- 1The First Hospital of Hunan University of Chinese Medicine, Changsha, China
- 2Hunan University of Chinese Medicine, Changsha, China
Heart failure (HF) has a severe impact on public health development due to high morbidity and mortality and is associated with imbalances in cardiac immunoregulation. Macrophages, a major cell population involved in cardiac immune response and inflammation, are highly heterogeneous and polarized into M1 and M2 types depending on the microenvironment. M1 macrophage releases inflammatory factors and chemokines to activate the immune response and remove harmful substances, while M2 macrophage releases anti-inflammatory factors to inhibit the overactive immune response and promote tissue repair. M1 and M2 restrict each other to maintain cardiac homeostasis. The dynamic balance of M1 and M2 is closely related to the Traditional Chinese Medicine (TCM) yin-yang theory, and the imbalance of yin and yang will result in a pathological state of the organism. Studies have confirmed that TCM produces positive effects on HF by regulating macrophage polarization. This review describes the critical role of macrophage polarization in inflammation, fibrosis, angiogenesis and electrophysiology in the course of HF, as well as the potential mechanism of TCM regulation of macrophage polarization in preventing and treating HF, thereby providing new ideas for clinical treatment and scientific research design of HF.
1 Introduction
Heart failure (HF) is the end stage of various heart diseases in which functional and/or structural abnormalities of the heart result in impaired ventricular filling and/or ejection capacity. The main clinical signs and symptoms are caused by stasis in the pulmonary and physical circulation and reduced cardiac output (Bozkurt et al., 2021; Heidenreich et al., 2022; McDonagh et al., 2024). The development of the disease is associated with a synergistic interplay of multiple mechanisms, including dysregulation of the neurohumoral system, oxygen-free radical burst, mitochondrial dysfunction, autophagy, apoptosis, and inflammatory response (Kleinbongard et al., 2010; Dick and Epelman, 2016; Antoine et al., 2017; van der Pol et al., 2019; Yamaguchi, 2019). With high morbidity and mortality rates, HF affects more than 64 million people worldwide and has become a significant public health problem worldwide (Savarese et al., 2023). Drug therapy is the primary treatment for HF, and more and more national HF guidelines recommend quadruple therapy as the primary drug therapy for HF, which can significantly improve the prognosis of patients with HF and open up a “new era” of HF treatment (Vaduganathan et al., 2020; Tromp et al., 2022). However, these medications still have many challenges and limitations, with many adverse effects and recurrent episodes of the disease when taken for an extended period. Traditional Chinese medicine (TCM), with its multi-target and multi-pathway characteristics, has good clinical efficacy in treating HF, especially in regulating the body balance and reducing side effects (Li et al., 2013; Liu et al., 2014).
HF activates the immune system regardless of the factor causing it (Torre-Amione, 2005; Bacmeister et al., 2019). Macrophages are an essential component of the immune system, and activated macrophages produce a variety of cytokines, chemokines, and enzymes that modulate the inflammatory response and promote tissue injury or repair (Murray and Wynn, 2011). After myocardial damage, the local release of chemokines in the tissue promotes the increase of the number of macrophages and differentiation into different phenotypes, thus playing various functions. The characteristics of M1 and M2 macrophage subsets have been well defined. M1 macrophages are pro-inflammatory cells, and M2 macrophages have anti-inflammatory effects (Shapouri-Moghaddam et al., 2018; Zhang H. et al., 2021). Studies have shown that M1 and M2 macrophages can be transformed into each other under different conditions during the course of HF, and the dynamic balance between the two is closely related to tissue damage and repair (Halade et al., 2018; Lv et al., 2020). Therefore, regulating the balance between the two types of macrophages may become essential for treating HF. This article will review the mechanism of macrophage polarization in HF and the research progress of TCM in regulating macrophage polarization for treating HF to provide a new therapeutic strategy.
2 Macrophage polarization and mechanisms
2.1 Origin and function of macrophages
Macrophages are widely distributed in various body tissues and include monocyte-derived macrophages and tissue-resident macrophages (TRMs) (Moreira Lopes et al., 2020). TRMs are derived from erythroid myeloid progenitors of the yolk sac and fetal monocyte progenitors and are replaced by renewal through in situ proliferation when the organism is in a stable state (Epelman et al., 2014; Gentek et al., 2014). When tissue inflammation or injury occurs, TRMs are consumed in large numbers, and monocytes migrate from the periphery to the tissue, acquire the ability to synthesize and secrete inflammatory mediators, and transform into macrophages that play a role in tissue injury (Jakubzick et al., 2017). The main functions of macrophages are pathogen clearance and antigen presentation. TRMs in different tissues have some functional heterogeneity. For example, osteoclasts in bone tissues have bone remodeling effects, and TRMs in the heart play a crucial role in the formation and development of cardiac tissue and blood vessels (Suzuki et al., 2020; Zaman et al., 2021).
2.2 Macrophage polarization
Macrophages are highly plastic and differentiate into different subtypes of macrophages with diverse functions under various microenvironments, a process known as macrophage polarization (Yunna et al., 2020). The current paradigm describes two macrophage subpopulations: classically activated M1 macrophages and alternatively activated M2 macrophages (Ruytinx et al., 2018).
2.2.1 Phenotype and function of M1 macrophages
M1 macrophages highly express surface protein markers such as CD16, CD32, CD40, CD68, CD80, CD86, F4/80 h and TRL-4 (Yao et al., 2019; Binatti et al., 2022). Pathogen-associated molecular patterns (PAMPs) such as lipopolysaccharide (LPS), interferon-gamma (INF-γ), tumor necrosis factor-α (TNF-α), and granulocyte-macrophage colony-stimulating factor activate intracellular downstream signaling pathways by binding to different receptors, which promotes M1 macrophage polarization, secretion, and proliferation (Brown et al., 2012). M1 macrophages secrete a large number of pro-inflammatory factors, such as IL-1β, TNF-α, and INF-γ, and chemokines (like chemokine CXC-motif ligand9 (CXCL9), CXCL10, CXCL11, and CXCL16). Simultaneously, immune stress increases reactive oxygen species (ROS) and inducible nitric oxide synthase (iNOS) levels, and the immune response of Th1 and Th17 cells is markedly increased. These responses have pro-inflammatory, anti-tumor, and antigen-presenting effects (Yunna et al., 2020; Tsai et al., 2021; Zhou et al., 2021).
2.2.2 Phenotype and function of M2 macrophages
M2 macrophages are anti-inflammatory and can be polarized into four subtypes: M2a, M2b, M2c and M2d. M2a macrophages are stimulated by IL-4 and IL-13, which express high levels of the CD206 and IL-1Ⅱreceptors. M2a secrete anti-inflammatory substances (such as IL-10, chemokine CC-motif ligand17 (CCL17), CCL18, CCL22, CCL24) and pro-fibrotic factors (TGF-β, insulin-like growth factor (IGF), and fibronectin) reduces chronic inflammation and promotes tissue regeneration and wound healing (Wang et al., 2019). M2b macrophages are mainly induced by immune complexes (ICs) and IL-1β, with high expression of protein markers such as CD86, IL-10R, IL-12R, and tumor necrosis factor superfamily member 14 (TNFSF14). M2b secretes pro-inflammatory factors, including IL-1β, IL-6, and TNF-α, and anti-inflammatory factors like IL-10. Because they have both pro-inflammatory and anti-inflammatory effects, M2b is also called a regulatory macrophage (Mosser and Edwards, 2008). M2c is induced by IL-10, TGF-β and glucocorticoids and is characterized by a high expression of CD163 and CD206 on the cell surface. M2c secretes IL-10 and TGF-β to inhibit inflammation and promote tissue repair, and at the same time, regulates T cells through the secretion of chemokines, such as CCL16 and CCL18, which exert a phagocytic effect on apoptotic cells (Tian L. et al., 2022). M2d, also known as tumor-associated macrophages, can be induced by a combination of TLRs and adenosine receptor agonists or by IL-6 alone and marked by proteins such as IL-10R and IL-12R. M2d secretes IL-10, vascular endothelial growth factor (VEGF), a major inflammatory factor in the tumor environment, promoting angiogenesis and contributing to tumor growth (Wang et al., 2019).
2.3 Molecular mechanisms of macrophage polarization
2.3.1 TLRs signaling pathway
Toll-like receptors (TLRs) play a crucial role in regulating macrophage polarization, with TLR4 being the most closely related to M1 macrophages. CD14, a membrane glycoprotein with glycosylated inositol (GPI), enhances the TLR4 signaling pathway, in addition, medullary differentiation protein 2 (MD-2) promotes TLR4 translocation (Park and Lee, 2013). LPS binds to LPS-binding protein (LBP) and recognizes a heterotrimer of CD14/TLR4/MD-2, which activates different downstream signaling pathways such as the myeloid differentiation primary response protein 88 (MyD88) pathway and the TIR structural domain-containing articulators inducing interferon-β (TRIF) signaling pathway (Ciesielska et al., 2021).
MyD88 binds to IL-1 receptor-associated kinase (IRAK) to form a complex (Ciesielska et al., 2021). Subsequently, the complex further interacts with tumor necrosis factor receptor-associated factor 6 (TRAF6), triggering a signaling cascade reaction with TAK1 kinase, which activates inhibitor kappa B kinase α/β (IKK α/β), leading to nuclear translocation of NF-κB transcription factors (Hawiger et al., 1999; Takaesu et al., 2000). The TRIF pathway activated by TLR4 first induces the activation of the ubiquitin ligase TRAF3, followed by the activation of tank-binding kinase 1 (TBK1) and IKKε. After TBK1 phosphorylates the pLxlS consensus motif of TRIF, interferon regulatory factor 3 (IRF3) is recruited. Nuclear translocation occurs after TBK1 phosphorylates IRF3 (Liu et al., 2015). After entering the nucleus, NF-κB and IRF3 bind to target gene binding sites, initiate and regulate the expression of inflammatory factors such as IL-1β and TNF-α, and promote M1 macrophage polarization (Ryu et al., 2022; Mussbacher et al., 2023).
2.3.2 JAK/STAT signaling pathway
Activation of the JAK/STAT signaling pathway regulates macrophage polarization. Ligands bind to corresponding macrophage receptors to form dimers, which recruit and phosphorylate JAK. Activated JAK causes tyrosine phosphorylation of bound receptors to form docking sites for STATs. Subsequently, STATs detach from the receptor and form homologous dimers through SH2 domain-phosphotyrosine interactions. The STATs dimer then undergoes nuclear translocation and binds to relevant target genes to induce macrophage polarization (Ge et al., 2021; Hu et al., 2021). The STAT family consists of seven members, of which STAT1 promotes macrophage polarization to the M1, and STAT3 and STAT6 mediate macrophage conversion to the M2.
The JAK/STAT1 pathway activation by IFN-γ is crucial for M1 macrophage polarization. Unlike IFN-γ, IFN-α/β activated the suppressor of cytokine signaling factor 3 (SOCS3), which prevented STAT1 phosphorylation and M1 polarization (Lawrence and Natoli, 2011). IL-4 activates the JAK/STAT6 pathway, and phosphorylated STAT6 can directly bind to KLF4 and PPAR-γ to mediate M2 polarization by initiating gene transcription (Gong et al., 2017; Chen et al., 2022). In addition, STAT6 increases the expression of the histone demethylase Jmjd3, which demethylates the lysine at position 27 of histone H3 and then acts on the transcription factor interferon regulatory factor 4 (IRF4) to mediate M2 polarization (Ming-Chin Lee et al., 2022). IL-10 is an essential immunosuppressive factor that promotes M2 polarization by activating the JAK/STAT3 pathway (Duncan et al., 2020).
2.3.3 PI3K/Akt signaling pathway
The PI3K/Akt signaling pathway is crucial for macrophage survival, proliferation, migration, and polarization. Signaling factors such as TLR4 and other pathogen recognition receptors, cytokines and chemokines, and Fc receptors activate class I PI3K to convert the second messenger phosphatidylinositol 4,5-diphosphate (PIP2) to phosphatidylinositol 4,5-triphosphate (PIP3) at the plasma membrane. PIP3 attracts Akt and rapamycin complex 2 (mTORC2) and facilitates the activation of Akt by mTORC2 (Vergadi et al., 2017). Activated Akt then activates mTORC1 by inhibiting tuberous sclerosis complex 1/2 (TSC1/2) (Covarrubias et al., 2015).
Macrophages have three distinct Akt subtypes: Akt1, Akt2, and Akt3. It has been observed that Akt1 and Akt2 have opposing effects on regulating macrophage polarization (Arranz et al., 2012). The activation of the PI3K/Akt1 pathway is considered a negative regulator of TLR and NF-kB signaling (Fukao and Koyasu, 2003). On the one hand, the PI3K/Akt1 pathway can upregulate the TLR4 signaling repressor IRAK-M by mediating the inactivation of TRAF6, thereby inhibiting TLR4 target genes and encouraging macrophage polarization toward M2. On the other hand, Akt1 can prevent NF-κB from nuclear transcription, which leads to M2 polarization (Kobayashi et al., 2002; Fan et al., 2010; Tian Y. et al., 2022). Oppositely, Akt2 can facilitate the polarization of macrophages to the M1 by promoting the nuclear translocation of NF-κB, while Akt2 deletion enhances the M2 macrophage phenotype (Babaev et al., 2014; Yin et al., 2023). As a downstream target of PI3K/Akt, mTORC also contributes to macrophage polarization. Studies on how mTORC1 regulates macrophage polarization show contradictory results, possibly due to different environmental activation conditions (Byles et al., 2013; Kimura et al., 2016). In contrast, the mechanism of mTORC2 is relatively straightforward, as it promotes M2 polarization by inducing the transcription factor IRF4 (Huang et al., 2016).
2.3.4 JNK signaling pathway
The JNK signaling pathway is crucial for the polarization and activation of macrophages (Zha et al., 2021). The JNK family contains three isoforms: JNK1, JNK2, and JNK3. Activated JNK controls target gene transcription and expression using downstream substrates such as AP-1 (Koga et al., 2019).
Activation of the JNK signaling pathway is a crucial step in initiating chronic low-grade inflammation (Park and Han, 2024). LPS activates JNK through TLR4/MyD88 signaling and promotes the activation of its downstream substrates, thereby mediating the polarization of M1 macrophages and producing inflammatory factors to promote an inflammatory response (Liu et al., 2018). Furthermore, JNK phosphorylates serine 707 to inactivate STAT6, which causes M1 polarization (Shirakawa et al., 2011). JNK is inhibited by IL-4 and IL-13, leading to macrophage polarization toward M2 and facilitating tumor development, angiogenesis, and metastasis (Cheng et al., 2023).
2.3.5 Notch signaling pathway
Notch receptors are highly conserved throughout evolution, participate in cell growth and development and maintain tissue homeostasis. The mammalian Notch signaling pathway is mainly activated by interactions between three delta-like ligands (DLL1, DLL3, and DLL4), two Jagged family ligands (JAG1 and JAG2), and four transmembrane receptors (Notch1-4) (Eun and Jeong, 2016). Recent studies have shown that the Notch signaling pathway is associated with macrophage activation and polarization (Vieceli Dalla Sega et al., 2019). The precise mechanism is that the intracellular portion (NICD) of Notch is released from the medial membrane into the nucleus following its binding to the ligand, owing to the actions of disintegrin metalloproteinase (ADAM) and gamma-secretase. NICD binds to recombinant signal binding protein-J (RBP-J) to promote M1 polarization. The Notch and TLRs pathways are integrated at the level of IRF8 protein synthesis. Xu et al. showed that the Notch pathway activates TLR4, which induces IRF8 expression and promotes M1 macrophage polarization through the IRAK2-MNK1-elF4E pathway (Xu et al., 2012).
The regulatory mechanisms of M1 and M2 macrophage polarization are detailed in Figures 1, 2.
3 Role of macrophage polarization in HF
Multiple complex physiopathologic processes accompany HF. Macrophages are the most numerous immune cells in the heart and are crucial for both preserving cardiac homeostasis and organizing tissue damage healing. After cardiac tissue is damaged, monocytes are recruited from the bone marrow and spleen to the injury site and differentiate into macrophages, which are involved in the various phases of inflammation, repair and remodeling of myocardial injury (Nahrendorf et al., 2007; Swirski et al., 2009). This article will discuss the role of macrophage polarization in HF from the following aspects. The detailed regulatory mechanisms are shown in Figure 3.
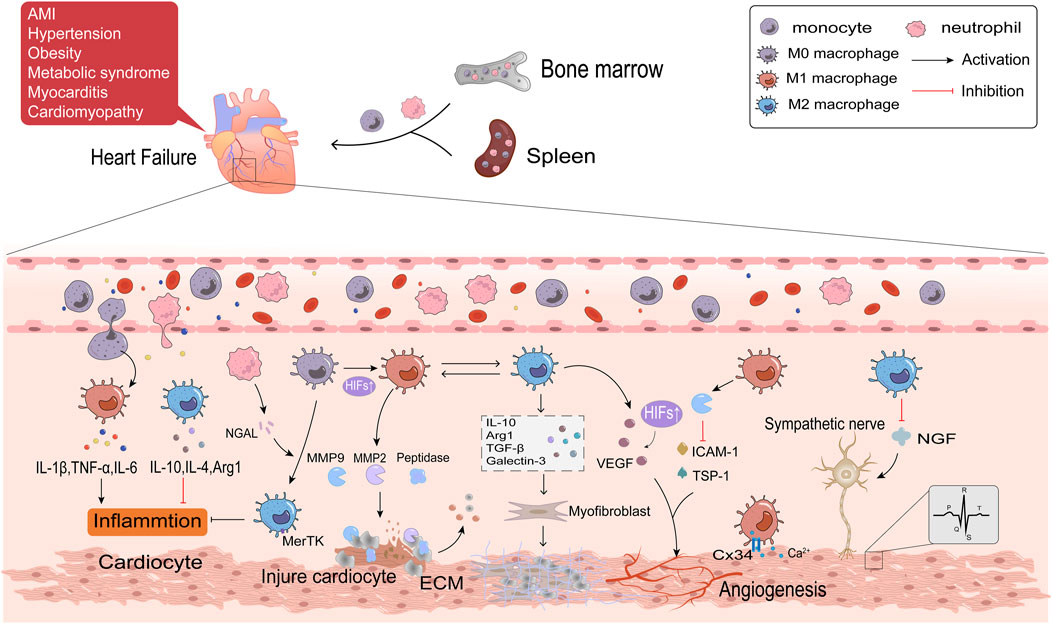
Figure 3. The regulatory mechanisms of macrophage polarization in inflammation, fibrosis, angiogenesis and cardiac electrophysiology of HF.
3.1 Macrophage polarization and inflammation in HF
Acute myocardial infarction (AMI) is the primary cause of HFrEF. Ischemia and subsequent reperfusion injury trigger acute inflammatory responses, which permanently damage cardiomyocytes. Obesity, hypertension, and metabolic syndromes are frequent causes of HFpEF. These diseases can all result in persistent systemic inflammation, which damages cardiomyocytes by producing pro-inflammatory cytokines. Circulating levels of inflammatory mediators have been connected with HF mortality (Mouton et al., 2020). Inflammation also is the primary cause of HF in patients with inflammatory cardiomyopathy or myocarditis (Hanna and Frangogiannis, 2020). This implies that HF is triggered by whatever pathological changes are associated with local and systemic activation of inflammatory signaling cascade responses (Adamo et al., 2020). An initial inflammatory response in HF is triggered by the innate immune response when ischemia and hypoxia cause cardiomyocyte necrosis. This reaction involves the release of danger-associated molecular patterns (DAMPs) such as mitochondrial DNA, activation of complement, and synthesis of inflammatory vesicles (Yang et al., 2015; Rhee and Lavine, 2020). The inflammatory pathway is continuously activated and produces large amounts of inflammatory factors and chemokines, like IL-1, IL-6, TNF-α, and CCL2 (Swirski and Nahrendorf, 2018). Elevated levels of inflammatory factors and chemokines attract neutrophils and monocytes generated in the bone marrow and spleen to travel with the blood to the ischemic myocardium to coordinate the initial inflammatory response and to clear dead cardiomyocytes (Tsou et al., 2007; Zuurbier et al., 2019). When AMI occurs, Ly6Chigh monocytes are the first monocyte population, which differentiate into M1 macrophages, produce protein hydrolases, and secrete matrix metalloproteinases (MMPs) that degrade dead or dying cardiomyocytes and extracellular matrix (ECM) (Nahrendorf et al., 2007; Nahrendorf and Swirski, 2013). The continuous infiltration of immune cells further aggravated oxygen depletion in the infarct area (Van den Bossche et al., 2017). Hypoxia-inducible factors (HIFs) rapidly accumulate in the nucleus of macrophages in response to a hypoxic microenvironment (Zeng et al., 2015). HIFs promotes transcription of the M1 macrophage gene profile and shifts glycolysis to become the primary mode of metabolic energy production in M1 macrophages by driving the expression of the glycolytic gene Slc2al (Fujisaka et al., 2013). Hypoxia stimulates M1 macrophages in the epicardial adipose tissue (EAT) in response to myocardial injury. These macrophages then further penetrate the myocardium and contribute to the exacerbation of cardiac inflammation (Hirata et al., 2011). Researches have indicated that patients with AMI and HFpEF have thicker EAT (Gruzdeva et al., 2018; Horckmans et al., 2018; Gorter et al., 2020). The M2 macrophages predominate in late AMI. In order to support the healing response to AMI, Ly6Clow monocytes are drawn to the myocardium to transform into M2 macrophages (Nahrendorf and Swirski, 2013). Reparative M2c macrophage is also induced by neutrophil gelatinase-associated lipid transport protein (NGAL) (Horckmans et al., 2017). M2c macrophages have a high cytosolic burying capacity and express the Mertk receptor specifically, facilitating tissue healing and inflammation (Wan et al., 2013). Necrotic cardiomyocytes are phagocytosed by macrophages, which provide fatty acids for macrophage mitochondrial respiration and generate NAD + to encourage M2 polarization (Zhang S. et al., 2019). M2 macrophages secrete anti-inflammatory factors such as IL-10, IL-4, and arginase 1 (Arg1), contributing to inflammation regression (Lv et al., 2020). Excessive inflammatory response aggravates a myocardial injury, inhibits tissue repair, promotes adverse LV remodeling, and thus promotes the progression of HF. Therefore, reducing inflammation has become a potent strategy for treating HF (Glezeva et al., 2015). It has been demonstrated that sodium-glucose cotransporter-2 inhibitors (SGLT2i) decrease HF events, particularly HFrEF, perhaps because of their anti-inflammatory properties by lowering plasma glucose and increasing plasma β-hydroxybutyric acid (Bonnet and Scheen, 2018). The β-hydroxybutyric acid promotes the M2 polarization through the STAT6 signaling pathway (Huang et al., 2022).
3.2 Macrophage polarization and myocardial fibrosis
Myocardial fibrosis, a common clinical state in many cardiovascular disorders leading to HF, is characterized by the activation of cardiac fibroblasts into myofibroblasts (Lee et al., 2017). Myofibroblasts generate ECM, a crucial factor in developing myocardial fibrosis (Nielsen et al., 2019; Liu Y. et al., 2020). Macrophages may be a key target for slowing the course of HF because of their bidirectional regulatory effect on ECM. M1 macrophages and Ly6Chigh monocytes secrete a variety of proteases, such as MMP2 and MMP9, to clear cell debris and degrade ECM during the acute phase of AMI. Phagocytosis of these cells is a prerequisite for the subsequent replacement of damaged tissue by granulation tissue (Zaidi et al., 2021). As the Warburg effect proceeds, the accumulated lactic acid in the cell causes histone lactation, which raises the M2 gene in macrophages and completes the transition of M1 to M2 macrophages (Zhang D. et al., 2019). Maintaining a balance between reparative and proinflammatory macrophages is crucial since the delayed conversion of M1 to M2 has been identified as one of the primary causes of adverse ventricular remodeling. Macrophages mainly exhibit the M2 phenotype in the repair stage of AMI, which stimulates fibroblasts, enhances ECM protein synthesis, and promotes collagen deposition by producing a multitude of cytokines, such as TGF-β, IL-10, galectin-3, and Arg1 (Hulsmans et al., 2018; Suthahar et al., 2018; Tengbom et al., 2021; Frangogiannis, 2024). TGF-β1 binds to the type II receptor (TGF-βRⅡ) on the surface of fibroblasts, activates the Smad3 signaling pathway, upregulates the expression of smooth muscle actin (α-SMA), and promotes the transformation of fibroblasts into myofibroblasts in the presence of the fibronectin domain EDA (Serini et al., 1998; Kong et al., 2014). In addition to directly activating fibroblasts, galectin-3 modulates immune-inflammatory responses, angiogenesis, and TGF-β amplification of pro-fibrotic signaling by forming lectin-sugar lattices on the cell surface (Chen et al., 2017; Xu GR. et al., 2020; Wang et al., 2023). Arg1 drives L-arginine metabolism to generate ornithine, polyamines, and proline, consequently contributing to fibroblast proliferation and collagen formation (Bhatta et al., 2017). IL-10 mediates the release of osteopontin (OPN) from macrophages through the STAT3-galectin3 pathway, and OPN activates fibroblasts (Shirakawa et al., 2018). Additionally, it has been demonstrated that IL-10 enhances heart function and myocardial wall compliance after myocardial infarction by lowering the collagen I/III ratio (Shirakawa et al., 2018). M2 macrophages produce IL-10 instantaneously in AMI. However, prolonged activation of M2 macrophages under pressure overload ultimately results in increased left ventricular wall thickness, excessive collagen deposition, and progression of diastolic dysfunction (Hulsmans et al., 2018; Zhang S. et al., 2019). M2 macrophages have the ability to differentiate directly into myofibroblasts or fibroblasts, which will accelerate the course of fibrosis. HAIDER et al. evaluated this macrophage-to-fibroblast transformation by examining typical fibroblast markers in MI mice, including type I collagen (COL1A1), prolyl 4-hydroxylase (P4H), fibroblast activating protein (FAP), and α-SMA (Haider et al., 2019). In addition, macrophages participate in angiotensin Ⅱ (AngⅡ) production by synthesizing renin and angiotensin-converting enzymes in stressed myocardium (Ovchinnikov et al., 2020). AngⅡ is an effective stimulant of myocardial fibroblasts. It has been discovered that the salocorticoid receptor inhibits AngⅡ-induced ventricular fibrosis by regulating the polarization of M2 macrophages (Usher et al., 2010). Therefore, the regulation of macrophage polarization will be significant in treating and preventing cardiac fibrosis.
3.3 Macrophage polarization and cardiac angiogenesis
When the heart is exposed to stressful stimuli such as pressure overload, ischemia, and hypoxia, compensatory responses are induced to maintain cardiac function, including cardiac hypertrophy and increased angiogenesis. Instead, continuous stimulation can result in maladaptive heart growth, such as fibrosis and thinning of blood vessels, which ultimately leads to the development of HF. Angiogenesis refers to the process of vascular endothelial cells forming new blood vessels after activation, proliferation, and migration under the influence of tissue microenvironment. Macrophages are essential regulators of cardiac angiogenesis. M1 macrophages mainly play an inhibitory role in angiogenesis. In the MI model, M1 macrophages eliminate plenty of exosomes, which highly express pro-inflammatory miRNA, such as miR-155. By suppressing Sirt1/AMPKα2-eNO and RAC1-PAK1/2 signaling pathways, M1 macrophages impair the angiogenesis capacity of endothelial cells, aggravate myocardial injury, and delay cardiac repair (Liu S. et al., 2020). Aging is an independent risk factor for HF. Overexpression of MMP9 derived from M1 macrophages decreases angiogenesis-related factors, such as the expression of intercellular adhesion factor (ICAM-1), platelet/endothelial cell molecule-1 (PECAM-1/CD31), and thrombospondin-1 (TSP1) in the left ventricle. This leads to the exacerbation of cardiac hypertrophy in the case of sparse blood vessels and promotes the progression of cardiac aging (Toba et al., 2017). M2 macrophages, as opposed to M1 macrophages, primarily support the development of new blood vessels. Vascular endothelial growth factor (VEGF), responsible for the rapid growth of collateral blood vessels, is released to compensate for ischemia and induce angiogenesis (Toba et al., 2017). M2 macrophages detect changes in the extracellular matrix environment through integrin α5 (Itga5) during the MI repair phase and activate the cascade of adhesion spot kinase and PI3K to upregulate VEGF-A to promote angiogenesis (Li et al., 2023). VEGF is also activated by HIF-1α, which helps restore blood flow to ischemic tissue (Dai et al., 2007). The proliferation of cardioresident macrophages (M2-like macrophages) in the first week of stress overload is essential for cardiac adaptation and function, and this may be linked to KLF4 by upregulating VEGF-A expression and downregulating anti-angiogenic factors like THBS1. In addition, the infiltration of exogenous macrophages (M1-like macrophages) is detrimental to disease development during the cardiac decompensation period (>4 weeks) in this model, and preventing their infiltration improves myocardial angiogenesis (Liao et al., 2018). Angiogenesis is critical to alleviate myocardial ischemia and hypoxia in HF patients and is an essential issue to be solved urgently in clinical.
3.4 Macrophage polarization and cardiac electrophysiology
Arrhythmia is one of the important clinical manifestations of HF, with high morbidity and mortality. The cardiac electrical conduction system is essential for maintaining normal heart rate and function and is a major factor in arrhythmia. Recent studies have demonstrated that macrophages are significant participants in cardiac electrophysiology, acting through various mechanisms, including direct or indirect interactions with other heart cells. Electrical impulses are generated from the SA node and transmitted to the ventricle through the atrioventricular node and conduction pathways. Connexin (Cx) is a significant medium for transmitting electrical signals between cells. Cx in the heart is mainly composed of Cx43, Cx40, Cx45 and Cx30.2, among which Cx43 has the highest content. Cx connects macrophages and cardiac conduction cells to form electrical coupling to regulate the electrophysiological activity of cardiomyocytes (Sugita and Fujiu, 2019). In the MI model, macrophages congregate at the edge of infarct tissue and are polarized into M1 macrophages. Upregulation of potassium channel KCa3.1 in M1 macrophages promotes Ca2+ influx to cardiomyocytes via Cx43, resulting in prolonged action potential duration APD (Fei et al., 2019). Macrophages can also exert paracrine effects on cardiac electrical activity through various cytokines. M1 macrophages secrete pro-inflammatory factors (TNF-α, IL-1β, and IL-6) to induce cardiac electrical remodeling, in which IL-1β has been shown to reduce the expression of atrial tremor protein (QKI) and further attenuate L-type Ca2+ current in cardiocytes to inhibit atrial fibrillation (Tili et al., 2015; Fei et al., 2019). Both the sympathetic and parasympathetic nerves innervate the heart. Arrhythmia is caused by abnormalities in autonomic innervation, and macrophages may be involved in this process (Stavrakis et al., 2020). A study in HF rats demonstrated that depletion of anti-inflammatory macrophages in the stellate ganglion reduces N-type Ca2+ currents and excitability of cardiac sympathetic postganglionic (CSP) neurons, thereby reducing cardiac sympathetic overactivation and ventricular arrhythmias in HF (Zhang D. et al., 2021). Nerve growth factor (NGF) is necessary to promote sympathetic nerve germination. M2 macrophages suppress ventricular arrhythmia caused by sympathetic nerve remodeling after myocardial infarction by reducing the expression of NGF (Liu et al., 2023; Yang et al., 2024) found that Sinapic acid (SA) stimulated the PPARγ pathway with concentration-dependent to promote M2 polarization and reduce NGF (Yang et al., 2022). In summary, macrophage polarization is a crucial regulatory factor of cardiac electrical conduction and is expected to be a primary target in the clinical treatment of arrhythmia.
4 Clinical dilemmas and treatment strategies for HF
HF can be classified into HFrEF and HFpEF based on the level of left ventricular ejection fraction (LVEF) measured by echocardiography at the time of the patient’s initial evaluation. Angiotensin-converting enzyme inhibitors (ACEI) or angiotensin receptor blockers (ARB)/angiotensin receptor-enkephalin inhibitors (ARNI), beta-blockers, salocorticoid receptor antagonists (MRAs), and SGLT2i constitute guidelines for guided drug therapy (GDMT) for patients with HFrEF. Quadruple therapy has been shown in numerous studies to dramatically lower the risk of death or hospitalization in people with HFrEF (Felker, 2020). Simultaneous initiation of the quadruple therapy and early reach of the target dose are central to the execution of GDMT (Heidenreich et al., 2022). However, a global survey shows that only 25% of patients are likely to start all drugs in GDMT at the same time (Savarese et al., 2024). Drug side effects such as hypotension, creatinine elevation, hyperkalemia, and bradycardia are the main clinical obstacles to the implementation of GDMT. Thus, based on the early intervention concept, it is advised to give preference to small-dose combinations, titrate the dosage, and create a customized regimen in stages based on the patient’s actual situation (Ge et al., 2022). It is important to note that mild hypotension and a slight rise in creatinine do not necessarily require a change in the HF treatment plan (Cautela et al., 2020; Savarese et al., 2024). Hyperkalemia is related to high mortality in HF patients, and part of the reason is that hyperkalemia prompts doctors to discontinue MRAs prematurely (Trevisan et al., 2021). According to researches, patients at risk for hyperkalemia can take advantage of novel potassium binders and the nephroprotective effects of SGLT2i to maintain treatment for MRAs (Pitt et al., 2011; Anker et al., 2015; Ferreira et al., 2021; Butler et al., 2022). Poor patient adherence to multidrug therapy is a non-clinical barrier to GDMT implementation, and outpatient follow-up is currently the most favored approach for this issue (Malmborg et al., 2023). In addition to chronic hypertension, HFpEF has been connected with multiple syndromes and microvascular dysfunction secondary to an enhanced systemic inflammatory state. In terms of the management of HFpEF patients, diuretics are first-line drugs to reduce volume load and blood stasis, and attention is paid to identifying and treating specific causes and the management of complications (McDonagh et al., 2021). Renin-angiotensin-aldosterone system (RAAS) inhibitors and SGLT2i have also demonstrated potential therapeutic advantages in HFpEF in recent years (Solomon et al., 2020; Vaduganathan et al., 2022). Unfortunately, there is no reliable evidence for treatments that improve adverse outcomes in HFpEF patients, which will be a huge challenge to address in future clinical practice. In terms of other treatments, combined with ivabradine, guanylate cyclase agonists, anticoagulants, positive inotropic drugs or TCM, and even cardiac resynchronization (CRT), implantable cardioverter defibrillator (ICD), and other devices are selected according to the individual conditions of patients to reduce the hospitalization rate and mortality of patients with HF.
5 The regulatory effect of TCM on macrophages in HF
According to TCM theory, deficiency of qi, blood, yin and yang of the heart, stagnation of qi, blood, and fluid are the pathomechanisms of HF. TCM offers specific benefits in treating HF and has unique advantages in reducing cardiac inflammation, delaying ventricular remodeling, promoting neovascularization, and suppressing arrhythmias (Cao et al., 2021; Dong et al., 2022a; Lu X. et al., 2022; Li et al., 2022). The dynamics of macrophage polarization into M1 and M2 according to the microenvironment can be elaborated by the yin-yang theory of TCM. Yin and yang oppose and restrain one another, just like M1 macrophages exacerbate cardiac inflammation and injury, while M2 macrophages reduce inflammation and repair damaged tissues. The two constrain each other and maintain the balance of yin and yang in the organism to ensure normal physiological functions. Under certain conditions, M1 and M2 transform into each other, called yin-yang transformation in TCM theory. The goal of controlling macrophage polarization is to bring M1 and M2 into balance, consistent with the concept of regulating the balance of yin and yang in TCM. Therefore, TCM regulating macrophage polarization is expected to be one of the most effective ways of treating HF.
5.1 TCM compounds alleviate HF by regulating macrophage polarization
According to the pathogenesis of HF in TCM theory, most of the TCM compounds used to treat HF have the effect of warming yang and benefiting qi, promoting blood and fluid circulation. With the deepening of HF research in recent years, more studies have shown that TCM compounds achieve anti-inflammatory, anti-fibrosis, and myocardial tissue protection by regulating the polarization of macrophages. The synergistic effect of complicated components in TCM compounds may explain their various therapeutic effects in HF treatment. Table 1 lists some TCM compounds that regulate the polarization of HF macrophages.
5.1.1 Qishen Granule
Qishen Granule (QSG) is a clinically approved TCM compound for the treatment of HF, which has the effect of warming yang and invigorating qi, promoting blood circulation and detoxifying. QSG consists of Astragalus mongholicus Bunge (Fabaceae; Astragali Radix), Salvia miltiorrhiza Bunge (Lamiaceae; Salviae miltiorrhizae radix et rhizoma), Lonicera japonica Thunb (Caprifoliaceae; Lonicerae Japonicae Flos), Aconitum carmichaelii Debeaux (Ranunculaceae; Aconiti Lateralis Radix Praeparata), Scrophularia ningpoensis Hemsl (Scrophulariaceae; Scrophulariae Radix) and Glycyrrhiza uralensis Fisch (Fabaceae; Glycyrrhizae Radix et Rhizoma). Clinical research has demonstrated that QSG is superior to placebo in decreasing NT-proBNP in CHF patients and enhancing the quality of life, the 6-min walk test (6MWD), the NYHA cardiac function grade, and the symptom score scale (Du et al., 2022). Lu et al. (2019) discovered that QSG could inhibit inflammation and fibrosis and improve the cardiac function of HF rats. Its mechanism was to reduce the recruitment and release of monocytes in damaged myocardial tissues, restrict the conversion of monocytes to M1 macrophages, and promote the M2 macrophage polarization by suppressing the AT1/MCP-1/CCR2 signaling pathway. Decreased M1 macrophages further inhibited TGF-β1/Smad3 pathway-mediated myocardial fibrosis, whereas an increase in M2 macrophages led to a rise in VEGF expression and proliferation in cardiac microvessels to alleviate ischemia and hypoxia in damaged myocardium. Based on previous studies, Li et al. demonstrated in vitro that QSG impeded the transformation of Ly6high monocytes to M1 macrophages, possibly by downregulating the expression of the TLR4-MyD88-NF-κB p65 pathway (Li et al., 2022).
5.1.2 Nuanxinkang
Nuanxinkang (NXK) is a simplified TCM compound derived from Xin Yang tablet, which contains Panax ginseng C.A.Mey. (Araliaceae; Ginseng Radix et Rhizoma Rubra) and Ilex pubescens Hook. et Arn (Aquifoliaceae; Ilex pubescentis radix et caulis). It has been used for decades in the treatment of HF because it effectively warms yang and invigorates qi, detoxifies, and activates blood circulation (Chen et al., 2019). Dong et al. demonstrated that in MIRI-induced HF mice, NXK reduced the percentage of cardiac M1 macrophage in the acute (days 0–3) and healing phases (days 3–14), lowered levels of blood and cardiac inflammatory cytokines, including TNF-α, IL-1β, and IL-6 to inhibit systemic and local inflammatory, and revealed the mechanism that NXK reduced M1 macrophage by suppressing phosphorylation and nuclear translocation of critical proteins of the IKKβ/IκBα/NF-κB pathway in vitro. Additionally, this experiment found that NXK increased M2 macrophages and promoted wound healing in the early but inhibited M2 and the chronic accumulation of TGF-β in the chronic phase (after day 28) to delay collagen fiber aggregation (Dong et al., 2022a). They further explored the regulatory effects of NXK on macrophage energy metabolism and found that NXK depressed the HIF-1α/PDK1 axis to reduce glycolysis in RAW264.7 cells, which ameliorated myocardial injury and slowed down the process of HF (Dong et al., 2022b).
5.1.3 Shexiang Tongxin Dropping Pill
Shexiang Tongxin Dropping Pill (STDP) is composed of Moschus berezovskii Flerov (Cervidae; Moschus), total ginsenoside of P. ginseng C. A. Mey. (Araliaceae; Ginseng Rhizoma et leafy), Bufo gargarizans Cantor (Bufonidae; Bufonis Venenum), Bos taurus domesticus Gmelin (Bovidae; Bovis Calculus), Selenarctos thibetanus G. Cuvier (Ursidae; Ursi Fellis Pulvis), Dryobalanops aromatica C.F.Gaertn (Dipterocarpaceae; Borneolum Syntheticum) and S. miltiorrhiza Bunge. As a prescription medication approved by The Chinese State Food and Drug Administration (SFDA), STDP has excellent therapeutic efficacy and has been widely used in the treatment of cardiovascular diseases (Lu et al., 2020; Lin et al., 2022). Clinical studies have shown that compared with trimetazidine alone, STDP combined with trimetazidine to treat ischemic HF can significantly reduce inflammatory factors and neurohormonal factors, restrain left ventricular remodeling, and improve heart function and symptoms of HF (Wu and Hu, 2019). Li et al. induced coronary microvascular dysfunction (CMD) rat and OGD/R-induced endothelial injury model, respectively, proving that STDP alleviates M1 macrophage-related inflammation and endothelial dysfunction by suppressing the Dectin-1/Syk/IRF5 pathway (Cui et al., 2023). According to another research, STDP stimulates M2 macrophages to release VEGF-A, which promotes microangiogenesis, increases blood flow, and improves cardiac function in CMD rats (Lu X. et al., 2022).
5.1.4 Naoxintong
Naoxintong (NXT) originated from the Buyang huanwu decoction in “Correction on the Errors of Mecical Works” by Wang Qingren in the Qing Dynasty. It has a total of 16 herbs and has the effect of promoting blood circulation. A systematic review and meta-analysis of RCT in 1589 CHF patients showed that NXT combined with conventional western medicine was better than conventional Western medicine alone, reduced BNP, and improved cardiac function indicators more effectively (Ban and Ke, 2020). According to reports, NXT protects the heart by encouraging angiogenesis, reducing oxidative stress and inflammation, and controlling the metabolism of fats and carbohydrates (Han et al., 2019). Naoxintong was found to improve heart function and reduce infarct size in MIRI by inhibiting the activation of NLRP3 inflammasome, restricting M1 macrophage polarization and IL-1β to prevent inflammation (Wang et al., 2017).
5.1.5 Shenfu injection
Shenfu injection (SFI) is a modified medication from Shenfu decoction, composed of P. ginseng C. A. Mey. (Araliaceae; Ginseng Radix et Rhizoma) and A. carmichaelii Debeaux can invigorate qi to engender blood and restore yang. SFI is frequently used clinically to treat HF, and a systematic review of RCT involved in 1042 CHF patients revealed that SFI combined with western medicine improved patients’ quality of life and activity tolerance more effectively than using western medicine alone (Zhou et al., 2023). Another similar systematic review and meta-analysis of RCT in 3231 HF patients showed that SFI adjuvant therapy was superior to conventional western medicine alone in terms of clinical efficacy and improved cardiac function indicators and could reduce the incidence of adverse reactions (Wu et al., 2022; Yang et al., 2024). Established the HF mice by intraperitoneal injection of isoproterenol (ISO) and observed the M1/M2 imbalance was mainly caused by the increase of M1 macrophages. SFI can inhibit M1 macrophage activation, significantly reduce TNF-α and IL-6 secretion, promote macrophage polarization towards M2, release Arg1 and IL-10, reduce inflammation, and improve cardiac function and ventricular remodeling by downregulating the TLR4/NF-κB signaling pathway (Yang et al., 2024).
5.1.6 QiShenYiQi pill
QiShenYiQi Pill (QSYQ) is a compound approved by the SFDA that benefits qi and promotes blood circulation. It is composed of A. mongholicus Bunge, S. miltiorrhiza Bunge, and Panax notoginseng (Burk.)F. H. Chen (Araliaceae; Notoginseng Radix), and Dalbergia odorifera T. Chen (Leguminosae; Dalbergiae Odoriferae Lignum). Numerous clinical investigations have shown that QSYQ is an effective treatment for HF. A systematic review and meta-analysis involving 895 patients with HFpEF revealed that western medicine combined with QSYQ could better decrease BNP and increase the rate of cardiac function improvement and 6-MWD compared with the western medicine group (Wang et al., 2021). Research has demonstrated that high-dose QSYQ (0.8 g/kg) reduces cardiac hypertrophy induced by pressure overload, restrains cardiomyocyte apoptosis, and the expression of our-and-a-half LIM domains protein 2 (FHL2), inhibits M1/M2 macrophage polarization and myocardial fibrosis mediated by RP S19/TGF-β1/Smad signaling pathway (Anwaier et al., 2022).
5.1.7 Xinyin Tablet
Xinyin Tablet (XYT) comprises P. ginseng C. A. Mey., A. mongholicus Bunge, Schisandra Chinensis (Turcz.)Baill. (Magnoliaceae; Schisandrae Chinensis Fructus), Leonurus japonicus Houtt. (Lamiaceae; Leonuri Herba), Descurainia sophia (L.). Webb ex Prantl (Brassicaceae; Descurainiae Semen), and I. pubescens Hook. et Arn. It is a hospital preparation approved by the Guangdong Food and Drug Administration (approval number: Yueyao Z20071178) and has been in clinical use for HF for several decades (Liu Q. et al., 2020). Clinical research has demonstrated that the combination of XYT can considerably improve the left ventricular systolic and cardiopulmonary function in male CHF patients when used by standardized western medicine treatment (Ye et al., 2019). The myocardial tissue of CHF mice resulting from transverse aortic constriction (TAC) exhibited significant collagen fiber deposition, a marked degree of myocardial fibrosis, and elevated expression of M2 macrophage markers. Through restricting the MLK3/JNK signaling pathway, XYT inhibits the transformation of macrophages into M2 and the release of anti-inflammatory factors, thereby reducing the synthesis of Col I and Col Ⅲ in myocardial tissue and abnormal deposition in the extracellular stroma, alleviating myocardial fibrosis and delaying ventricular remodeling (Liu et al., 2021).
5.1.8 Fangji Fuling Decoction
Fangji Fuling Decoction (FFD), derived from “Synopsis of Golden Chamber” by Zhang Zhongjing in the Han Dynasty, is composed of five herbs: Stephania tetrandra S.Moore (Menispermaceae; Stephaniae Tetrandrae Radix), Poria cocos (Schw.) Wolf (Polyporaceae; Poria), Cinnamomum verum J.Presl (Lauraceae; Cinnamomi Ramulus), A. mongholicus Bunge and G. uralensis Fisch. It has the effect of warming yang, invigorating qi and promoting blood and fluid circulation, and is a frequently used compound in clinical treatment of HF (Wang and Lu, 2015). According to studies, FFD inhibit inflammation, decrease collagen deposition in mice with myocardial fibrosis, increase the expression of the anti-inflammatory factor IL-10, decrease the expression of pro-inflammatory factors TNF-α, IL-1β and IL-6, and promote the transformation of macrophage from M1 to M2 (Shi et al., 2023).
5.2 Active ingredient of single TCM alleviate HF by regulating macrophage polarization
Through the analysis of the rule of medicine in the treatment of HF by TCM, it is concluded that the main effect of medicine for HF is to warm yang and benefit qi, promote blood circulation and remove blood stasis. The active ingredients of single TCM are the material basis for its effect. The studies demonstrated how TCM active ingredients modulate M1/M2 macrophage polarization balance in various ways, potentially aiding in the treatment of HF. Table 2 lists some active ingredients of single TCM that regulate the polarization of HF macrophages.
5.2.1 Puerarin and tanshinone ⅡA
Puerarin (Pue), the primary active ingredient of Pueraria lobata (Willd.) Ohwi (Leguminosae; Puerariae Lobatae Radix) has a wide range of pharmacological effects and is closely related to cardiovascular diseases. Studies have shown that Pue inhibits cardiac hypertrophy by reducing the generation of ROS and inhibiting the activation of ERK1/2, p38 MAPK and NF-κB pathways (Chen et al., 2014). Tanshinone ⅡA is one of the active ingredients of S. miltiorrhiza Bunge, which has therapeutic value in alleviating cardiac oxidative stress, inflammation and fibrosis (Lu TC. et al., 2022). The combination of tanshinone ⅡA and Pue in treating ischemic heart disease has a synergistic effect. The two combined at a 1:1 ratio for 28 days have been shown in an experiment to significantly improve cardiac dysfunction resulting from AMI. This improvement may be attributed to the inhibition of M1 macrophage and the promotion of M2 macrophage during the early stages of inflammation, reducing collagen synthesis and inhibiting myocardial fibrosis and ventricular remodeling (Gao et al., 2019).
5.2.2 Dihydrotanshinone Ⅰ
Dihydrotanshinone I (DHT) is another active ingredient of S. miltiorrhiza Bunge, which has a protective effect on myocardial injury. DHT significantly reduces the oxidative stress damage of H9C2 cells induced by OGD/R to reduce cell apoptosis (Wang et al., 2024). Doxorubicin (DOX) -induced cardiac inflammation is a high-risk factor for HF. Wang et al. discovered that DHT improves DOX-induced cardiac dysfunction by regulating the mTOR-TFEB-NF-κB signaling pathway, reducing M1 macrophage polarization and the release of TNF-α and IL-1β, and controlling inflammation (Wang et al., 2020).
5.2.3 Salvianolic acid B
Salvianolic acid B (Sal B) is also one of the active components of S. miltiorrhiza Bunge, which has anti-oxidation, anti-MIRI, and anti-atherosclerosis effects and has positive impacts on cardia-cerebrovascular disease (He et al., 2023). It has been reported that in MIRI mice, Sal B reduces M1 macrophages and increases M2 macrophages after reperfusion for 3 days by inhibiting mTORC1-induced glycolysis, thus reducing collagen deposition, improving cardiac dysfunction, and restricting inflammation following MIRI (Zhao et al., 2020).
5.2.4 Curcumin
Curcumin, the primary active ingredient of Curcuma longa L. (Zingiberaceae; Curcumae Longae Rhizoma), is a regulator of macrophage polarization, which plays a cardioprotective role through various signaling pathways (Zhou et al., 2015). Studies have found that curcumin activates the STAT6 pathway through the secretion of IL-4 and IL-13, promotes the polarization of M2 macrophages, reduces the infiltration of proinflammatory cells, and enhances the cardiac function indicators of dilated cardiomyopathy induced by autoimmune myocarditis (Gao et al., 2015). Subsequently, Yan et al. investigated how curcumin affected macrophage polarization in MI mice, and the results showed that curcumin decreased M1 macrophages, TNF-α, IL-1β and IL-6 through the AMPK pathway, increased M2 macrophages and IL-10, inhibited early inflammation, and thus impaired myocardial remodeling after 3 months of MI (Yan et al., 2021).
5.2.5 Latifolin
Dalbergia odorifera T. Chen is an herb that promotes blood circulation and relieves pain. Latifolin is one of the active ingredients of D. odorifera T. Chen. According to pharmacological research, Latifolin possesses antithrombotic, anti-inflammatory, and antioxidant properties (Yang et al., 2013). Latifolin suppressed the expression of M1 biomarkers (iNOS, CD86), enhanced the expression of M2 biomarkers (CD206, IL-10, IL-4R), and decreased the secretion of TNF-α, IL-1β, and IL-6 to block cardiac inflammation caused by DOX plays a role in cardiac protection (Zhang N. et al., 2020).
5.2.6 Arctigenin
Arctigenin (ATG) is the essential ingredient in Arctium lappa L. (Asteraceae, Arctii Fructus), possessing anti-oxidative stress, anti-cancer and anti-inflammatory properties (Gu et al., 2012; Xu X. et al., 2020; Guo et al., 2020). Ni et al. revealed that ATG reduces cardiac inflammation, enhances cardiac function, shrinks infarct size, prevents M1/M2c macrophage polarization, and promotes M2a/M2b/M2d polarization in MI mice. Utilizing RNA-Seq analysis, they proceeded to ascertain the regulatory mechanism of ATG on macrophages. Their findings were validated in subsequent in vitro and in vivo experiments, which indicated that ATG regulation of macrophage polarization was associated with inhibition of the JAK-STAT and NF-κb pathways induced by NFAT5 genes (Ni et al., 2020).
5.3 Acupuncture alleviates HF by regulating macrophage polarization
Acupuncture, a common complementary and integrative therapy, has been used clinically by millions worldwide. It specifically improves angina pectoris, palpitations, and other systemic diseases. Anti-inflammation is one of the therapeutic mechanisms of acupuncture related to regulating macrophage polarization. In AMI mice induced by LAD ligation, electroacupuncture pretreatment at Neiguan (PC6) for 3 days inhibited the activation of the NLRP3 inflammasome, promoted M2 macrophages polarization, and reduced the degree of inflammation after AMI injury, thereby reducing infarct size and improving cardiac function (Zhang T. et al., 2020). Hua et al. detected and sampled MIRI rats at 6 h, 24 h, and 3 days after reperfusion, which further proved that electroacupuncture accelerated the M2 macrophages polarization, promoted the transition from the acute proinflammatory phase to the anti-inflammatory repair phase after MIRI, and ultimately produced cardiac protection (Bai et al., 2023).
6 Conclusion and prospects
With the prevalence of coronary heart disease, hypertension, obesity, diabetes, and other diseases, the morbidity and mortality of HF are increasing annually, which seriously affects people’s lives and social development. At present, there are still many barriers in the treatment of HF, so it is urgent to develop new therapeutic strategies.
In recent years, the theory of immune inflammation has gained widespread attention. Macrophages are a crucial component of the immune system that is capable of rapidly detecting and reacting to environmental changes, regulating inflammation, and repairing tissues by polarizing into M1 or M2 macrophages. During the course of HF, macrophages sense early cardiac injury through various signaling molecules. M1 macrophages secrete pro-inflammatory factors mediating early inflammation, removing pathogens and damaged myocardium to exert a cardioprotective effect. However, excessive or persistent inflammation results in massive death of cardiomyocytes and aggravates cardiac injury. As the disease progresses, M2 macrophages release anti-inflammatory factors to suppress the inflammation, promote fibroblast activation and collagen deposition, and repair damaged heart, but immoderate fibrosis causes cardiac stiffness and deterioration of cardiac function. The regenerative capacity of microvessels and the electrophysiological activity of cardiomyocytes are critical to cardiac function and closely related to macrophage polarization. It follows that maintaining a balance of M1/M2 macrophages is a potential target for treating HF.
TCM has a broad clinical foundation for treating and preventing HF, and long-term practice has shown its safety and effectiveness. The function of M1 and M2 macrophages is mutually restricted, consistent with TCM yin-yang theory. The pathogenesis of HF is usually the decline of heart yang qi and the stagnation of blood and fluid. Therefore, TCM, which regulates the polarization of macrophages, has the effect of warming yang, invigorating qi, and promoting blood and fluid circulation. Although TCM has become increasingly aware of the function that macrophage polarization plays in HF, there are few studies on TCM compound regulation of macrophage polarization. In addition, existing studies mainly focus on inflammation and are limited to detecting M1/M2 macrophage surface protein markers and inflammatory factors secreted by macrophages. Some studies also ignored the regulatory pathway mechanism, which leads to an inadequate integration of evidence chains. It is unable to meet the methodical understanding of TCM treatment for HF. Furthermore, most researches are restricted to animal and cellular levels and lack clinical evidence. TCM has the characteristics of multi-component, multi-target and multi-link action, and has varying functions in different stages of disease development. In the future, it is necessary to strengthen further the basic and clinical research of TCM intervention in HF, especially TCM compounds. Meanwhile, the mechanism of TCM regulating macrophage polarization in different pathological stages of HF will be explored to enrich the scientific connotation of TCM treatment of HF and highlight the scientific and effectiveness of TCM yin-yang theory.
Author contributions
ZZ: Writing–original draft, Writing–review and editing. MW: Writing–review and editing. SL: Writing–review and editing. SD: Writing–review and editing. JL: Writing–review and editing.
Funding
The author(s) declare that financial support was received for the research, authorship, and/or publication of this article. This study was supported by the Key Projects of Scientific Research Program of Hunan Provincial Administration of Traditional Chinese Medicine (D2023005,2021011), the Key Projects of Scientific Research of Hunan Provincial Department of Education (21A0234), 2023 Double First-Class Discipline Construction-Research on Prevention and Treatment of Cardio-Cerebral Diseases by Traditional Chinese Medicine (6001001006).
Acknowledgments
All the pictures in this article are drawn in Adobe Illustrator 2021, thanks to the support provided by the software.
Conflict of interest
The authors declare that the research was conducted in the absence of any commercial or financial relationships that could be construed as a potential conflict of interest.
Publisher’s note
All claims expressed in this article are solely those of the authors and do not necessarily represent those of their affiliated organizations, or those of the publisher, the editors and the reviewers. Any product that may be evaluated in this article, or claim that may be made by its manufacturer, is not guaranteed or endorsed by the publisher.
Supplementary material
The Supplementary Material for this article can be found online at: https://www.frontiersin.org/articles/10.3389/fphar.2024.1434654/full#supplementary-material
References
Adamo, L., Rocha-Resende, C., Prabhu, S. D., and Mann, D. L. (2020). Reappraising the Role of Inflammation in Heart Failure. Nat. Rev. Cardiol. 17 (5), 269–285. doi:10.1038/s41569-019-0315-x
Anker, S. D., Kosiborod, M., Zannad, F., Piña, I. L., McCullough, P. A., Filippatos, G., et al. (2015). Maintenance of Serum Potassium With Sodium Zirconium Cyclosilicate (Zs-9) In Heart Failure Patients: Results from a Phase 3 Randomized, Double-Blind, Placebo-Controlled Trial. Eur. J. Heart Fail 17 (10), 1050–1056. doi:10.1002/ejhf.300
Antoine, S., Vaidya, G., Imam, H., and Villarreal, D. (2017). Pathophysiologic Mechanisms In Heart Failure: Role of The Sympathetic Nervous System. Am. J. Med. Sci. 353 (1), 27–30. doi:10.1016/j.amjms.2016.06.016
Anwaier, G., Xie, T. T., Pan, C. S., Yan, L., Wang, D., Weng, D. Z., et al. (2022). QiShenYiQi pill ameliorates cardiac fibrosis after pressure overload-induced cardiac hypertrophy by regulating FHL2 and the macrophage RP S19/TGF-β1 signaling pathway. Front. Pharmacol. 13, 918335. doi:10.3389/fphar.2022.918335
Arranz, A., Doxaki, C., Vergadi, E., Martinez de la Torre, Y., Vaporidi, K., Lagoudaki, E. D., et al. (2012). Akt1 and Akt2 Protein Kinases Differentially Contribute To Macrophage Polarization. Proc. Natl. Acad. Sci. U. S. A. 109 (24), 9517–9522. doi:10.1073/pnas.1119038109
Babaev, V. R., Hebron, K. E., Wiese, C. B., Toth, C. L., Ding, L., Zhang, Y., et al. (2014). Macrophage Deficiency of Akt2 Reduces Atherosclerosis In LDLR Null Mice. J. Lipid Res. 55 (11), 2296–2308. doi:10.1194/jlr.M050633
Bacmeister, L., Schwarzl, M., Warnke, S., Stoffers, B., Blankenberg, S., Westermann, D., et al. (2019). Inflammation and Fibrosis in Murine Models of Heart Failure. Basic Res. Cardiol. 114 (3), 19. doi:10.1007/s00395-019-0722-5
Bai, H., Xu, S. L., Shi, J. J., Ding, Y. P., Liu, Q. Q., Jiang, C. H., et al. (2023). Electroacupuncture Preconditioning Protects Against Myocardial Ischemia-Reperfusion Injury By Modulating Dynamic Inflammatory Response. Heliyon 9 (9), e19396. doi:10.1016/j.heliyon.2023.e19396
Ban, J. F., and Ke, R. (2020). Meta-Analysis of Naoxintong Capsules Combined With Western Medicine In Treatment of Chronic Heart Failur. China J. Chin. materia medica 45 (17), 4254–4261. doi:10.19540/j.cnki.cjcmm.20200224.501
Bhatta, A., Yao, L., Xu, Z., Toque, H. A., Chen, J., Atawia, R. T., et al. (2017). Obesity-Induced Vascular Dysfunction and Arterial Stiffening Requires Endothelial Cell Arginase 1. Cardiovasc Res. 113 (13), 1664–1676. doi:10.1093/cvr/cvx164
Binatti, E., Gerussi, A., Barisani, D., and Invernizzi, P. (2022). The Role of Macrophages in Liver Fibrosis: New Therapeutic Opportunities. Int. J. Mol. Sci. 23 (12), 6649. doi:10.3390/ijms23126649
Bonnet, F., and Scheen, A. J. (2018). Effects of SGLT2 Inhibitors on Systemic and Tissue Low-Grade Inflammation: The Potential Contribution To Diabetes Complications And Cardiovascular Disease. Diabetes and Metabolism 44 (6), 457–464. doi:10.1016/j.diabet.2018.09.005
Bozkurt, B., Coats, A. J. S., Tsutsui, H., Abdelhamid, C. M., Adamopoulos, S., Albert, N., et al. (2021). Universal Definition and Classification of Heart Failure: A Report of The Heart Failure Society of America, Heart Failure Association of The European Society of Cardiology, Japanese Heart Failure Society And Writing Committee of The Universal Definition of Heart Failure: Endorsed By The Canadian Heart Failure Society, Heart Failure Association of India, Cardiac Society of Australia And New Zealand, and Chinese Heart Failure Association. Eur. J. heart Fail. 23 (3), 352–380. doi:10.1002/ejhf.2115
Brown, B. N., Ratner, B. D., Goodman, S. B., Amar, S., and Badylak, S. F. (2012). Macrophage Polarization: An Opportunity for Improved Outcomes In Biomaterials And Regenerative Medicine. Biomaterials 33 (15), 3792–3802. doi:10.1016/j.biomaterials.2012.02.034
Butler, J., Anker, S. D., Lund, L. H., Coats, A. J. S., Filippatos, G., Siddiqi, T. J., et al. (2022). Patiromer For The Management of Hyperkalemia In Heart Failure With Reduced Ejection Fraction: The DIAMOND Trial. Eur. Heart J. 43 (41), 4362–4373. doi:10.1093/eurheartj/ehac401
Byles, V., Covarrubias, A. J., Ben-Sahra, I., Lamming, D. W., Sabatini, D. M., Manning, B. D., et al. (2013). The TSC-mTOR Pathway Regulates Macrophage Polarization. Nat. Commun. 4, 2834. doi:10.1038/ncomms3834
Cao, X., Zhou, M., Liu, H., Chen, X., Li, X., and Jia, S. (2021). Clinical Efficacy and Safety of Shensong Yangxin Capsule-Amiodarone Combination on Heart Failure Complicated By Ventricular Arrhythmia: A Meta-Analysis of Randomized Controlled Trials. Front. Pharmacol. 12, 613922. doi:10.3389/fphar.2021.613922
Cautela, J., Tartiere, J. M., Cohen-Solal, A., Bellemain-Appaix, A., Theron, A., Tibi, T., et al. (2020). Management of Low Blood Pressure in Ambulatory Heart Failure With Reduced Ejection Fraction Patients. Eur. J. Heart Fail 22 (8), 1357–1365. doi:10.1002/ejhf.1835
Chen, G., Pan, S. Q., Shen, C., and Zhang, X. m. (2014). Puerarin Inhibits Angiotensin II-Induced Cardiac Hypertrophy via the Redox-Sensitive ERK1/2, p38 and NF-κB pathways. Acta Pharmacol. Sin. 35 (4), 463–475. doi:10.1038/aps.2013.185
Chen, R., Wang, J., Dai, X., Wu, S., Huang, Q., Jiang, L., et al. (2022). Augmented PFKFB3-Mediated Glycolysis By Interferon-γ Promotes Inflammatory M1 Polarization Through the JAK2/STAT1 Pathway In Local Vascular Inflammation in Takayasu Arteritis. Arthritis Res. Ther. 24 (1), 266. doi:10.1186/s13075-022-02960-1
Chen, W. S., Cao, Z., Leffler, H., Nilsson, U. J., and Panjwani, N. (2017). Galectin-3 Inhibition by a Small-Molecule Inhibitor Reduces Both Pathological Corneal Neovascularization and Fibrosis. Investigative Ophthalmol. Vis. Sci. 58 (1), 9–20. doi:10.1167/iovs.16-20009
Chen, Z., Luo, T., Zhang, L., Zhou, Z., Huang, Y., Lu, L., et al. (2019). A Simplified Herbal Formula for the Treatment of Heart Failure: Efficacy, Bioactive Ingredients, And Mechanisms. Pharmacol. Res. 147, 104251. doi:10.1016/j.phrs.2019.104251
Cheng, Y., Zhong, X., Nie, X., Gu, H., Wu, X., Li, R., et al. (2023). Glycyrrhetinic Acid Suppresses Breast Cancer Metastasis By Inhibiting M2-Like Macrophage Polarization Via Activating JNK1/2 Signaling. Phytomedicine 114, 154757. doi:10.1016/j.phymed.2023.154757
Ciesielska, A., Matyjek, M., and Kwiatkowska, K. (2021). TLR4 and CD14 Trafficking and its Influence on LPS-Induced Pro-Inflammatory Signaling. Cell. Mol. life Sci. 78 (4), 1233–1261. doi:10.1007/s00018-020-03656-y
Covarrubias, A. J., Aksoylar, H. I., and Horng, T. (2015). Control of Macrophage Metabolism and Activation by mTOR and Akt Signaling. Seminars Immunol. 27 (4), 286–296. doi:10.1016/j.smim.2015.08.001
Cui, L., Liu, Y., Hu, Y., Dong, J., Deng, Q., Jiao, B., et al. (2023). Shexiang Tongxin Dropping Pill alleviates M1 Macrophage Polarization-Induced Inflammation and Endothelial Dysfunction To Reduce Coronary Microvascular Dysfunction Via the Dectin-1/Syk/IRF5 Pathway. J. Ethnopharmacol. 316, 116742. doi:10.1016/j.jep.2023.116742
Dai, Y., Xu, M., Wang, Y., Pasha, Z., Li, T., and Ashraf, M. (2007). HIF-1alpha induced-VEGF Overexpression In Bone Marrow Stem Cells Protects Cardiomyocytes Against Ischemia. J. Mol. Cell. Cardiol. 42 (6), 1036–1044. doi:10.1016/j.yjmcc.2007.04.001
Dick, S. A., and Epelman, S. (2016). Chronic Heart Failure and Inflammation: What do We Really Know? Circulation Res. 119 (1), 159–176. doi:10.1161/CIRCRESAHA.116.308030
Dong, X., Jiang, J., Lin, Z., Wen, R., Zou, L., Luo, T., et al. (2022a). Nuanxinkang Protects Against Ischemia/Reperfusion-Induced Heart Failure Through Regulating IKKβ/IκBα/NF-κB-Mediated Macrophage Polarization. Phytomedicine 101, 154093. doi:10.1016/j.phymed.2022.154093
Dong, X., Weng, R. J., Jiang, J. L., Ling, Z. J., Guan, Z. J., Li, X., et al. (2022b). Effect and mechanism of Nuanxinkang on Heart Failure After Myocardial Ischemia-Reperfusion. Chin. J. Traditional Chin. Med. 40 (12), 166–170+303. doi:10.13193/j.issn.1673-7717.2022.12.035
Du, K., Liu, J., Tan, N., Huang, X., Wang, J., Zhao, H., et al. (2022). The Effects of Qishen Granules For Patients With Chronic Heart Failure: A Multicenter Randomized Double-Blind Placebo-Controlled Trial. Front. Pharmacol. 13, 1017734. doi:10.3389/fphar.2022.1017734
Duncan, S. A., Sahu, R., Dixit, S., Singh, S. R., and Dennis, V. A. (2020). Suppressors of Cytokine Signaling (SOCS)1 and SOCS3 Proteins Are Mediators Of Interleukin-10 Modulation of Inflammatory Responses Induced by Chlamydia Muridarum and its Major Outer Membrane Protein (MOMP) in Mouse J774 Macrophages. Mediat. Inflammatiom. 2020, 7461742. doi:10.1155/2020/7461742
Epelman, S., Lavine, K. J., and Randolph, G. J. (2014). Origin and Functions of Tissue Macrophages. Immunity 41 (1), 21–35. doi:10.1016/j.immuni.2014.06.013
Eun, H. S., and Jeong, W. I. (2016). Dual Notch Signaling in Proinflammatory Macrophage Activation. Hepatology 63 (4), 1381–1383. doi:10.1002/hep.28386
Fan, W., Morinaga, H., Kim, J. J., Bae, E., Spann, N. J., Heinz, S., et al. (2010). FoxO1 Regulates Tlr4 Inflammatory Pathway Signalling in Macrophages. EMBO J. 29 (24), 4223–4236. doi:10.1038/emboj.2010.268
Fei, Y. D., Wang, Q., Hou, J. W., Li, W., Cai, X. X., Yang, Y. L., et al. (2019). Macrophages Facilitate Post Myocardial Infarction Arrhythmias: Roles of Gap Junction and KCa3.1. Theranostics 9 (22), 6396–6411. doi:10.7150/thno.34801
Felker, G. M. (2020). Building the Foundation For A New Era of Quadruple Therapy in Heart Failure. Circulation 141 (2), 112–114. doi:10.1161/CIRCULATIONAHA.119.044578
Ferreira, J. P., Zannad, F., Pocock, S. J., Anker, S. D., Butler, J., Filippatos, G., et al. (2021). Interplay of Mineralocorticoid Receptor Antagonists and Empagliflozin in Heart Failure: EMPEROR-reduced. J. Am. Coll. Cardiol. 77 (11), 1397–1407. doi:10.1016/j.jacc.2021.01.044
Frangogiannis, N. G. (2024). TGF-β as a Therapeutic Target in The Infarcted And Failing Heart: Cellular Mechanisms, Challenges, And Opportunities. Expert Opin. Ther. Targets 28 (1-2), 45–56. doi:10.1080/14728222.2024.2316735
Fujisaka, S., Usui, I., Ikutani, M., Aminuddin, A., Takikawa, A., Tsuneyama, K., et al. (2013). Adipose Tissue Hypoxia Induces Inflammatory M1 Polarity of Macrophages in an HIF-1α-Dependent and HIF-1α-Independent manner in Obese Mice. Diabetologia 56 (6), 1403–1412. doi:10.1007/s00125-013-2885-1
Fukao, T., and Koyasu, S. (2003). PI3K and Negative Regulation of TLR Signaling. Trends Immunol. 24 (7), 358–363. doi:10.1016/s1471-4906(03)00139-x
Gao, S., Li, L., Li, L., Ni, J., Guo, R., Mao, J., et al. (2019). Effects of the combination of Tanshinone IIA And Puerarin on Cardiac Function and Inflammatory Response In Myocardial Ischemia Mice. J. Mol. Cell. Cardiol. 137, 59–70. doi:10.1016/j.yjmcc.2019.09.012
Gao, S., Zhou, J., Liu, N., Wang, L., Gao, Q., Wu, Y., et al. (2015). Curcumin induces M2 macrophage polarization by secretion IL-4 and/or IL-13. J. Mol. Cell. Cardiol. 85, 131–139. doi:10.1016/j.yjmcc.2015.04.025
Ge, J. B., Cui, Y., Yang, J. F., Gao, X. F., Li, Y., et al. (2022). Expert consensus on clinical decision pathways for "new quadruple" drug therapy in chronic heart failure. Chin. Circulation J. 37 (08), 769–781. doi:10.3969/j.issn.1000-3614.2022.08.003
Ge, X., Meng, Q., Wei, L., Liu, J., Li, M., Liang, X., et al. (2021). Myocardial Ischemia-Reperfusion Induced Cardiac Extracellular Vesicles Harbour Proinflammatory Features and Aggravate Heart Injury. J. Extracell. Vesicles 10 (4), e12072. doi:10.1002/jev2.12072
Gentek, R., Molawi, K., and Sieweke, M. H. (2014). Tissue Macrophage Identity and Self-Renewal. Immunol. Rev. 262 (1), 56–73. doi:10.1111/imr.12224
Glezeva, N., Voon, V., Watson, C., Horgan, S., McDonald, K., Ledwidge, M., et al. (2015). Exaggerated Inflammation and Monocytosis Associate With Diastolic Dysfunction In Heart Failure With Preserved Ejection Fraction: Evidence Of M2 Macrophage Activation In Disease Pathogenesis. J. cardiac Fail. 21 (2), 167–177. doi:10.1016/j.cardfail.2014.11.004
Gong, M., Zhuo, X., and Ma, A. (2017). STAT6 Upregulation Promotes M2 Macrophage Polarization To Suppress Atherosclerosis. Med. Sci. Monit. basic Res. 23, 240–249. doi:10.12659/msmbr.904014
Gorter, T. M., van Woerden, G., Rienstra, M., Dickinson, M. G., Hummel, Y. M., Voors, A. A., et al. (2020). Epicardial Adipose Tissue and Invasive Hemodynamics In Heart Failure With Preserved Ejection Fraction. JACC Heart Fail. 8 (8), 667–676. doi:10.1016/j.jchf.2020.06.003
Gruzdeva, O., Uchasova, E., Dyleva, Y., Borodkina, D., Akbasheva, O., Belik, E., et al. (2018). Relationships Between Epicardial Adipose Tissue Thickness And Adipo-Fibrokine Indicator Profiles Post-Myocardial Infarction. Cardiovasc. Diabetol. 17 (1), 40. doi:10.1186/s12933-018-0679-y
Gu, Y., Qi, C., Sun, X., Ma, X., Zhang, H., Hu, L., et al. (2012). Arctigenin Preferentially Induces Tumor Cell Death Under Glucose Deprivation By Inhibiting Cellular Energy Metabolism. Biochem. Pharmacol. 84 (4), 468–476. doi:10.1016/j.bcp.2012.06.002
Guo, S., Chen, Y., Shi, S., Wang, X., Zhang, H., Zhan, Y., et al. (2020). Arctigenin, a novel TMEM16A Inhibitor for Lung Adenocarcinoma Therapy. Pharmacol. Res. 155, 104721. doi:10.1016/j.phrs.2020.104721
Haider, N., Boscá, L., Zandbergen, H. R., Kovacic, J. C., Narula, N., González-Ramos, S., et al. (2019). Transition of Macrophages To Fibroblast-Like Cells In Healing Myocardial Infarction. J. Am. Coll. Cardiol. 74 (25), 3124–3135. doi:10.1016/j.jacc.2019.10.036
Halade, G. V., Norris, P. C., Kain, V., Serhan, C. N., and Ingle, K. A. (2018). Splenic Leukocytes Define The Resolution Of Inflammation In Heart Failure. Sci. Signal. 11 (520), eaao1818. doi:10.1126/scisignal.aao1818
Han, J., Tan, H., Duan, Y., Chen, Y., Zhu, Y., Zhao, B., et al. (2019). The Cardioprotective Properties and The Involved Mechanisms of NaoXinTong Capsule. Pharmacol Res. 141, 409–417. doi:10.1016/j.phrs.2019.01.024
Hanna, A., and Frangogiannis, N. G. (2020). Inflammatory Cytokines and Chemokines as Therapeutic Targets In Heart Failure. Cardiovasc. drugs Ther. 34 (6), 849–863. doi:10.1007/s10557-020-07071-0
Hawiger, J., Veach, R. A., Liu, X., Timmons, S., and Ballard, D. W. (1999). IκB kinase complex is an intracellular target for Endotoxic lipopolysaccharide in Human monocytic cells. Blood 94 (5), 1711–1716. doi:10.1182/blood.v94.5.1711.417k20_1711_1716
He, G., Chen, G., Liu, W., Ye, D., Liu, X., Liang, X., et al. (2023). Salvianolic Acid B: A Review of Pharmacological Effects, Safety, Combination Therapy, New Dosage Forms, And Novel Drug Delivery Routes. Pharmaceutics 15 (9), 2235. doi:10.3390/pharmaceutics15092235
Heidenreich, P. A., Bozkurt, B., Aguilar, D., Allen, L. A., Byun, J. J., Colvin, M. M., et al. (2022). 2022 AHA/ACC/HFSA guideline for the Management of Heart Failure: Executive Summary: A Report of the American College of Cardiology/American Heart Association Joint Committee on Clinical Practice Guidelines. Circulation 145 (18), e876–e894. doi:10.1161/CIR.0000000000001062
Hirata, Y., Tabata, M., Kurobe, H., Motoki, T., Akaike, M., Nishio, C., et al. (2011). Coronary Atherosclerosis Is Associated With Macrophage Polarization In Epicardial Adipose Tissue. J. Am. Coll. Cardiol. 58 (3), 248–255. doi:10.1016/j.jacc.2011.01.048
Horckmans, M., Bianchini, M., Santovito, D., Megens, R. T. A., Springael, J. Y., Negri, I., et al. (2018). Pericardial Adipose Tissue Regulates Granulopoiesis, Fibrosis, And Cardiac Function After Myocardial Infarction. Circulation 137 (9), 948–960. doi:10.1161/CIRCULATIONAHA.117.028833
Horckmans, M., Ring, L., Duchene, J., Santovito, D., Schloss, M. J., Drechsler, M., et al. (2017). Neutrophils Orchestrate Post-Myocardial Infarction Healing By Polarizing Macrophages Towards A Reparative Phenotype. Eur. heart J. 38 (3), 187–197. doi:10.1093/eurheartj/ehw002
Hu, X., Li, J., Fu, M., Zhao, X., and Wang, W. (2021). The JAK/STAT Signaling Pathway: From Bench To Clinic. Signal Transduct. Target. Ther. 6 (1), 402. doi:10.1038/s41392-021-00791-1
Huang, C., Wang, J., Liu, H., Huang, R., Yan, X., Song, M., et al. (2022). Ketone body β-Hydroxybutyrate Ameliorates Colitis By Promoting M2 Macrophage Polarization Through the STAT6-Dependent Signaling Pathway. BMC Med. 20 (1), 148. doi:10.1186/s12916-022-02352-x
Huang, S. C., Smith, A. M., Everts, B., Colonna, M., Pearce, E. L., Schilling, J. D., et al. (2016). Metabolic Reprogramming mediated by the mTORC2-IRF4 signaling Axis is Essential for Macrophage Alternative Activation. Immunity 45 (4), 817–830. doi:10.1016/j.immuni.2016.09.016
Hulsmans, M., Sager, H. B., Roh, J. D., Valero-Muñoz, M., Houstis, N. E., Iwamoto, Y., et al. (2018). Cardiac Macrophages Promote Diastolic Dysfunction. J. Exp. Med. 215 (2), 423–440. doi:10.1084/jem.20171274
Jakubzick, C. V., Randolph, G. J., and Henson, P. M. (2017). Monocyte Differentiation and Antigen-Presenting Functions. Nat. Rev. Immunol. 17 (6), 349–362. doi:10.1038/nri.2017.28
Kimura, T., Nada, S., Takegahara, N., Okuno, T., Nojima, S., Kang, S., et al. (2016). Polarization of M2 Macrophages Requires Lamtor1 that Integrates Cytokine And Amino-Acid Signals. Nat. Commun. 7, 13130. doi:10.1038/ncomms13130
Kleinbongard, P., Heusch, G., and Schulz, R. (2010). TNFalpha in Atherosclerosis, Myocardial Ischemia/Reperfusion and Heart Failure. Pharmacol. Ther. 127 (3), 295–314. doi:10.1016/j.pharmthera.2010.05.002
Kobayashi, K., Hernandez, L. D., Galán, J. E., Janeway, C. A., Medzhitov, R., and Flavell, R. A. (2002). IRAK-M is a Negative Regulator of Toll-like Receptor Signaling. Cell. 110 (2), 191–202. doi:10.1016/s0092-8674(02)00827-9
Koga, Y., Tsurumaki, H., Aoki-Saito, H., Sato, M., Yatomi, M., Takehara, K., et al. (2019). Roles of cyclic AMP response Element binding activation in the ERK1/2 and p38 MAPK signalling pathway in central Nervous System, Cardiovascular System, Osteoclast Differentiation and Mucin and Cytokine Production. Int. J. Mol. Sci. 20 (6), 1346. doi:10.3390/ijms20061346
Kong, P., Christia, P., and Frangogiannis, N. G. (2014). The Pathogenesis Of Cardiac Fibrosis. Cell. Mol. Life Sci. 71 (4), 549–574. doi:10.1007/s00018-013-1349-6
Lawrence, T., and Natoli, G. (2011). Transcriptional Regulation of Macrophage Polarization: Enabling Diversity With Identity. Nat. Rev. Immunol. 11 (11), 750–761. doi:10.1038/nri3088
Lee, T. M., Chang, N. C., and Lin, S. Z. (2017). Dapagliflozin, a selective SGLT2 Inhibitor, Attenuated Cardiac Fibrosis By Regulating the Macrophage Polarization Via STAT3 SIGNALIng in Infarcted rat hearts. Free Radic. Biol. Med. 104, 298–310. doi:10.1016/j.freeradbiomed.2017.01.035
Li, R., Chen, B., Kubota, A., Hanna, A., Humeres, C., Hernandez, S. C., et al. (2023). Protective Effects of Macrophage-Specific Integrin Α5 In Myocardial Infarction Are Associated With Accentuated Angiogenesis. Nat. Commun. 14 (1), 7555. doi:10.1038/s41467-023-43369-x
Li, X., Zhang, J., Huang, J., Ma, A., Yang, J., Li, W., et al. (2013). A Multicenter, Randomized, Double-Blind, Parallel-Group, Placebo-Controlled Study Of The Effects Of Qili Qiangxin Capsules In Patients With Chronic Heart Failure. J. Am. Coll. Cardiol. 62 (12), 1065–1072. doi:10.1016/j.jacc.2013.05.035
Li, Y., Li, X., Chen, X., Sun, X., Liu, X., Wang, G., et al. (2022). Qishen Granule (QSG) Inhibits Monocytes Released From the Spleen and Protect Myocardial Function via the TLR4-MyD88-NF-κB p65 Pathway in Heart Failure Mice. Front. Pharmacol. 13, 850187. doi:10.3389/fphar.2022.850187
Liao, X., Shen, Y., Zhang, R., Sugi, K., Vasudevan, N. T., Alaiti, M. A., et al. (2018). Distinct Roles of Resident And Nonresident Macrophages In Nonischemic Cardiomyopathy. Proc. Natl. Acad. Sci. U. S. A. 115 (20), E4661–E4669. doi:10.1073/pnas.1720065115
Lin, Y. J., Jiao, K. L., Liu, B., Fang, L., and Meng, S. (2022). Antiplatelet and myocardial protective effect of Shexiang Tongxin Dropping Pill in Patients Undergoing Percutaneous coronary intervention: A Randomized Controlled Trial. J. Integr. Medicine-jim 20 (2), 126–134. doi:10.1016/j.joim.2022.01.001
Liu, Q., Huang, X., Tian, M., Dong, X., Kang, J., Liao, H., et al. (2020c). Effectiveness and safety of Xinyin tablet in treatment of chronic heart failure: A Protocol of Systematic Review And Meta-Analysis Of Randomized Controlled Trials. Med. Baltim. 99 (51), e23759. doi:10.1097/MD.0000000000023759
Liu, Q., Li, Y., Shi, Y., Tan, J., Yan, W., Zhang, J., et al. (2023). The protective Effect of Gamma Aminobutyric acid B Receptor activation on sympathetic Nerve Remodeling Via the regulation of M2 Macrophage Polarization After Myocardial Infarction. Rev. Port. Cardiol. 42 (2), 125–135. doi:10.1016/j.repc.2021.10.011
Liu, Q., Wang, J. Y., Deng, B., Zhang, L., Yang, Z. Q., Wang, L. J., et al. (2021). Xinyin tablets regulate macrophage polarization through MLK3/JNK Signaling to Improve the Mechanism of Myocardial Fibrosis In Chronic Heart Failure. Chin. J. Traditional Chin. Med. 36 (10), 6064–6068.
Liu, S., Cai, X., Wu, J., Cong, Q., Chen, X., Li, T., et al. (2015). Phosphorylation of Innate Immune Adaptor Proteins MAVS, STING, and TRIF induces IRF3 activation. Science 347 (6227), aaa2630. doi:10.1126/science.aaa2630
Liu, S., Chen, J., Shi, J., Zhou, W., Wang, L., Fang, W., et al. (2020b). M1-like macrophage-derived exosomes suppress angiogenesis and exacerbate Cardiac Dysfunction in a Myocardial Infarction Microenvironment. Basic Res. Cardiol. 115 (2), 22. doi:10.1007/s00395-020-0781-7
Liu, S., Lu, C., Liu, Y., Zhou, X., Sun, L., Gu, Q., et al. (2018). Hyperbaric Oxygen Alleviates the Inflammatory Response Induced by LPS Through Inhibition of NF-κB/MAPKs-CCL2/CXCL1 Signaling Pathway in Cultured Astrocytes. Inflammation 41 (6), 2003–2011. doi:10.1007/s10753-018-0843-2
Liu, Y., Wang, Z., Xiao, W., and Li, Y. (2014). Use of Gated Myocardial Perfusion Imaging to Assess Clinical Value of Xinmailong Injection In Chronic Congestive Heart Failure. J. traditional Chin. Med. 34 (5), 555–559. doi:10.1016/s0254-6272(15)30062-5
Liu, Y., Xu, J., Wu, M., Kang, L., and Xu, B. (2020a). The effector Cells and Cellular Mediators of Immune System Involved In Cardiac Inflammation And Fibrosis After Myocardial Infarction. J. Cell. physiology 235 (12), 8996–9004. doi:10.1002/jcp.29732
Lu, T. C., Wu, Y. H., Chen, W. Y., and Hung, Y. C. (2022b). Targeting Oxidative Stress and Endothelial Dysfunction Using Tanshinone IIA for the Treatment of Tissue Inflammation and Fibrosis. Oxidative Med. Cell. Longev. 2022, 2811789. doi:10.1155/2022/2811789
Lu, W., Wang, Q., Sun, X., He, H., Wu, Y., Liu, Y., et al. (2019). Qishen Granule Improved Cardiac Remodeling via Balancing M1 and M2 Macrophages. Front. Pharmacol. 10, 1399. doi:10.3389/fphar.2019.01399
Lu, X., Yao, J., Li, C., Cui, L., Liu, Y., Liu, X., et al. (2022a). Shexiang Tongxin Dropping Pills Promote Macrophage Polarization-Induced Angiogenesis Against Coronary Microvascular Dysfunction via PI3K/Akt/mTORC1 Pathway. Front. Pharmacol. 13, 840521. doi:10.3389/fphar.2022.840521
Lu, Y., Chu, X., Zhang, J., Zhao, Y., Jin, C., Zhu, J., et al. (2020). Effect of Shexiang Tongxin Dropping Pill on Stable Coronary Artery Disease Patients With Normal Fractional Flow Reserve and Coronary Microvascular Disease: A Study Protocol. Med. Baltim. 99 (38), e22126. doi:10.1097/MD.0000000000022126
Lv, J., Chen, J., Wang, M., and Yan, F. (2020). Klotho alleviates Indoxyl Sulfate-Induced Heart Failure And Kidney Damage By Promoting M2 macrophage polarization. Aging 12 (10), 9139–9150. doi:10.18632/aging.103183
Malmborg, M., Assad Turky Al-Kahwa, A., Kober, L., Torp-Pedersen, C., Butt, J. H., Zahir, D., et al. (2023). Specialized Heart Failure Clinics Versus Primary Care: Extended Registry-Based Follow-Up Of The Northstar Trial. PLoS One 18 (6), e0286307. doi:10.1371/journal.pone.0286307
McDonagh, T. A., Metra, M., Adamo, M., Gardner, R. S., Baumbach, A., Böhm, M., et al. (2021). 2021 ESC Guidelines for the diagnosis and treatment of acute and chronic heart failure. Eur. heart J. 42 (36), 3599–3726. doi:10.1093/eurheartj/ehab368
McDonagh, T. A., Metra, M., Adamo, M., Gardner, R. S., Baumbach, A., Burri, H., et al. (2024). 2023 Focused Update of the 2021 ESC Guidelines for the Diagnosis and Treatment of Acute And Chronic Heart Failure: Developed By The Task Force for The Diagnosis And Treatment of Acute And Chronic Heart Failure of the European Society of Cardiology (ESC) With the special contribution of the Heart Failure Association (HFA) of the ESC. Eur. J. heart Fail. 26 (1), 5–17. doi:10.1002/ejhf.3024
Ming-Chin Lee, K., Achuthan, A. A., De Souza, D. P., Lupancu, T. J., Binger, K. J., Lee, M. K. S., et al. (2022). Type I interferon Antagonism of the JMJD3-IRF4 Pathway Modulates Macrophage Activation and Polarization. Cell. Rep. 39 (3), 110719. doi:10.1016/j.celrep.2022.110719
Moreira Lopes, T. C., Mosser, D. M., and Gonçalves, R. (2020). Macrophage Polarization In Intestinal Inflammation and Gut Homeostasis. Inflamm. Res. 69 (12), 1163–1172. doi:10.1007/s00011-020-01398-y
Mosser, D. M., and Edwards, J. P. (2008). Exploring the Full Spectrum of Macrophage Activation. Nat. Rev. Immunol. 8 (12), 958–969. doi:10.1038/nri2448
Mouton, A. J., Li, X., Hall, M. E., and Hall, J. E. (2020). Obesity, Hypertension, and Cardiac Dysfunction: Novel Roles of Immunometabolism in Macrophage Activation and Inflammation. Circulation Res. 126 (6), 789–806. doi:10.1161/CIRCRESAHA.119.312321
Murray, P. J., and Wynn, T. A. (2011). Protective and pathogenic functions of macrophage subsets. Nat. Rev. Immunol. 11 (11), 723–737. doi:10.1038/nri3073
Mussbacher, M., Derler, M., Basílio, J., and Schmid, J. A. (2023). NF-κB in Monocytes And Macrophages - An Inflammatory Master Regulator In Multitalented Immune Cells. Front. Immunol. 14, 1134661. doi:10.3389/fimmu.2023.1134661
Nahrendorf, M., and Swirski, F. K. (2013). Monocyte and Macrophage Heterogeneity in the Heart. Circulation Res. 112 (12), 1624–1633. doi:10.1161/CIRCRESAHA.113.300890
Nahrendorf, M., Swirski, F. K., Aikawa, E., Stangenberg, L., Wurdinger, T., Figueiredo, J. L., et al. (2007). The healing myocardium Sequentially Mobilizes Two Monocyte Subsets with Divergent and Complementary Functions. J. Exp. Med. 204 (12), 3037–3047. doi:10.1084/jem.20070885
Ni, S. H., Sun, S. N., Zhou, Z., Li, Y., Huang, Y. S., Li, H., et al. (2020). Arctigenin Alleviates Myocardial Infarction Injury Through Inhibition of the NFAT5-related inflammatory Phenotype of Cardiac Macrophages/Monocytes in Mice. Lab. Investig. 100 (4), 527–541. doi:10.1038/s41374-019-0340-8
Nielsen, S. H., Mouton, A. J., DeLeon-Pennell, K. Y., Genovese, F., Karsdal, M., and Lindsey, M. L. (2019). Understanding cardiac Extracellular Matrix Remodeling To Develop Biomarkers Of Myocardial Infarction outcomes. Matrix Biol. 75-76, 43–57. doi:10.1016/j.matbio.2017.12.001
Ovchinnikov, A. G., Arefieva, T. I., Potekhina, A. V., Filatova, A. Y., Ageev, F. T., and Boytsov, S. A. (2020). The Molecular and Cellular Mechanisms Associated with a Microvascular Inflammation in the Pathogenesis of Heart Failure with Preserved Ejection Fraction. Acta Naturae 12 (2), 40–51. doi:10.32607/actanaturae.10990
Park, B. S., and Lee, J. O. (2013). Recognition of lipopolysaccharide pattern by TLR4 complexes. Exp. Mol. Med. 45 (12), e66. doi:10.1038/emm.2013.97
Park, J. E., and Han, J. S. (2024). Improving the Effect of Ferulic Acid on Inflammation and Insulin Resistance by Regulating the JNK/ERK and NF-κB Pathways in TNF-α-Treated 3T3-L1 Adipocytes. Nutrients 16 (2), 294. doi:10.3390/nu16020294
Pitt, B., Anker, S. D., Bushinsky, D. A., Kitzman, D. W., Zannad, F., Huang, I. Z., et al. (2011). Evaluation of the efficacy and safety of RLY5016, a Polymeric Potassium Binder, In A Double-Blind, Placebo-Controlled Study In Patients with Chronic Heart Failure (the PEARL-HF) Trial. Eur. Heart J. 32 (7), 820–828. doi:10.1093/eurheartj/ehq502
Rhee, A. J., and Lavine, K. J. (2020). New Approaches to Target Inflammation in Heart Failure: Harnessing Insights from Studies of Immune Cell Diversity. Annu. Rev. Physiology 82, 1–20. doi:10.1146/annurev-physiol-021119-034412
Ruytinx, P., Proost, P., Van Damme, J., and Struyf, S. (2018). Chemokine-Induced Macrophage Polarization in Inflammatory Conditions. Front. Immunol. 9, 1930. doi:10.3389/fimmu.2018.01930
Ryu, S., Sidorov, S., Ravussin, E., Artyomov, M., Iwasaki, A., Wang, A., et al. (2022). The Matricellular Protein SPARC Induces Inflammatory Interferon-Response In Macrophages During Aging. Immunity 55 (9), 1609–1626.e7. doi:10.1016/j.immuni.2022.07.007
Savarese, G., Becher, P. M., Lund, L. H., Seferovic, P., Rosano, G. M. C., and Coats, A. J. S. (2023). Global Burden of Heart Failure: A Comprehensive And Updated Review of Epidemiology. Cardiovasc Res. 118 (17), 3272–3287. doi:10.1093/cvr/cvac013
Savarese, G., Lindberg, F., Christodorescu, R. M., Ferrini, M., Kumler, T., Toutoutzas, K., et al. (2024). Physician perceptions, attitudes, and Strategies Towards Implementing Guideline-Directed Medical Therapy In Heart Failure with Reduced Ejection Fraction. A Survey of the Heart Failure Association of the ESC and the ESC Council for Cardiology Practice. Eur. J. Heart Fail 26, 1408–1418. doi:10.1002/ejhf.3214
Serini, G., Bochaton-Piallat, M. L., Ropraz, P., Geinoz, A., Borsi, L., Zardi, L., et al. (1998). The fibronectin domain ED-A is Crucial for Myofibroblastic Phenotype Induction by Transforming Growth Factor-Beta1. J. Cell. Biol. 142 (3), 873–881. doi:10.1083/jcb.142.3.873
Shapouri-Moghaddam, A., Mohammadian, S., Vazini, H., Taghadosi, M., Esmaeili, S. A., Mardani, F., et al. (2018). Macrophage Plasticity, Polarization, and Function in Health and Disease. J. Cell. physiology 233 (9), 6425–6440. doi:10.1002/jcp.26429
Shi, L. P., Deng, J. W., Yin, E. Q., Chen, X., and Du, X. Q. (2023). Effect of Fangji Fuling Decoction on Macrophage Polarization and Oxidative Stress in mice with Myocardial Fibrosis. J. Chin. Exp. Formulae. 29 (24), 11–18. doi:10.13422/j.cnki.syfjx.20231112
Shirakawa, K., Endo, J., Kataoka, M., Katsumata, Y., Yoshida, N., Yamamoto, T., et al. (2018). IL (Interleukin)-10-STAT3-Galectin-3 Axis Is Essential for Osteopontin-Producing Reparative Macrophage Polarization After Myocardial Infarction. Circulation 138 (18), 2021–2035. doi:10.1161/CIRCULATIONAHA.118.035047
Shirakawa, T., Kawazoe, Y., Tsujikawa, T., Jung, D., Sato, S. i., and Uesugi, M. (2011). Deactivation of STAT6 through serine 707 Phosphorylation by JNK. J. Biol. Chem. 286 (5), 4003–4010. doi:10.1074/jbc.M110.168435
Solomon, S. D., Vaduganathan, M., L Claggett, B., Packer, M., Zile, M., Swedberg, K., et al. (2020). Sacubitril/Valsartan Across the Spectrum of Ejection Fraction in Heart Failure. Circulation 141 (5), 352–361. doi:10.1161/CIRCULATIONAHA.119.044586
Stavrakis, S., Kulkarni, K., Singh, J. P., Katritsis, D. G., and Armoundas, A. A. (2020). Autonomic Modulation of Cardiac Arrhythmias: Methods to Assess Treatment and Outcomes. JACC Clin. Electrophysiol. 6 (5), 467–483. doi:10.1016/j.jacep.2020.02.014
Sugita, J., and Fujiu, K. (2019). Emerging Function of Cardiac Macrophages Ushers in a New Era for the Electrophysiology of the Heart. Circ. Rep. 1 (12), 558–563. doi:10.1253/circrep.CR-19-0103
Suthahar, N., Meijers, W. C., Silljé, H. H. W., Ho, J. E., Liu, F. T., and de Boer, R. A. (2018). Galectin-3 Activation and Inhibition in Heart Failure and Cardiovascular Disease: An Update. Theranostics 8 (3), 593–609. doi:10.7150/thno.22196
Suzuki, A., Minamide, M., Iwaya, C., Ogata, K., and Iwata, J. (2020). Role of Metabolism in Bone Development and Homeostasis. Int. J. Mol. Sci. 21 (23), 8992. doi:10.3390/ijms21238992
Swirski, F. K., and Nahrendorf, M. (2018). Cardioimmunology: the immune system in cardiac homeostasis and disease. Nat. Rev. Immunol. 18 (12), 733–744. doi:10.1038/s41577-018-0065-8
Swirski, F. K., Nahrendorf, M., Etzrodt, M., Wildgruber, M., Cortez-Retamozo, V., Panizzi, P., et al. (2009). Identification of splenic reservoir Monocytes and their deployment to Inflammatory sites. Science. 325 (5940), 612–616. doi:10.1126/science.1175202
Takaesu, G., Kishida, S., Hiyama, A., Yamaguchi, K., Shibuya, H., Irie, K., et al. (2000). TAB2, A Novel Adaptor Protein, Mediates Activation of TAK1 MAPKKK by linking TAK1 to TRAF6 in the IL-1 signal transduction pathway. Mol. Cell. 5 (4), 649–658. doi:10.1016/s1097-2765(00)80244-0
Tengbom, J., Cederström, S., Verouhis, D., Böhm, F., Eriksson, P., Folkersen, L., et al. (2021). Arginase 1 is Upregulated at Admission in patients with ST-elevation myocardial Infarction. J. Intern. Med. 290 (5), 1061–1070. doi:10.1111/joim.13358
Tian, L., Yu, Q., Liu, D., Chen, Z., Zhang, Y., Lu, J., et al. (2022a). Epithelial-mesenchymal Transition of Peritoneal Mesothelial Cells Is Enhanced by M2c Macrophage Polarization. Immunol. Investig. 51 (2), 301–315. doi:10.1080/08820139.2020.1828911
Tian, Y., Liu, B., Li, Y., Zhang, Y., Shao, J., Wu, P., et al. (2022b). Activation of RARα Receptor Attenuates Neuroinflammation After SAH via Promoting M1-to-M2 Phenotypic Polarization of Microglia and Regulating Mafb/Msr1/PI3K-Akt/NF-κB Pathway. Front. Immunol. 13, 839796. doi:10.3389/fimmu.2022.839796
Tili, E., Chiabai, M., Palmieri, D., Brown, M., Cui, R., Fernandes, C., et al. (2015). Quaking and miR-155 Interactions in Inflammation and Leukemogenesis. Oncotarget 6 (28), 24599–24610. doi:10.18632/oncotarget.5248
Toba, H., Cannon, P. L., Yabluchanskiy, A., Iyer, R. P., D'Armiento, J., and Lindsey, M. L. (2017). Transgenic Overexpression of Macrophage Matrix metalloproteinase-9 Exacerbates Age-Related Cardiac Hypertrophy, Vessel Rarefaction, Inflammation, and Fibrosis. Am. J. physiology. Heart circulatory physiology 312 (3), H375–H383. doi:10.1152/ajpheart.00633.2016
Torre-Amione, G. (2005). Immune Activation in Chronic Heart Failure. Am. J. Cardiol. 95 (11A), 3C–40C. doi:10.1016/j.amjcard.2005.03.006
Trevisan, M., Fu, E. L., Xu, Y., Savarese, G., Dekker, F. W., Lund, L. H., et al. (2021). Stopping Mineralocorticoid Receptor Antagonists After Hyperkalaemia: Trial Emulation In Data From Routine Care. Eur. J. Heart Fail 23 (10), 1698–1707. doi:10.1002/ejhf.2287
Tromp, J., Ouwerkerk, W., van Veldhuisen, D. J., Hillege, H. L., Richards, A. M., van der Meer, P., et al. (2022). A Systematic Review and Network Meta-Analysis of Pharmacological Treatment of Heart Failure with Reduced Ejection Fraction. JACC. Heart Fail. 10 (4), 73–84. doi:10.1016/j.jchf.2021.09.004
Tsai, C. F., Chen, G. W., Chen, Y. C., Shen, C. K., Lu, D. Y., Yang, L. Y., et al. (2021). Regulatory Effects of Quercetin on M1/M2 Macrophage Polarization and Oxidative/Antioxidative Balance. Nutrients 14 (1), 67. doi:10.3390/nu14010067
Tsou, C. L., Peters, W., Si, Y., Slaymaker, S., Aslanian, A. M., Weisberg, S. P., et al. (2007). Critical roles for CCR2 and MCP-3 in Monocyte Mobilization From Bone Marrow and Recruitment to Inflammatory Sites. J. Clin. investigation 117 (4), 902–909. doi:10.1172/JCI29919
Usher, M. G., Duan, S. Z., Ivaschenko, C. Y., Frieler, R. A., Berger, S., Schütz, G., et al. (2010). Myeloid Mineralocorticoid Receptor Controls Macrophage Polarization and Cardiovascular Hypertrophy and Remodeling in mice. J. Clin. investigation 120 (9), 3350–3364. doi:10.1172/JCI41080
Vaduganathan, M., Claggett, B. L., Jhund, P. S., Cunningham, J. W., Pedro Ferreira, J., Zannad, F., et al. (2020). Estimating Lifetime Benefits of Comprehensive Disease-Modifying Pharmacological Therapies In Patients With Heart Failure With Reduced Ejection Fraction: A Comparative Analysis Of Three Randomised Controlled Trials. Lancet 396 (10244), 121–128. doi:10.1016/S0140-6736(20)30748-0
Vaduganathan, M., Docherty, K. F., Claggett, B. L., Jhund, P. S., de Boer, R. A., Hernandez, A. F., et al. (2022). SGLT-2 Inhibitors in Patients With Heart Failure: A Comprehensive Meta-Analysis Of Five Randomised Controlled Trials. Lancet (London, Engl.) 400 (10354), 757–767. doi:10.1016/S0140-6736(22)01429-5
Van den Bossche, J., O'Neill, L. A., and Menon, D. (2017). Macrophage Immunometabolism: Where Are We (Going)? Trends Immunol. 38 (6), 395–406. doi:10.1016/j.it.2017.03.001
van der Pol, A., van Gilst, W. H., Voors, A. A., and van der Meer, P. (2019). Treating Oxidative Stress In Heart Failure: Past, Present and Future. Eur. J. heart Fail. 21 (4), 425–435. doi:10.1002/ejhf.1320
Vergadi, E., Ieronymaki, E., Lyroni, K., Vaporidi, K., and Tsatsanis, C. (2017). Akt Signaling Pathway in Macrophage Activation and M1/M2 Polarization. J. Immunol. 198 (3), 1006–1014. doi:10.4049/jimmunol.1601515
Vieceli Dalla Sega, F., Fortini, F., Aquila, G., Campo, G., Vaccarezza, M., and Rizzo, P. (2019). Notch Signaling Regulates Immune Responses in Atherosclerosis. Front. Immunol. 10, 1130. doi:10.3389/fimmu.2019.01130
Wan, E., Yeap, X. Y., Dehn, S., Terry, R., Novak, M., Zhang, S., et al. (2013). Enhanced Efferocytosis of Apoptotic Cardiomyocytes Through Myeloid-Epithelial-Reproductive Tyrosine Kinase Links Acute Inflammation Resolution to Cardiac Repair After Infarction. Circulation Res. 113 (8), 1004–1012. doi:10.1161/CIRCRESAHA.113.301198
Wang, L., Zhang, S., Wu, H., Rong, X. L., and Guo, J. (2019). M2b Macrophage Polarization and its Roles in Diseases. J. Leukoc. Biol. 106 (2), 345–358. doi:10.1002/JLB.3RU1018-378RR
Wang, M., and Lu, S. (2015). Clinical Observation on the Intervention of Fangji Poria Decoction on Patients with Chronic Heart Failure (heart-qi Yang Deficiency Syndrome). J. Emerg. Traditional Chin. Med. 24 (02), 355–357.
Wang, M., Shan, Y., Wu, C., Cao, P., Sun, W., Han, J., et al. (2021). Efficacy and Safety of Qishen Yiqi Dripping Pill for Heart Failure with Preserved Ejection Fraction: A Systematic Review and Meta-Analysis. Front. Pharmacol. 11, 626375. doi:10.3389/fphar.2020.626375
Wang, X., Gaur, M., Mounzih, K., Rodriguez, H. J., Qiu, H., Chen, M., et al. (2023). Inhibition of Galectin-3 Post-Infarction Impedes Progressive Fibrosis By Regulating Inflammatory Profibrotic Cascades. Cardiovasc. Res. 119 (15), 2536–2549. doi:10.1093/cvr/cvad116
Wang, X., Wang, Q., Li, W., Zhang, Q., Jiang, Y., Guo, D., et al. (2020). TFEB-NF-κB Inflammatory Signaling Axis: A Novel Therapeutic Pathway Of Dihydrotanshinone I in Doxorubicin-Induced Cardiotoxicity. J. Exp. Clin. Cancer Res. 39 (1), 93. doi:10.1186/s13046-020-01595-x
Wang, Y., Yan, X., Mi, S., Li, Z., Zhu, H., Sun, X., et al. (2017). Naoxintong Attenuates Ischaemia/Reperfusion Injury Through Inhibiting Nlrp3 Inflammasome Activation. J. Celluar Mol. Med. 21 (1), 4–12. doi:10.1111/jcmm.12915
Wang, Y. C., Shao, Y. D., Shao, C. L., Guan, X. Q., Lu, P. P., Ning, K., et al. (2024). Dihydrotanshinone I Reduces H9c2 Cell Damage by Regulating AKT and MAPK Signaling Pathways. Vitro Cell. Dev. Biology-animal 60 (1), 89–97. doi:10.1007/s11626-023-00839-2
Wu, G., and Hu, L. (2019). Effects of Shexiang Tongxin dropping Pills Combined With Trimetazidine Hydrochloride on Neurohormone-Cytokines And Left Ventricular Remodeling In Patients With Ischemic Heart Failure. Liaoning J. Traditional Chin. Med. 46 (04), 677–680. doi:10.13192/j.issn.1000-1719.2019.04.002
Wu, Y., Li, S., Li, Z., Mo, Z., Luo, Z., Li, D., et al. (2022). Efficacy and Safety of Shenfu Injection for The Treatment of Post-Acute Myocardial Infarction Heart Failure: A Systematic Review And Meta-Analysis. Front. Pharmacol. 13, 1027131. doi:10.3389/fphar.2022.1027131
Xu, G. R., Zhang, C., Yang, H. X., Sun, J. H., Zhang, Y., Yao, T. T., et al. (2020a). Modified Citrus Pectin Ameliorates Myocardial Fibrosis And Inflammation Via Suppressing Galectin-3 and TLR4/MyD88/NF-κB Signaling Pathway. Biomed. Pharmacother. 126, 110071. doi:10.1016/j.biopha.2020.110071
Xu, H., Zhu, J., Smith, S., Foldi, J., Zhao, B., Chung, A. Y., et al. (2012). Notch-RBP-J signaling regulates the transcription factor IRF8 to promote inflammatory macrophage polarization. Nat. Immunol. 13 (7), 642–650. doi:10.1038/ni.2304
Xu, X., Piao, H. N., Aosai, F., Zeng, X. Y., Cheng, J. H., Cui, Y. X., et al. (2020b). Arctigenin Protects Against Depression By Inhibiting Microglial Activation and Neuroinflammation via HMGB1/TLR4/NF-κB and TNF-α/TNFR1/NF-κB Pathways. Br. J. Pharmacol. 177 (22), 5224–5245. doi:10.1111/bph.15261
Yamaguchi, O. (2019). Autophagy in the Heart. Circulation J. 83 (4), 697–704. doi:10.1253/circj.CJ-18-1065
Yan, S., Zhou, M., Zheng, X., Xing, Y., Dong, J., Yan, M., et al. (2021). Anti-Inflammatory Effect of Curcumin on the Mouse Model of Myocardial Infarction through Regulating Macrophage Polarization. Mediat. Inflamm. 2021, 9976912. doi:10.1155/2021/9976912
Yang, M., Tan, Y. Q., Zhang, J. Y., Zhang, Y., Su, L. Q., Hu, Z. X., et al. (2024) Shenfu Injection Reduces Inflammation In Chronic Heart Failure by Regulating Macrophage Polarization through TLR4/NF-κB Pathwa. Chin. J. traditional Chin. Med. 13, 810587. doi:10.19540/j.cnki.cjcmm.20240305.401
Yang, M., Xiong, J., Zou, Q., Wang, X., Hu, K., and Zhao, Q. (2022). Sinapic Acid Attenuated Cardiac Remodeling After Myocardial Infarction by Promoting Macrophage M2 Polarization Through the PPARγ Pathway. Front. Cardiovasc. Med. 9, 915903. doi:10.3389/fcvm.2022.915903
Yang, X., Cui, L., White, J., Kuck, J., Ruchko, M. V., Wilson, G. L., et al. (2015). Mitochondrially Targeted Endonuclease III has a Powerful anti-Infarct Effect in an in vivo rat Model of Myocardial Ischemia/Reperfusion. Basic Res. Cardiol. 110 (2), 3. doi:10.1007/s00395-014-0459-0
Yang, Z., Mei, C., He, X., and Sun, X. (2013). Advance in studies on Chemical Constitutions, Pharmacological Mechanism and Pharmacokinetic profile of Dalbergiae Odoriferae Lignum. China J. Chin. Materia Medica 38 (11), 1679–1683.
Yao, Y. L., Xu, X. H., and Jin, L. (2019). Macrophage Polarization in Physiological and Pathological Pregnancy. Front. Immunol. 10, 792. doi:10.3389/fimmu.2019.00792
Ye, T. C., Wang, L. J., Yang, Z. Q., Wu, H., Chen, H. Y., Xian, S. X., et al. (2019). Effect of Xinyin Tablet on Cardiopulmonary Function Of Patients With Heart Failure of Qi Yin Deficiency and blood stasis and water stopping typy. Traditional Chin. Drugs Res. Clin. Pharmacol. 30 (09), 1129–1132. doi:10.19378/j.issn.1003-9783.2019.09.018
Yin, J., Hu, T., Xu, L., Zhang, L., Zhu, J., Ye, Y., et al. (2023). Hsa_circRNA_103124 upregulation in Crohn's disease promoted macrophage M1 Polarization To Maintain an Inflammatory Microenvironment Via Activation Of The AKT2 and TLR4/NF-κB Pathways. Int. Immunopharmacol. 123, 110763. doi:10.1016/j.intimp.2023.110763
Yunna, C., Mengru, H., Lei, W., and Weidong, C. (2020). Macrophage M1/M2 Polarization. Eur. J. Pharmacol. 877, 173090. doi:10.1016/j.ejphar.2020.173090
Zaidi, Y., Aguilar, E. G., Troncoso, M., Ilatovskaya, D. V., and DeLeon-Pennell, K. Y. (2021). Immune regulation of Cardiac Fibrosis Post Myocardial Infarction. Cell. Signal. 77, 109837. doi:10.1016/j.cellsig.2020.109837
Zaman, R., Hamidzada, H., Kantores, C., Wong, A., Dick, S. A., Wang, Y., et al. (2021). Selective Loss Of Resident Macrophage-Derived Insulin-Like Growth Factor-1 Abolishes Adaptive Cardiac Growth to Stress. Immunity 54 (9), 2057–2071.e6. doi:10.1016/j.immuni.2021.07.006
Zeng, H., Vaka, V. R., He, X., Booz, G. W., and Chen, J. X. (2015). High-fat diet Induces Cardiac Remodelling and Dysfunction: Assessment of the Role Played by SIRT3 loss. J. Cell. Mol. Med. 19 (8), 1847–1856. doi:10.1111/jcmm.12556
Zha, Z., Cheng, Y., Cao, L., Qian, Y., Liu, X., Guo, Y., et al. (2021). Monomeric CRP Aggravates Myocardial Injury After Myocardial Infarction by Polarizing the Macrophage to Pro-Inflammatory Phenotype Through JNK Signaling Pathway. J. Inflamm. Res. 14, 7053–7064. doi:10.2147/JIR.S316816
Zhang, D., Hu, W., Tu, H., Hackfort, B. T., Duan, B., Xiong, W., et al. (2021b). Macrophage Depletion in Stellate Ganglia Alleviates Cardiac Sympathetic Overactivation and Ventricular Arrhythmogenesis by Attenuating Neuroinflammation in Heart Failure. Basic Res. Cardiol. 116 (1), 28. doi:10.1007/s00395-021-00871-x
Zhang, D., Tang, Z., Huang, H., Zhou, G., Cui, C., Weng, Y., et al. (2019b). Metabolic Regulation of Gene Expression by Histone Lactylation. Nature 574 (7779), 575–580. doi:10.1038/s41586-019-1678-1
Zhang, H., Li, Z., and Li, W. (2021a). M2 Macrophages Serve as Critical Executor of Innate Immunity in Chronic Allograft Rejection. Front. Immunol. 12, 648539. doi:10.3389/fimmu.2021.648539
Zhang, N., Shou, B., Chen, L., Lai, X., Luo, Y., Meng, X., et al. (2020a). Cardioprotective Effects of Latifolin Against Doxorubicin-Induced Cardiotoxicity by Macrophage Polarization in Mice. J. Cardiovasc. Pharmacol. 75 (6), 564–572. doi:10.1097/FJC.0000000000000827
Zhang, S., Weinberg, S., DeBerge, M., Gainullina, A., Schipma, M., Kinchen, J. M., et al. (2019a). Efferocytosis Fuels Requirements of Fatty Acid Oxidation and the Electron Transport Chain to Polarize Macrophages for Tissue Repair. Cell. Metab. 29 (2), 443–456. doi:10.1016/j.cmet.2018.12.004
Zhang, T., Yang, W. X., Wang, Y. L., Yuan, J., Qian, Y., Sun, Q. M., et al. (2020b). Electroacupuncture Preconditioning Attenuates Acute Myocardial Ischemia Injury Through Inhibiting NLRP3 Inflammasome Activation in mice. Life Sci. 248, 117451. doi:10.1016/j.lfs.2020.117451
Zhao, M., Li, F., Jian, Y., Wang, X., Yang, H., Wang, J., et al. (2020). Salvianolic Acid B regulates Macrophage Polarization in Ischemic/Reperfused Hearts by Inhibiting mTORC1-Induced Glycolysis. Eur. J. Pharmacol. 871, 172916. doi:10.1016/j.ejphar.2020.172916
Zhou, J., Chen, S., Xu, M., Guo, Y., Xia, X., Qin, Q., et al. (2023). Adjuvant Treatment With Shenfu Injection Improve Quality of Life in Chronic Heart failure Patients: A Meta-Analysis of Randomized Controlled Trials. Heliyon 9 (10), e20594. doi:10.1016/j.heliyon.2023.e20594
Zhou, J., Liu, W., Zhao, X., Xian, Y., Wu, W., Zhang, X., et al. (2021). Natural Melanin/Alginate Hydrogels Achieve Cardiac Repair through ROS Scavenging and Macrophage Polarization. Adv. Sci. 8 (20), e2100505. doi:10.1002/advs.202100505
Zhou, Y., Zhang, T., Wang, X., Wei, X., Chen, Y., Guo, L., et al. (2015). Curcumin Modulates Macrophage Polarization Through the Inhibition of the Toll-Like Receptor 4 Expression and its Signaling Pathways. Cell Physiology Biochem. 36 (2), 631–641. doi:10.1159/000430126
Keywords: macrophage polarization, heart failure, traditional Chinese medicine, mechanisms, research progress
Citation: Zhu Z, Wang M, Lu S, Dai S and Liu J (2024) Role of macrophage polarization in heart failure and traditional Chinese medicine treatment. Front. Pharmacol. 15:1434654. doi: 10.3389/fphar.2024.1434654
Received: 21 May 2024; Accepted: 01 July 2024;
Published: 18 July 2024.
Edited by:
Wei Peng, Chengdu University of Traditional Chinese Medicine, ChinaReviewed by:
Shihao Zheng, Beijing University of Chinese Medicine, ChinaJunping Zhang, First Teaching Hospital of Tianjin University of Traditional Chinese Medicine, China
Copyright © 2024 Zhu, Wang, Lu, Dai and Liu. This is an open-access article distributed under the terms of the Creative Commons Attribution License (CC BY). The use, distribution or reproduction in other forums is permitted, provided the original author(s) and the copyright owner(s) are credited and that the original publication in this journal is cited, in accordance with accepted academic practice. No use, distribution or reproduction is permitted which does not comply with these terms.
*Correspondence: Jianhe Liu, bGpodGNtMUAxNjMuY29t