- 1College of Pharmacy, Inner Mongolia Medical University, Hohhot, Inner Mongolia, China
- 2Department of Pharmacy, Baotou Medical College, Baotou, Inner Mongolia, China
- 3Central laboratory, Inner Mongolia Autonomous Region Hospital of Traditional Chinese Medicine, Hohhot, Inner Mongolia, China
- 4Department of Mongolia Medicine Study, Institute of Traditional Medicine and Technology of Mongolia, Ulaanbaatar, Mongolia
- 5Liaoning Provincial Universities Key Laboratory of Environmental Science and Technology, School of Environment and Chemical Engineering, Dalian Jiaotong University, Dalian, China
The Lamiaceae genus Dracocephalum, with over 30 species, is believed to have considerable medicinal properties and is widely used in Eurasian ethnomedicine. Numerous studies have researched on the geographical distribution, metabolite identification, and bioactivity of Dracocephalum species, especially amidst debates concerning the taxonomy of its closely related genera Hyssopus and Lallemantia. These discussions present an opportunity for pharmacophylogenetic studies of these medicinal plants. In this review, we collated extensive literature and data to present a multifaceted view of the geographical distribution, phylogenetics, phytometabolites and chemodiversity, ethnopharmacological uses, and pharmacological activities of Dracocephalum, Hyssopus, and Lallemantia. We found that these genera were concentrated in Europe, with species adapted to various climatic zones. These genera shared close phylogenetic relationships, with Dracocephalum and Hyssopus displaying intertwined patterns in the phylogenetic tree. Our review assessed more than 900 metabolites from these three genera, with terpenoids and flavonoids being the most abundant. Researchers have recently identified novel metabolites within Dracocephalum, expanding our understanding of its chemical constituents. Ethnopharmacologically, these genera have been traditionally used for treating respiratory, liver and gall bladder diseases. Extracts and metabolites from these genera exhibit a range of pharmacological activities such as hepatoprotective, anti-inflammation, antimicrobial action, anti-hyperlipidaemia, and anti-tumour properties. By integrating phylogenetic analyses with network pharmacology, we explored the intrinsic links between metabolite profiles, traditional efficacy, and modern pharmacology of Dracocephalum and its related genera. This study contributes to the discovery of potential medicinal value from closely related species of Dracocephalum and aids in the development and sustainable use of medicinal plant resources.
1 Introduction
The Lamiaceae family is the sixth most diverse in terms of species and the tenth most diverse in terms of genera among angiosperms (Christenhusz and Byng, 2016). With its cosmopolitan distribution, this botanical group is used extensively in various fields. For example, lavender [Lamiaceae; Lavandula angustifolia Mill.], which is known for its ornamental cultivation and aromatic qualities, has attracted considerable scholarly attention (Gu et al., 2021). Another member of this family, fresh mint [Lamiaceae; Mentha piperita L.], is highly valued for its culinary use (Hsu et al., 2010). Historical records from various regions and ethnic groups have confirmed the traditional medicinal uses of Lamiaceae species. In Traditional Chinese Medicine, the dried roots and rhizomes of Salvia miltiorrhiza Bunge [Lamiaceae; Salviae miltiorrhizae radix et rhizoma] is lauded for its therapeutic properties, which encompass the promotion of blood circulation, the alleviation of menstrual discomfort, heat-clearing, and the reduction of swelling (Deng et al., 2016). In the southern regions of India, Leucas ciliata Benth [Lamiaceae] have been documented for their efficacy in wound healing and as a snakebites remedy (Akshatha et al., 2021). Additionally, the native American plant Callicarpa americana L [Lamiaceae] has been traditionally used by several indigenous tribes in the southeastern United States for its febrifuge, stomachic, and anti-dysenteric properties (Dettweiler et al., 2020).
Globally, the importance of Lamiaceae plants is undeniably prominent. The genera Dracocephalum, Hyssopus, and Lallemantia belong to the Lamiaceae family. In recent years, the phylogenetic relationships among these three genera have attracted the attention of researchers and their taxonomy has been reassessed from multiple perspectives, including bioinformatics, geography, and plant morphology. Among them, Dracocephalum has the greatest number of species, characterized by flowers that are typically blue-purple with occasional whites, and bracts, that are often obovate and frequently feature acute teeth or spines, rarely being entire. The floral structure is described as tubular or campanulate-tubular, either straight or slightly curved, and adorned with 15 veins and five teeth (Figure 1). As close relatives of the Dracocephalum genus, the flowers of Hyssopus and Lallemantia genera also typically have a tubular calyx, 15 veins, five teeth, and a bilabiate corolla, with four stamens and a stigma that is bifid at the apex. However, significant differences between the stems and leaves were observed. Plants of these three genera contain several valuable metabolites. Notably, Dracocephalum is known for its rich content of essential oils, flavonoids, glycosides, triterpenoids, organic acids, and esters (Lv et al., 2022). The ‘A Quick-Consultative Dictionary of World Medicinal Plants’ documents the nomenclature, medicinal parts, habitats, and therapeutic properties of the medicinal plants within the Dracocephalum genus. A substantial proportion of these records are from diverse provinces across China, where they have garnered attention for their roles in traditional Chinese medicine, particularly for their heat-clearing, blood-cooling, and cough-relieving effects (Jiang, 2015; Yao et al., 2020; Yan et al., 2020).
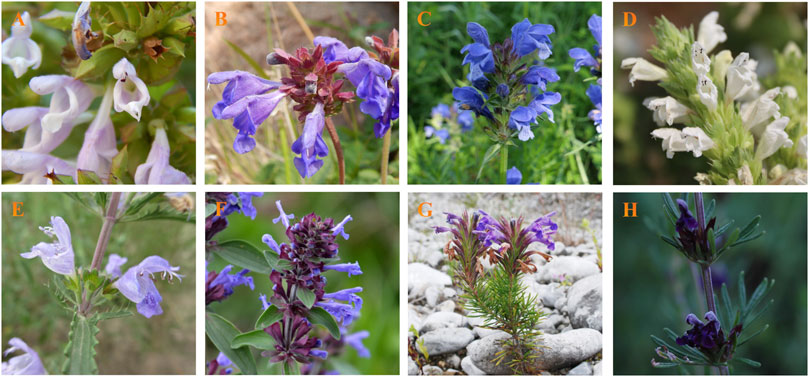
Figure 1. Species within the Dracocephalum genus, recognized for their medicinal properties, display certain distinctive features in the field of plant morphology. D. rigidulum (A), D. rupestre (B), D. argunense (C), D. heterophyllum (D), D. moldavica (E), (D) nutans (F), D. forrestii (G), D. taliense (H). The picture comes from shooting.
As living standards progressively increase, societal emphasis on health and wellbeing increases. This trend is mirrored by an increasing interest in herbal resources. However, the growing demand for herbal resources could result in the overexploitation of wild resources and a shortage of medicinal plants that are on the verge of extinction. Thus, sustainable use of medicinal plants has become a pivotal research domain in contemporary society. This field demands a robust foundation of precise knowledge and strategic application of theoretical frameworks, as exemplified by the discipline of pharmacophylogeny. The concept of pharmacophylogeny was proposed by Xiao Peigen in the 1980s and is an emerging discipline that studies the correlation between the molecular phylogeny of medicinal plants, chemical metabolites, pharmacological activities, and traditional applications. Pharmacophylogeny is advantageous owing to its interdisciplinarity and interpenetration feature its research objects, which involve multidisciplinary fields (Chen et al., 2005; Hao and Xiao, 2020). Establishing this subject as an essential guide for the conservation and development of medicinal plant resources is crucial, and justified through long-term research (Gong et al., 2022; Hao et al., 2022). Pharmacophylogeny proposes that phylogenetically closer taxonomic groups, such as families, tribes, or genera, are more likely to possess similar chemical profiles, which contribute to more analogous bioactivities. Pharmacophylogeny principles have been applied to various aspects of imported drug resource substitution, the search for new drugs, and production practices to expand the development and utilization of medicinal resources (Li, 2021; Hao et al., 2022).
In recent years, many studies have been conducted on the genus Dracocephalum, covering various aspects such as the geographical distribution of its species, their phylogenetic relationships with other Lamiaceae genera, its metabolites, and its pharmacological activities. Our review meticulously compiled extensive information on Dracocephalum species and their closely related genera to clarify the current status of Dracocephalum as a medicinal plant through the lens of pharmacophylogenetic principles. This compendium is intended not only to deepen our understanding of Dracocephalum and its relatives but also to encourage the sustainable management and application of these precious botanical resources.
For this review, we have primarily referenced ‘A Quick-Consultative Dictionary of World Medicinal Plants’ and conducted independent searches for the species names across PubMed (https://pubmed.ncbi.nlm.nih.gov/), Google Scholar (https://scholar.google.com), and the China National Knowledge Infrastructure (https://kns.cnki.net/) database, focusing on the most current and authoritative literature. All plant names mentioned in this article have been confirmed on the WFO Plant List (https://wfoplantlist.org/), ensuring that they are accepted species names. The geographical coordinates were sourced from the Global Biodiversity Information Facility (https://www.gbif.org/zh/). Species distribution data were integrated from the WFO, WORLD PLANTS (https://www.worldplants.de/), and iPlant (http://www.iplant.cn/) databases, facilitating the delineation of climatic conditions. Phylogenetic tree sequences are derived from the National Center for Biotechnology Information (NCBI) (https://www.ncbi.nlm.nih.gov/) and the scholarly work of Chen G. W. et al. (2022).
2 Geographical distribution and phylogenetics
2.1 Geographical distribution
The genus Dracocephalum, comprising over 60 species, is distributed across the Eurasian and North American tectonic plates. The speciation of Dracocephalum exhibits regional differentiation, featuring four primary distribution areas on the Eurasian plate and one main area on the Indian and American plates. The genus Dracocephalum exhibits an endemic distribution, with robust its adaptability observed predominantly within the climatic zones of the Northern Hemisphere, specifically in temperate continental climate areas.
In the Asian continent, the genus Dracocephalum shows a rich diversity of species that exhibit distinct climatic differentiation and a geographical distribution gradient from east to west. Dracocephalum’s distribution transitions from temperate zones to more demanding alpine mountainous regions, with this genus predominantly thriving in temperate continental climates. These climates are marked by significant seasonal variations, pronounced temperature fluctuations, and moderate, seasonally concentrated rainfall, which, along with the region’s topography, shape the ecological spread of Dracocephalum across alpine, temperate monsoon, and Mediterranean climates.
The aridification of the Asian interior and the uplift of the Qinghai-Tibet Plateau (QTP) are substantial geological and climatic events that have influenced the speciation and distribution of Dracocephalum. Interior aridification likely drove the rapid radiation of the genus, whereas the uplift of the QTP initiated its dispersal and diversification in the plateau and surrounding areas (Chen Y. P. et al., 2022). The flora of Central and West Asia are closely related to the QTP, with some species hypothesized to have originated from the plateau and later migrated to other regions (Wen et al., 2014; Zhang et al., 2017). The alpine climate of the QTP, influenced by its distance from the ocean, high terrain, and low temperatures, has fostered a conducive environment for the growth and diversification of this genus. Interestingly, despite the southern part of the American Plate fallings within the temperate continental climate zone, there are no records of Dracocephalum in this region, as the genus’s adaptive radiation of this genus may be unable to cross the tropical climate zones of the American Plate.
The spatial distribution pattern (Figures 2A, C) shows that Dracocephalum has a broader distribution than the Lallemantia and Hyssopus; however, the latter three genera share overlapping ranges within Europe. Dracocephalum is found across Asia and Europe, whereas Lallemantia and Hyssopus are predominantly present in Southern European countries such as Greece and Italy, in addition to other regions across Europe. The distribution of Dracocephalum also extends to the Americas, particularlynorthern Mexico. From the perspective of suitable ecological zones, the genera Dracocephalum, Lallemantia, and Hyssopus are well adapted across much of Europe. Dracocephalum, in particular, is amenable to cultivation and thrives along the Mediterranean coast, Central Asia, West Asia, and the western regions of North America. However, most areas south of the equator were not conducive to the cultivation and growth of these three genera (Figures 2B, D).
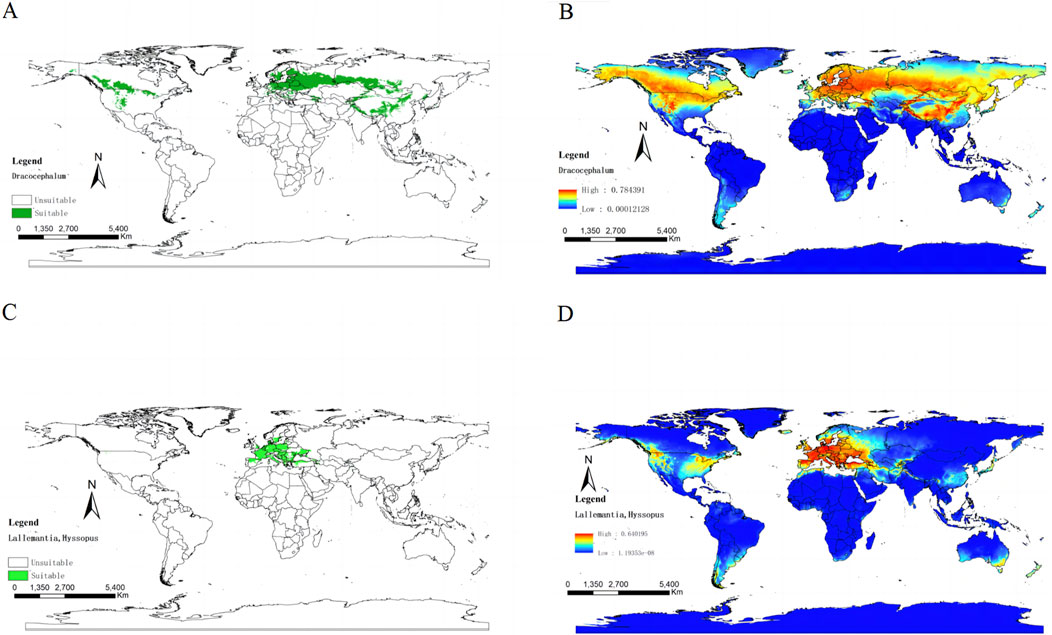
Figure 2. Ecologically suitable regions of genus Dracocephalum (Green distribution is suitable for the growth area of Dracocephalum). (A). Suitability distribution of Dracocephalum. From blue to red, the suitability of Dracocephalum gradually increases, and yellow is the transition area (B). Ecologically suitable regions of genus Lallemantia and Hyssopus (Green distribution is suitable for the growth area of Lallemantia and Hyssopus). (C). Suitability distribution of Lallemantia and Hyssopus. From blue to red, the suitability of Lallemantia and Hyssopus gradually increases, and yellow is the transition area (D).
2.2 Phylogenetics
The genus Dracocephalum encompasses a diverse array of over 60 species, approximately 30 of which are recognized for their medicinal properties. The majority of these medicinal species are meticulously documented in the authoritative publication ‘A Quick-Consultative Dictionary of World Medicinal Plants’, as well as in the traditional medical literature of numerous ethnic groups and within a variety of scholarly texts. Currently, phylogenetic analysis is widely applied in pharmacophylogeny, especially in the exploration and organization of traditional Chinese medicine (Hao et al., 2017; Gong et al., 2022). The medicinal plants of Dracocephalum are underpinned by a rich and extensive genomic sequence foundation, which renders them invaluable subjects for extensive and scholarly research.
In 2022, Chen et al. conducted an exhaustive study employing a range of genetic sequences, including chloroplast sequences (rpl32-trnL, trnL-trnF, ycf1, ycf1-rps15), nuclear genes (ITS and ETS), and low-copy nuclear gene sequences (AT3G09060, AT1G09680) (Chen Y. P. et al., 2022). Despite the topological structures of the resultant phylogenetic trees demonstrating variability, these findings suggest that the taxonomic classification of Dracocephalum species is a matter of ongoing debate. This is particularly true when considering the phylogenetic interrelationships among Hyssopus, Lallemantia, and Dracocephalum, which present both morphological and taxonomic challenges.
The ITS sequence, which is a standard in pharmacophylogeny analysis, was pivotal in our study (Hao et al., 2017; Wang et al., 2019). We used the Neighbor-Joining (NJ) model to construct phylogenetic trees (Supplementary Figure S1) from these sequences (Supplementary Table S1). These result indicates that within the medicinal flora of Dracocephalum and its closest botanical allies, Hyssopus is consistently intermingled with the Dracocephalum lineage, whereas Lallemantia may be more appropriately classified taxonomically as a distinct entity. However, it is important to note that the phylogenetic branches derived from this model had relatively low bootstrap values. In a stark comparison, Chen G. W. et al. (2022) leveraged the Pentapeptide Repeat (PPR) motif and applied the Bayesian Inference (BI) algorithm to uncover a tree with significantly robust branch support. By extracting the topological structure of the medicinal plant branches from their analysis, we delineated a novel phylogenetic tree for the genus Dracocephalum and its related medicinal species (Figure 3). Within the Dracocephalum genus, species such as Dracocephalum argunense Fisch. ex Rchb. and Dracocephalum bipinnatum Rupr., demonstrated adaptability to two diverse climatic conditions. It is worth noting that, Dracocephalum moldavica L. and Dracocephalum nutans L. have the ability to inhabit three separate climatic zones. Furthermore, Dracocephalum ruyschiana L. had an even broader distribution across the five climatic regions. In contrast to medicinal plants in the genera Hyssopus and Lallemantia, which share a close phylogenetic affinity with Dracocephalum, only Hyssopus officinalis L. and Lallemantia royleana (Benth.) Benth. are documented to have distributions spanning multiple climatic zones. This adaptability highlights the ecological plasticity of these species and underscores their potential for medicinal use in various environmental contexts.
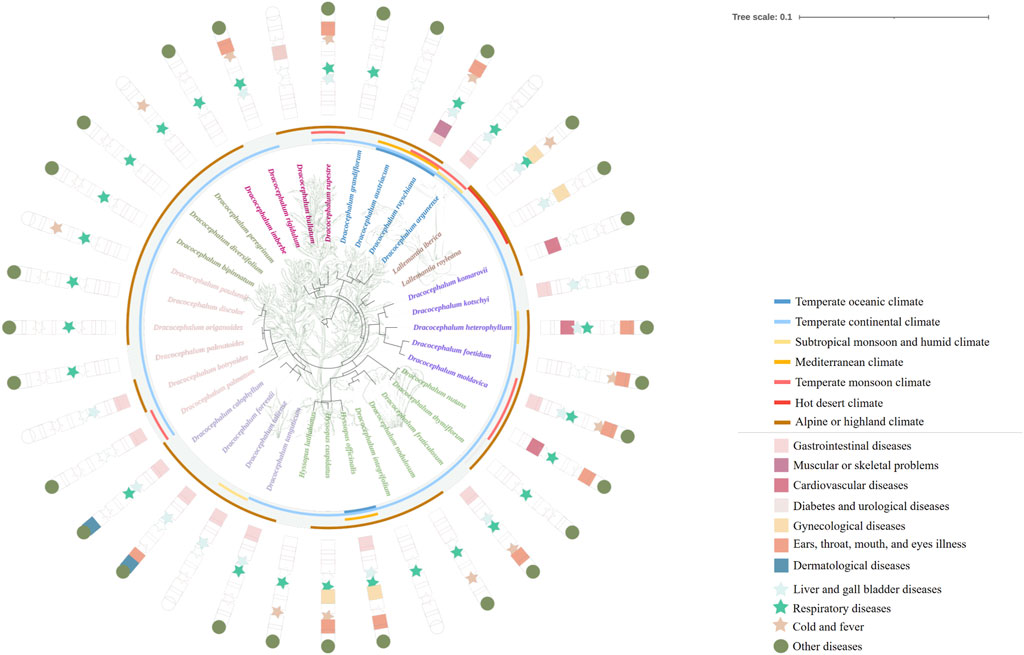
Figure 3. This refined phylogenetic tree expands upon the original framework constructed by Chen et al., with selective pruning to emphasize species of Dracocephalum and its closely related genera, recognized for their medicinal properties and historical use in healing practices. The outermost ring of the visualisation encapsulates the climatic distribution characteristics of these plants, collated from the World Plants database [https://www.worldplants.de/world-plants-complete-list/complete-plant-list] and the iPlant database [http://www.iplant.cn/]. The annotations on the outer layer provide insights into the medicinal efficacy attributed to these species, offering a visual representation of their therapeutic potential. The traditional classification of efficacy is shown in Supplementary Table S2, and the climate information of each region is shown in Supplementary Table S5.
3 Phytometabolites and chemodiversity
Our survey systematically catalogued the chemical metabolites from a total of seventeen Dracocephalum species, three Hyssopus species, and two Lallemantia species, resulting in the identification of more than 900 metabolites (Supplementary Tables S3, S4). The predominant metabolites are terpenoids, flavonoids, phenylpropanoids, and phenolic acids. Fatty acids, alkaloids, and steroids, being less prevalent, were categorized under the ‘Others’ grouping (as depicted in Figure 4A). Our findings, which only provide a consolidated summary, consider the variability in extraction methods reported in the literature. Notably, within the genus Dracocephalum, D. moldavica exhibited the greatest metabolite diversity, while in Hyssopus, Hyssopus cuspidatus Boriss. had the most characterized metabolites. In the genus Lallemantia, the number of reported metabolites for L. royleana significantly surpassed that for Lallemantia iberica (M.Bieb.) Fisch. & C.A.Mey., with a focus on the aerial parts of the plants rather than on the seeds (Figure 4B). Most studies have focused on identifying chemical metabolites in naturally dried whole plants of Dracocephalum species, with D. moldavica, Dracocephalum heterophyllum Benth., and D. nutans being the most studied examples (Jiang, 2015). Some studies have specifically analyzed whole plants during the flowering stage, such as Dracocephalum kotschyi Boiss., Dracocephalum palmatum Stephan ex Willd., Dracocephalum austriacum L. and Dracocephalum botryoides Steven, which may correspond to the peak times of traditional medicinal activity (Olennikov et al., 2017; Kashchenko et al., 2022). Additionally, some investigations have concentrated on particular plant organs, such as leaves or roots (Poursalavati et al., 2021; Kashchenko et al., 2022). Concurrently, many novel metabolites are continually being discovered within this flora, highlighting the dynamic chemical landscape of this genus. This disparity may be attributed to variations in metabolic pathways, ecological preferences, or evolutionary factors that have shaped the chemical diversity of these plants. It is important to acknowledge that current research on the metabolites of Dracocephalum and its closely related genera are not exhaustive, indicating the need for further comprehensive studies. However, the extensive geographical distribution of Dracocephalum species, along with the significant altitudinal gradients they inhabit, make them an excellent model for studying the relationship between medicinal plant metabolites and environmental factors such as latitude, longitude, and altitude. This research is crucial for understanding the influence of environmental factors on the chemical profiles of medicinal plants and has implications for biodiversity conservation and the discovery of new pharmaceutical metabolites.
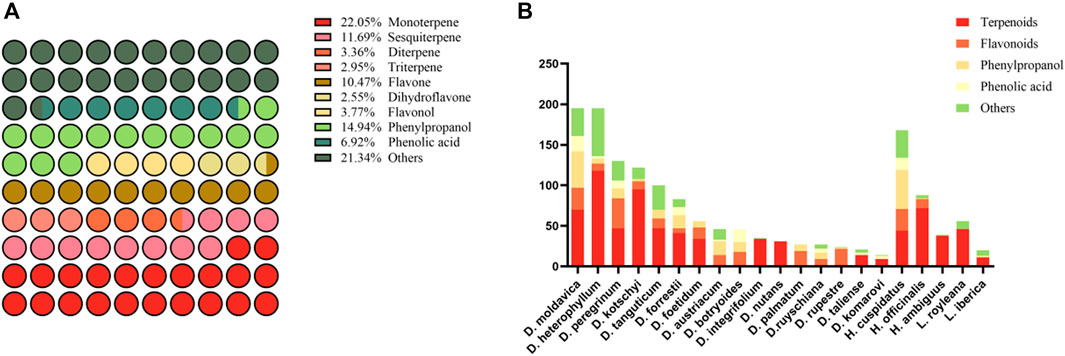
Figure 4. A proportional diagram of all metabolites collected from the medicinal plants of the Dracocephalum genus and its closely related genera (A), and information on each collected medicinal plant metabolite (B).
In this review, we focused on terpenoids and flavonoids, which constitute the two most diverse classes of metabolites in the aforementioned genera. Moreover, during our literature review, we found that researchers were often able to successfully isolate and characterize new metabolites from the genus Dracocephalum. Among these, some have already made initial contributions to the advancement of human health, whereas others are yet to be investigated because of their unknown activities.
3.1 Terpenoids
Terpenoids are ubiquitous throughout the plant kingdom and play integral roles in both primary and secondary metabolic processes. They are crucial for a myriad of biological functions, such as signal transduction, reproduction, communication, and adaptation of plants to environmental changes, including climate change, as well as their defense mechanisms (Tholl, 2015; Atriya et al., 2023). Terpenoid metabolites within plants can attract pollinating insects and deter or resist predators and other organisms that may harm the plant foliage. Plants use the color and/or aroma of these volatile metabolites to entice pollinators and animals, thereby facilitating seed dispersal (Boncan et al., 2020; Tetali, 2019). Certain terpenoids not only regulate plant growth and development and respond to environmental pressures but also play a central role in the physiological activities of plants (Chen et al., 2020). Additionally, the majority of plant terpenoids possess antimicrobial and insecticidal properties (Yamaguchi, 2022).
In this study, we compiled a collection of 283 terpenoids from the Dracocephalum genus, including 216 monoterpenes, 112 sesquiterpenes, 24 diterpenes, and 29 triterpenes. This collection highlights the rich chemical diversity of this class of metabolites and their potential significance in biological and ecological contexts.
3.1.1 Monoterpenes and sesquiterpenes
Monoterpenes and sesquiterpenes are prevalent in the essential oils of plants and represent the most abundant and varied classes within the genera Dracocephalum, Hyssopus, and Lallemantia. In this literature review, with the exception of essential oils from Dracocephalum tanguticum Maxim. extracted via supercritical carbon dioxide, which had a combined GC/MS-determined proportion of monoterpenes, oxygenated monoterpenes, sesquiterpenes, and oxygenated sesquiterpenes of less than 70% (Zhang et al., 2007), species such as D. heterophyllum, D. moldavica, and D. nutans all surpassed this percentage threshold (Zhang et al., 2008; Fattahi et al., 2021; Ashrafi et al., 2017). GC-MS analysis of Dracocephalum integrifolium Bunge from various regions revealed that these four metabolite classes comprised an average of 95.86% of the total essential oil content (Zhou et al., 2019). Some of these metabolites exhibited toxicity, suggesting their potential for weed management and resistance to pests and pathogens, thereby warranting consideration for the development of novel natural pesticides.
Specific metabolites, such as citral and nerolidol, which are the most abundant in the essential oil of D. kotschyi, may also account for its notable in vitro antibacterial activity against gram-negative bacteria (Chahardoli et al., 2019). Among Hyssopus, Lallemantia, and Dracocephalum, there was certain overlap in the presence of monoterpene and sesquiterpene metabolites. Furthermore, some metabolites have been identified that are distinguished solely by their optical isomers within these genera. However, the diversity of these shared metabolites is generally less pronounced in Hyssopus and Lallemantia than in Dracocephalum. This distinction underscores the rich chemical landscape of Dracocephalum, which may provide a more extensive reservoir of bioactive metabolites for future research and medicinal exploitation.
3.1.2 Diterpenes and triterpenes
The variety of diterpenes and triterpenes detected in Dracocephalum plants was far less than that of the monoterpenes and sesquiterpenes. However, it is noteworthy that komaroviquinone, isolated by Uchiyama et al. (2003) from Dracocephalum komarovii Lipsky in the early 21st century, has gained particular prominence. In the context of Chagas disease treatment, the natural product komaroviquinone and its derivatives have emerged as promising candidates, offering a novel therapeutic strategy. This study highlights the efficacy of komaroviquinone, which has shown significant anti-trypanosomal activity against Trypanosoma cruzi, the causative agent of Chagas. The potency of this metabolite is especially remarkable, as it demonstrates higher antiprotozoal activity than the standard drug benznidazole, without concurrent toxicity to host cells (Suto et al., 2015). Dracocephalum forrestii W.W.Sm. is currently the plant with the most abundant triterpene metabolites, whereas betulinic acid and ursolic acid have been detected in three or more Dracocephalum species. During plant desiccation, there may be a general accumulation phenomenon of these antioxidant-rich triterpenoid metabolites (Abbasi et al., 2019). This antioxidant effect originates from the plants themselves, indicating that throughout evolution, plants have developed the capability to synthesize these compounds to protect themselves from oxidative stress.
3.2 Flavonoids
In Dracocephalum, 160 flavonoid metabolites were collected, including 94 flavone, 44 flavonol and 22 dihydroflavonoid metabolites. The pioneering documentation of flavonoids within the Dracocephalum, as found in current literature, originates from the work of Shamyrina et al., who characterized the presence of apigenin and its glucoside derivative, apigenin 7-β-D-glucopyranoside from D. nutans (Shamyrina et al., 1975). Subsequently, metabolites such as cosmosiin, pedalitin, and pedaliin were successively isolated from D. tanguticum by Zhang et al. (1994). Flavonoids were most widely distributed in vanilla, including isorhamnetin, kaempferol, tilianin, xanthomicrol, 8-hydroxy-salvigenin, chrysoeriol, etc (Sharafi et al., 2014; Zhang et al., 2019). The most widely distributed metabolite are luteolin and its derivatives, which have been identified in Dracocephalum (Sharafi et al., 2014). Cosmosiin has also been identified in D. moldavica, D. tanguticum, and D. palmatum (Zhang et al., 2019). Most flavonoids are distributed throughout the plant, but some tend to accumulate in the tissue of specific species. For example, Apigenin-7-O-glucoside is predominantly accumulated in the leaves of Dracocephalum rupestre Hance (Ferreira et al., 2016); (2S)-Isosakuranetin 7-O-β-D-(6ʺ-o-malonyl) glucopyranoside and (2S) -Poncirin can only be detected in the aboveground part of Dracocephalum fruticulosum Stephan ex Willd. (Sabrin et al., 2021); chrysoeriol O-β-D-glucopyranoside was specifically distributed in the aboveground part of D. nutans (Conrad et al., 2009); acacetin-7-O-(3-O-malonyl)-β-D-glucopyranoside and acacetin-7-O-(2-O-malonyl)-β-D-glucuronopyranoside are distributed in the aboveground portion of Dracocephalum foetidum Bunge (Selenge et al., 2014). In addition, several flavanone glucosides deserve attention. For example, (2S) -isosakuranetin 7-O-β-D-(6ʺ-o-malonyl) glucopyranoside, (2S) -poncirin, and isosakuranin, present in D. fruticulosum, naringenin-7-O-β-D-glucuronopyranoside, present in D. palmatum (Sabrin et al., 2021; Olennikov et al., 2013). Naringenin-7-O-β-D-glucuronopyranoside is one of the main substances controlling the bitter taste of plants, which may be a factor affecting the flavor of medicinal materials (Matoušek et al., 2012).
3.3 Chemical metabolites of the first classification
Over the past 20 years, investigators of the Dracocephalum genus have made significant strides in successfully isolating and characterizing a plethora of new metabolites. In addition to the aforementioned komaroviquinone, Saeidnia et al. reported the isolation of two novel monoterpene glycosides from D. kotschyi: limonen-10-ol 10-O-β-D-glucopyranoside and limonen-10-ol 10-O-β-D-glucopyranosyl-(1→2)-β-D-glucopyranoside. Regrettably, these metabolites did not exhibit the potent trypanocidal activity observed for limonene-10-aL (Saeidnia et al., 2004). In 2008, Dai et al. extracted 1′-methyl-2′-hydroxyethyl ferulate (a ferulic acid ester) from Dracocephalum peregrinum L., which demonstrated a modest inhibitory effect on nitric oxide (NO) production. Cellular viability assays using MTT [3-(4,5-dimethylthiazol-2-yl)-2,5-diphenyltetrazolium bromide] indicated that this metabolite did not significantly affect RAW264.7, at efficacious concentrations (Dai et al., 2008).
In 2009, Fu et al. identified three flavonoid glycosides (Peregrinumin A, B, and C) and one cyanogenic glycoside (Peregrinumcin A) from D. peregrinum. These metabolites displayed commendable anti-inflammatory activity in vitro, effectively suppressing the production of nitric oxide (NO) in RAW 264.7 and 293 cells activated by lipopolysaccharide (LPS). Notably, Peregrinumin A and Peregrinumcin A exhibit robust anti-inflammatory effects at a dose of 100 mg/mL (Fu et al., 2009). In the same year, Wang et al. isolated four novel spermidine glycosides, dracotanosides A-D, from D. tanguticum, yet their biological activities and functions were not elucidated (Wang et al., 2009). In 2009, Li et al. discovered four new metabolites from D. forrestii, among which only 3,4,5-trimethoxyphenylethanol β-D-glucopyranoside demonstrated modest anti-inflammatory effects (Li et al., 2009).
In 2011, Zeng et al. reported that among the four glycosides found in D. tanguticum, benzyl-6-[(2E)-2-butenoate]-β-D-glucopyranoside exhibited moderate inhibitory activity against NO with an IC50 value of 64.33 µM (Zeng et al., 2011). In 2017, Deng et al. identified two metabolites from Dracocephalum taliense Forrest ex W.W.Sm., one being the abietane diterpenoid 12-methoxy-18-hydroxy-sugiol and the other a highly oxygenated ursane triterpenoid named 2α,3α-dihydroxy-11α,12α-epoxy-urs-28,13β-olide. While these did not show significant anti-inflammatory activity, the latter metabolite displayed potential antitumor activity, exhibiting marked cytotoxic effects on the HepG2 (human liver cancer cell line) and NCI-H1975 (human lung adenocarcinoma cell line) with IC50 values of 6.58 ± 0.14 µM and 7.17 ± 0.26 µM, respectively (Deng et al., 2017). In 2019, Ma et al. discovered three new phenylacetamide glycosides, dratanguticumides A-C, from D. tanguticum. These metabolites showed moderate antihyperglycemic activity, as determined by the glucose consumption rate in 3T3-L1 adipocytes, with rates of 20.80% ± 1.47%, 21.48% ± 2.44%, and 21.57% ± 1.35%, respectively (Ma et al., 2020). In 2021, Zhang et al. identified eight lignans from D. moldavica whose biological activities remain to be elucidated (Zhang et al., 2021).
4 Ethnopharmacological uses
The genus Dracocephalum, along with its closely related genera Hyssopus and Lallemantia, presents a rich tapestry of ethnomedical uses across various plant parts such as the roots, stems, leaves, flowers, and seeds. Our comprehensive compilation verified 32 medicinal species within Dracocephalum, complemented by four within Hyssopus and two within Lallemantia, all taxonomically confirmed in the field of botanical science. The application of the whole herb is notably dominant in Dracocephalum and Hyssopus, representing a substantial 71.9% and 75.0% of the medicinal species from these genera, respectively. Conversely, the medicinal accounts for Lallemantia are solely attributed to its seeds (Table 2). To delineate the traditional medicinal attributes of these genera in more detail, we draw on the previous classification (Li et al., 2012a). The traditional medicinal properties have been categorized into 11 therapeutic applications, which encompass a spectrum of conditions, including gastrointestinal diseases; musculoskeletal issues; cardiovascular diseases; diabetes and urological disorders; gynaecological conditions; ailments of the ears, throat, mouth, and eyes; dermatological conditions; liver and gall bladder diseases; respiratory diseases; colds and fevers; and others (see Supplementary Table S2). We have consolidated this information and presented it visually in Figure 3, which clearly demonstrates that the traditional efficacy of these three genera is predominantly concentrated in addressing respiratory diseases, liver and gall bladder diseases.
4.1 Tibetan plateau and adjacent regions ethnomedicines
Some Dracocephalum species are distributed across the QTP and its surrounding regions, establishing themselves as an indispensable element of local and regional ethnopharmacopeia. These plants have become an integral part of traditional medicine for neighboring ethnic groups and countries, underscoring their importance in the ethnomedical landscape.
In Tibetan pharmacopeias dating back to the 17th century, a record exists of a Tibetan medicinal material known as “Priyangu,” characterized by its flowers that resemble fluttering blue flags and its whole plant possessing effects in alleviating liver heat and hemostasis. In contemporary Tibetan medicine, D. tanguticum isconsidered the mainstream variety of “Priyangu” (Tashi et al., 2022). However, some scholars from China’s ethnic minorities suggest that because of ambiguous botanical descriptions in ancient Tibetan medical literature, Dracocephalum calophyllum Hand.-Mazz. and Dracocephalum isabellae Forrest ex W.W.Sm. may also be used as “Priyangu” to alleviate liver heat (He et al., 2015). Unfortunately, literature regarding the chemical metabolites and pharmacological properties D. calophyllum and D. isabellae is lacking.
D. forrestii is a Tibetan medicinal plant that is indigenous to the mountainous regions of Yunnan Province, China. The aerial parts of D. forrestii are used as diuretic, astringent, and antipyretic agents (Weremczuk-Jeżyna et al., 2020). Given its use as a substitute for D. tanguticum, D. forrestii has emerged as a medicinal plant that warrants in-depth investigation of its pharmacological activities and clinical applications. D. taliense is a species endemic to Yunnan Province. Local communities utilize the entire plant of D. taliense for the treatment of hepatic disorders. The plant has demonstrated significant efficacy particularly in cases of hepatitis and jaundice, as well as for the regulation of gastrointestinal health (Deng et al., 2017).
D. heterophyllum is recognized as one of the traditional Tibetan medicinal plants within the Dracocephalum genus. In the traditional Tibetan medical system, D. heterophyllum is also known as “Ao-Ga” or “Ji-Mei-Qing-Bao,” and has been utilized by Tibetan physicians to treat various conditions, including jaundice, liver diseases, coughs, lymphangitis, oral ulcers, and dental disorders (Li et al., 2022). Additionally, the Uyghur people employ D. heterophyllum to treat certain cardiovascular and respiratory diseases (Jiang et al., 2018).
D. integrifolium is predominantly found in Central Asia and is referred to as “Marzan Juxi” in traditional Uyghur herbal medicine, where it is used for the treatment of coughs and asthma (Zhou et al., 2019). D. peregrinum is widely distributed across Russia, Mongolia, and Northern China. In the traditional medicine of the Kazakh people in Xinjiang, D. peregrinum is known as “Tekanbasjelanbas,” and the entire plant is commonly used for treating colds and liver diseases (Yan et al., 2020; Dai et al., 2008).
4.2 Ethnopharmacological heritage beyond the Tibetan plateau
Beyond the QTP and its surrounding regions, we provide a comprehensive overview of the utilization of Dracocephalum medicinal plants in other countries and regions.
For example, D. rupestre is widely distributed across various provinces in China, including Hebei, Shanxi, Qinghai, Inner Mongolia, and Liaoning. According to records in the “Chinese Herbal Medicine Dictionary,” the entire plant of D. rupestre is used to treat conditions such as externally contracted wind-heat, headache with chills fever, cough, jaundice, and hepatitis. Additionally, the tender stems and leaves of D. rupestre form a traditional Chinese medicinal food, known as “Maojian” tea (Wang et al., 2022).
D. austriacum, and D. botryoides are characteristic Dracocephalum species found in the Caucasus region. In Azerbaijan, these two species are considered medicinal. Local populations add the aerial parts of these plants into noodle soups to treat respiratory and gastrointestinal disorders. In local folk medicine, herbal decoctions of D. botryoides are used to treat liver diseases, gastritis, and ulcers, whereas those of D. austriacum possess anti-inflammatory properties and aid in wound healing (Kashchenko et al., 2022).
D. komarovii predominantly grows in the high-altitude regions of the Western Tian Shan Mountain range. In Uzbekistan, D. komarovii is known as “buzbosh,” and the local population utilizes the aerial parts of the plant to prepare a tea that is traditionally consumed to address various ailments, including inflammatory conditions and hypertension (Uchiyama et al., 2006).
D. kotschyi is a traditionally used medicinal plant in Iran, referred to as “Zarrin-giah” in Persian. In the traditional Iranian medical system, this plant is used for its antispasmodic, antihyperlipidemic, and analgesic properties. It is also used to treat symptoms such as headaches, fever, inflammation, bruising, rheumatism, gastric disorders, and liver diseases, and serves as an analgesic for complications related to kidney function. In Iran, it is boiled to alleviate rheumatic pain and to facilitate wound healing. Furthermore, it is recognized for its capacity to strengthen the immune system (Ghavam et al., 2021; Heydari et al., 2019).
From the Caucasus to Siberia and China, the whole herb or seedling of D. calophyllum has been reported to have effects on clearing the heat of the liver, stomach, and lungs, stopping bleeding, healing sores, eliminating dampness, relieving itching, and treating dizziness, visceral pus, prurigo rheumatism, hematochezia, hematuria, sore mouth incompatibility, edema, and ascites (Jiang, 2015).
D. moldavica is recognized as a Dracocephalum species with the most extensive folk medicinal applications, for which we collected data. In the northern Iranian Boulze Mountains, D. moldavica is commonly known by the name ‘Badarshoo.’ It is used as a food additive in yogurt or as a processed herb. It can effectively treat stomach and liver diseases, headaches, and congestion. In regions spanning Eastern Europe, Siberia, Mongolia, and China, D. moldavica has been used extensively for its traditional therapeutic properties. These include its applications in clearing heat and detoxifying, cooling the blood and purging internal ‘fire,’ alleviating pain, arresting bleeding, and providing relief from cough and asthma. Additionally, it is recognized for its expectorant effects and capacity to protect liver health (Morshedloo et al., 2020; Zhang et al., 2018). In China, D. moldavica is the main composition in certain clinical preparations, such as Qinggan Qiwei powder, Niuhuang Shisanwei pill, and Liganhewei pill (Xing et al., 2021).
In addition, H. officinalis essential oils have therapeutic effects on coughing, loss of appetite, fungal infections, spasmodic diseases, and antibacterial activity. The biological activity and aroma of essential oils indicate that they can serve as potential antioxidant food ingredients (Sharifi-Rad et al., 2022). Various compositional metabolites of L. iberica and L. royleana seeds such as proteins, oils, fatty acids, and carbohydrates play a significant role in their commercial value of the seeds. The oils, composition of fatty acids (linolenic acid, linoleic acid, and oleic acid), and mucilage are the main resources in Lallemantia seeds that are used by the food and pharmaceutical industries (Paravar et al., 2023). L. royleana is used as a diuretic, nourishing, aphrodisiac, and cough suppressant in traditional and folk medicine in Iran, and is used to treat various neurological, liver, and kidney diseases (Mohammed et al., 2022). The traditional application of the plant indicates that the medicinal value of Dracocephalum mainly focuses on clearing away heat and protecting the liver, which echoes the anti-inflammatory and hepatoprotective effects of Dracocephalum in modern pharmacological research, possibly through phenols, flavonoids, and terpenoids in Dracocephalum. Therefore, combining the anti-inflammatory and hepatoprotective effects of Dracocephalum in clinical practice, and effectively improving its clinical utilization rate will be the focus of Dracocephalum plant research.
5 Pharmacological activities
The pharmacological properties of Dracocephalum have attracted considerable attention in recent years. The main pharmacological activities of Dracocephalum include hepatoprotective, anti-inflammatory, antimicrobial, anti-hyperlipidemic, and antitumor. In addition, recent research has reported novel pharmacological effects. Table 3 lists some in vitro and in vivo pharmacological models and related dosage information used to clarify the pharmacological activities of Dracocephalum. The main pharmacological activities of Dracocephalum are as follows.
5.1 Hepatoprotective
Elevated levels of serum alanine aminotransferase (ALT) and aspartate aminotransferase (AST) are important markers of LPS and CCl4-induced liver injury (Weber et al., 2003; Xiong et al., 2017). Zhu et al. (2018) established a Kunming mice liver injury model induced by acute CCl4 and confirmed that the extract of phenols from D. rupestre reversed the increase in serum ALT and AST levels induced by CCl4 in a dose-dependent manner, and lowered them to near-normal levels (Zhu et al., 2018). D. heterophyllum can reduce plasma ALT, AST, IFN-γ, and TNF-α concentration in experimental autoimmune uveitis (EAU) mouse models at a dose of 20 mg/kg, indicating that D. heterophyllum has certain advantages in protecting against liver damage (Zheng et al., 2016). The MTT cell viability experiment showed that the extract of H. officinalis, at a concentration of 500 μg/mL, did not exhibit hepatotoxicity in mouse FL83B hepatocytes. Furthermore, this concentration of the H. officinalis extract displayed a protective effect against both palmitic acid-induced and acetaminophen-induced hepatotoxicity. This suggests that it may contribute to the mitigation or prevention of hepatocellular damage induced by these chemical agents to a certain extent.
5.2 Anti-inflammatory activity
Anti-inflammatory drugs are the second largest class of drugs after anti-infective drugs. Therefore, exploring the anti-inflammatory effects of natural products has become a new trend in the research and development of novel anti-inflammatory drugs (Li et al., 2012b). The anti-inflammatory effects of Dracocephalum have also been extensively studied, including the anti-inflammatory mechanism underlying its traditional use for treating typhoid fever, common cold, cardiovascular diseases, gastritis, sore throat, rheumatism, pulse disease, and scabies (Sadraei et al., 2017; Nie et al., 2021).
Current research has shown that at the third and fourth hours after administration of carrageenan, the highest dose of 200 mg/kg of methanol extract from H. officinalis has a significant inhibitory effect on rat foot edema (Mićović et al., 2022). In vitro inflammatory models are often established using LPS-stimulated macrophages (RAW 264.7 cells). Toshmatov et al. isolated new Monoterpene glucosides—komarovin B and komarovin C—from D. komarovii, and found that they inhibited LPS-induced NO production in macrophages to alleviate inflammation at concentrations of 1, 10, 50, and 100 μM (Toshmatov et al., 2019). The anti-inflammatory experiment using RAW 264.7 cells showed that dracocephalumoid A, uncinatone, trichotomone F, and caryopterisoid C from D. moldavica can inhibit LPS-induced TNF-α, IL-1β or the production of NO has a significant inhibitory effect, with IC50 values ranging from 1.12 to 5.84 μM (Nie et al., 2021).
5.3 Antimicrobial
Studies have shown that D. moldavica contains geranyl acetate, geranial, and neral, and can effectively inhibit Staphylococcus aureus, Escherichia coli, Salmonella typhimurium, and Listeria monocytogenes (Aćimović et al., 2022). Essential oil of H. officinalis effectively shows antifungal activity at concentrations of 500 and 1,000 μg/mL, with the main active metabolites being isopiperitenone, pinocampheol, and α-pinene (Harčárová et al., 2021). Rosmarinic acid, methyl rosmarinate, butyl rosmarinate, and salvigenin significantly inhibit E. coli, Candida albicans, and S. aureus growth in H. cuspidatus (Shomirzoeva et al., 2020). The antibacterial activity of D. kotschyi is mainly indicated by its inhibition of S. aureus and E. coli (Moridi Farimani et al., 2017). L. iberica showed antibacterial activity against E. coli, Pseudomonas aeruginosa, S. aureus and Enterobacter aerogenes, and the largest inhibitory activity was observed against P. aeruginosa and E. aerogenes (Yilmaz Kardas et al., 2023).
5.4 Antihyperlipidemic
Through a rat model, Aslian et al. validated that the D. kotschyi can upregulate the expression of p-FOXO1, p-AKT, and PPARγ and downregulate the expression of FOXO1, p-JNK, and SREBP-1 at concentrations of 0.25 and 0.5 mL/rat to exert antihyperlipidemic activity. In the pathogenesis and treatment of hyperlipidemia, multiple key signaling factors play significant roles. PPARγ, as a primary regulator of adipocyte differentiation and lipid metabolism, can promote the storage of fatty acids and the breakdown of lipids when activated, contributing to the reduction of blood lipid levels. FOXO1, acting as a transcription factor, affects lipid metabolism by inhibiting the activity of PPARγ. Phosphorylated FOXO1 (p-FOXO1) is usually associated with the suppression of FOXO1 activity, and its increase may help to reduce the negative impact of FOXO1 on lipid metabolism. Additionally, SREBP-1 influences blood lipid levels by regulating the synthesis of cholesterol and fatty acids. The activation of the AKT signaling pathway, particularly in the form of p-AKT, is crucial for improving insulin resistance and regulating lipid metabolism. The activation of p-JNK is often related to cellular stress responses and inflammatory reactions, and it may exacerbate insulin resistance by promoting the activation of FOXO1 (Aslian and Yazdanparast, 2018). The total flavonoid extract of D. moldavica can significantly improve hyperlipidemia in rats by regulating TG, LDLC, HDLC, ICAM-1, VCAM-1, PCNA, and other indicators at doses of 21, 42, and 84 mg/kg and exhibited a dose-dependent effect (Quan et al., 2017). In addition, the study on the protective effects of L. royleana seed polysaccharides against liver and kidney injury in rats induced by a high-cholesterol diet indicates their potential to safeguard hepatic and renal functions and tissue integrity, thereby providing a novel avenue for natural products in the management of hyperlipidemia (Mohammed et al., 2022).
5.5 Antitumor
Generally, when evaluating the antitumor activity of natural products, we mainly investigate their ability to inhibit tumor cell proliferation and promote immune cells to secrete cytokines acting on tumor cells (Zhang et al., 2019). Studies have shown that the methanolic extract of H. officinalis can increase apoptosis, reduce cell division, reduce tumor volume, and prolong survival in C6 glioma cells, mainly due to the upregulation of p53 and p21 mRNA expression (Khaksar et al., 2022). The D. taliense was tested for its antitumor activity against HepG2 cells using flavonoids at concentrations of 10, 20, 40, and 80 µM (Deng et al., 2017).
5.6 Other pharmacological activities
The hypoglycemic effects of D. tanguticum were confirmed in 3T3-L1 cells. It exerted moderate effects at a final concentration of 25 μM (Ma et al., 2020). The total flavonoids of D. moldavica (25, 50, 100 mg/kg/d) can upregulate MDA, SOD, and GSH-Px expression and downregulate IL-6, IL-8, and TNF-α expression in the brain tissue of model rats, effectively improving myocardial ischemia (Su et al., 2010). Frosmaric acid and oleanolic acid in D. moldavica also improve memory impairment (Deepa et al., 2020), whereas tilianin, luteolin, and apigenin improve vascular dementia (Liu M. et al., 2021). The antiviral effects of isosakuranetin glycosides and phenylpropanoid oligomers from D. foetidum, D. nutans, and D. fruticulosum have been confirmed through in vitro experiments (Sabrin et al., 2021). In animal models of systemic and local allergic reactions, Kim et al. found that the water extract of D. argunense regulates TNF-α and IL-6 in a dose-dependent manner, indicating its anti-allergic effect (Kim and Shin, 2006).
6 Discussion
Dracocephalum is a large and complex group of plants, and the intricate relationships between it and closely related genera can be discerned from the classification methods applied by scholars throughout history (Chen Y. P. et al., 2022). Preliminary pharmacophylogeny studies suggest that a certain regularity exists among medicinal plants of the genus Dracocephalum; species that are phylogenetically closer tend to exhibit some aggregation in terms of geographical distribution, chemical metabolites (the distribution pattern of these metabolites), and therapeutic effects (as reflected in ethnopharmacology and pharmacological activity) (Abbasi et al., 2022). We used Microsoft Excel 2010 software for statistical analysis and Cytoscape 3.7.2 software for visual presentation, establishing a network diagram (Figure 5). This diagram integrated data on plant species, metabolite profiles, pharmacological activities, traditional uses, and medicinal components of 21 medicinal plants from genus Dracocephalum and its related genera for a visual analysis. This approach facilitated the exploration of their untapped potential. D. moldavica, D. heterophyllum, D. forrestii, H. cuspidatus, and H. officinalis all show higher connectivity. Extensive research has been conducted on the metabolites and pharmacological effects of these species, which have significant traditional applications and are known for their terpenoids, flavonoids, and phenylpropanoids with high chemical connectivity. These metabolites have been found to possess hepatoprotective properties, as well as anti-inflammatory, antitumor, and antimicrobial effects (Figure 5). D. moldavica and H. cuspidatus had the most reported types of metabolites, with relatively balanced proportions. D. heterophyllum, D. kotschyi, and D. forrestii have several reported metabolites, particularly terpenoids in the studied types. In contrast, reports on D. austriacum, D. rupestre, D. botryoides, and D. palmatum are predominantly related to their flavonoid and phenylpropanoid contents. This phenomenon indirectly reflects the medicinal tendencies of these species, illustrating a potential dialectical relationship between the traditional efficacy and metabolite diversity.
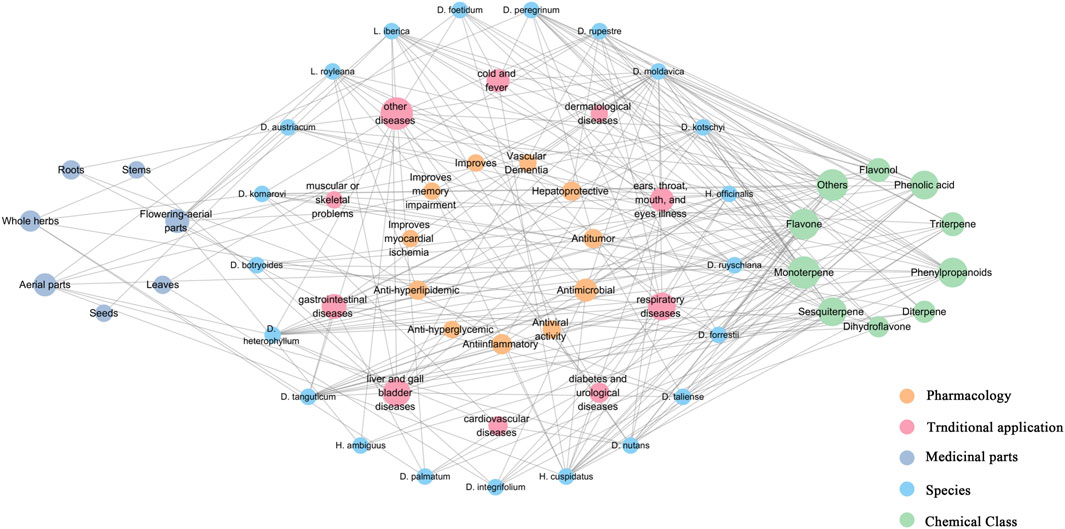
Figure 5. Network analysis of genus Dracocephalum-chemical metabolites-traditional application and pharmacological activities. (We used Microsoft Excel 2010 software for statistical analysis and Cytoscape 3.7.2 software for visual presentation and established a network diagram. Nodes represent medicinal plants, chemical metabolites, traditional applications, and medicinal parts of Dracocephalum, and edges are connected to represent their mutual relationship; Connectivity indicates the number of edges directly associated with a node in the network diagram. The greater the connectivity, the wider the network of the node.
The phylogenetic tree established by Chen G. W. et al. (2022) featured nine clades, eight of which contained species of medicinal varieties. However, the functions of many of these medicinal plants are only documented in traditional applications, without clear pharmacological mechanisms. D. moldavica is recognized as the Dracocephalum species with the richest known diversity of compounds and the most extensive pharmacological activities. However, some of its traditional medicinal claims remain unverified. Phylogenetic analysis has demonstrated that D. moldavica, D. heterophyllum, and D. kotschyi are closely related. In traditional ethnomedical practices, these species are utilized for the treatment of liver and gall bladder diseases. Specifically, D. moldavica, D. heterophyllum, and D. kotschyi are reputed to possess therapeutic effects against hepatitis, jaundice, and liver pain, respectively. However, in our survey, we identified only studies concerning the ethanol extract of D. heterophyllum and its impact on ConA-Induced Acute Hepatitis. Therefore, many extended discussions on the efficacy of medicinal plants are based on traditional applications. Medicinally related species include D. foetidum and D. komarovii, as well as some closely related plants that have not yet been documented, including Dracocephalum stamineum Kar. & Kir., Dracocephalum renati Emb., and Dracocephalum spinulosum Popov. The lack of documentation for these species may be attributed to their perceived lack of significant medicinal properties or because their potential remains undeveloped or has not been fully investigated. Medicinal plants, such as D. nutans, D. integrifolium, and H. cuspidatus are closely related and form a single branch in the phylogenetic tree. They are all effective in treating respiratory diseases; therefore, we speculate that closely related species such as Dracocephalum psammophilum C.Y.Wu & W.T.Wang and Hyssopus seravschanicus (Dubj.) Pazij may have a similar efficacy. Similarly, the clade comprising D. calophyllum, D. forrestii, D. taliense, and D. tanguticum is known to exhibit therapeutic effects on gastrointestinal conditions, raising the question of whether Dracocephalum propinquum W.W.Sm. and Dracocephalum microphyton Y.P.Chen, Y.S.Chen & C.L.Xiang share similar beneficial properties, meriting further investigation. Furthermore, although neurological disorders are grouped within the category of “Other diseases,” the shared efficacy of L. royleana and L. iberica in addressing neurological as well as hepatic and renal diseases is particularly noteworthy. Consequently, the closely related species Lallemantia peltata (L.) Fisch. & C.A.Mey. and Dracocephalum scrobiculatum Regel should also garner the interest of researchers because of their potential applications in this area. In addition, D. rupestre, which has the potential for use in medicine and food, can be used as a tea. According to the theory of consanguinity, we speculate that D. bipinnatum and Dracocephalum adylovii I.I.Malzev are likely homologous plants used in medicine and food. Therefore, there is an urgent need to provide a suitable theoretical and technical system for medicinal and edible homologous items, enhance the scientific connotations of medicinal and edible homologous items, and allow people to use medicinal and edible homologous items to meet the needs of contemporary health and disease (Hao et al., 2022; He et al., 2022; Zhang et al., 2022; Pan et al., 2022; Selvaraj and Gurumurthy, 2022; Yao et al., 2022).
The genus Dracocephalum originated from the steppe-desert biomes of Central and West Asia, and the alpine region of the QTP. It has a long history of medicinal uses. Previous studies showed that this genus spread from East and West Asia to QTP alpine region during the Pliocene. Drought on the Eurasian continent has promoted rapid radiation in the region, and the uplift of QTP has promoted the distribution and diversity of species in this genus. D. forrestii occurs only in the northwestern mountains of Yunnan Province, China. D. taliense is distributed across Dali, Yunnan. D. integrifolium is found primarily in Xinjiang, China. D. nutans is found throughout Siberia, Eastern Europe, and Kashmir. It is also seen in Heilongjiang, Inner Mongolia, and northern Xinjiang. D. moldavica, and D. heterophyllum are found in Inner Mongolia, Gansu, Qinghai, and in Eastern Europe. Dracocephalum paulsenii Briq., and Dracocephalum origanoides Stephan ex Willd. are distributed in Xinjiang and Russia. Hyssopus latilabiatus C.Y.Wu & H.W.Li, H. cuspidatus, D. integrifolium, and D. nutans are distributed in Xinjiang. H. officinalis is distributed in Europe, Algeria, Morocco, Iran and other places, whereas D. moldavica, D. rupestre, D. heterophyllum, and H. officinalis overlap in Inner Mongolia, Shanxi, Gansu, and Qinghai. H. cuspidatus has been found in North Africa, India, Mongolia, Russia, and China (Deepa et al., 2020). L. iberica originated in the Caucasus and the Middle East and is widely distributed in some western parts of Europe and Asia, while L. royleana grows in Iran and almost all parts of Middle East. The genus Hyssopus is widely distributed in Central and West Asia, Siberia, and Europe, whereas the genus Lallemantia is distributed in West Asia and L. royleana is further extended to Central Asia, which is consistent with our research. We preliminary verified the rationality of combining Hyssopus and Lallemantia with Dracocephalum through the spatial distribution pattern map and the suitable Ecotope (Figure 1). From the perspective of spatial distribution patterns, the distribution of genus Dracoephalum is wider than that of Hyssopus and Lallemantia, but the two have overlapping distributions in the European region. From the perspective of suitable Ecotope, Dracocephalum, Lallemantia and Hyssopus were suitable for growing in western North America and western Asia. Consistent with the results of our previous phylogenetic studies, Sect. Dracocephalum, Sect. Sinodracon, and Sect. Keimodracon are phylogenetically close to each other and can be used to verify the phylogenetic relationships between the Hyssopus, Lallemantia, and Dracocephalum genera at the geographical distribution level (Chen Y. P. et al., 2022).
Dracocephalum grandiflorum L., D. integrifolium, D. nutans, D. origanoides, D. paulsenii, D. forrestii, D. heterophyllum, D. moldavica, D. grandiflorum, D. bipinnatum, D. peregrinum, D. rupestre, Dracocephalum rigidulum Hand.-Mazz., L. iberica and Hyssopus species share similarities in clearing heat, detoxifying, cooling blood, relieving bleeding, treating bronchitis, and relieving cough and asthma. D. forrestii has traditional efficacy in the treatment of cardiovascular diseases and rheumatoid arthritis, which has not yet been proven in pharmacological studies. Both modern pharmacological research and traditional application have shown that D. rupestre, D. nutans, D. taliense, D. tanguticum, D. peregrinum, D. heterophyllum, H. officinalis, L. iberica, and L. royleana possess hepatoprotective activities. The Encyclopedia of Chinese Medicine records that the aboveground parts of D. moldavica are used to treat all liver disorders (Editorial Board of Chinese Medical Encyclopedia, 1992). Terpenoids and flavonoids are abundant in closely related species, among which, monoterpenes are the most common, and the dominant flavonoids are flavones and their glycosides (Table 1). We speculate that the traditional therapeutic properties of clearing heat and detoxifying, treating bronchitis, relieving cough, and relieving asthma may be related to the content of monoterpenes and flavonoids in D. heterophyllum, D. rupestre, D. forrestii, D. integrifolium, D. nutans, D. origanoides, L. iberica, H. latilabiatus, H. cuspidatus, which supported the anti-inflammatory activity of H. cuspidatus to a certain extent. According to the theory of pharmacosystematics, D. integrifolium, D. nutans, D. rigidulum, D. origanoides, D. forrestii, and L. royleana may be a new potential resource for the development of anti-inflammatory drugs. Both L. royleana and D. moldavica exhibit antibacterial, and anti-hyperlipidemic activities, possibly owing to the presence of soluble fiber and polysaccharides. Moreover, triterpenoids were concentrated in H. cuspidatus, D. taliense and D. forrestii of Sect. Sinodracon, but Ser. Angustifolii and Sect. Sinodracon and Sect. Idiodracon exhibited antibacterial, anti-tussive, and anti-asthmatic effects, implying that known triterpenoids might not be the only active substances, and other effective metabolites are yet to be identified (Tables 2, 3). Both Sect. Calodracon and Sect. Dracocephalum contains essential oils, seven-substituted flavonoid glycosides and three-substituted flavanol glycosides (Table 1). Ser. Officinales Boris., Sect. Calodracon, and Sect. Dracocephalum have the common effects of protecting the liver, clearing heat and toxic materials, relieving cough and asthma, and eliminating phlegm. Additionally, Sect. Calodracon and Ser. Officinales Boris. have the common effects of cooling the blood, and purging fire. Based on these effects, Sect. Calodracons can also prevent bleeding and promote granulation. Therefore, these phylogenetically close species may have similar pharmacodynamic bases, such as chemical composition and therapeutic effects, which are relevant to their clinical efficacy. Phytochemistry research has identified 19 species of Dracocephalum, Lallemantia, and Hyssopus, and found that most of them contain terpenoids, flavonoids, and phenylpropanoids. These metabolites are key substances for medicinal plants to exert pharmacological effects, with good pharmacological activity and potential anti-inflammatory, and hepatoprotective effects. In addition, plant metabolites not only have pharmacological effects but also determine their traditional applications. The genus Dracocephalum is widely used for the treatment of cough, fever, and liver and gall bladder diseases. Owing to the similar pharmacological activities of the three genera, Lallemantia and Hyssopus have similar traditional therapeutic effects. Based on the latest phylogenetic and biogeography studies of Dracocephalum, we provide further evidence for the merger of Lallemania and Hyssopus into Dracocephalum and lay a foundation for finding plants with the medicinal value of this kind in the future.
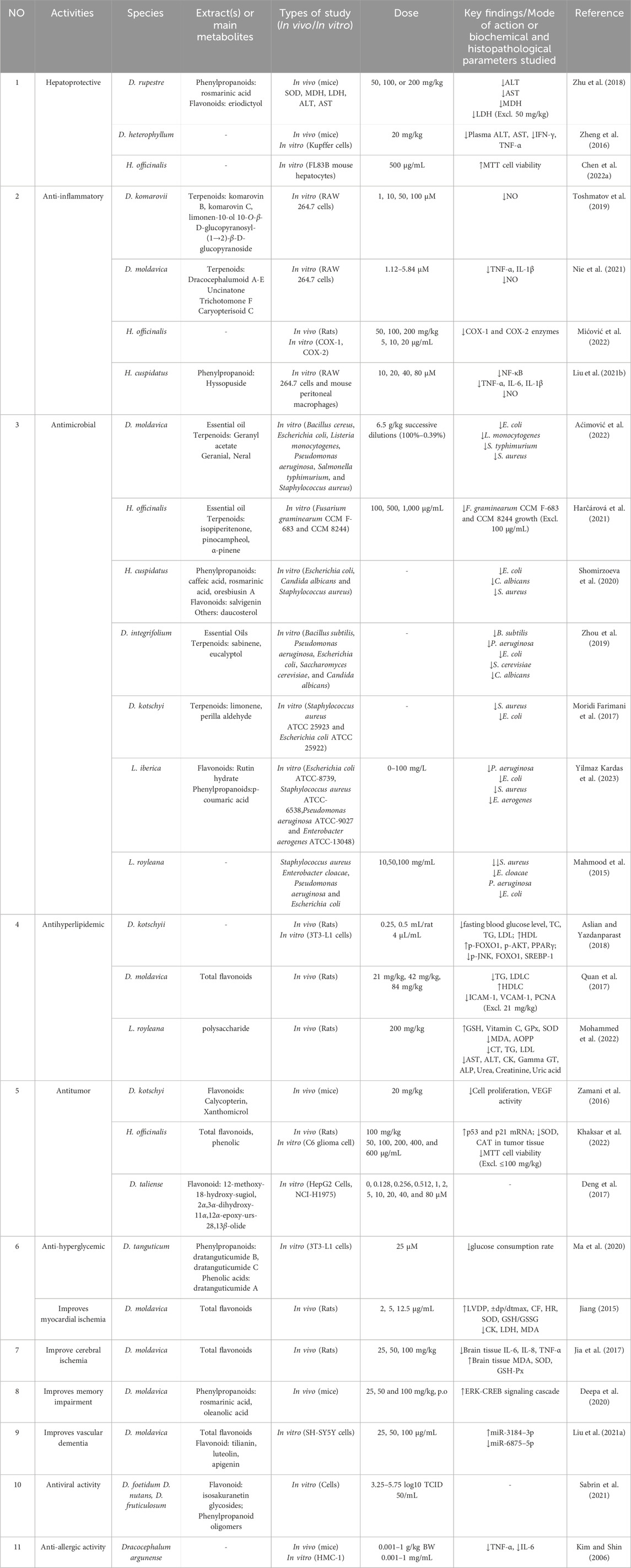
Table 3. Pharmacological activities of the medicinal plants of genus Dracocephalum and its related genera.
7 Conclusion and prospects
In conclusion, we have shown that Hyssopus, Lallemantia, and Dracocephalum are closely related in many aspects, including molecular phylogeny, species distribution model, chemical composition, pharmacological activity, and traditional application. The division of these two genera into Dracocephalum was reasonable. Most flavonoids have flavonoid glycosides or flavonoid glycosides as their parent nuclear structure; therefore they have anti-inflammatory properties, but their pharmacological effects differ owing are different due to the different positions of the substituents. Modern pharmacological studies have justified some traditional medicinal uses, which may be partly attributed to the bioactivities of terpenoids, flavonoids, phenylpropanoids and phenolic acid, however, pharmacological mechanisms should be revealed to provide a scientific explanation for the traditional curative effect of Dracocephalum medicinal plants. In contrast, there are relatively few studies on the chemical metabolites of Dracocephalum, drug safety, effectiveness, and corresponding toxicological mechanisms, and the rationale for disease treatment remains uncertain. Although some species are used in traditional therapeutics, they lack verification of modern pharmacological research. Many metabolites have been identified using LC-MS, including indole alkaloids, flavonoids, terpenoids, and phenolic acids; however, some species of the genus Dracocephalum remain understudied with respect to their chemical metabolites. This review focused on Sect. Sinodracon, Sect. Idiodracon, Sect. Calodracon, and Sect. Dracocephalum. The commonly recorded efficacies of these sections are clearing away heat and toxic materials, relieving cough and asthma, eliminating phlegm, and protecting the liver. While studying the phylogenetic relationships of the genus Dracocephalum, we found that species in the Hyssopus and Lallemantia genera share a similar chemical composition, pharmacological activity, and traditional therapeutic effects. Network pharmacology has made some contributions to clarify the distance of plant genetic relationship from the perspective of mechanism.
In this review, the phamacophylogenetic relationship among Hyssopus, Lallemantia, and Dracocephalum is discussed from many standpoints, and on this basis, the related target pathways are predicted through network pharmacology, which helps to re-examine traditional usage, design/optimize experiments, to further unearth the associations among evolutionary relationship, chemical composition, and pharmacological activity. Based on previous studies, ecological adaptability evaluation was carried out to provide a research basis for the future cultivation of Dracocephalum, and clinical substitution of drugs among Hyssopus, Lallemantia, and Dracocephalum. Beyond this, there is considerable scope for future investigation into the conservation and utilization of plant resources, as well as the relationships of medicinal plants among these genera. For example, by employing species distribution prediction models in conjunction with various databases, it was possible to forecast potential suitable growth areas for endangered plants in these genera, followed by targeted conservation and cultivation efforts (Zhang et al., 2023). Furthermore, the application of genomics, transcriptomics, and metabolomics were crucial for exploring the origins of plant medicinal metabolism. Yang et al. (2023) sequenced the genomes of three Solanaceae species that produce hyoscyamine and scopolamine (HS) and one species that does not, revealing a shared biosynthetic pathway for HS across distantly related lineages, contributing to our understanding of HS biosynthesis. Correspondingly, the biosynthetic pathway of komaroviquinone, a distinctive pharmacological effect present from D. kotschyi, merits thorough investigation. This study not only enriches the theory of pharmacy but also provides a reference for the medicinal application of Dracocephalum medicinal plants. Unfortunately, for a long time, the medicinal value of the 60 species of Dracocephalum has not aroused enough attention or in-depth research; only a few species of chemical and pharmacological activities have been given due attention. For this reason, it is particularly urgent to find suitable substitutes and appropriate cultivation techniques. In this study, the genetic relationship between species of Dracocephalum was studied under the guidance of the genetic theory of medicinal plants, and it was skillfully transformed into the genetic relationship of modern scientific drugs, which was more helpful to understand and explore the medicinal value of Dracocephalum, and also provided the reference for the development of other medicinal plants. In addition, the internal relationships among the medicinal plants of Dracocephalum are still unclear, and these areas deserve further study in the future.
Author contributions
HL: Visualization, Writing–original draft, Writing–review and editing. XF: Writing–original draft. YZ: Writing–original draft. GL: Data curation, Investigation, Writing–original draft. CZ: Resources, Supervision, Writing–original draft. Aruhan: Investigation, Visualization, Writing–original draft. T-AD.: Investigation, Visualization, Writing–original draft. NZ: Resources, Supervision, Writing–original draft. DH: Conceptualization, Methodology, Resources, Supervision, Writing–original draft. ML: Conceptualization, Methodology, Resources, Supervision, Writing–original draft.
Funding
The author(s) declare that financial support was received for the research, authorship, and/or publication of this article. This research was financially supported by the project of “Belt and Road” Traditional Medicinal Plant Species Inventory and Database Construction (2018FY100700); Standardized cultivation demonstration, promotion, and technical training for bulk, geographically authentic medicinal materials in Inner Mongolia (2023GLLH0113); the National Key Research and Development Program of China (2021YFE0190100); the National Natural Science Foundation of China (No. 81903925); the project of Education Department of Inner Mongolia Autonomous Region (NJZY22053).
Conflict of interest
The authors declare that the research was conducted in the absence of any commercial or financial relationships that could be construed as a potential conflict of interest.
Publisher’s note
All claims expressed in this article are solely those of the authors and do not necessarily represent those of their affiliated organizations, or those of the publisher, the editors and the reviewers. Any product that may be evaluated in this article, or claim that may be made by its manufacturer, is not guaranteed or endorsed by the publisher.
Supplementary material
The Supplementary Material for this article can be found online at: https://www.frontiersin.org/articles/10.3389/fphar.2024.1449426/full#supplementary-material
References
Abbasi, B. H., Siddiquah, A., Tungmunnithum, D., Bose, S., Younas, M., Garros, L., et al. (2019). Isodon rugosus (wall. ex Benth.) codd in vitro cultures: establishment, phytochemical characterization and in vitro antioxidant and anti-aging activities. Int. J. Mol. Sci. 20 (2), 452. doi:10.3390/ijms20020452
Abbasi, N., Fattahi, M., Ghosta, Y., and Sefidkon, F. (2022). Volatile compounds and antifungal activity of Dracocephalum moldavica L. at different phenological stages. J. Essent. Oil Res. 34 (1), 87–95. doi:10.1080/10412905.2021.1975577
Aćimović, M., Šovljanski, O., Šeregelj, V., Pezo, L., Zheljazkov, V. D., Ljujić, J., et al. (2022). Chemical composition, antioxidant, and antimicrobial activity of dracocephalum moldavica L. Essential oil and hydrolate. Plants (Basel) 11 (7), 941. doi:10.3390/plants11070941
Aihaiti, K., Li, J., Yaermaimaiti, S., Liu, L., Xin, X., and Aisa, H. A. (2022). Non-volatile compounds of Hyssopus cuspidatus Boriss and their antioxidant and antimicrobial activities. Food Chem. 374, 131638. doi:10.1016/j.foodchem.2021.131638
Akshatha, J. V., SantoshKumar, H. S., Prakash, H. S., and Nalini, M. S. (2021). In silico docking studies of α-amylase inhibitors from the anti-diabetic plant Leucas ciliata Benth. and an endophyte, Streptomyces longisporoflavus. 3 Biotech. 11 (2), 51. doi:10.1007/s13205-020-02547-0
Ashrafi, B., Ramak, P., Ezatpour, B., and Talei, G. R. (2017). Investigation on chemical composition, antimicrobial, antioxidant, and cytotoxic properties of essential oil from Dracocephalum kotschyi Boiss. Afr. J. traditional, complementary, Altern. Med. 14 (3), 209–217. doi:10.21010/ajtcam.v14i3.23
Aslian, S., and Yazdanparast, R. (2018). Hypolipidemic activity of Dracocephalum kotschyi involves FOXO1 mediated modulation of PPARγ expression in adipocytes. Lipids Health Dis. 17 (1), 245. doi:10.1186/s12944-018-0893-3
Atriya, A., Majee, C., Mazumder, R., Choudhary, A. N., Salahuddin, , Mazumder, A., et al. (2023). Insight into the various approaches for the enhancement of bioavailability and pharmacological potency of terpenoids: a review. Curr. Pharm. Biotechnol. 24 (10), 1228–1244. doi:10.2174/1389201024666221130163116
Boncan, D. A. T., Tsang, S. S. K., Li, C., Lee, I. H. T., Lam, H. M., Chan, T. F., et al. (2020). Terpenes and terpenoids in plants: interactions with environment and insects. Int. J. Mol. Sci. 21 (19), 7382. doi:10.3390/ijms21197382
Chahardoli, A., Karimi, N., Fattahi, A., and Salimikia, I. (2019). Biological applications of phytosynthesized gold nanoparticles using leaf extract of Dracocephalum kotschyi. J. Biomed. Mater. Res. Part A 107 (3), 621–630. doi:10.1002/jbm.a.36578
Cham, R., Abtahi, S. A., Jafarinia, M., and Yasrebi, J. (2022). Physiological responses of Dracocephalum kotschyi Boiss. to drought stress and Bio-fertilizers. South Afr. J. Bot. 148, 180–189. doi:10.1016/j.sajb.2022.04.008
Chen, G. W., Chen, T. Y., and Yang, P. M. (2022a). Differential effect of herbal tea extracts on free fatty acids-ethanol- and acetaminophen-induced hepatotoxicity in FL83B hepatocytes. Drug Chem. Toxicol. 45 (1), 347–352. doi:10.1080/01480545.2019.1692026
Chen, K., Li, G. J., Bressan, R. A., Song, C. P., Zhu, J. K., and Zhao, Y. (2020). Abscisic acid dynamics, signaling, and functions in plants. J. Integr. plant Biol. 62 (1), 25–54. doi:10.1111/jipb.12899
Chen, S. B., Peng, Y., Chen, S. L., and Xiao, P. G. (2005). Introduction of pharmaphylogeny. Mod. Trad. Chin. Med. Mat. Medica-World Sci. Technol. 7 (6), 97–103.
Chen, Y. P., Turdimatovich, T. O., Nuraliev, M. S., Lazarević, P., Drew, B. T., and Xiang, C. L. (2022b). Phylogeny and biogeography of the northern temperate genus Dracocephalum s.l. (Lamiaceae). Cladistics 38 (4), 429–451. doi:10.1111/cla.12502
Christenhusz, M. J. M., and Byng, J. W. (2016). The number of known plants species in the world and its annual increase. Phytotaxa 261 (3), 201–217. doi:10.11646/phytotaxa.261.3.1
Conrad, J., Förster-Fromme, B., Constantin, M. A., Ondrus, V., Mika, S., Mert-Balci, F., et al. (2009). Flavonoid glucuronides and a chromone from the aquatic macrophyte Stratiotes aloides. J. Nat. Prod. 72 (5), 835–840. doi:10.1021/np800769g
Dai, L. M., Zhao, C. C., Jin, H. Z., Tang, J., Shen, Y. H., Li, H. L., et al. (2008). A new ferulic acid ester and other constituents from Dracocephalum peregrinum. Archives pharmacal Res. 31 (10), 1325–1329. doi:10.1007/s12272-001-2113-2
Deepa, P., Bae, H. J., Park, H. B., Kim, S. Y., Choi, J. W., Kim, D. H., et al. (2020). Dracocephalum moldavica attenuates scopolamine-induced cognitive impairment through activation of hippocampal ERK-CREB signaling in mice. J. Ethnopharmacol. 253, 112651. doi:10.1016/j.jep.2020.112651
Deng, A. P., Guo, L. P., Zhan, Z. L., and Huang, L. Q. (2016). Decipherment of ancient literature about Danshen. Zhongguo Zhong yao za zhi 41 (22), 4274–4279. doi:10.4268/cjcmm20162230
Deng, Y., Hua, J., Wang, W., Zhan, Z., Wang, A., and Luo, S. (2017). Cytotoxic terpenoids from the roots of Dracocephalum taliense. Molecules 23 (1), 57. doi:10.3390/molecules23010057
Dettweiler, M., Melander, R. J., Porras, G., Risener, C., Marquez, L., Samarakoon, T., et al. (2020). A clerodane diterpene from Callicarpa americana resensitizes methicillin-resistant Staphylococcus aureus to β-lactam antibiotics. ACS Infect. Dis. 6 (7), 1667–1673. doi:10.1021/acsinfecdis.0c00307
Editorial Board of Chinese Medical Encyclopedia (1992). Chinese medical Encyclopedia: Mongolian medicine. Shanghai: Shanghai Scientific &Technical, 206–207.
Fattahi, A., Shakeri, A., Tayarani-Najaran, Z., Kharbach, M., Segers, K., Heyden, Y. V., et al. (2021). UPLC-PDA-ESI-QTOF-MS/MS and GC-MS analysis of Iranian Dracocephalum moldavica L. Food Sci. and Nutr. 9 (8), 4278–4286. doi:10.1002/fsn3.2396
Ferreira, E. deO., Fernandes, M. Y., Lima, N. M., Neves, K. R., Carmo, M. R., Lima, F. A., et al. (2016). Neuroinflammatory response to experimental stroke is inhibited by eriodictyol. Behav. Brain Res. 312, 321–332. doi:10.1016/j.bbr.2016.06.046
Fu, P., Zhao, C. C., Tang, J., Shen, Y. H., Xu, X. K., and Zhang, W. D. (2009). New flavonoid glycosides and cyanogenic glycosides from Dracocephalum peregrinum. Chem. Pharm. Bull. 57 (2), 207–210. doi:10.1248/cpb.57.207
Ghannadi, A., and Zolfaghari, B. (2003). Compositional analysis of the essential oil of Lallemantia royleana (Benth. In wall.) Benth. From Iran. Flavour Fragr. J. 18, 237–239. doi:10.1002/ffj.1215
Ghavam, M., Manconi, M., Manca, M. L., and Bacchetta, G. (2021). Extraction of essential oil from Dracocephalum kotschyi Boiss. (Lamiaceae), identification of two active compounds and evaluation of the antimicrobial properties. J. Ethnopharmacol. 267, 113513. doi:10.1016/j.jep.2020.113513
Gong, X., Yang, M., He, C. N., Bi, Y. Q., Zhang, C. H., Li, M. H., et al. (2022). Plant pharmacophylogeny: review and future directions. Chin. J. Integr. Med. 28 (6), 567–574. doi:10.1007/s11655-020-3270-9
Gu, C. Y., Pan, R., Abid, M., Zang, H. Y., Yang, X., and Chen, Y. (2021). First report of blackleg disease caused by Epicoccum sorghinumon on lavender (Lavandula stoechas) in China. Plant Dis. [Epub ahead of print]. doi:10.1094/PDIS-10-20-2280-PDN
Guo, M., Gu, L., Hui, H., Li, X., and Jin, L. (2022). Extracts of dracocephalum tanguticum maxim ameliorate acute alcoholic liver disease via regulating transcription factors in mice. Front. Pharmacol. 13, 830532. doi:10.3389/fphar.2022.830532
Hao, D. C., Gu, X., and Xiao, P. (2017). Anemone medicinal plants: ethnopharmacology, phytochemistry and biology. Acta Pharm. Sin. B 7 (2), 146–158. doi:10.1016/j.apsb.2016.12.001
Hao, D. C., and Xiao, P. G. (2020). Pharmaceutical resource discovery from traditional medicinal plants: pharmacophylogeny and pharmacophylogenomics. Chin. Herb. Med. 12 (2), 104–117. doi:10.1016/j.chmed.2020.03.002
Hao, D. C., Xu, L. J., Zheng, Y. W., Lyu, H. Y., and Xiao, P. G. (2022). Mining therapeutic efficacy from treasure chest of biodiversity and chemodiversity: pharmacophylogeny of ranunculales medicinal plants. Chin. J. Integr. Med. 28 (12), 1111–1126. doi:10.1007/s11655-022-3576-x
Harčárová, M., Čonková, E., Proškovcová, M., Váczi, P., Marcinčáková, D., and Bujňák, L. (2021). Comparison of antifungal activity of selected essential oils against Fusarium graminearum in vitro. Ann. Agric. Environ. Med. 28 (3), 414–418. doi:10.26444/aaem/137653
He, C., Zhao, X., Yao, R., and Xiao, P. (2022). Food-medicine can promote cross-culture communication between East and West. Chin. Herb. Med. 15 (1), 3–5. doi:10.1016/j.chmed.2022.12.003
He, J. W., Cao, L., Zhou, H. R., Zhong, W. H., and Zhong, G. Y. (2015). Analysis of varieties and standards of labiatae medicinal plants used in Tibetan medicine. China J. Chin. Materia Medica 40 (7), 1419–1424. doi:10.4268/cjcmm20150738
Heydari, P., Yavari, M., Adibi, P., Asghari, G., Ghanadian, S. M., Dida, G. O., et al. (2019). Medicinal properties and active constituents of dracocephalum kotschyi and its significance in Iran: a systematic review. Evidence-based complementary Altern. Med. eCAM 2019, 9465309. doi:10.1155/2019/9465309
Hsu, W. Y., Simonne, A., Jitareerat, P., and Marshall, M. R., Jr. (2010). Low-dose irradiation improves microbial quality and shelf life of fresh mint (Mentha piperita L.) without compromising visual quality. J. Food Sci. 75 (4), M222–30. doi:10.1111/j.1750-3841.2010.01568.x
Huang, L. Q. (2022). Chinese pharmacopoeia of traditional Chinese medicine resources-volume 4. Beijing: Beijing Science and Technology Press, 230–233.
Jia, C. Y., Aibti, P., Sun, J. N., A, R., and Zhang, H. Y. (2022). GC-MS analysis of volatile oil from Hyssopus cuspidatus and Hyssopus latilabiatus. Food Drug 24, 319–322. doi:10.3969/j.issn.1672-979X.2022.04.007
Jia, J. X., Zhang, Y., Wang, Z. L., Yan, X. S., Jin, M., Huo, D. S., et al. (2017). The inhibitory effects of Dracocephalum moldavica L. (DML) on rat cerebral ischemia reperfusion injury. J. Toxicol. Environ. health. Part A 80 (22), 1206–1211. doi:10.1080/15287394.2017.1367139
Jiang, H., He, W., and Zhang, C. (2018). Effect of total flavonoids of Dracocephalum heterophyllum Benth on norepinephrine-induced cardiomyocyte hypertrophy in rats. Chin. J. Pharmacol. Toxicol. 31 (9), 291–297. doi:10.19577/j.1007-4406.2018.05.001
Jiang, J. W. (2015). A quick-consultative dicitionary of world medicinal plants. China: China medical science press, 327–328.
Kashchenko, N. I., Jafarova, G. S., Isaev, J. I., Olennikov, D. N., and Chirikova, N. K. (2022). Caucasian dragonheads: phenolic compounds, polysaccharides, and bioactivity of dracocephalum austriacum and dracocephalum botryoides. Plants-Basel 11 (16), 2126. doi:10.3390/plants11162126
Khaksar, S., Kiarostami, K., and Alinaghi, S. (2022). The effects of methanol extracts of Hyssopus officinalis on model of induced glioblastoma multiforme (GBM) in rats. J. Mol. Neurosci. 72 (9), 2045–2066. doi:10.1007/s12031-022-02058-y
Khamesipour, F., Razavi, S. M., Hejazi, S. H., and Ghanadian, S. M. (2021). In vitro and in vivo Anti-Toxoplasma activity of Dracocephalum kotschyi essential oil. Food Sci. Nutr. 9 (1), 522–531. doi:10.1002/fsn3.2021
Kim, S. H., and Shin, T. Y. (2006). Effect of Dracocephalum argunense on mast-cell-mediated hypersensitivity. Int. Arch. Allergy Immunol. 139 (2), 87–95. doi:10.1159/000090383
Lee, S. B., Cha, K. H., Kim, S. N., Altantsetseg, S., Shatar, S., Sarangerel, O., et al. (2007). The antimicrobial activity of essential oil from Dracocephalum foetidum against pathogenic microorganisms. J. Microbiol. (Seoul, Korea) 45 (1), 53–57. Available at: https://pubmed.ncbi.nlm.nih.gov/17342056/.
Li, C., Dang, J., Lv, Y., Fang, Y., Ma, C., Wang, Q., et al. (2022). The isolation and preparation of samwinol from dracocephalum heterophyllum and prevention on aβ(25-35)-induced neuroinflammation in PC-12 cells. Int. J. Mol. Sci. 23 (19), 11572. doi:10.3390/ijms231911572
Li, M., Liu, Y., Wang, G., Zheng, Z., Cui, Z., Huang, L., et al. (2012a). Ethnopharmacological survey of medicinal plants in Baotou,Inner Mongolia,China. Chin. Herb. Med. (04), 301–313. doi:10.3969/j.issn.1674-6348.2012.04.007
Li, P. (2021). “An exploration of multi-omics-based Pharmacophylogeny: The genera Dichocarpum W.T. Wang et Hsiao and Paeonia L. as two cases,”. [Doctoral dissertation] (Beijing: Peking Union Medical College), 11.
Li, S. M., Yang, X. W., Li, Y. L., Shen, Y. H., Feng, L., Wang, Y. H., et al. (2009). Chemical constituents of Dracocephalum forrestii. Planta medica 75 (15), 1591–1596. doi:10.1055/s-0029-1185868
Li, S. S., and Zhu, Y. (2012b). Research progress of anti-inflammatoy action in active ingredient of Chinese drugs. Chin. Arch. Tradit. Chin. Med. 30, 143–146. doi:10.13193/j.archtcm.2012.01.145.lishsh.059
Liu, M., Shan, G., Jiang, H., Zeng, L., Zhao, K., Li, Y., et al. (2021a). Identification of miRNA and their regulatory effects induced by total flavonoids from dracocephalum moldavica in the treatment of vascular dementia. Front. Pharmacol. 12, 796628. doi:10.3389/fphar.2021.796628
Liu, X., Su, J., Wang, G., Zheng, L., Wang, G., Sun, Y., et al. (2021b). Discovery of phenolic glycoside from hyssopus cuspidatus attenuates LPS-induced inflammatory responses by inhibition of iNOS and COX-2 expression through suppression of NF-κB activation. Int. J. Mol. Sci. 22 (22), 12128. doi:10.3390/ijms222212128
Lv, Y., Li, C., Wang, Z., Wang, Q., Li, G., and Dang, J. (2022). Preparative isolation of antioxidative furanocoumarins from Dracocephalum heterophyllum and their potential action target. J. Sep. Sci. 45 (24), 4375–4387. doi:10.1002/jssc.202200309
Ma, E. G., Wu, H. Y., Hu, L. J., Wei, M., Mou, L. Y., and Li, G. P. (2020). Three new phenylacetamide glycosides from Dracocephalum tanguticum Maxim and their anti-hyperglycemic activity. Nat. Prod. Res. 34 (13), 1827–1835. doi:10.1080/14786419.2018.1562451
Mahmood, S., Hayat, M. Q., Sadiq, A., Ishtiaq, S., Malik, S., and Ashraf, M. A. (2015). Antibacterial activity of Lallemantia royleana (Benth.) indigenous to Pakistan. Afr. J. Microbiol. Res. 9, 800–813.
Matoušek, J., Kocábek, T., Patzak, J., Füssy, Z., Procházková, J., and Heyerick, A. (2012). Combinatorial analysis of lupulin gland transcription factors from R2R3Myb, bHLH and WDR families indicates a complex regulation of chs_H1 genes essential for prenylflavonoid biosynthesis in hop (Humulus Lupulus L.). BMC plant Biol. 12, 27. doi:10.1186/1471-2229-12-27
Mićović, T., Katanić Stanković, J. S., Bauer, R., Nöst, X., Marković, Z., Milenković, D., et al. (2022). In vitro, in vivo and in silico evaluation of the anti-inflammatory potential of Hyssopus officinalis L. subsp. aristatus (Godr.) Nyman (Lamiaceae). J. Ethnopharmacol. 293, 115201. doi:10.1016/j.jep.2022.115201
Mohammed, A. F., Amal, F., Intissar, K., Malek, A., Manel, N., Rim, K., et al. (2022). Hypocholesterolemic effects of a polysaccharide isolated from Balangu (Lallemantia royleana) seeds in Wistar rat fed on a cholesterol-containing diet. Cell. Mol. Biol. (Noisy-le-Grand, France) 68 (10), 38–46. doi:10.14715/cmb/2022.68.10.6
Moridi Farimani, M., Mirzania, F., Sonboli, A., and Moghaddam, F. M. (2017). Chemical composition and antibacterial activity of Dracocephalum kotschyi essential oil obtained by microwave extraction and hydrodistillation. Int. J. Food Prop. 20 (Suppl. 1), 306–315. doi:10.1080/10942912.2017.1295987
Morshedloo, M. R., Amani Machiani, M., Mohammadi, A., Maggi, F., Aghdam, M. S., Mumivand, H., et al. (2020). Comparison of drying methods for the extraction of essential oil from dragonhead (Dracocephalum moldavica L., Lamiaceae). J. Essent. Oil Res. 33, 162–170. doi:10.1080/10412905.2020.1848652
Nie, L., Li, R., Huang, J., Wang, L., Ma, M., Huang, C., et al. (2021). Abietane diterpenoids from Dracocephalum moldavica L. and their anti-inflammatory activities in vitro. Phytochemistry 184, 112680. doi:10.1016/j.phytochem.2021.112680
Olennikov, D. N., Chirikova, N. K., Kashchenko, N. I., Gornostai, T. G., Selyutina, I. Y., and Zilfikarov, I. N. (2017). Effect of low temperature cultivation on the phytochemical profile and bioactivity of arctic plants: a case of Dracocephalum palmatum. Int. J. Mol. Sci. 18 (12), 2579. doi:10.3390/ijms18122579
Olennikov, D. N., Chirikova, N. K., Okhlopkova, Z. M., and Zulfugarov, I. S. (2013). Chemical composition and antioxidant activity of Tánara Ótó (Dracocephalum palmatum Stephan), a medicinal plant used by the North-Yakutian nomads. Molecules 18 (11), 14105–14121. doi:10.3390/molecules181114105
Pan, H., Le, M., He, C., Yang, C. S., and Ling, T. (2022). Dark tea: a popular beverage with possible medicinal application. Chin. Herb. Med. 15 (1), 33–36. doi:10.1016/j.chmed.2022.08.005
Paravar, A., Maleki Farahani, S., and Rezazadeh, A. (2023). Morphological, physiological and biochemical response of Lallemantia species to elevated temperature and light duration during seed development. Heliyon 9 (4), e15149. doi:10.1016/j.heliyon.2023.e15149
Poursalavati, A., Rashidi-Monfared, S., and Ebrahimi, A. (2021). Toward understanding of the methoxylated flavonoid biosynthesis pathway in Dracocephalum kotschyi Boiss. Sci. Rep. 11 (1), 19549. doi:10.1038/s41598-021-99066-6
Quan, Y. N., Wang, X. C., Guo, X. H., Yuan, Y., and Cheng, J. (2017). The effect of total flavones of dracocephalum moldavica on atherosclerosis lesions of apolipoprotein E gene deficient mice. Chin. J. Arter. 25 (09), 874–878. Available at: http://www.dmzzbjb.net/zgdmyhzzen/article/abstract/20170902.
Sabrin, M. S., Selenge, E., Takeda, Y., Batkhuu, J., Ogawa, H., Jamsransuren, D., et al. (2021). Isolation and evaluation of virucidal activities of flavanone glycosides and rosmarinic acid derivatives from Dracocephalum spp. against feline calicivirus. Phytochemistry 191, 112896. doi:10.1016/j.phytochem.2021.112896
Sadraei, H., Asghari, G., Khanabadi, M., and Minaiyan, M. (2017). Anti-inflammatory effect of apigenin and hydroalcoholic extract of Dracocephalum kotschyi on acetic acid-induced colitis in rats. Res. Pharm. Sci. 12 (4), 322–329. doi:10.4103/1735-5362.212050
Saeidnia, S., Gohari, A. R., Uchiyama, N., Ito, M., Honda, G., and Kiuchi, F. (2004). Two new monoterpene glycosides and trypanocidal terpenoids from Dracocephalum kotschyi. Chem. Pharm. Bull. 52 (10), 1249–1250. doi:10.1248/cpb.52.1249
Saren, G. W., and Saren, G. L. R. (2002). Pharmacognosy study of Mongolian medicine Ergun-Briyanggu. J. Med. and Pharm. Chin. Minorities 02, 33. doi:10.16041/j.cnki.cn15-1175.2002.02.030
Selenge, E., Murata, T., Tanaka, S., Sasaki, K., Batkhuu, J., and Yoshizaki, F. (2014). Monoterpene glycosides, phenylpropanoids, and acacetin glycosides from Dracocephalum foetidum. Phytochemistry 101, 91–100. doi:10.1016/j.phytochem.2014.02.007
Selvaraj, S., and Gurumurthy, K. (2022). An overview of probiotic health booster-kombucha tea. Chin. Herb. Med. 15 (1), 27–32. doi:10.1016/j.chmed.2022.06.010
Semerdjieva, I. B., Burducea, M., Astatkie, T., Zheljazkov, V. D., and Dincheva, I. (2019). Essential oil composition of ruta graveolens L. Fruits and hyssopus officinalis subsp. aristatus (godr.) nyman biomass as a function of hydrodistillation time. Molecules 24 (22), 4047. doi:10.3390/molecules24224047
Shamyrina, A. A., Peshkova, V. A., and Shergina, N. I. (1975). Flavonoids of dracocephalum nutans. Chem. Nat. Compd. 11 (2), 262. doi:10.1007/BF00570691
Sharafi, A., Sohi, H. H., Azadi, P., and Sharafi, A. A. (2014). Hairy root induction and plant regeneration of medicinal plant Dracocephalum kotschyi. Physiology Mol. Biol. plants 20 (2), 257–262. doi:10.1007/s12298-013-0217-z
Sharifi-Rad, J., Quispe, C., Kumar, M., Akram, M., Amin, M., Iqbal, M., et al. (2022). Hyssopus essential oil: an update of its phytochemistry, biological activities, and safety pofile. Oxid. Med. Cell Longev. 2022, 8442734. doi:10.1155/2022/8442734
Shomirzoeva, O., Li, J., Numonov, S., Atolikshoeva, S., and Aisa, H. A. (2020). Chemical components of Hyssopus cuspidatus Boriss.: isolation and identification, characterization by HPLC-DAD-ESI-HRMS/MS, antioxidant activity and antimicrobial activity. Nat. Prod. Res. 34 (4), 534–540. doi:10.1080/14786419.2018.1488710
Sokolov, P. D. (1991). “Plant resources of ussr. Flowering plants, its chemical composition, application,” in Families hippuridaceae-lobeliaceae (St-Petersburg: Nauka), 19–24.
Su, Y. W., Chao, S. H., Lee, M. H., Ou, T. Y., and Tsai, Y. C. (2010). Inhibitory effects of citronellol and geraniol on nitric oxide and prostaglandin E₂production in macrophages. Planta Med. 76 (15), 1666–1671. doi:10.1055/s-0030-1249947
Suto, Y., Nakajima-Shimada, J., Yamagiwa, N., Onizuka, Y., and Iwasaki, G. (2015). Synthesis and biological evaluation of quinones derived from natural product komaroviquinone as anti-Trypanosoma cruzi agents. Bioorg. and Med. Chem. Lett. 25 (15), 2967–2971. doi:10.1016/j.bmcl.2015.05.022
Tahir, M., Khushtar, M., Fahad, M., and Rahman, M. A. (2018). Phytochemistry and pharmacological profile of traditionally used medicinal plant Hyssop (Hyssopus officinalis L.). J. Appl. Pharm. Sci. 8 (7), 132–140. doi:10.7324/JAPS.2018.8721
Tashi, T., Cidan, , Zhao, X., Tenzin, Z., Sangji, D., Dawa, D., et al. (2022). The study on the herbology of the traditional Tibetan medicinal herbs dracocephalum tanguticum. J. Med. Pharm. Chin. Minorities 28 (1), 43–46. doi:10.16041/j.cnki.cn15-1175.2022.01.021
Tetali, S. D. (2019). Terpenes and isoprenoids: a wealth of compounds for global use. Planta 249 (1), 1–8. doi:10.1007/s00425-018-3056-x
Tholl, D. (2015). Biosynthesis and biological functions of terpenoids in plants. Adv. Biochem. engineering/biotechnology 148, 63–106. doi:10.1007/10_2014_295
Toshmatov, Z. O., Li, J., Eshbakova, K. A., Tang, D., Xin, X. L., and Aisa, H. A. (2019). New monoterpene glucosides from Dracocephalum komarovi and their anti-inflammatory activity. Phytochem. Lett. 33, 102–105. doi:10.1016/j.phytol.2019.08.005
Uchiyama, N., Kiuchi, F., Ito, M., Honda, G., Ashurmetov, O. A., Khodzhimatov, O. K., et al. (2006). Trypanocidal constituents of dracocephalum komarovi. Tetrahedron 62 (18), 4355–4359. doi:10.1016/j.tet.2006.02.067
Uchiyama, N., Kiuchi, F., Ito, M., Honda, G., Takeda, Y., Khodzhimatov, O. K., et al. (2003). New icetexane and 20 norabietane diterpenes with trypanocidal activity from Dracocephalum komarovi. J. Nat. Prod. 66 (1), 128–131. doi:10.1021/np020308z
Wang, F., Dong, P. Z., Pei, X. P., and Lian, Y. L. (2022). Research progress of dracocephalum rupestre hance. Chin. J. Ethnomedicine Ethnopharmacy 31 (22), 50–55. Available at: https://kns.cnki.net/kcms2/article/abstract?v=su5nt4ZpZVTbTPlaqthWfyAKAjaW1vEVwDeAZukeX5b7AZaDw_VucMCxKn9XiUYG9MVo9Rx-ziRDqQTlEoHwSaK65IZVczAL-Ay-ZJvv6Y2fbZgLl3ujGLsdO0AR_lZzrckxJ0ySBDMSbVje7lI9pn5YsSBD4M7WeR2wI1odJ9L1PTJZgXyjo9kZdNVve9wAAeBA292bjwA=&uniplatform=NZKPT&language=CHS.
Wang, S. Q., Ren, D. M., Xiang, F., Wang, X. N., Zhu, C. J., Yuan, H. Q., et al. (2009). Dracotanosides A-D, spermidine glycosides from Dracocephalum tanguticum: structure and amide rotational barrier. J. Nat. Prod. 72 (6), 1006–1010. doi:10.1021/np900140s
Wang, Z., Linghu, K. G., Hu, Y., Zuo, H., Yi, H., Xiong, S. H., et al. (2019). Deciphering the pharmacological mechanisms of the huayu-qiangshen-tongbi formula through integrating network pharmacology and in vitro pharmacological investigation. Front. Pharmacol. 10, 1065. doi:10.3389/fphar.2019.01065
Weber, L. W. D., Boll, M., and Stampfl, A. (2003). Hepatotoxicity and mechanism of action of haloalkanes: carbon tetrachloride as a toxicological model. Crit. Rev. Toxicol. 33 (2), 105–136. doi:10.1080/713611034
Wen, J., Zhang, J. Q., Nie, Z. L., Zhong, Y., and Sun, H. (2014). Evolutionary diversifications of plants on the Qinghai-Tibetan plateau. Front. Genet. 5, 4. doi:10.3389/fgene.2014.00004
Weremczuk-Jeżyna, I., Lisiecki, P., Gonciarz, W., Kuźma, Ł., Szemraj, M., Chmiela, M., et al. (2020). Transformed shoots of dracocephalum forrestii W.W. Smith from different bioreactor systems as a rich source of natural phenolic compounds. Mol. 25 (19), 4533. doi:10.3390/molecules25194533
Xing, J. H., Zhao, A. G., Bao, F. G., and Liu, Q. L. (2021). Stnuy on standardized planting technology of Mongolian medicine Cymbidium Mongolica. J. North Pharm. 18 (8), 1–3. Available at: https://kns.cnki.net/kcms2/article/abstract?v=su5nt4ZpZVRRszG34RD52N9uWqtK17QGNybih09YAKSLjAujQPlxL2vefCI8JGGMPkOLQ8265bei_vm6y2D9XG5xrnV99cTULghU-oDh3lUnT9NVouzB_d3vTXNtoOCFNyutE3Bkzckb3dZVLFu9o-L59nJhvckmm5X86NztMooI7iVR9h5-FJWSv4vMI0wHlfkvS3nyxXk=&uniplatform=NZKPT&language=CHS.
Xiong, X., Ren, Y., Cui, Y., Li, R., Wang, C., and Zhang, Y. (2017). Obeticholic acid protects mice against lipopolysaccharide-induced liver injury and inflammation. Biomed. Pharmacother. 96, 1292–1298. doi:10.1016/j.biopha.2017.11.083
Yamaguchi, T. (2022). Antibacterial effect of the combination of terpenoids. Archives Microbiol. 204 (8), 520. doi:10.1007/s00203-022-03142-y
Yan, Y., Liu, X., Gao, J., Wu, Y., and Li, Y. (2020). Inhibition of TGF-β signaling in gliomas by the flavonoid diosmetin isolated from dracocephalum peregrinum L. Molecules 25 (1), 192. doi:10.3390/molecules25010192
Yan, Y. L. (2020). “Chemical constituents extraction and antitumor activity of dracocephalum peregrinum linn,”. [Doctoral dissertation] (Northeast Normal University), 1–2. doi:10.27011/d.cnki.gdbsu.2020.001747
Yang, J., Wu, Y., Zhang, P., Ma, J., Yao, Y. J., Ma, Y. L., et al. (2023). Multiple independent losses of the biosynthetic pathway for two tropane alkaloids in the Solanaceae family. Nat. Commun. 14 (1), 8457. doi:10.1038/s41467-023-44246-3
Yao, J., Zhao, F., Xu, Y., Zhao, K., Quan, H., Su, Y., et al. (2020). Complete chloroplast genome sequencing and phylogenetic analysis of two dracocephalum plants. Biomed. Res. Int. 2020, 4374801. doi:10.1155/2020/4374801
Yao, R., He, C., and Xiao, P. (2022). Food and medicine continuum' in the East and West: old tradition and current regulation. Chin. Herb. Med. 15 (1), 6–14. doi:10.1016/j.chmed.2022.12.002
Yilmaz Kardas, B., Diken, M. E., Bayhan, H., Acar, M., and Dogan, S. (2023). Cytoprotective, antimutagenic/antirecombinogenic and antibacterial properties of Lallemantia iberica extracts. J. Sci. food Agric. 103 (4), 1901–1911. doi:10.1002/jsfa.12257
Zamani, S. S., Hossieni, M., Etebari, M., Salehian, P., and Ebrahimi, S. A. (2016). Pharmacokinetics of calycopterin and xanthmicrol, two polymethoxylated hydroxyflavones with anti-angiogenic activities from Dracocephalum kotschyi Bioss. Daru J. Fac. Pharm. Tehran Univ. Med. Sci. 24 (1), 22. doi:10.1186/s40199-016-0161-x
Zeng, Q., Chang, R., Qin, J., Cheng, X., Zhang, W., and Jin, H. (2011). New glycosides from Dracocephalum tanguticum maxim. Archives pharmacal Res. 34 (12), 2015–2020. doi:10.1007/s12272-011-1202-0
Zhang, C., Li, H., Yun, T., Fu, Y., Liu, C., Gong, B., et al. (2008). Chemical composition, antimicrobial and antioxidant activities of the essential oil of Tibetan herbal medicine Dracocephalum heterophyllum Benth. Nat. Prod. Res. 22 (1), 1–11. doi:10.1080/14786410701619076
Zhang, G. Y., Ling, J. Y., and Cui, Z. J. (2007). Supercritical CO2 extraction of essential oil from Dracocephalum tanguticum Max-im and analysis by GC-MS. J. Liq. Chromatogr. Relat. Technol. 30 (2), 287–292. doi:10.1080/10826070601064607
Zhang, H., Wang, S., Liu, Q., Zheng, H., Liu, X., Wang, X., et al. (2021). Dracomolphin A-E, new lignans from Dracocephalum moldavica. Fitoterapia 150, 104841. doi:10.1016/j.fitote.2021.104841
Zhang, H. H., Meng, X. X., Liu, Y. L., Chen, S. L., and Huang, L. F. (2023). Prediction of global potential growth areas for Panax ginseng based on GMPGIS system and MaxEnt model. China J. Chin. Materia Medica 48 (18), 4959–4966. doi:10.19540/j.cnki.cjcmm.20230514.102
Zhang, J. L., Yan, R. j., Yu, N., Zhang, X., Chen, D. J., Wu, T., et al. (2018). A new caffeic acid tetramer from the Dracocephalum moldavica L. Nat. Prod. Res. 32, 370–373. doi:10.1080/14786419.2017.1359168
Zhang, M., Lei, J., Wang, Y., Zhang, J., and Liu, D. (2022). Ethnopharmacology, phytochemistry and pharmacology of Benincasae Exocarpium: a review. Chin. Herb. Med. 15 (1), 15–26. doi:10.1016/j.chmed.2022.10.001
Zhang, M. L., Zeng, X. Q., Sanderson, S. C., Byalt, V. V., and Sukhorukov, A. P. (2017). Insight into central asian flora from the cenozoic tianshan montane origin and radiation of lagochilus (Lamiaceae). PloS one 12 (9), e0178389. doi:10.1371/journal.pone.0178389
Zhang, X. F., Hu, B. L., and Wang, S. X. (1994). The chemical constituents from Dracocephalum tanguticum Maxim. Acta Bot-anica Sin. 08, 645–648.
Zhang, X. P., Shao, J. J., Ma, D. L., Liu, F., LiuM, M., and Cui, Q. H. (2019). Research on antitumor active components and mechanisms of natural products. Acta Pharm. Sin. 54 (11), 1949–1957. doi:10.16438/j.0513-4870.2019-0669
Zheng, W., Wang, Q., Lu, X., Shi, Q., Zou, J., Tao, Y., et al. (2016). Protective effects of dracocephalum heterophyllum in ConA-induced acute hepatitis. Mediat. Inflamm. 2016, 2684321. doi:10.1155/2016/2684321
Zhou, S., Wei, C., Zhang, C., Han, C., Kuchkarova, N., and Shao, H. (2019). Chemical composition, phytotoxic, antimicrobial and insecticidal activity of the essential oils of dracocephalum integrifolium. Toxins 11 (10), 598. doi:10.3390/toxins11100598
Zhu, C. S., Liu, K., Wang, J. L., Li, J. F., Liu, M. F., Hao, N., et al. (2018). Antioxidant activities and hepatoprotective potential of Dracocephalum rupestre Hance extract against CCl4-induced hepatotoxicity in Kunming mice. J. Food Biochem. 42 (2), e12484. doi:10.1111/jfbc.12484
Keywords: Dracocephalum, pharmacophylogeny, geographical distribution, plant metabolites, pharmacological activities
Citation: Liu H, Feng X, Zhao Y, Lv G, Zhang C, Aruhan , Damba T-A, Zhang N, Hao D and Li M (2024) Pharmacophylogenetic relationships of genus Dracocephalum and its related genera based on multifaceted analysis. Front. Pharmacol. 15:1449426. doi: 10.3389/fphar.2024.1449426
Received: 15 June 2024; Accepted: 09 September 2024;
Published: 03 October 2024.
Edited by:
Chunnian He, Chinese Academy of Medical Sciences and Peking Union Medical College, ChinaCopyright © 2024 Liu, Feng, Zhao, Lv, Zhang, Aruhan, Damba, Zhang, Hao and Li. This is an open-access article distributed under the terms of the Creative Commons Attribution License (CC BY). The use, distribution or reproduction in other forums is permitted, provided the original author(s) and the copyright owner(s) are credited and that the original publication in this journal is cited, in accordance with accepted academic practice. No use, distribution or reproduction is permitted which does not comply with these terms.
*Correspondence: Minhui Li, cHJvZl9saW1pbmh1aUB5ZWFoLm5ldA==; Dacheng Hao, aGFvQGRqdHUuZWR1LmNu