- 1Institute of Urology, Lanzhou University Second Hospital, Lanzhou University, Lanzhou, China
- 2Institute of Urology, The Affiliated Luohu Hospital of Shenzhen University, Shenzhen University, Shenzhen, China
- 3Institute of Urology, South China Hospital, Health Science Center, Shenzhen University, Shenzhen, China
Background: Statins, which are medications that lower lipid levels, are extensively used to decrease cardiovascular disease risk. Recently, the use of statins in cancer prevention has attracted considerable interest. However, it is still unclear whether the use of statins has a causal effect on bladder cancer.
Methods: The two-sample Mendelian Randomization (MR) was performed to infer the causal relationship between statin therapy (atorvastatin, simvastatin, and rosuvastatin) and bladder cancer. Single-nucleotide polymorphisms (SNP)-based genome-wide association studies (GWAS) of statins (atorvastatin, simvastatin, and rosuvastatin) were gathered from the UK Biobank, involving 462,933 participants. We acquired summary-level genetic data on bladder cancer from a European cohort of 175,121 individuals. The inverse variance weighted (IVW) method was the main analytical technique used, supplemented by MR-Egger, weighted median, weighted mode, and simple mode to estimate causal effects. Additionally, sensitivity analyses were conducted to verify the robustness and reliability of our findings.
Results: Based on the IVW analysis, we identified a significant causal association between rosuvastatin use and a decreased risk of bladder cancer, with genetic analysis inferring the substantial reduction in odds (OR = 3.52E-19, 95% CI: 5.48E-32–2.26E-06, p = 0.005). In contrast, the IVW results did not reveal a statistically significant relationship between the genetically estimated use of atorvastatin (OR = 7.42E-03, 95% CI: 6.80E-06–8.084, p = 0.169) or simvastatin (OR = 0.135, 95% CI: 0.008–2.330, p = 0.168) and bladder cancer risk.
Conclusion: We investigated the causal link between statin therapy (atorvastatin, simvastatin, and rosuvastatin) and bladder cancer using a two-sample Mendelian Randomization analysis among the European population. Our findings indicated that genetically predicted use of rosuvastatin was associated with a decreased risk of bladder cancer, whereas no significant genetically predicted causal effects were observed for atorvastatin and simvastatin use.
1 Introduction
Bladder cancer (BCa), frequently diagnosed as a malignant urological tumor, originates mainly from malignant transitional epithelial cells (Dyrskjot et al., 2023). In 2022, this cancer accounted for 84,825 new cases in the United States and 91,893 in China, while Europe reported 204,000 new cases in 2020 (Dyba et al., 2021; Xia et al., 2022). Known as the costliest malignancy to manage, bladder cancer poses a significant healthcare challenge due to its tendency for frequent relapses and the static nature of treatment advancements, necessitating expensive ongoing monitoring and multiple interventions (Leal et al., 2016; Richters et al., 2020). In the United States, the annual total cost of cancer was $183 billion in 2015, and it is projected to increase to $246 billion by 2030. Among these costs, the annual medical burden of bladder cancer was approximately $7.93 billion, with an expected increase to $11.6 billion by 2030 (Mariotto et al., 2020). Similarly, in the European Union member states, the total cost of cancer was €152.8 billion in 2012, with the medical burden of bladder cancer accounting for approximately €5.24 billion (Leal et al., 2016). Effective early prevention, screening, and accurate diagnosis are pivotal in lessening the burden of this disease on society (Lenis et al., 2020). In the realm of cancer prevention, particularly through chemoprevention, medications commonly used for metabolic and cardiovascular conditions have been noticed for their beneficial impacts on the anticancer process (Gronich and Rennert, 2013; Morales and Morris, 2015).
Statins, which are 3-Hydroxy-3-methylglutaryl-coenzyme A reductase (HMGCoAR) inhibitors, effectively reduce lipids and are the primary treatment for hypercholesterolemia by blocking liver-based endogenous cholesterol production (Igel et al., 2002; Ziaeian and Fonarow, 2017). These medications might also serve a chemopreventive function against cancer, as a decrease in cholesterol could restrict the cell proliferation necessary for cancer development and spread (Rosch and McCully, 2013). As the critical enzyme in the mevalonate pathway, HMGCoAR supports essential cellular growth and survival processes (Mullen et al., 2016). Furthermore, statins are known to obstruct Ras/Rho pathways, thereby curtailing various cancer-promoting signaling routes (Ahmadi et al., 2017; Patel and Kashfi, 2022). A nested case-control study within the UK Clinical Practice Research Datalink (CPRD) found that current statins use correlates with a 12% reduction in the risk of biliary tract cancers compared to non-use (Liu et al., 2019). However, few randomized controlled trials (RCTs) have been conducted to evaluate the effect of statins on bladder cancer (Symvoulidis et al., 2023).
Mendelian randomization (MR) is an analytical method increasingly employed to determine causal relationships between exposures and outcomes (Davies et al., 2018). This technique uses genetic variations as instrumental variables (IV) to firmly establish causality between exposures and outcomes (Davey Smith and Hemani, 2014; Burgess et al., 2015). By leveraging the random distribution of these genetic variations, MR effectively minimizes the impact of confounding factors and reverse causality. This approach emulates the randomization seen in RCTs, thereby circumventing the confounding effects and potential biases associated with traditional RCTs (Lawlor et al., 2008). Utilizing data from Genome-wide association studies (GWAS), MR has been extensively applied in various public health sectors (Sekula et al., 2016; Hemani et al., 2018). Accordingly, a two-sample MR method was used to investigate the causal link between statin usage and bladder cancer, thus providing a more robust basis for clinical decision-making through GWAS data insights.
2 Methods
2.1 Study design and ethics statement
In this study, MR analysis was utilized to explore the causal relationship between statin use and bladder cancer (Figure 1). MR analysis is based on public GWAS data, and we utilized publicly available GWAS datasets for atorvastatin, simvastatin, and rosuvastatin usage in this MR analysis. The use of atorvastatin, simvastatin, and rosuvastatin served as the exposure variables in our study. The study design and reporting conformed to using STROBE-MR (Skrivankova et al., 2021a; Skrivankova et al., 2021b). MR analysis employs instrumental variables (IVs) to evaluate causal links between exposures and outcomes. This method hinges on three critical assumptions (Lawlor et al., 2008). The first assumption requires that the genetic variants (single-nucleotide polymorphisms, SNPs) used as IVs must have a strong association with the exposure (statins). The second assumption states that these genetic variants should not be linked with any confounders affecting the relationship between the exposure and outcomes. Finally, the third assumption mandates that the genetic variants should influence the outcome solely through their effect on the exposure, excluding any other indirect pathways. Since our data were sourced from previously conducted research and publicly available databases, obtaining further ethical approval from an ethics committee was not necessary.
2.2 Data source
We obtained summary statistics for statin use and bladder cancer from the International Oncology Unit (IEU) Open GWAS project (https://gwas.mrcieu.ac.uk/datasets/). The data for atorvastatin, simvastatin, and rosuvastatin came from the MRC-IEU consortium (Bycroft et al., 2018; Hemani et al., 2018). Specifically, the atorvastatin dataset (ukb-b-10008) included 13,851 cases and 449,082 controls; the simvastatin dataset (ukb-b-11268) included 52,427 cases and 410,506 controls; and the rosuvastatin dataset (ukb-b-13664) included 2,870 cases and 460,063 controls. To avoid population overlap in the exposure and outcome assessments, we sourced GWAS summary-level data linked to BCa from a FinnGen biobank cohort of European descent (1,115 cases and 174,006 controls) via the IEU Open GWAS database (Kurki et al., 2023). We exclusively used datasets of European ancestry to mitigate bias due to population stratification. Detailed information about these four GWAS datasets is available in Table 1.
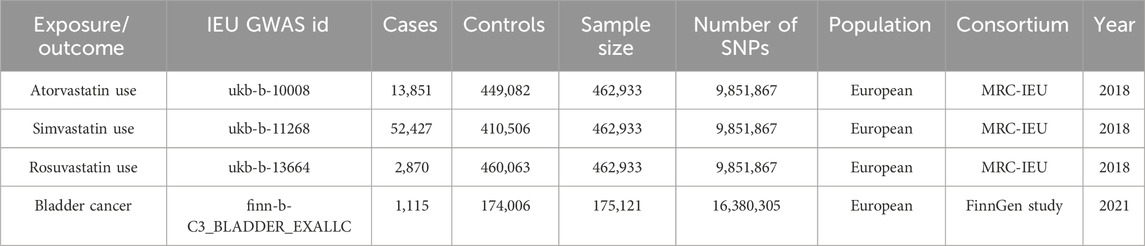
Table 1. Characteristics of statin (atorvastatin, simvastatin, and rosuvastatin) use and bladder cancer GWAS cohorts in this study.
2.3 Selection of instrumental variables
Drawing from the GWAS summary data mentioned earlier, a rigorous procedure was followed to select suitable SNPs as IVs. Initially, SNPs had to demonstrate a strong association with the exposure, achieving a genome-wide significance with a p-value < 5 × 10−8. Secondly, to prevent results skewed by linkage disequilibrium (LD), a clumping process was implemented with an R2 cutoff of 0.001 and a window size of 10,000 kb. Thirdly, the Phenoscanner database (http://www.phenoscanner.medschl.cam.ac.uk/) was employed to identify genetic variants linked to potential confounders. SNPs closely related to the potential confounders, including smoking, body mass index, waist-to-hip ratio, and type 2 diabetes mellitus were excluded (p-value < 5 × 10−8). The remaining SNPs were correspondingly chosen as IVs for exposes (Cheng et al., 2023). Fourthly, if these specific SNPs were absent in the outcome GWAS dataset, proxy SNPs (with a high LD, R2 > 0.8, with the target SNPs) were sought as alternatives. Finally, to ensure the consistency of effect alleles between the exposure and outcome datasets, harmonization was carried out to exclude palindromic and ambiguous SNPs with non-matching alleles. Additionally, to robustly adhere to the first key assumption, the F-statistic for each SNP was calculated individually; SNPs with F statistics < 10 were deemed weak IVs and excluded from further analysis (Burgess et al., 2011; Li et al., 2023; 2024). Following these stringent filters, the selected SNPs were utilized as the definitive IVs for the ensuing two-sample MR study.
2.4 Statistical analysis
In our research, we utilized a range of methods to analyze the causal connections and effects between exposure and outcome, including inverse-variance weighted (IVW) (Burgess et al., 2016), MR-Egger (Bowden et al., 2015), weighted median (Bowden et al., 2016), weighted mode (Hartwig et al., 2017), and simple mode (Zhang et al., 2023). Each method is suited to different scenarios. The IVW method uses SNPs’ Wald estimators to determine the influence of exposure on outcome. We primarily rely on the IVW approach when there is no pleiotropy, or when pleiotropy is balanced, to derive reliable causal estimates. If significant heterogeneity among the IVs was detected (p < 0.05), a random effects model was applied; otherwise, a fixed effects model was employed (Burgess et al., 2016). The MR-Egger regression provides credible estimates under conditions of IV pleiotropy (Bowden et al., 2015). The Weighted Median method, not requiring the Instrument Strength Independent of Direct Effect (InSIDE) assumption, offers significant improvements over MR-Egger by achieving unbiased effect estimates and lower type I error through evaluation of the weighted median of instrumental variable ratio estimate (Bowden et al., 2016). The weighted mode method is effective for MR causal inference assuming most IVs are valid (Hartwig et al., 2017), while the simple mode method generally yields less robust results compared to IVW(Zhang et al., 2023). All these methods were executed and visually presented using R version 4.3.1 with the “MRPRESSO” and “TwoSampleMR” packages, considering a p-value under 0.05 as statistically significant.
2.5 Sensitivity analysis
We conducted MR-Egger regression to evaluate pleiotropy in the instrumental variables, considering pleiotropy confirmed when the p-value fell below 0.05. We also implemented the MR-PRESSO test to further assess pleiotropy and identify outliers. In instances where the MR-PRESSO test revealed significant horizontal pleiotropy, we removed the implicated outlier variants and repeated the MR analysis. To quantify heterogeneities uncovered by both the IVW and MR-Egger regression methods, we calculated Cochran’s Q statistic, with a p-value of less than 0.05 indicating significant heterogeneity. Additionally, we executed a “leave-one-out” sensitivity analysis to ascertain whether any single SNP could significantly skew the overall causal inference.
3 Results
3.1 Selection of instrumental variables
Adhering to strict criteria for instrumental SNP selection, we identified appropriate SNPs as IVs that met three key assumptions. We identified 22 SNPs highly correlated with atorvastatin use, 39 SNPs highly correlated with simvastatin use, and 6 SNPs highly correlated with rosuvastatin use. These SNPs served as IVs for exposure, with each SNP displaying an F-statistic greater than 10, indicating a minimal likelihood of weak IV bias. Detailed descriptions of the included SNPs are provided in the Supplementary Tables S1-S3.
3.2 Causal effects of atorvastatin use on bladder cancer
Table 2 displayed the outcomes of the MR analysis on the causal effects of atorvastatin use on bladder cancer. The inverse variance weighted (IVW) method revealed no causal connection between atorvastatin use and bladder cancer (OR = 7.42E-03, 95% CI: 6.80E-06–8.084, p = 0.169), a finding supported by additional methods including MR-Egger, Weighted Median, Weighted Mode, and Simple Mode. These results were graphically represented in the forest plot (Figure 2) and the scatter plot (Figure 3). The forest plot delineated the effect estimates and their confidence intervals for each SNP, while the scatter plot illustrated the relationship between atorvastatin use and bladder cancer using the instrumental variables. Thus, our analysis indicated that there was no significant causal effect of atorvastatin use on bladder cancer.
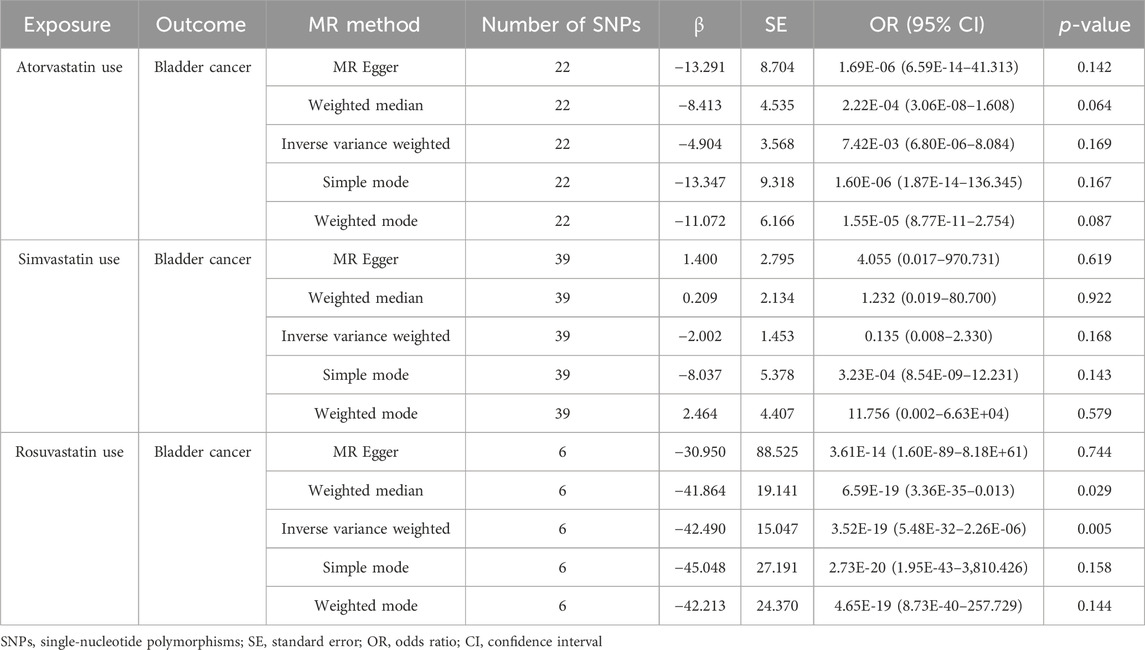
Table 2. MR analysis of the causality of statin (atorvastatin, simvastatin, and rosuvastatin) use on Bladder cancer.
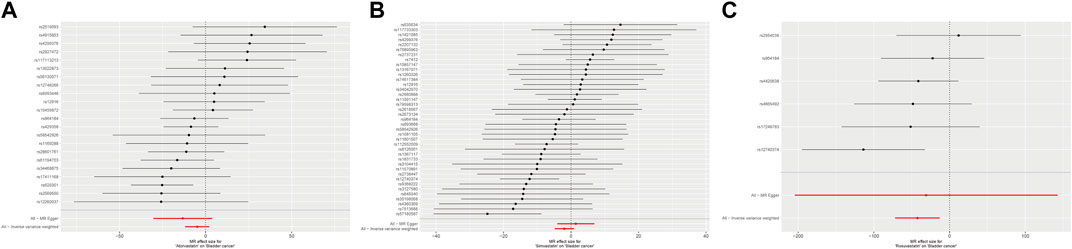
Figure 2. Forest plot of the causal effects of atorvastatin (A), simvastatin (B), and rosuvastatin (C) use associated SNPs on bladder cancer.
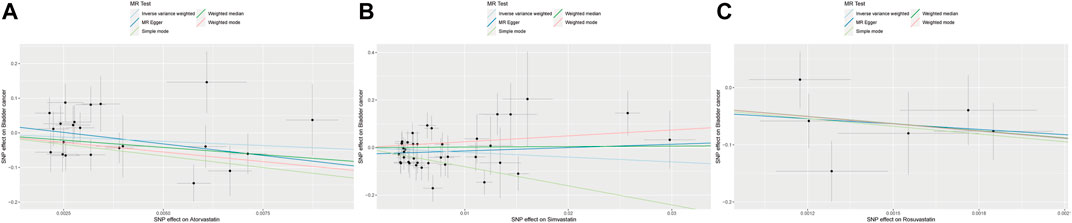
Figure 3. Scatter plot of the causal relationships between atorvastatin (A), simvastatin (B), and rosuvastatin (C) use on bladder cancer. The regression slopes of the lines represent the magnitude of the causal effect.
Additionally, the MR-Egger regression intercept analysis (p = 0.304) and the MR-PRESSO global test (p = 0.121) did not show significant pleiotropy (Table 3). The Cochran Q-test results from both the MR-Egger (p = 0.137) and IVW (p = 0.128) methods also demonstrated no heterogeneity in our findings. The funnel plots, presented in Supplementary Figure S1. Furthermore, the leave-one-out analysis verified that excluding any single SNP did not significantly influence the estimated causal relationship (Supplementary Figure S2).
3.3 Causal effects of simvastatin use on bladder cancer
The results from the MR analysis investigating the causal effects of simvastatin on bladder cancer were presented in Table 2. The IVW method indicated that simvastatin use had no causal impact on bladder cancer (OR = 0.135, 95% CI: 0.008–2.330, p = 0.168), a conclusion corroborated by the MR-Egger, Weighted Median, Weighted Mode, and Simple Mode analyses. Figures 2, 3 respectively illustrated these findings through a forest plot and a scatter plot, reinforcing the lack of a significant causal relationship between simvastatin use and bladder cancer.
Further evaluations using the MR-Egger regression intercept analysis for assessing pleiotropy among the instrumental variables showed no significant pleiotropic effects (p = 0.164) (Table 3). Although the p-value of MR-PRESSO global test was less than 0.05, MR-PRESSO global test showed that no significant outliers. The outlier-corrected causal estimate showed the NA. Heterogeneity assessments conducted through the MR-Egger (p = 0.095) and IVW (p = 0.074) methods also indicated no significant heterogeneity among the instrumental variables. The funnel plots depicted in Supplementary Figure S1. Additionally, the leave-one-out analysis verified the stability of the causal association estimate, demonstrating that it was unaffected by the exclusion of any individual SNP (Supplementary Figure S2).
3.4 Causal effects of rosuvastatin use on bladder cancer
The outcomes of the MR analysis of the causality of rosuvastatin use on bladder cancer were encapsulated in Table 2. IVW revealed a statistically significant negative causal impact of A rosuvastatin use on bladder cancer (OR = 3.52E-19, 95% CI: 5.48E-32–2.26E-06, p = 0.005). Simultaneously, a relationship following the same trend was discerned through the weighted median method (OR = 6.59E-19, 95% CI = 3.36E-35–0.013, p = 0.029). These results were graphically represented in both the forest plot (Figure 2) and the scatter plot (Figure 3). Given that IVW and weighted median method hold an edge in preserving superior estimation precision over the MR-Egger method in MR analysis, the outcomes from the MR analysis provide support for a potential causal association between rosuvastatin use on bladder cancer.
Additionally, the MR-Egger regression intercept analysis (p = 0.901) and the MR-PRESSO global test (p = 0.487) did not show significant pleiotropy (Table 3). The Cochran Q-test results from both the MR-Egger (p = 0.285) and IVW (p = 0.410) methods also demonstrated no heterogeneity in our findings. The funnel plots were shown in Supplementary Figure S1. Furthermore, the leave-one-out analysis demonstrated that the causal association estimate was not influenced by the exclusion of any individual SNP (Supplementary Figure S2). These outcomes provide confidence in the validity and robustness of the causal inference derived from the MR analysis.
4 Discussion
In this research, we utilized a two-sample MR analysis based on GWAS summary-level data to assess the causal impact of statin therapy (atorvastatin, simvastatin, and rosuvastatin) on bladder cancer. The MR analysis indicated that rosuvastatin usage had a genetically determined causal effect on reducing bladder cancer risk. However, there were no causal effects on bladder cancer risk from the use of atorvastatin or simvastatin.
In the present epidemiological research, the influence of statins on cancer risk remains uncertain. A population-based, nested, case-control study with 3,129 patients and 16,976 controls from the PHARMO database was conducted to investigate the potential protective effect of statin therapy on cancer risk. This study reported a 20% reduction in cancer risk associated with statin use (adjusted odds ratio [OR], 0.80; 95% CI, 0.66–0.96) (Graaf et al., 2004). Additionally, a multi-regional observational study from the BioBank Japan cohort indicated that statin monotherapy was effective in reducing all-cause and cancer mortality (Yokomichi et al., 2017). In contrast, a matched case-control study using the General Practice Research Database observed no significant relationship between current statin use and the risk of 13 types of cancer (Kaye and Jick, 2004). Another investigation involving 4,913 cancer patients and 3,900 controls found no evidence to support a positive or negative association between statin use and 10 cancer types (Coogan et al., 2007).
Further research by Jun-Jun Yeh et al., utilizing Taiwan’s National Health Insurance Research Database, assessed the effects of statins on cancer risk among patients with interstitial lung disease (ILD) and pulmonary fibrosis. Their findings suggested a lower risk of bladder cancer associated with statin use in this population (Yeh et al., 2021). A multicenter study on patients with T1 high-grade non-muscle invasive urothelial bladder cancer showed that statin use was independently associated with a lower risk of recurrence (HR: 0.80, 95% CI: 0.67–0.95; p = 0.009), implying a beneficial effect on recurrence rates (Ferro et al., 2021). Additionally, a retrospective cohort study of individuals aged 66 and over, diagnosed with non-muscle invasive bladder cancer (NMIBC) between 1992 and 2012, demonstrated that statin users had better overall survival compared to nonusers (Richard et al., 2017). However, a population-based case-control study in Taiwan, including 325 bladder cancer cases and 1,300 controls, did not provide evidence supporting any beneficial or detrimental associations between statin use and bladder cancer risk (Kuo et al., 2012). A retrospective analysis of 1,117 patients treated with transurethral resection of the bladder (TURB) for NMIBC at three institutions from 1996 to 2007 assessed the impact of statin use on patient outcomes and the efficacy of intravesical BCG therapy. The findings indicated that statin use did not affect outcomes differently compared to non-use, nor did it impact the efficacy of BCG immunotherapy (Crivelli et al., 2013). And a meta-analysis including 10 studies found a non-significant increase in bladder cancer risk among statin users compared with non-users, and no association between statin use and BCa local control, recurrence, survival or mortality (Symvoulidis et al., 2023). Another meta-analysis including 13 studies also suggested that there was no association between statin use and risk of BCa(Zhang et al., 2013). It is noteworthy that these studies did not differentiate the effects based on specific statins.
Atorvastatin demonstrated notable antiproliferative and pro-apoptotic effects in human bladder cancer cells (Kamat and Nelkin, 2005). It also induced autophagy in these cells in vitro. Additionally, when used with autophagy inhibitors, atorvastatin’s cytotoxicity was enhanced, further promoting apoptotic cell death (Kang et al., 2014). In animal studies, Belmiro Parada et al. explored the chemopreventive efficacy of atorvastatin against nitrosamine-induced rat bladder cancer and observed a significant inhibitory impact on cancer development, likely due to its antioxidant, anti-proliferative, and anti-inflammatory actions (Parada et al., 2012). Despite these findings, no clinical trials have confirmed atorvastatin’s protective effect in bladder cancer patients. Similarly, our findings did not support a causal link between atorvastatin use and reduced bladder cancer risk.
Simvastatin inhibited bladder cancer cell metastasis by blocking epithelial-mesenchymal transition (EMT) and disrupting AKT/GSK3β pathways, while also suppressing cell proliferation and causing G1/G0 phase cell cycle arrest through the Peroxisome Proliferator-Activated Receptor (PPAR)γ signaling pathway (Wang et al., 2016). Additionally, the combination of simvastatin with romidepsin synergistically killed bladder cancer cells, with mechanisms involving ER stress induction, AMPK activation, histone acetylation, and enhanced PPARγ expression (Okubo et al., 2021). Moreover, Pleomorphic adenoma gene like-2 (PLAGL2) facilitated bladder cancer progression via RACGAP1/RhoA GTPase/YAP1 signaling, and its proproliferative and prometastatic effects were negated by the RhoA inhibitor simvastatin (Chen et al., 2023). Contrarily, a 10-year multicentric retrospective study in Lebanon established a duration-response relationship between simvastatin use and bladder cancer risk (OR = 1.189), revealing a detrimental link with the increased duration of simvastatin intake (Chalhoub et al., 2023). Our findings showed that there was no causal link between simvastatin use and bladder cancer risk among the European population.
Rosuvastatin triggered autophagic responses in human papillary thyroid cancer B-CPAP cells at lower doses, with a transition to apoptosis observed as rosuvastatin concentrations increased (Zeybek et al., 2011). When used alone or in a combined strategy with difluoromethylornithine, rosuvastatin significantly inhibited colon adenocarcinomas in male F344 rats induced by azoxymethane (AOM) and enhanced the functionality of natural killer (NK) cells (Janakiram et al., 2016). Furthermore, rosuvastatin prevented spheroid formation and epithelial-mesenchymal transition (EMT) in the prostate cancer PC-3 cell line (Deezagi and Safari, 2020) and exhibited antiangiogenic and antitumor properties that curtailed prostate cancer growth (Wang et al., 2010). A population-based cohort study from the Database of Clalit Health Services indicated that extended use of rosuvastatin was linked to a lower risk of prostate cancer (HR 0.22, 95% CI 0.08–0.75) (Lustman et al., 2014). In this study, MR analysis suggested that rosuvastatin use has a genetically determined causal effect in reducing bladder cancer risk.
Our research has several noteworthy strengths. Initially, we assessed the impact of three specific statins on bladder cancer risk using a two-sample MR analysis, potentially offering more profound insights for subsequent research. Given statins’ known benefits in lowering cardiovascular risk through their antidyslipidemic properties, their potential protective effects against bladder cancer could translate into significant medical and socioeconomic advantages for patients with common risk factors. Furthermore, Mendelian Randomization, which utilizes extensive data from Genome-Wide Association Studies (GWAS) to simulate a randomized controlled trial, offers a cost-effective alternative to observational studies and minimizes the risk of reverse causation. Additionally, the selection of our instrumental variable SNP, which occurs randomly at conception, helps eliminate confounding bias. Lastly, by choosing participants from the European demographic, we aimed to decrease potential biases stemming from population stratification.
This study is subject to several limitations that must be considered when interpreting and generalizing the results. Initially, while the study population addressed racial discrepancies, it remains uncertain if these findings can be extended to different racial groups and geographic areas. Additional GWAS studies across diverse regions might yield stronger evidence concerning the relationship between statin use and bladder cancer risk. Additionally, our reliance on summary-level data precluded the possibility of analyzing non-linear relationships or effects that vary across subgroups. Moreover, we accessed only the GWAS summary-level data for atorvastatin, simvastatin, and rosuvastatin, which limits our understanding of how other statins might affect bladder cancer risk. Then, the funnel plot for the instrumental variables is asymmetric, indicating bias and confounding in the findings. Lastly, the MR method used was restricted to establishing causal connections and did not allow for the investigation of the mechanisms underlying these associations, which would require more comprehensive studies.
5 Conclusion
In this study, we presented evidence supporting a potential genetically determined causal link between rosuvastatin use and a decreased risk of bladder cancer in the European population, using two-sample Mendelian Randomization analysis. The results indicated that rosuvastatin usage was linked to a lower risk of bladder cancer, while no significant genetically predicted causal effects were observed for atorvastatin or simvastatin. The results of this study were not sufficient to support the conclusion that statins were associated with low incidence of bladder cancer. Additional research into the mechanisms is required to elucidate the intricate relationship between statin treatment and bladder cancer risk.
Data availability statement
The original contributions presented in the study are included in the article/Supplementary Material, further inquiries can be directed to the corresponding authors.
Author contributions
RL: Conceptualization, Data curation, Formal Analysis, Investigation, Software, Writing–original draft. GH: Conceptualization, Data curation, Formal Analysis, Investigation, Software, Writing–original draft. YFL: Formal Analysis, Software, Writing–original draft. MH: Formal Analysis, Software, Writing–original draft. YH: Formal Analysis, Software, Writing–original draft. YGL: Formal Analysis, Software, Writing–original draft. GL: Funding acquisition, Methodology, Project administration, Resources, Supervision, Writing–review and editing. SW: Funding acquisition, Methodology, Project administration, Resources, Supervision, Writing–review and editing.
Funding
The author(s) declare that financial support was received for the research, authorship, and/or publication of this article. This work was partially supported by the Shenzhen Key Medical Discipline (No. SZXK021) and the Sanming Project of Medicine in Shenzhen (No. SASM202201024).
Conflict of interest
The authors declare that the research was conducted in the absence of any commercial or financial relationships that could be construed as a potential conflict of interest.
Publisher’s note
All claims expressed in this article are solely those of the authors and do not necessarily represent those of their affiliated organizations, or those of the publisher, the editors and the reviewers. Any product that may be evaluated in this article, or claim that may be made by its manufacturer, is not guaranteed or endorsed by the publisher.
Supplementary material
The Supplementary Material for this article can be found online at: https://www.frontiersin.org/articles/10.3389/fphar.2024.1427318/full#supplementary-material
References
Ahmadi, Y., Ghorbanihaghjo, A., and Argani, H. (2017). The balance between induction and inhibition of mevalonate pathway regulates cancer suppression by statins: a review of molecular mechanisms. Chem. Biol. Interact. 273, 273–285. doi:10.1016/j.cbi.2017.06.026
Bowden, J., Davey Smith, G., and Burgess, S. (2015). Mendelian randomization with invalid instruments: effect estimation and bias detection through Egger regression. Int. J. Epidemiol. 44 (2), 512–525. doi:10.1093/ije/dyv080
Bowden, J., Davey Smith, G., Haycock, P. C., and Burgess, S. (2016). Consistent estimation in mendelian randomization with some invalid instruments using a weighted median estimator. Genet. Epidemiol. 40 (4), 304–314. doi:10.1002/gepi.21965
Burgess, S., Daniel, R. M., Butterworth, A. S., Thompson, S. G., and Consortium, E.P.-I. (2015). Network Mendelian randomization: using genetic variants as instrumental variables to investigate mediation in causal pathways. Int. J. Epidemiol. 44 (2), 484–495. doi:10.1093/ije/dyu176
Burgess, S., Dudbridge, F., and Thompson, S. G. (2016). Combining information on multiple instrumental variables in Mendelian randomization: comparison of allele score and summarized data methods. Stat. Med. 35 (11), 1880–1906. doi:10.1002/sim.6835
Burgess, S., Thompson, S. G., and Collaboration, C. C. G. (2011). Avoiding bias from weak instruments in Mendelian randomization studies. Int. J. Epidemiol. 40 (3), 755–764. doi:10.1093/ije/dyr036
Bycroft, C., Freeman, C., Petkova, D., Band, G., Elliott, L. T., Sharp, K., et al. (2018). The UK Biobank resource with deep phenotyping and genomic data. Nature 562 (7726), 203–209. doi:10.1038/s41586-018-0579-z
Chalhoub, I. G., Boulos, R. T., Dagher, Y. G., El Helou, S., Haifa, K. G., Atallah, B., et al. (2023). Statins, commonly coprescribed drugs, and concomitant risk factors: a protective, neutral, or harmful association with common cancer types development: a 10-year multicentric retrospective lebanese study. Med. Baltim. 102 (39), e34562. doi:10.1097/MD.0000000000034562
Chen, H., Yang, W., Li, Y., and Ji, Z. (2023). PLAGL2 promotes bladder cancer progression via RACGAP1/RhoA GTPase/YAP1 signaling. Cell Death Dis. 14 (7), 433. doi:10.1038/s41419-023-05970-2
Cheng, Z., Ye, F., Liang, Y., Xu, C., Zhang, Z., Ou, Y., et al. (2023). Blood lipids, lipid-regulatory medications, and risk of bladder cancer: a Mendelian randomization study. Front. Nutr. 10, 992608. doi:10.3389/fnut.2023.992608
Coogan, P. F., Rosenberg, L., and Strom, B. L. (2007). Statin use and the risk of 10 cancers. Epidemiology 18 (2), 213–219. doi:10.1097/01.ede.0000254694.03027.a1
Crivelli, J. J., Xylinas, E., Kluth, L. A., da Silva, R. D., Chrystal, J., Novara, G., et al. (2013). Effect of statin use on outcomes of non-muscle-invasive bladder cancer. BJU Int. 112 (2), E4–E12. doi:10.1111/bju.12150
Davey Smith, G., and Hemani, G. (2014). Mendelian randomization: genetic anchors for causal inference in epidemiological studies. Hum. Mol. Genet. 23 (R1), R89–R98. doi:10.1093/hmg/ddu328
Davies, N. M., Holmes, M. V., and Davey Smith, G. (2018). Reading Mendelian randomisation studies: a guide, glossary, and checklist for clinicians. BMJ 362, k601. doi:10.1136/bmj.k601
Deezagi, A., and Safari, N. (2020). Rosuvastatin inhibit spheroid formation and epithelial-mesenchymal transition (EMT) in prostate cancer PC-3 cell line. Mol. Biol. Rep. 47 (11), 8727–8737. doi:10.1007/s11033-020-05918-1
Dyba, T., Randi, G., Bray, F., Martos, C., Giusti, F., Nicholson, N., et al. (2021). The European cancer burden in 2020: incidence and mortality estimates for 40 countries and 25 major cancers. Eur. J. Cancer 157, 308–347. doi:10.1016/j.ejca.2021.07.039
Dyrskjot, L., Hansel, D. E., Efstathiou, J. A., Knowles, M. A., Galsky, M. D., Teoh, J., et al. (2023). Bladder cancer. Nat. Rev. Dis. Prim. 9 (1), 58. doi:10.1038/s41572-023-00468-9
Ferro, M., Marchioni, M., Lucarelli, G., Vartolomei, M. D., Soria, F., Terracciano, D., et al. (2021). Association of statin use and oncological outcomes in patients with first diagnosis of T1 high grade non-muscle invasive urothelial bladder cancer: results from a multicenter study. Minerva Urol. Nephrol. 73 (6), 796–802. doi:10.23736/S2724-6051.20.04076-X
Graaf, M. R., Beiderbeck, A. B., Egberts, A. C., Richel, D. J., and Guchelaar, H. J. (2004). The risk of cancer in users of statins. J. Clin. Oncol. 22 (12), 2388–2394. doi:10.1200/JCO.2004.02.027
Gronich, N., and Rennert, G. (2013). Beyond aspirin-cancer prevention with statins, metformin and bisphosphonates. Nat. Rev. Clin. Oncol. 10 (11), 625–642. doi:10.1038/nrclinonc.2013.169
Hartwig, F. P., Davey Smith, G., and Bowden, J. (2017). Robust inference in summary data Mendelian randomization via the zero modal pleiotropy assumption. Int. J. Epidemiol. 46 (6), 1985–1998. doi:10.1093/ije/dyx102
Hemani, G., Zheng, J., Elsworth, B., Wade, K. H., Haberland, V., Baird, D., et al. (2018). The MR-Base platform supports systematic causal inference across the human phenome. Elife 7, e34408. doi:10.7554/eLife.34408
Igel, M., Sudhop, T., and von Bergmann, K. (2002). Pharmacology of 3-hydroxy-3-methylglutaryl-coenzyme A reductase inhibitors (statins), including rosuvastatin and pitavastatin. J. Clin. Pharmacol. 42 (8), 835–845. doi:10.1177/009127002401102731
Janakiram, N. B., Mohammed, A., Bryant, T., Zhang, Y., Brewer, M., Duff, A., et al. (2016). Potentiating NK cell activity by combination of Rosuvastatin and Difluoromethylornithine for effective chemopreventive efficacy against Colon Cancer. Sci. Rep. 6, 37046. doi:10.1038/srep37046
Kamat, A. M., and Nelkin, G. M. (2005). Atorvastatin: a potential chemopreventive agent in bladder cancer. Urology 66 (6), 1209–1212. doi:10.1016/j.urology.2005.06.075
Kang, M., Jeong, C. W., Ku, J. H., Kwak, C., and Kim, H. H. (2014). Inhibition of autophagy potentiates atorvastatin-induced apoptotic cell death in human bladder cancer cells in vitro. Int. J. Mol. Sci. 15 (5), 8106–8121. doi:10.3390/ijms15058106
Kaye, J. A., and Jick, H. (2004). Statin use and cancer risk in the general Practice research database. Br. J. Cancer 90 (3), 635–637. doi:10.1038/sj.bjc.6601566
Kuo, C. C., Chiu, H. F., Lee, I. M., Kuo, H. W., Lee, C. T., and Yang, C. Y. (2012). Statin use and the risk of bladder cancer: a population-based case-control study. Expert Opin. Drug Saf. 11 (5), 733–738. doi:10.1517/14740338.2012.712960
Kurki, M. I., Karjalainen, J., Palta, P., Sipila, T. P., Kristiansson, K., Donner, K. M., et al. (2023). FinnGen provides genetic insights from a well-phenotyped isolated population. Nature 613 (7944), 508–518. doi:10.1038/s41586-022-05473-8
Lawlor, D. A., Harbord, R. M., Sterne, J. A., Timpson, N., and Davey Smith, G. (2008). Mendelian randomization: using genes as instruments for making causal inferences in epidemiology. Stat. Med. 27 (8), 1133–1163. doi:10.1002/sim.3034
Leal, J., Luengo-Fernandez, R., Sullivan, R., and Witjes, J. A. (2016). Economic burden of bladder cancer across the European union. Eur. Urol. 69 (3), 438–447. doi:10.1016/j.eururo.2015.10.024
Lenis, A. T., Lec, P. M., Chamie, K., and Mshs, M. D. (2020). Bladder cancer: a review. JAMA 324 (19), 1980–1991. doi:10.1001/jama.2020.17598
Li, R., Peng, L., Deng, D., Li, G., and Wu, S. (2023). Potential causal association between aspirin use and erectile dysfunction in European population: a Mendelian randomization study. Front. Endocrinol. (Lausanne) 14, 1329847. doi:10.3389/fendo.2023.1329847
Li, R., Peng, L., Deng, D., Li, G., and Wu, S. (2024). Causal relationship between Alzheimer's disease and prostate cancer: a bidirectional Mendelian randomization analysis. Front. Endocrinol. (Lausanne) 15, 1354528. doi:10.3389/fendo.2024.1354528
Liu, Z., Alsaggaf, R., McGlynn, K. A., Anderson, L. A., Tsai, H. T., Zhu, B., et al. (2019). Statin use and reduced risk of biliary tract cancers in the UK Clinical Practice Research Datalink. Gut 68 (8), 1458–1464. doi:10.1136/gutjnl-2018-317504
Lustman, A., Nakar, S., Cohen, A. D., and Vinker, S. (2014). Statin use and incident prostate cancer risk: does the statin brand matter? A population-based cohort study. Prostate Cancer Prostatic Dis. 17 (1), 6–9. doi:10.1038/pcan.2013.34
Mariotto, A. B., Enewold, L., Zhao, J., Zeruto, C. A., and Yabroff, K. R. (2020). Medical care costs associated with cancer survivorship in the United States. Cancer Epidemiol. Biomarkers Prev. 29 (7), 1304–1312. doi:10.1158/1055-9965.EPI-19-1534
Morales, D. R., and Morris, A. D. (2015). Metformin in cancer treatment and prevention. Annu. Rev. Med. 66, 17–29. doi:10.1146/annurev-med-062613-093128
Mullen, P. J., Yu, R., Longo, J., Archer, M. C., and Penn, L. Z. (2016). The interplay between cell signalling and the mevalonate pathway in cancer. Nat. Rev. Cancer 16 (11), 718–731. doi:10.1038/nrc.2016.76
Okubo, K., Miyai, K., Kato, K., Asano, T., and Sato, A. (2021). Simvastatin-romidepsin combination kills bladder cancer cells synergistically. Transl. Oncol. 14 (9), 101154. doi:10.1016/j.tranon.2021.101154
Parada, B., Reis, F., Pinto, A., Sereno, J., Xavier-Cunha, M., Neto, P., et al. (2012). Chemopreventive efficacy of Atorvastatin against nitrosamine-induced rat bladder cancer: antioxidant, anti-proliferative and anti-inflammatory properties. Int. J. Mol. Sci. 13 (7), 8482–8499. doi:10.3390/ijms13078482
Patel, K. K., and Kashfi, K. (2022). Lipoproteins and cancer: the role of HDL-C, LDL-C, and cholesterol-lowering drugs. Biochem. Pharmacol. 196, 114654. doi:10.1016/j.bcp.2021.114654
Richard, P. O., Ahmad, A. E., Bashir, S., Hamilton, R. J., Nam, R. K., Leao, R., et al. (2017). Effect of statins as a secondary chemopreventive agent among individuals with non-muscle-invasive bladder cancer: a population-based analysis. Urol. Oncol. 35 (6), 342–348. doi:10.1016/j.urolonc.2016.12.009
Richters, A., Aben, K. K. H., and Kiemeney, L. (2020). The global burden of urinary bladder cancer: an update. World J. Urol. 38 (8), 1895–1904. doi:10.1007/s00345-019-02984-4
Rosch, P. J., and McCully, K. (2013). Statin use and reduced cancer-related mortality. N. Engl. J. Med. 368 (6), 576. doi:10.1056/NEJMc1214827
Sekula, P., Del Greco, M. F., Pattaro, C., and Kottgen, A. (2016). Mendelian randomization as an approach to assess causality using observational data. J. Am. Soc. Nephrol. 27 (11), 3253–3265. doi:10.1681/ASN.2016010098
Skrivankova, V. W., Richmond, R. C., Woolf, B. A. R., Davies, N. M., Swanson, S. A., VanderWeele, T. J., et al. (2021a). Strengthening the reporting of observational studies in epidemiology using mendelian randomisation (STROBE-MR): explanation and elaboration. BMJ 375, n2233. doi:10.1136/bmj.n2233
Skrivankova, V. W., Richmond, R. C., Woolf, B. A. R., Yarmolinsky, J., Davies, N. M., Swanson, S. A., et al. (2021b). Strengthening the reporting of observational studies in epidemiology using mendelian randomization: the STROBE-MR statement. JAMA 326 (16), 1614–1621. doi:10.1001/jama.2021.18236
Symvoulidis, P., Tsioutis, C., Zamboglou, C., and Agouridis, A. P. (2023). The effect of statins on the incidence and prognosis of bladder cancer: a systematic review and meta-analysis. Curr. Oncol. 30 (7), 6648–6665. doi:10.3390/curroncol30070488
Wang, C., Tao, W., Wang, Y., Bikow, J., Lu, B., Keating, A., et al. (2010). Rosuvastatin, identified from a zebrafish chemical genetic screen for antiangiogenic compounds, suppresses the growth of prostate cancer. Eur. Urol. 58 (3), 418–426. doi:10.1016/j.eururo.2010.05.024
Wang, G., Cao, R., Wang, Y., Qian, G., Dan, H. C., Jiang, W., et al. (2016). Simvastatin induces cell cycle arrest and inhibits proliferation of bladder cancer cells via PPARγ signalling pathway. Sci. Rep. 6, 35783. doi:10.1038/srep35783
Xia, C., Dong, X., Li, H., Cao, M., Sun, D., He, S., et al. (2022). Cancer statistics in China and United States, 2022: profiles, trends, and determinants. Chin. Med. J. Engl. 135 (5), 584–590. doi:10.1097/CM9.0000000000002108
Yeh, J. J., Lai, J. N., Lin, C. L., Hsu, C. Y., and Kao, C. H. (2021). Time-dependent propensity-matched general population study of the effects of statin use on cancer risk in an interstitial lung disease and pulmonary fibrosis cohort. BMJ Open 11 (10), e047039. doi:10.1136/bmjopen-2020-047039
Yokomichi, H., Nagai, A., Hirata, M., Tamakoshi, A., Kiyohara, Y., Kamatani, Y., et al. (2017). Statin use and all-cause and cancer mortality: BioBank Japan cohort. J. Epidemiol. 27 (3S), S84–S91. doi:10.1016/j.je.2016.12.011
Zeybek, N. D., Gulcelik, N. E., Kaymaz, F. F., Sarisozen, C., Vural, I., Bodur, E., et al. (2011). Rosuvastatin induces apoptosis in cultured human papillary thyroid cancer cells. J. Endocrinol. 210 (1), 105–115. doi:10.1530/JOE-10-0411
Zhang, K., Li, A., Zhou, J., Zhang, C., and Chen, M. (2023). Genetic association of circulating C-reactive protein levels with idiopathic pulmonary fibrosis: a two-sample Mendelian randomization study. Respir. Res. 24 (1), 7. doi:10.1186/s12931-022-02309-x
Zhang, X. L., Geng, J., Zhang, X. P., Peng, B., Che, J. P., Yan, Y., et al. (2013). Statin use and risk of bladder cancer: a meta-analysis. Cancer Causes Control 24 (4), 769–776. doi:10.1007/s10552-013-0159-3
Keywords: Mendelian Randomization, statin, bladder cancer, causal analysis, GWAS
Citation: Li R, Huang G, Li Y, Huang M, Huang Y, Li Y, Li G and Wu S (2024) Assessing the role of statin therapy in bladder cancer: evidence from a Mendelian Randomization study. Front. Pharmacol. 15:1427318. doi: 10.3389/fphar.2024.1427318
Received: 03 May 2024; Accepted: 02 July 2024;
Published: 19 July 2024.
Edited by:
Lei Yin, Shanghai Jiaotong University School of Medicine, ChinaCopyright © 2024 Li, Huang, Li, Huang, Huang, Li, Li and Wu. This is an open-access article distributed under the terms of the Creative Commons Attribution License (CC BY). The use, distribution or reproduction in other forums is permitted, provided the original author(s) and the copyright owner(s) are credited and that the original publication in this journal is cited, in accordance with accepted academic practice. No use, distribution or reproduction is permitted which does not comply with these terms.
*Correspondence: Guangzhi Li, Z3Vhbmd6aGlsaUBlbWFpbC5zenUuZWR1LmNu; Song Wu, c29uZ3d1X2x6dUAxMjYuY29t
†These authors have contributed equally to this work