- 1Department of Traditional Chinese Veterinary Medicine, College of Veterinary Medicine, Southwest University, Chongqing, China
- 2Weifang Academy of Agricultural Sciences, Institute of Animal Husbandry, Shandong, China
- 3Hanzhong Animal Disease Prevention and Control Center, Shaanxi, China
- 4Chi Institute of Traditional Chinese Veterinary Medicine, Southwest University, Chongqing, China
Ethnopharmacological relevance: Danggui Buxue decoction (DBD) is a traditional Chinese herbal formula. According to the theory of traditional Chinese medicine, the combination of Astragali Radix (AR) and Angelica sinensis (AS) is a classic prescription of tonifying qi and enriching blood. DBD has the functions of hematopoietic, immune enhancement and inflammation inhibition, usually used to treat qi and blood deficiency symptoms.
Aim of the study: Cyclophosphamide (CY) can inhibit humoral and cellular immunity, leading to the overall immune disorder of the body, resulting in immunosuppressive (IS). Pre-laboratory studies confirmed the immunomodulatory effects of DBD, but its mechanisms have not been thoroughly studied. In this study, the main purpose was to determine the effects of DBD on the immune function and intestinal mucosal barrier function of IS mice induced by CY, and initially explored the immunomodulatory mechanism of DBD.
Materials and methods: 100 g of AR and 20 g of AS were accurately weighed and 0.5 g/mL of the DBD was obtained by boiling, filtration and rotary evaporation. Then, mice in the DBD group were administered 5 g/kg of DBD by gavage, positive group were administered 40 mg/kg of levamisole hydrochloride, whereas those in the control and model groups were given the corresponding volume of normal saline by gavage for 1 week. At the end of the experiment, blood, spleen, thymus, ileum and cecum contents of all the experimental mice were collected aseptically. IS mouse model induced by intraperitoneal injection of 80 mg/kg CY for three consecutive days. Pathomorphology was used to observe the physical barrier of the intestine, flow cytometry to detect splenic lymphocytes, immunohistochemistry to determine the content of intestinal barrier-associated proteins, ELISA to measure the secretion of ileal SIgA, qRT-PCR to detect the mRNA expression of immune-related genes in the intestine, and high-throughput sequencing and analysis of cecum contents.
Results: DBD alleviated spleen tissue damage and restored impaired immune functions, such as increased thymus index and CD4+/CD8+ subsets of spleen lymphocytes. In addition, DBD could increase ileum villi length and the ratio of villi length to crypt depth (V/C), and decrease crypt depth. Moreover, DBD administration up-regulated the expression of ZO-1, Occludin, Claudin-1, MUC-2 mRNA in ileum. And the secretions of sIgA and ZO-1 in ileum were also significantly improved. Furthermore, the administration of DBD can increase the diversity of gut microbiota, improve the composition of intestinal flora and increase the relative abundance of beneficial genus, such as Bacteroides.
Conclusion: DBD alleviated CY-induced immune damage by decreasing the ratio of spleen index to CD4+/CD8+ of T lymphocyte subsets. And the intestinal barrier function of mice was by improves improving the intestinal morphology of the ileum and up-regulating the expression levels of ZO-1, MUC-2 and SIgA. DBD regulates CY-induced gut microbiota dysregulation in mice by increasing species diversity and richness, regulating the phylum, class and order levels of Bacteroidetes.
1 Introduction
Host defense is one of the most basic functions of organisms, protecting humans and animals from infectious diseases (Yu et al., 2021; Sudeep et al., 2023). However, due to the damage of the immune system composed of cells, tissues and organs involved in the immune response, an immune abnormal state of the body’s ability to respond to antigens is called immunosuppression (Feng et al., 2021). Immunosuppression can lead to the gradual decline of the body’s immune capacity, weakened drug response, and more susceptibly infected by pathogenic microorganisms, which is difficult to treat, resulting in increased morbidity and mortality (Han et al., 2023; Yao et al., 2023a). Therefore, it is of great significance to search for safe immunomodulators to alleviate immunosuppressive diseases.
Cyclophosphamide (CY) is an active alkylating agent that is clinically used to treat diseases such as leukemia (Kumar and Venkatesh, 2016). However, CY can inhibit humoral and cellular immunity, leading to the overall immune disorder of the body, resulting in immunosuppressive side effects. Therefore, CY is commonly used in modern research to establish immunosuppressive animal models (Cui et al., 2022a; Nam et al., 2022; Nan et al., 2023). Intraperitoneal injection of CY induced weight loss, decreased immune organ index, cellular immune factors, immunoglobulin and immune cell activity in mice. CY also altered the gut microbiota composition and damaged the intestinal mucosa in mice (Huang et al., 2021; Zhang et al., 2023).
Chinese herbal medicine has been widely concerned for its safety, accessibility, anti-inflammatory and immunomodulatory effects. According to deficiency theory of TCM and earlier reports, long-term deficiency of qi and blood will lead to immunosuppression (Pan et al., 2023), so according to the principle of Chinese medicine treatment for immunosuppression diseases, the method of supplementing qi and blood should be adopted. Danggui Buxue decoction (DBD) first recorded in the book “Neiwaishang Bianhuo Lun” written by Li Dongyuan in the Jin and Yuan dynasties, it is a famous prescription for supplementing qi and blood (Li et al., 2021; Zhou et al., 2022). It is composed of Astragalus mongholicus Bunge (Astragali Radix, AR) and Angelica sinensis (Oliv.) (Angelica sinensis, AS) (The plant name has been checked with http://www.worldfloraonline.org) (Liu et al., 2021a; Wang et al., 2022) with a ratio of 5:1. AR has the effect of tonifying qi and strengthening superficial resistance of the body, promoting diuresis, detoxifying, discharging pus, astringing sores and promoting tissue regeneration. AS has the effect of enriching blood and activating blood circulation, regulating menstruation to relieve pain, moistening bowels and relieving constipation. The combination of these two herbal medicines is a classic prescription of tonifying qi and enriching blood. Modern research believes that qi is closely related to immunity. In traditional Chinese medicine syndrome differentiation and treatment, immune deficiency belongs to asthenic syndrome, that is, lack of vital qi will lead to the invasion of evil qi. Modern pharmacological studies have confirmed that DBD has the functions of hematopoietic, immune enhancement and inflammation inhibition (Kwan et al., 2021). At present, the pharmacological effects of DBD are mainly in its promotion of hematopoietic and anti-tumor aspects (Liu et al., 2021b; Xiang et al., 2023), while the studies on the regulation of animal immunity are relatively few. Previous laboratory studies discussed the effect of different doses of DBD on the immunity of type O foot-and-mouth disease vaccine and intestinal mucosal immunity. The results showed that 5 g/kg DBD had the best effect (Zhou et al., 2022). However, the immunomodulatory effects of DBD and its mechanisms have not been thoroughly studied, especially the immune-protective mechanisms under the state of immunosuppression is still unclear.
Therefore, the main purpose of this study was to investigate the immunomodulatory effect and mechanism of DBD by CY induced IS mouse model. We used pathological examination, ELISA, molecular biology techniques, high-throughput sequencing and other methods to determine the effects of DBD on the immune function and intestinal mucosal barrier function of IS mice, and initially explored the immunomodulatory mechanism of DBD. We believe that this study not only helps to reveal the immunomodulatory mechanism of DBD, but also provides a lot of scientific evidence for the development of new immune enhancers.
2 Materials and methods
2.1 Drugs and reagents
Astragali radix [Fabaceae; Astragalus mongholicus Bunge](210303) was purchased from Gansu Guocao Pharmaceutical Co., LTD.; Angelica sinensis [Apiaceae; Angelica sinensis (Oliv.)] (201201) was purchased from Chongqing Yafeng Pharmaceutical Co., LTD.; Cyclophosphamide (C849559) and levamisole hydrochloride (L812625) were purchased from Shanghai Maclin Biochemical Technology Co., LTD.; Rat Anti-Mouse CD3ε-APC (1535-11), Rat Anti-Mouse CD4-FITC (1540-02s), and Rat Anti-Mouse CD8-PE (1550-09) were purchased from Southern United States Biotech Inc.; Mouse spleen lymphocyte isolation kit (LTS1092PK), purchased from Tianjin Hao Yang Biological Products Technology Co., LTD. HRP-Goat Anti-Rabbit IgG (ZB-2301) was purchased from Zhongshan Jinqiao. Rabbit Anti-ZO-1 antibody (bs-1329R) from Beijing Boosen Biotechnology Co., LTD. Mouse SIgA Elisa kit (BPE20416) was purchased from Shanghai Langton Biotechnology Co., LTD. RNAiso Plus (9109), PrimeScript RT reagent kit with gDNA eraser (RR047A), TB Green premix ex taq II (Tli RNaseH Plus) (RR820A), Purchased from Baori Doctor Physical Technology (Beijing) Co., LTD.; Mouse ATCB internal reference primer (B661302-0001), purchased from Bioengineering (Shanghai) Co., LTD.
2.2 Animal and experimental design
Forty six-week-old specific pathogen-free (SPF) male Kunming mice with a body weight of 26 ± 2 g were purchased from Sibeifu (Beijing) Biotechnology Co., LTD (Animal license NO. SCXK [Beijing] 2019-0010). The animals were housed at a temperature of 22°C ± 2°C and relative humidity of 55% ± 10%, subjected to a 12-h/12-h light/dark cycle, and provided access to food and water ad libitum for 1 week. All animal experiments were performed with approval by the Laboratory Animal Ethics Committee of Southwest University (permit number: IACUC-20211020-01). And all the experimental animals were euthanized at the end of the experiment.
2.3 Preparation of DBD
DBD was prepared according to the method previously obtained (Zhou et al., 2022). In short, accurately weigh 100 g of AR and 20 g of AS, soak in 8 times distilled water at high temperature for 30 min to boil, and then gently simmer for 1 h. Strain the liquid and add the residue to an equal amount of distilled water for another 1 h. The two extracts were mixed and concentrated to obtain a crude drug content of 0.5 g/mL using a rotary evaporator.
2.4 Animal modeling and drug administration
After adaptive feeding, 40 mice were randomly divided into control group, model group, positive group and DBD group, with 10 mice per group. Mice in the model, positive and DBD groups were intraperitoneally injected with 80 mg/kg CY for 3 consecutive days to establish the IS model. Then, mice in the DBD group were administered 5 g/kg of DBD by gavage, positive group were administered 40 mg/kg of levamisole hydrochloride, whereas those in the control and model groups were given the corresponding volume of normal saline by gavage for 1 week. At the end of the experiment, blood, spleen, thymus, ileum and cecum contents of all the experimental mice were collected aseptically.
2.5 Body weight and immune organ index
Each mouse was weighed daily. And at the end of the experiment, spleen and thymus of all mice were collected aseptically and weighed. The organ index is calculated as follows:
Organ index = organ weight (mg)/mouse weight (g).
2.6 Flow cytometry
The spleen was ground with a syringe piston and passed through a 70 μm cell screen to form a cell suspension. The cells were gently washed twice in an ice bath with PBS to adjust the cell concentration to 1×106/mL. CD3-APC, CD4-FITC, and CD8-PE were added with 1 μL each, and incubated on ice for 30 min in dark light, then suspended twice with 1 mL PBS, centrifuged (2500 rpm, 5 min), abandoned the supernatant, and added 800 μL PBS to blow and mixed well. Finally, lymphocytes were detected by flow cytometry.
2.7 Hematoxylin-eosin staining (HE)
The ileum tissue was fixed in 4% neutral formaldehyde, trimmed to a suitable size, washed with running water overnight, dehydrated with ethanol gradient, and embedded in paraffin. The paraffin-embedded ileum was cut into 5 μm sections for HE staining, and the villi length and crypt depth were measured by ImageJ software. The ratio of villi length to crypt depth was calculated.
2.8 Immunohistochemistry staining (IHC)
The paraffin-embedded ileum was cut into 5 μm slices and fixed on adhesive slides. After gradient dehydration with ethanol, endogenous peroxidase was removed with 3% hydrogen peroxide, and antigen repair was performed in sodium citrate buffer solution. The ileum was closed with 5%BSA for 30 min and incubated overnight with Rabbit Anti-ZO-1 antibody at 4°C. On the second day, Goat Anti-Rabbit IgG droplets labeled by HRP were added to the slide, stained by DAB and HE, and observed by microscope.
2.9 Enzyme-linked immunosorbent assay (ELISA)
Mouse ileum tissue was homogenized, supernatant was obtained by centrifugation, and SIgA content was detected according to manufacturer’s instructions.
2.10 Real-time reverse transcription–polymerase chain reaction (qRT-PCR)
Total ileum RNA was extracted by TRIZOL method and reversed-converted into cDNA. mRNA expression levels of ZO-1, Claudin-1, Occludin, MUC-2 and IgA in ileum tissue were detected by real-time fluorescence quantitative PCR. Using β-actin as internal reference gene, the primer sequences are shown in Table 1 and the data were analyzed using 2−△△ct method.
2.11 16S rRNA sequencing of gut microbiota
Gut microbiota community gene DNA was extracted from mouse cecum contents according to the instructions of fecal DNA extraction kit. Universal primers 338F (5′-ACTCCTACGGGAGGCAGCAG-3′) and 806R (5′-GGACTACHVGGGTWTCTAAT-3′) were used to amplify the V3-V4 region of bacterial 16s rRNA gene. The PCR products were sequenced and analyzed on a high-throughput sequencer. The sequencing results were filtered and spliced (Pear software), and the sequences with 97% similarity were clustered into operation classification units (OTU). The sequences were compared with Silva138 database (Silva138), and R software was used for mapping.
2.12 Statistical analysis
All data were expressed as the mean ± standard error (SE) and analyzed using GraphPad Prism 8. Statistical significance was determined using IBM SPPS 22.0, and differences were considered significant at p < 0.05.
3 Results
3.1 Evaluation of the IS mice model
The status and weight changes of mice before and after CY injection were recorded to evaluate whether the model was successfully established. Compared with the control group, the mice injected with CY showed symptoms such as lethargy. After CY injection, the mice body weight began to decrease linear and significantly different compared with that before the injection (Figure 1A). Moreover, the thymus index significantly decreased and the spleen index significantly increased (Figure 1B) in the model group compared with the control group (p < 0.05). These results indicated that the IS mice model was successfully established.
3.2 Effect of DBD lymphocyte subsets of spleen in IS mice
As shown in Figure 2A, the distribution analysis of splenic lymphocyte subsets of mice in each group showed that splenic lymphocyte, CD3+ cells and CD4+/CD8+ T cells were obviously grouped. According to Figure 2B, the CD4+/CD8+ ratio of spleen lymphocytes in the model group was significantly lower than that in the control group, positive group and DBD group (p < 0.05).
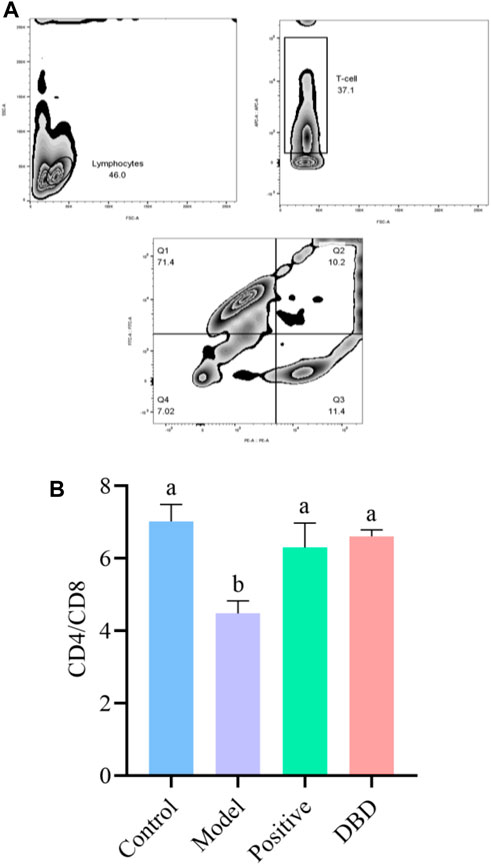
Figure 2. Effect of DBD on lymphocyte subsets of spleen in IS mice. (A) zebra plot of flow cytometry; (B) CD4+/CD8+ subsets.
3.3 Effect of DBD on the morphology of ileum in IS mice
HE staining was used to observe the morphological and structural changes of the ileum tissue of experimental mice in each group, and the test results were shown in Figure 3. Compared with the control group, the villi length of was shorter, the intestinal recess was deeper and the ratio of V/C was significantly decreased in model group (p < 0.05). However, compared with the model group, the depth of crypt was decreased, villus length and V/C was increased in DBD group (p < 0.05).
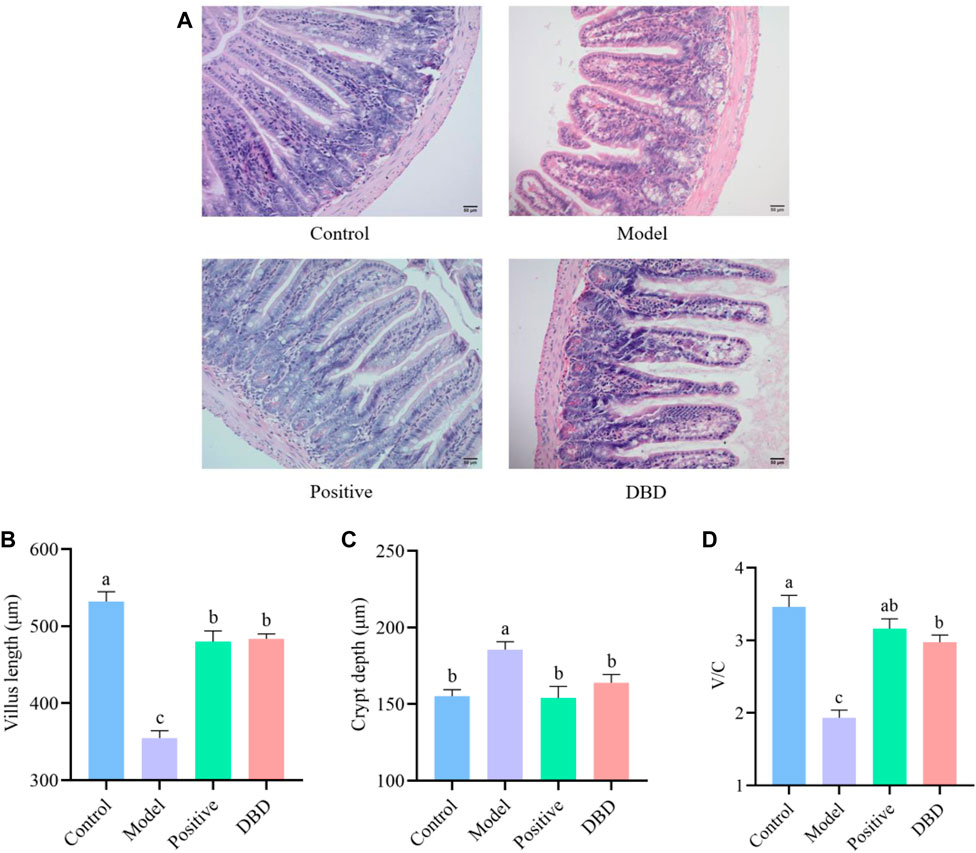
Figure 3. Effect of DBD on ileum morphology in IS mice. (A) HE; (B) Villus length; (C) Crypt depth; (D) V/C.
3.4 Effect of DBD on intestinal mucosal barrier in IS mice
We evaluated the effects of DBD on intestinal mucosal barrier function in IS mice by detecting physical barrier, chemical barrier and immune barrier. Compared with the control group, the SIgA secretion was significantly reduced in model group, while SIgA secretion in ileum was improved after DBD treatment (Figure 4A). As shown in Figure 4B, CY can significantly downregulate mRNA expression levels of ZO-1, Occludin, Claudin-1, MUC-2 and IgA (p < 0.05). After DBD treatment, the mRNA expression levels of ZO-1, MUC-2 and IgA significantly upregulated (p < 0.05). The results of IHC (Figures 4C, D) were similar to those of qRT-PCR. Compared with control group, CY decreased the expression level of ileum ZO-1 protein and increased intestinal permeability, while DBD improved intestinal permeability (p < 0.05).
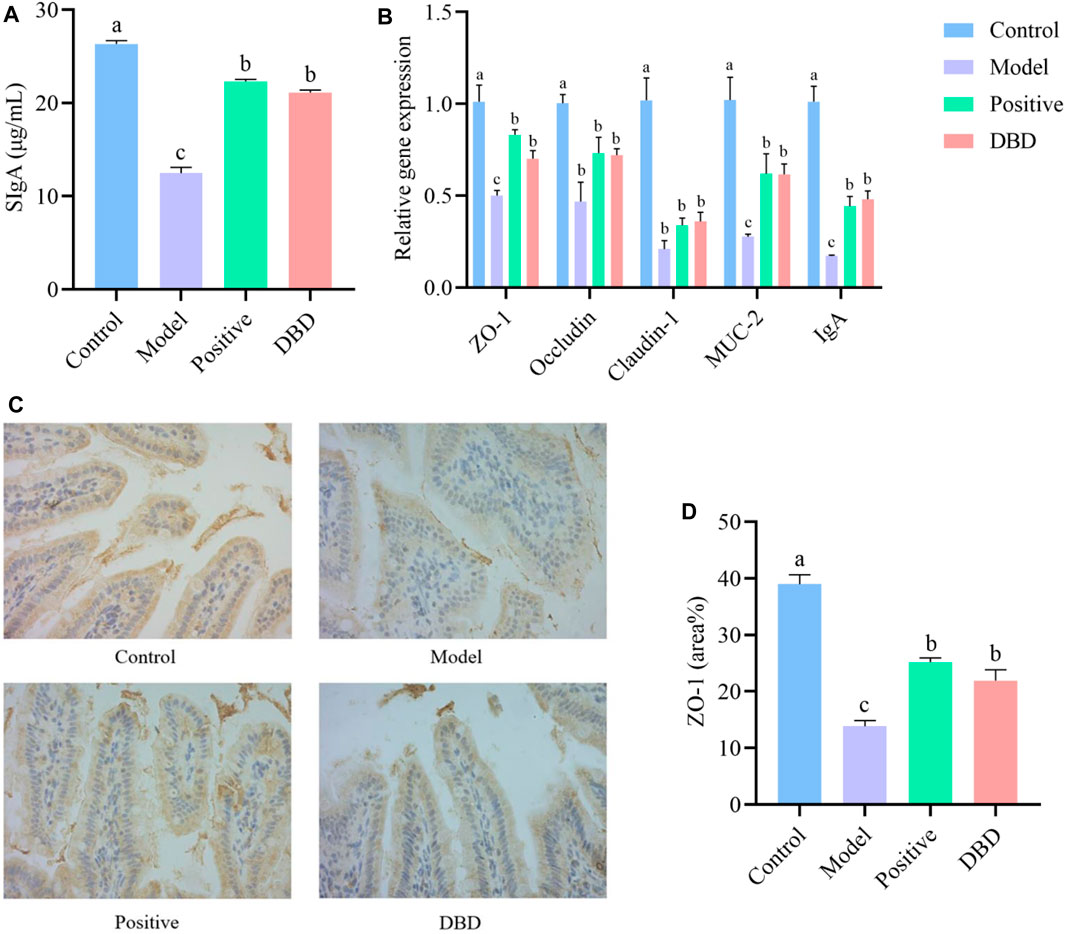
Figure 4. Effect of DBD on intestinal mucosal barrier in IS mice. (A) SIgA secretion; (B) mRNA expression levels; (C) IHC; (D) ZO-1 protein area.
3.5 Effect of DBD on gut microbiota structure in IS mice
As shown in Figure 5, a total of 3121 OTU were obtained, including 1102 shared OTU in the four groups. There were 1347, 1692, 1781 OTU in control group compared with model group, positive group and DBD group, respectively. The number of unique OTU in model group was the highest, but the positive group and DBD group was similar to control group.
3.6 Analysis of alpha diversity of gut microbiota in IS mice by DBD
Alpha diversity was mainly used to analyze the diversity of gut microbiota among each group. As shown in Figure 6, the dilution curve (Figure 6A), Shannon index curve (Figure 6B), class abundance curve (Figure 6C) and species accumulation curve (Figure 6D) tend to be flat, indicating sufficient sequencing data and high species richness and evenness. Compared with the control group, the indexes of Chao 1 (Figure 7A), Simpson (Figure 7B) and Shannon (Figure 7C) in the model group was decreased, among which Simpson and Shannon indexes decreased significantly (p < 0.05). This indicating that the species abundance and diversity of cecal colonies decreased. Compared with the model group, the indexes of Chao 1, Simpson and Shannon of mice in positive group and DBD group were significantly increased (p < 0.05). This indicates that DBD could effectively alleviate the decline in cecum colony abundance and diversity caused by CY.
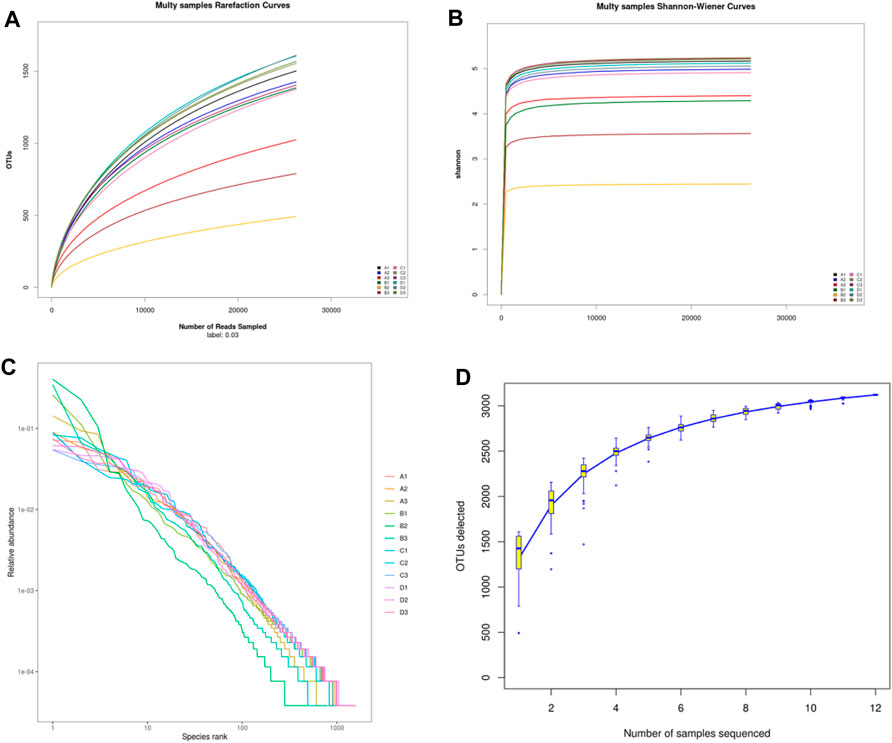
Figure 6. Alpha diversity curve. (A) Multy samples rarefaction curves; (B) Multy samples Shannon-wiener curves; (C) Rank abundance curve; (D) Specaccum accumulation curve.
3.7 Analysis of beta diversity of gut microbiota in IS mice by DBD
The Beta diversity index further analyzed the effects of CY treatment on the richness and diversity of intestinal microbiota in mice. PCA (Figure 8A) and PCoA (Figure 8B) analyses are distance-based Beta diversity analyses, that is, the closer the distance between samples, the more similar the sample composition. As shown in Figures 8A, B, within a certain range, PCA and PCoA maps showed that the microbial composition of each group was similar, and the microbial composition of each group was clustering. In general, the distance between the model and control, positive and DBD group was relatively far, means the differences were obvious. NMDS analysis (Figure 8C) were consistent with those of PCA and PCoA. These results showed that DBD could improve the changes of gut microbiota in mice induced by CY.
3.8 Effect of DBD on gut microbiota composition of IS mice
In order to further analyze the potential protective mechanism of DBD on IS mice, 16s rRNA sequencing was used to analyze the effect of DBD on gut microbiota of IS mice. A total of 18 of phylum levels, 38 of class levels, 88 of order levels, 148 of family levels, 286 of genus levels and 202 of species levels were identified in this experiment.
At the phylum level (Figure 9A), the dominant bacteria are mainly composed of Firmicutes, Bacteroidota, Proteobacteria, Desulfobacterota and Campilobacterota. There was significant difference between the model group and other groups in Bacteroidota and Proteobacteria (p < 0.05). Firmicutes/Bacteroidota in the model group showed an increasing trend, but there was no significant difference compared with other group (p > 0.05). However, there was significant difference between the model group and the control group in Firmicutes level (p < 0.05).
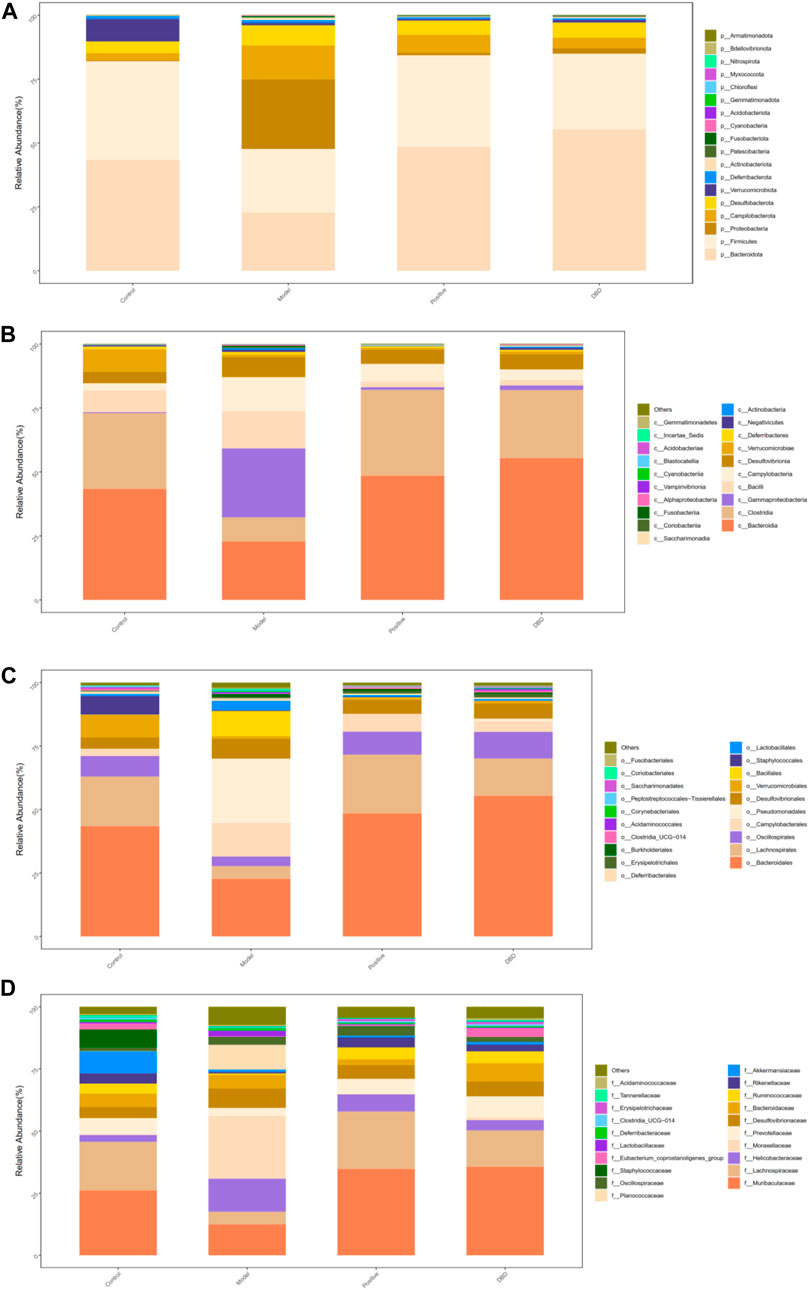
Figure 9. Gut microbiota composition. (A) phylum level; (B) class level; (C) order level; (D) family level.
At the class level (Figure 9B), Bacteroidia, Clostridia, Gammaproteobacteria, Bacilli and Campylobacteria are the main bacteroidia. There were significant differences between the model group and the other groups in Bacteroidia, Gammaproteobacteria and Clostridia (p < 0.05).
At the order level (Figure 9C), the dominant bacteria are mainly composed of Bacteroidales, Lachnospirales, Oscillospirales, Campylobacterales and Pseudomonadales. Compared with the control group, positive group and DBD group the model group had significantly differences in Bacteroidales and Pseudomonadales. There were significant differences in Lachnospirales level between the model group and the control group (p < 0.05).
At the family level (Figure 9D), Muribaculaceae, Lachnospiraceae, Helicobacteraceae, Moraxellaceae and Prevotellaceae are the main bacteroidia. Compared with control group, positive group and DBD group, the difference of model group in Moraxellaceae family level was statistically significant (p < 0.05). There was significant difference in Lachnospiraceae between the model group and the control group (p < 0.05).
3.9 Multivariate statistical analysis of DBD on IS mice
The LDA distribution bar chart (Figure 10A) shows the species with LDA scores greater than the set value with differences, i.e., the biomaker with statistical differences. LEfSe analysis (Figure 10B) was used to detect different strains between groups. As can be seen from Figure 10A, a total of 33 taxonomic groups of different levels were identified in this experiment, and they had different degrees of richness in control group, model group, positive group and DBD group. Among them, in the control group, the main bacteria species were Acidobacteriales and Family_XIIIUCG_001. The model group was composed of Clostridiales and Clostridiaceae. The number of taxa in the positive group was the lowest, and the most significant species were Actinomyces and Prevotella_shahii. Bacteroidota, Bacteroidia and Bacteroidales were the most abundant species in the positive group. As can be seen from Figure 10B, Acidobacteriales and Acidobacteriae, Clostridiaceae and Clostridiales are significantly difference and play an important role in the control group and the model groups, respectively. The positive groups had four significant differences played an important role bacteroidia, there are Actinomycetaceae, Actinomycetales, Bacillaceae and Sporolactobacillaceae. And DBD groups were Bacteroidales, Bacteroidia, Saccharimonadaceae, Saccharimonadales, Saccharimonadia and Eubacterium_coprostanoligene.
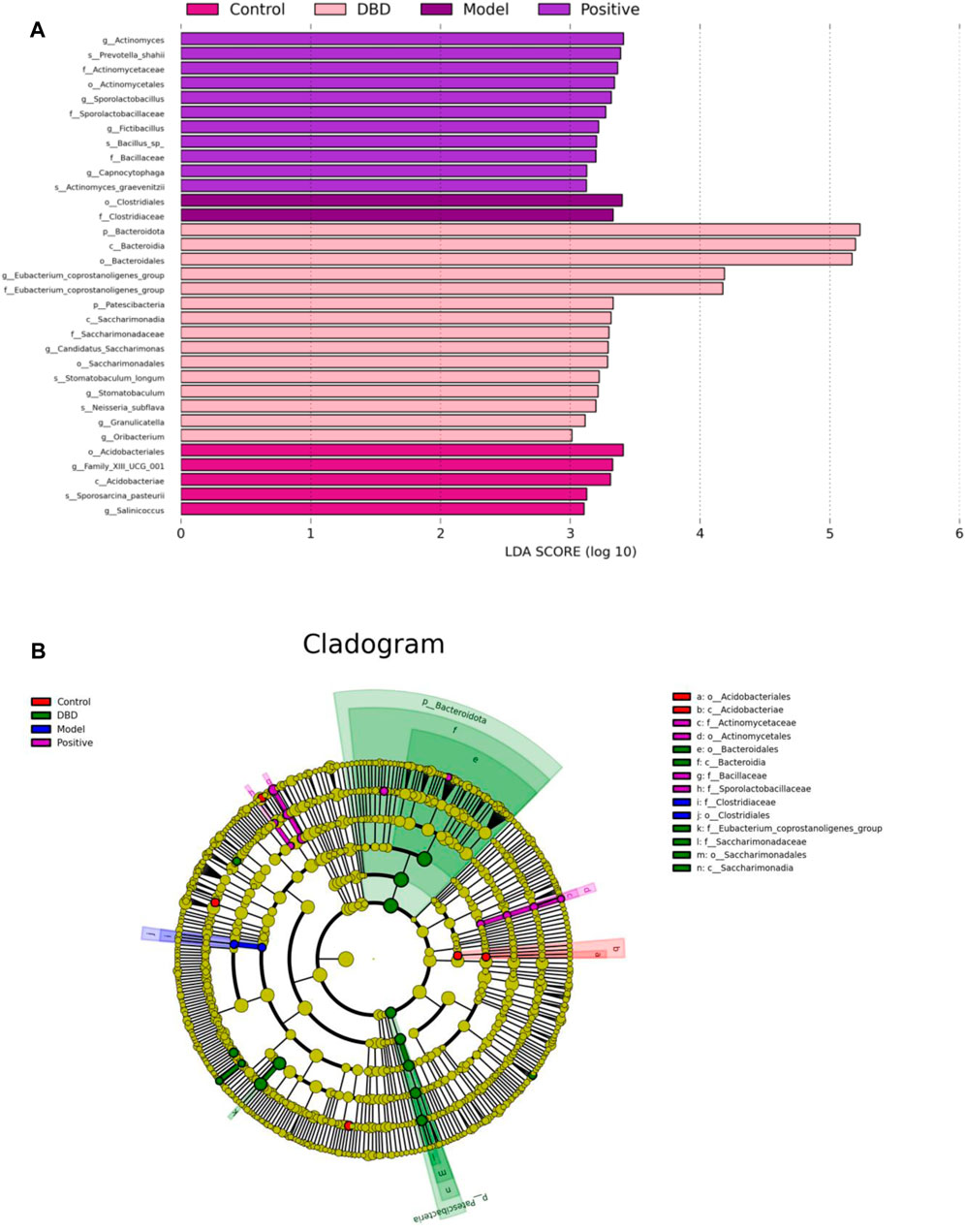
Figure 10. LEfSe statistical difference analysis. (A) LDA distribution bar graph; (B) LEfSe branching diagram.
4 Discussion
The immune system is a complex network of immune organs such as the spleen and thymus. As a natural barrier of the body, the immune system has the role of immune surveillance and defense. The intestinal barrier can eliminate the invasion of products and maintaining body health and homeostasis (Elmassry et al., 2022; Yan et al., 2022). Gut microbiota is a complex and changeable microcosm that inhabits the gut and participates in host immune regulation, protects the intestinal barrier and affects the functioning of the body. Many studies have shown that gut microbes are a key component of the gut barrier and play a key role in the development and regulation of immune function (Li et al., 2022; Yao et al., 2023b).
In this study, the weight and thymus index of CY-induced mice were decreased, which was consistent with the results of Chen et al. (Chen et al., 2021). It was proved that the CY induced IS model was successfully established in this experiment. Different from Liu et al. (Liu et al., 2022), this experiment found that spleen index of all experimental mice increased after injection of CY, which may be due to pathological enlargement of spleen caused by CY. The mechanism may be that the immunosuppressive effect of CY obstructs the development of thymus and the proliferation, differentiation and maturation of T lymphocytes (Xu et al., 2023). In the recovery period, the spleen showed obvious compensatory hyperplasia (Zhao et al., 2013). The ratio of CD4+ and CD8+ cells is one of the important indexes to evaluate the immune function of the body. The results of this experiment showed that intraperitoneal injection of CY in mice could cause the imbalance of T lymphocyte subsets in the animal body, resulting in cellular immune disorder, while DBD could increase the number of lymphocytes, and make CD4+/CD8+ close to normal by regulating the number of CD3+, CD4+ and CD8+ cells, so as to maintain the balance of immune function in the body.
CY is a widely used chemotherapy drug, and many studies have shown that it can damage intestinal mucosa (Chen et al., 2022). It can cause significant shortening of intestinal villi length, while crypt cells secrete a large number of immature cells in a short time when intestinal villi are destroyed, resulting in increased crypt depth (Liu et al., 2022). In this study, we confirmed that CY can cause villi damage in mice ileum tissue. The results of this study showed that DBD could improve the intestinal injury caused by CY to a certain extent, reduce the depth of crypts, increase the length of villi and V/C value, and make the intestinal mechanical barrier structure tend to be complete. Studies have shown that down-regulating the expression of ZO-1, Occludin and Claudin-1 can increase intestinal permeability and lead to enteric-related diseases (Al-Sadi et al., 2011; Lynn et al., 2020). The results of this study are similar, therefore, the improvement of intestinal physical barrier function by DBD may be related to the upregulation of mRNA and protein expression levels of ileum tight junction protein ZO-1. Previous studies have shown that MUC-2 is an important component of intestinal mucin layer (Okumura and Takeda, 2017; Cai et al., 2022), which can resist the invasion of enteroviruses, and its absence will affect mucosal repair. This explains the results of the model group of this experiment, CY can downregulate the expression level of intestinal MUC-2 mRNA, while DBD significantly upregulates the expression level of MUC-2 mRNA, indicating that DBD enhances the function of intestinal chemical barrier in mice to a certain extent. The SIgA results were consistent with the conclusion proved by Cai et al. (Cai et al., 2022) in vivo and in vitro experiments, which showed that spicosaccharide could enhance the secretion of SIgA to restore CY and lead to the decline of immune function. The results of this study showed that DBD increased the expression level of IgA and the concentration of SIgA in the ileum, thereby improving the function of intestinal immune barrier in IS mice.
Gut microbes are a key component of the gut barrier and play a key role in the development and regulation of immune function (Li et al., 2019; Tang et al., 2022). Intestinal microecological disturbance caused by changes in gut microbiota diversity, composition, structure and function will have a negative impact on the body and induce inappropriate immune activation. Therefore, the search for drugs that can regulate gut flora has important implications for body function. Many studies have shown that Chinese herbal medicines and their extracts have positive regulatory effects on the gut microbiota of animals (Pan et al., 2022; Wu et al., 2022). The high diversity of gut microbiota is conducive to the maintenance of intestinal stability. Alpha diversity analysis of intestinal species can reflect the richness and diversity of microbial community, and the decrease of richness and diversity is often associated with abnormal immune function (Tang et al., 2018). In this study, it was found that CY could lead to the decrease of Chao 1 index, Simpson index and Shannon index of cecal microflora in mice, which was similar to the results of Cui et al. (Cui et al., 2022a), that is, the diversity and richness of intestinal microflora in cecal contents decreased (Che et al., 2022; Cui et al., 2022b). At the phylum level, Firmicutes and Bacteroides, as the main dominant phyla, accounted for the highest relative abundance ratio in the gut microbiota detected in this study, and were the main components of the gut microbiota. Some of the bacteria in Firmicutes can enhance the immunity of the body by promoting the fermentation of polysaccharides and cellulose respectively. Bacteroidetes is one of the important groups of bacteria that maintain intestinal microbial homeostasis in animals. When Bacteroidetes change, its related effects will also change. An elevated ratio of Firmicutes to Bacteroidetes (F/B) is often considered a criterion for gut microbiota dysregulation. In this study, DBD can reduce the ratio of Firmicutes to Bacteroidetes, possibly by increasing Bacteroidetes and thereby decreasing F/B value. Bacteroidetes, as beneficial bacteria for intestinal colonization, plays an important role in inhibiting the proliferation of intestinal bacteria and producing short-chain fatty acids. Therefore, the increase of Bacteroidetes by DBD plays a beneficial role in the health of gut microbiota. At the family level, Lachnospiraceae can indirectly promote the production of short-chain fatty acids and play an anti-inflammatory and immunomodulatory role. The results of this study showed that compared with the blank control group, the content of Lachnospiraceae bacteria in the model group was significantly reduced. Studies have shown that Trichospirillaceae is one of the families that produce butyrate, which has many positive properties as it represents an important source of nutrients for intestinal cells, stimulates intestinal mucin production, improves tight junction protein integrity, and reduces inflammation. Thus, we speculate that CY may reduce butyrate production by decreasing Trichomillaceae, which leads to a decrease in inflammatory response as well as in mucin and tightenin.
The results of different levels of gut microbiota in mice showed that there were statistical differences between the model group and the control group, the positive group and the DBD group in Bacteroidota, Bacteroidia and Bacteroidales. According to the diversified statistical analysis, the significant differences in the groups of DBD were Bacteroidota, Bacteroidia and Bacteroidales. Therefore, we speculated that DBD may restore the balance of gut microbiota by regulating the level of phyla, class and order of Bacteroides.
5 Conclusion
In this study, DBD alleviated CY-induced immune damage by increasing body weight and thymus index and decreasing the ratio of spleen index to CD4+/CD8+ of T lymphocyte subsets. And the intestinal barrier function of mice was by improves improving the intestinal morphology of the ileum and up-regulating the expression levels of ZO-1, MUC-2 and SIgA. DBD regulates CY-induced gut microbiota dysregulation in mice by increasing species diversity and richness, regulating the phylum, class and order levels of Bacteroidetes. In conclusion, DBD can protect gut health by regulating intestinal mucosal barrier and gut microbiota structure in mice (Figure 11).
Data availability statement
The data presented in the study are deposited in the NCBI repository, accession number PRJNA1147448.
Ethics statement
The animal study was approved by Laboratory Animal Ethics Committee of Southwest University. The study was conducted in accordance with the local legislation and institutional requirements.
Author contributions
HH: Data curation, Formal Analysis, Methodology, Writing–original draft. YX: Data curation, Visualization, Writing–review and editing. XL: Formal Analysis, Investigation, Writing–review and editing. FG: Writing–review and editing. PY: Formal Analysis, Writing–review and editing. YL: Formal Analysis, Writing–review and editing. LZ: Methodology, Writing–review and editing. HD: Data curation, Writing–review and editing. SB: Formal Analysis, Writing–review and editing. LC: Funding acquisition, Project administration, Supervision, Writing–review and editing.
Funding
The author(s) declare that financial support was received for the research, authorship, and/or publication of this article. This work was supported by National Center of Technology Innovation for Pigs (NCTIP-XD/C17), Chongqing Technology Innovation and Application Development Special Key Project (cstc2019jsccx-lyjsBX0003 and cstc2021jscx-lyjsAX0008) and Natural Science Foundation project of Chongqing Science and Technology Bureau (CSTB2022NSCQ-MSX0470).
Conflict of interest
The authors declare that the research was conducted in the absence of any commercial or financial relationships that could be construed as a potential conflict of interest.
Publisher’s note
All claims expressed in this article are solely those of the authors and do not necessarily represent those of their affiliated organizations, or those of the publisher, the editors and the reviewers. Any product that may be evaluated in this article, or claim that may be made by its manufacturer, is not guaranteed or endorsed by the publisher.
References
Al-Sadi, R., Khatib, K., Guo, S., Ye, D., Youssef, M., and Ma, T. (2011). Occludin regulates macromolecule flux across the intestinal epithelial tight junction barrier. Am. J. physiology. Gastrointest. liver physiology 300 (6), G1054–G1064. doi:10.1152/ajpgi.00055.2011
Cai, G., Yang, Y., Gu, P., Li, K., Adelijiang, W., Zhu, T., et al. (2022). The secretion of sIgA and dendritic cells activation in the intestinal of cyclophosphamide-induced immunosuppressed mice are regulated by Alhagi honey polysaccharides. Phytomedicine Int. J. phytotherapy Phytopharm. 103, 154232. doi:10.1016/j.phymed.2022.154232
Che, Q., Luo, T., Shi, J., He, Y., and Xu, D. (2022). Mechanisms by which traditional Chinese medicines influence the intestinal flora and intestinal barrier. Front. Cell. Infect. Microbiol. 12, 863779. doi:10.3389/fcimb.2022.863779
Chen, T., Shen, M., Yu, Q., Chen, Y., Wen, H., Lu, H., et al. (2022). Purple red rice anthocyanins alleviate intestinal damage in cyclophosphamide-induced mice associated with modulation of intestinal barrier function and gut microbiota. Food Chem. 397, 133768. doi:10.1016/j.foodchem.2022.133768
Chen, X., Sun, W., Xu, B., Wu, E., Cui, Y., Hao, K., et al. (2021). Polysaccharides from the roots of Millettia speciosa champ modulate gut health and ameliorate cyclophosphamide-induced intestinal injury and immunosuppression. Front. Immunol. 12, 766296. doi:10.3389/fimmu.2021.766296
Cui, Y., Sun, W., Li, Q., Wang, K., Wang, Y., Lv, F., et al. (2022a). Effects of caulis spatholobi polysaccharide on immunity, intestinal mucosal barrier function, and intestinal microbiota in cyclophosphamide-induced immunosuppressive chickens. Front. Vet. Sci. 9, 833842. doi:10.3389/fvets.2022.833842
Cui, Y., Zhang, L., Lu, C., Dou, M., Jiao, Y., Bao, Y., et al. (2022b). Effects of compound small peptides of Chinese medicine on intestinal immunity and cecal intestinal flora in CTX immunosuppressed mice. Front. Microbiol. 13, 959726. doi:10.3389/fmicb.2022.959726
Elmassry, M. M., Zayed, A., and Farag, M. A. (2022). Gut homeostasis and microbiota under attack: impact of the different types of food contaminants on gut health. Crit. Rev. Food. Sci. Nutr. 62 (3), 738–763. doi:10.1080/10408398.2020.1828263
Feng, L., Lijia, Z., Xi, F. A. I. S., Wen, H., and Ying, L. (2021). Immunomodulatory activity of carboxymethyl pachymaran on immunosuppressed mice induced by cyclophosphamide. Molecules 26 (19), 5733. doi:10.3390/molecules26195733
Han, Y., Huang, J., Zhao, C., Zhang, F., Gu, Y., Wang, C., et al. (2023). Hericium erinaceus polysaccharide improves the microstructure, immune function, proliferation and reduces apoptosis of thymus and spleen tissue cells of immunosuppressed mice. Biosci. Biotechnol. Biochem. 87 (3), 279–289. doi:10.1093/bbb/zbac198
Huang, J., Huang, J., Li, Y., Wang, Y., Wang, F., Qiu, X., et al. (2021). Sodium alginate modulates immunity, intestinal mucosal barrier function, and gut microbiota in cyclophosphamide-induced immunosuppressed BALB/c mice. J. Agric. Food. Chem. 69 (25), 7064–7073. doi:10.1021/acs.jafc.1c02294
Kumar, V. P., and Venkatesh, Y. P. (2016). Alleviation of cyclophosphamide-induced immunosuppression in Wistar rats by onion lectin (Allium cepa agglutinin). J. Ethnopharmacol. 186, 280–288. doi:10.1016/j.jep.2016.04.006
Kwan, K. K. L., Dong, T. T. X., and Tsim, K. W. K. (2021). Danggui buxue Tang, a Chinese herbal decoction containing astragali radix and angelicae sinensis radix, improves mitochondrial bioenergetics in osteoblast. Phytomedicine Int. J. phytotherapy Phytopharm. 88, 153605. doi:10.1016/j.phymed.2021.153605
Li, C., Zhu, F., Wang, S., Wang, J., and Wu, B. (2021). Danggui buxue decoction ameliorates inflammatory bowel disease by improving inflammation and rebuilding intestinal mucosal barrier. Evidence-based complementary Altern. Med. eCAM. 2021, 8853141. doi:10.1155/2021/8853141
Li, X., Shen, L., and Ji, H. (2019). Astragalus alters gut-microbiota composition in type 2 diabetes mice: clues to its pharmacology. Diabetes, metabolic syndrome Obes. targets Ther. 12, 771–778. doi:10.2147/DMSO.S203239
Li, Y., Udayakumar, V., Sathuvan, M., Liu, Y., Liu, X., Zhang, Y., et al. (2022). Effects of laminarin zwitterionic carboxylate and sulfonate on the intestinal barrier function and gut microbiota. Carbohydr. Polym. 278, 118898. doi:10.1016/j.carbpol.2021.118898
Liu, H., Yang, H., Qin, Z., Chen, Y., Yu, H., Li, W., et al. (2021a). Exploration of the danggui buxue decoction mechanism regulating the balance of ESR and AR in the TP53-AKT signaling pathway in the prevention and treatment of POF. Evidence-based complementary Altern. Med. eCAM. 2021, 4862164. doi:10.1155/2021/4862164
Liu, J., Wang, J., Zhou, S., Huang, D., Qi, G., and Chen, G. (2022). Ginger polysaccharides enhance intestinal immunity by modulating gut microbiota in cyclophosphamide-induced immunosuppressed mice. Int. J. Biol. Macromol. 223 (Pt A), 1308–1319. doi:10.1016/j.ijbiomac.2022.11.104
Liu, Y., Ju, Y., and Qin, X. (2021b). Studies on the compatibility mechanism and material basis of Danggui Buxue Decoction against anemia mice using metabonomics and network pharmacology. J. Pharm. Pharmacol. 73 (6), 767–777. doi:10.1093/jpp/rgab016
Lynn, K. S., Peterson, R. J., and Koval, M. (2020). Ruffles and spikes: control of tight junction morphology and permeability by claudins. Biochimica biophysica acta. Biomembr. 1862 (9), 183339. doi:10.1016/j.bbamem.2020.183339
Nam, J. H., Choi, J., Monmai, C., Rod-In, W., Jang, A., You, S., et al. (2022). Immune-enhancing effects of crude polysaccharides from Korean ginseng berries on spleens of mice with cyclophosphamide-induced immunosuppression. J. Microbiol. Biotechnol. 32 (2), 256–262. doi:10.4014/jmb.2110.10021
Nan, H., Yue, Q., Tingyu, L., Yue, Z., Chang, L., Jinhui, W., et al. (2023). Immunomodulatory effects and mechanisms of Tiepishihu Xiyangshen granules on cyclophosphamide induced immuno-suppression via TLR4/MAPKs and PI3K/AKT/FOXO3a signal pathways. J. Ethnopharmacol. 307, 116192. doi:10.1016/j.jep.2023.116192
Okumura, R., and Takeda, K. (2017). Roles of intestinal epithelial cells in the maintenance of gut homeostasis. Exp. and Mol. Med. 49 (5), e338. doi:10.1038/emm.2017.20
Pan, L., Fu, T., Cheng, H., Mi, J., Shang, Q., and Yu, G. (2022). Polysaccharide from edible alga Gloiopeltis furcata attenuates intestinal mucosal damage by therapeutically remodeling the interactions between gut microbiota and mucin O-glycans. Carbohydr. Polym. 278, 118921. doi:10.1016/j.carbpol.2021.118921
Pan, Z., Chen, P., Wu, H., Wang, L., Xian, S., and Chen, Z. (2023). Mechanism of boosting qi,activating blood and soothing the liver method in treating psycho-cardiological disease:based on immuno-inflammatory regulation. J. Trad. Chin. Med. 64 (12), 1211–1217. doi:10.13288/j.11-2166/r.2023.12.006
Sudeep, H. V., Gouthamchandra, K., Ramanaiah, I., Raj, A., Naveen, P., and Shyamprasad, K. (2023). A standardized extract of Echinacea purpurea containing higher chicoric acid content enhances immune function in murine macrophages and cyclophosphamide-induced immunosuppression mice. Pharm. Biol. 61 (1), 1211–1221. doi:10.1080/13880209.2023.2244000
Tang, C., Sun, J., Zhou, B., Jin, C., Liu, J., Kan, J., et al. (2018). Effects of polysaccharides from purple sweet potatoes on immune response and gut microbiota composition in normal and cyclophosphamide treated mice. Food Funct. 9 (2), 937–950. doi:10.1039/c7fo01302g
Tang, Y., Pu, Q., Zhao, Q., Zhou, Y., Jiang, X., and Han, T. (2022). Effects of fucoidan isolated from laminaria japonica on immune response and gut microbiota in cyclophosphamide-treated mice. Front. Immunol. 13, 916618. doi:10.3389/fimmu.2022.916618
Wang, W., Fan, L., Ge, F., Li, Z., Zhu, J., Yin, K., et al. (2022). Effects of Danggui Buxue decoction on host gut microbiota and metabolism in GK rats with type 2 diabetes. Front. Microbiol. 13, 1029409. doi:10.3389/fmicb.2022.1029409
Wu, R., Shen, Q., Li, P., and Shang, N. (2022). Sturgeon chondroitin sulfate restores the balance of gut microbiota in colorectal cancer bearing mice. Int. J. Mol. Sci. 23 (7), 3723. doi:10.3390/ijms23073723
Xiang, Z., Xin, X., Pei, Z., Xiang, W., Zhaoliang, H., Wenrui, Z., et al. (2023). Crude polysaccharide from Danggui Buxue decoction enhanced the anti-tumor effect of gemcitabine by remodeling tumor-associated macrophages. Int. J. Biol. Macromol. 242 (P4), 125063. doi:10.1016/j.ijbiomac.2023.125063
Xu, Q., Yang, W., Wang, Y., Wu, F., Dai, J., Qin, M., et al. (2023). Study on immunomodulatory effect of prepared radix rehmanniae polysaccharide on cyclophosphamide-induced immunosuppression in mice. China Animal Husb. and Veterinary Med. 50 (02), 693–703. doi:10.16431/j.cnki.1671-7236.2023.02.027
Yan, A., Ding, H., Liu, J., Bi, C., Han, Z., Wang, Z., et al. (2022). Black Lycium barbarum polysaccharide attenuates LPS-induced intestine damage via regulation gut microbiota. Front. Microbiol. 13, 1080922. doi:10.3389/fmicb.2022.1080922
Yao, J., Liu, J., He, Y., Liu, L., Xu, Z., Lin, X., et al. (2023a). Systems pharmacology reveals the mechanism of Astragaloside IV in improving immune activity on cyclophosphamide-induced immunosuppressed mice. J. Ethnopharmacol. 313, 116533. doi:10.1016/j.jep.2023.116533
Yao, S., Yang, X., Wu, W., Jiang, Q., Deng, S., Zheng, B., et al. (2023b). Effect of Paecilomyces cicadae polysaccharide Pc0-1 on cyclophosphamide-induced immunosuppression and regulation of intestinal flora in mice. Food Biosci. 51, 102340. doi:10.1016/j.fbio.2022.102340
Yu, Y., Mo, S., Shen, M., Chen, Y., Yu, Q., Li, Z., et al. (2021). Sulfated modification enhances the immunomodulatory effect of Cyclocarya paliurus polysaccharide on cyclophosphamide-induced immunosuppressed mice through MyD88-dependent MAPK/NF-κB and PI3K-Akt signaling pathways. Food Res. Int. 150, 110756. doi:10.1016/j.foodres.2021.110756
Zhang, Q., Tang, X., Zhang, X., He, Y., and Li, Y. (2023). Immunomodulatory effect of two hydrolysates of chitin on cyclophosphamide-induced mice via immune response enhancement and gut microbiota modulation. J. Funct. Foods. 109, 105799. doi:10.1016/j.jff.2023.105799
Zhao, T., Mao, G., Mao, R., Zou, Y., Zheng, D., Feng, W., et al. (2013). Antitumor and immunomodulatory activity of a water-soluble low molecular weight polysaccharide from Schisandra chinensis (Turcz.) Baill. Food Chem. Toxicol. Int. J. Publ. Br. Industrial Biol. Res. Assoc. 55, 609–616. doi:10.1016/j.fct.2013.01.041
Keywords: Danggui buxue decoction, immunosuppression, intestinal mucosal immunity, gut microbiota, immune function
Citation: Huang H, Xie Y, Li X, Gui F, Yang P, Li Y, Zhang L, Du H, Bi S and Cao L (2024) Danggui Buxue decoction regulates the immune function and intestinal microbiota of cyclophosphamide induced immunosuppressed mice. Front. Pharmacol. 15:1420411. doi: 10.3389/fphar.2024.1420411
Received: 20 April 2024; Accepted: 30 July 2024;
Published: 19 August 2024.
Edited by:
Karl Tsim, Hong Kong University of Science and Technology, Hong Kong SAR, ChinaReviewed by:
Jiaguo Liu, Nanjing Agricultural University, ChinaZhe Ren, Fujian Agriculture and Forestry University, China
Copyright © 2024 Huang, Xie, Li, Gui, Yang, Li, Zhang, Du, Bi and Cao. This is an open-access article distributed under the terms of the Creative Commons Attribution License (CC BY). The use, distribution or reproduction in other forums is permitted, provided the original author(s) and the copyright owner(s) are credited and that the original publication in this journal is cited, in accordance with accepted academic practice. No use, distribution or reproduction is permitted which does not comply with these terms.
*Correspondence: Liting Cao, caoliting@swu.edu.cn