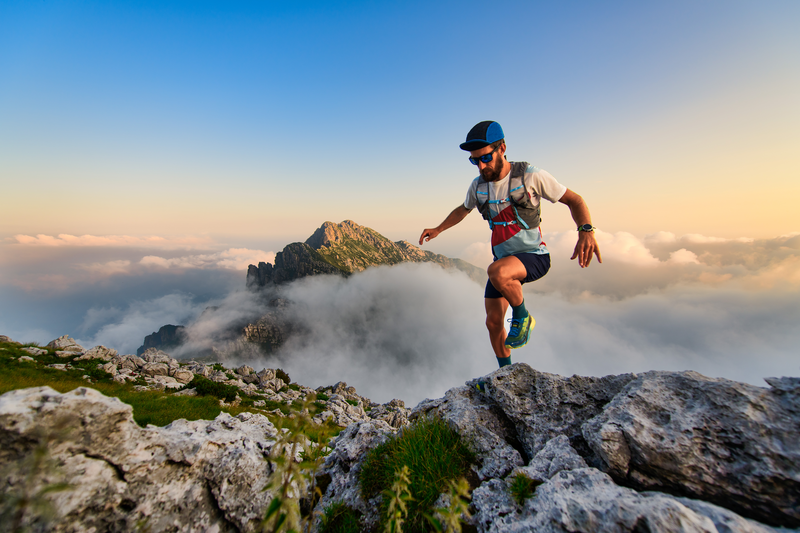
94% of researchers rate our articles as excellent or good
Learn more about the work of our research integrity team to safeguard the quality of each article we publish.
Find out more
ORIGINAL RESEARCH article
Front. Pharmacol. , 02 August 2024
Sec. Experimental Pharmacology and Drug Discovery
Volume 15 - 2024 | https://doi.org/10.3389/fphar.2024.1429423
Introduction: The antibacterial protein PAG14 was extracted from a metabolite of Bacillus G14 isolated from mangrove plants.
Methods: In this study, Pseudomonas aeruginosa, Pasteurell multocide, Enterobacter aerogenes, and Enterococcus faecalis were used as indicator bacteria to screen endophytes that exhibited antibacterial activity. The endophyte culture conditions were optimized to enhance productivity. Subsequently, the culture supernatant was salted using ammonium sulfate, followed by purification using dextran gel chromatography and ion exchange column techniques. Finally, the structures of antibacterial proteins were identified using mass spectrometry.
Results and Discussion: The optimal culture conditions for Bacillus G14 were 2% mannitol, 0.5% fish peptone, 0.05% KH2PO4 + 0.05% K2HPO4 + 0.025% MnSO4·H2O. The antibacterial substances exhibited stability within the temperature range of 30–40°C and pH range of 5.0–7.0, while displaying sensitivity toward enzymes. The antibacterial activity decreased as the duration of UV irradiation increased. The antibacterial protein PAG14, isolated from the culture broth of Bacillus G14 through purification using dextran gel and ion-exchange columns, was identified as a class III bacteriocin using LC-MS/MS, similar to Lysozyme C. These findings serve as a theoretical foundation for the investigation and application of bacteriocins in food products.
Endophytic bacteria mainly refer to endophytic bacteria in plant tissues, organs, and intercellular spaces. The metabolic pathways unique to plant endophytes has been demonstrated to facilitate the production of a plethora of bioactive substances with novel structures and potent antibacterial effects, thereby serving as a valuable resource for the development of novel antibacterial drugs (Jouda et al., 2016). Bacillus species are widely distributed and can be found in various sources, including soil, water, air, and animal intestines, showing diversity and universality (Gond et al., 2015). Senadeera et al. (2012) first proposed the method of “OSMAC,” by changing the culture conditions biologically or abiotically to activate silencing biosynthetic gene clusters to produce new metabolites, secondary metabolites of more structural types were obtained. Bacteriocins are a class of antimicrobial proteins or polypeptides synthesized by bacterial ribosomes (Heilbronner et al., 2021). The secretion of bacteriocins varies among different Bacillus strains, exhibiting diversity not only in terms of molecular weight, but also in morphological structure and physical and chemical properties. Bacillus bacteriocins are another major class of bacteriocins, following Lactobacillus bacteriocins (Abriouel et al., 2011), and numerous bacteriocins have been discovered to date (Mercado and Olmos, 2022). For instance, Bacillus cereus can secrete various types of bacteria, such as cerein 7, cerein 7 B, and cerein MRX1 (Oscáriz and Pisabarro, 2000; Bizani and Brandelli, 2002; Oscáriz et al., 2006; Sebei et al., 2007). The literature shows that the isolation and culture conditions of bacteriocin produced by Bacillus atrophicum and Bacillus amyloliquefaciens were optimized, which effectively increased the production of bacteriocin, and further expanded the understanding of the potential applicability of bacteriocin (Elazzazy et al., 2024). Bacillus bacteriocins exhibit non-toxic and innocuous properties and are highly effective in minimizing the likelihood of drug resistance development (Kumariya et al., 2019). Bacteriocins exhibit a broad antibacterial spectrum, exceptional thermal stability, and resistance under both acidic and alkaline conditions (Cotter et al., 2013; Özel et al., 2018).
At present, many different bacteriocins have been isolated from Bacillus sp., which have been reviewed (Basi-Chipalu et al., 2022; Vaca et al., 2022); however, there are deficiencies in their structure and stability of Bacillus bacteriocins. The stability study of Bacillus bacteriocins provided strong support for structural studies of Bacillus bacteriocins. In this study, strain G14 with a broad-spectrum antibacterial effect was isolated from mangrove plants and identified as Bacillus velezensis by whole gene sequencing analysis. The study had shown that the bactericidal activity of B. velezensis bacteriocin is wider than the other bacteriocins (Vaca et al., 2023). The culture conditions of the strain were optimized to determine the stability of active antibacterial substances. Bacillus G14 culture supernatant was purified using dextran gel chromatography and ion-exchange columns. The structure of the purified antibacterial protein was identified using mass spectrometry. The experimental scheme of this study was shown in Figure 1. It provides valuable theoretical references for subsequent applications (such as food, health supplements, animal husbandry, feed production, and aquaculture) as well as research and development.
To improve bacteriocin production, the following substances were selected for medium optimization according to a preliminary experiment. Glucose, mannitol, soluble starch, lactose, fructose, and sucrose were purchased from Shanghai Maclin Biotechnology Co., Ltd., China. Beef paste, peptone, casein peptone, fish peptone, beef powder, and tryptone were purchased from Beijing Aoboxing Biotechnology Co., Ltd., China. NaCl, MnSO4, CaCl2, KCl, MnSO4·H2O, NaH2PO4, Na2PO4, KH2PO4, K2HPO4 were produced by Shanghai Yien Chemical Technology Co., LTD., China.
Pseudomonas aeruginosa (CVCC393), Enterobacter aerogenes (ATCC13048), Enterococcus faecalis (ATCC29212), and Pasteurea multocida (ATCC9027) were used as indicator bacteria and purchased from Beijing Reserve Biotechnology Co., Ltd., China.
Bacteria were isolated from the tissues of mangrove plants, and endophyte G14 was screened for its antibacterial activity against P. aeruginosa, Pasteurella multocida, E. aerogenes and E. faecalis, was selected and identified as B. velezensis. Bacillus G14 was inoculated into LB medium and cultured overnight at 30°C, 180 r/min. It was added to various culture media at a 2% concentration and incubated for 24 h under the same conditions. Antibacterial effects were detected using the agar plate method. Indicator bacteria were introduced into the LB solid medium, and a sterile perforator was used. The supernatant of the culture medium was filtered by 0.22 μm filter membrane and added into the agar plate. The diameter of the inhibition zone was measured after culture at 28°C for 24 h.
The carbon source medium was composed of 10.0 g/L peptone, 3.0 g/L beef powder, and 5.0 g/L NaCl, while the basic medium was supplemented with 4% mannitol, lactose, fructose, sucrose, glucose, and soluble starch respectively.
The optimal carbon source, mannitol, was added to the nitrogen-source medium. The basal medium for the nitrogen source consisted of 40.0 g/L mannitol and 5.0 g/L NaCl, while the basic medium was enriched with 2% beef extract, peptone, casein peptone, fish peptone, beef powder, and tryptone individually.
The composition of the inorganic salt base medium was as follows: 40.0 g/L mannitol, 20.0 g/L fish peptone, and the basal medium was supplemented with 0.5% NaCl, 0.5% MnSO4, 0.5% CaCl2, 0.5% KCl, 0.5% MnSO4·H2O, 0.5% NaH2PO4, 0.5% Na2PO4, 0.5% KH2PO4, 0.5% K2HPO4, 0.25% NaH2PO4 + 0.25% Na2HPO, 0.2% KH2PO4 + 0.2% K2HPO4 + 0.1% MnSO4·H2O, 0.2% NaH2PO4 + 0.2% Na2HPO4 + 0.1% MnSO4·H2O, respectively.
The optimal carbon and nitrogen sources and inorganic salt were selected as the basic media. To optimize the amount of the carbon source additive, mannitol was added at mass volume fractions of 1%, 2%, 4%, 8%, and 16%. The optimal amount of fish peptone, a nitrogen source additive, was determined based on the concentrations of 0.5%, 1%, 2%, 4%, and 8%. The optimal amount of inorganic salt additive, consisting of KH2PO4 + K2HPO4 + MnSO4·H2O, was added based on the percentages of 0.1%, 0.2%, 0.5%, 1% and 2% for further optimization. The antibacterial activities of the culture supernatants were determined under identical experimental conditions. All experiments were conducted in triplicates.
The optimal amounts of carbon, nitrogen, and inorganic salts were determined based on the results of a single-factor experiment. Subsequently, an orthogonal experimental design was used to perform a three-factor, three-level analysis to select the optimal quantities of carbon, nitrogen, and inorganic salt additives. All experiments were conducted in triplicates.
The supernatant of the culture liquid was adjusted to pH values of 8.0, 9.0, 10.0, 11.0, 12.0, 13.0 and 14.0 using a solution of NaOH with a concentration of 1 mol/L, and to pH values of 1.0, 2.0, 3.0, 4.0, 5.0 and 6.0 using a solution of HCl with a concentration of 1 mol/L respectively. After incubation at a temperature of 4°C for a duration of 12 h, the pH was restored back to neutral, the antibacterial activity was detected.
The supernatant of the culture liquid was subjected to thermal treatments at 40°C, 60°C, 80°C, and 100°C for a duration of 30 min each, followed by a sterilization process at 121°C for an additional 30 min to detect the antibacterial activity.
The culture supernatant was treated with 1 mg/mL pepsin, trypsin, papain, or protease K. Subsequently, the antibacterial activity was detected following incubation in a water bath at 37°C for 1 h.
The supernatant of the culture liquid was exposed to a 15 W UV lamp at a distance of approximately 15 cm for 1, 2, 3, 4 and 5 h to detect antibacterial activity.
Bacillus G14 preserved at −80°C was inoculated into LB liquid medium and cultured at 30°C for 24 h as the first generation. The second generation was obtained by marking and culturing the LB solid culture at 30°C for 24 h. A single colony from the second generation was inoculated into the LB liquid medium to obtain a third generation. Following this method, the culture was transferred to the 11th generation. The 1st, 3rd, 5th, 7th, 9th, and 11th generations of bacteria were fermented and cultured to determine their antibacterial activities.
The supernatant of the culture liquid was stored at 4°C and −20°C, and 5 time points were set for each storage condition, which were 0, 3, 6, 9, and 12 days, respectively. The next step was to detect the antimicrobial activity over time. Samples were collected at different time points for analysis.
These tests were conducted in triplicate using the untreated culture broth supernatant as the positive control.
The optimized culture conditions for culturing Bacillus G14 included a liquid volume of 30 mL, an inoculation volume of 2%, a culture temperature of 30°C, and a culture time of 120 h. The culture solution was centrifuged at 4°C at 8,000 × g for 30 min and subsequently filtered using a 0.22 μm filter membrane. The sterile supernatant was packed into a 1 KDa dialysis bag and concentrated 10 times. The antibacterial activity of the concentrated culture supernatant was verified using the solid AGAR drilling method with Streptococcus as the indicator bacteria.
The supernatant was salted out with 50%, 60%, 70%, and 80% saturation ammonium sulfate respectively, centrifuged at 4°C at 8,000 × g for 30 min, and the precipitated proteins were collected. Ammonium sulfate was removed by dialysis. The retained molecular weight of the dialysis bag was 1 kDa, and the bag was placed in PBS (a phosphate-buffered saline (PBS) solution (pH 5.5 for dialysis. After dialysis, the antibacterial protein was filtered by 0.22 μm filter membrane. The solid AGAR drilling method was used to verify the antibacterial activity of the protein, and the optimal concentration of ammonium sulfate salting was determined based on different antibacterial effects.
The antibacterial proteins were purified using ACHROM Firin Plus [Taidu Biotechnology (Suzhou) Co., Ltd., China]. Using a Tideroe-GF75 gel chromatographic column with deionized water as the mobile phase equilibrium chromatography column. The crude protein extraction solution was sampled at a flow rate of 0.55 mL/min and detected at 280 nm, followed by the collection of protein isolates. The antibacterial activities of the different isolated proteins were verified using the solid AGAR drilling method.
A Titrap Q HP ion-exchange column was used to isolate and purify the antibacterial proteins. The purified column was equilibrated with 0.05 mol/L of sterile NaCl, and samples were collected at a flow rate of 3 mL/min for detection at 280 nm. The antibacterial activities of the different isolated proteins were verified using the solid AGAR drilling method.
Purified antibacterial protein PAG14 powder, which underwent vacuum freeze-drying, was sent to Beijing Beitapak Biotechnology Co., Ltd.
The samples were reduced and alkylated, followed by their addition to a dithiothreitol (DTT) solution to achieve a final concentration of 10 mmol/L. The samples underwent reduction in a water bath at 56°C for 1 h. The IAA (Iodoacetamide, IAA) solution was prepared at a final concentration of 50 mmol/L, and the reaction was allowed to proceed in the dark for 40 min. The samples were desalted using a self-filling column prior to LC-MS/MS analysis (Thermo Fisher Scientific). For the identification of polypeptide fragments. The primary mass spectrometry scan range spanned from 350 to 1800 (m/z) with a resolution of 70,000, whereas the secondary MS/MS resolution was set at 17,500.
Amino acid sequences of PAG14 were obtained using MaxQuant software (Max-Planck-Institute of Biochemistry, Germany) and the Universal Protein Resource (UniProt; https://www.uniprot. org/blast/) and the Antimicrobial Peptide Database (APD; https://aps. unmc.edu/AP/) were used to compare the sequences with known AMP amino acid sequences of antimicrobial peptides (Zou et al., 2018).
The results of the single-component screening of the culture medium are presented in Table 1. Mannitol as a single carbon source, fish peptone as a single nitrogen source and KH2PO4+K2HPO4+MnSO4·H2O as a single inorganic salt, they were effective against all four indicator bacteria and had the largest inhibition zone diameter, which had the best influence on inhibitory effect, respectively.
Table 1. Inhibition effect of carbon source, nitrogen source and inorganic salt on culture solution.
As shown in Table 2, the additive amounts of mannitol were 2%, 4%, and 8%, and the culture solution had an inhibitory effect on the four indicator bacteria. The additive amounts of fish peptone were 0.5%, 1%, and 2%; the culture solution had an inhibitory effect on all four indicator bacteria, and the inhibition zone diameter was larger. The added amounts of inorganic salts were 0.125%, 0.25%, and 0.5%, and the culture solution exhibited inhibitory effects on all four indicator bacteria, with larger inhibition zone diameters indicating stronger inhibitory effects. In summary, three additive amounts of mannitol, fish peptone, and inorganic salt were selected for the subsequent orthogonal experiments.
Table 2. Inhibition effect of mannitol, fish peptone and inorganic salt addition on culture solution.
The orthogonal experiment of L9 (34) was designed by using SPSS software with mannitol, fish peptone, KH2PO4 + K2HPO4 + MnSO4·H2O as test factors. The design scheme is presented in Table 3.
As shown in Table 4, among experimental groups 1–9 designed by the orthogonal experiment, group 1 exhibited the largest antibacterial circle diameter and the most effective antibacterial effect. Therefore, the optimal culture conditions for strain G14 were determined to be 2% mannitol, 0.5% fish peptone, and 0.05% KH2PO4 + 0.05% K2HPO4 + 0.025% MnSO4·H2O.
As shown in Figure 2A, the pH of the control group corresponded to the pH of the culture solution (5.5) during bacterial isolation and culture. Different pH values exhibited significant variations in their impact on the antibacterial activity compared to the control group. When the pH of the culture medium was below 5.0 or above 7.0, there was a gradual decline in the antibacterial efficacy with changing pH levels. However, at pH 5.5, the culture solution exhibited the highest antibacterial activity.
Figure 2. Results of stability determination of culture solution. (A) Acid-base stability, (B) Temperature stability, (C) Enzyme stability, (D) UV stability. Note: * indicates significant difference (P < 0.05), ** indicates highly significant difference (P < 0.01). Results of stability determination of culture solution (continued). (E) Genetic stability, (F) 4°C storage stability, (G) −20°C storage stability. Where Control is the control check.
As shown in Figures 2B, the temperature of the control group was 30°C when the bacteria were isolated and cultured. Compared with the control group, the inhibitory effect of the culture liquid decreased at 40–60°C after being treated at different temperatures, and the antibacterial activity of the culture liquid against P. aeruginosa, E. aerogenes and E. faecalis was significantly different compared with the control group (P < 0.01). No significant differences were observed in P. multocida. At temperatures ranging from 80°C to 121°C, there was a complete loss of antibacterial activity against P. aeruginosa. Additionally, a substantial decrease in antibacterial activity against E. aerogenes and E. faecalis was observed (P < 0.01), and the antibacterial activity against P. multocida disappeared at 121°C. The results showed that the culture solution was stable at 30–60°C.
As shown in Figure 2C, the control group was not subjected to enzyme treatment. Compared to the control group, the antibacterial activity of the culture broth treated with trypsin and pepsin against P. multocida and E. faecalis decreased. The antibacterial activity of the culture broth treated with protease K showed a decline against P. multocida. Furthermore, papain treatment decreases the antibacterial activity of the culture broth against E. aerogenes and E. faecalis. However, it completely disappeared for P. aeruginosa and P. multocida. Henceforth, it can be concluded that papain displayed higher sensitivity towards antimicrobial protein.
Compared to the control group, the antibacterial activity of the culture solution decreased within 4 h under UV light after different durations of UV treatment, as depicted in Figure 2D. The antibacterial activity of the culture solution against P. aeruginosa was significantly different (P < 0.01), and the antibacterial activity of the culture solution against P. aeruginosa completely disappeared at 5–6 h. Simultaneously, a significant decrease was noted in the antibacterial activity against E. aerogenes (P < 0.01).
As depicted in Figure 2E, the antibacterial activity of the culture solution against P. multocida gradually decreased from the 5th generation onward when compared to that of the control group (1st generation). However, no significant antibacterial activity was observed against the other indicator strains after passaging.
As depicted in Figures 2F, G, the antibacterial activity of the culture liquid stored at 4°C and −20°C for different durations, remained relatively stable compared to the control group (day 0).
Crude protein was obtained by salting, resolubilizing, and dialyzing the Bacillus G14 culture supernatant. After purification by dextran gel electrophoresis, the results showed that peak 3 protein exhibited antibacterial activity (Figure 3). The results for peak protein 3 after ion purification are shown in Figure 4. Peak 3 protein showed potent antibacterial activity against P. aeruginosa with an inhibition zone diameter of 13 mm (Figure 5). SDS-PAGE revealed a purified protein strip with a molecular mass ranging from approximately 11–17 KDa (Figure 4), indicating that the extracted antibacterial protein, PAG14, had a molecular weight within this range.
Figure 5. Gel electrophoresis of antibacterial protein PAG14. M: Non-pre-stained protein Marker (10–200 kDa); L: antibacterial protein PAG14.
The mass spectrum structure of the antibacterial protein PAG14 was analyzed using the Byonic software, as presented in Table 5. Based on the LC-MS/MS results, the identified proteins were comprehensively evaluated considering the score value, sequence coverage rate, and relative molecular mass. Consequently, it was presumed that the antibacterial substance belonged to the class III bacteriocin Lysozyme C. It has been reported in literature that bacteriocin Lysozyme C had a molecular mass of approximately 16.5 KDa (Ling et al., 2024), which further supports the findings obtained from SDS-PAGE analysis.
The efficiency of bacteriocin production through bacterial strain culture is related to the performance of the strain itself, but is also influenced by various factors, such as culture conditions and processes. The bacteria initially entered the logarithmic growth phase, with exponential growth in bacterial numbers and production of secondary metabolites. The strain reached a stable phase after 18 h and maximum production at approximately 22 h (Liu et al., 2021; Kim et al., 2022). Subsequently, as strain growth plateaued, bacteriocin production ceased. Culture media play a crucial role in bacteriocin production, because nutrients directly affect the expression and synthesis of bacteriocins and other secondary metabolites. The production of secondary metabolites by Bacillus is mainly affected by the nutrient composition of the medium (carbon, nitrogen source, and inorganic salt), culture conditions (temperature, pH, culture time, and inoculation amount), and highly complex metabolic regulation mechanisms. The production of inhibitory substances by microorganisms varies under different culture conditions. In this study, a single-factor orthogonal experiment was conducted to investigate the impact of optimal carbon and nitrogen sources, inorganic salts, and their ratios on the antibacterial activity of Bacillus G14 culture liquid. The optimum conditions were 2% mannitol, 0.5% fish peptone, 0.05% KH2PO4 + 0.05% K2HPO4 + 0.025% MnSO4·H2O, and the culture solution exhibited the best inhibitory effect. A study conducted by Anthony et al. revealed that elevated levels of yeast extract and NaCl, along with alkaline pH, high temperature, and vigorous agitation, significantly enhanced bacteriocin production in B. licheniformis AnBa9 (Anthony et al., 2009). Media and culture conditions must be carefully chosen to maximize bacteriocin production in Bacillus species.The selection of nutrients and the acquisition of appropriate concentration in the single factor culture medium provided a direct basis for the production of antibacterial substances by Bacillus G14.
The stability experiment of Bacillus G14 demonstrated that the antibacterial substance remained stable within a temperature range of 30–40°C during culture, whereas its activity significantly declined when exposed to temperatures exceeding 80°C. Hong et al. found that the activity of Bacteriocin from the B. subtilis was 100% after incubation at 0–50°C for 12 h but decreased to 70% after incubation at 70°C for 12 h (Hong et al., 2022). In contrast, the antimicrobial activity of a substance produced by Bacillus subtilis against B. cereus was lost after incubation at 80°C or 100°C for 1 h, and the activity against Listeria monocytogenes decreased by 50% after incubation at 60–80°C for 15 min (Kamoun et al., 2005; Kindoli et al., 2012). The culture material of Bacillus G14 was decomposed by UV irradiation, resulting in decreased antibacterial activity with increasing irradiation time. The pH stability test of the antibacterial substance from Bacillus G14 revealed that its activity was higher within a pH range of 5.0–7.0 in the culture solution, suggesting that acidic conditions could enhance the yield of the antibacterial substance. The stability of bacteriocins limits their application. For example, lactostreptococcin (Nisin) has strong activity in acidic environments, but loses activity in neutral and alkaline environments (Haider et al., 2022). It has been previously reported that the activity of the antimicrobial substance produced by B. subtilis SC-8 is lower at pH 3 than at pH 4–10. Bacthruricin F4, produced by Bacillus thuringiensis showed 40% residual antimicrobial activity at pH 8 and approximately 80% at pH 3 (Lee et al., 2010). The bacteriocin isolated from Bacillus BS2 was stable at pH 4–9 (Lee et al., 2022). The antibacterial substance of Bacillus G14 was sensitive to trypsin, pepsin, papain, and protease K. The antimicrobial activity of bacteriocins is lost upon exposure to proteolytic enzymes such as protease, proteinase K, and pronase E, confirming that the purified substance is proteinaceous (Chen et al., 2018). The antibacterial activity of Bacillus G14 against P. multocida decreased significantly in 7–11 generations. These excellent properties make Bacillus G14 bacteriocins have potential application prospects in feed processing and drug therapy.
Bacteriocins are ribosomally synthesized peptides that exert antibacterial effects against strains of the same species, or species that are more distantly related to the bacteriocin-producer (Cleveland et al., 2001). Bacteriocins have great potential in pharmaceutical, agricultural, and biochemical engineering industries (Iqbal et al., 2016). Rapid advancements in protein separation and purification technologies have led to the increasing recognition and application of a greater number of active substances derived from Bacillus. Wei et al. isolated subtilin JS-4 from B. subtilis, which inhibited the growth of Listeria (Wei et al., 2021). Kim et al. (2024) isolated Bacillus bacteriocins from fermented food and found that they inhibited the growth of aerobic bacteria and fungi on cheese surfaces. Pinontoan et al. (2018) isolated B. subtilis G8 with fibrinolytic activity from natto. Watanakij et al. (2020) isolated the extracellular fraction of B. subtilis BCC 42005 isolated from African locust bean potentially possessed aflatoxin B-1-degrading ability. Bacteriocins increase the permeability of the cell membrane, cause electrolyte outflow from the cell, interfere with proton dynamics, and cause ATP leakage (Willey and Van, 2007). According to their molecular structure characteristics of bacteriocins, Bacillus bacteriocins can be divided into three classes (Zgheib et al., 2020; Farid et al., 2023): Class I, modified bacteriocins, also known as lanthiobacitins, small molecule polypeptides (<5 ku); Class II, unmodified bacteriocins, and linear small molecule polypeptides (0.77–10 ku). Class III is a heat-labile protein-like bacteriocin (>10 ku), which is an unmodified protein of high molecular mass, including antibacterial proteins that cannot be classified owing to incomplete genetic, molecular, or amino acid structure data (i.e., bacteriocins). The mechanism of action of these bacteriocins involves inducing gram-positive bacterial cell wall alterations and gram-negative bacterial outer membrane disorganization, changing their structures, and causing cell lysis (Abriouel et al., 2011). Vaca et al. (2022) showed that most of the antibacterial substances synthesized by Bacillus were bacteriocins or bacteriocin-like substances. In this study, the antibacterial protein PAG14, isolated from the metabolite of Bacillus G14, was classified as a class III bacteriocin by LC-MS/MS, similar to Lysozyme C. Studies had shown that B. velezensis and B. subtilis encode several related secondary metabolite gene clusters, which can effectively inhibit the growth activity of Gram-negative bacteria (Xiang et al., 2021; Soni et al., 2022). We found that the secondary metabolites of B. velezensis G14 can inhibit a variety of pathogens which has broad application prospect in the future development.
The optimal culture conditions of G14 were determined to be 2% mannitol, 0.5% fish peptone, 0.05% KH2PO4 + 0.05% K2HPO4 + 0.025% MnSO4·H2O, with the antibacterial substances exhibiting stability at temperatures ranging from 30 to 40°C. The activity of these substances decreased with increasing UV irradiation time, whereas their effectiveness was highest when the pH value of the culture solution ranged between 5.0 and 7.0. Additionally, it was discovered that the antimicrobial substances were sensitive to trypsin, pepsin, papain and protease K enzymes. Furthermore, a class III bacteriocin produced by Bacillus G14 was purified and identified as being responsible for producing the antibacterial protein PAG14, which exhibits strong inhibitory effects on P. aeruginosa, with a molecular mass of approximately 11–17 kDa.
The original contributions presented in the study are included in the article/supplementary material, further inquiries can be directed to the corresponding authors.
JP: Writing–original draft. XX: Methodology, Writing–original draft. TF: Software, Writing–original draft. HM: Data curation, Writing–original draft. YL: Data curation, Writing–original draft. SL: Data curation, Writing–original draft. MY: Software, Writing–original draft. YD: Writing–review and editing. YM: Funding acquisition, Writing–review and editing.
The author(s) declare that financial support was received for the research, authorship, and/or publication of this article. This research was supported by Natural Science Foundation of Guangdong Province (2023A1515012181), and the Guangdong Provincial Department of Education Special Project for Key Fields of Ordinary Colleges and Universities (2021ZDZX4003).
The authors declare that the research was conducted in the absence of any commercial or financial relationships that could be construed as a potential conflict of interest.
All claims expressed in this article are solely those of the authors and do not necessarily represent those of their affiliated organizations, or those of the publisher, the editors and the reviewers. Any product that may be evaluated in this article, or claim that may be made by its manufacturer, is not guaranteed or endorsed by the publisher.
Abriouel, H., Franz, CMAP, Ben Omar, N., and Gálvez, A. (2011). Diversity and applications of Bacillus bacteriocins. FEMS Microbiol. Rev. 35, 201–232. doi:10.1111/j.1574-6976.2010.00244.x
Anthony, T., Rajesh, T., Kayalvizhi, N., and Gunasekaran, P. (2009). Influence of medium components and fermentation conditions on the production of bacteriocin(s) by Bacillus licheniformis AnBa9. Bioresour. Technol. 100, 872–877. doi:10.1016/j.biortech.2008.07.027
Basi-Chipalu, S., Sthapit, P., and Dhital, S. (2022). A review on characterization, applications and structure-activity relationships of Bacillus species-produced bacteriocins. Drug Discov. Ther. 16, 55–62. doi:10.5582/ddt.2021.01087
Bizani, D., and Brandelli, A. (2002). Characterization of a bacteriocin produced by a newly isolated Bacillus sp. Strain 8 A. J. Appl. Microbiol. 93, 512–519. doi:10.1046/j.1365-2672.2002.01720.x
Chen, L., Gu, Q., Li, P., Li, Y., Song, D., and Yang, J. (2018). Purification and characterization of plantaricin ZJ316, a novel bacteriocin against Listeria monocytogenes from Lactobacillus plantarum ZJ316. J. Food Prot. 81, 1929–1935. doi:10.4315/0362-028X.JFP-18-306
Cleveland, J., Montville, T. J., Nes, I. F., and Chikindas, M. L. (2001). Bacteriocins: safe, natural antimicrobials for food preservation. Int. J. Food Microbiol. 71, 1–20. doi:10.1016/S0168-1605(01)00560-8
Cotter, P. D., Ross, R. P., and Hill, C. (2013). Bacteriocins—a viable alternative to antibiotics? Nat. Rev. Microbiol. 11, 95–105. doi:10.1038/nrmicro2937
Elazzazy, A. M., Mobarki, M. O., Baghdadi, A. M., Bataweel, N. M., and Al-Hejin, A. M. (2024). Optimization of culture conditions and batch process control for the augmented production of bacteriocin by Bacillus species. Microorganisms 4, 651. doi:10.3390/microorganisms12040651
Farid, N., Waheed, A., and Motwani, S. (2023). Synthetic and natural antimicrobials as a control against foodborne pathogens: a review. Heliyon 9, e17021. doi:10.1016/j.heliyon.2023.e17021
Gond, S. K., Bergen, M. S., Torres, M. S., and White, J. F. (2015). Endophytic Bacillus spp. produce antifungal lipopeptides and induce host defence gene expression in maize. Microbiol. Res. 172, 79–87. doi:10.1016/j.micres.2014.11.004
Haider, T., Pandey, V., Behera, C., Kumar, P., Gupta, P. N., and Soni, V. (2022). Nisin and nisin-loaded nanoparticles: a cytotoxicity investigation. Drug Dev. Ind. Pharm. 48, 310–321. doi:10.1080/03639045.2022.2111438
Heilbronner, S., Krismer, B., Brötz-Oesterhelt, H., and Peschel, A. (2021). The microbiome-shaping Roles of bacteriocins. Nat. Rev. Microbiol. 19, 726–739. doi:10.1038/s41579-021-00569-w
Hong, S. W., Kim, J. H., Cha, H. A., Chung, K. S., Bae, H. J., Park, W. S., et al. (2022). Identification and characterization of a bacteriocin from the newly isolated Bacillus subtilis HD15 with inhibitory effects against Bacillus cereus. J. Microbiol. Biotechn 32, 1462–1470. doi:10.4014/jmb.2208.08006
Iqbal, M., Tao, Y., Xie, S., Zhu, Y., Chen, D., Wang, X., et al. (2016). Aqueous two-phase system (ATPS): an overview and advances in its applications. Biol. Proced. Online 18, 18. doi:10.1186/s12575-016-0048-8
Jouda, J. B., Mawabo, I. K., Notedji, A., Mbazoa, C. D., Nkenfou, J., Wandji, J., et al. (2016). Anti-mycobacterial activity of polyketides from Penicillium sp. endophyte isolated from Garcinia nobilis against Mycobacteriumsmegmatis. Int. J. Mycobact. 5, 192–196. doi:10.1016/j.ijmyco.2016.02.007
Kamoun, F., Mejdoub, H., Aouissaoui, H., Reinbolt, J., Hammani, A., and Jaoua, S. (2005). Purification, amino acid sequence and characterization of Bacthuricin F4, a new bacteriocin produced by Bacillus thuringiensis. J. Appl. Microbiol. 98, 881–888. doi:10.1111/j.1365-2672.2004.02513.x
Kim, J., Ahn, J., and Ahn, C. (2022). Characterization of novel bacteriocin produced by bacteriocinogenic Carnobacterium maltaromaticum isolated from raw milk. Microb. Pathog. 173, 105872. doi:10.1016/j.micpath.2022.105872
Kim, J. H., Lee, E. S., Kim, B. M., Ham, J. S., and Oh, M. H. (2024). Simple purification and antimicrobial properties of bacteriocin-like inhibitory substance from Bacillus species for the biopreservation of cheese. Foods 13, 10. doi:10.3390/foods13010010
Kindoli, S., Lee, H. A., Kim, J. H., and Kim, J. H. (2012). Properties of a bacteriocin from Bacillus subtilis H27 isolated from Cheonggukjang. J. Microbiol. Biotechn 21, 1745–1751. doi:10.1007/s10068-012-0232-9
Kumariya, R., Garsa, A. K., Rajput, Y. S., Sood, S. K., Akhtar, N., and Patel, S. (2019). Bacteriocins: classification, synthesis, mechanism of action and resistance development in food spoilage causing bacteria. Microb. Pathog. 128, 171–177. doi:10.1016/j.micpath.2019.01.002
Lee, G., Heo, S., Kim, T., Na, H. E., Park, J., Lee, E., et al. (2022). Discrimination of Bacillus subtilis from other Bacillus species using specific oligonucleotide primers for the pyruvate carboxylase and shikimate dehydrogenase genes. J. Microbiol. Biotechnol. 32, 1011–1016. doi:10.4014/jmb.2205.05014
Lee, N. K., Yeo, I. C., Park, J. W., Kang, B. S., and Hahm, Y. T. (2010). Isolation and characterization of a novel analyte from Bacillus subtilis SC-8 antagonistic to Bacillus cereus. J. Biosci. Bioeng. 110, 298–303. doi:10.1016/j.jbiosc.2010.03.002
Ling, X., Lv, J., Chen, F., Qin, X., Wu, M., Bai, F., et al. (2024). Expression characteristics and in vitro antibacterial properties of C-type lysozyme in crucian carp infected with Aeromonas salmonicida. Heliyon 10, e24044. doi:10.1016/j.heliyon.2024.e24044
Liu, G. R., Nie, R., Liu, Y. S., Li, X., Duan, J. J., Hao, X., et al. (2021). Bacillus subtilis BS-15 effectively improves plantaricin production and the regulatory biosynthesis in lactiplantibacillus plantarum RX-8. Front. Microbiol. 12, 772546. doi:10.3389/fmicb.2021.772546
Mercado, V., and Olmos, J. (2022). Bacteriocin production by Bacillus species: isolation, characterization, andapplication. Probiotics Antimicro 14, 1151–1169. doi:10.1007/s12602-022-09966-w
Oscáriz, J. C., Cintas, L., Holo, H., Lasa, I., Nes, I. F., and Pisabarro, A. G. (2006). Purification and sequencing of cerein 7B, a novel bacteriocin produced by Bacillus cereus Bc7. FEMS Microbiol. Lett. 254, 108–115. doi:10.1111/j.1574-6968.2005.00009.x
Oscáriz, J. C., and Pisabarro, A. G. (2000). Characterization and mechanism of action of cerein 7, a bacteriocin produced by Bacillus cereus Bc7. J. Appl. Microbiol. 89, 361–369. doi:10.1046/j.1365-2672.2000.01123.x
Özel, B., Şimşek, Ö., Akçelik, M., and Saris, P. E. J. (2018). Innovative approaches to nisin production. Appl. Microbiol. Biot. 102, 6299–6307. doi:10.1007/s00253-018-9098-y
Pinontoan, R., Elvina, S. A., and Jo, J. (2018). Fibrinolytic characteristics of the Bacillus subtilis G8 isolated from natto. Biosci. Microb. Food H. 40, 144–149. doi:10.12938/bmfh.2020-071
Sebei, S., Zendo, T., Boudabous, A., Nakayama, J., and Sonomoto, K. (2007). Characterization, N-terminal sequencing and classification of cerein MRX1, a novel bacteriocin purified from a newly isolated bacterium: Bacillus cereus MRX1. J. Appl. Microbiol. 103, 1621–1631. doi:10.1111/j.1365-2672.2007.03395.x
Senadeera, S. P. D., Wiyakrutta, S., Mahidol, C., Ruchirawat, S., and Kittakoop, P. (2012). A novel tricyclic polyketide and its biosynthetic precursor azaphilone derivatives from the endophytic fungus Dothideomycete sp. Org. Biomol. Chem. 10, 7220–7226. doi:10.1039/c2ob25959a
Soni, R., Keharia, H., Dunlap, C., Pandit, N., and Doshi, J. (2022). Functional annotation unravels probiotic properties of a poultry isolate, Bacillus velezensis CGS1.1. LWT-Food Sci.Technol. 153, 112471. doi:10.1016/j.lwt.2021.112471
Vaca, J., Ortiz, A., and Sansinenea, E. (2022). Bacillus sp. Bacteriocins: natural weapons against bacterial enemies. Curr. Med. Chem. 29, 2093–2108. doi:10.2174/0929867328666210527093041
Vaca, J., Ortiz, A., and Sansinenea, E. (2023). A study of bacteriocin like substances comparison produced by different species of Bacillus related to B. cereus group with specific antibacterial activity against foodborne pathogens. Arch. Microbiol. 205, 13. doi:10.1007/s00203-022-03356-0
Watanakij, N., Visessanguan, W., and Petchkongkaew, A. (2020). Aflatoxin B1-degrading activity from Bacillus subtilis BCC 42005 isolated from fermented cereal products Bacillus subtilis BCC 42005 isolated from fermented cereal products. Food Addit. Contam. A 37, 1579–1589. doi:10.1080/19440049.2020.1778182
Wei, Z. H., Shan, C. J., Zhang, L. X., Ge, D. E., Wang, Y., Xia, X. D., et al. (2021). A novel subtilin-like lantibiotics subtilin JS-4 produced by Bacillus subtilis JS-4, and its antibacterial mechanism against Listeria monocytogenes. LWT - Food Sci. Technol. 142, 110993. doi:10.1016/j.lwt.2021.110993
Willey, J. M., and Van, D. (2007). Lantibiotics: peptides of diverse structure and function. Annu. Rev. Microbiol. 61, 477–501. doi:10.1146/annurev.micro.61.080706.093501
Xiang, Y. Z., Li, X. Y., Zheng, H. L., Chen, J. Y., Lin, L. B., and Zhang, Q. L. (2021). Purification and antibacterial properties of a novel bacteriocin against Escherichia coli from Bacillus subtilis isolated from blueberry ferments. LWT-Food Sci.Technol. 146, 111456. doi:10.1016/j.lwt.2021.111456
Zgheib, H., Drider, D., and Belguesmia, Y. (2020). Broadening and enhancing bacteriocins activities by association with bioactive substances. Int. J. Env. Res. Pub. He. 17, 7835. doi:10.3390/ijerph17217835
Keywords: mangroves, endophytic bacteria, culture, bacteriocin, antibacterial activity
Citation: Peng J, Xie X, Fan T, Ma H, Li Y, Luo S, Yu M, Ding Y and Ma Y (2024) Optimization of culture conditions for endophytic bacteria in mangrove plants and isolation and identification of bacteriocin. Front. Pharmacol. 15:1429423. doi: 10.3389/fphar.2024.1429423
Received: 09 May 2024; Accepted: 22 July 2024;
Published: 02 August 2024.
Edited by:
Yingjie Yu, Beijing University of Chemical Technology, ChinaReviewed by:
Kao Li, China University of Petroleum (Huadong), ChinaCopyright © 2024 Peng, Xie, Fan, Ma, Li, Luo, Yu, Ding and Ma. This is an open-access article distributed under the terms of the Creative Commons Attribution License (CC BY). The use, distribution or reproduction in other forums is permitted, provided the original author(s) and the copyright owner(s) are credited and that the original publication in this journal is cited, in accordance with accepted academic practice. No use, distribution or reproduction is permitted which does not comply with these terms.
*Correspondence: Yuexia Ding, ZGluZ3l1ZXhpYTIwMDZAMTYzLmNvbQ==; Yi Ma, bWF5aTc2MUAxNjMuY29t
†These authors have contributed equally to this work
Disclaimer: All claims expressed in this article are solely those of the authors and do not necessarily represent those of their affiliated organizations, or those of the publisher, the editors and the reviewers. Any product that may be evaluated in this article or claim that may be made by its manufacturer is not guaranteed or endorsed by the publisher.
Research integrity at Frontiers
Learn more about the work of our research integrity team to safeguard the quality of each article we publish.