- School of Pharmacy, Shandong University of Traditional Chinese Medicine, Jinan, China
Introduction: Morroniside (Mor) is a bioactive compound found in Corni Fructus (CF) [Cornaceae; Cornus officinalis Siebold & Zucc.], which has been used as medicine and food in China, Korea, and Japan for over 2,000 years. This review summarizes recent progress on Mor, specifically focusing on its distribution, isolation, detection, and various pharmacological effects.
Methods: A literature survey on Mor was conducted using electronic databases such as PubMed, ScienceDirect, CNKI, and Google Scholar. After removing TCM prescription-related standards, medicinal herb processing-related research, and other irrelevant works of literature, we obtained relevant information on Mor’s biological and pharmacological properties.
Results: The main conclusions are as follows: Mor is widely distributed in the plant kingdom; the methods for extracting and isolating Mor are well established; and the technology for detecting it is accurate. Mor exhibits numerous pharmacological effects. Along with CF, Mor has shown renoprotective effects against diabetes, hepatoprotective effects against diabetes, triptolide, and nonalcoholic steatohepatitis, and boneprotective effects against osteoporosis and osteoarthritis. In addition, researchers have also explored other pharmacological effects of Mor, including neuroprotective effects against focal cerebral ischemia, spinal cord injury, and Alzheimer’s disease; cardioprotective effects against acute myocardial infarction; protection of the digestive system from gastritis, inflammatory bowel disease, and colitis; protection of the skin by promoting hair growth, wound healing, and flap survival; and protection of the lungs from acute lung injury and pulmonary fibrosis. Moreover, Mor has anti-obesity effects, anti-inflammatory effects in the eye, and improves follicular development.
Discussion: Overall, this review provides a comprehensive understanding of the pharmacological effects of Mor, from which the limitations of the current research can be understood, which will help facilitate future research.
1 Introduction
Corni Fructus (CF) was first recorded in Shen Nong Materia Medica and extensively used as a medicine and food in China, Korea, and Japan. Its main functional indications are replenishing the liver and kidney and arresting the loss of essence (Chinese Pharmacopoeia, 2020). Liu Wei Di Huang Wan (六味地黄丸) (Ge et al., 2018) and Jin Gui Shen Qi Wan (金匮肾气丸) (Xu and Cao, 2015) are the famous representatives of replenishing the liver and kidney.
Morroniside (Mor), an iridoid glycoside (Figure 1), is the primary bioactive and representative ingredient in CF. With the continuous expansion of the pharmacological effects of CF, Mor has attracted attention. The multiple pharmacological effects, including neuroprotective, bone-protective, cardioprotective, renoprotective, and hepatoprotective effects, suggest that Mor has considerable potential value in the prevention and treatment of diseases such as focal cerebral ischemia, spinal cord injury, Alzheimer’s disease, osteoporosis, osteoarthritis, acute myocardial infarction, and diabetes. This review provides the latest and most comprehensive data on Mor’s extraction, detection, and pharmacology, which will help facilitate further research and provide a reference resource for its clinical application.
2 Sources of Mor
2.1 Distribution of Mor
The compound Mor is found in a wide variety of plants. To date, 17 plant species have been reported to contain Mor. Detailed information and images of these plants can be found in Table 1. These species include Sambucus williamsii (Liang et al., 2011), Chione venosa (Lendl et al., 2005), Adina pilulifera (Xue et al., 2007), Lonicera macranthoides (Sun et al., 2011), Sarracenia purpurea (Cieniak et al., 2015), Mussaenda luteola (Mohamed et al., 2016), L. japonica (Cai et al., 2019), Angelica gigas, Glycyrrhiza uralensis, G. glabra, G. inflata, Schisandra chinensis (Ahn et al., 2020), Gentiana straminea (Zhou et al., 2021), Patrinia scabra (Ma et al., 2015), Caulophyllum robustum (Li et al., 2015), and Gentiana olivieri Griseb (Maituoheti et al., 2023). They belong to class Angiospermae and are broadly categorized into Apiaceae, Berberidaceae, Caprifoliaceae, Fabaceae, Gentianaceae, Rubiaceae, Sarraceniaceae, and Viburnaceae. Mor has a large reserve in the plant kingdom; therefore, developing and utilizing these plants would be beneficial. In addition, researchers discovered that the 3-hydroxy-3-methylglutaryl-CoA synthase (HMGS) gene plays a crucial role in the synthesis of Mor in Cornus officinalis (Zhang et al., 2023) and that the C. officinalis geranyl pyrophosphate synthase (CoGPPS) is another critical gene involved in the biosynthesis of Mor (Chen J. et al., 2024). This discovery offers valuable insight into the genetic mechanism responsible for the production of Mor in this plant.
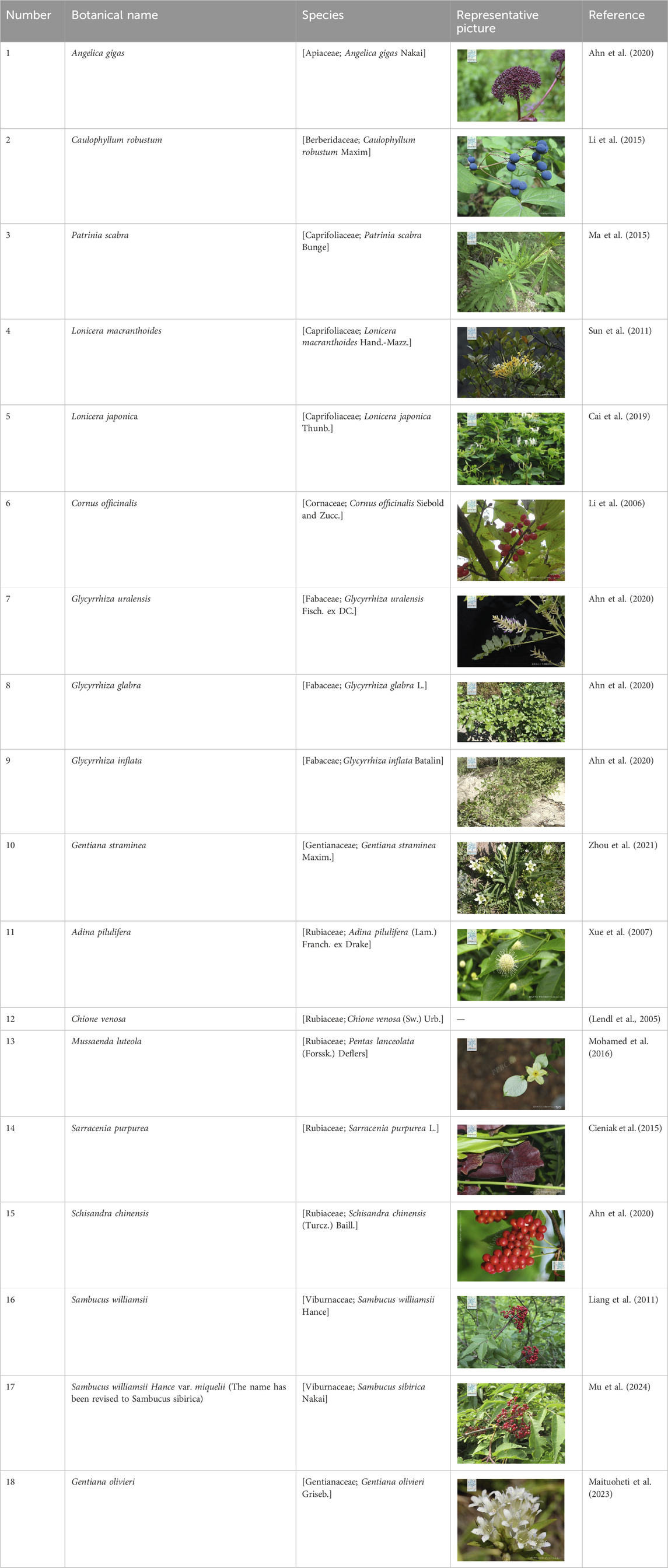
Table 1. Plant species information and their respective photos (representative pictures provided by https://www.plantplus.cn/cn).
2.2 Isolation and purification of Mor
Mor is mainly extracted and separated from CF, and this article reviews the techniques and simpler steps used in purification. Isolation and purification of Mor are performed as follows: CF is extracted with hot water and precipitated by adding alcohol (four times the amount of water). Subsequently, the extract is concentrated using a rotary evaporator and isolated using AB-8 macroporous resin. Afterward, 30% ethanol eluent is pooled and passed through silica gel with detection using thin-layer chromatography; then, the mixture is purified by high-performance liquid chromatography (HPLC) to 98% (Li et al., 2006). In a simplified process, Mor is obtained from CF in a two-step separation process. In brief, a fraction of 40% ethanol is collected using a macroporous absorption resin column, following ultrasonic extraction with 50% methanol. Subsequently, high-speed countercurrent chromatography (CCC) separation is performed to achieve a final purity of 97.8% (Liu et al., 2009). Another extraction method consists of refluxing with 75% ethanol. Afterward, 50% aqueous ethanol eluent is concentrated after the water elution becomes colorless using D101 macroporous resin. The mixture is subjected to CCC separation to achieve a final purity of 99.1% (Liang et al., 2013). Moreover, CF was crushed and extracted with 70% ethanol. The resulting extracts were filtered, evaporated, and freeze-dried. The fraction was obtained after being subjected to Diaion HP-20 chromatography using 30% ethanol and was further separated using a C18 MPLC (Oh et al., 2024). With the rapid progress in technology, particularly in the development of polymer materials, the isolation and purification of Mor from plants are anticipated to become more convenient, efficient, and eco-friendly. This encourages greater exploration and study of Mor, increasing possibilities for its utilization.
2.3 Detection of Mor
The detection of Mor is essential for the quality control of CF, and upgrading detection technology makes the detection method more accurate, simple, and convenient. The detailed detection methods are summarized in Table 2 Using micellar electrokinetic capillary chromatography (MEKC), Mor has been isolated from eight other components in CF (Wang et al., 2003) and Liu Wei Di Huang Wan (Zhao et al., 2007). Additionally, Mor has been detected using the diode array detector (DAD) and variable wavelength detector (VWD) at 218 nm (Du et al., 2008), evaporative light-scattering detector (ESLD), and electrospray ionization mass spectrometry (ESI-MS) (Ye et al., 2009). Paper spray mass spectrometry (PS-MS) (Guo et al., 2017) and near-infrared spectroscopy (NIRS) (Gong et al., 2015) have been applied to evaluate CF quality. Pre-treatment methods have been improved to simultaneously detect as many iridoid glycosides as possible. Specifically, ionic liquid-based vortex-assisted matrix solid-phase dispersion (Du et al., 2018), molecularly imprinted solid-phase extraction (Ji et al., 2018) have been used to detect iridoid glycosides in CF and Liu Wei Di Huang Wan. The specialization of pre-treatment technology and the diversification of detection technology allow Mor to be analyzed qualitatively and quantitatively more rapidly and accurately. Moreover, a qualitative analysis of Mor was conducted among 134 compounds in the Shandong Yangxin capsule using ultra-high-performance liquid chromatography–Fourier-transform ion cyclotron resonance mass spectrometry (UPLC-FT-ICR-MS) (Fu et al., 2024). Another study demonstrated that ultra-performance liquid chromatography coupled with linear quadrupole ion trap-orbitrap mass spectrometry (UHPLC-LTQ-Orbitrap-MS) was able to identify 130 components of CF, including Mor (Sun et al., 2023).
MEKC effectively combines the advantages of chromatography and electrophoresis, making it widely used in protein and peptide separations. In recent years, the separation and detection of small molecules have become a new research direction, and its coupling with MS also provides the possibility for the detection of Mor. The convenience and accuracy of the HPLC system, in combination with DAD or ELSD, make it the most widely used method for qualitative and quantitative analyses of Mor. UPLC-FT-ICR-MS and UHPLC-LTQ-Orbitrap-MS can be used for the qualitative analysis of Mor very accurately, which provides strong technical support for the expansion of medicinal plant resources of Mor and the serum pharmacology and pharmacokinetics of prescriptions containing Mor.
3 Pharmacological effects of Mor
3.1 Protective effects of Mor on the nervous system
The protective effect of Mor on the nervous system can be classified into four categories: protection against focal cerebral ischemia, spinal cord injury, Alzheimer’s disease, and neuroprotective effects. The vital targets and putative pathways of Mor on the nervous system are summarized in Figures 2, 3, detailed pharmacological effects was listed in Table 3, and therelevant mechanisms are described as follows.
3.1.1 Protection of Mor against focal cerebral ischemia
Cerebral ischemia can result in death or permanent disability; the limited blood flow to the brain cuts off oxygen and other nutrients, causing cerebral ischemia (Girnar and Mahajan, 2021). Cerebral ischemia therapy is closely linked to angiogenesis, neurogenesis, blood–brain barrier (BBB), and cerebral blood flow (Ahad et al., 2020; Herpich and Rincon, 2020). Animal models of transient middle cerebral artery occlusion (MCAO) and permanent occlusion of extracranial vessels have been created to simulate cerebral ischemia conditions.
In MCAO rats, Mor improved neurobehavioral scores (Zea-Longa, Ludmila Belayer, and prehensile traction), reduced brain infarction volume, minimized oxidative stress by regulating MDA, glutathione (GSH), and superoxide dismutase (SOD), and reduced apoptosis by decreasing MMP2, MMP9, and caspase-3 while increasing the ratio of Bcl-2/Bax (Wang W. et al., 2010; Zeng et al., 2018).
Angiogenesis relies on the proliferation of endothelial progenitor cells (EPCs) (Wang et al., 2021). In MCAO, Mor increased vWF+ vessels and CD34+ cells, indicating an increase of new blood vessels in the brain, and angiogenic promoters (Ang-1, Tie-2, NRP-1, FGF-2, HGF, and VEGF) elevated EPC proliferation (Sun et al., 2014a; Wei et al., 2016; Liu et al., 2019). Previous studies on the long-term effects of Mor on angiogenesis in MCAO have shown that new vessels were generated after 14 days in the peri-infarcted cortex, and angiogenesis-related proteins, such as vascular endothelial growth factor receptor 2 (VEGFR2), ephrin-B2, Erk1/2, and Src, were increased. Additionally, in MCAO, regional cerebral blood flow dynamics and the number of vessels of the leptomeningeal anastomoses were improved by Mor, which indicated that the new vessels improved microvascular circulation (Liu et al., 2016).
Neurogenesis plays another important role in ischemia stroke treatment (Ruan et al., 2015). In MCAO rats, Ludmila Belayer demonstrated that the neurological function was improved by Mor, as well as the increase in endogenous neural stem cells marked with Ki-67 and nestin (a predominant protein marker for neural stem and progenitor cells) in the ischemic ipsilateral dorsolateral corner of the subventricular zone, the unilateral ventricle wall, and peri-infarct cortex. Furthermore, Mor increased the expression of Wnt3a, β-catenin, and Tcf-4, along with activating the downstream transcription factors Pax6 and Ngn2, but did not influence Tbr2 expression (Sun et al., 2014b). In addition, Mor promoted the proliferation of neural stem cells from oxygen-glucose deficiency and differentiation into neurons, as marked by Map2 and GFAP; after transfected with shRNA-EphB4, Mor still promoted the proliferation and differentiation with the weakened efforts (Sun et al., 2019). The accumulation of cyclin D1 caused by cerebral ischemia promotes programmed cell death by activating CDKs (Wen et al., 2005); Mor downregulated cyclin D1 and CDK6 to protect the brain (Liu et al., 2013). In HT-22 cells deprived of transitory oxygen and glucose, Mor increased viability and suppressed oxidative stress by reducing ROS and MDA while increasing SOD and GSH. Furthermore, Mor upregulated Nrf2, HO-1, and Bcl-2, downregulated Bax, cleaved caspase-3, and cleaved caspase-9 (Zhang L. et al., 2022). Therefore, Mor promotes neurogenesis to protect cerebral ischemia, which primarily promotes neuro differentiation, minimizes programmed cell death, reduces oxidative stress, and inhibits apoptosis.
The transcriptomic analysis of the brain following ischemia revealed that the microglia contributed 75% of the differentially expressed genes, which were primarily related to activity, differentiation, metastasis, and inflammation (Khan et al., 2017). Mor induced M2 polarization in primary microglial cells by increasing the expression of Agr1, C206, IL-4, and IL-10. Furthermore, Mor only affected IL-10 expression in microglial cells compared with astrocytes and neural cells. In another study, among various inhibitors, only p38 inhibitors reduced IL-10 expression in MCAO mice. At the same time, intracerebroventricular injection of Mor increased IL-10 expression in the cortical penumbra area, which reduced the infarction size reversed by exendin(9–39), suggesting that Mor is a GLP-1R agonist. Mor protects microglial cells by inducing M2 polarization and IL-10 expression in M2 microglia, possibly mediated by the cAMP/PKA/p38β pathway (Liu et al., 2021b).
3.1.2 Protection of Mor against spinal cord injury
Spinal cord injury (SCI) results in permanent neurological impairment with nearly no effective treatment, leading to severe lifelong disabilities and a significant burden to individuals, families, and society (Sachdeva et al., 2018). In OLN-93 cells (a cell line of oligodendrocytes) induced by H2O2, Mor diminished oxidative stress by attenuating ROS and MDA and increased mitochondrial membrane potential (MMP) by suppressing SOD2 and iNOS. Mor resisted apoptosis by upregulating Bcl-2 and downregulating cleaved caspase-3 and Bax. In addition, LY294002, an inhibitor of the PI3K/AKT pathway, inhibits the protective effect of Mor described above; therefore, Mor may protect SCI against oxidative stress and apoptosis via the PI3K/AKT signaling pathway (Li et al., 2021).
In another study, Mor has been shown to significantly improve the locomotor function of SCI rats, according to the Basso, Beattie, and Bresnahan locomotor rating scale, reduce the lesion area, improve the preservation of myelin, and alleviate motor neuron loss. In addition, Mor protected nerve cells from apoptosis by reducing caspase-3 expression and extenuated oxidative stress by increasing antioxidant enzymes (SOD, CAT, and GSH) and inhibiting oxygen free radicals (H2O2, MDA, 8-OHDG, and 3-NT) (Duan et al., 2021). According to RNA sequencing (RNA-seq) results, the specific mechanism is related to Mor’s anti-inflammatory and anti-apoptotic effects in SCI rats (Shi et al., 2022).
3.1.3 Protection of Mor against Alzheimer’s disease
Alzheimer’s disease is a common geriatric disease with considerable individual, social, and economic burden, which is increasingly attracting attention due to the growing elderly population (Author Anonymous, 2021). Studies have demonstrated that the pathogenesis of Alzheimer’s disease is related to abnormal deposition of β-amyloid protein, neurofibrillary tangles (NFTs) caused by tau protein hyperphosphorylation, neuroinflammatory response, mitochondrial dysfunction, and abnormal synaptic transduction function (DeTure and Dickson, 2019). Tau protein hyperphosphorylation in Alzheimer’s disease is attributed to low PP2A expression (Liu et al., 2005).
In P301S mice, a widely used transgenic model of tauopathy, the CF extract attenuated tau hyperphosphorylation at Thr205, Ser212, Ser262, Thr231, Ser235, and Ser404 (Yang et al., 2020). In AutoDock and surface plasmon resonance simulation tests, Mor showed high binding free energies and interactions as an inhibitor of acetylcholinesterase, butyrylcholinesterase, and beta-secretase 1 for treating Alzheimer’s disease (Bhakta et al., 2016). Calpain is abnormally activated in NFTs, N2a cells, and P301S mice; Mor inhibited the activity of calpain and glycogen synthase kinase 3β (GSK-3β) and enhanced the activity of PP2A (Guo et al., 2022).
In okadaic acid, a PP2A inhibitor, stimulated SK-N-SH cells, Mor attenuated tau hyperphosphorylation (Ser199/202, Thr205, Thr212, Ser214, and Thr217) by increasing PP2A and tau 5. Importantly, in HEK293 cells where PP2Ac expression was silenced by PP2Ac siRNA, the reduced effect of Mor on tau hyperphosphorylation suggests that Mor directly activates the PP2A protein. Additionally, the ratio of PME-1 (a methylesterase) to LCMT-1 (a leucine carboxyl methyltransferase) was significantly decreased by Mor, which inhibited PP2Ac demethylation. Mor could decrease the phosphorylation of Src, a membrane-associated protein tyrosine kinase that promotes the phosphorylation of PP2Ac at Tyr307 (Yang et al., 2016). Mor also reduced tau protein hyperphosphorylation by activating PP2A or decreasing GSK-3β.
The activation of GLP-1 receptors (GLP-1R) plays protective roles against multiple neurodegenerative disorders, including Alzheimer’s disease and Parkinson’s disease, as well as diabetes mellitus and ischemia (Grieco et al., 2019). In H2O2-induced microglia N9 and HEK293 cells, the protective effects of Mor were antagonized by exendin(9–39), a selective GLP-1 receptor antagonist, suggesting that Mor acts as a GLP-1R agonist (Meng et al., 2017). In the neuropathic pain rat model established by ligating the L5/L6 spinal nerves, Mor elevated the tolerance of the paw to electronic von Frey filaments and radiant heat by activating IL-10 and β-endorphin, which was blocked by IL-10 and β-endorphin antibodies (Tang et al., 2020). Therefore, Mor exerts therapeutic effects in neuropathy via the spinal microglial expression of IL-10 and β-endorphin after GLP-1 activation.
3.1.4 Other neuroprotective effects of Mor
In H2O2-induced SH-SY5Y cells (a subline of human neuroblastoma cells), Mor increased GSH, SOD, and mitochondrial membrane potential and reduced reactive oxygen species (ROS), nitrite content, lipid peroxidation, and intracellular accumulation of Ca2+. Moreover, Mor upregulated Bcl-2 and decreased caspase-3, caspase-9, and Bax (Wang et al., 2008; Wang et al., 2009; Zhang et al., 2017). In rat pheochromocytoma cells (PC12 cells), which are used to study neurotoxic activity (Wiatrak et al., 2020), Mor upregulated Bcl-2, downregulated Bax, cytochrome C, and cleaved caspase-3, alleviated cell death, and decreased the phosphorylation of JNK and p38MAPK simultaneously (Chen et al., 2018). The results demonstrated that Mor inhibits the activation of apoptosis, inflammatory, and oxidative stress to protect nerve cells.
In depression model rats established by forcibly disrupting their regular routine, Mor improved the behavioral test score in open-field and sugar water preference experiments, reduced pathological damage to the prefrontal cortex, and decreased levels of IL-1β, TNF-α, and NF-κBp65, implying that Mor exerts neuroprotective effects by reducing neuroinflammatory (Mo, 2020).
Mor demonstrates the ability to protect neurons and decelerate the progression of Parkinson’s disease (PD). In the 1-methyl-4-phenyl-1,2,3,6-tetrahydropyridine (MPTP)-induced PD model, Mor activates the Nrf2/HO-1 signaling pathway, leading to the decrease of MDA, ROS production, lipid peroxidation, and mitochondrial damage and the increase of GSH and GPX4, to prevent ferroptosis caused by lipid oxidation (Li M. et al., 2023).
3.2 Effects of Mor on bone protection
In TCM, the kidney is believed to influence bone, and CF’s function in nourishing the kidney is believed to have a miraculous effect on strengthening bones. Bone-related diseases can be classified into osteoporosis and osteoarthritis; the former is related to bone metabolism (Lane, 2006), while the latter is primarily associated with cartilage (Glyn-Jones et al., 2015). This section discusses the protective effects of Mor in osteoporosis and osteoarthritis, the detailed pharmacological effects was listed in Table 4, and the detailed mechanism of action can be seen in Figures 4, 5.
3.2.1 Effects of Mor on osteoporosis
Osteoporosis typically occurs in elderly and menopausal women, whereas idiopathic osteoporosis primarily occurs in adolescents. CF and Liu Wei Di Huang Wan are commonly used to treat postmenopausal osteoporosis (Gong et al., 2019; Kim et al., 2022).
Mouse embryo osteoblast precursor cells (MC3T3-E1 cells) can differentiate into osteoblasts and osteocytes, and they co-regulate bone formation and dissolution (Xu et al., 2020). Mor itself exerts no effects on the proliferation of MC3T3-E1 cells (Li et al., 2010); however, among 14 Mor derivatives, the introduction of a methyl group at position 7 and substitution with the beta configuration proved to be better than the alpha configuration (Han et al., 2018).
Mor can promote bone formation by increasing bone formation markers (ALP, Col-I, and osteocalcin) in MC3T3-E1 cells. Mor also upregulates Bcl-2 and downregulates caspase-3, caspase-9, and RANKL (Li et al., 2010). PI3K and mTOR inhibitors reversed MC3T3-E1 cell differentiation to mature osteoblasts, and mTOR overexpression can enhance osteoblast differentiation suppressed by PI3K inhibitors (Liu et al., 2021a). Hence, the anti-osteoporosis mechanism of Mor may be associated with apoptosis and autophagy. Additionally, osteoblast formation enhanced by Mor was also reversed by PI3K or mTOR inhibitors (Liu et al., 2021a). Further studies have found that Mor can promote mTOR activity and autophagy; Beclin1 or Atg13 agents, which enhance autophagy, may help improve protein levels in MC3T3-E1 cells. Moreover, Mor enhanced Atg13 expression, and mTOR overexpression reversed the expression of Beclin1. TAT-Beclin1 (inducers of autophagy) amplified the positive effect of Mor on bone parameters, such as the trabecular area and OCN expression in OVX mice (Li et al., 2022).
In ovariectomy-induced mice, the micro-CT images indicated that Mor reversed bone mineral density decline and structural compartment loss (Lee et al., 2021).
Mor shortened the adherence time of bone marrow stromal cells (BMSCs) and accelerated the formation of typical BMSC colonies to promote bone formation (Hu et al., 2013). High glucose causes the dysfunction of bone formation in type 1 diabetes mellitus. In high glucose-induced BMSCs, Mor reversed the osteogenic differentiation of BMSCs and increased the expression of osteospecific genes (Alp, Bmp2, Col-I, Opn, Ocn, and Runx2). In addition, Mor suppressed advanced glycation end product (AGE) formation and receptor for advanced glycation end product (RAGE) expression by triggering Glo1, whereas the Glo1 inhibitor (BBGCP2) partially reversed the suppressive effect of Mor on AGE-RAGE signaling (Uribarri et al., 2007).
In the type 1 diabetic rat model, Mor improved the mass and microarchitecture of the distal femur trabecular bone, as well as the bone volume/total volume, trabecular number, trabecular thickness, cortical bone area, and cortical thickness in the micro-CT image. Moreover, AGE-RAGE, Glo1, and osteo-related proteins, including RUNX2, Glo1, and Ocn in rat distal femurs, were all regulated by Mor. Therefore, Mor attenuated HG-mediated BMSC dysfunction partly by inhibiting AGE-RAGE signaling and activating Glo1 (Sun et al., 2020).
Mor attenuated chronic inflammation-triggered bone loss in mouse models by enhancing bone density and bone microstructure and inhibiting the expression of IL6, IL1β, and ALP. Moreover, in BMSCs, Mor downregulated IL-6 and IL-1β and upregulated the osteogenic mediators Runx2 and OCN. These effects were attributed to its inhibition of TRAF6-mediated NF-κB and MAPK signaling pathways (Xiao et al., 2023).
In the glucocorticoid-induced osteoporosis zebrafish model, MOR improved vertebral loss and increased the expression of osteoblastogenesis factors such as ALP, Runx2, and Col-I. Additionally, sodium-glucose cotransporter 2 (SGLT2) played a vital role in the anti-osteoporosis of Mor (Yang et al., 2023).
3.2.2 Effects of Mor on osteoarthritis
Osteoarthritis affects the elderly population, physical activity, and metabolic syndrome. The leading causes of osteoarthritis are degenerative lesions and persistently low levels of joint inflammation and matrix degradation (Abramoff and Caldera, 2020).
Chondrocytes are directly responsible for cartilage formation, metabolism, and repair (Chen H. et al., 2021). Mor could enhance chondrocyte viability and promote matrix synthesis by upregulating the expression of PCNA (responsible for proliferative activity), Col-II (the foundation of cartilage), and aggrecan (the key proteoglycan in articular cartilage). Furthermore, Mor activated Akt and Erk (Cheng et al., 2015).
In IL-1β-induced chondrocytes, Mor inhibited inflammation by downregulating Cox-2, MMP3, and MMP13. PGE2 and collagenase were reduced following treatment with Mor (Park E. et al., 2021). In another study, Mor inhibited apoptosis by reducing cleaved caspase-3 expression and suppressed pyroptosis by decreasing cleaved caspase-1, NLRP3, and GSDMD expression; in addition, Mor stimulated cartilage matrix synthesis by regulating Col-II and MMP13 (Yu et al., 2021). Studies have verified the protective effects of Mor by inhibiting autophagy in chondrocytes via the PI3K/mTOR pathway. PI3K and mTOR inhibitors significantly reversed the autophagy suppressed by Mor but did not affect the protective role of Mor in chondrocytes (Xiao et al., 2020).
Medial meniscus (DMM)-damaged mice are established by opening the medial joint capsule of the knee and transecting the medial meniscus after opening the medial meniscotibial ligament (Glasson et al., 2007). In this model, intra-articular injection of Mor elevated the level of proteoglycans in the cartilage matrix and ameliorated the cartilage damage. In addition, Mor increased Akt and Erk expression (Cheng et al., 2015) and inhibited sclerosis and cartilage degradation by reducing inflammatory mediators, such as Cox-2, MMP3, and MMP13 (Park E. et al., 2021).
Micro-CT imaging has shown that Mor attenuated osteoarthritis progression and stimulated cartilage matrix synthesis by increasing Col-II and decreasing MMP13. Mor also reduced p-IκBα and reversed the translocation of p65 into the nucleus; Mor might inhibit chondrocyte pyroptosis and apoptosis by inhibiting NF-κB signaling from preventing cartilage matrix degradation (Yu et al., 2021).
The prolonged use of glucocorticoids induces osteonecrosis of the femoral head (GIONFH). In dexamethasone-induced dysfunction in stem and endothelial cells and GIONFH rats, Mor mitigated stem and endothelial cell dysfunction through the PI3K/AKT and Bax/Bcl-2/Caspase3 signaling pathway, suggesting that Mor may be a potential agent for GIONFH (Jiang et al., 2024).
Intervertebral disc (IVD) degeneration (IVDD) is a leading cause of chronic low back pain and disability. Nucleus pulposus (NP) cell senescence is closely related to IVDD. In lumbar spine instability surgery-induced mice and H2O2-induced NP cells, Mor has been found to reduce SA-β-gal activities and the expression of p53 and p21, which are indicators of senescence. Mor suppressed the activation of Hippo signaling by inhibiting p-Mst1/2 and p-Lats1/2 and increasing Yap/Taz. In the mouse IVDD model, the inhibition of Hippo signaling by Mor was further confirmed. Mor protected against NP cell senescence to alleviate IVDD progression by inhibiting the ROS-Hippo-p53 pathway (Zhou et al., 2022).
3.3 Renoprotective effects of Mor
Along with the main indications of CF, Mor also has a therapeutic effect on diabetes, which is referred to as Xiao-Ke in TCM. The detailed mechanism of action of the rephroprotective action is shown in Figure 6 with the detailed pharmacological effects was listed in Table 5. With the intervention of Mor, human umbilical vein endothelial cell (HUVEC) survival was recovered from high ambient glucose, the number of cells in the S-phase was increased, and morphological damage was alleviated, suggesting that Mor can inhibit diabetic angiopathies (Xu et al., 2004). In the AGE-induced rat renal mesangial cell model, Mor improved cell morphology, inhibited proliferation, and reduced oxidative stress by regulating ROS, SOD, and GSH (Xu et al., 2006). Moreover, Mor recovered the morphological damage and cellular ultrastructure in AGE-induced rat glomerular mesangial cells and inhibited RAGE, p38MAPK, NF-κB, and TGF-β expression (Lv et al., 2016).
In streptozotocin-induced diabetes mellitus rats, Mor inhibited hyperglycemia by decreasing serum glucose and urinary protein, increasing serum albumin and total protein, and reducing serum urea nitrogen, creatinine clearance, serum glycosylated protein, and serum and renal thiobarbituric acid reactive substances. Moreover, the expressions of AGEs and AGER decreased in diabetic rats after Mor administration (Yokozawa et al., 2008).
Db/db mice have gene-encoding mutations at the leptin receptor, with high susceptibility to obesity and type 2 diabetes mellitus, and hence are suitable for the study of type 2 diabetes mellitus and metabolic liver and kidney disease (Suriano et al., 2021). In db/db mice, Mor decreased the overproduced glucose, triglyceride, and cholesterol and the expression of SREBP-1, SREBP-2, ROS, and TBARS (a by-product of lipid peroxidation) and increased GSH/GSSG. Moreover, Mor reduced NF-κB, Cox-2, and iNOS. Therefore, Mor inhibits metabolic disorders (hyperglycemia and dyslipidemia), oxidative stress, and inflammation in diabetic kidneys (Park et al., 2010; Yokozawa et al., 2010).
Podocyte, a type of glomerular visceral epithelial cell, play a critical role in filtration and are involved in glomerulopathies induced by diabetic nephropathy; in H2O2-injured podocytes, Mor reduced apoptosis by downregulating Bax and decreasing the Bax/Bcl-2 ratio. Furthermore, Mor inhibited autophagy by increasing LC3-II expression and reducing p62, mTOR, and NOX4. Additionally, Mor restrained the apoptosis by suppressing caspase-3 and cleaved caspase-3 (Gao et al., 2020).
In mouse renal tubular epithelial cells (mRTECs) damaged by sodium palmitate or HG, Mor reduced the accumulation of lipids and cholesterol by upregulating PGC-1α, LXR, ABCA1, ABCG1, and ApoE. This suggests that Mor promotes cholesterol efflux in mRTECs via the PGC-1α/LXR pathway (Gao et al., 2021).
In KKAy mice, which is an ideal animal model for early-to-mid-stage type 2 diabetic nephropathy, Mor normalized renal lipid metabolism by improving podocyte cholesterol efflux and regulating podocyte cholesterol uptake through upregulating the PGC-1α/LXRs/ABCA1 and PGC-1α/PPARγ/CD36 signaling pathways, respectively. In podocyte cells induced with PA or HG, Mor alleviated lipid accumulation through the exact mechanism (Yu et al., 2024).
Mor reduces lipid deposition in diabetic nephropathy by inhibiting the NF-κB/TNF-α/SREBP1c signaling pathway. In KKAy mice and PA-stimulated HK-2 cells, Mor inhibited the activation of NF-κBp65, reduced the levels of TNF-α and SREBP1c, lowered the production of lipid components such as ACC, FAS, and SCD1, and ultimately reduced renal lipid accumulation (Zhu et al., 2023).
3.4 Hepatoprotective effects of Mor
In traditional Chinese medicine theory, CF is considered a liver-nourishing tonic. Modern clinical trials have also confirmed its hepatoprotective effect (Sangsefidi et al., 2021; Bayram et al., 2024). There are also an increasing number of studies on the hepatoprotective effects of Mor, and the detailed mechanism of hepatoprotective action is shown in Figure 7, with the detailed pharmacological effects was listed in Table 6.
Diabetes damages the structure and function of the liver, and in turn, liver injury impairs glucose tolerance and causes diabetes. Using db/db mice, Mor has been shown to reduce the levels of glucose, triglyceride, and total cholesterol; regulate the activity of ROS, GSH, and GSSG (Park et al., 2009); and inhibit the expression of NF-κB, Cox-2, iNOS, and SREBP-1 and -2 (promotors of the expression of genes related to fatty acid synthase and cholesterol (Brown and Goldstein, 1997) but increase the expression of PPARa, which is related to the anti-obesity and the regulatory enzymes of fatty acid oxidation (Lin et al., 2022). Mor decreased the levels of glucose, ALT, and AST in serum, as well as ROS and lipid peroxidation in the liver of db/db mice, and significantly reduced the expression of oxidative stress-related proteins, such as NADPH oxidase subunits (Nox-4 and p22phox), Nrf2, HO-1, NF-κB, Cox-2, and iNOS, inflammation-related proteins (MCP-1 and ICAM-1) and apoptosis-related proteins (Bax and cytochrome C) (Park et al., 2011). Mor can regulate lipid metabolism, inflammation, and oxidative stress in the liver to improve diabetes.
In triptolide-induced liver injury, Mor reduced the levels of ALT and AST. In vitro, Mor reversed the growth inhibition, morphological changes, apoptosis, and nucleus deformation of HepG2 cells it also restored the expression of Nrf2 and HO-1, indicating that Mor improved triptolide-induced liver injury by preventing or alleviating oxidative stress (Zhou Y. et al., 2018).
In CCl4 and HFD-induced liver fibrosis mice, Mor reduced α-SMA, collagen, and GATA expressions by targeting GATA3 and LAL, thereby inhibiting HSC activation (An et al., 2022). In the NASH mouse model established by a high-fat and high-fructose diet, Mor attenuated hepatic lipid metabolism disorders and inhibited NLRP3 inflammasome activation by promoting AMPKα phosphorylation-mediated lipophagy and fatty acid oxidation. The consistent results performed in PA-treated cell models suggest that Mor may be a potential agent to inhibit NASH progression by promoting lipophagy and inhibiting inflammasome activation (Zhang et al., 2024).
Insulin resistance is another factor involved in diabetes. In HepG2 cells, Mor promoted glucose uptake, as well as in the HepG2 cell insulin resistance model. The results indicate that Mor can lower blood sugar by improving insulin resistance to alleviate type 2 diabetes-associated symptoms (Huang et al., 2017).
3.5 Cardiovascular protective effects of Mor
Acute myocardial infarction remains a major cause of mortalityworldwide despite substantial improvements in prognosis (Reed et al., 2017). The specific pharmacological effects of MOR in cardiovascular protective function are shown in Table 7. In STZ combined with high-fat diet-induced T2DM mice, Mor reduced TC and increased SOD and NOS (Feng et al., 2016), prevented HG-induced rat cardiomyocyte injury, and reduced apoptosis by modulating Bax and Bcl-2 and decreasing caspase-3 (Pi et al., 2017). In STZ combined with MACO mice, Mor improved heart function, reduced Mb, CK-MB, and cTNⅠ, regulated inflammation and oxidative stress by reducing IL-6, TNF-α, and MDA and enhancing IL-10 and SOD, and increased the expression of AMPKα1, PGC-1α, and GLUT4 (Li et al., 2018).
Morroniside can prevent myocardial injury due to ischemia and hypoxia. In an acute myocardial infarction (AMI) rat model induced by ligating the anterior descending coronary artery, Mor exhibited cardioprotective effects by improving cardiac function and decreasing CK-MB, LDH, ɑ-HBDH, AST, and cTnT; this protection may be related to anti-inflammatory activity. Mor decreased IL-6, IL-1β, and TNF-α, NF-κB (Yu and Wang, 2018; Yu et al., 2018) but increased IL-4 and IL-10 (Zhou et al., 2019). Moreover, Mor reduced apoptosis by regulating Bax and Bcl-2 (Bai et al., 2017) and caspase-3, caspase-8, and caspase-9 (Cui et al., 2018; Zhou et al., 2019) and alleviated oxidative stress by increasing SOD, GSH, Nrf2, and HO-1 while lowering MDA (Zhou et al., 2019). Another study found that Mor relieved inflammatory factors such as IL-8, IL-17, and hs-CRP and attenuated myocardial fibrosis by the downregulation of Smad3, Smad2, and TGF-β1 (Chen Z. et al., 2021). In H9c2 cells, Mor can inhibit cardiomyocyte apoptosis and autophagic death caused by hypoxia by inhibiting p-JNK and p-BCL2 at Ser70 and Ser87 and dissociation of BCL2–Beclin1 and BCL2–Bax complexes. In addition, Mor ameliorated the reduction of mitochondrial membrane potential (Ke et al., 2023). Morroniside could enhance cardiomyocyte cell cycle activity by upgrading cell cycle proteins (cyclin D1, CDK4, cyclin A2, and cyclin B1) in AMI rats (Zheng et al., 2023).
In the infarct border zone of AMI rats, Mor improved the level of Ang-1 and FGF-2, indicating that Mor promoted angiogenesis (Wang et al., 2019). Mor could also increase the newly generated endothelial cells and arterioles simulated by vascular endothelial growth factor A (VEGFA), an inducer and regulator of angiogenesis, and vascular endothelial growth factor receptor 2 (VEGFR2). The results were verified in rat coronary artery endothelial cells deprived of oxygen and glucose (Liu et al., 2020).
In adenosine diphosphate-induced rabbits, Mor has anti-coagulant properties in adenosine diphosphate, arachidonic acid, and platelet, activating factors that induce platelet aggregation (Wang X. et al., 2010). Mor also inhibited the increase in platelet Ca2+ (Ai et al., 2012) and decreased cyclooxygenase (Sun et al., 2012) and thromboxane B2 (Zuo et al., 2012) levels. In MACO rats, Mor reduced platelet aggregation (Cheng et al., 2013), whole blood and plasma viscosity (Pan et al., 2013), fibrinogen and prothrombin time and activated partial thromboplastin time, thrombin time (Yuan et al., 2013), and hematocrit percentage (Ai et al., 2014). These results suggest that Mor can improve cardiovascular and cerebrovascular diseases by altering the function of blood clotting.
3.6 Protective effect of Mor on the digestive system
In rats with chronic atrophic gastritis, Mor increased levels of gastrin and decreased levels of motilin. Mor also reduced inflammation by lowering TNF-α, IL-6, and IL-1β. In gastric mucosal cells, Mor decreased the expression of Bax, cleaved caspase-3, cleaved caspase-9, p-NF-κBp65, and p-IKKα/β and increased the levels of Bcl-2 and IκB-α. These results suggest that Mor has the potential to combat chronic atrophic gastritis by inhibiting inflammation and apoptosis (Zhang and Wang, 2019).
The protective effects of Mor in colitis are based on its anti-inflammatory activity. In acute colitis mice stimulated by the injection of dextran sodium sulfate, Mor reduced inflammation by decreasing IL-1β, IL-6, and TNF-α expression, improved epithelial defects, and repaired the intestinal mucosal barrier by increasing tight junction proteins (ZO-1, claudin-3, occludins, E-cadherin, and Muc2); additionally, Mor downregulated the overexpression of p-STAT3 and p-p65. In LPS-induced HCT116 cells (a type of human colon cancer cell) and HIEC-6 cells (an epithelial cell from the small intestine), the pro-inflammatory cytokines (IL-1β, IL-6, TNF-α, and IFN-γ) were decreased in the Mor-treated group (Yuan et al., 2020). In the inflammatory bowel disease (IBD) induced by LPS in human colon mucosal epithelial cells (NCM460), Mor reduced apoptosis, inflammation, and oxidative stress by regulating Bax/Bcl-2, inhibiting TNF-α, IL-1β, IL-6, SOD, MDA, T-AOC, and MPO levels. Additionally, Mor suppressed NLRP3 levels and NF-κB pathway activation (Zhang S. et al., 2022).
3.7 Protective effect of Mor on the integumentary system
Mor can regulate the hair anagen phase and protect hair follicles to prevent hair loss. In outer root sheath cells, Mor accelerated proliferation by expanding the S and G2 phases and increasing migration, which was partly reversed by DKK1, a Wnt/β-catenin signaling inhibitor. In addition, Mor increased the level of Wnt10b, β-catenin, and lef1. In vivo, Mor injection accelerated the onset of anagen and delayed catagen by increasing the diameter of the hair bulbs and skin thickness. In addition, the level of β-catenin was increased in hair follicles (Zhou L. et al., 2018).
Mor can promote wound healing and improve graft healing of the skin. Mor increased the ischemic skin flap survival in rats and elevated flap perfusion by 200%; these effects are related to promoting neovascularization by increasing VEGF and reducing ROS by regulating SOD and MDA (Lin et al., 2020). Mor facilitates the expedited healing of skin wounds by stimulating the proliferation of human epidermal stem cells (EpSCs) and the re-epithelialization of wounds in mice. This is achieved by upregulating β-catenin via GLP-1R-mediated PKA, PKA/PI3K/AKT, and PKA/ERK signaling pathways in EpSCs. Consequently, heightened β-catenin transcriptional activity triggers the transcription of c-Myc, cyclin D1, and cyclin E1, thereby inducing the proliferation of EpSCs (Yu et al., 2024).
In addition, Mor decreased melanin synthesis in human malignant melanoma cells (A375) and keratinocyte co-culture system by inhibiting tyrosinase activity (Yu and Chen, 2013), inhibited A375 proliferation, and promoted apoptosis by downregulating cyclin D1 and Bcl-2 and upregulating P21 and Bax (Li et al., 2017).
3.8 Other pharmacological activities of Mor
Other pharmacological effects of Mor are clearly shown in Table 8. In a previous study, CF extract inhibited oxidative stress in LPS-induced RAW 264.7 cells (Quah et al., 2020), Mor inhibited the polygonal spindle-shaped pseudopodia and phagocytosis, prevented LPS binding to Toll-like receptor 4 (TLR4), suppressed myeloid differentiation factor 88 (Myd88), reversed the accumulation of NF-κBp65 and degradation of IκB-α, and inhibited pro-inflammatory mediators and cytokines (NO, PGE2, TNF-α, and IL-1β). In addition, Mor inhibited ROS generation by enhancing the expression of Nrf2 and HO-1. Consequently, Mor exerts anti-inflammatory and antioxidant effects by targeting the TLR4/NF-κB and Nrf2/HO-1 signaling pathways (Park C. et al., 2021). In another study, five derivatives of Mor were evaluated in TNF-a-stimulated HUVECs; 7-O-dodecyl morroniside downregulated the expression of E-selectin (Takeda et al., 2010). Moreover, Mor exhibited allergy-preventive effects in mice induced by hen-egg white lysozyme, and the mechanism involves its C-8 position, which possesses the sp3 atom, in comparison to compounds ith similar structure (Oku et al., 2011).
Mor has a positive protective effect on the lungs, including acute lung injury, pulmonary fibrosis, and lung cancer. Mor decreased IL-6, IL-1β, and TNF-α levels in LPS-stimulated rat lung cell lines (E-6TN) and RAW 264.7 cells, suggesting its potential to alleviate lung inflammation. Additionally, in mice with LPS-induced acute lung injury, Mor relieved pulmonary edema, oxidative stress, and inflammatory factors such as IL-6, IL-1β, and TNF-α. In mice with bleomycin-induced pulmonary fibrosis, Mor reduced collagen deposition and hydroxyproline accumulation by increasing the CD4+/CD8+ ratio and decreasing the levels of TGF-β1, α-SMA, and type I collagen (Chen et al., 2022). Additionally, Mor has different effects on normal lung cells (human embryonic lung fibroblast, HELF) and lung cancer cells (A549), only in H2O2-induced HELF Mor promoted cell proliferation, improved cell morphology, inhibited apoptosis, and reduced retinoblastoma protein content, indicating that Mor is a potential ameliorative agent in lung cancer (Chen et al., 2014).
Mor has been found to have an anti-obesity effect. It decreases the expression of genes associated with the formation of fat cells (Pparg, Cebpa, Fabp4, Plin2, Fasn, and Srebp1) in 3T3-L1 cells and ADSCs, which prevents the differentiation of fat cells and the accumulation of fat droplets. This results in lower levels of fat in the cells and a higher release of glycerol. In animal models of obesity (ovary removal (OVX) and HFD-induced obesity), Mor reduced the rate of weight gain and the presence of fat vacuoles and fatty tissue areas (Oh et al., 2024).
Mor has the effect of reducing inflammation in the eye. The human iris pigment epithelium (IPE) plays a vital role in the iris structure. Mor has demonstrated cell proliferation stimulation, apoptosis inhibition, and reduction of levels of inflammatory cytokines such as TNF-α, IL-6, and IL-8 in LPS-IPE cells. In addition, Mor has been observed to suppress the activation of the TLR4/JAK2/STAT3 signaling pathway (Li W. J. et al., 2023). In endotoxin-induced uveitis mice, Mor reduced inflammation by decreasing IL-1β, IL-6, and TNF-α and inhibiting the expression of iNOS, p-JAK2, and p-STAT3 while promoting the expression of Arg-1. This suggests Mor can prevent uveitis inflammation by inhibiting the JAK/STAT pathway to promote M2 polarization (Li W. et al., 2023).
Researchers have also found that Mor regulates and protects follicular development. In H2O2-induced granulosa cells, Mor reversed survival inhibition and regulated intracellular oxidative stress by decreasing ROS and MDA and increasing SOD, GSH, and CAT. Additionally, Mor inhibited apoptosis by downregulating caspase-3 and autophagy by decreasing LC3-II/LC3-I and beclin-1 levels while increasing p62. Moreover, Mor could activate the PI3K/AKT/mTOR pathway, confirmed by LY294002 and rapamycin (PI3K and mTOR inhibitors) (Deng et al., 2021). In another study, Mor improved the quality of oocytes by reducing oxidative stress in H2O2-induced ovarian granulosa cells (GCs). Mor decreased the levels of ROS, MDA, and 8-OHdG, upregulated p-Nrf2, and promoted the nuclear translocation of Nrf2, which in turn activated the antioxidants SOD and NQO1. Additionally, Mor reduced apoptosis by regulating Bax, Bcl-2, cleaved caspase-9, and cleaved caspase-3 through the p38 and JNK pathways (Ma et al., 2022).
4 Conclusion
According to the traditional medicinal use of CF, Mor also demonstrates similar pharmacological effects, and more of its effects have been identified and developed further. The protective effects primarily researched include those on neurodegenerative diseases, focal cerebral ischemia, spinal cord injury, and Alzheimer’s disease. Mor reduces oxidative stress, reduces inflammation, regulates apoptosis, and plays a neuroprotective role primarily via the Nrf2/HO-1 signaling, Bax/Bcl-2, cytochrome C, caspase-3/9, MMP2/3/9, and NF-κB pathways. Mor protects focal cerebral ischemia by promoting angiogenesis and neurogenesis; the former is via Src/Erk, Ang-1/VEGFR signaling and later restrains programmed cell death, oxidative stress, and apoptosis via the Nrf2/HO-1, caspase-3/8/9, and Wnt/β-catenin signaling. Mor prevents Alzheimer’s disease by reducing tau phosphorylation via PI3K/Akt, calpain/GSK-3β, and Src/PP2Ac pathways. In terms of bone and joint protection, the mechanisms of the protective effects on osteoporosis or osteoarticular disease involve regulation of the signaling of caspase-3/9, Bax/Bcl-2, PI3K/Akt/mTOR, Glo1-AGE-RAGE, AKT/Erk, and NF-κB signaling. In addition, the protective effects on the liver and kidney are mediated by regulating lipid metabolism, inflammation, apoptosis, autophagy, and oxidative stress via the AGE/AGER, LXR/ApoE/ABCA1, mTOR, Bax/Bcl-2, caspase-3/9, Nrf2/HO-1, and iNOS/Cox-2 pathways; Mor also promotes hair growth by increasing β-catenin and improves chronic atrophic gastritis and colitis by inhibiting inflammation and apoptosis.; Moreover, Mor increases flap survival, promotes wound healing, and promotes hair growth. Furthermore, Mor improves lung damage, inflammation in the eye, and follicular development. The consistency between the traditional effectiveness of CF and Mor suggests the importance of exploring the pharmacodynamic effects according to the theory of TCM. Additionally, the expansion of Mor’s pharmacodynamic effects indicates the need to broaden the scope and treatment directions within TCM.
5 Research limitations and perspectives
Mor is the representative iridoid glycoside in CF; due to its special structure, Mor cannot be chemically synthesized. However, it can be isolated and purified from CF, and the isolation and purification technology is relatively well-developed and straightforward. Given the rapid advancements in polymer materials and their utilization in traditional Chinese medicine chemistry, separating and purifying Mor will soon become simpler and more efficient. In addition, high-resolution mass spectrometry can be utilized to quickly investigate Mor’s plant resources, thereby supporting its development.
Notably, Mor’s structure modification demonstrates diverse pharmacodynamic outcomes. Therefore, conducting a qualitative structure–activity relationship study of Mor is essential for enhancing efficacy, minimizing toxicity, and generating valuable insights for further research.
The study of many pharmacological mechanisms of action of Mor requires more exposure, including its toxicological studies. The therapeutic potential of Mor was explored through existing research and the mechanisms underlying its pharmacological effects. However, the toxicological effect of Mor should be clarified, including chronic and acute toxicities, to guide its clinical use and development better. In addition, we need ongoing high-quality studies to expand the pharmacological effects of Mor, and more comprehensive clinical trials are needed to confirm the long-term efficacy of Mor in human diseases.
The clinical data for Mor are crucial for research as clinical trials are more effective in confirming the safety and efficacy of drugs than cell and animal experiments. Although Chinese herbal formulations such as the Liu Wei Di Huang pill, which is the main ingredient of CF, are commonly used in clinical practice in Asia, there needs to be more separate randomized, double-masked, controlled clinical trials for Mor. Therefore, it is essential to further improve the clinical data to promote its clinical application. Additionally, given the protective effect of Mor on multiple diseases and organs, CF may have more significant medicinal and edible value in future research and utilization.
Author contributions
PS: writing–original draft, conceptualization, data curation, formal analysis, investigation, methodology, project administration, resources, software, supervision, validation, and visualization. BZ: data curation, supervision, writing–review and editing, methodology, project administration, validation, and visualization. SZ: conceptualization, data curation, writing–review and editing, investigation, and methodology. QG: funding acquisition, supervision, writing–review and editing, conceptualization, and project administration.
Funding
The author(s) declare that financial support was received for the research, authorship, and/or publication of this article. This work was supported by the Natural Science Foundation Project of Shandong Province (ZR2021MH123), “National General Survey of Traditional Chinese Medicine Resources” special subsidy for public health services of traditional Chinese medicine in 2018; Key R&D plan of Shandong Province “Selection of new varieties of elderberry with high yield and high quality (2020LZGC0905);” Shandong Provincial Traditional Chinese Medicine Science and Technology Project “Study on the mechanism of Astragalus polysaccharide inhibiting the occurrence of liver fibrosis through PD-1 regulating NK cell function (2020Q004);” and Shandong Provincial Medical and Health Science and Technology Development Project “Mechanism of STAT3 inhibition of liver fibrosis by regulating PD-1*NK cells (202002070991)”.
Conflict of interest
The authors declare that the research was conducted in the absence of any commercial or financial relationships that could be construed as a potential conflict of interest.
Publisher’s note
All claims expressed in this article are solely those of the authors and do not necessarily represent those of their affiliated organizations, or those of the publisher, the editors, and the reviewers. Any product that may be evaluated in this article, or claim that may be made by its manufacturer, is not guaranteed or endorsed by the publisher.
Supplementary material
The Supplementary Material for this article can be found online at: https://www.frontiersin.org/articles/10.3389/fphar.2024.1423062/full#supplementary-material
References
Abramoff, B., and Caldera, F. E. (2020). Osteoarthritis: pathology, diagnosis, and treatment options. Med. Clin. North Am. 104 (2), 293–311. doi:10.1016/j.mcna.2019.10.007
Ahad, M. A., Kumaran, K. R., Ning, T., Mansor, N. I., Effendy, M. A., Damodaran, T., et al. (2020). Insights into the neuropathology of cerebral ischemia and its mechanisms. Rev. Neurosci. 31 (5), 521–538. doi:10.1515/revneuro-2019-0099
Ahn, S. J., Kim, H. J., Lee, A., Min, S. S., In, S., and Kim, E. (2020). Determination of 12 herbal compounds for estimating the presence of Angelica gigas root, Cornus fruit, licorice root, pueraria root, and Schisandra fruit in foods by LC-MS/MS. Food Addit. Contam. Part A Chem. Anal. Control Expo. Risk Assess. 37 (9), 1437–1448. doi:10.1080/19440049.2020.1778187
Ai, H., Sun, F., Wang, X., Zhang, Y., Zhang, L., Cheng, N., et al. (2014). Effect of morroniside on hematocrit percentage in rat of focal cerebral ischemia/reperfusion. Chin. J. Rehabilitation Theory Pract. 20 (03), 230–232. doi:10.3969/j.issn.1006-9771.2014.03.008
Ai, H., Zuo, W., Wang, X., and Wang, W. (2012). Morroniside inhibiting Ca2+ after platelet aggregation induced by adenosine diphosphate in rabbits. Chin. J. Rehabilitation Theory Pract. 18 (02), 131–133. doi:10.3969/j.issn.1006-9771.2012.02.011
An, L., Zhang, M., Lin, Y., Jiang, T., Xu, K., Xiao, S., et al. (2022). Morroniside, a novel GATA3 binding molecule, inhibits hepatic stellate cells activation by enhancing lysosomal acid lipase expression. Phytomedicine 103, 154199. doi:10.1016/j.phymed.2022.154199
Author Anonymous (2021). 2021 Alzheimer's disease facts and figures. Alzheimers Dement. 17 (3), 327–406. doi:10.1002/alz.12328
Bai, Y., Tang, S., Wu, L., Xia, H., and Shi, X. (2017). The protection and related mechanism of morroniside in rat model of myocardial ischemic reperfusion injury. Chin. J. General Pract. 15 (09), 1466–1468. doi:10.16766/j.cnki.issn.1674-4152.2017.09.003
Bayram, H. M., Iliaz, R., and Gunes, F. E. (2024). Effects of Cornus mas L. on anthropometric and biochemical parameters among metabolic associated fatty liver disease patients: randomized clinical trial. J. Ethnopharmacol. 318 (Pt B), 117068. doi:10.1016/j.jep.2023.117068
Bhakta, H. K., Park, C. H., Yokozawa, T., Min, B. S., Jung, H. A., and Choi, J. S. (2016). Kinetics and molecular docking studies of loganin, morroniside and 7-O-galloyl-D-sedoheptulose derived from Corni fructus as cholinesterase and β-secretase 1 inhibitors. Arch. Pharm. Res. 39 (6), 794–805. doi:10.1007/s12272-016-0745-5
Brown, M. S., and Goldstein, J. L. (1997). The SREBP pathway: regulation of cholesterol metabolism by proteolysis of a membrane-bound transcription factor. Cell. 89 (3), 331–340. doi:10.1016/s0092-8674(00)80213-5
Cai, H., Cao, G., and Cai, B. (2013). Rapid simultaneous identification and determination of the multiple compounds in crude Fructus Corni and its processed products by HPLC-MS/MS with multiple reaction monitoring mode. Pharm. Biol. 51 (3), 273–278. doi:10.3109/13880209.2012.720689
Cai, Z., Wang, C., Zou, L., Liu, X., Chen, J., Tan, M., et al. (2019). Comparison of multiple bioactive constituents in the flower and the caulis of Lonicera japonica based on UFLC-QTRAP-MS/MS combined with multivariate statistical analysis. Molecules 24 (10), 1936. doi:10.3390/molecules24101936
Chen, H., Tan, X. N., Hu, S., Liu, R. Q., Peng, L. H., Li, Y. M., et al. (2021a). Molecular mechanisms of chondrocyte proliferation and differentiation. Front. Cell. Dev. Biol. 9, 664168. doi:10.3389/fcell.2021.664168
Chen, J., Tan, X., Guo, G., Wang, P., Zhang, H., Lv, S., et al. (2024a). Cloning and expression analysis of key enzyme gene CoGPPS involved in iridoid glycoside synthesis in Cornus officinalis. DNA Cell. Biol. 43 (3), 125–131. doi:10.1089/dna.2023.0335
Chen, J., Xu, D., Yu, X., Zhao, F., Xu, D., Zhang, X., et al. (2014). Discrepancy between the effects of morronside on apoptosis in human embryonic lung fibroblast cells and lung cancer A549 cells. Oncol. Lett. 7 (4), 927–932. doi:10.3892/ol.2014.1850
Chen, K., Lu, Y., Liu, C., Zhang, L., Fang, Z., and Yu, G. (2018). Morroniside prevents H2O2 or Aβ1-42-induced apoptosis via attenuating JNK and p38 MAPK phosphorylation. Eur. J. Pharmacol. 834, 295–304. doi:10.1016/j.ejphar.2018.07.047
Chen, L., Ma, Q., Zhang, G., Lei, Y., Wang, W., Zhang, Y., et al. (2022). Protective effect and mechanism of loganin and morroniside on acute lung injury and pulmonary fibrosis. Phytomedicine 99, 154030. doi:10.1016/j.phymed.2022.154030
Chen, Y., Chen, M., Zhu, W., Zhang, Y., Liu, P., and Li, P. (2024b). Morroniside attenuates podocytes lipid deposition in diabetic nephropathy: a network pharmacology, molecular docking and experimental validation study. Int. Immunopharmacol. 138, 112560. doi:10.1016/j.intimp.2024.112560
Chen, Z., Shen, C., and Li, S. (2021b). Study on the improvement and mechanism of monod glycoside on myocardial fibrosis in rat model of myocardial infarction. J. Clin. Exp. Med. 20 (06), 584–588. doi:10.3969/j.issn.1671-4695.2021.06.007
Cheng, H., Sun, F., Sun, Z., Wang, X., Ai, H., Zhang, L., et al. (2013). Effect of morroniside on platelet aggregation in focal cerebral ischemia/reperfusion in rats. Chin. J. Rehabilitation Theory Pract. 19 (02), 101–103. doi:10.3969/j.issn.1006-9771.2013.02.001
Cheng, L., Zeng, G., Liu, Z., Zhang, B., Cui, X., Zhao, H., et al. (2015). Protein kinase B and extracellular signal-regulated kinase contribute to the chondroprotective effect of morroniside on osteoarthritis chondrocytes. J. Cell. Mol. Med. 19 (8), 1877–1886. doi:10.1111/jcmm.12559
Chinese Pharmacopoeia (2020). Pharmacopoeia of the people's Republic of China. Beijing: China Medico-Pharmaceutical Science and Technology Publishing House.
Cieniak, C., Walshe-Roussel, B., Liu, R., Muhammad, A., Saleem, A., Haddad, P. S., et al. (2015). Phytochemical comparison of the water and ethanol leaf extracts of the Cree medicinal plant, Sarracenia purpurea L. (Sarraceniaceae). J. Pharm. Pharm. Sci. 18 (4), 484–493. doi:10.18433/j35w27
Cui, J., Sun, F., Liu, T., Guo, D., Wang, W., and Shi, J. (2018). Effects of morroniside on oxidative stress and cardiomyocyte apoptosis in rats with acute myocardial infarction. J. Zunyi Med. Univ. 41 (02), 132–139. doi:10.14169/j.cnki.zunyixuebao.2018.0027
Deng, D., Yan, J., Wu, Y., Wu, K., and Li, W. (2021). Morroniside suppresses hydrogen peroxide-stimulated autophagy and apoptosis in rat ovarian granulosa cells through the PI3K/AKT/mTOR pathway. Hum. Exp. Toxicol. 40 (4), 577–586. doi:10.1177/0960327120960768
DeTure, M. A., and Dickson, D. W. (2019). The neuropathological diagnosis of Alzheimer's disease. Mol. Neurodegener. 14 (1), 32. doi:10.1186/s13024-019-0333-5
Du, K., Li, J., Bai, Y., An, M., Gao, X. M., and Chang, Y. X. (2018). A green ionic liquid-based vortex-forced MSPD method for the simultaneous determination of 5-HMF and iridoid glycosides from Fructus Corni by ultra-high performance liquid chromatography. Food Chem. 244, 190–196. doi:10.1016/j.foodchem.2017.10.057
Du, W., Cai, H., Wang, M., Ding, X., Yang, H., and Cai, B. (2008). Simultaneous determination of six active components in crude and processed Fructus Corni by high performance liquid chromatography. J. Pharm. Biomed. Anal. 48 (1), 194–197. doi:10.1016/j.jpba.2008.04.021
Duan, F., Shi, Y., Chen, J., Song, X., Shen, L., Qi, Q., et al. (2021). The neuroprotective role of morroniside against spinal cord injury in female rats. Neurochem. Int. 148, 105105. doi:10.1016/j.neuint.2021.105105
Feng, X., Zhao, W., Pi, W., and Cai, B. (2016). Protective effect of the active ingredient of dogwood on the myocardium of diabetic mice. Chin. J. Gerontology 36 (19), 4681–4682. doi:10.3969/j.issn.1005-9202.2016.19.004
Fu, Z., Zhang, Y., Jin, T., Wang, Z., Zhao, C., and Zhao, M. (2024). A comprehensive quality evaluation strategy of Shensong Yangxin capsules based on qualitative, fingerprint and quantitative analyses. Biomed. Chromatogr. 38 (4), e5832. doi:10.1002/bmc.5832
Gao, J., Liu, P., Shen, Z., Xu, K., Wu, C., Tian, F., et al. (2021). Morroniside promotes PGC-1α-mediated cholesterol efflux in sodium palmitate or high glucose-induced mouse renal tubular epithelial cells. Biomed. Res. Int. 2021, 9942152. doi:10.1155/2021/9942152
Gao, X., Liu, Y., Wang, L., Sai, N., Liu, Y., and Ni, j. (2020). Morroniside inhibits H2O2-induced podocyte apoptosis by down-regulating NOX4 expression controlled by autophagy in vitro. Front. Pharmacol. 11, 533809. doi:10.3389/fphar.2020.533809
Ge, J. R., Xie, L. H., Chen, J., Li, S. Q., Xu, H. J., Lai, Y. L., et al. (2018). Liuwei Dihuang Pill treats postmenopausal osteoporosis with shen (kidney) yin deficiency via anus kinase/signal transducer and activator of transcription signal pathway by up-regulating cardiotrophin-like cytokine factor 1 expression. Chin. J. Integr. Med. 24 (6), 415–422. doi:10.1007/s11655-016-2744-2
Girnar, G. A., and Mahajan, H. S. (2021). Cerebral ischemic stroke and different approaches for treatment of stroke. Future J. Pharm. Sci. 7 (1), 134. doi:10.1186/s43094-021-00289-1
Glasson, S. S., Blanchet, T. J., and Morris, E. A. (2007). The surgical destabilization of the medial meniscus (DMM) model of osteoarthritis in the 129/SvEv mouse. Osteoarthr. Cartil. 15 (9), 1061–1069. doi:10.1016/j.joca.2007.03.006
Glyn-Jones, S., Palmer, A. J., Agricola, R., Price, A. J., Vincent, T. L., Weinans, H., et al. (2015). Osteoarthritis. Lancet 386 (9991), 376–387. doi:10.1016/s0140-6736(14)60802-3
Gong, H., Li, S., Xie, C., Lei, J., Chen, Z., Duan, X., et al. (2015). Rapid determination of morroniside in fructus corni by near infrared spectroscopy combined with TQ software. Nat. Prod. Res. Dev. 27 (02), 277–281+293. doi:10.16333/j.1001-6880.2015.02.017
Gong, R., Ren, S., Chen, M., Wang, Y., Zhang, G., Shi, L., et al. (2019). Bioinformatics analysis reveals the altered gene expression of patients with postmenopausal osteoporosis using liuweidihuang pills treatment. Biomed. Res. Int. 2019, 1907906. doi:10.1155/2019/1907906
Grieco, M., Giorgi, A., Gentile, M. C., d'Erme, M., Morano, S., Maras, B., et al. (2019). Glucagon-like peptide-1: a focus on neurodegenerative diseases. Front. Neurosci. 13, 1112. doi:10.3389/fnins.2019.01112
Guo, K., Yang, C., and Zhang, L. (2022). The reduction of tau hyperphosphorylation by cornel iridoid glycosides is mediated by their influence on Calpain activity. Evid. Based Complement. Altern. Med. 2022, 9213046. doi:10.1155/2022/9213046
Guo, Y., Gu, Z., Liu, X., Liu, J., Ma, M., Chen, B., et al. (2017). Rapid analysis of corni fructus using paper spray-mass spectrometry. Phytochem. Anal. 28 (4), 344–350. doi:10.1002/pca.2681
Han, H., Li, Z., Qu, N., Chen, S., and Dong, P. (2018). Synthesis and promotion of the osteoblast proliferation effect of morroniside derivatives. Molecules 23 (6), 1412. doi:10.3390/molecules23061412
Herpich, F., and Rincon, F. (2020). Management of acute ischemic stroke. Crit. Care Med. 48 (11), 1654–1663. doi:10.1097/ccm.0000000000004597
Hu, N., Ren, S., Li, W., Zhang, T., and Zhao, C. (2013). Morroniside promotes bone marrow mesenchymal stem cell proliferation in rats. Mol. Med. Rep. 7 (5), 1565–1570. doi:10.3892/mmr.2013.1399
Huang, M., Ge, C., and Yu, S. (2017). Effects of loganin and morroniside extracted from Cornus officinalis on HepG2 cells and their insulin resistance model. Mod. Food Sci. Technol. 33 (04), 13–18. doi:10.13982/j.mfst.1673-9078.2017.4.003
Ji, W., Wang, R., Mu, Y., and Wang, X. (2018). Superhydrophilic molecularly imprinted polymers based on a single cross-linking monomer for the recognition of iridoid glycosides in Di-huang pills. Anal. Bioanal. Chem. 410 (25), 6539–6548. doi:10.1007/s00216-018-1257-6
Jiang, H., Wang, W., Mao, Y., Jiang, L., Yu, J., Zhu, X., et al. (2024). Morroniside-mediated mitigation of stem cell and endothelial cell dysfunction for the therapy of glucocorticoid-induced osteonecrosis of the femoral head. Int. Immunopharmacol. 127, 111421. doi:10.1016/j.intimp.2023.111421
Ke, X., Yu, S., Situ, S., Lin, Z., and Yuan, Y. (2023). Morroniside inhibits Beclin1-dependent autophagic death and Bax-dependent apoptosis in cardiomyocytes through repressing BCL2 phosphorylation. Vitro Cell. Dev. Biol. Anim. 59 (4), 277–288. doi:10.1007/s11626-023-00768-0
Khan, A., Ju, F., Xie, W., Tariq Hafeez, M., Cheng, X., Yang, Z., et al. (2017). Transcriptomic analysis reveals differential activation of microglial genes after ischemic stroke in mice. Neuroscience 348, 212–227. doi:10.1016/j.neuroscience.2017.02.019
Kim, J., Lee, C. G., Yun, S. H., Hwang, S., Jeon, H., Park, E., et al. (2022). Inhibitory effect of ulmus davidiana and Cornus officinalis extracts on osteoporotic bone loss in vitro and in vivo. Med. Kaunas. 58 (4), 466. doi:10.3390/medicina58040466
Lane, N. E. (2006). Epidemiology, etiology, and diagnosis of osteoporosis. Am. J. Obstet. Gynecol. 194 (2 Suppl. l), S3–S11. doi:10.1016/j.ajog.2005.08.047
Lee, C. G., Kim, J., Yun, S. H., Hwang, S., Jeon, H., Park, E., et al. (2021). Anti-osteoporotic effect of morroniside on osteoblast and osteoclast differentiation in vitro and ovariectomized mice in vivo. Int. J. Mol. Sci. 22 (19), 10642. doi:10.3390/ijms221910642
Lendl, A., Werner, I., Glasl, S., Kletter, C., Mucaji, P., Presser, A., et al. (2005). Phenolic and terpenoid compounds from Chione venosa (sw.) urban var. venosa (Bois Bandé). Phytochemistry 66 (19), 2381–2387. doi:10.1016/j.phytochem.2005.07.002
Li, F., Song, X., Xu, J., Shi, Y., Hu, R., Ren, Z., et al. (2021). Morroniside protects OLN-93 cells against H(2)O(2)-induced injury through the PI3K/Akt pathway-mediated antioxidative stress and antiapoptotic activities. Cell. Cycle 20 (7), 661–675. doi:10.1080/15384101.2021.1889186
Li, G., Xu, N., Liu, X., Wu, Z., Lv, S., and Kuang, H. (2015). Saponin constituents from roots and rhizmes of Caulophyllum robustum. Chin. Traditional Herb. Drugs 46 (10), 1431–1436. doi:10.7501/j.issn.0253-2670.2015.10.005
Li, M., Wang, W., Wang, P., Yang, K., Sun, H., and Wang, X. (2010). The pharmacological effects of morroniside and loganin isolated from Liuweidihuang Wan, on MC3T3-E1 cells. Molecules 15 (10), 7403–7414. doi:10.3390/molecules15107403
Li, M., Zhang, J., Jiang, L., Wang, W., Feng, X., Liu, M., et al. (2023a). Neuroprotective effects of morroniside from Cornus officinalis sieb. Et zucc against Parkinson's disease via inhibiting oxidative stress and ferroptosis. BMC Complement. Med. Ther. 23 (1), 218. doi:10.1186/s12906-023-03967-0
Li, N., Li, S., Zhang, N., Wang, J., and Chen, J. (2017). Proliferation and apoptosis regulatory mechanism of morroniside on human malignant melanoma cells (in Chinese). Lett. Biotechnol. 28 (02), 128–132. doi:10.3969/j.issn.1009-0002.2017.02.012
Li, W., Liu, L., Zhang, Z., and Lu, H. (2023b). Morroniside ameliorates endotoxin-induced uveitis by regulating the M1/M2 polarization balance of macrophages. J. Immunol. Res. 2023, 1252873. doi:10.1155/2023/1252873
Li, W. J., Liu, L., and Lu, H. (2023c). Morroniside ameliorates lipopolysaccharide-induced inflammatory damage in iris pigment epithelial cells through inhibition of TLR4/JAK2/STAT3 pathway. Int. J. Ophthalmol. 16 (12), 1928–1934. doi:10.18240/ijo.2023.12.03
Li, X., Huo, C., Yin, W., and Zhang, L. (2006). Preparation of loganin and morroniside reference substances from Fructus Corni. Chin. Traditional Herb. Drugs (08), 1168–1170.
Li, X., Zhang, T., and Feng, Y. (2018). Morroniside protects diabetic rat heart against ischemia-reperfusion injury via activating AMPKα/PGC-1α/GLUT4 pathway. Chin. J. Immunol. 34 (07), 990–995.
Li, X., Zhu, Y., Lin, X., Chen, C., Liu, H., and Shi, Y. (2022). Beclin1-and Atg13-dependent autophagy activation and morroniside have synergistic effect on osteoblastogenesis. Exp. Biol. Med. (Maywood) 247, 1764–1775. doi:10.1177/15353702221116879
Liang, J., He, J., Zhu, S., Zhao, W., Zhang, Y., Ito, Y., et al. (2011). Preparation of main iridoid glycosides in fructus corni by macroporous resin column chromatogaphy and countercurrent chromatography. Chin. J. Nat. Med. 9 (01), 26–29. doi:10.1080/10826076.2012.683914
Liang, J., He, J., Zhu, S., Zhao, W., Zhang, Y., Ito, Y., et al. (2013). Preparation of main iridoid glycosides in fructus corni by macroporous resin column chromatography and countercurrent chromatography. J. Liq. Chromatogr. Relat. Technol. 36 (8), 983–999. doi:10.1080/10826076.2012.683914
Lin, Y., Lin, B., and Lin, D. (2020). Effects of morroniside on the viability of random skin flaps in rats. J. Investig. Surg. 33 (2), 182–188. doi:10.1080/08941939.2018.1479007
Lin, Y., Wang, Y., and Li, P. F. (2022). PPARα: an emerging target of metabolic syndrome, neurodegenerative and cardiovascular diseases. Front. Endocrinol. (Lausanne) 13, 1074911. doi:10.3389/fendo.2022.1074911
Liu, F., Grundke-Iqbal, I., Iqbal, K., and Gong, C. X. (2005). Contributions of protein phosphatases PP1, PP2A, PP2B and PP5 to the regulation of tau phosphorylation. Eur. J. Neurosci. 22 (8), 1942–1950. doi:10.1111/j.1460-9568.2005.04391.x
Liu, H., Li, X., Lin, J., and Lin, M. (2021a). Morroniside promotes the osteogenesis by activating PI3K/Akt/mTOR signaling. Biosci. Biotechnol. Biochem. 85 (2), 332–339. doi:10.1093/bbb/zbaa010
Liu, H., Ou, M., and Han, Q. (2021b). Microglial M2 polarization mediated the neuroprotective effect of morroniside in transient MCAO-induced mice. Front. Pharmacol. 12, 784329. doi:10.3389/fphar.2021.784329
Liu, L., Sun, A., Wu, S., and Liu, R. (2009). Preparative purification of morroniside and loganin from Fructus corni by combination of macroporous absorption resin and HSCCC. J. Chromatogr. Sci. 47 (5), 333–336. doi:10.1093/chromsci/47.5.333
Liu, M., Xu, D., Sun, F., Liu, T., Li, Y., and Wang, W. (2019). Protective effects of morroniside in rats at 24 h after focal cerebral ischemia-reperfusion. Chin. J. Comp. Med. 29 (02), 19–24. doi:10.3969/j.issn.1671-7856.2019.02.004
Liu, T., Sun, F., Ai, H., Zhang, L., and Wang, W. (2013). Effects of morroniside on the expression of CyclinD1 and CDK6 in Hippocampus of rats treated with focal cerebral ischemia/reperfusion injury. Acta Neuropharmacol. 3 (04), 1–7.
Liu, T., Sun, F., Cui, J., Zheng, S., Li, Z., Guo, D., et al. (2020). Morroniside enhances angiogenesis and improves cardiac function following acute myocardial infarction in rats. Eur. J. Pharmacol. 872, 172954. doi:10.1016/j.ejphar.2020.172954
Liu, T., Xiang, B., Guo, D., Sun, F., Wei, R., Zhang, G., et al. (2016). Morroniside promotes angiogenesis and further improves microvascular circulation after focal cerebral ischemia/reperfusion. Brain Res. Bull. 127, 111–118. doi:10.1016/j.brainresbull.2016.09.004
Lv, G., Lv, X., Tao, Y., and Xu, H. (2016). Effect of morroniside on glomerular mesangial cells through AGE-RAGE pathway. Hum. Cell. 29 (4), 148–154. doi:10.1007/s13577-015-0128-0
Ma, Q., Shi, X., Fan, B., Shen, W., Liu, D., and Wang, X. (2015). Chemical constituents of n-butanol fraction from Patrinia scabra. Chin. Traditional Herb. Drugs 46 (11), 1593–1596. doi:10.7501/j.issn.0253-2670.2015.11.005
Ma, Y., Hao, G., Lin, X., Zhao, Z., Yang, A., Cao, Y., et al. (2022). Morroniside protects human granulosa cells against H(2)O(2)-induced oxidative damage by regulating the Nrf2 and MAPK signaling pathways. Evid. Based Complement. Altern. Med. 2022, 8099724. doi:10.1155/2022/8099724
Maituoheti, R., Rouzimaimaiti, R., Tang, D., Zhao, J., and Aisa, H. A. (2023). Seco-iridoid glycosides from the Gentiana olivieri Griseb and their bioactivities. Phytochemistry 215, 113839. doi:10.1016/j.phytochem.2023.113839
Meng, X., HaiYun, W., Hao, L., Nian, G., YiRui, W., and YongXiang, W. (2017). Morroniside, a secoiridoid glycoside from Cornus officinalis, attenuates neuropathic pain by activation of spinal glucagon-like peptide-1 receptors. Br. J. Pharmacol. 174 (7), 580–590. doi:10.1111/bph.13720
Mo, X. (2020). Efficacy of morroniside on depression-like behavior and neuroinflammation of depression model rats. Clin. J. Chin. Med. 12 (34), 19–21. doi:10.3969/j.issn.1674-7860.2020.34.006
Mohamed, S. M., Backheet, E. Y., Bayoumi, S. A., and Ross, S. A. (2016). New cycloartane saponin and monoterpenoid glucoindole alkaloids from Mussaenda luteola. Fitoterapia 110, 129–134. doi:10.1016/j.fitote.2016.03.009
Mu, L., Gao, J., Zhang, Q., Kong, F., Lian, Y., Li, X., et al. (2024). Mechanism of action of Sambucus williamsii Hance var. miquelii in the treatment of osteoporosis analyzed by UHPLC-HRMS/MS combined network pharmacology and experimental validation. Fitoterapia 176, 106003. doi:10.1016/j.fitote.2024.106003
Oh, K. I., Lim, E., Uprety, L. P., Jeong, J., Jeong, H., Park, E., et al. (2024). Anti-adipogenic and anti-obesity effects of morroniside in vitro and in vivo. Biomed. Pharmacother. 176, 116762. doi:10.1016/j.biopha.2024.116762
Oku, H., Ogawa, Y., Iwaoka, E., and Ishiguro, K. (2011). Allergy-preventive effects of chlorogenic acid and iridoid derivatives from flower buds of Lonicera japonica. Biol. Pharm. Bull. 34 (8), 1330–1333. doi:10.1248/bpb.34.1330
Pan, H., Sun, F., Wang, X., Wang, W., Ai, H., Zhang, L., et al. (2013). Effect of morroniside on blood viscosity in focal cerebral ischemia/reperfusion in rats. Chin. J. Rehabilitation Theory Pract. 19 (02), 107–109. doi:10.3969/j.issn.1006-9771.2013.02.003
Park, C., Cha, H. J., Lee, H., Kim, G. Y., and Choi, Y. H. (2021a). The regulation of the TLR4/NF-κB and Nrf2/HO-1 signaling pathways is involved in the inhibition of lipopolysaccharide-induced inflammation and oxidative reactions by morroniside in RAW 264.7 macrophages. Arch. Biochem. Biophys. 706, 108926. doi:10.1016/j.abb.2021.108926
Park, C. H., Noh, J. S., Kim, J. H., Tanaka, T., Zhao, Q., Matsumoto, K., et al. (2011). Evaluation of morroniside, iridoid glycoside from Corni Fructus, on diabetes-induced alterations such as oxidative stress, inflammation, and apoptosis in the liver of type 2 diabetic db/db mice. Biol. Pharm. Bull. 34 (10), 1559–1565. doi:10.1248/bpb.34.1559
Park, C. H., Noh, J. S., Tanaka, T., and Yokozawa, T. (2010). Effects of morroniside isolated from Corni Fructus on renal lipids and inflammation in type 2 diabetic mice. J. Pharm. Pharmacol. 62 (3), 374–380. doi:10.1211/jpp.62.03.0013
Park, C. H., Yamabe, N., Noh, J. S., Kang, K. S., Tanaka, T., and Yokozawa, T. (2009). The beneficial effects of morroniside on the inflammatory response and lipid metabolism in the liver of db/db mice. Biol. Pharm. Bull. 32 (10), 1734–1740. doi:10.1248/bpb.32.1734
Park, E., Lee, C. G., Han, S. J., Yun, S. H., Hwang, S., Jeon, H., et al. (2021b). Antiosteoarthritic effect of morroniside in chondrocyte inflammation and destabilization of medial meniscus-induced mouse model. Int. J. Mol. Sci. 22 (6), 2987. doi:10.3390/ijms22062987
Pi, W., Feng, X., Ye, L., and Cai, B. (2017). Combination of morroniside and diosgenin prevents high glucose-induced cardiomyocytes apoptosis. Molecules 22 (1), 163. doi:10.3390/molecules22010163
Quah, Y., Lee, S. J., Lee, E. B., Birhanu, B. T., Ali, M. S., Abbas, M. A., et al. (2020). Cornus officinalis ethanolic extract with potential anti-allergic, anti-inflammatory, and antioxidant activities. Nutrients 12 (11), 3317. doi:10.3390/nu12113317
Reed, G. W., Rossi, J. E., and Cannon, C. P. (2017). Acute myocardial infarction. Lancet 389 (10065), 197–210. doi:10.1016/s0140-6736(16)30677-8
Ruan, L., Wang, B., ZhuGe, Q., and Jin, K. (2015). Coupling of neurogenesis and angiogenesis after ischemic stroke. Brain Res. 1623, 166–173. doi:10.1016/j.brainres.2015.02.042
Sachdeva, R., Gao, F., Chan, C. C. H., and Krassioukov, A. V. (2018). Cognitive function after spinal cord injury: a systematic review. Neurology 91 (13), 611–621. doi:10.1212/wnl.0000000000006244
Sangsefidi, Z. S., Yarhosseini, F., Hosseinzadeh, M., Ranjbar, A., Akhondi-Meybodi, M., Fallahzadeh, H., et al. (2021). The effect of (Cornus mas L.) fruit extract on liver function among patients with nonalcoholic fatty liver: a double-blind randomized clinical trial. Phytother. Res. 35 (9), 5259–5268. doi:10.1002/ptr.7199
Shi, Y. J., Sheng, W. J., Xue, M. T., Duan, F. X., Shen, L., Ding, S. Q., et al. (2022). Effect of morroniside on the transcriptome profiles of rat in injured spinal cords. Gene 823, 146338. doi:10.1016/j.gene.2022.146338
Sun, F., Wang, W., Cheng, H., Wang, Y., Li, L., Xue, J., et al. (2014a). Morroniside improves microvascular functional integrity of the neurovascular unit after cerebral ischemia. PLoS One 9 (6), e101194. doi:10.1371/journal.pone.0101194
Sun, F., Wang, W., Liu, T., Wu, Z., and Guo, M. (2019). Mechanism of morroniside in regulating neural stem cell proliferation and differentiation in vitro. Acta Neuropharmacol. 9 (04), 32.
Sun, F., Wang, W., Zuo, W., Xue, J., Xu, J., Ai, H., et al. (2014b). Promoting neurogenesis via Wnt/β-catenin signaling pathway accounts for the neurorestorative effects of morroniside against cerebral ischemia injury. Eur. J. Pharmacol. 738, 214–221. doi:10.1016/j.ejphar.2014.05.019
Sun, M. Y., Feng, X., Lin, X. H., Yin, M., Zhao, X. Z., Chen, Y., et al. (2011). Studies on the chemical constituents from stems and leaves of Lonicera macranthoides. Zhong Yao Cai 34 (2), 218–220.
Sun, P., Wei, S., and Wang, X. (2012). Effects of morroniside on cycloxygenase after platelet aggregation induced by adenosine diphosphate in rabbits. Chin. J. Rehabilitation Theory Pract. 18 (04), 331–332. doi:10.3969/j.issn.1006-9771.2012.04.005
Sun, X., Xue, S., Cui, Y., Li, M., Chen, S., Yue, J., et al. (2023). Characterization and identification of chemical constituents in Corni Fructus and effect of storage using UHPLC-LTQ-Orbitrap-MS. Food Res. Int. 164, 112330. doi:10.1016/j.foodres.2022.112330
Sun, Y., Zhu, Y., Liu, X., Chai, Y., and Xu, J. (2020). Morroniside attenuates high glucose-induced BMSC dysfunction by regulating the Glo1/AGE/RAGE axis. Cell. Prolif. 53 (8), e12866. doi:10.1111/cpr.12866
Suriano, F., Vieira-Silva, S., Falony, G., Roumain, M., Paquot, A., Pelicaen, R., et al. (2021). Novel insights into the genetically obese (ob/ob) and diabetic (db/db) mice: two sides of the same coin. Microbiome 9 (1), 147. doi:10.1186/s40168-021-01097-8
Takeda, Y., Tanigawa, N., Sunghwa, F., Ninomiya, M., Hagiwara, M., Matsushita, K., et al. (2010). Morroniside cinnamic acid conjugate as an anti-inflammatory agent. Bioorg Med. Chem. Lett. 20 (16), 4855–4857. doi:10.1016/j.bmcl.2010.06.095
Tang, X., Wu, H., Mao, X., Li, X., and Wang, Y. (2020). The GLP-1 receptor herbal agonist morroniside attenuates neuropathic pain via spinal microglial expression of IL-10 and β-endorphin. Biochem. Biophys. Res. Commun. 530 (3), 494–499. doi:10.1016/j.bbrc.2020.05.080
Uribarri, J., Cai, W., Peppa, M., Goodman, S., Ferrucci, L., Striker, G., et al. (2007). Circulating glycotoxins and dietary advanced glycation endproducts: two links to inflammatory response, oxidative stress, and aging. J. Gerontol. A Biol. Sci. Med. Sci. 62 (4), 427–433. doi:10.1093/gerona/62.4.427
Wang, Q. N., Zou, Z. X., Wang, X. P., Zhang, Q., Zhao, Y. Q., Duan, L., et al. (2021). Endothelial progenitor cells induce angiogenesis: a potential mechanism underlying neovascularization in encephaloduroarteriosynangiosis. Transl. Stroke Res. 12 (2), 357–365. doi:10.1007/s12975-020-00834-9
Wang, S. F., Chen, X. G., Hu, Z. D., and Ju, Y. (2003). Analysis of three effective components in Fructus corni and its preparations by micellar electrokinetic capillary chromatography. Biomed. Chromatogr. 17 (5), 306–311. doi:10.1002/bmc.247
Wang, T., Cui, J., Liu, T., Sun, F., and Tao, Y. (2019). Effects of morroniside on expression of angiopoietin-1 and fibroblast growth factor-2 in infarcted myocardium in rats with acute myocardial infarction. Chin. Heart J. 31 (03), 266–269. doi:10.12125/j.chj.201902016
Wang, W., Huang, W., Li, L., Liu, C., Sun, F., An, Y., et al. (2008). Morroniside prevents peroxide-induced apoptosis by induction of endogenous glutathione in human neuroblastoma cells. Cell. Mol. Neurobiol. 28 (2), 293–305. doi:10.1007/s10571-007-9168-7
Wang, W., Sun, F., An, Y., Ai, H., Zhang, L., Huang, W., et al. (2009). Morroniside protects human neuroblastoma SH-SY5Y cells against hydrogen peroxide-induced cytotoxicity. Eur. J. Pharmacol. 613 (1-3), 19–23. doi:10.1016/j.ejphar.2009.04.013
Wang, W., Xu, J., Li, L., Wang, P., Ji, X., Ai, H., et al. (2010a). Neuroprotective effect of morroniside on focal cerebral ischemia in rats. Brain Res. Bull. 83 (5), 196–201. doi:10.1016/j.brainresbull.2010.07.003
Wang, X., Chen, N., and Wang, W. (2010b). Effects of morroniside on platelet aggregation in rabbit. Chin. J. Rehabilitation Theory Pract. 16 (11), 1029–1030.
Wei, R., Tian, X., Sun, F., Zhu, Z., Liu, T., Wang, Y., et al. (2016). Effects of morroniside on the expression of the HGF and vWF in peri-infarct cortex after cerebral ischemia-reperfusion in rats. Chin. J. Comp. Med. 26 (03), 24–28. doi:10.3969/j.issn.1671-7856.2016.03.006
Wen, Y., Yang, S., Liu, R., and Simpkins, J. W. (2005). Cell-cycle regulators are involved in transient cerebral ischemia induced neuronal apoptosis in female rats. FEBS Lett. 579 (21), 4591–4599. doi:10.1016/j.febslet.2005.07.028
Wiatrak, B., Kubis-Kubiak, A., Piwowar, A., and Barg, E. (2020). PC12 cell line: cell types, coating of culture vessels, differentiation and other culture conditions. Cells 9 (4), 958. doi:10.3390/cells9040958
Xiao, J., Han, Q., Yu, Z., Liu, M., Sun, J., Wu, M., et al. (2023). Morroniside inhibits inflammatory bone loss through the TRAF6-mediated NF-κB/MAPK signalling pathway. Pharm. (Basel) 16 (10), 1438. doi:10.3390/ph16101438
Xiao, Z., Wang, J., Chen, S., and Feng, Y. (2020). Autophagy promotion enhances the protective effect of Morroniside on human OA chondrocyte. Biosci. Biotechnol. Biochem. 84 (5), 989–996. doi:10.1080/09168451.2020.1717925
Xu, F., and Cao, L. (2015). Discussion on the formula and syndrome of Jinkui Shenqi Pill and its clinical application. China J. Traditional Chin. Med. Pharm. 30 (03), 931–932. doi:10.19803/j.1672-8629.20220512
Xu, F., Li, W., Yang, X., Na, L., Chen, L., and Liu, G. (2020). The roles of epigenetics regulation in bone metabolism and osteoporosis. Front. Cell. Dev. Biol. 8, 619301. doi:10.3389/fcell.2020.619301
Xu, H., Shen, J., Liu, H., Shi, Y., Li, L., and Wei, M. (2006). Morroniside and loganin extracted from Cornus officinalis have protective effects on rat mesangial cell proliferation exposed to advanced glycation end products by preventing oxidative stress. Can. J. Physiol. Pharmacol. 84 (12), 1267–1273. doi:10.1139/y06-075
Xu, H. Q., Hao, H. P., Zhang, X., and Pan, Y. (2004). Morroniside protects cultured human umbilical vein endothelial cells from damage by high ambient glucose. Acta Pharmacol. Sin. 25 (4), 412–415.
Xue, J. Y., Li, Y. L., Fan, Z. Y., Su, M. X., and Cen, Y. Z. (2007). Studies on the chemical constituents of Adina pilulifera. Zhong Yao Cai 30 (9), 1084–1086. doi:10.3321/j.issn:1001-4454.2007.09.013
Yang, C., Kuai, X., Gao, W., Yu, J., Wang, Q., Lia, L., et al. (2016). Morroniside-induced PP2A activation antagonizes tau hyperphosphorylation in a cellular model of neurodegeneration. J. Alzheimers Dis. 51 (1), 33–44. doi:10.3233/jad-150728
Yang, C., Luo, Y., Guo, K., Zheng, C., Li, L., and Zhang, L. (2020). Cornel iridoid glycoside regulates modification of tau and alleviates synaptic abnormalities in aged P301S mice. Curr. Med. Sci. 40 (6), 1040–1046. doi:10.1007/s11596-020-2285-y
Yang, H. Z., Dong, R., Jia, Y., Li, Y., Luo, G., Li, T., et al. (2023). Morroniside ameliorates glucocorticoid-induced osteoporosis and promotes osteoblastogenesis by interacting with sodium-glucose cotransporter 2. Pharm. Biol. 61 (1), 416–426. doi:10.1080/13880209.2023.2173787
Ye, J., Zhang, X., Dai, W., Yan, S., Huang, H., Liang, X., et al. (2009). Chemical fingerprinting of Liuwei Dihuang Pill and simultaneous determination of its major bioactive constituents by HPLC coupled with multiple detections of DAD, ELSD and ESI-MS. J. Pharm. Biomed. Anal. 49 (3), 638–645. doi:10.1016/j.jpba.2008.12.009
Yokozawa, T., Kang, K. S., Park, C. H., Noh, J. S., Yamabe, N., Shibahara, N., et al. (2010). Bioactive constituents of Corni Fructus: the therapeutic use of morroniside, loganin, and 7-O-galloyl-D-sedoheptulose as renoprotective agents in type 2 diabetes. Drug Discov. Ther. 4 (4), 223–234.
Yokozawa, T., Yamabe, N., Kim, H. Y., Kang, K. S., Hur, J. M., Park, C. H., et al. (2008). Protective effects of morroniside isolated from Corni Fructus against renal damage in streptozotocin-induced diabetic rats. Biol. Pharm. Bull. 31 (7), 1422–1428. doi:10.1248/bpb.31.1422
Yu, B., and Wang, W. (2018). Cardioprotective effects of morroniside in rats following acute myocardial infarction. Inflammation 41 (2), 432–436. doi:10.1007/s10753-017-0699-x
Yu, B., Zhang, G., An, Y., and Wang, W. (2018). Morroniside on anti-inflammation activities in rats following acute myocardial infarction. Korean J. Physiol. Pharmacol. 22 (1), 17–21. doi:10.4196/kjpp.2018.22.1.17
Yu, C., Yu, S., Liu, Z., Xu, L., Zhang, Z., Wan, J., et al. (2024). Morroniside promotes skin wound re-epithelialization by facilitating epidermal stem cell proliferation through GLP-1R-mediated upregulation of β-catenin expression. Acta Biochim. Biophys. Sin. (Shanghai) 56 (7), 1072–1084. doi:10.3724/abbs.2024070
Yu, H., and Chen, J. (2013). Effects of morroniside on the in vitro model of the Melanoma Cells and keratinocyte co-culture system. Chin. J. Aesthetic Med. 22 (08), 833–835. doi:10.15909/j.cnki.cn61-1347/r.2013.08.001
Yu, H., Yao, S., Zhou, C., Fu, F., Luo, L., Du, W., et al. (2021). Morroniside attenuates apoptosis and pyroptosis of chondrocytes and ameliorates osteoarthritic development by inhibiting NF-κB signaling. J. Ethnopharmacol. 266, 113447. doi:10.1016/j.jep.2020.113447
Yuan, J., Cheng, W., Zhang, G., Ma, Q., Lia, X., Zhang, B., et al. (2020). Protective effects of iridoid glycosides on acute colitis via inhibition of the inflammatory response mediated by the STAT3/NF-кB pathway. Int. Immunopharmacol. 81, 106240. doi:10.1016/j.intimp.2020.106240
Yuan, T., Sun, F., Wang, X., Ai, H., Wang, W., and Gong, D. (2013). Effect of morroniside on coagulation in focal cerebral ischemia/reperfusion in rats. Chin. J. Rehabilitation Theory Pract. 19 (02), 104–106. doi:10.3969/j.issn.1006-9771.2013.02.002
Zeng, G., Ding, W., Li, Y., Sun, M., and Deng, L. (2018). Morroniside protects against cerebral ischemia/reperfusion injury by inhibiting neuron apoptosis and MMP2/9 expression. Exp. Ther. Med. 16 (3), 2229–2234. doi:10.3892/etm.2018.6457
Zhang, C., Tong, Q., Liu, K., Mao, T., Song, Y., Qu, Y., et al. (2024). Morroniside delays the progression of non-alcoholic steatohepatitis by promoting AMPK-mediated lipophagy. Phytomedicine 129, 155703. doi:10.1016/j.phymed.2024.155703
Zhang, J., and Wang, H. (2019). Morroniside protects against chronic atrophic gastritis in rat via inhibiting inflammation and apoptosis. Am. J. Transl. Res. 11 (9), 6016–6023.
Zhang, J., Wang, Y., Liu, X., Liu, H., Zhao, X., Lv, S., et al. (2023). Expression analysis of CoHMGS in Cornus officinalis and subcellular localization of the enzyme it encodes. DNA Cell. Biol. 42 (2), 91–96. doi:10.1089/dna.2022.0527
Zhang, J. X., Wang, R., Xi, J., Shen, L., Zhu, A. Y., Qi, Q., et al. (2017). Morroniside protects SK-N-SH human neuroblastoma cells against H2O2-induced damage. Int. J. Mol. Med. 39 (3), 603–612. doi:10.3892/ijmm.2017.2882
Zhang, L., Wang, H., Liu, Y., Wang, L., Pan, W., and Yuan, B. (2022a). Morroniside protects HT-22 cells against oxygen-glucose deprivation/reperfusion through activating the Nrf2/HO-1 signaling pathway. J. Recept Signal Transduct. Res. 42 (1), 9–15. doi:10.1080/10799893.2020.1837872
Zhang, S., Lai, Q., Liu, L., Yang, Y., and Wang, J. (2022b). Morroniside alleviates lipopolysaccharide-induced inflammatory and oxidative stress in inflammatory bowel disease by inhibiting NLRP3 and NF-κB signaling pathways. Allergol. Immunopathol. Madr. 50 (6), 93–99. doi:10.15586/aei.v50i6.674
Zhao, X., Wang, Y., and Sun, Y. (2007). Simultaneous determination of four bioactive constituents in Liuwei Dihuang Pills by micellar electrokinetic chromatography. J. Pharm. Biomed. Anal. 44 (5), 1183–1186. doi:10.1016/j.jpba.2007.04.025
Zheng, S., Liu, T., Chen, M., Sun, F., Fei, Y., Chen, Y., et al. (2023). Morroniside induces cardiomyocyte cell cycle activity and promotes cardiac repair after myocardial infarction in adult rats. Front. Pharmacol. 14, 1260674. doi:10.3389/fphar.2023.1260674
Zhou, C., Yao, S., Fu, F., Bian, Y., Zhang, Z., Zhang, H., et al. (2022). Morroniside attenuates nucleus pulposus cell senescence to alleviate intervertebral disc degeneration via inhibiting ROS-Hippo-p53 pathway. Front. Pharmacol. 13, 942435. doi:10.3389/fphar.2022.942435
Zhou, D., Lv, D., Zhang, H., Cheng, T., Wang, H., Lin, P., et al. (2021). Quantitative analysis of the profiles of twelve major compounds in Gentiana straminea Maxim. Roots by LC-MS/MS in an extensive germplasm survey in the Qinghai-Tibetan plateau. J. Ethnopharmacol. 280, 114068. doi:10.1016/j.jep.2021.114068
Zhou, J., Zhang, L., Lei, X., and Zhang, R. (2019). Protective effect of morroniside on myocardial injury and oxidative stress in neonatal rats with hypoxic-ischemic brain injury. Anat. Res. 41 (04), 256–262.
Zhou, L., Wang, H., Jing, J., Yu, L., Wu, X., and Lu, Z. (2018a). Morroniside regulates hair growth and cycle transition via activation of the Wnt/β-catenin signaling pathway. Sci. Rep. 8 (1), 13785. doi:10.1038/s41598-018-32138-2
Zhou, Y., Sun, Y., Li, P., Qin, G., Cheng, Q., Liu, Y., et al. (2018b). Monoside antagonizes triptolide-induced hepatocyte apoptosis via the anti-oxidative stress pathway. Nan Fang. Yi Ke Da Xue Xue Bao 38 (8), 949–955. doi:10.3969/j.issn.1673-4254.2018.08.08
Zhu, W., Chen, M., Wang, Y., Chen, Y., Zhang, Y., Wang, Y., et al. (2023). Regulation of renal lipid deposition in diabetic nephropathy on morroniside via inhibition of NF-KB/TNF-a/SREBP1c signaling pathway. Chem. Biol. Interact. 385, 110711. doi:10.1016/j.cbi.2023.110711
Zuo, W., Wang, X., and Ai, H. (2012). Effects of morroniside on inhibiting thromboxane B2 after platelet aggregation induced by adenosine diphosphate in rabbits. Chin. J. Rehabilitation Theory Pract. 18 (04), 329–330. doi:10.3969/j.issn.1006-9771.2012.04.004
Glossary
Keywords: morroniside, sources, pharmacology, distribution, detection
Citation: Shi P, Zheng B, Zhang S and Guo Q (2024) A review of the sources and pharmacological research of morroniside. Front. Pharmacol. 15:1423062. doi: 10.3389/fphar.2024.1423062
Received: 26 April 2024; Accepted: 19 August 2024;
Published: 04 September 2024.
Edited by:
Juei-Tang Cheng, Chang Jung Christian University, TaiwanReviewed by:
Denglang Zou, Qinghai Normal University, ChinaWen Wang, Capital Medical University, China
Copyright © 2024 Shi, Zheng, Zhang and Guo. This is an open-access article distributed under the terms of the Creative Commons Attribution License (CC BY). The use, distribution or reproduction in other forums is permitted, provided the original author(s) and the copyright owner(s) are credited and that the original publication in this journal is cited, in accordance with accepted academic practice. No use, distribution or reproduction is permitted which does not comply with these terms.
*Correspondence: Qingmei Guo, qmguo@sina.com