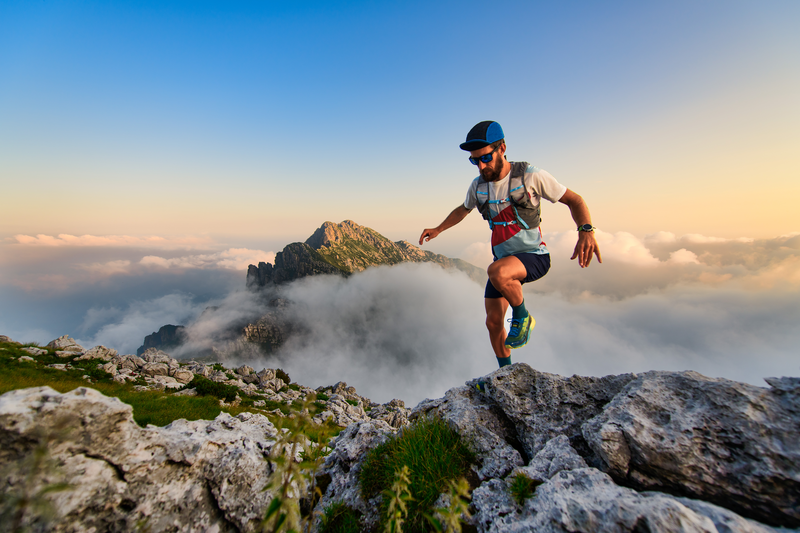
95% of researchers rate our articles as excellent or good
Learn more about the work of our research integrity team to safeguard the quality of each article we publish.
Find out more
ORIGINAL RESEARCH article
Front. Pharmacol. , 16 August 2024
Sec. Ethnopharmacology
Volume 15 - 2024 | https://doi.org/10.3389/fphar.2024.1424803
Background and aim: Pathological changes in the central nervous system (CNS) begin before the clinical symptoms of Alzheimer’s Disease (AD) manifest, with the hippocampus being one of the first affected structures. Current treatments fail to alter AD progression. Traditional Chinese medicine (TCM) has shown potential in improving AD pathology through multi-target mechanisms. This study investigates pathological changes in AD hippocampal tissue and explores TCM active components that may alleviate these changes.
Methods: GSE5281 and GSE173955 datasets were downloaded from GEO and normalized to identify differentially expressed genes (DEGs). Key functional modules and hub genes were analyzed using Cytoscape and R. Active TCM components were identified from literature and the Pharmacopoeia of the People’s Republic of China. Enrichment analyses were performed on target genes overlapping with DEGs.
Result: From the datasets, 76 upregulated and 363 downregulated genes were identified. Hub genes included SLAMF, CD34, ELN (upregulated) and ATP5F1B, VDAC1, VDAC2, HSPA8, ATP5F1C, PDHA1, UBB, SNCA, YWHAZ, PGK1 (downregulated). Literature review identified 33 active components from 23 herbal medicines. Target gene enrichment and analysis were performed for six components: dihydroartemisinin, berberine, naringenin, calycosin, echinacoside, and icariside II.
Conclusion: Mitochondrial to synaptic vesicle dysfunction pathways were enriched in downregulated genes. Despite downregulation, UBB and SNCA proteins accumulate in AD brains. TCM studies suggest curcumin and echinacoside may improve hippocampal pathology and cognitive impairment in AD. Further investigation into their mechanisms is needed.
Alzheimer’s disease (AD) is a progressive neurodegenerative disorder and the most common form of dementia (Power et al., 2019). Over the past 3 decades, both the prevalence and mortality rates of AD have increased significantly (Li et al., 2022). In 2019, an estimated 55 million people worldwide were living with dementia, and the annual cost of diagnosing and treating AD reached $1.3 trillion (World Health Organization, 2023). This makes AD a leading cause of disability among older adults. The diagnosis of AD primarily relies on biomarkers such as amyloid-beta (Aβ) deposition, Tau pathology, and neurodegenerative or neuronal lesions (Jack et al., 2018), along with clinical manifestations including cognitive decline and psychoneurobehavioral changes (McKhann et al., 2011). When the cerebral cortex regions responsible for language and social behavior are affected, patients often lose the ability to live independently (National Institute on Aging). Retrospective studies have confirmed that AD lesions can be detected in the central nervous system (CNS) more than 20 years before the onset of clinical symptoms (Bateman et al., 2012), a stage defined as “preclinical AD” (Sperling et al., 2011). Following this prolonged period of preclinical decline, patients with AD eventually develop complex cognitive deficits (Monsell et al., 2014).
The hippocampus, as the brain structure where lesions are first detected, has received significant attention in the treatment of AD due to its prolonged pathological process. The hippocampus is crucial for learning and memory (Squire, 1992) and is involved in complex behavioral processes such as reinforcement learning and assisted decision-making (Ballard et al., 2019). Hippocampal lesions are considered a vital pathological process in AD. The accumulation of neurofibrillary tangles (NFTs) and Aβ plaques in the hippocampus is central to AD pathology (Calvo-Flores Guzmán et al., 2020). In the early stages of AD, damage to the connectivity between memory-related neurons in the hippocampus occurs (National Institute on Aging), and the degree of volumetric hippocampal atrophy is positively correlated with cognitive decline in AD patients (Mormino et al., 2009). Clinical studies have examined the microstructure of the hippocampus and assessed AD lesions using various analytical methods such as diffusion tensor imaging (DTI) (Zarei et al., 2013), seed-based correlation analysis (SCA) (Fellgiebel and Yakushev, 2011), and multivariate morphometric statistics (MMS) (Zheng et al., 2023). Clinical treatments for AD, including cholinesterase inhibitors and N-methyl-D-aspartate antagonists, alleviate clinical cognitive and behavioral symptoms but do not alter the progression of the disease (Passeri et al., 2022).
Traditional Chinese medicine (TCM) has a long history in treating dementia. Clinical studies and in vitro experiments have confirmed that many TCM therapies can alleviate cognitive symptoms in AD patients and improve the pathological damage associated with AD (Pei et al., 2020). TCM exerts a synergistic effect in AD patients by targeting multiple pathways involved in the pathological progression of AD (Zhang Y. et al., 2015), including the ubiquitin-proteasome pathway, the autophagy-lysosome pathway, and the NF-κB pathway (Ding et al., 2022).TCM has been proven effective in the early prevention of AD and in enhancing brain activity in individuals with AD (Zhang et al., 2019).
In this study, we focused on hippocampal tissue-specific expressed genes and comprehensively applied bioinformatics analyses to screen and identify hub genes and signaling pathways associated with AD hippocampal lesions. Additionally, we conducted a literature review to investigate the potential targets and mechanisms of action of TCM components in improving cognition in AD.
Figure 1 shows a flow diagram that outlines the various stages of our research process. In the Gene Expression Omnibus (GEO) database, the search terms “Alzheimer’s disease [MeSH Terms],” “Alzheimer’s disease,” “AD Alzheimer’s disease,” “AD,” “Alzheimer Dementia,” “Senile Dementia,” and “Homo sapiens” were used. The search aimed to obtain GSE (Gene Expression Omnibus Series) datasets, including high-throughput sequencing and microarray data, which were then screened according to the following criteria:
Inclusion criteria: 1) GSE dataset information or references specify inclusion criteria for AD and control samples; 2) GSE contains hippocampal tissue; 3) GSE includes both AD and control samples; 4) GSE data are RNA expression levels.
Exclusion criteria: No raw data available.
For sample selection of included GSE studies: 1) GSM (Gene Expression Omnibus Sample) samples of hippocampal tissue were included, and the total sample size should be greater than 10; 2) GSMs without clinical information were excluded.
Data pre-processing for the microarray dataset was performed using R-based software (R version 4.3.2). After reading the raw data with the “affy” package (Gautier et al., 2004), background correction was applied using the Robust Multiarray Average (RMA) method (Irizarry et al., 2003), and gene expression averages were calculated for the same gene probe detection results. Differential expression analysis was conducted using the “limma” package (Ritchie et al., 2015).
For high-throughput data, pre-processing was carried out using FastQC and Trimmomatic (Bolger et al., 2014) for quality control and assessment of raw data. After filtering out low-quality results, the data were converted to count form using Hierarchical Indexing for Spliced Alignment of Transcripts 2 (HISAT2) (Kim et al., 2015). Differential expression analysis was then performed using the “DESeq2” package (Love et al., 2014) (R version 4.3.2), and genes with an adjusted p-value <0.05 were selected as differentially expressed genes (DEGs). Heatmaps and volcano plots for each included dataset were generated using the “ggplot2” package (Wickham, 2016).
Changes in gene expression were assessed based on the sign of the log fold change (logFC). The intersection of upregulated and downregulated DEGs from each dataset was selected for further analysis.
Gene Ontology (GO) and Kyoto Encyclopedia of Genes and Genomes (KEGG) pathway analyses were performed using the ‘clusterProfiler’ package (Yu et al., 2012) (R version 4.3.2). Thresholds of P < 0.05 and q < 0.05 were set for both GO and KEGG analyses to identify pathways with significant differences. The results were visualized using the “enrichplot” (Yu, 2021) and “ggplot2” packages.
The intersection of DEGs from each GSE dataset was analyzed using the STRING (https://string-db.org/) online database (Szklarczyk et al., 2023) to construct protein-protein interaction (PPI) networks with moderate confidence (scores >0.4). The PPI networks were visualized using Cytoscape software (Shannon et al., 2003) (version 3.10.1). Cytoscape’s plug-in, Molecular Complex Detection (MCODE)(Bader and Hogue, 2003), was used to analyze key functional modules with the following selection criteria: K-core = 2, degree cutoff = 2, max depth = 100, and node score cutoff = 0.2. The “clusterProfiler” package was used for KEGG and GO analyses of the genes in each module.
The cytoHubba plugin of Cytoscape (v 3.9.0) was used to score each node gene using 10 randomly selected algorithms, including Degree, EPC (Edge Percolated Component), MNC (Maximum Neighbourhood Component), BNC (Biological Network Centrality), BottleNeck, EcCentricity, Closeness, Betweenness, Clustering Coefficient, MCC (Maximum Clique Centrality), Radiality, and Stress (Chin et al., 2014). The top 15 hub genes from each algorithm were used to identify hub genes, which were then visualized using the ‘UpSetR’ package (Conway et al., 2017).
Studies were identified through a comprehensive search in the following databases: China National Knowledge Infrastructure (CNKI), Wan Fang database, SinoMed, China Science and Technology Journal Database, PubMed, Cochrane Library, and Web of Science. The search covered studies on TCM for AD published from the inception of the databases to January 2024, using a combination of subject terms and free terms. The results were included in EndNote X9 (version 3.3) for screening.
We reviewed experimental animal studies on the treatment of AD with TCM in rats and mice. The literature inclusion criteria were as follows: 1) The Morris water maze test showed a significant difference in behavioral tests between the treatment group and the model group (p < 0.05); 2) Hippocampal tissue analysis from the experimental animals showed a significant difference between the TCM treatment group and the model group (P < 0.05); 3) The therapeutic drug was a TCM active component or a single Chinese medicine decoction piece.
Exclusion criteria were as follows: 1) Animal models other than rats and mice; 2) AD models constructed by the investigators themselves through surgery.
For literature that does not list the specific chemical composition of TCM: 1) By comparing with the relevant parts of the Pharmacopoeia of the People’s Republic of China 2020 edition (Pharmacopoeia) (https://ydz.chp.org.cn/#/main), if the extraction method in the literature is the same as that in the Pharmacopoeia, the active components of TCM specified in the Pharmacopoeia were used for the analyses; 2) Relevant literature on the active components of the corresponding TCM was searched for assessment.
An initial screening was performed by two authors (Du Yida, He Chunying) based on the titles and abstracts of the literature retrieved from the database to exclude irrelevant studies, including clinical trials, reviews, non-TCM treatments, and pharmacological studies. The literature was then screened a second time using the full-text content based on the inclusion and exclusion criteria. Disagreements in the assessment of the content were adjudicated by a third author (Zhan Min).
Information on the design of the AD versus control model, sample size, therapeutic agents, and treatment duration of the included studies was extracted. Using P < 0.05 as a criterion, behavioral test results with significant differences and outcome indicators related to the hippocampus of experimental animals were screened as baseline information.
The Chemical Abstracts Service (CAS) (https://commonchemistry.cas.org/) or the National Drug Reference Standards (http://aoc.nifdc.org.cn/sell/home/) were used to search for the names of the TCM components in the included studies to obtain the CAS numbers. Both the CAS numbers and the names of the TCM components were searched in the PubChem database (https://pubchem.ncbi.nlm.nih.gov/) to identify the target genes. The target genes of the active components of TCM that were present in each DEG (adjusted P-value <0.05) of the included GSE datasets were selected. Core modules were analyzed for GO and KEGG enrichment using STRING to construct the PPI network, and Cytoscape’s MCODE plugin was used for further analysis. The target genes of the remaining TCM agents were directly enriched and analyzed as before.
A total of 35 patients from two GSE datasets (GSE5281, GSE173955) were included. Detailed information on test platforms, sample size, and patient age for these datasets is shown in the table below (Table 1).
After analyzing GSE5281 using the R software “limma” package and GSE173955 using the “DESeq2” package, the DEGs of the two GSE datasets were obtained. The heatmap and volcano plot of the two GSE datasets are shown in (Figure 2). Taking the intersection of DEGs from the two GSE datasets resulted in 76 upregulated genes (adjusted P-value <0.05, logFC >0) and 363 downregulated genes (adjusted P -value <0.05, logFC <0). For the logFC value and the adjusted P -value of upregulated and downregulated genes, see (Supplementary Material S2).
Figure 2. Heatmaps and volcano plots of the GSE dataset. (A,B): Heatmaps of gene expression of GSE173955 and GSE5281; (C,D): Volcano plots of DEGs of GSE173955 and GSE5281 (adjusted P-value < 0.05).
GO annotations of DEGs consisted of three categories: CC (Cellular Component), BP (Biological Process), and MF (Molecular Function), which were used to analyze DEG functional enrichment. KEGG analysis was conducted to determine the relationship between DEGs and signaling pathways.
After GO and KEGG enrichment analyses of all upregulated gene processes, only the results of the biological process and molecular function categories of the GO analysis were obtained. The upregulated genes are involved in epidermal cell differentiation and developmental pathways in terms of biological processes, while in terms of molecular function, they are associated with prostaglandin signaling (PTGIR, PTGER1) (Table 2).
After cluster analysis of the downregulated genes by MCODE, the higher-scoring clustering modules were subjected to GO and KEGG enrichment analyses. The top 10 significantly enriched terms in each GO category were identified (Benjamini and Yosef, 1995).
The enrichment results were broadly classified into three categories: 1) Mitochondrial structure-function related pathways, including mitochondrial ribosomes, mitochondrial gene expression, and mitochondrial endomembrane composition; 2) Cellular energy metabolism pathways, including cellular respiration, ATPase activity, and oxidative phosphorylation; 3) Synaptic vesicle related pathways. See (Table 3) and (Figure 3). This is consistent with previous studies that found mitochondrial damage and presynaptic vesicle loss in the synapses of nerve cells in AD patients, and the two often influence each other (Wang et al., 2023). In addition, downregulated genes were enriched for a variety of degenerative neuropathies, including Alzheimer’s disease, Parkinson’s disease, prion diseases, and Huntington’s disease. For specific results of enrichment analyses of upregulated genes and individual modules of downregulated genes, see (Supplementary Material S3).
Figure 3. Major functional modules of downregulated genes. (A) Downregulated gene module 1 (MCODE score = 11750, number of genes = 17); (B) Downregulated gene module 2 (MCODE score = 8552, number of genes = 30).
In total, 76 upregulated genes and 363 downregulated genes were analyzed separately to characterize potential PPIs using the online STRING database. PPIs with a moderate confidence score ≥0.4 were selected and then imported into Cytoscape for further complex network analysis (Figure 4). The MCC, DMNC, MNC, Degree, EPC, BottleNeck, EcCentricity, Closeness, Radiality, Betweenness, Stress, and Clustering Coefficient algorithms of CytoHubba were used to integrate and rank the hub genes. The top 15 hub genes from each algorithm were used to screen hub genes through the ‘UpSetR’ package (Figure 5).
Figure 4. PPI network diagram of up- and downregulated genes. (A) Upregulated genes; (B) Uownregulated genes.
Figure 5. Screening of up- and downregulated hub genes using the “UpSetR” package. The highlighted regions in red boxes indicate the hubgenes identified through the “UpSetR” package. (A) Upregulated genes; (B) Downregulated genes.
Ultimately, three upregulated hub genes (SLAMF, CD34, ELN) and ten downregulated hub genes (ATP5F1B, VDAC1, VDAC2, HSPA8, ATP5F1C, PDHA1, UBB, SNCA, YWHAZ, PGK1) were filtered, uploaded to STRING, and mapped (Figure 6). After determining the hub genes, we used the UniProt Knowledgebase/Swiss-Prot (UniProtKB/Swiss-Prot) database to retrieve detailed information and provide comments about these genes, as shown in (Table 4).
Following the differential expression analysis of hippocampal tissue samples from AD patients, we explored the effects of TCM on hippocampal pathology in AD animal models. After rigorously and systematically searching the databases, 1560 unique records were identified in EndNote, and the titles and abstracts were screened. The full texts of 120 articles were assessed for eligibility, and 35 studies were included in this study (Figure 7). See (Supplementary Material S4) for the baseline information from the included studies. The risk of bias in the included studies was assessed using the 10 items of SYRCLE’s Risk of Bias Tool (Hooijmans et al., 2014), with the results presented in (Supplementary Material S5).
A total of 35 papers were included (Zhou, 2009; Wang H., 2011; Wang P. W., 2011; Nie, 2011; Wei, 2013; Zhang Z.-W. et al., 2015; Dang, 2015; Fan, 2015; Li, 2015; Li et al., 2016; Yuan, 2016; Chen C., 2017; Chen Y. W., 2017; Gao, 2017; Yan, 2017; Yang, 2017; Gu, 2018; Liu, 2018; Shi et al., 2018; Yu, 2018; Chen, 2019; Liu, 2019; Piao et al., 2019; Yang, 2019; Zhang, 2019; Zhu, 2019; Kong F. G., 2020; Kong Y. Y., 2020; Jiang et al., 2020; Ranran et al., 2020; Wang XF. et al., 2021; Wu, 2021; Wang, 2022; Zhao, 2022; He, 2023). Of these, 32 papers used APP/PS1 mice as AD model animals and C57/BL6J mice or wild-type mice as controls. Three papers used Senescence-Accelerated Mouse Prone 8 (SAMP8) mice as AD model animals and Senescence-Accelerated Mouse Resistant 1 (SAMR1) mice as controls. All animals were 3–9 months old. Among them, 10 (28.57%) were less than 3 months old, 17 (48.57%) were 3–6 months old, and 6–9 (22.86%) were 6–9 months old, as shown in (Figure 8). In total, 33 active components from 23 TCMs were included. Thirteen active components from seven TCMs were identified by reviewing Pharmacopoeia and other relevant studies. See (Supplementary Material S6) for the Latin names of TCMs and the active components of TCMs in English and Chinese.
Figure 8. Age Distribution of Experimental Animals in the Included Studies Altogether, nine active components of TCM were not found as target genes in PubChem: β-asarone (Nie, 2011), tetrahydroxystilbene glucoside (Chen C., 2017), schisandrin (Piao et al., 2019), smilagenin (Yang, 2019), safflower yellow B (Shi et al., 2018), 3,6′-disinapoyl sucrose (Wang XF. et al., 2021), pinoresinol diglucoside (Yu, 2018), rehmannioside D (Zhao, 2022), and onjisaponin B (Li et al., 2016). Valganciclovir (Liu, 2019) and neoeriocitrin (Gu, 2018), on the other hand, lack gene targets from humans.
Analyzing the changes in hippocampal tissue-related indices from the included studies revealed that various active components of TCM effectively improved Aβ deposition and Tau phosphorylation lesions in hippocampal tissue. These TCM components ameliorate histopathological damage in the hippocampus of AD model mice through various pathways, such as regulating glial cell activity, attenuating oxidative stress, reducing cellular inflammation, and acting on the insulin and estrogen pathways.
Based on the results of the enrichment analyses of DEGs, various TCM components were identified with mechanisms of action related to mitochondrial autophagy and synaptic function.
For late-stage AD model mice, TCM treatments improved neuronal autophagy and synaptic function. Geniposide (6 months old) (Zhang, 2019) enhanced autophagy by inhibiting the mTOR signaling pathway, improving hippocampal lesions in AD model animals, as evidenced by increased expression of LC3-II and Beclin1, and decreased p62 protein levels. Total alkaloids of Dendrobium nobile Lindl. (7 months old) (Yang, 2017) and tetrahydroxy-stilbene glucoside (4–5 months old) (Chen C., 2017) increased synaptophysin protein content in hippocampal tissue, improving synaptic function. Additionally, tetrahydroxy-stilbene glucoside has a protective effect on nerve cells and is effective in neurological diseases such as Parkinson’s disease and vascular dementia (Wang et al., 2019).
Various TCM components demonstrated therapeutic effects at the neuronal and hippocampal tissue levels in AD model mice at different pathological stages: 1) For neuronal inflammation, drugs such as calycosin (7–8 months old) (Gao, 2017) and curcumin (8 months old) (Chen Y. W., 2017) reduced inflammatory responses in hippocampal neuronal cells; 2) In hippocampal tissue, components such as dihydroartemisinin (6 months old) (He, 2023), berberine (6 months old) (Wu, 2021), total alkaloids of D. nobile Lindl. (7 months old) (Yang, 2017), and forsythoside B (8 months old) (Kong F. G., 2020) modulated glial cell activity; curcumin (3 months old) (Wang H., 2011; Dang, 2015), tetrahydroxy-stilbene glucoside (4–5 months old) (Chen C., 2017), and total alkaloids of D. nobile Lindl. (7 months old) (Yang, 2017) improved synaptic function in hippocampal neuronal cells; 3) Some TCM components are related to hormonal pathways: curcumin (3 months old) (Wang H., 2011; Dang, 2015) and valganciclovir (6 months old) (Liu, 2019) modulated the insulin pathway, while extracts of Eucommia ulmoides Oliv. (3 months old) (Yu, 2018), Drynaria roosii Nakaike (3 months old) (Gu, 2018), and Cullen corylifolium (L.) Medik. (3 months old) (Liu, 2018) exerted neuroprotective effects through the estrogenic pathway; 4) Extracts of Rehmannia glutinosa (Gaertn.) DC (4 months old)(Zhao, 2022) and dihydroartemisinin (6 months old) (He, 2023) repaired blood-brain barrier damage.
A total of 30.79% of the target genes of the included components were found in the DEGs of the GSE173955 and GSE5281 datasets, with the exception of geniposide (Zhang, 2019), forsythoside A (Wang, 2022), and notopterol (Jiang et al., 2020) (Table 5). Among them, dihydroartemisinin (He, 2023) had the highest percentage (49.53%) and number (53) of target genes in DEGs. The downregulated hub genes are included in the target genes of dihydroartemisinin (He, 2023) (HSPA8, PGK1) and echinacoside (Yuan, 2016) (SNCA). The TCM components with a high number of target genes contained in the DEGs were analyzed using the MCODE plug-in, and then the clustering module was used for GO and KEGG pathway enrichment. We searched the UniProt Knowledgebase/Swiss-Prot database to annotate the target genes of each active component of TCM. See (Supplementary Material S7).
In animal experiments (He, 2023), DHA, the first-generation derivative of artemisinin, was found to inhibit the TLR4/MyD88/NF-kB pathway, reduce the inflammatory response in the hippocampal tissue of 6-month-old AD model mice, and decrease the permeability of the blood-brain barrier. The visualization results of the target genes of DHA are shown in (Table 6). The enrichment analysis results and the upregulation and downregulation of target genes in the differential expression analysis mentioned earlier are presented in (Table 7).
The target genes of DHA are enriched in pathways related to ribosomal structure and the Hypoxia-inducible factor 1 (HIF-1) signaling pathway and are downregulated in the DEGs. According to PubChem’s target gene information, cell experiments have confirmed that DHA interacts with various ribosomal proteins. Additionally, DHA interacts with Enolase 1 (ENO1), L-lactate dehydrogenase A (LDHA), and Phosphoglycerate Kinase 1 (PGK1), which are involved in the HIF-1 signaling pathway (Ravindra et al., 2015), though the mechanisms of action require further investigation. Furthermore, DHA targets HNRNPK and PPIA (Ravindra et al., 2015), promoting the degradation of Myc protein (Wang et al., 2015), which is associated with the endogenous apoptosis signaling pathway. DHA also promotes ROS generation in Molt-4 cells, thereby inducing an increase in SOD2, reflecting its cytotoxic effects (Bhatt et al., 2021).
The earliest mention of Artemisia annua L. for the treatment of various types of malaria was in the Zhouhou Beiji Fang. Currently, DHA is widely used in the clinical treatment of malaria and plays a powerful role in inhibiting a variety of inflammation-related diseases (Yu et al., 2021). Chinese medicine considers A. annua L. to have a cooling effect, commonly used in the treatment of febrile diseases (Zhang et al., 2016). This study found that MYC is an upregulated DEG in AD, consistent with the co-localization of phosphorylated c-Myc with abnormal Tau protein deposition in AD pathology (Ferrer et al., 2001a). DHA selectively induced the degradation of c-Myc in a proteasome-dependent manner in tumor cells overexpressing c-Myc (Lu et al., 2010), but its effect on c-Myc in neuronal cells still requires further investigation. Additionally, DHA can alleviate lipopolysaccharide-induced neuroinflammation and reduce neuronal damage in the hippocampus of mice by inhibiting the PI3K/AKT signaling pathway (Gao et al., 2020).
Additionally, DHA has been shown to reduce blood-brain barrier permeability in a sepsis model by increasing the expression of the tight junction protein occludin (OCLN) (Liu et al., 2022). However, the mechanisms by which it affects blood-brain barrier permeability in AD still require further investigation.
Berberine, an active component in the TCM Coptis, has been shown to inhibit the endoplasmic reticulum stress PERK/eIF2α pathway (Wu, 2021), reducing Aβ42 deposition and Tau hyperphosphorylation in the hippocampal neurons of 6-month-old APP/PS1 mice. The visualization results of the target genes of berberine are shown in (Table 8), and the enrichment analysis results are presented in (Table 9).
According to the target gene information from PubChem, in a Parkinson’s disease cell model, berberine inhibits the activation of the NF-κB pathway by modulating the LINC00943/miR-142-5p/KPNA4 axis, thereby reducing the nuclear translocation of the transcription factor p65 (RELA) (Li et al., 2021). Additionally, other target genes are linked to berberine’s mechanisms in treating various cancers. Specifically, genes enriched in the neutrophil extracellular trap (NET) pathway, such as H3C7, H3C10, and HAT1, play a role in berberine’s therapeutic effects on acute myeloid leukemia (Wang et al., 2016).
Berberine exerts neuroprotective effects through various molecular mechanisms by modulating multiple signaling pathways, including NF-κB and JNK (Tian et al., 2023). Research on berberine and its target genes reveals that in a lipopolysaccharide-stimulated RAW264.7 macrophage model, berberine inhibits the NF-κB signaling pathway by activating SIRT1 (Zhang et al., 2017) and exerts anti-inflammatory effects via the p300 (EP300)/p65lys310 axis (Zhang S. et al., 2023). Additionally, EGFR exhibits significant immunoreactivity around neuroplaque in AD patients (Birecree et al., 1988). Molecular docking analysis shows stable binding between berberine and the EGFR target, although no significant differences in EGFR mRNA levels were observed in the brain tissues of AD model mice (3 × Tg AD mice) (Wei et al., 2023).
Modern Chinese medicine believes that the pathogenesis of AD can be summarized as “toxins damaging brain collaterals” (Su et al., 2011). The 2023“Guideline for the Diagnosis and Treatment of Alzheimer’s Disease with Integrated Chinese and Western Medicine” suggests that Huanglian Jiedu Decoction (with Coptis as the principal Chinese medicine decoction piece) can clear heat and detoxify, improving cognition in patients with MMSE scores ≤10. Other studies have found that berberine reduces Aβ production by promoting autophagy (Huang et al., 2017), decreases abnormal Tau protein phosphorylation (Chen Y. et al., 2020), and alleviates mitochondrial dysfunction, thereby improving AD pathology (Wong et al., 2021).
The included studies found that D. roosii Nakaike extract with more than 50% flavonoid content exerted neuroprotective effects through the ERβ/P38 pathway (Gu, 2018), reducing cellular inflammation and neuronal apoptosis in the hippocampus, and increasing levels of choline acetyltransferase (ChAT) and acetylcholine (Ach). Referring to the Pharmacopoeia, we consider naringenin as the active component in D. roosii Nakaike.
Naringin is enriched in pathways related to the cellular response to tumor necrosis factor, protein kinase activator activity, membrane raft, and lipid and atherosclerosis (Table 10). Among the enriched genes, CYP1B1 and CYBA are associated with ROS production in AD pathology. CYP1B1, as part of the cytochrome P450 family, promotes oxidative stress in AD (Chen YY. et al., 2020), and cell experiments have confirmed that naringin is a weak inhibitor of CYP1B1 (Shimada et al., 2010). CYBA is an important component of the NADPH oxidase complex (Afsar et al., 2023), responsible for ROS generation, and naringin can inhibit the TNF-α-induced overexpression of CYBA mRNA and protein (Li et al., 2014). The target genes in other enriched pathways are derived from studies on naringin’s mechanisms in treating various cancers.
Multiple behavioral tests have confirmed that naringin, the active component in Drynaria roosii Nakaike, can improve cognition in AD model animals (Poudineh et al., 2022). Naringin has been shown to ameliorate AD neurocyte structural lesions through mechanisms such as reducing Aβ and Tau protein phosphorylation (Meng et al., 2021), modulating the glutamate system (Wang et al., 2013), and chelating excess metals (Prakash et al., 2013). Chinese medicine believes that D. roosii Nakaike has the effect of tonifying the kidneys and strengthening bones. Clinically, it is often used in TCM to treat skeletal diseases such as osteoporosis, osteoarthritis, and fractures (Deng et al., 2022), as well as oral bone-related diseases such as periodontitis and pulpitis (Huang et al., 2023). In an early case report, oral administration of D. roosii Nakaike alone improved limb weakness and cognitive impairment in an AD patient (Yang, 2004).
In vivo, calycosin (active component of Astragalus propinquus Schischkin) has antioxidative stress and anti-inflammatory neuroprotective effects on the hippocampal neurons of 7–8 month old APP/PS1 mice. In vitro, calycosin can attenuate Aβ-induced oxidative stress and inflammation through the PKC/Nrf2 pathway (Gao, 2017).
The active component of Astragalus propinquus Schischkin, calycosin, targets genes in hepatocellular carcinoma cells that are enriched in pathways related to mitigating intracellular oxidative stress (Zhang et al., 2013) (Table 11). Other studies have shown that intraperitoneal injection of calycosin in APP/PS1 mice reduces hippocampal Aβ, Tau, interleukin-1beta (IL-1β), tumor necrosis factor-alpha (TNF-α), acetylcholinesterase, and malondialdehyde levels in a dose-dependent manner (Song et al., 2017). TCM posits that AD involves a deficiency of qi and blood (Li and Gao, 2017), and Astragalus propinquus Schischkin is commonly used in TCM clinical practice for treating AD due to its qi-tonifying and yang-raising properties according to Pharmacopoeia. Astragalus propinquus Schischkin is frequently prescribed in TCM for AD treatment (Song et al., 2019). Other active components of Astragalus, primarily including astragaloside IV (Wu Y. et al., 2021) and astragalus polysaccharides (Yao et al., 2014), have shown potential in ameliorating AD pathology by inhibiting Aβ production and aggregation, preventing neuronal damage or apoptosis, and reducing neuroinflammation (Wang et al., 2024).
According to the Pharmacopoeia, both verbascoside and echinacoside are recognized as active components of cistanche (Cistanche deserticola Y.C.Ma or Cistanche tubulosa (Schenk) Wight). Prophylactic administration of cistanche glycosides increased the resistance of hippocampal neuronal cells to oxidative damage in 5-month-old AD model mice (Yuan, 2016). We performed GO and KEGG enrichment analyses to further investigate the potential roles of the target genes of verbascoside and echinacoside.
Verbascoside targets genes are enriched in pathways related to cellular oxidative stress and aging (Table 12). It inhibits the overexpression of SOD2 mRNA induced by oxidized LDL (Kostyuk et al., 2011). Target genes such as ARNT, MAPK1, RELA (Potapovich et al., 2011), and EGFR (Pastore et al., 2012) are associated with skin inflammatory responses. Other target genes have been identified through cell experiments in prostate cancer (Wu CH. et al., 2021) and acute promyelocytic leukemia (Lee et al., 2007).
Echinacoside is enriched in pathways related to neurological disorders, including synaptic transmission and the regulation of neuronal apoptosis (Table 13). Echinacoside significantly increases the phosphorylation levels of TrkA (NTRK1) and ERK2 (MAPK1) (Zhu et al., 2013), activating the extracellular signal-regulated kinase (ERK) signaling pathway to protect neurons. Additionally, it modulates the ROS/ATF3/CHOP pathway to reduce the expression of apoptotic genes DDIT3 (CHOP) and SNCA (α-synuclein, α-syn), thereby inhibiting apoptosis in Parkinson’s disease cell models (Zhao et al., 2016).
Upon reviewing the literature, we found that the precursor of nerve growth factor (pro-NGF) is increased in the hippocampus (Hock et al., 2000), while the expression of NGF and the TrkA gene (NTRK1) is decreased in cholinergic basal forebrain neurons (Mufson et al., 2008; Mufson et al., 1995), potentially related to reduced retrograde transport of NGF (Schindowski et al., 2008). Additionally, studies have shown that the expression levels of ERK2 (MAPK1) in the hippocampus of AD patients remain unchanged, but phosphorylated ERK2 is increased (Ferrer et al., 2001b; Khezri et al., 2023), which is associated with Tau protein phosphorylation (Kerr et al., 2006). Lewy-related pathology (LRP) in the brains of AD patients is immunohistochemically co-localized with α-syn (SNCA) and Tau pathology (Twohig and Nielsen, 2019a). While α-syn is involved in multiple presynaptic mechanisms, endogenous α-syn depletion has been found to exacerbate neurodegeneration (Chandra et al., 2005).
Our differential expression analysis identified DDIT3 as a downregulated DEG (adjusted P-value = 0.001234713 < 0.05) in GSE5281, which contradicts other studies showing increased expression of CHOP (DDIT3) and other ER stress-related proteins in the hippocampus of AD patients. This discrepancy may be related to the unfolded protein response (UPR) (Ismael et al., 2021): UPR upregulates CHOP via the PERK/eIF2α/ATF4 pathway (Hetz and Mollereau, 2014), with activation occurring in the early stages of neurofibrillary degeneration (Hoozemans et al., 2009), while the GSE5281 samples represent late-stage AD pathology (Braak stages V-VI) (Miller et al., 2008).
TCM posits that “the kidney stores essence and essence houses will,” with kidney deficiency being a primary pathogenesis of cognitive impairment in AD (Zhang M. et al., 2023). According to the Pharmacopoeia, Cistanche deserticola Y.C.Ma has properties of tonifying the kidneys and aphrodisiac, invigorating the blood, and moisturizing the intestines and acting as a laxative. It is included in multiple TCM formulations for treating AD (Wang X. et al., 2021; Dong, 2017; Qi et al., 2017; Ma F. Y., 2018) and can alleviate the common symptom of constipation in AD patients (Wang et al., 2022). Other animal studies have shown that total glycosides of Cistanche inhibit neuronal apoptosis and enhance free radical scavenging in AD model mice (Gu et al., 2016). Calycosin’s neuroprotective effects are associated with regulating neuroinflammation via the NF-κB-p65 pathway (Chen et al., 2022), while echinacoside modulates the PERK/eIF2α pathway to alleviate ER stress in APP/PS1 mice (Dai et al., 2020).
In 9-month-old APP/PS1 mice, icariin II inhibits BACE1 protein levels by regulating hippocampal and cortical PERK/eIF2α phosphorylation levels and PPARγ protein expression, thereby reducing Aβ production. It also inhibits PDE5A expression to protect neuronal cells (Yan, 2017). GO analysis of icariin II target genes revealed enrichment in pathways related to peptide hormone response, protein kinase activity, and cell-substrate adhesion. KEGG analysis indicated involvement in cancer-related pathways.
According to PubChem, Icariin II affects enriched target genes involved in the mechanisms of prostate cancer (Lee et al., 2009), non-small cell lung cancer (Song et al., 2012), and osteosarcoma (Geng et al., 2014) in disease model cells (Table 14). In vivo and in vitro studies have found that Icariin II reduces neuronal damage in AD model animals by activating the BDNF/TrkB/CREB signaling pathway (Liu et al., 2018) and alleviates LPS-induced neuroinflammation by inhibiting the TLR4/MyD88/NF-κB pathway (Zhou et al., 2019).
Chinese medical theory posits that “the kidney nourishes marrow, and the brain is the sea of marrow.” As one ages, the essence and qi in the kidneys gradually decline, leading to brain marrow deficiency and resulting in cognitive impairments such as memory loss (Li et al., 2006). Epimedium brevicornu Maxim. is known for its function of “tonifying the kidney and essence” (Long et al., 2024) and is frequently mentioned in literature analyzing the usage patterns of Chinese medicine decoction pieces for treating AD (Wu S. et al., 2021; Sun et al., 2022; Zhong et al., 2021).
Some of the target genes of the active components of TCM included in the study intersect minimally with DEGs but have effects in reducing Aβ deposition while inhibiting neuroinflammation in APP/PS1 mice. Among these, 1-Deoxynojirimycin has been shown to reduce the expression of neuroinflammatory factors in the hippocampal tissue of 3-month-old SAMP8 mice, possibly through the upregulation of the BDNF/TrkB signaling pathway in the hippocampus (Chen, 2019). Psoralen extract may alleviate the expression of inflammatory factors in the hippocampal tissue of 3-month-old APP/PS1 mice via the ERβ/ERK signaling pathway (Liu, 2018). Additionally, molecular docking studies have indicated that psoralen, an active components of Psoralea corylifolia L. (Somani et al., 2015), and derivatives of 1-Deoxynojirimycin possess cholinesterase inhibitory activity (Ahuja-Casarín et al., 2021).
Multiple studies included in the literature have investigated the mechanisms of curcumin in treating AD. Curcumin, an active component of the Chinese medicine decoction piece Curcuma longa L., has been reported to improve insulin signaling pathway disorders in 3-month-old APP/PS1 mice by activating the PI3K/AKT pathway downstream of insulin receptor substrate 1 (IRS-1), thereby reducing Aβ expression and the formation of Aβ oligomers (ADDLs) in the hippocampal CA1 region (Wang P. W., 2011; Dang, 2015). In 8-month-old APP/PS1 mice, curcumin treatment lowered the levels of IL-1β and TNF-α in the hippocampus and inhibited neuroinflammatory responses (Chen Y. W., 2017), suggesting that curcumin has therapeutic effects in both early and late stages of AD pathology.
Further studies revealed that curcumin can reduce Aβ-activated microglial inflammatory cytokine mRNA and protein levels by inhibiting the ERK1/2 and p38 kinase signaling pathways (Shi et al., 2015). Additionally, curcumin activates the Nrf2 pathway and inhibits the nuclear translocation of NF-κB, reducing LPS-induced NF-κB luciferase activity (Fagiani et al., 2020). Curcumin also binds to Aβ, increasing the helical propensity of Aβ peptides (Salamanova et al., 2021) and inhibiting Aβ aggregation, which suggests its potential as an early diagnostic probe for AD (Chen et al., 2018).
It is currently believed that glycogen synthase kinase-3β (GSK-3β) links senile plaques and neurofibrillary tangles in the pathological changes of AD. In vitro experiments have shown that Aβ activates GSK-3β signaling (Takashima et al., 1996a; Takashima et al., 1996b), leading to abnormal APP processing and synaptic dysfunction (Deng et al., 2014). Additionally, GSK-3β, as one of the Tau kinases (Fuster-Matanzo et al., 2012), directly associates with Tau protein to form functional units (Sun et al., 2002; Chun et al., 2004). Hyperphosphorylated Tau further activates GSK-3β by increasing oxidative stress, neuroinflammation, and apoptosis (Saeki et al., 2011).
Curcumin inhibits Aβ-induced activation of GSK-3β in human neuroblastoma SH-SY5Y cells. The specific mechanism involves curcumin reducing the mRNA and protein expression of PTEN, a negative regulator of PIP3, which in turn increases the levels of the PIP3 phosphorylated substrate Akt. Activated Akt, as an upstream regulator of GSK-3β, inhibits the phosphorylation of GSK-3β at the Ser9 site (Huang et al., 2014).
This study selected GSE datasets (GSE5281, GSE173955) with clearly diagnosed AD, hippocampal tissue samples, and sample sizes of ≥10. We found that the DEGs in the hippocampal tissue of AD patients are enriched in pathways related to mitochondrial structure, mitochondrial energy metabolism, and synaptic vesicles. Using the Cytoscape plugin cytoHubba combined with the “UpSet” package, we identified hub genes, and identified the upregulated hub genes (SLAMF6, CD34, ELN) and downregulated hub genes (ATP5F1B, VDAC1, VDAC2, HSPA8, ATP5F1C, PDHA1, UBB, SNCA, YWHAZ, PGK1). Additionally, we reviewed studies that investigated the improvement of cognitive impairment and hippocampal tissue pathology in AD model animals after treatment with TCM. We analyzed the mechanisms by which these active components of TCM ameliorate AD pathology.
Among the upregulated hub genes, SLAMF6 belongs to the signaling lymphocytic activation molecule (SLAM) family receptors, which are expressed in various immune cells, including T cells, B cells, and NK cells (Yigit et al., 2019). It is a susceptibility gene for systemic lupus erythematosus (Zhong and Veillette, 2008). The upregulation of SLAMF6 may be related to the complex changes in immune cells during neurodegenerative diseases (Heneka, 2020).
The cell-surface protein CD34 is a marker for vascular endothelial cells, hematopoietic progenitor cells, and endothelial progenitor cells (Nielsen and McNagny, 2008). Studies have shown that the number of circulating CD34+ bone marrow progenitor cells (BMPCs) decreases with age (Hajjar et al., 2016). However, an increase in circulating CD34+ progenitor cells has been observed in the peripheral blood of early AD patients (Bigalke et al., 2011). Additionally, the counts of circulating CD34+ BMPCs and early endothelial progenitor cells (EPCs) are negatively correlated with MMSE scores in patients with moderate to severe AD (Stellos et al., 2010). Research suggests that the upregulation of CD34 may be associated with the repair of vascular injury in the central nervous system and treatment with cholinesterase inhibitors (Romaus-Sanjurjo et al., 2023).
The ELN gene encodes elastin, a component of the extracellular matrix (ECM) in the central nervous system, which contributes to the mechanical strength and elasticity of the ECM. Elastin plays a crucial role in neurogenesis, neuronal migration, and other neural cell structures and activities (Ma et al., 2020). As elastin ages, it degrades into elastin-derived peptides (EDPs). In vivo and in vitro experiments confirm that elastin-like peptides (ELPs) significantly increase Aβ levels in the hippocampus of AD model mice and AD model cells (Ma et al., 2019), suggesting that an increase in EDPs is associated with the pathological progression of AD. Furthermore, the degradation of elastin in the leptomeningeal arterioles of AD patients increases progressively from Braak stages II to VI, while degradation is less pronounced in medium-sized arteries (Merlini et al., 2016). It is hypothesized that the upregulation of ELN may help maintain the ECM and the structure of small blood vessels in the central nervous system.
Among the downregulated hub genes involved in cellular energy metabolism, phosphoglycerate kinase (PGK) is a central nervous system glycolytic enzyme. Its isoform, PGK1, acts as a rate-limiting enzyme in the second phase of glycolysis (Danshina et al., 2010), regulating energy production and redox balance. Pyruvate dehydrogenase E1 component subunit alpha (PDHA1) is a key component of the pyruvate dehydrogenase complex (PDC)(Patel et al., 2014; Patel et al., 2014), linking glycolysis to the tricarboxylic acid cycle in mitochondria. The downregulation of PGK1 and PDHA1 aligns with the metabolic dysfunction observed in AD pathology. Current studies indicate that significantly reduced glucose metabolism in brain regions such as the hippocampus and temporal cortex is a precursor symptom of AD (Mosconi et al., 2008), involving impaired glycolytic function (An et al., 2018). Furthermore, decreased pyruvate dehydrogenase complex activity has been found in the prefrontal cortex of AD patients, which is negatively correlated with the clinical dementia rating (CDR) (Bubber et al., 2005). The role of PGK1 in AD pathology requires further investigation. Some studies suggest that activation of PGK1 promotes autophagic degradation of various pathological aggregates (Chen et al., 2023), PGK1 can bind to Aβ in vitro and co-deposit in plaques (Rahman et al., 2015; Drummond et al., 2017).
In terms of mitochondrial energy metabolism, the downregulated hub genes ATP5F1B and ATP5F1C encode subunits of ATP synthase (Jonckheere et al., 2012), which are crucial components of mitochondrial ATP synthesis. The downregulation of ATP5F1B and ATP5F1C is associated with mitochondrial dysfunction in AD patients. Numerous studies have shown extensive mitochondrial abnormalities in the AD brain (Swerdlow, 2018), including reduced expression of ATP synthase in the hippocampal tissue of AD patients (Schägger and Ohm, 1995). Another study found a 61% reduction in the expression of mitochondrial electron transport chain subunit genes in the hippocampal CA1 region compared to controls (Liang et al., 2008). Regarding mitochondrial structure, the voltage-dependent anion channel (VDAC) is a pore-forming protein on the outer mitochondrial membrane and a key participant in mitochondria-mediated apoptosis (Shoshan-Barmatz et al., 2010). VDAC is also a primary regulator of metabolite exchange between the cytosol and mitochondria, with its isoforms including VDAC1 and VDAC2 (Zinghirino et al., 2021). In transgenic mice, interactions between VDAC1 and Aβ and phosphorylated Tau were observed in the cortex and hippocampus (Manczak and Reddy, 2012), which may contribute to mitochondrial dysfunction in AD pathogenesis. VDAC also co-localizes with full-length APP and Aβ in the frontal cortex of AD patients, and VDAC1 levels progressively increase in the cortex (Manczak and Reddy, 2012). While many studies suggest that reduced expression of VDAC1 may benefit synaptic activity and improve AD pathology (Manczak et al., 2013), it has been found that VDAC1 expression varies across different brain regions in AD patients (Yoo et al., 2001). The mechanisms by which VDAC affects AD and its potential as a drug target require further investigation.
14-3-3 proteins are a family of highly conserved proteins abundantly expressed in the brain, accounting for approximately 1% of total soluble brain proteins (Pair and Yacoubian, 2021). YWHAZ encodes the zeta (ζ) isoform of the 14-3-3 protein family (with delta (δ) as its phosphorylated form) (Aitken et al., 1995), which has a complex relationship with AD pathology. In vitro studies have found that 14-3-3ζ may negatively regulate Aβ-mediated toxicity (Nelson and Alkon, 2007), and increased levels of insoluble 14-3-3ζ have been detected in NFTs in the hippocampal tissue of AD patients (Umahara et al., 2004). Additionally, 14-3-3ζ maintains cytoskeletal dynamics by inhibiting the binding of phosphorylated cofilin to filamentous actin in AD pathology (Kim et al., 2009; Mizuno, 2013). The expression of 14-3-3ζ in various brain regions of AD patients remains unclear. Some studies have found increased levels of 14-3-3ζ in the cerebrospinal fluid of AD patients, which correlate with elevated phosphorylated Tau 181 (P-Tau) levels (Qiang et al., 2024). Moreover, significant upregulation of 14-3-3ζ has been observed in the frontal and temporal cortices of AD patients (Qureshi et al., 2013), while other studies have reported that the average levels of all 14-3-3 isoforms in the prefrontal cortex of AD patients are lower than those in controls, with no significant difference in the expression level of 14-3-3ζ (Gu et al., 2020). We found that the YWHAZ gene is downregulated in the hippocampal tissue of AD patients, but further research is needed to determine changes in 14-3-3ζ protein levels.
Ubiquitin (Ub) is involved in various cellular pathways, including signal transduction and proteasomal degradation (Hershko and Ciechanover, 1998; Park and Ryu, 2014). UBB is one of the genes encoding polyubiquitin proteins (Wiborg et al., 1985) and contributes significantly to the total ubiquitin protein pool in brain tissue (Ryu et al., 2007). In vitro experiments have shown that disruption of the UBB gene leads to reduced self-renewal capacity of neural stem cells, affecting their differentiation into neurons (Park et al., 2020). Animal studies have found that UBB is highly expressed in the mouse olfactory bulb, hippocampus, and hypothalamus (Park et al., 2012). The ubiquitin-proteasome system (UPS) is the primary mechanism for protein quality control within cells (Pohl and Dikic, 2019). The frameshift form of the polyubiquitin protein encoded by the UBB gene, UBB+1, inhibits the ubiquitin-proteasome pathway (Lindsten et al., 2002). UBB+1 is present in the brain tissues of all AD patients (Ebrahimi-Fakhari et al., 2012) and co-localizes with pathological changes in Tau protein, involving the early induction of Aβ deposition and phosphorylated Tau protein aggregation in AD (Hol et al., 2005). UBB+1 also participates in the regulation of gene expression. Transcriptome analysis indicates that low expression of UBB+1 increases the expression of genes involved in ubiquitin-related processes and autophagy pathways (Chen et al., 2021). Therefore, our finding of UBB downregulation in the hippocampus of AD patients may be related to the accumulation of UBB+1 in hippocampal tissue.
α-syn is expressed in presynaptic terminals of neurons (Iwai et al., 1995) and is closely associated with various presynaptic processes (George et al., 1995). Strong mRNA expression of α-syn has been observed in the hippocampus of rats (Maroteaux and Scheller, 1991). α-syn is the main protein component of Lewy bodies, which are hallmarks of Parkinson’s disease (Spillantini et al., 1997). Accumulation of α-syn and Lewy body-related pathology has been found in the brains of more than half of AD patients (Lippa et al., 1998; Hamilton, 2000; Arai et al., 2001). Even in the absence of Lewy body-related pathology, significant increases in intracellular soluble α-syn monomers and oligomers have been observed in the inferior temporal cortex of AD patients (Larson et al., 2012), and elevated α-syn levels have also been detected in the cerebrospinal fluid of AD patients (Twohig and Nielsen, 2019b). Research shows that α-syn induces intracellular Tau aggregation (Spencer et al., 2016) and contributes to Aβ pathology (Masliah et al., 2001), consistent with abundant α-syn in the core of Aβ plaques (Masliah et al., 1996) and the co-localization of α-syn-positive inclusions with neurofibrillary tangles (Arai et al., 2001). Although studies commonly report α-syn accumulation in the central nervous system of AD patients, animal experiments have shown that the loss of endogenous α-syn exacerbates neurodegeneration (Qi et al., 2017). Our study found that SNCA gene expression is downregulated in the hippocampal tissue of AD patients, which may be associated with late-stage AD pathology.
HSPA8 encodes Heat Shock Cognate Protein 70 (HSC70), a molecular chaperone of the heat shock protein (HSP) family (Stricher et al., 2013).HSC70 recognizes substrate proteins in chaperone-mediated autophagy (CMA) (Liao et al., 2021), and the carboxyl-terminus of Hsc70 interacting protein (CHIP) connects to the UPS to target various proteins for degradation (Meimaridou et al., 2009).HSC70 has a complex relationship with AD pathology. Experiments have shown that Tau protein fragments interact with HSC70 and are degraded via the CMA pathway (Wang et al., 2009). Enhancing the binding of HSC70 to Tau protein can significantly reduce Tau levels in cells (Young et al., 2016).In addition to promoting Tau protein degradation, HSC70 is also associated with the neuroprotective effects of soluble APPα (sAPPα) fragments generated by APP cleavage. Increased HSC70 mRNA expression was observed in SH-SY5Y cells treated with sAPPα (Masi et al., 2023).On the other hand, experiments have found that inhibiting HSC70 activity can reduce amyloid deposition and PHF-Tau formation in the CA1 region of AD model mice (Yang and Tohda, 2018). The carboxyl-terminus of Hsc70 interacting protein (CHIP) enhances the ubiquitination and clearance of Neurofilament Medium Chain (NF-M) which may relate to neurodegeneration in AD (Wang et al., 2011). Currently, a significant increase in HSC70 levels has been found in the cytoplasmic and membrane regions of the inferior temporal gyrus in AD patients, accompanied by a decrease in heat shock gactor 1 (HSF-1), suggesting that the elevated HSC70 levels may not be due to increased transcription (Piedrahita et al., 2016). Other studies have found significantly reduced HSPA8 mRNA expression in the hippocampal tissue of AD patients (Silva et al., 2014), which is consistent with the differential expression analysis results of this study.
After analyzing the included studies, we found that various TCM improved behavioral disorders and alleviated hippocampal lesions at different stages of the AD model mice. Curcumin showed efficacy in both early and late stages of AD pathology in APP/PS1 mice, which is due to its effects on Aβ, Tau pathology, and neuroinflammation inhibition. In our target gene analysis of the active components of traditional Chinese medicines, including dihydroartemisinin, berberine, naringin, calycosin, verbascoside, echinacoside, and icariside II, we found that echinacoside involves the ERK and ROS/ATF3/CHOP pathways for its neuroprotective effects. However, the specific targets of echinacoside for AD pathology require further investigation. Other TCM, such as E. brevicornu Maxim., Coptis chinensis Franch., and Astragalus propinquus Schischkin, are important drugs in the current clinical treatment of AD in Chinese medicine, but the majority of their target genes are derived from studies on non-neurological diseases.
Based on our analysis of studies and experiments related to the active components of TCM, we found that berberine (Wu, 2021), icariside II (Yan, 2017), and echinacoside (Dai et al., 2020) can inhibit the phosphorylation of the PERK/eIF2α pathway. The endoplasmic reticulum (ER) maintains cellular protein homeostasis, and the accumulation of unfolded proteins within the cell causes ER stress, which activates the unfolded protein response (UPR) (Uddin et al., 2020). However, ER stress also creates a positive feedback loop with neuroinflammation, leading to neurodegenerative diseases, including AD (Santos and Ferreira, 2018). During ER stress, PERK activation leads to the phosphorylation of eIF2α (Sudhakar et al., 2000), which reduces protein accumulation in the ER by inhibiting mRNA translation (Ohno, 2014). RNA-binding proteins (RBPs) are involved in the formation of stress granules (SGs) (Jackson et al., 2010). Current research has shown a significant increase in phosphorylated PERK and eIF2α in the hippocampal tissues of AD patients (Stutzbach et al., 2013). Long-term overactivation of the PERK/eIF2α pathway leads to reduced protein synthesis, pathological Tau protein phosphorylation, and Aβ production (Moradi Majd et al., 2020). Additionally, the interaction between Tau and SGs results in the formation of insoluble Tau protein aggregates (Vanderweyde et al., 2016). The RNA binding protein (RBP) cascade hypothesis of neurodegeneration proposes that SGs persist for a long time during the pathological process of AD and other diseases (Wolozin and Ivanov, 2019), gradually making proteins like Tau highly stable in their conformations, ultimately leading to neurodegeneration. Among the active components of TCM, berberine (Wu, 2021), icariside II (Yan, 2017), and echinacoside (Dai et al., 2020) were found to reduce the levels of phosphorylated PERK and phosphorylated eIF2α in the hippocampal tissues of APP/PS1 mice. Furthermore, the MicroScale Thermophoresis (MST) Assay demonstrated that echinacoside has a high affinity for human PERK (Dai et al., 2020), suggesting echinacoside’s potential role in regulating protein homeostasis in AD pathology.
This study specifically focused on single-component TCM treatments to assess their effects on hippocampal pathology in AD. Consequently, the reviewed literature may not fully encompass all TCM treatments and formulation patterns used clinically for AD. Additionally, due to the considerable variation in the number of target genes for each active component, we prioritized those with significant overlap with the DEGs for analysis. This approach may have excluded some TCM components with potential therapeutic effects on AD, necessitating further investigation.
To address these limitations, future research on TCM treatments for AD should prioritize identifying and validating the target genes of active components commonly used in clinical treatments through in vivo and in vitro experiments. Furthermore, animal studies should independently assess the effects of TCM on pathological changes in different brain regions of AD model animals.
In summary, this study conducted a bioinformatic analysis on the screened microarray and high-throughput data, identifying upregulated and downregulated hub genes. Among them, we found that the genes encoding α-syn (SNCA), polyubiquitin (UBB), and mitochondrial outer membrane proteins VDAC (VDAC1, VDAC2) are downregulated hub genes in the hippocampal tissue of AD patients. Based on enriched pathways and hub genes, we found that synaptic function in the hippocampal tissue of AD patients is impaired, associated with structural and functional damage to neuronal mitochondria and disruptions in cellular energy metabolism, consistent with previous findings in several studies on central AD pathology (Swerdlow, 2018; Wang et al., 2014). In the included studies, geniposide (Zhang, 2019), total alkaloids from Dendrobium nobile (Yang, 2017), and tetrahydroxy-stilbene-glucoside (Chen C., 2017) were found to be related to the enriched pathways of DEGs in this study, specifically affecting mitochondrial autophagy and synaptic function in the hippocampal tissue of AD model mice.
Based on target gene analysis, we believe that the active component echinacoside from traditional Chinese medicine has therapeutic potential for AD pathology. Cistanche is a widely used TCM for treating AD. Echinacoside, an active component of Cistanche, has been identified through target gene analysis to interact with specific targets (NTRK1, MAPK1, SNCA, DDIT3) in Parkinson’s disease model cells, which are also DEGs in our study. Furthermore, current research indicates that echinacoside inhibits phosphorylation of the PERK/eIF2α pathway, crucial for maintaining intracellular protein homeostasis. Therefore, we propose that echinacoside holds significant therapeutic potential for addressing AD pathology. However, the therapeutic effects of the aforementioned TCMs on AD require further investigation in future clinical and molecular biology studies.
The original contributions presented in the study are included in the article/Supplementary Material, further inquiries can be directed to the corresponding author.
The manuscript presents research on animals that do not require ethical approval for their study.
ZC: Conceptualization, Formal Analysis, Software, Validation, Writing–original draft. ZM: Data curation, Investigation, Writing–original draft. DY: Data curation, Investigation, Writing–original draft. HC: Data curation, Investigation, Writing–original draft. HX: Data curation, Investigation, Writing–original draft. LY: Data curation, Visualization, Writing–original draft. WH: Data curation, Investigation, Writing–original draft. SL: Project administration, Supervision, Writing–review and editing.
The author(s) declare that financial support was received for the research, authorship, and/or publication of this article. This work supported by the National Natural Science Foundation of China (NSFC) General Program, Project No. 81873168.
The authors declare that the research was conducted in the absence of any commercial or financial relationships that could be construed as a potential conflict of interest.
All claims expressed in this article are solely those of the authors and do not necessarily represent those of their affiliated organizations, or those of the publisher, the editors and the reviewers. Any product that may be evaluated in this article, or claim that may be made by its manufacturer, is not guaranteed or endorsed by the publisher.
The Supplementary Material for this article can be found online at: https://www.frontiersin.org/articles/10.3389/fphar.2024.1424803/full#supplementary-material
Afsar, A., Chacon Castro, M. D. C., Soladogun, A. S., and Zhang, L. (2023). Recent development in the understanding of molecular and cellular mechanisms underlying the etiopathogenesis of Alzheimer's disease. Int. J. Mol. Sci. 24 (8), 7258. doi:10.3390/ijms24087258
Ahuja-Casarín, A. I., Merino-Montiel, P., Vega-Baez, J. L., Montiel-Smith, S., Fernandes, M. X., Lagunes, I., et al. (2021). Tuning the activity of iminosugars: novel N-alkylated deoxynojirimycin derivatives as strong BuChE inhibitors. J. Enzyme Inhib. Med. Chem. 36 (1), 138–146. doi:10.1080/14756366.2020.1847101
Aitken, A., Howell, S., Jones, D., Madrazo, J., and Patel, Y. (1995). 14-3-3 alpha and delta are the phosphorylated forms of raf-activating 14-3-3 beta and zeta. in vivo stoichiometric phosphorylation in brain at a Ser-Pro-Glu-Lys MOTIF. J. Biol. Chem. 270 (11), 5706–5709. doi:10.1074/jbc.270.11.5706
An, Y., Varma, V. R., Varma, S., Casanova, R., Dammer, E., Pletnikova, O., et al. (2018). Evidence for brain glucose dysregulation in Alzheimer's disease. Alzheimers Dement. 14 (3), 318–329. doi:10.1016/j.jalz.2017.09.011
Arai, Y., Yamazaki, M., Mori, O., Muramatsu, H., Asano, G., and Katayama, Y. (2001). Alpha-synuclein-positive structures in cases with sporadic Alzheimer's disease: morphology and its relationship to tau aggregation. Brain Res. 888 (2), 287–296. doi:10.1016/s0006-8993(00)03082-1
Bader, G. D., and Hogue, C. W. (2003). An automated method for finding molecular complexes in large protein interaction networks. BMC Bioinforma. 4, 2. doi:10.1186/1471-2105-4-2
Ballard, I. C., Wagner, A. D., and McClure, S. M. (2019). Hippocampal pattern separation supports reinforcement learning. Nat. Commun. 10 (1), 1073. doi:10.1038/s41467-019-08998-1
Bateman, R. J., Xiong, C., Benzinger, T. L., Fagan, A. M., Goate, A., Fox, N. C., et al. (2012). Clinical and biomarker changes in dominantly inherited Alzheimer's disease. N. Engl. J. Med. 367 (9), 795–804. doi:10.1056/NEJMoa1202753
Benjamini, Y., and Yosef, H. (1995). Controlling the False discovery rate: a practical and powerful approach to multiple testing. J. R. Stat. Soc. Ser. B Methodol. 57 (1), 289–300. doi:10.1111/j.2517-6161.1995.tb02031.x
Bhatt, S., Puli, L., and Patil, C. R. (2021). Role of reactive oxygen species in the progression of Alzheimer's disease. Drug Discov. Today 26 (3), 794–803. doi:10.1016/j.drudis.2020.12.004
Bigalke, B., Schreitmüller, B., Sopova, K., Paul, A., Stransky, E., Gawaz, M., et al. (2011). Adipocytokines and CD34 progenitor cells in Alzheimer's disease. PLoS One 6 (5), e20286. doi:10.1371/journal.pone.0020286
Birecree, E., Whetsell, W. O., Stoscheck, C., King, L. E., and Nanney, L. B. (1988). Immunoreactive epidermal growth factor receptors in neuritic plaques from patients with Alzheimer's disease. J. Neuropathol. Exp. Neurol. 47 (5), 549–560. doi:10.1097/00005072-198809000-00006
Bolger, A. M., Lohse, M., and Usadel, B. (2014). Trimmomatic: a flexible trimmer for Illumina sequence data. Bioinformatics 30 (15), 2114–2120. doi:10.1093/bioinformatics/btu170
Bubber, P., Haroutunian, V., Fisch, G., Blass, J. P., and Gibson, G. E. (2005). Mitochondrial abnormalities in Alzheimer brain: mechanistic implications. Ann. Neurol. 57 (5), 695–703. doi:10.1002/ana.20474
Calvo-Flores Guzmán, B., Elizabeth Chaffey, T., Hansika Palpagama, T., Waters, S., Boix, J., Tate, W. P., et al. (2020). The interplay between beta-amyloid 1-42 (Aβ1-42)-Induced hippocampal inflammatory response, p-tau, vascular pathology, and their synergistic contributions to neuronal death and behavioral deficits. Front. Mol. Neurosci. 13, 522073. doi:10.3389/fnmol.2020.552073
Carlsen, R. M., and Hoffman, C. (2021). “GSE173955: analysis of human blood transcriptomes in response to mRNA COVID-19 vaccine,” in Gene expression Omnibus (National Center for Biotechnology Information). Available at: https://www.ncbi.nlm.nih.gov/geo/query/acc.cgi?acc=GSE173955.
Chandra, S., Gallardo, G., Fernández-Chacón, R., Schlüter, O. M., and Südhof, T. C. (2005). Alpha-synuclein cooperates with CSPalpha in preventing neurodegeneration. Cell. 123 (3), 383–396. doi:10.1016/j.cell.2005.09.028
Chen, C. (2017a). Study on the effects of stilbene glycosides on APP/PS1 double transgenic mice and its pharmacological mechanism. Master’s thesis. Beijing, China: Capital Medical University Available at: https://kns.cnki.net/kcms2/article/abstract?v=8WLnD7pOpNFbrTUp2VQKpahPFGeYUeOSFjYwMd315CMk_C9ZXfKEn2VT_2twzZxsYloGz5dxUEqtC82Qku1LcYsytOKKXdIDnvYck4v81Zv60zZLATF1NIn6Qej2JJdCQZD_MZkFxJwX0-_rUkS1qQ==&uniplatform=NZKPT&language=CHS.
Chen, H., Li, Y., Gao, J., Cheng, Q., Liu, L., and Cai, R. (2023). Activation of Pgk1 results in reduced protein aggregation in diverse neurodegenerative conditions. Mol. Neurobiol. 60 (9), 5090–5101. doi:10.1007/s12035-023-03389-6
Chen, M., Du, Z. Y., Zheng, X., Li, D. L., Zhou, R. P., and Zhang, K. (2018). Use of curcumin in diagnosis, prevention, and treatment of Alzheimer's disease. Neural Regen. Res. 13 (4), 742–752. doi:10.4103/1673-5374.230303
Chen, S., Liu, H., Wang, S., Jiang, H., Gao, L., Wang, L., et al. (2022). The neuroprotection of verbascoside in Alzheimer's disease mediated through mitigation of neuroinflammation via blocking NF-κB-p65 signaling. Nutrients 14 (7), 1417. doi:10.3390/nu14071417
Chen, W. K. (2019). Study on the neuroprotective effect and mechanism of 1-deoxy-myo-inositol in Alzheimer's disease. Nanjing, China: Nanjing University of Chinese Medicine. Available at: https://kns.cnki.net/kcms2/article/abstract?v=8WLnD7pOpNGnirnI1ZB2jUdNUO0gKXxXe1Aaq7W54e8rVYDcoDuKicLvNXV65c8MRggMljg8mbcsZKn8eu2ACCUcOC-tER27jG5KAgirmUzc83o7EI7lMHiJUKfsTwWbbqkpN0f1Y71hBDBGz74lug==&uniplatform=NZKPT&language=CHS.
Chen, X., Muñoz-Arellano, A. J., and Petranovic, D. (2021). UBB+1 reduces amyloid-β cytotoxicity by activation of autophagy in yeast. Aging (Albany NY) 13 (21), 23953–23980. doi:10.18632/aging.203681
Chen, Y., Chen, Y., Liang, Y., Chen, H., Ji, X., and Huang, M. (2020a). Berberine mitigates cognitive decline in an Alzheimer's Disease Mouse Model by targeting both tau hyperphosphorylation and autophagic clearance. Biomed. Pharmacother. 121, 109670. doi:10.1016/j.biopha.2019.109670
Chen, Y. W. (2017b). The effect of curcumin on behavior and inflammation mechanism in APP/PS1 double transgenic mice, Master’s thesis. Beijing, China: Beijing University of Chinese Medicine). Available at: https://kns.cnki.net/kcms2/article/abstract?v=8WLnD7pOpNHsCH3NFRwsQmoD1VQqgeYsZ6qaKmorr0NJN7OUqYJSOSSQ3qeTcYA7oMXfycvCkMj7ObH1NgDS85dd33Z1ffiayfCCtOX1LLKMPXBplBRgBA7PPzmpk5TBmLySIzdvNkZr96wU7Bipag==&uniplatform=NZKPT&language=CHS.
Chen, Y. Y., Wang, M. C., Wang, Y. N., Hu, H. H., Liu, Q. Q., Liu, H. J., et al. (2020b). Redox signaling and Alzheimer's disease: from pathomechanism insights to biomarker discovery and therapy strategy. Biomark. Res. 8, 42. doi:10.1186/s40364-020-00218-z
Chin, C. H., Chen, S. H., Wu, H. H., Ho, C. W., Ko, M. T., and Lin, C. Y. (2014). cytoHubba: identifying hub objects and sub-networks from complex interactome. BMC Syst. Biol. 8 (Suppl. 4), S11. doi:10.1186/1752-0509-8-S4-S11
Chun, J., Kwon, T., Lee, E. J., Kim, C. H., Han, Y. S., Hong, S. K., et al. (2004). 14-3-3 Protein mediates phosphorylation of microtubule-associated protein tau by serum- and glucocorticoid-induced protein kinase 1. Mol. Cells 18 (3), 360–368. doi:10.1016/s1016-8478(23)13125-6
Conway, J. R., Lex, A., and Gehlenborg, N. (2017). UpSetR: an R package for the visualization of intersecting sets and their properties. Bioinformatics 33 (18), 2938–2940. doi:10.1093/bioinformatics/btx364
Dai, Y., Han, G., Xu, S., Yuan, Y., Zhao, C., and Ma, T. (2020). Echinacoside suppresses amyloidogenesis and modulates F-actin remodeling by targeting the ER stress sensor PERK in a mouse model of Alzheimer's disease. Front. Cell. Dev. Biol. 8, 593659. doi:10.3389/fcell.2020.593659
Dang, H. Z. (2015). The effect of curcumin on the insulin signaling pathway in APP/PS1 double transgenic mice. (Doctoral thesis). Beijing, China: Beijing University of Chinese Medicine. Available at: https://kns.cnki.net/kcms2/article/abstract?v=8WLnD7pOpNHmkM16CMZa-bUn2_N-7slPefZGdktOLUtiV4UQdiSvORprPnHCcldoHhSG-qiHHWnxPdU2oKiqeCYa0KI1JsXIm9O-4lM7C52rxtcC7sstH-3Ny1F2xTyExovzlRrxy4zDnBJZX_WbZQ==&uniplatform=NZKPT&language=CHS.
Danshina, P. V., Geyer, C. B., Dai, Q., Goulding, E. H., Willis, W. D., Kitto, G. B., et al. (2010). Phosphoglycerate kinase 2 (PGK2) is essential for sperm function and male fertility in mice. Biol. Reprod. 82 (1), 136–145. doi:10.1095/biolreprod.109.079699
Deng, Q., Qiao, X., Li, Z., Peng, R., Zhang, K., Luo, L., et al. (2022). Research progress of gusuibu (Drynariae rhizoma) and its active ingredients in the treatment of skeletal system diseases. J. Liaoning Univ. Traditional Chin. Med. 24 (07), 1–5. doi:10.13194/j.issn.1673-842x.2022.07.001
Deng, Y., Xiong, Z., Chen, P., Wei, J., Chen, S. S., and Yan, Z. (2014). β-amyloid impairs the regulation of N-methyl-D-aspartate receptors by glycogen synthase kinase 3. Neurobiol. Aging 35, 449–459. doi:10.1016/j.neurobiolaging.2013.08.031
Ding, M. R., Qu, Y. J., Hu, B., and An, H. M. (2022). Signal pathways in the treatment of Alzheimer's disease with traditional Chinese medicine. Biomed. Pharmacother. 152, 113208. doi:10.1016/j.biopha.2022.113208
Dong, Y. (2017). Mechanism of neuroprotective effect of Jin Siwei based on cholinergic nerves and oxidative stress injury. Beijing, China: Beijing University of Chinese Medicine. Available at: https://kns.cnki.net/kcms2/article/abstract?v=qwZretP9BaGbwbx7aIfMNNDseiqxjajpsnT2FhSWZUV0GhH_fTs5dKHT_pce_vbarTRcWSbTPrnzQ4vH7Qvc5doRVIWWXvE3tVH3kCj3_6eaT2VDJL9HuiioRmTWtbTLdHW7NydaVJuZ_eKXf9LqQAThUmyHWSQv&uniplatform=NZKPT&language=CHS.
Drummond, E., Nayak, S., Faustin, A., Pires, G., Hickman, R. A., Askenazi, M., et al. (2017). Proteomic differences in amyloid plaques in rapidly progressive and sporadic Alzheimer's disease. Acta Neuropathol. 133 (6), 933–954. doi:10.1007/s00401-017-1691-0
Ebrahimi-Fakhari, D., Wahlster, L., and McLean, P. J. (2012). Protein degradation pathways in Parkinson's disease: curse or blessing. Acta Neuropathol. 124 (2), 153–172. doi:10.1007/s00401-012-1004-6
Fagiani, F., Catanzaro, M., Buoso, E., Basagni, F., Di Marino, D., Raniolo, S., et al. (2020). Targeting cytokine release through the differential modulation of Nrf2 and NF-κB pathways by electrophilic/non-electrophilic compounds. Front. Pharmacol. 11, 1256. doi:10.3389/fphar.2020.01256
Fan, H. (2015). The effect of curcumin on Aβ and ADDLs in APPswe/PS1dE9 double transgenic mice. Master’s thesis. Beijing, China: Beijing University of Chinese Medicine. Available at: https://kns.cnki.net/kcms2/article/abstract?v=8WLnD7pOpNFN3ZjQ8_33-SwigUMBnZz4OuB27jdMNW6ErtSS8pMHg-U-EHNq4bpxGcELA3-KzuG08QWM05n-o30hpiP5ghb3941K_7jziPSp8yT3CPdZgmz9LvZ2o08AgKhZrBGrz1N5EcPhVj342A==&uniplatform=NZKPT&language=CHS.
Fellgiebel, A., and Yakushev, I. (2011). Diffusion tensor imaging of the hippocampus in MCI and early Alzheimer's disease. J. Alzheimers Dis. 26 (Suppl. 3), 257–262. doi:10.3233/JAD-2011-0001
Ferrer, I., Blanco, R., Carmona, M., and Puig, B. (2001a). Phosphorylated c-MYC expression in Alzheimer disease, Pick's disease, progressive supranuclear palsy and corticobasal degeneration. Neuropathol. Appl. Neurobiol. 27 (5), 343–351. doi:10.1046/j.1365-2990.2001.00348.x
Ferrer, I., Blanco, R., Carmona, M., Ribera, R., Goutan, E., Puig, B., et al. (2001b). Phosphorylated map kinase (ERK1, ERK2) expression is associated with early tau deposition in neurones and glial cells, but not with increased nuclear DNA vulnerability and cell death, in Alzheimer disease, Pick's disease, progressive supranuclear palsy and corticobasal degeneration. Brain Pathol. 11 (2), 144–158. doi:10.1111/j.1750-3639.2001.tb00387.x
Fuster-Matanzo, A., Llorens-Martín, M., Jurado-Arjona, J., Avila, J., and Hernández, F. (2012). Tau protein and adult hippocampal neurogenesis. Front. Neurosci. 6, 104. doi:10.3389/fnins.2012.00104
Gao, J. (2017). The mechanism of puerarin in alleviating cognitive impairment in APP/PS1 mice through the PKC/Nrf2 pathway. Doctoral thesis. Changchun, China: Jilin University. Available at: https://kns.cnki.net/kcms2/article/abstract?v=8WLnD7pOpNGJDJ-jzmHUrUy-sSjP9bAV4l89i2f9oOUMqT7NkanywLgyoNzxjNosGc2ljhA9TCiSfgDCvP6z4LqFLCtVkwpT56-McHtCUtW6WFCcDS0EY-nCtAOf3-e0weKxMBZPlMx62eFjv96kLA==&uniplatform=NZKPT&language=CHS.
Gao, Y., Cui, M., Zhong, S., Feng, C., Nwobodo, A. K., Chen, B., et al. (2020). Dihydroartemisinin ameliorates LPS-induced neuroinflammation by inhibiting the PI3K/AKT pathway. Metab. Brain Dis. 35 (4), 661–672. doi:10.1007/s11011-020-00533-2
Gautier, L., Cope, L., Bolstad, B. M., and Irizarry, R. A. (2004). affy--analysis of Affymetrix GeneChip data at the probe level. Bioinformatics 20 (3), 307–315. doi:10.1093/bioinformatics/btg405
Geng, Y. D., Yang, L., Zhang, C., and Kong, L. Y. (2014). Blockade of epidermal growth factor receptor/mammalian target of rapamycin pathway by Icariside II results in reduced cell proliferation of osteosarcoma cells. Food Chem. Toxicol. 73, 7–16. doi:10.1016/j.fct.2014.08.002
George, J. M., Jin, H., Woods, W. S., and Clayton, D. F. (1995). Characterization of a novel protein regulated during the critical period for song learning in the zebra finch. Neuron 15 (2), 361–372. doi:10.1016/0896-6273(95)90040-3
Gu, C., Yang, X., and Huang, L. (2016). Cistanches herba: a neuropharmacology review. Front. Pharmacol. 7, 289. doi:10.3389/fphar.2016.00289
Gu, Q., Cuevas, E., Raymick, J., Kanungo, J., and Sarkar, S. (2020). Downregulation of 14-3-3 proteins in Alzheimer's disease. Mol. Neurobiol. 57 (1), 32–40. doi:10.1007/s12035-019-01754-y
Gu, T. (2018). Study on the protective effect and mechanism of Bone Repair Extract on APP/PS1 double transgenic AD model mice and Aβ-damaged PC12 cells. Master’s thesis. Harbin, China: Heilongjiang University of Chinese Medicine. doi:10.27127/d.cnki.ghlzu.2018.000105
Hajjar, I., Goldstein, F. C., Waller, E. K., Moss, L. D., and Quyyumi, A. (2016). Circulating progenitor cells is linked to cognitive decline in healthy adults. Am. J. Med. Sci. 351 (2), 147–152. doi:10.1016/j.amjms.2015.11.009
Hamilton, R. L. (2000). Lewy bodies in Alzheimer's disease: a neuropathological review of 145 cases using alpha-synuclein immunohistochemistry. Brain Pathol. 10 (3), 378–384. doi:10.1111/j.1750-3639.2000.tb00269.x
He, J. (2023). Study on the molecular mechanism of dihydroartemisinin improving cognitive impairment in Alzheimer's disease based on the “gut microbiota-gut-brain axis”. Master’s thesis. Chongqing, China: Chongqing Medical University. doi:10.27674/d.cnki.gcyku.2023.000092
Heneka, M. T. (2020). An immune-cell signature marks the brain in Alzheimer's disease. Nature 577 (7790), 322–323. doi:10.1038/d41586-019-03892-8
Hershko, A., and Ciechanover, A. (1998). The ubiquitin system. Annu. Rev. Biochem. 67, 425–479. doi:10.1146/annurev.biochem.67.1.425
Hetz, C., and Mollereau, B. (2014). Disturbance of endoplasmic reticulum proteostasis in neurodegenerative diseases. Nat. Rev. Neurosci. 15 (4), 233–249. doi:10.1038/nrn3689
Hock, C., Heese, K., Hulette, C., Rosenberg, C., and Otten, U. (2000). Region-specific neurotrophin imbalances in Alzheimer disease: decreased levels of brain-derived neurotrophic factor and increased levels of nerve growth factor in hippocampus and cortical areas. Arch. Neurol. 57 (6), 846–851. doi:10.1001/archneur.57.6.846
Hol, E. M., van Leeuwen, F. W., and Fischer, D. F. (2005). The proteasome in Alzheimer's disease and Parkinson's disease: lessons from ubiquitin B+1. Trends Mol. Med. 11 (11), 488–495. doi:10.1016/j.molmed.2005.09.001
Hooijmans, C. R., Rovers, M. M., de Vries, R. B., Leenaars, M., Ritskes-Hoitinga, M., and Langendam, M. W. (2014). SYRCLE's risk of bias tool for animal studies. BMC Med. Res. Methodol. 14, 43. doi:10.1186/1471-2288-14-43
Hoozemans, J. J., van Haastert, E. S., Nijholt, D. A., Rozemuller, A. J., Eikelenboom, P., and Scheper, W. (2009). The unfolded protein response is activated in pretangle neurons in Alzheimer's disease hippocampus. Am. J. Pathol. 174 (4), 1241–1251. doi:10.2353/ajpath.2009.080814
Huang, H. C., Tang, D., Xu, K., and Jiang, Z. F. (2014). Curcumin attenuates amyloid-β-induced tau hyperphosphorylation in human neuroblastoma SH-SY5Y cells involving PTEN/Akt/GSK-3β signaling pathway. J. Recept Signal Transduct. Res. 34 (1), 26–37. doi:10.3109/10799893.2013.848891
Huang, M., Jiang, X., Liang, Y., Liu, Q., Chen, S., and Guo, Y. (2017). Berberine improves cognitive impairment by promoting autophagic clearance and inhibiting production of β-amyloid in APP/tau/PS1 mouse model of Alzheimer's disease. Exp. Gerontol. 91, 25–33. doi:10.1016/j.exger.2017.02.004
Huang, Y., Peng, X., and Zhou, X. (2023). Progress in research into the effect of Rhizoma Drynariae on the treatment of bone-related diseases in the oral cavit. Int. J. Stomatology 50 (06), 679–685. doi:10.7518/gjkq.2023107
Irizarry, R. A., Hobbs, B., Collin, F., Beazer-Barclay, Y. D., Antonellis, K. J., Scherf, U., et al. (2003). Exploration, normalization, and summaries of high density oligonucleotide array probe level data. Biostatistics 4 (2), 249–264. doi:10.1093/biostatistics/4.2.249
Ismael, S., Wajidunnisa, S. K., McDonald, M. P., Liao, F. F., and Ishrat, T. (2021). ER stress associated TXNIP-NLRP3 inflammasome activation in hippocampus of human Alzheimer's disease. Neurochem. Int. 148, 105104. doi:10.1016/j.neuint.2021.105104
Iwai, A., Masliah, E., Yoshimoto, M., Ge, N., Flanagan, L., de Silva, H. A., et al. (1995). The precursor protein of non-A beta component of Alzheimer's disease amyloid is a presynaptic protein of the central nervous system. Neuron 14 (2), 467–475. doi:10.1016/0896-6273(95)90302-x
Jack, C. R. Jr, Bennett, D. A., Blennow, K., Carrillo, M. C., Dunn, B., Haeberlein, S. B., et al. (2018). NIA-AA Research Framework: toward a biological definition of Alzheimer's disease. Alzheimers Dement. 14 (4), 535–562. doi:10.1016/j.jalz.2018.02.018
Jackson, R. J., Hellen, C. U., and Pestova, T. V. (2010). The mechanism of eukaryotic translation initiation and principles of its regulation. Nat. Rev. Mol. Cell. Biol. 11 (2), 113–127. doi:10.1038/nrm2838
Jiang, X. W., Liu, W. W., Wu, Y. T., Wu, Q., Lu, H. Y., Xu, Z. H., et al. (2020). Notopterygium incisum extract (NRE) rescues cognitive deficits in APP/PS1 Alzhneimer's disease mice by attenuating amyloid-beta, tau, and neuroinflammation pathology. J. Ethnopharmacol. 249, 112433. doi:10.1016/j.jep.2019.112433
Jonckheere, A. I., Smeitink, J. A., and Rodenburg, R. J. (2012). Mitochondrial ATP synthase: architecture, function and pathology. J. Inherit. Metab. Dis. 35 (2), 211–225. doi:10.1007/s10545-011-9382-9
Kerr, F., Rickle, A., Nayeem, N., Brandner, S., Cowburn, R. F., and Lovestone, S. (2006). PTEN, a negative regulator of PI3 kinase signalling, alters tau phosphorylation in cells by mechanisms independent of GSK-3. FEBS Lett. 580 (13), 3121–3128. doi:10.1016/j.febslet.2006.04.064
Khezri, M. R., Yousefi, K., Esmaeili, A., and Ghasemnejad-Berenji, M. (2023). The role of ERK1/2 pathway in the pathophysiology of Alzheimer's disease: an overview and update on new developments. Cell. Mol. Neurobiol. 43 (1), 177–191. doi:10.1007/s10571-022-01191-x
Kim, D., Langmead, B., and Salzberg, S. L. (2015). HISAT: a fast spliced aligner with low memory requirements. Nat. Methods 12 (4), 357–360. doi:10.1038/nmeth.3317
Kim, J. S., Huang, T. Y., and Bokoch, G. M. (2009). Reactive oxygen species regulate a slingshot-cofilin activation pathway. Mol. Biol. Cell. 20 (11), 2650–2660. doi:10.1091/mbc.e09-02-0131
Kong, F. G. (2020a). The protective effect and mechanism of Forsythoside B on Alzheimer's disease. Master’s thesis. Changchun, China: Jilin University. doi:10.27162/d.cnki.gjlin.2020.002010
Kong, Y. Y. (2020b). Study on the protective effect and mechanism of paeoniflorin in transgenic mice with Alzheimer's disease. Master’s thesis. Guangzhou, China: Guangdong Pharmaceutical University. doi:10.27690/d.cnki.ggdyk.2020.000118
Kostyuk, V. A., Potapovich, A. I., Suhan, T. O., de Luca, C., and Korkina, L. G. (2011). Antioxidant and signal modulation properties of plant polyphenols in controlling vascular inflammation. Eur. J. Pharmacol. 658 (2-3), 248–256. doi:10.1016/j.ejphar.2011.02.022
Larson, M. E., Sherman, M. A., Greimel, S., Kuskowski, M., Schneider, J. A., Bennett, D. A., et al. (2012). Soluble α-synuclein is a novel modulator of Alzheimer's disease pathophysiology. J. Neurosci. 32 (30), 10253–10266. doi:10.1523/JNEUROSCI.0581-12.2012
Lee, K. S., Lee, H. J., Ahn, K. S., Kim, S. H., Nam, D., Kim, D. K., et al. (2009). Cyclooxygenase-2/prostaglandin E2 pathway mediates icariside II induced apoptosis in human PC-3 prostate cancer cells. Cancer Lett. 280 (1), 93–100. doi:10.1016/j.canlet.2009.02.024
Lee, K. W., Kim, H. J., Lee, Y. S., Park, H. J., Choi, J. W., Ha, J., et al. (2007). Acteoside inhibits human promyelocytic HL-60 leukemia cell proliferation via inducing cell cycle arrest at G0/G1 phase and differentiation into monocyte. Carcinogenesis 28 (9), 1928–1936. doi:10.1093/carcin/bgm126
Li, C., and Gao, A. (2017). A review on treating Alzheimer's disease in TCM. Clin. Res. Traditional Chin. Med. 9 (28), 139–141. doi:10.3969/j.issn.1674-7860.2017.28.070
Li, F. (2015). The molecular mechanism of β-asarone against synaptic injury in Alzheimer's disease via the ROCK pathway. Doctoral thesis. Guangzhou, China: Guangzhou University of Chinese Medicine. Available at: https://kns.cnki.net/kcms2/article/abstract?v=8WLnD7pOpNEB8AdalDwzZETrgU-YGM_vrlogtoHi5taLDgMtIevOZlLNz9uLJtL5toW5lnbBSRykMoxDIUhENLc30VlWRUosqEBfpCWa_Xeq8y8fBLm_quW6_WF4XhyFPHG9Me6im4DP15HBAwsUqA==&uniplatform=NZKPT&language=CHS.
Li, L., Wei, H., Zhang, L., Chu, J., and Zhao, L. (2006). Modern biological basis of Chinese medical theory that 'kidney nourishes marrow and brain is sea of marrow. China J. Chin. Materia Medica 31 (17), 1397–1400. https://qikan.cqvip.com/Qikan/Article/Detail?id=22770516
Li, W., Wang, C., Peng, J., Liang, J., Jin, Y., Liu, Q., et al. (2014). Naringin inhibits TNF-α induced oxidative stress and inflammatory response in HUVECs via Nox4/NF-κ B and PI3K/Akt pathways. Curr. Pharm. Biotechnol. 15 (12), 1173–1182. doi:10.2174/1389201015666141111114442
Li, X., Cui, J., Yu, Y., Li, W., Hou, Y., Wang, X., et al. (2016). Traditional Chinese nootropic medicine radix polygalae and its active constituent onjisaponin B reduce β-amyloid production and improve cognitive impairments. PLoS One 11 (3), e0151147. doi:10.1371/journal.pone.0151147
Li, X., Feng, X., Sun, X., Hou, N., Han, F., and Liu, Y. (2022). Global, regional, and national burden of Alzheimer's disease and other dementias, 1990-2019. Front. Aging Neurosci. 14, 937486. doi:10.3389/fnagi.2022.937486
Li, X., Su, Y., Li, N., Zhang, F. R., and Zhang, N. (2021). Berberine attenuates MPP+-Induced neuronal injury by regulating linc00943/miR-142-5p/KPNA4/NF-κB pathway in SK-N-sh cells. Neurochem. Res. 46 (12), 3286–3300. doi:10.1007/s11064-021-03431-w
Liang, W. S., Reiman, E. M., Valla, J., Dunckley, T., Beach, T. G., Grover, A., et al. (2008). Alzheimer's disease is associated with reduced expression of energy metabolism genes in posterior cingulate neurons. Proc. Natl. Acad. Sci. U. S. A. 105 (11), 4441–4446. doi:10.1073/pnas.0709259105
Liao, Z., Wang, B., Liu, W., Xu, Q., Hou, L., Song, J., et al. (2021). Dysfunction of chaperone-mediated autophagy in human diseases. Mol. Cell. Biochem. 476 (3), 1439–1454. doi:10.1007/s11010-020-04006-z
Lindsten, K., de Vrij, F. M., Verhoef, L. G., Fischer, D. F., van Leeuwen, F. W., Hol, E. M., et al. (2002). Mutant ubiquitin found in neurodegenerative disorders is a ubiquitin fusion degradation substrate that blocks proteasomal degradation. J. Cell. Biol. 157 (3), 417–427. doi:10.1083/jcb.200111034
Lippa, C. F., Fujiwara, H., Mann, D. M., Giasson, B., Baba, M., Schmidt, M. L., et al. (1998). Lewy bodies contain altered alpha-synuclein in brains of many familial Alzheimer's disease patients with mutations in presenilin and amyloid precursor protein genes. Am. J. Pathol. 153 (5), 1365–1370. doi:10.1016/s0002-9440(10)65722-7
Liu, F., Liu, J., Xiang, H., Sun, Z., Li, Y., Li, X., et al. (2022). Dihydroartemisinin protects blood-brain barrier permeability during sepsis by inhibiting the transcription factor SNAI1. Clin. Exp. Pharmacol. Physiol. 49 (9), 979–987. doi:10.1111/1440-1681.13683
Liu, J. C. (2018). Study on the protective effect and mechanism of Psoralea Corylifolia extract on APP/PS1 transgenic AD model mice and Aβ-damaged PC12 cells. Master’s thesis. Harbin, China: Heilongjiang University of Chinese Medicine. doi:10.27127/d.cnki.ghlzu.2018.000051
Liu, S., Li, X., Gao, J., Liu, Y., Shi, J., Gong, Q., et al. (2018). Icariside II, a phosphodiesterase-5 inhibitor, attenuates beta-amyloid-induced cognitive deficits via BDNF/TrkB/CREB signaling. Cell. Physiol. Biochem. 49 (3), 985. doi:10.1159/000493232
Liu, S. W. (2019). Study on the mechanism of Valerenic Acid monomer of traditional Chinese medicine in Alzheimer's disease. Doctoral thesis. Changchun, China: Changchun University of Chinese Medicine. doi:10.26980/d.cnki.gcczc.2019.000022
Long, J., Huang, N., and Luo, Y. (2024). Research progress in the treatment of Alzheimer's disease with yinyanghuo based on the theory of shenzhugushengsui. Pharmacol. Clin. Chin. Materia Medica, 1–14. doi:10.13412/j.cnki.zyyl.20240425.002
Love, M. I., Huber, W., and Anders, S. (2014). Moderated estimation of fold change and dispersion for RNA-seq data with DESeq2. Genome Biol. 15 (12), 550. doi:10.1186/s13059-014-0550-8
Lu, J. J., Meng, L. H., Shankavaram, U. T., Zhu, C. H., Tong, L. J., Chen, G., et al. (2010). Dihydroartemisinin accelerates c-MYC oncoprotein degradation and induces apoptosis in c-MYC-overexpressing tumor cells. Biochem. Pharmacol. 80 (1), 22–30. doi:10.1016/j.bcp.2010.02.016
Ma, C., Su, J., Sun, Y., Feng, Y., Shen, N., Li, B., et al. (2019). Significant upregulation of Alzheimer's β-amyloid levels in a living system induced by extracellular elastin polypeptides. Angew. Chem. Int. Ed. Engl. 58 (51), 18703–18709. doi:10.1002/anie.201912399
Ma, F. (2018a). Mechanistic study of the treatment of Alzheimer's disease with Bushen formula (Doctoral dissertation, Beijing University of Chinese Medicine). Available at: https://kns.cnki.net/kcms2/article/abstract?v=qwZretP9BaGd1-nwjE-IsSPOy9d0TVrWpax7s_NTuSHkFatuMzc7yhFiPGu66y9Ka6bwHijdVrzAE27SZuJAj-WujLYZtpqsQoaqEkCll4_CVMI-bUlZ3MuX1yoerahzJAc8MPz1oQWmGlSIVB-lEpZyX2Pjcur-_5CFtk7kcio=&uniplatform=NZKPT&language=CHS.
Ma, F. Y. (2018b). Mechanism study of kidney tonifying prescription in the treatment of Alzheimer's disease. Beijing University of Chinese Medicine. Available at: https://kns.cnki.net/kcms2/article/abstract?v=qwZretP9BaHfswCjAAj6xF1PD_ENDfZZxlSIayzP2Gw0TCOdKduV74NaEg3M6YLN3IEZXYPjBMGfGR-RbkEcszOBrI-wU-LM6kNNArQYA3T1IEpBJXFAUFfagOvZLwRLDVndG3XoyYCrk-M9gzkCM37NcSHDXJIghFhFCYmbedA=.
Ma, J., Ma, C., Li, J., Sun, Y., Ye, F., Liu, K., et al. (2020). Extracellular matrix proteins involved in Alzheimer's disease. Chemistry 26 (53), 12101–12110. doi:10.1002/chem.202000782
Manczak, M., and Reddy, P. H. (2012). Abnormal interaction of VDAC1 with amyloid beta and phosphorylated tau causes mitochondrial dysfunction in Alzheimer's disease. Hum. Mol. Genet. 21 (23), 5131–5146. doi:10.1093/hmg/dds360
Manczak, M., Sheiko, T., Craigen, W. J., and Reddy, P. H. (2013). Reduced VDAC1 protects against Alzheimer's disease, mitochondria, and synaptic deficiencies. J. Alzheimers Dis. 37 (4), 679–690. doi:10.3233/JAD-130761
Maroteaux, L., and Scheller, R. H. (1991). The rat brain synucleins; family of proteins transiently associated with neuronal membrane. Brain Res. Mol. Brain Res. 11 (3-4), 335–343. doi:10.1016/0169-328x(91)90043-w
Masi, M., Biundo, F., Fiou, A., Racchi, M., Pascale, A., and Buoso, E. (2023). The labyrinthine landscape of APP processing: state of the art and possible novel soluble APP-related molecular players in traumatic brain injury and neurodegeneration. Int. J. Mol. Sci. 24 (7), 6639. doi:10.3390/ijms24076639
Masliah, E., Iwai, A., Mallory, M., Uéda, K., and Saitoh, T. (1996). Altered presynaptic protein NACP is associated with plaque formation and neurodegeneration in Alzheimer's disease. Am. J. Pathol. 148 (1), 201–210. https://pubmed.ncbi.nlm.nih.gov/8546207/
Masliah, E., Rockenstein, E., Veinbergs, I., Sagara, Y., Mallory, M., Hashimoto, M., et al. (2001). beta-amyloid peptides enhance alpha-synuclein accumulation and neuronal deficits in a transgenic mouse model linking Alzheimer's disease and Parkinson's disease. Proc. Natl. Acad. Sci. U. S. A. 98 (21), 12245–12250. doi:10.1073/pnas.211412398
McKhann, G. M., Knopman, D. S., Chertkow, H., Hyman, B. T., Jack, C. R., Kawas, C. H., et al. (2011). The diagnosis of dementia due to Alzheimer's disease: recommendations from the National Institute on Aging-Alzheimer's Association workgroups on diagnostic guidelines for Alzheimer's disease. Alzheimers Dement. 7 (3), 263–269. doi:10.1016/j.jalz.2011.03.005
Meimaridou, E., Gooljar, S. B., and Chapple, J. P. (2009). From hatching to dispatching: the multiple cellular roles of the Hsp70 molecular chaperone machinery. J. Mol. Endocrinol. 42 (1), 1–9. doi:10.1677/JME-08-0116
Meng, X., Fu, M., Wang, S., Chen, W., Wang, J., and Zhang, N. (2021). Naringin ameliorates memory deficits and exerts neuroprotective effects in a mouse model of Alzheimer's disease by regulating multiple metabolic pathways. Mol. Med. Rep. 23 (5), 332. doi:10.3892/mmr.2021.11971
Merlini, M., Wanner, D., and Nitsch, R. M. (2016). Tau pathology-dependent remodelling of cerebral arteries precedes Alzheimer's disease-related microvascular cerebral amyloid angiopathy. Acta Neuropathol. 131 (5), 737–752. doi:10.1007/s00401-016-1560-2
Miller, J. A., Oldham, M. C., and Geschwind, D. H. (2008). “GSE5281: gene expression in hippocampal subregions from Alzheimer's disease patients and controls,” in Gene expression Omnibus (National Center for Biotechnology Information). Available at: https://www.ncbi.nlm.nih.gov/geo/query/acc.cgi?acc=GSE5281.
Mizuno, K. (2013). Signaling mechanisms and functional roles of cofilin phosphorylation and dephosphorylation. Cell. Signal 25 (2), 457–469. doi:10.1016/j.cellsig.2012.11.001
Monsell, S. E., Mock, C., Hassenstab, J., Roe, C. M., Cairns, N. J., Morris, J. C., et al. (2014). Neuropsychological changes in asymptomatic persons with Alzheimer disease neuropathology. Neurology 83 (5), 434–440. doi:10.1212/WNL.0000000000000650
Moradi Majd, R., Mayeli, M., and Rahmani, F. (2020). Pathogenesis and promising therapeutics of Alzheimer disease through eIF2α pathway and correspondent kinases. Metab. Brain Dis. 35 (8), 1241–1250. doi:10.1007/s11011-020-00600-8
Mormino, E. C., Kluth, J. T., Madison, C. M., Rabinovici, G. D., Baker, S. L., Miller, B. L., et al. (2009). Episodic memory loss is related to hippocampal-mediated beta-amyloid deposition in elderly subjects. Brain 132 (5), 1310–1323. doi:10.1093/brain/awn320
Mosconi, L., Pupi, A., and De Leon, M. J. (2008). Brain glucose hypometabolism and oxidative stress in preclinical Alzheimer's disease. Ann. N. Y. Acad. Sci. 1147, 180–195. doi:10.1196/annals.1427.007
Mufson, E. J., Conner, J. M., and Kordower, J. H. (1995). Nerve growth factor in Alzheimer's disease: defective retrograde transport to nucleus basalis. Neuroreport 6 (7), 1063–1066. doi:10.1097/00001756-199505090-00028
Mufson, E. J., Counts, S. E., Perez, S. E., and Ginsberg, S. D. (2008). Cholinergic system during the progression of Alzheimer's disease: therapeutic implications. Expert Rev. Neurother. 8 (11), 1703–1718. doi:10.1586/14737175.8.11.1703
National Institute on Aging (n.d.). What happens to the brain in Alzheimer's disease? Available at: https://www.nia.nih.gov/health/what-happens-brain-alzheimers-disease.
Nelson, T. J., and Alkon, D. L. (2007). Protection against beta-amyloid-induced apoptosis by peptides interacting with beta-amyloid. J. Biol. Chem. 282 (43), 31238–31249. doi:10.1074/jbc.M705558200
Nie, H. (2011). Effect of active components in Acorus gramineus on learning and memory and the neuroprotective pathway of clusterin in APP/PS1 double transgenic mice doctoral dissertation. Doctoral dissertation. Guangzhou, China: Guangzhou University of Chinese Medicine. Available at: https://kns.cnki.net/kcms2/article/abstract?v=8WLnD7pOpNHHOArH7fC1jf5dZOSzSgKCVT0tBKFe_DkFdnGmfYb17EcdB6QV2VkDJVJ3hfngmggenw-JUY8LtUIVVFlW359MBPibwFeVXtqx4YcBBRQ7hYorQUsckUyanmlApeLBjzY64s7QrcvoNA==.
Nielsen, J. S., and McNagny, K. M. (2008). Novel functions of the CD34 family. J. Cell. Sci. 121 (Pt 22), 3683–3692. doi:10.1242/jcs.037507
Ohno, M. (2014). Roles of eIF2α kinases in the pathogenesis of Alzheimer's disease. Front. Mol. Neurosci. 7, 22. doi:10.3389/fnmol.2014.00022
Pair, F. S., and Yacoubian, T. A. (2021). 14-3-3 proteins: novel pharmacological targets in neurodegenerative diseases. Trends Pharmacol. Sci. 42 (4), 226–238. doi:10.1016/j.tips.2021.01.001
Park, C. W., Jung, B. K., and Ryu, K. Y. (2020). Disruption of the polyubiquitin gene Ubb reduces the self-renewal capacity of neural stem cells. Biochem. Biophys. Res. Commun. 527 (2), 372–378. doi:10.1016/j.bbrc.2020.04.042
Park, C. W., Ryu, H. W., and Ryu, K. Y. (2012). Locus coeruleus neurons are resistant to dysfunction and degeneration by maintaining free ubiquitin levels although total ubiquitin levels decrease upon disruption of polyubiquitin gene Ubb. Biochem. Biophys. Res. Commun. 418 (3), 541–546. doi:10.1016/j.bbrc.2012.01.063
Park, C. W., and Ryu, K. Y. (2014). Cellular ubiquitin pool dynamics and homeostasis. BMB Rep. 47 (9), 475–482. doi:10.5483/bmbrep.2014.47.9.128
Passeri, E., Elkhoury, K., Morsink, M., Broersen, K., Linder, M., Tamayol, A., et al. (2022). Alzheimer's disease: treatment strategies and their limitations. Int. J. Mol. Sci. 23 (22), 13954. doi:10.3390/ijms232213954
Pastore, S., Lulli, D., Fidanza, P., Potapovich, A. I., Kostyuk, V. A., De Luca, C., et al. (2012). Plant polyphenols regulate chemokine expression and tissue repair in human keratinocytes through interaction with cytoplasmic and nuclear components of epidermal growth factor receptor system. Antioxid. Redox Signal 16 (4), 314–328. doi:10.1089/ars.2011.4053
Patel, M. S., Nemeria, N. S., Furey, W., and Jordan, F. (2014). The pyruvate dehydrogenase complexes: structure-based function and regulation. J. Biol. Chem. 289 (24), 16615–16623. doi:10.1074/jbc.R114.563148
Pei, H., Ma, L., Cao, Y., Wang, F., Li, Z., Liu, N., et al. (2020). Traditional Chinese medicine for Alzheimer's disease and other cognitive impairment: a review. Am. J. Chin. Med. 48 (3), 487–511. doi:10.1142/S0192415X20500251
Piao, Z.-Y., Song, L., Yao, L.-F., Peng, Y., Bai, X., Yu, S., et al. (2019). Effect of schisandrin on learning and memory abilities and their mechanism in APP/PS1 dual transgenic dementia mice. Chin. J. Neuromedicine 18 (4), 325–330. doi:10.3760/cma.j.issn.1671-8925.2019.04.001
Piedrahita, D., Castro-Alvarez, J. F., Boudreau, R. L., Villegas-Lanau, A., Kosik, K. S., Gallego-Gomez, J. C., et al. (2016). β-Secretase 1's targeting reduces hyperphosphorilated tau, implying autophagy actors in 3xTg-AD mice. Front. Cell. Neurosci. 9, 498. doi:10.3389/fncel.2015.00498
Pohl, C., and Dikic, I. (2019). Cellular quality control by the ubiquitin-proteasome system and autophagy. Science 366 (6467), 818–822. doi:10.1126/science.aax3769
Potapovich, A. I., Lulli, D., Fidanza, P., Kostyuk, V. A., De Luca, C., Pastore, S., et al. (2011). Plant polyphenols differentially modulate inflammatory responses of human keratinocytes by interfering with activation of transcription factors NFκB and AhR and EGFR-ERK pathway. Toxicol. Appl. Pharmacol. 255 (2), 138–149. doi:10.1016/j.taap.2011.06.007
Poudineh, M., Ghotbi, T., Azizi, F., Karami, N., Zolfaghari, Z., Gheisari, F., et al. (2022). Neuropharmaceutical properties of naringin against Alzheimer's and Parkinson's diseases: naringin protection against AD and PD. Galen. Med. J. 11, e2337. doi:10.31661/gmj.v11i.2337
Power, R., Prado-Cabrero, A., Mulcahy, R., Howard, A., and Nolan, J. M. (2019). The role of nutrition for the aging population: implications for cognition and Alzheimer's disease. Annu. Rev. Food Sci. Technol. 10, 619–639. doi:10.1146/annurev-food-030216-030125
Prakash, A., Shur, B., and Kumar, A. (2013). Naringin protects memory impairment and mitochondrial oxidative damage against aluminum-induced neurotoxicity in rats. Int. J. Neurosci. 123 (9), 636–645. doi:10.3109/00207454.2013.785542
Qi, Y., Jiang, H., Li, J., Wang, G., Wang, Y., Qin, W., et al. (2017). Yizhi Congming Decoction attenuates learning and memory deficits through reducing tau hyperphosphorylation in Aβ-induced AD mice. Chin. Tradit. Pat. Med. 39 (10), 1999–2003. doi:10.3969/j.issn.1001-1528.2017.10.002
Qiang, Q., Skudder-Hill, L., Toyota, T., Huang, Z., Wei, W., and Adachi, H.Alzheimer's Disease Neuroimaging Initiative (2024). CSF 14-3-3 zeta(ζ) isoform is associated with tau pathology and cognitive decline in Alzheimer's disease. J. Neurol. Sci. 457, 122861. doi:10.1016/j.jns.2023.122861
Qureshi, H. Y., Li, T., MacDonald, R., Cho, C. M., Leclerc, N., and Paudel, H. K. (2013). Interaction of 14-3-3ζ with microtubule-associated protein tau within Alzheimer's disease neurofibrillary tangles. Biochemistry 52 (37), 6445–6455. doi:10.1021/bi400442d
Rahman, M. M., Zetterberg, H., Lendel, C., and Härd, T. (2015). Binding of human proteins to amyloid-β protofibrils. ACS Chem. Biol. 10 (3), 766–774. doi:10.1021/cb5008663
Ranran, W., Zhu, T., Feng, Z., Wang, M., Aoxue, M. I. N., Heng, L. I., et al. (2020). Effects of long-term icariin treatment on neuroinflammation in APP/PS1 mice. J. Shandong Univ. Heal. Sci. 58(4): 71–77. doi:10.6040/j.issn.1671-7554.0.2019.1458
Ravindra, K. C., Ho, W. E., Cheng, C., Godoy, L. C., Wishnok, J. S., Ong, C. N., et al. (2015). Untargeted proteomics and systems-based mechanistic investigation of artesunate in human bronchial epithelial cells. Chem. Res. Toxicol. 28 (10), 1903–1913. doi:10.1021/acs.chemrestox.5b00105
Ritchie, M. E., Phipson, B., Wu, D., Hu, Y., Law, C. W., Shi, W., et al. (2015). Limma powers differential expression analyses for RNA-sequencing and microarray studies. Nucleic Acids Res. 43 (7), e47. doi:10.1093/nar/gkv007
Romaus-Sanjurjo, D., Custodia, A., Ouro, A., and Sobrino, T. (2023). CD34+ progenitor cells as diagnostic and therapeutic targets in Alzheimer's disease. Neural Regen. Res. 18 (3), 535–536. doi:10.4103/1673-5374.346485
Ryu, K. Y., Maehr, R., Gilchrist, C. A., Long, M. A., Bouley, D. M., Mueller, B., et al. (2007). The mouse polyubiquitin gene UbC is essential for fetal liver development, cell-cycle progression and stress tolerance. EMBO J. 26 (11), 2693–2706. doi:10.1038/sj.emboj.7601722
Saeki, K., Machida, M., Kinoshita, Y., Takasawa, R., and Tanuma, S. (2011). Glycogen synthase kinase-3β2 has lower phosphorylation activity to tau than glycogen synthase kinase-3β1. Biol. Pharm. Bull. 34 (1), 146–149. doi:10.1248/bpb.34.146
Salamanova, E., Atanasova, M., Dimitrov, I., and Doytchinova, I. (2021). Effects of curcumin and ferulic acid on the folding of amyloid-β peptide. Molecules 26 (9), 2815. doi:10.3390/molecules26092815
Santos, L. E., and Ferreira, S. T. (2018). Crosstalk between endoplasmic reticulum stress and brain inflammation in Alzheimer's disease. Neuropharmacology 136 (Pt B), 350–360. doi:10.1016/j.neuropharm.2017.11.016
Schägger, H., and Ohm, T. G. (1995). Human diseases with defects in oxidative phosphorylation. 2. F1F0 ATP-synthase defects in Alzheimer disease revealed by blue native polyacrylamide gel electrophoresis. Eur. J. Biochem. 227 (3), 916–921. doi:10.1111/j.1432-1033.1995.tb20219.x
Schindowski, K., Belarbi, K., and Buée, L. (2008). Neurotrophic factors in Alzheimer's disease: role of axonal transport. Genes. Brain Behav. 7 (1), 43–56. doi:10.1111/j.1601-183X.2007.00378.x
Shannon, P., Markiel, A., Ozier, O., Baliga, N. S., Wang, J. T., Ramage, D., et al. (2003). Cytoscape: a software environment for integrated models of biomolecular interaction networks. Genome Res. 13 (11), 2498–2504. doi:10.1101/gr.1239303
Shi, X., Zheng, Z., Li, J., Xiao, Z., Qi, W., Zhang, A., et al. (2015). Curcumin inhibits Aβ-induced microglial inflammatory responses in vitro: involvement of ERK1/2 and p38 signaling pathways. Neurosci. Lett. 594, 105–110. doi:10.1016/j.neulet.2015.03.045
Shi, X. M., Zhang, H., Zhou, Z. J., Ruan, Y. Y., Pang, J., Zhang, L., et al. (2018). Effects of safflower yellow on beta-amyloid deposition and activation of astrocytes in the brain of APP/PS1 transgenic mice. Biomed. Pharmacother. 98, 553–565. doi:10.1016/j.biopha.2017.12.099
Shimada, T., Tanaka, K., Takenaka, S., Murayama, N., Martin, M. V., Foroozesh, M. K., et al. (2010). Structure-function relationships of inhibition of human cytochromes P450 1A1, 1A2, 1B1, 2C9, and 3A4 by 33 flavonoid derivatives. Chem. Res. Toxicol. 23 (12), 1921–1935. doi:10.1021/tx100286d
Shoshan-Barmatz, V., De Pinto, V., Zweckstetter, M., Raviv, Z., Keinan, N., and Arbel, N. (2010). VDAC, a multi-functional mitochondrial protein regulating cell life and death. Mol. Asp. Med. 31 (3), 227–285. doi:10.1016/j.mam.2010.03.002
Silva, P. N., Furuya, T. K., Braga, I. L., Rasmussen, L. T., Labio, R. W., Bertolucci, P. H., et al. (2014). Analysis of HSPA8 and HSPA9 mRNA expression and promoter methylation in the brain and blood of Alzheimer's disease patients. J. Alzheimers Dis. 38 (1), 165–170. doi:10.3233/JAD-130428
Somani, G., Kulkarni, C., Shinde, P., Shelke, R., Laddha, K., and Sathaye, S. (2015). In vitro acetylcholinesterase inhibition by psoralen using molecular docking and enzymatic studies. J. Pharm. Bioallied Sci. 7 (1), 32–36. doi:10.4103/0975-7406.148775
Song, J., Shu, L., Zhang, Z., Tan, X., Sun, E., Jin, X., et al. (2012). Reactive oxygen species-mediated mitochondrial pathway is involved in Baohuoside I-induced apoptosis in human non-small cell lung cancer. Chem. Biol. Interact. 199 (1), 9–17. doi:10.1016/j.cbi.2012.05.005
Song, K., Zhang, Q., Wang, X., and Liu, Y. (2019). Study on medication law of modern Chinese medicine in the treatment of Alzheimer's disease based on data mining. World Sci. Technol. - Mod. Traditional Chin. Med. 21 (07), 1424–1429. doi:10.11842/wst.2019.07.021
Song, L., Li, X., Bai, X. X., Gao, J., and Wang, C. Y. (2017). Calycosin improves cognitive function in a transgenic mouse model of Alzheimer's disease by activating the protein kinase C pathway. Neural Regen. Res. 12 (11), 1870–1876. doi:10.4103/1673-5374.219049
Spencer, B., Desplats, P. A., Overk, C. R., Valera-Martin, E., Rissman, R. A., Wu, C., et al. (2016). Reducing endogenous α-synuclein mitigates the degeneration of selective neuronal populations in an Alzheimer's disease transgenic mouse model. J. Neurosci. 36 (30), 7971–7984. doi:10.1523/JNEUROSCI.0775-16.2016
Sperling, R. A., Aisen, P. S., Beckett, L. A., Bennett, D. A., Craft, S., Fagan, A. M., et al. (2011). Toward defining the preclinical stages of Alzheimer's disease: recommendations from the National Institute on Aging-Alzheimer's Association workgroups on diagnostic guidelines for Alzheimer's disease. Alzheimers Dement. 7 (3), 280–292. doi:10.1016/j.jalz.2011.03.003
Spillantini, M. G., Schmidt, M. L., Lee, V. M., Trojanowski, J. Q., Jakes, R., and Goedert, M. (1997). Alpha-synuclein in Lewy bodies. Nature 388 (6645), 839–840. doi:10.1038/42166
Squire, L. R. (1992). Memory and the hippocampus: a synthesis from findings with rats, monkeys, and humans. Psychol. Rev. 99 (2), 195–231. doi:10.1037/0033-295x.99.2.195
Stellos, K., Panagiota, V., Sachsenmaier, S., Trunk, T., Straten, G., Leyhe, T., et al. (2010). Increased circulating progenitor cells in Alzheimer's disease patients with moderate to severe dementia: evidence for vascular repair and tissue regeneration? J. Alzheimers Dis. 19 (2), 591–600. doi:10.3233/JAD-2010-1261
Stricher, F., Macri, C., Ruff, M., and Muller, S. (2013). HSPA8/HSC70 chaperone protein: structure, function, and chemical targeting. Autophagy 9 (12), 1937–1954. doi:10.4161/auto.26448
Stutzbach, L. D., Xie, S. X., Naj, A. C., Albin, R., Gilman, S., Lee, V. M., et al. (2013). The unfolded protein response is activated in disease-affected brain regions in progressive supranuclear palsy and Alzheimer's disease. Acta Neuropathol. Commun. 1, 31. doi:10.1186/2051-5960-1-31
Su, R., Han, Z., Fan, J., and Zhang, Y. (2011). Significance of the "toxin damaging brain network" theory in the field of traditional Chinese medicine research on Alzheimer's disease. J. Traditional Chin. Med. 52 (16), 1370–1371. doi:10.13288/j.11-2166/r.2011.16.008
Sudhakar, A., Ramachandran, A., Ghosh, S., Hasnain, S. E., Kaufman, R. J., and Ramaiah, K. V. (2000). Phosphorylation of serine 51 in initiation factor 2 alpha (eIF2 alpha) promotes complex formation between eIF2 alpha(P) and eIF2B and causes inhibition in the guanine nucleotide exchange activity of eIF2B. Biochemistry 39 (42), 12929–12938. doi:10.1021/bi0008682
Sun, W., Qureshi, H. Y., Cafferty, P. W., Sobue, K., Agarwal-Mawal, A., Neufield, K. D., et al. (2002). Glycogen synthase kinase-3beta is complexed with tau protein in brain microtubules. J. Biol. Chem. 277 (14), 11933–11940. doi:10.1074/jbc.M107182200
Sun, X., Huang, C., and Li, Z. (2022). A study on medication regularities of national patented TCM compound prescription in the treatment of Alzheimer's disease. Clin. Res. Traditional Chin. Med. 14 (31), 42–46. doi:10.3969/j.issn.1674-7860.2022.31.009
Swerdlow, R. H. (2018). Mitochondria and mitochondrial cascades in Alzheimer's disease. J. Alzheimers Dis. 62 (3), 1403–1416. doi:10.3233/JAD-170585
Szklarczyk, D., Kirsch, R., Koutrouli, M., Nastou, K., Mehryary, F., Hachilif, R., et al. (2023). The STRING database in 2023: protein–protein association networks and functional enrichment analyses for any sequenced genome of interest. Nucleic Acids Res. 51 (D1), D638–D646. doi:10.1093/nar/gkac1000
Takashima, A., Noguchi, K., Michel, G., Mercken, M., Hoshi, M., Ishiguro, K., et al. (1996a). Exposure of rat hippocampal neurons to amyloid beta peptide (25–35) induces the inactivation of phosphatidyl inositol-3 kinase and the activation of tau protein kinase I/glycogen synthase kinase-3 beta. Neurosci. Lett. 203, 33–36. doi:10.1016/0304-3940(95)12257-5
Takashima, A., Sato, M., Mercken, M., Tanaka, S., Kondo, S., Honda, T., et al. (1996b). Localization of Alzheimer-associated presenilin 1 in transfected COS-7 cells. Biochem. Biophys. Res. Commun. 227, 423–426. doi:10.1006/bbrc.1996.1523
Tian, E., Sharma, G., and Dai, C. (2023). Neuroprotective properties of berberine: molecular mechanisms and clinical implications. Antioxidants (Basel) 12 (10), 1883. doi:10.3390/antiox12101883
Twohig, D., and Nielsen, H. M. (2019a). α-synuclein in the pathophysiology of Alzheimer's disease. Mol. Neurodegener. 14 (1), 23. doi:10.1186/s13024-019-0320-x
Twohig, D., and Nielsen, H. M. (2019b). α-synuclein in the pathophysiology of Alzheimer's disease. Mol. Neurodegener. 14 (1), 23. doi:10.1186/s13024-019-0320-x
Uddin, M. S., Tewari, D., Sharma, G., Kabir, M. T., Barreto, G. E., Bin-Jumah, M. N., et al. (2020). Molecular mechanisms of ER stress and UPR in the pathogenesis of Alzheimer's disease. Mol. Neurobiol. 57 (7), 2902–2919. doi:10.1007/s12035-020-01929-y
Umahara, T., Uchihara, T., Tsuchiya, K., Nakamura, A., Iwamoto, T., Ikeda, K., et al. (2004). 14-3-3 proteins and zeta isoform containing neurofibrillary tangles in patients with Alzheimer's disease. Acta Neuropathol. 108 (4), 279–286. doi:10.1007/s00401-004-0885-4
Vanderweyde, T., Apicco, D. J., Youmans-Kidder, K., Ash, P. E. A., Cook, C., Lummertz da Rocha, E., et al. (2016). Interaction of tau with the RNA-binding protein TIA1 regulates tau pathophysiology and toxicity. Cell. Rep. 15 (7), 1455–1466. doi:10.1016/j.celrep.2016.04.045
Wang, C. Y. (2022). Study on the effect of Forsythoside A on alleviating Alzheimer's disease through the Nrf2/GPX4 pathway. Doctoral thesis. Changchun, China: Jilin University. doi:10.27162/d.cnki.gjlin.2022.000193
Wang, D. M., Yang, Y. J., Zhang, L., Zhang, X., Guan, F. F., and Zhang, L. F. (2013). Naringin enhances CaMKII activity and improves long-term memory in a mouse model of Alzheimer's disease. Int. J. Mol. Sci. 14 (3), 5576–5586. doi:10.3390/ijms14035576
Wang, F., Fei, M., Hu, W. Z., Wang, X. D., Liu, S., Zeng, Y., et al. (2022). Prevalence of constipation in elderly and its association with dementia and mild cognitive impairment: a cross-sectional study. Front. Neurosci. 15, 821654. doi:10.3389/fnins.2021.821654
Wang, H. (2011a). The effect of curcumin on brain energy metabolism in APP/PS1 double transgenic mice. Master’s thesis. Beijing, China: Beijing University of Chinese Medicine. Available at: https://kns.cnki.net/kcms2/article/abstract?v=8WLnD7pOpNEeoy5QidlrwcQkc4yvRVKYNOt1z_33-GW1mkBnY0oOPeCI8p3nicp9qnJ83dNZ4JIOOxgxeO0teqcojVBqQzU0jiM5mGCoqOiviQDWko7KbEXDUdR97GcpRdRoNN8_L63sz7OTkl6mtQ==&uniplatform=NZKPT&language=CHS.
Wang, H., Chi, J., Jiang, X., and Yuan, T. (2019). Advances in the pharmacology and clinical research of stilbene glycoside extracted from Polygonum multiflorum. Chin. Archives Traditional Chin. Med. 37 (10), 2464–2469. doi:10.13193/j.issn.1673-7717.2019.10.039
Wang, H., Sharma, L., Lu, J., Finch, P., Fletcher, S., and Prochownik, E. V. (2015). Structurally diverse c-Myc inhibitors share a common mechanism of action involving ATP depletion. Oncotarget 6 (18), 15857–15870. doi:10.18632/oncotarget.4327
Wang, P. W. (2011b). The neuroprotective effect of curcumin on APP/PS1 double transgenic mice. Doctoral thesis. Beijing, China: Beijing University of Chinese Medicine. Available at: https://kns.cnki.net/kcms2/article/abstract?v=8WLnD7pOpNG-cYV_5dpx_vC7ofdB9awshvp0pJ3w1Uf2ogAY-TX7w36Ynbme6dY8OW1E457s3CYviPlOoJSUXY6Z-ZF_ejG4hx61NWx3C4r6vq5QDGLHiPW_kDDAdwWq83dVMXo0_zxOAHxZ72OrwQ==&uniplatform=NZKPT&language=CHS.
Wang, Q., Song, F., Zhang, C., Zhao, X., Zhu, Z., Yu, S., et al. (2011). Carboxyl-terminus of Hsc70 interacting protein mediates 2,5-hexanedione-induced neurofilament medium chain degradation. Biochem. Pharmacol. 81 (6), 793–799. doi:10.1016/j.bcp.2010.12.021
Wang, W., Zhao, F., Lu, Y., Siedlak, S. L., Fujioka, H., Feng, H., et al. (2023). Damaged mitochondria coincide with presynaptic vesicle loss and abnormalities in alzheimer’s disease brain. acta neuropathol. Commun. 11, 54. doi:10.1186/s40478-023-01552-7
Wang, X., Wang, W., Li, L., Perry, G., Lee, H. G., and Zhu, X. (2014). Oxidative stress and mitochondrial dysfunction in Alzheimer's disease. Biochim. Biophys. Acta 1842 (8), 1240–1247. doi:10.1016/j.bbadis.2013.10.015
Wang, X., Yao, X., Ma, S., and Shi, J. (2021b). Network pharmacological study on mechanism of Compound Congrong Yizhi Capsule in treatment of Alzheimer's disease. Chin. J. New Drugs Clin. Remedies 32 (02), 172–181. doi:10.19378/j.issn.1003-9783.2021.02.004
Wang, X. F., Xiao, H. H., Wu, Y. T., Kong, L., Chen, J. C., Yang, J. X., et al. (2021a). Active constituent of Polygala tenuifolia attenuates cognitive deficits by rescuing hippocampal neurogenesis in APP/PS1 transgenic mice. BMC Complement. Med. Ther. 21 (1), 267. doi:10.1186/s12906-021-03437-5
Wang, Y., Martinez-Vicente, M., Krüger, U., Kaushik, S., Wong, E., Mandelkow, E. M., et al. (2009). Tau fragmentation, aggregation and clearance: the dual role of lysosomal processing. Hum. Mol. Genet. 18 (21), 4153–4170. doi:10.1093/hmg/ddp367
Wang, Z., Liu, Y., Xue, Y., Hu, H., Ye, J., Li, X., et al. (2016). Berberine acts as a putative epigenetic modulator by affecting the histone code. Toxicol Vitro 36, 10–17. doi:10.1016/j.tiv.2016.06.004
Wang, Z., Ma, D., Wang, M., Liu, T., Gao, F., Li, Y., et al. (2024). Research progress on mechanism of Astragali Radix and its active ingredients in prevention and treatment of Alzheimer's disease. Chin. Tradit. Herb. Drugs, 55 (06), 2094–2100.
Wei, P. (2013). The effect of curcumin on the expression of Shank1 and PSD95 in AD mice. Master’s thesis. Beijing, China: Beijing University of Chinese Medicine. Available at: https://kns.cnki.net/kcms2/article/abstract?v=8WLnD7pOpNG-ZZylnudvM-wQIxmLNBiKDtmekqPyu5tcaAMqqIBR0RTAathYSF-eMjUG72redl6Nnu8xPuscTrhMjdGvOySHIha3oxWqUtS93CPL60bgUpayooPKIQesCkk3JA8rq3D5aqd0abEdnw==&uniplatform=NZKPT&language=CHS.
Wei, W., Yao, J. X., Zhang, T. T., Wen, J. Y., Zhang, Z., Luo, Y. M., et al. (2023). Network pharmacology reveals that Berberine may function against Alzheimer's disease via the AKT signaling pathway. Front. Neurosci. 17, 1059496. doi:10.3389/fnins.2023.1059496
Wiborg, O., Pedersen, M. S., Wind, A., Berglund, L. E., Marcker, K. A., and Vuust, J. (1985). The human ubiquitin multigene family: some genes contain multiple directly repeated ubiquitin coding sequences. EMBO J. 4 (3), 755–759. doi:10.1002/j.1460-2075.1985.tb03693.x
Wickham, H. (2016). ggplot2: elegant graphics for data analysis. 2nd ed. Springer. Available at: https://ggplot2.tidyverse.org.
Wolozin, B., and Ivanov, P. (2019). Stress granules and neurodegeneration. Nat. Rev. Neurosci. 20 (11), 649–666. doi:10.1038/s41583-019-0222-5
Wong, L. R., Tan, E. A., Lim, M. E. J., Shen, W., Lian, X. L., Wang, Y., et al. (2021). Functional effects of berberine in modulating mitochondrial dysfunction and inflammatory response in the respective amyloidogenic cells and activated microglial cells - in vitro models simulating Alzheimer's disease pathology. Life Sci. 282, 119824. doi:10.1016/j.lfs.2021.119824
World Health Organization (2023). Dementia [fact sheet]. Available at: https://www.who.int/news-room/fact-sheets/detail/dementia.
Wu, C. H., Chen, C. H., Hsieh, P. F., Lee, Y. H., Kuo, W. W., Wu, R. C., et al. (2021b). Verbascoside inhibits the epithelial-mesenchymal transition of prostate cancer cells through high-mobility group box 1/receptor for advanced glycation end-products/TGF-β pathway. Environ. Toxicol. 36 (6), 1080–1089. doi:10.1002/tox.23107
Wu, S., Zhu, H., and Chen, X. (2021c). Analysis of medication patterns for Alzheimer's disease based on a modern traditional Chinese medicine literature database. Zhejiang J. Integr. Traditional Chin. West. Med. 31 (06), 568–572. doi:10.3969/j.issn.1005-4561.2021.06.021
Wu, Y. (2021). Study on the molecular mechanism of berberine affecting the cognitive function of APP/PS1 mice. Doctoral thesis. Wuhan, China: Huazhong University of Science and Technology. doi:10.27157/d.cnki.ghzku.2021.006532
Wu, Y., Qiu, S., Kang, Y., Gao, M., Chen, M., Zhang, Y., et al. (2021a). The protective effects and mechanisms of astragaloside IV on memory disorder in mice induced by scopolamine. J. Shandong First Med. Univ. Shandong Acad. Med. Sci. 42 (10), 734–738. doi:10.27157/d.cnki.ghzku.2021.006532
Yan, L. L. (2017). Study on the effects of icariin on learning and memory impairment in Alzheimer's disease model mice and its mechanism. Master’s thesis. Zunyi, China: Zunyi Medical College. Available at: https://kns.cnki.net/kcms2/article/abstract?v=8WLnD7pOpNGKTBP1WejHd6nG0L8u74lBKmLMEnEzwgamzBuYzIIt8HWspnGbtAQDh47kmoatAwhphV4NzXnvh8KaPHSHmQnjBY0K_UlElyN6UK7tSg0POiQKlUMY4ChNxnYP3GiHva3riSKVllinKw==&uniplatform=NZKPT&language=CHS.
Yang, D. (2004). Drynaria fortunei is effective in treating Alzheimer's disease. J. Traditional Chin. Med. (04), 249. doi:10.13288/j.11-2166/r.2004.04.003
Yang, S. S. (2019). Study on the acetylation mechanism of Saponin treatment in Alzheimer's disease. Shanghai, China: Shanghai Jiao Tong University. Available at: doi:10.27307/d.cnki.gsjtu.2019.004603
Yang, X., and Tohda, C. (2018). Heat shock cognate 70 inhibitor, VER-155008, reduces memory deficits and axonal degeneration in a mouse model of Alzheimer's disease. Front. Pharmacol. 9, 48. doi:10.3389/fphar.2018.00048
Yang, X. H. (2017). Study on the effect of Dendrobium nobile alkaloids on learning and memory of APP/PS1 transgenic mice and related factors. Zunyi, China: Zunyi Medical College. Available at: https://kns.cnki.net/kcms2/article/abstract?v=8WLnD7pOpNEC6EvftGNEV3VDt5fRemtteWUGw8BswoRN_XjrUO5PiI7XzDlM8W2P5ofO6bePmqGlOJrShYlgh8a4XdI1iPj36FnNhsZ4cueWBjTeqS3bajMnTMUNtQBuLcgGoQ6ib5uRIAV37Z4_-w==&uniplatform=NZKPT&language=CHS.
Yao, H., Gu, L., and Guo, J. (2014). Study on effect of Astragali Radix polysaccharides in improving learning and memory functions in aged rats and its mechanism. China J. Chin. Materia Medica 39 (11), 2071–2075. doi:10.4268/cjcmm20141125
Yigit, B., Wang, N., Herzog, R. W., and Terhorst, C. (2019). SLAMF6 in health and disease: implications for therapeutic targeting. Clin. Immunol. 204, 3–13. doi:10.1016/j.clim.2018.10.013
Yoo, B. C., Fountoulakis, M., Cairns, N., and Lubec, G. (2001). Changes of voltage-dependent anion-selective channel proteins VDAC1 and VDAC2 brain levels in patients with Alzheimer's disease and Down syndrome. Electrophoresis 22 (1), 172–179. doi:10.1002/1522-2683(200101)22:1<172::AID-ELPS172>3.0.CO;2-P
Young, Z. T., Rauch, J. N., Assimon, V. A., Jinwal, U. K., Ahn, M., Li, X., et al. (2016). Stabilizing the hsp70-tau complex promotes turnover in models of tauopathy. Cell. Chem. Biol. 23 (8), 992–1001. doi:10.1016/j.chembiol.2016.04.014
Yu, R., Jin, G., and Fujimoto, M. (2021). Dihydroartemisinin: a potential drug for the treatment of malignancies and inflammatory diseases. Front Oncol. 11, 722331. doi:10.3389/fonc.2021.722331
Yu, G., Wang, L. G., Han, Y., and He, Q. Y. (2012). clusterProfiler: an R package for comparing biological themes among gene clusters. OMICS 16 (5), 284–287. doi:10.1089/omi.2011.0118
Yu, R., Jin, G., and Fujimoto, M. (2021). Dihydroartemisinin: a potential drug for the treatment of malignancies and inflammatory diseases. Front. Oncol. 11, 722331. doi:10.3389/fonc.2021.722331
Yu, X. F. (2018). Study on the protective effect and mechanism of Eucommia Extract on APP/PS1 transgenic AD model mice and Aβ-damaged PC12 cells. Master’s thesis. Harbin, China: Heilongjiang University of Chinese Medicine. doi:10.27127/d.cnki.ghlzu.2018.000065
Yuan, L. (2016). Age-related changes in oxidative/antioxidative properties and neurotrophic factor expression in the hippocampus of mice; the effects of traditional Chinese medicine components on learning and memory ability, oxidative/antioxidative balance in APP/PS1 transgenic mice [Dissertation]. Chongqing: Chongqing Medical University. doi:10.7666/d.D01120429
Zarei, M., Beckmann, C. F., Binnewijzend, M. A., Schoonheim, M. M., Oghabian, M. A., Sanz-Arigita, E. J., et al. (2013). Functional segmentation of the hippocampus in the healthy human brain and in Alzheimer's disease. Neuroimage 66, 28–35. doi:10.1016/j.neuroimage.2012.10.071
Zhang, D., Wang, S., Zhu, L., Tian, Y., Wang, H., Zhuang, Y., et al. (2013). Profiling of hepatocellular carcinoma cell cycle regulating genes targeted by calycosin. Biomed. Res. Int. 2013, 317926. doi:10.1155/2013/317926
Zhang, H., Shan, Y., Wu, Y., Xu, C., Yu, X., Zhao, J., et al. (2017). Berberine suppresses LPS-induced inflammation through modulating Sirt1/NF-κB signaling pathway in RAW264.7 cells. Int. Immunopharmacol. 52, 93–100. doi:10.1016/j.intimp.2017.08.032
Zhang, J., Yang, C., Wei, D., Li, H., Leung, E. L., Deng, Q., et al. (2019). Long-term efficacy of Chinese medicine Bushen Capsule on cognition and brain activity in patients with amnestic mild cognitive impairment. Pharmacol. Res. 146, 104319. doi:10.1016/j.phrs.2019.104319
Zhang, M., Qu, Y., and An, H. (2023b). Analysis on the change characteristics of kidney deficiency syndrome in the proqressive development of Alzheimer's disease. Jilin J. Chin. Med. 43 (11), 1361–1364. doi:10.13463/j.cnki.jlzyy.2023.11.026
Zhang, S., Xu, P., Zhu, Z., Zhou, L., Li, J., Zhou, R., et al. (2023a). Acetylation of p65Lys310 by p300 in macrophages mediates anti-inflammatory property of berberine. Redox Biol. 62, 102704. doi:10.1016/j.redox.2023.102704
Zhang, X., Zhao, Y., Huang, X., Qiu, Z. D., Guo, L. P., Qu, X. B., et al. (2016). A review of research on genuine medicinal materials of Artemisia annua. China J. Chin. Materia Medica 41 (11), 2015–2018. doi:10.4268/cjcmm20161107
Zhang, Y., Lin, C., Zhang, L., Cui, Y., Gu, Y., Guo, J., et al. (2015a). Cognitive improvement during treatment for mild Alzheimer's disease with a Chinese herbal formula: a randomized controlled trial. PLoS One 10 (6), e0130353. doi:10.1371/journal.pone.0130353
Zhang, Z. H. (2019). Study on the protective effect of Genipin on APP/PS1 mice behavior damage and pathological changes—suppressing mTOR signaling pathway to enhance autophagy. Doctoral thesis. Taiyuan, China: Shanxi Medical University. Available at: https://kns.cnki.net/kcms2/article/abstract?v=8WLnD7pOpNHTaExJYB65R6cRduLEudLxPFrQd14MyV7-kJGLq1faIO1lw7cA8zY1Sr87UKLn7J264IYLln-IXo6N-mxHLtGkxhOK2SkyZtq6VBY5I-Wo1HApYA-sH1PAZ5Wd7TBBCDdpGEeKL8P3pg==&uniplatform=NZKPT&language=CHS.
Zhang, Z.-W., Zhang, T., and Zhu, H. (2015b). Icarin upregulates brain-derived neurotrophic factors in the hippocampus of the senescence-acceler-ated mouse. Chin. J. Clin. Physicians 17 (2), 178–182. doi:10.3760/cma.j.issn.1008-1372.2015.02.007
Zhao, D. P. (2022). Study on the protective effect of Rehmannia on blood-brain barrier in APP/PS1 mice and the clinical application of Chinese medicine formula with Rehmannia as monarch medicine in Alzheimer's disease. Doctoral thesis. Nanjing, China: Nanjing University of Chinese Medicine. Available at: doi:10.27253/d.cnki.gnjzu.2022.000683
Zhao, Q., Yang, X., Cai, D., Ye, L., Hou, Y., Zhang, L., et al. (2016). Echinacoside protects against MPP(+)-Induced neuronal apoptosis via ROS/ATF3/CHOP pathway regulation. Neurosci. Bull. 32 (4), 349–362. doi:10.1007/s12264-016-0047-4
Zheng, W., Liu, H., Li, Z., Li, K., Wang, Y., Hu, B., et al. (2023). Classification of Alzheimer's disease based on hippocampal multivariate morphometry statistics. CNS Neurosci. Ther. 29 (9), 2457–2468. doi:10.1111/cns.14189
Zhong, D., Li, L., Jiang, C., and Song, Z. (2021). Research on medication rule of modern doctors in the treatment of Alzheimer disease based on data mining. China Med. Her. 18 (18), 131–135. Available at: https://kns.cnki.net/kcms2/article/abstract?v=6RtRr0kVassD2YZQMjI4Xnx-eefD8IttBJYrJPXQB1FZJKxBOl_QX3tedBJoOwSpdwU01t2U7bgFZZ-7SPh2XROXmdQ5mmgnf3GotI3YaTyX7WwUAPzZDxAkP6zVIlBFuEX2wFc5oDlmOEjo5B8CF87Gzqg1aJtCd0RHOXo1PrGc3XULaiuirBjJY3H9k0t9yFJo-sEJBzI=&uniplatform=NZKPT&language=CHS.
Zhong, M. C., and Veillette, A. (2008). Control of T lymphocyte signaling by Ly108, a signaling lymphocytic activation molecule family receptor implicated in autoimmunity. J. Biol. Chem. 283 (28), 19255–19264. doi:10.1074/jbc.M800209200
Zhou, J., Deng, Y., Li, F., Yin, C., Shi, J., and Gong, Q. (2019). Icariside II attenuates lipopolysaccharide-induced neuroinflammation through inhibiting TLR4/MyD88/NF-κB pathway in rats. Biomed. Pharmacother. 111, 315–324. doi:10.1016/j.biopha.2018.10.201
Zhou, W. Q. (2009). Study on the effect of icariin on the learning and memory of rapidly aging SAMP8 mice and its mechanism. Master’s thesis. Beijing, China: Chinese Academy of Medical Sciences. Available at: https://kns.cnki.net/kcms2/article/abstract?v=8WLnD7pOpNFTeDh3Bh1ET6AsOpyiyvKYQGtDMKWlohnr0eR4sJ56gCdxp6zRENVUOdE8reoyQeGg-fCPWAvm6VqvmfdNpXZoJGzyVfQANifw2G9obs15LYRZtVQwONt_KHgxODOcjLoFBmeQh_4C4w==&uniplatform=NZKPT&language=CHS.
Zhu, M., Lu, C., and Li, W. (2013). Transient exposure to echinacoside is sufficient to activate Trk signaling and protect neuronal cells from rotenone. J. Neurochem. 124 (4), 571–580. doi:10.1111/jnc.12103
Zhu, T. R. (2019). Study on the effect of Icariin on lymphocyte-mediated immune-inflammatory response in Alzheimer's disease. Doctoral thesis. Jinan, China: Shandong University. doi:10.27272/d.cnki.gshdu.2019.000112
Keywords: GEO dataset, bioinformatics, mitochondrial autophagy, echinacoside, SNCA, UBB
Citation: Zhiyan C, Min Z, Yida D, Chunying H, Xiaohua H, Yutong L, Huan W and Linjuan S (2024) Bioinformatic analysis of hippocampal histopathology in Alzheimer’s disease and the therapeutic effects of active components of traditional Chinese medicine. Front. Pharmacol. 15:1424803. doi: 10.3389/fphar.2024.1424803
Received: 02 May 2024; Accepted: 17 July 2024;
Published: 16 August 2024.
Edited by:
Valentina Echeverria Moran, United States Department of Veterans Affairs, United StatesReviewed by:
Mirco Masi, Italian Institute of Technology (IIT), ItalyCopyright © 2024 Zhiyan, Min, Yida, Chunying, Xiaohua, Yutong, Huan and Linjuan. This is an open-access article distributed under the terms of the Creative Commons Attribution License (CC BY). The use, distribution or reproduction in other forums is permitted, provided the original author(s) and the copyright owner(s) are credited and that the original publication in this journal is cited, in accordance with accepted academic practice. No use, distribution or reproduction is permitted which does not comply with these terms.
*Correspondence: Sun Linjuan, c3VubGluanVhbjg4OEAxNjMuY29t
Disclaimer: All claims expressed in this article are solely those of the authors and do not necessarily represent those of their affiliated organizations, or those of the publisher, the editors and the reviewers. Any product that may be evaluated in this article or claim that may be made by its manufacturer is not guaranteed or endorsed by the publisher.
Research integrity at Frontiers
Learn more about the work of our research integrity team to safeguard the quality of each article we publish.