclusions.
The data that support the findings of this study are available from the corresponding author upon reasonable request.
Ethical approval was not required for the study involving humans in accordance with the local legislation and institutional requirements. Written informed consent to participate in this study was not required from the participants or the participants’ legal guardians/next of kin in accordance with the national legislation and the institutional requirements.
XB: Data curation, Formal analysis, Funding acquisition, Writing–original draft, Writing–review and editing. NL: Formal analysis, investigation, Writing–original draft. YL: Investigation, Methodology, Validation, Writing–original draft. XZ: Funding acquisition, Validation, Writing–review and editing. JY: Project administration, Visualization, Writing–review and editing. YH: Project administration, Visualization, Writing–review and editing. HY: Validation, Writing–review and editing. QW: Validation, Writing–review and editing. XW: Data Curation, Writing–review and editing. JiW: Investigation, Writing–review and editing. GC: Investigation, Writing–review and editing. JuW: Conceptualization, Project administration, Supervision, Writing–review and editing. YW: Data Curation, Writing–review and editing. JZ: Project administration, Conceptualization, Supervision, Writing–review and editing.
The author(s) declare that financial support was received for the research, authorship, and/or publication of this article. The study was supported by an Investigator-Initiated Trial (Project number: 2023QD029) from Huashan Hospital, Fudan University funded by Sumitomo Pharmaceuticals (Suzhou) Co., Ltd., Shanghai “Science and Technology Innovation Action Plan” Rising Star Program (Sailing Program, Project number: 23YF1404000), Shanghai Municipal Hospital Development Center (Project number: SHDC2022CRS004B).
Author JW was employed by Sumitomo Pharmaceuticals (Suzhou) Co., Ltd.
The remaining authors declare that the research was conducted in the absence of any commercial or financial relationships that could be construed as a potential conflict of interest.
The authors declare that this study was funded by Sumitomo Pharmaceuticals (Suzhou) Co., Ltd. The funder’s involvement in the study included providing the dataset for modeling, data curation and manuscript revision.
All claims expressed in this article are solely those of the authors and do not necessarily represent those of their affiliated organizations, or those of the publisher, the editors and the reviewers. Any product that may be evaluated in this article, or claim that may be made by its manufacturer, is not guaranteed or endorsed by the publisher.
The Supplementary Material for this article can be found online at: https://www.frontiersin.org/articles/10.3389/fphar.2024.1456741/full#supplementary-material
Bhavnani, S. M., Zhang, L., Hammel, J. P., Rubino, C. M., Bader, J. C., Sader, H. S., et al. (2019). Pharmacokinetic/pharmacodynamic target attainment analyses to support intravenous and oral lefamulin dose selection for the treatment of patients with community-acquired bacterial pneumonia. J. Antimicrob. Chemother. 74, iii35–iii41. doi:10.1093/jac/dkz089
Bhavnani, S. M. Z. L., and Rubino, C. M. (1976). “Pharmacokinetic-pharmacodynamic (PK-PD) target attainment analyses to support oral lefamulin dose selection in the treatment of patients with community-acquired bacterial pneumonia (CABP),” in ID week 2016.
Chahine, E. B., and Sucher, A. J. (2020). Lefamulin: the first systemic pleuromutilin antibiotic. Ann. Pharmacother. 54, 1203–1214. doi:10.1177/1060028020932521
Covvey, J. R., and Guarascio, A. J. (2022). Clinical use of lefamulin: a first-in-class semisynthetic pleuromutilin antibiotic. J. Intern Med. 291, 51–63. doi:10.1111/joim.13378
Eraikhuemen, N., Julien, D., Kelly, A., Lindsay, T., and Lazaridis, D. (2021). Treatment of community-acquired pneumonia: a focus on lefamulin. Infect. Dis. Ther. 10, 149–163. doi:10.1007/s40121-020-00378-3
Hu, Y., Wei, Q., Bian, X., Yang, X., Yu, J., Wang, J., et al. (2023). Pharmacokinetic, pharmacokinetic/pharmacodynamic, and safety investigations of lefamulin in healthy Chinese subjects. Antibiot. (Basel) 12, 1391. doi:10.3390/antibiotics12091391
Levison, M. E., and Levison, J. H. (2009). Pharmacokinetics and pharmacodynamics of antibacterial agents. Infect. Dis. Clin. North Am. 23, 791–815. vii. doi:10.1016/j.idc.2009.06.008
Nabriva Therapeutics (2019). Xenleta (lefamulin) [NDA/BLA multi-disciplinary review and evaluation]. U.S. Food Drug Adm. Website. Available at: https://www.accessdata.fda.gov/drugsatfda_docs/nda/2019/211672Orig1s000,%20211673Orig1s000MultidisciplineR.pdf (Accessed August 12, 2021).
Paukner, S., Gelone, S. P., Arends, S. J. R., Flamm, R. K., and Sader, H. S. (2019). Antibacterial activity of lefamulin against pathogens most commonly causing community-acquired bacterial pneumonia: SENTRY antimicrobial surveillance Program (2015-2016). Antimicrob. Agents Chemother. 63. doi:10.1128/AAC.02161-18
Rodvold, K. A. (2019). Introduction: lefamulin and pharmacokinetic/pharmacodynamic rationale to support the dose selection of lefamulin. J. Antimicrob. Chemother. 74, iii2–iii4. doi:10.1093/jac/dkz084
Veve, M. P., and Wagner, J. L. (2018). Lefamulin: review of a promising novel pleuromutilin antibiotic. Pharmacotherapy 38, 935–946. doi:10.1002/phar.2166
Wicha, W. L. C., Seltzer, E., Prince, W. T., and Gelone, S. (2017). “Pharmacokinetics and safety of an oral, immediate-release (IR) tablet formulation of lefamulin in fed and fasted healthy subjects,” in Poster presented at 27th European congress of clinical microbiology and infectious diseases. Vienna.
Wicha, W. W., Prince, W. T., Lell, C., Heilmayer, W., and Gelone, S. P. (2019b). Pharmacokinetics and tolerability of lefamulin following intravenous and oral dosing. J. Antimicrob. Chemother. 74, iii19–iii26. doi:10.1093/jac/dkz087
Wicha, W. W., Strickmann, D. B., and Paukner, S. (2019a). Pharmacokinetics/pharmacodynamics of lefamulin in a neutropenic murine pneumonia model with Staphylococcus aureus and Streptococcus pneumoniae. J. Antimicrob. Chemother. 74, iii11–iii18. doi:10.1093/jac/dkz086
Wu, S., Zheng, Y., Guo, Y., Yin, D., Zhu, D., and Hu, F. (2020). In vitro activity of lefamulin against the common respiratory pathogens isolated from mainland China during 2017-2019. Front. Microbiol. 11, 578824. doi:10.3389/fmicb.2020.578824
Zhanel, G. G., Deng, C., Zelenitsky, S., Lawrence, C. K., Adam, H. J., Golden, A., et al. (2021). Lefamulin: a novel oral and intravenous pleuromutilin for the treatment of community-acquired bacterial pneumonia. Drugs 81, 233–256. doi:10.1007/s40265-020-01443-4
Keywords: lefamulin, Chinese population, population pharmacokinetic, pharmacokinetic/pharmacodanamic, community-acquired bacterial pneumonia
Citation: Bian X, Li N, Li Y, Zhu X, Yu J, Hu Y, Yang H, Wei Q, Wu X, Wang J, Cao G, Wu J, Wang Y and Zhang J (2024) Lefamulin dosing optimization using population pharmacokinetic and pharmacokinetic/pharmacodynamic assessment in Chinese patients with community-acquired bacterial pneumonia. Front. Pharmacol. 15:1456741. doi: 10.3389/fphar.2024.1456741
Received: 29 June 2024; Accepted: 26 September 2024;
Published: 25 October 2024.
Edited by:
Huanzhong Ding, South China Agricultural University, ChinaReviewed by:
Matthias Hoch, Novartis Institutes for BioMedical Research, SwitzerlandCopyright © 2024 Bian, Li, Li, Zhu, Yu, Hu, Yang, Wei, Wu, Wang, Cao, Wu, Wang and Zhang. This is an open-access article distributed under the terms of the Creative Commons Attribution License (CC BY). The use, distribution or reproduction in other forums is permitted, provided the original author(s) and the copyright owner(s) are credited and that the original publication in this journal is cited, in accordance with accepted academic practice. No use, distribution or reproduction is permitted which does not comply with these terms.
*Correspondence: Jing Zhang, emhhbmdqNjFAZnVkYW4uZWR1LmNu
†These authors have contributed equally to this work
Disclaimer: All claims expressed in this article are solely those of the authors and do not necessarily represent those of their affiliated organizations, or those of the publisher, the editors and the reviewers. Any product that may be evaluated in this article or claim that may be made by its manufacturer is not guaranteed or endorsed by the publisher.
Research integrity at Frontiers
Learn more about the work of our research integrity team to safeguard the quality of each article we publish.