- 1College of Pharmacy, Heilongjiang University of Chinese Medicine, Harbin, China
- 2First Affiliated Hospital, Heilongjiang University of Chinese Medicine, Harbin, China
- 3College of Jiamusi, Heilongjiang University of Chinese Medicine, Jiamusi, China
Silybum marianum (L.) Gaertn, a herbaceous plant with a long history in traditional medicine for the treatment of hepatobiliary diseases, particularly in Europe, which has attracted attention for its remarkable therapeutic effect. This review systematically summarizes the research progress in the botany, phytochemistry, pharmacology, comprehensive utilization and synthetic biology of S. marianum. Up to now, more than 20 types of flavonolignan components have been isolated from S. marianum. In addition, the rearch on fatty acids and triterpenoids is also constantly improving. Among them, silybin is the most active compound in flavonolignans components. Its pharmacological effects in vivo and in vitro include anti-inflammatory, antioxidant, anti-tumour, hypoglycaemic, neuroprotective and immunoregulatory properties. The use of coniferyl alcohol and taxifolin as substrates to produce silybin and isosilybin under the action of enzyme catalysis is the commonly used biosynthetic pathway of silymarin, which provides support for a comprehensive analysis of the synthetic pathway of silymarin. In addition to medicinal use, the extracts of plants also have broad application prospects in the production of food, healthcare products, cosmetics and other aspects. In addition, the chemical composition, pharmacological mechanism and synthetic biology of S. marianum need to be further studied, which is very important for its clinical efficacy and resource development.
1 Introduction
Silybum marianum (L.) Gaertn is an annual or biennial herb of the genus Silybum in the family Asteraceae. It is native to the southern Europe, Asia Minor and northern Africa (Morazzoni and Bombardelli, 1995; Marmouzi et al., 2021). We obtained the geographical distribution of S. marianum in the world from the GBIF online database (www.gbif.org). (Figure 1). This plant is able to adapt to harsh environments such as cold (zone 8b), drought, salinity (Martinelli, 2019; Papadimou and Golia, 2024).
The achenes of S. marianum are bitter taste, cool nature, and have the effects of clearing heat, protecting liver and gallbladder. It can be utilized for the treatment of jaundice, damp heat of the liver and gallbladder, as well as other related conditions (Committee, 2020; Yu et al., 2023). The chemical constituents found in these achenes mainly consist of flavonolignans, terpenoids, and oil, with flavonolignans representing the largest proportion (Pferschy-Wenzig et al., 2023). Silymarin is a group of flavonolignans including silybin, isosilybin, silydianin, and silychristin, which are formed through the dehydration-condensation process of dihydroflavonols and phenylpropanoid derivatives to produce flavonolignans compounds (Biedermann et al., 2014). Silymarin exhibit hepatoprotective, anticancer, antioxidant, anti-inflammatory, hypoglycemic, neuroprotective, cardioprotective, and immunomodulatory effects (Karimzadeh et al., 2024; Ma et al., 2024). S. marianum has been used as a medicinal plant for thousands of years and was first recorded for use in liver protection and venomous snake bites (Morazzoni and Bombardelli, 1995). With the development of modern medicine, the mechanism of action of silymarin has become clearer. Silymarin can resist liver cell fibrosis, protect the liver cell membrane, and promote the repair or regeneration of liver cells when used as a hepatoprotective drug (de Avelar et al., 2023).
When utilized as an anti-cancer medicines, silymarin can block the cell cycle of tumor cells, typically in the G1 phase, and induce apoptosis through multiple mechanisms (Faixová et al., 2023; Mao et al., 2023). As research and applications of active ingredients like silymarin continue to expand and gain traction, traditional extraction methods are struggling to keep up with demand (Yang et al., 2020). The use of medicinal plants for multiple purposes can result in higher economic returns. In addition to medicinal use, S. marianum can also be used for oil extraction, a raw material for protein powder, animal fodder, and honey, which making S.marianum highly versatile and enhancing economic value (Bencze-Nagy et al., 2023).
With the continuous deepening of research on this medicinal plant, more and more effective components have been found and isolated. In addition to medicinal use, more utilization value of plants is also constantly being discovered. However, there are still some omissions in the research of S. marianum. For example, many of the active ingredients needed to be proven in S. marianum that have not yet been isolated and it is necessary to explore the biological functions of various active ingredients, meanwhile, it is absolutely imperative that using science and technology to develop higher value-added. In this review, the botany, phytochemistry, pharmacology, synthetic biology and comprehensive utilization of S. marianum in recent years are summarized comprehensively and deeply, which provides novel idea for further clinical application and resource development of S. marianum.
2 Methodology
For this review, a comprehensive literature search was conducted up to 24 February 2024. Most of the literature research was conducted through the following five online scientific databases: PubMed, Google Scholar, SciFinder, Web of Science and CNKI. The keywords used to search were: “Silybum marianum,” “Phytochemistry,” “Pharmacology,” “Synthetic biology,” and “Comprehensive utilization”. In addition, the names of all phytochemical compounds were used in the search. The review also included results from the Flora of China (http://www.iplant.cn), and relevant conference proceedings written in English and Chinese. And the chemical structures were accurately depicted using the KingDraw software.
3 Botanical characteristics
S. marianum is an annual or biennial herbaceous plant with a height of 1–2 m (Figure 2). The stems are erect, striped, multi-branched, with sparse fine hairs or hairless. The rosette basal leaves and the lower stems and leaves have petioles. The leaf shape is oval or inverted lanceolate, about 0.5 m long and 0.3 m wide, and the leaf margin shape is plumose shallow lobes, deep lobes or full lobes; the stems and leaves in the middle and upper parts are small, the leaf shape is lanceolate or long ovate, the leaf margin is pinnate shallow crack and the edge is shallow wavy round tooth crack, the base is gradually pointed and heart-shaped, the upper stems and leaves are small, undivided, lanceolate, and the base amplexicaul shows heart-shape. Variegated green and white are glabrous, the texture of the leaves is thin, and the edges have hard yellow needles with about 5 mm long. he plant has a large capitulum, and the bracts are 3–5 cm long, spherical or ovoid. The middle and outer layers of the entire bracts are oval to lanceolate, with needles on the top and the edge, and no needles on the edge of the base. The upper part may have hard attachments, and their edges and bases have sharp spines, about 1–2 mm long, and the sharp spines at the top are about 5 mm long; the bracts of the inner layer are lanceolate, about 25–30 mm long. Bracts have no acicular edges, no apical attachments, and apex is pointed. The whole bract does not grow fluff, and the texture of the middle and outer bracts are hard and leathery. The flowers are mainly red-purple, with a small amount of white, about 30 mm long, and the thin tube section is about 20 mm long. The filament is thick and short. The achenes are flattened, brownish, and finely ellipsoidal, about 6–8 mm long and 2–3 mm wide, with dark brown spots or stripes. The upper part of the achenes have a edge, which has no serrations. The flowering and fruiting period is from May to August.
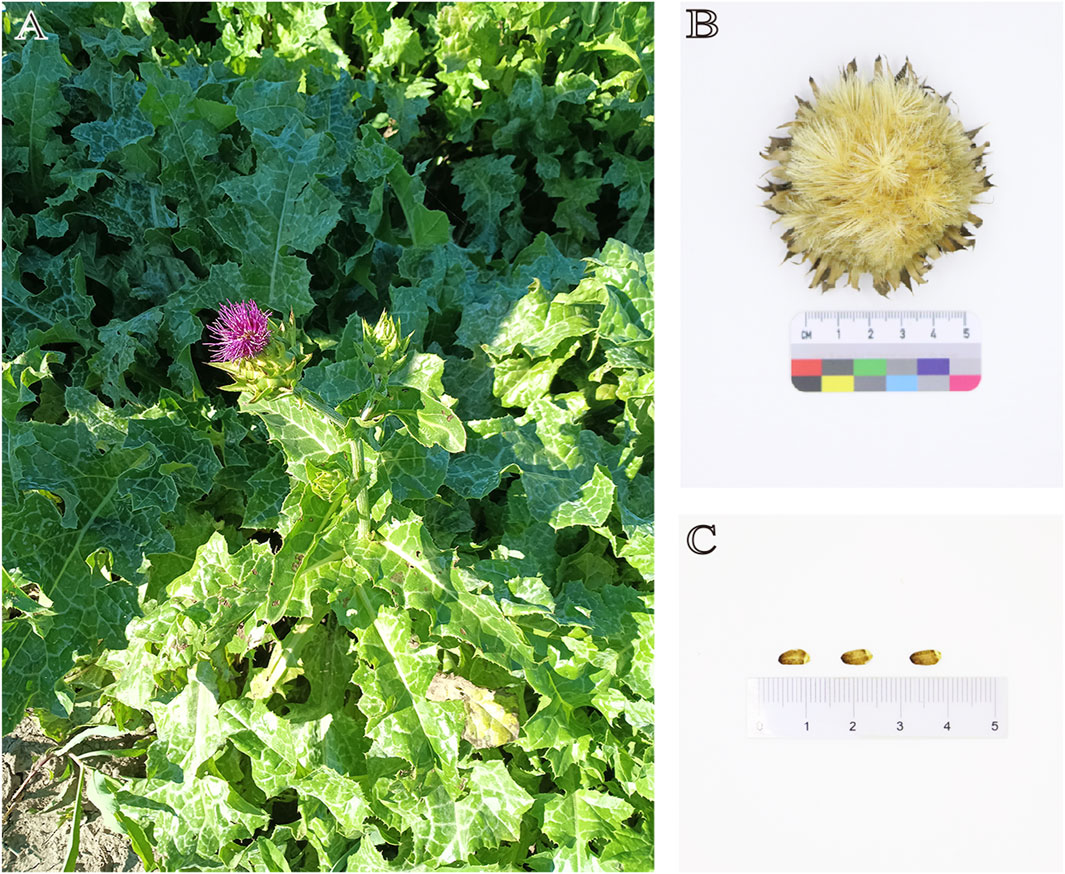
Figure 2. (A) The plant of S. marianum; (B) Dried flower of S. marianum; (C) Achenes of S. marianum.
4 Phytochemistry
4.1 Flavonoids
Silymarin is a mixture of several flavonolignans including silybin, dehydrosilybin, isosilybin, silydianin, and silicristin, among which silybin having the highest content of these compounds (Csupor et al., 2016) (Figure 3). In 1968, Wagner et al. were the first to isolate a pharmacologically active silymarin constituent from achenes of S. marianum (Wagner et al., 1968). In the same year, Pelter et al. first isolated silybin (Pelter and Hänsel, 1968). Silicristin, the second component of S. marianum was discovered by Wagner et al., in 1971 (Wagner et al., 1971) and its structure was determined 3 years later (Pelter et al., 1977). In 1976, silydianin was reported by Wagner et al. (Wagner et al., 1976). The regional isomer of silydianin, isosilydianin, was first reported by Arnone et al., in 1979 (Arnone et al., 1979). Subsequently, isosilicristin was reported by Kaloga et al., in 1981 (Kaloga, 1981). In 2003, the corresponding isomers of silybins A, silybins B, isosilybin A, and isosilybin B were isolated. Achenes of S. marianum contain silicristin A, silicristin B, 2,3-cis-silybin A, 2,3-cis-silybin B, and more than 20 types of flavonolignans. In addition, S. marianum contains flavonoids component such as quercetin, saccharin, naringenin, flavanolignans, kaempferol, and dihydropyran-4-one, among others (Begum et al., 2010).
4.2 Oil compounds
Oil compounds in achenes of S. marianum account for a large proportion, about 25%∼30%, of which linoleic acid content is about 46.46% ± 0.26% (Chen and Wang, 1998; Zhang, 2011) (Figure 4). There are some differences in the types and contents of fatty acids in S. marianum of different producing areas. For instance, the content of grease in achenes of S. marianum cultivated in Egypt is about 35%. In addition, behenic acid and arachidic acid were isolated. Stearic acid and myristic acid have also been isolated from the achenes of S. marianum that produced in India (Zarrouk et al., 2019).
4.3 Others
Achenes of S. marianum also contains about 20% protein and 30% starch. S. marianum also contains a small amount of triterpenoids, dozens of polyacetylene and polyolefin compounds, alkaloids and sterols (Chen and Wang, 1998; Begum et al., 2010; MacDonald-Ramos et al., 2021; Javeed et al., 2022).
5 Pharmacological activity
5.1 Liver effects
5.1.1 Treatment of liver damage caused by Alcohol
Alcoholic liver disease often presents oxidative stress, inflammation, liver injury, and liver fibrosis (Stolf et al., 2017). Alcohol causes an imbalance in hepatic lipid synthesis, loss, and degradation, leading to the formation of fatty liver (Ball and Kowdley, 2005). Alcohol can produce the more toxic acetaldehyde through its metabolism. These two substances degrade the ferritin proteins in rat hepatocytes, thereby releasing free Fe2+. This process inhibits the expression of the Glutathione peroxidase 4 protein, reduces mitophagy, and increases iron death. Silymarin reduces the level of malondialdehyde, reactive oxygen species (ROS), Fe2+, and maintains a normal number of mitochondria. This helps to reduce hepatocyte apoptosis and protect the liver (Rambaldi et al., 2007).
5.1.2 Protecting liver cell membranes
Silymarin protects liver cell membranes by inhibiting lipid peroxidation, which maintains fluidity. It also prevents the specific binding of mycotoxins, such as ghost penitoxin peptide and α-goitrogens, to receptors on liver cell membranes. This inhibits damage to cell membranes, restrains transmembrane transport of toxins, and blocks hepatic-intestinal recycling of toxins, thus enhancing the resistance of the liver cell membranes (Saller et al., 2008; Abenavoli et al., 2010). Studies have shown that silymarin can restore the increase in superficial fluidity of hepatic microsomal and mitochondrial membranes induced by carbon tetrachloride, as well as the decrease in deep fluidity (Abenavoli et al., 2018).
5.1.3 Anti-hepatic fibrosis
Liver fibrosis is typically initiated by inflammation of liver tissue and necrosis of liver cells (Saller et al., 2001; Abenavoli et al., 2010). Previous research has demonstrated that pre-collagen type III peptide (PIIIP) is a reliable indicator of the severity of hepatic fibrosis. Silymarin treatment decreases serum PIIIP levels. Silymarin may inhibit hepatic fibrosis by reducing reactive oxygen species activity and mitigating hepatocellular injury and liver tissue inflammation (Abenavoli et al., 2018; Zhai et al., 2019).
5.1.4 Promoting the repair and regeneration of hepatocytes
Silymarin specifically binds and activates the estradiol receptor in hepatocytes, which enhances the activity of RNA polymerase I in the nucleus and promotes the transcription of ribosomal RNA. An increase in the number of ribosomes promotes the synthesis of structural proteins and enzymes and indirectly promotes DNA synthesis, contributing to the repair and regeneration of hepatocytes (Flora et al., 1998) (Figure 5).
5.2 Anti-cancer effects
5.2.1 Hepatocellular carcinoma
Hepatocellular carcinoma is the most common primary cancer and is one of the leading causes of cancer-related deaths worldwide. It can occur due to various reasons, including alcohol consumption, fatty liver disease, chronic liver disease, and viral hepatitis B or A. Hepatocellular carcinoma develops gradually through genomic alterations that alter the hepatocyte morphology. The cells progress from an intermediate form to cancerous cells over time (Marengo et al., 2016).
Research has shown that silymarin can effectively decrease the rat liver Lipoperoxides (LOP) content, increase the liver Glutathione (GSH) content, and protect the liver from oxidative stress (Ramasamy and Agarwal, 2008). Additionally, the reduction in LOP content stabilizes the permeability of the liver membrane, which helps to maintain the antioxidant capacity of the liver and the level of ribosomal RNA synthesis (Kwon et al., 2013). Silymarin significantly reduces the expression of the proliferation marker Proliferation cell nuclear antigen (Ki-67) in liver tissue. It also prevents the elevation of serum tumor markers such as alpha fetoprotein and carcinoembryonic antigen in rats and inhibits the occurrence of hepatocellular carcinoma (Pradhan and Girish, 2006). Overexpression of Hepatocyte growth factor (HGF) and Cellular-mesenchymal epithelial transition factor (c-Met) occurred after stimulation of hepatocellular carcinoma cells (HCC) with Tetrachloromethane and Diethylnitrosamine. The combination of HGF and c-Met leads to the phosphorylation of downstream effectors, such as PI3K/Akt, RAS/MAPK, nonreceptor tyrosine kinase, and Focal adhesion kinase, which promotes the survival, proliferation, invasion, and metastasis of HCC (Han et al., 2019). Silymarin inhibits the extracellular binding of HGF to c-Met by downregulating c-Met on the cell membrane and inhibiting HGF expression. This leads to inhibition of tumor cell growth, proliferation, metastasis, and other processes (Lau et al., 2016).
Overexpression of PI3K/Akt induces intrahepatic metastasis in hepatocellular HCC and vascular invasion (Rosário and Birchmeier, 2003). PI3K is converted from phosphatidylinositol bisphosphate to phosphatidylinositol trisphosphate through its binding of Phosphatidylinositol-3-kinase (PI3K) to articulin via SH2/SH3 structural domains. Akt is then phosphorylated, which in turn phosphorylates several cellular target proteins, including mTOR and glycogen synthase kinase 3, ultimately promoting cell cycle progression (Shaw and Cantley, 2006).
Activation of the PI3K/Akt/mTOR pathway contributes to HCC progression in liver fibrosis and hepatocellular carcinoma cells. Silymarin binds to enzymes of the PI3K family, thereby inhibiting the activation of the Akt and mTOR families (Peng et al., 2017). The production and development of HCC are also related to the dysregulation of Wnt/β-catenin signaling pathway (Zhang M. et al., 2018). Activation of the Wnt/β-catenin signaling pathway inhibits the degradation of β-catenin, leading to its cytoplasmic accumulation and translocation to the nucleus. β-catenin also complexes with transcription factors such as TCF/Lef, which can activate downstream target genes associated with malignant tumor development, such as Recombinant Protein, Cancer-myc, and cyclin D, thereby stimulating cancer cell proliferation and metastasis (Dahmani et al., 2011). Silymarin inhibits the proliferation of HCC by reducing Wnt mRNA expression and downregulating the level of β-catenin protein (Nusse and Clevers, 2017). (Figure 6)
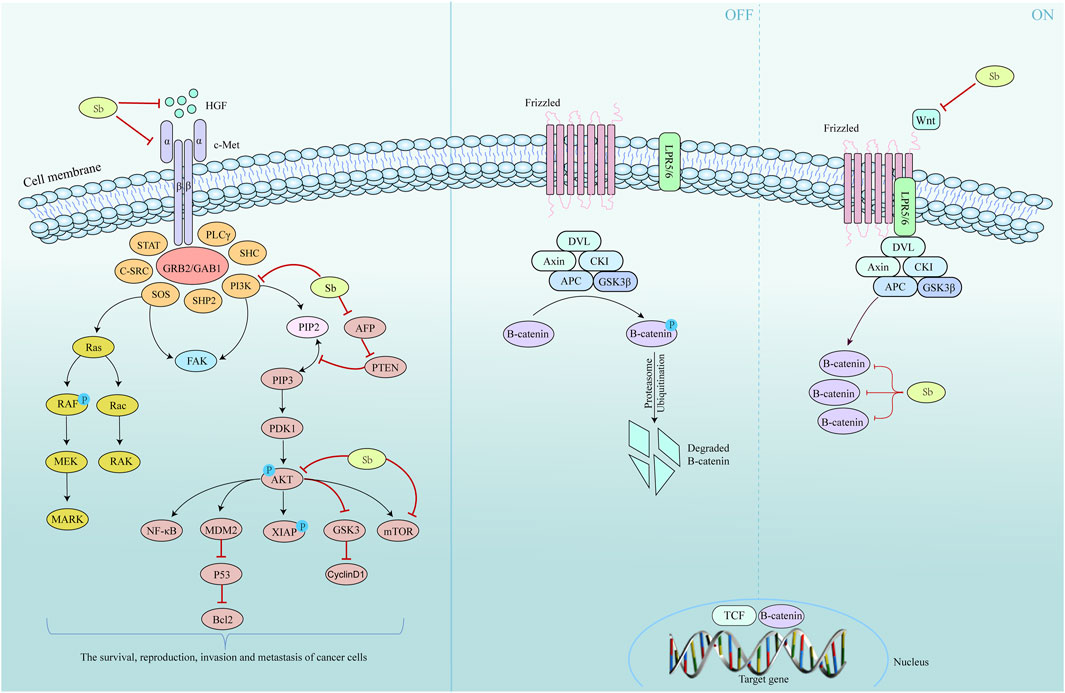
Figure 6. Mechanism of S. marianum in the treatment of liver cancer. Left is the HGF/c-Met and the PI3K/Akt/mTOR pathway, and the Wnt/β-catenin pathway on the right.
5.2.2 Gastric cancer
This research found that silymarin exhibited different mechanisms of action in three types of gastric cancer cells, namely, SGC-7901, BGC-823, and HGC-27 (Zhang et al., 2013b). In SGC-7901 cells, silymarin increased the expression of p53 and p21 and decreased the expression of Cyclin-dependent kinases 1 (CDK1). This resulted in the reduction of the CDK1-Cyclin B1 complex, causing the cells to stagnate in the G2/M phase. Additionally, silymarin induces apoptosis in SGC-7901 cells independent of the caspase pathway. In BGC-823 cells, silymarin had a limited effect, slightly reducing CDK1 and activating cysteinyl aspartate specific proteinase 3 (caspase 3) to a small extent. Therefore, silymarin had a weak effect on the induction of apoptosis in BGC-823 cells. Silymarin significantly inhibits the proliferation of HGC-27 cells, reduces the expression levels of CDK1 and Cyclin B1, causes G2/M phase cycle arrest, and activates caspase 3 to cause Poly ADP-ribose polymerase cleavage as well as caspase 8 and caspase 9, ultimately leading to apoptosis of tumor cells (Cartee et al., 2001).
5.2.3 Lung cancer
Silymarin targets several cytokines, including Interferon gamma (IFN-γ), Interferon beta-1 (IF-1β), and Tumor necrosis factor (TNF-α), by binding to Signal transducer and activator of transcription 3 (STAT3). This inhibits the expression of vascular endothelial growth factor by regulating COX-2 and Inducible nitric oxide synthase (Singh et al., 2006; Chittezhath et al., 2008). Silymarin can reduce the size and number of lung cancer cells through its anti-angiogenic activity. This is achieved by decreasing the production of cytokines in tumor-associated macrophages and inhibiting the activation of NFκB and STAT3 in lung cancer cells (Tyagi et al., 2009; Wang et al., 2020a; Verdura et al., 2021).
5.2.4 Kidney cancer
Silymarin has been shown to inhibit the growth of SN12K1 kidney cancer cells (Hii et al., 1998). At low concentrations, silymarin affected renal cancer cell morphology and inhibited DNA synthesis. At high concentrations, silymarin increased the release of lactate dehydrogenase (LDH) and induced apoptosis and necrosis of SN12K1 renal cancer cells. The effects of silymarin on renal cancer cells were studied in vivo using a model created by transplanting the renal cancer cells into healthy animals. After comparing the results with those of the control group, silymarin administration was found to reduce both the weight and size of the tumors (Cheung et al., 2007).
5.2.5 Bladder cancer
Silymarin effectively induced the expression of Cip1/p21 and Kip1/p27 proteins while decreasing the expression of CDK2, CDK4, and CDK6 and the cell cycle proteins Cyclin D1, Cyclin D3, and Cyclin E. It also increases the mutual binding of Cyclin-dependent kinases inhibitors (CDKI) and CDK, inhibits the kinase activity of CDKs, and ultimately arrests the cell cycle of bladder cancer in the G1 phase (Singh et al., 2002). Furthermore, increased doses of silymarin can decrease the levels of pCdc25c, Cdc25c, pCdc2, Cdc2, and Cyclin B1 proteins in TCC-SUP tumor cells, resulting in cell cycle arrest in the G2/M phase (Tan et al., 2002). Silymarin has varying effects on various bladder cancer cell types TCC-SUP cells can induce G1 and G2/M phase blockage, whereas T-24 cells can only block G1 phase blockage. Silymarin can significantly induce apoptosis in TCC-SUP cells, but the effect is not significant in T-24 cells (Tyagi et al., 2004).
5.2.6 Cervical cancer
The research found that silymarin treatment resulted in a four-fold increase in the number of HeLa cells in the G2/M phase compared to that in the control group. This suggests that silymarin may inhibit the progression of HeLa cells in cervical cancer and induce apoptosis, as evidenced by the presence of apoptotic precursors, such as cell crumpling. Silymarin downregulated CDK1 and CDK2 protein levels in a concentration-dependent manner and induced apoptosis in HeLa breast cancer cells in a time and concentration-dependent manner (Fan et al., 2011). It can activate the mitochondrial apoptotic pathway, leading to a decrease in B lymphocyte chemoattractant protein levels, release of cytochrome C from the mitochondria into the cytoplasmic matrix, and activation of caspase 9. It can activate the membrane receptor pathway of apoptosis, which upregulates the protein levels of Fatty Acid Synthase (Fas) gene and Fas ligand and activates Caspase 8 (Zhang et al., 2012).
5.2.7 Prostate cancer
Prostate cancer cells diffuse and infiltrate the prostate mesenchyme instead of forming localized tumors due to the secretion of prostate-specific antigens (PSA) into the prostate mesenchyme. This promotes the cleavage of Insulin-like growth factor-binding protein 3 and Insulin-like growth factor 1 (IGF-1), as well as the activation of transforming growth factor b and other growth factors in the extracellular matrix (ECM). These factors promote tumor cell growth and lead to tumor progression (Wang et al., 1997). Silymarin reduced intracellular and secreted PSA levels in human prostate cancer LNCaP cells and inhibited dihydrotestosterone-induced PSA production and cell growth. It can also inhibit malignant tumors by overexpressing cell-cycle proteins. Silymarin significantly decreased the levels of the cell cycle proteins D1, CDK4, and CDK6, leading to reduced kinase activity. Additionally, there was a significant increase in Cip1/p21 and Kip1/p27 (Poluha et al., 1996), which led to an increase in their binding to CDK2. In turn, this resulted in a significant reduction in CDK2 and cyclin E kinase activities, ultimately causing tumor cells to arrest at the G1 phase and inhibiting the growth of LNCaP cells (Mueller et al., 1997). Aldehyde dehydrogenase 1 family, member A1 (ALDH1A1) is an aldehyde oxidase that can be targeted by silymarin for the treatment of prostate cancer. It regulates the synthesis of trans- and 9-cis-retinoic acid, which inhibits the proliferation and differentiation of tumor-promoting macrophages stimulated by cancer cells. In addition, it also acts as an oncogene in prostate cancer (Yoshida et al., 1992). Studies have shown a positive correlation between ALDH1A1 expression in prostate cancer tissue and Recombinant retinoic acid receptor alpha (RARα) and Erythroblastosis-twenty six 1 (Ets1). RARα can bind to the Ets1 promoter and induce the expression of Ets1 mRNA and protein in cancer cells (Raouf et al., 2000). Ets1 affects the degradation of the extracellular matrix, which can facilitate the metastasis of tumor cells. The overexpression of Ets1 is closely related to the deterioration of prostate cancer (Li et al., 2012). ALDH1A1 promotes the invasion and metastasis of prostate cancer by activating RARα, which further activates Ets1. Silymarin inhibits the expression of ALDH1A1 in prostate cancer, which in turn inhibits the further activation of RARα and Ets1. Thus inhibiting the growth and metastasis of prostate cancer (Nazir et al., 2019).
5.2.8 Skin cancer
There was a strong correlation between elevated P53 levels and apoptosis induction in chronic ultraviolet radiation b (UVB)-exposed skin and tumors treated with silymarin (Matsumura and Ananthaswamy, 2004). P53 induces apoptosis in human keratinocytes at high doses of UV irradiation and activates the UV-induced repair of DNA damage at low doses of irradiation (Cotton and Spandau, 1997). p53 promotes cell repair and survival while promoting apoptosis. Similarly, silymarin protects cells from UV-induced apoptosis during acute injury and promotes apoptosis during chronic UV-induced injury (Dhanalakshmi et al., 2004). Silymarin upregulates Kip1/p27 and Cip1/p21 expression in tumors, which decreases the protein levels of CDK2, CDK4, Cyclin E, Cyclin A, and Cyclin D1, ultimately leading to tumor cell cycle arrest and reduce proliferation (Fotedar et al., 2004). Treatment with silymarin results in a significant increase in the phosphorylation of extracellular regulated protein kinases, JNK1/2, and p38 in the tumor samples. Activation of ERK induces cell cycle arrest by inducing the expression of the CDK inhibitors Cip1/p21 and Kip1/27, which activate the apoptotic response of JNK1/2 and p38 kinases. In HaCaT cells, p38 is activated by the release of cytochrome c into the cytosol, which in turn activates caspase-3 to mediate apoptosis in UVB (Polyak et al., 1994). Survivin molecular antagonists have been shown to induce caspase-dependent cell death, enhance apoptosis, and exhibit anticancer activity in vivo. Additionally, silymarin has been shown to reduce Survivin levels in tumors. In conclusion, silymarin can inhibit skin cell carcinogenesis by inhibiting DNA synthesis, cell proliferation, blocking the cell cycle, and inducing apoptosis (Bachelder et al., 1999).
5.2.9 Breast cancer
Silymarin inhibites both MCF-7 and SK-BR-3 breast cancer cell lines A low dose of silymarin can strongly inhibit MCF-7, leading to cell autophagy and apoptosis by down-regulating the expression of Estrogen Receptor α (ERα) in MCF-7 cells (Zheng et al., 2017). Additionally, silymarin upregulates the expression of ERβ, which induces apoptosis via the mitochondrial pathway. However, silymarin has shown to only weakly inhibit the growth of SK-BR-3 cells. Studies have suggested that this may be related to the protein tyrosine kinase molecule Human epidermal growthFactor receptor 2 (Her-2) in breast cancer cells (Templeton et al., 2014). The expression level of Her-2 is considered an important indicator of the degree of malignancy and prognosis of breast cancer (Kurokawa et al., 2000). The expression of the Her-2 molecule has been found to increase the proliferation of SK-BR-3 breast cancer cells and decrease their sensitivity to silymarin (Hermanto et al., 2001).
5.2.10 Colon cancer
Silymarin inhibits the growth of colon cancer cells by blocking the cell cycle via multiple mechanisms. Specifically, it upregulates kip1/p27, a key member of CDKI that counteracts proliferative signals. Tumor cells with low or no CDKI expression exhibited uncontrolled growth. Silymarin increased the mRNA and protein expression of kip1/p27. Additionally, it can increases the protein expression of Cip1/p21 without relying on the regulation of the p35 oncoprotein. It also blocked the G1 phase of HT-29 colon cancer cells (Sherr, 1996). Cdc25C acts as a mitotic activator by dephosphorylating cdc2/p34. The activity of cdc2/p34 kinase is enhanced in human cancers. Higher doses of silybin reduce cdc2/p34 kinase activity, decrease the protein expression of cdc25C, cdc2/p34, and cyclin B1, and block HT-29 colon cancer cells in the G2 phase (Graves et al., 2000). Longer treatment with silymarin also causes HT-29 tumor cells to undergo apoptosis independent of the caspase pathway (Chinni et al., 2001). (Table 1).
5.3 Antioxidant effects
Silymarin enhances the antioxidant capacity of the body via several mechanisms. First, it directly scavenges the free radicals. Secondly, it inhibits ROS-generating enzymes, thereby preventing free radical production. Additionally, silymarin activates a series of antioxidant enzymes, such as NF-E2-related factor 2 and Nuclear factor kappa B (NF-κB), to maintain an optimal redox balance in cells. Silymarin can activate the molecules responsible for protecting organisms, such as Heat shock protein, Thioredoxin, and sirtuins, providing additional protection during oxidative stress (Surai, 2015). Studies have shown that increased production of reactive oxygen metabolites is a significant cause of sepsis. Free radicals, in addition to causing direct tissue damage, may lead to the accumulation of leukocytes in tissue, which activate neutrophils and cause further damage. This disease causes a systemic inflammatory response that may ultimately progress to systemic multi-organ failure (Sener et al., 2005a; Sener et al., 2005b). Silymarin reduces oxidative organ damage induced by sepsis by inhibiting neutrophil infiltration, which in turn blocks the release of cytokines such as leukotrienes (Lts) and Interleukin-1 (IL-1) (Toklu et al., 2008). Severe burns can trigger an inflammatory response that damages the affected tissue. This damage can lead to sepsis and multi-organ failure in severe cases (Sayeed, 1998; Schwacha and Chaudry, 2002). Burns results in a significant increase in pro-inflammatory factors TNF-a and LDH, leading to an increase in MDA levels and a decrease in GSH levels in the skin. Silymarin can reverse these effects by inhibiting burn-induced oxidative damage to the skin. Additionally, silymarin can reverse the morphological damage caused by burns on the skin (Toklu et al., 2007). Oxidative stress is considered a crucial mechanism in Doxorubicin (DOX)-induced cardiomyocyte damage. High levels of ROS promote autophagy, whereas low levels inhibit it. DOX causes a slight increase in ROS, and moderate autophagy helps maintain intracellular homeostasis by degrading redundant, aged, and misfolded proteins, releasing energy, or removing damaged organelles (Taghiabadi et al., 2012). This suggests that low ROS production is crucial for the inhibition of DOX-induced autophagy. Additionally, silymarin counteracts myocardial injury by activating IL6ST/JAK2/STAT3, which helps eliminate ROS and restore autophagy (Li W. et al., 2022).
5.4 Inhibition of NO production
Cytotoxic NO production increases in pathological states of liver damage. Excessive NO levels can cause hypoxemia and hyperdynamic cycles. Additionally, NO reacts with O2 to produce nitrite, an unstable and weak acid that decomposes into a strongly toxic NO2 group. Silymarin inhibits the production of NO by Kupffer cells, thereby reducing the amount of NO2 and other toxic groups that protect the body (Chittezhath et al., 2008).
5.5 Anti-inflammatory
The anti-gastric ulcer activity of silymarin is attributed to its inhibition of enzymatic peroxidation in the lipoxygenase pathway, which inhibits leukotriene synthesis (Alarcon de la Lastra et al., 1992). Silymarin has free radical scavenging activity. It can also regulate arachidonic acid cascade and inhibit the production of Prostaglandins and Leukotrienes, thus effectively inhibiting the development of arthritis (Gupta et al., 2000).
5.6 Immunomodulatory effects
Research on alcohol-induced liver disease revealed that ethanol metabolism generates acetaldehyde adducts that activate the immune system as foreign antigens. This can lead to an increase in the number and activity of Cytotoxic T lymphocyte (CTL) and Natural killer cells (NK) in the body, thereby exacerbating immune damage to hepatocytes (Yasuda et al., 1999). Following silymarin treatment, there was a decrease in the number of CTL and NK cells in the blood, as well as a reduction in their activity. This suggests that the drug possesses immunomodulatory properties. Additionally, studies have shown that silymarin can inhibit the proliferation of CD4 cell lymphocytes and the production of IL-2 and IFN-γ in mice (Gharagozloo et al., 2010).
5.7 Neuroprotective effects
Silymarin may serve as a neuroprotective agent for treating various neurological disorders such as Alzheimer’s disease, Parkinson’s disease, and cerebral ischemia (Borah et al., 2013). Alzheimer’s is characterized by cognitive impairment and the deposition of extracellular amyloid fibrils in senile plaques. Silymarin attenuates these symptoms in antibody-induced animal models of Alzheimer’s disease. Silymarin administration significantly improved cognitive abnormalities, particularly memory impairment, and significantly reduced extracellular amyloid fibrillar deposition in senile plaques (Lu et al., 2009). Parkinson’s disease is characterized by the loss of dopaminergic neurons in the dense part of the substantia nigra and abnormal motor behavior. According to a previous research, silymarin has been shown to significantly increase dopamine and serotonin levels in the hippocampal and cortical regions and inhibit monoamine oxidase-b. This suggests that silymarin counteracts dopamine loss in patients with Parkinson’s (Singhal et al., 2011).
5.8 Treatment of insulin resistance
Insulin resistance refers to the weakening of the physiological role of insulin in the body, and obesity is often the main cause of insulin resistance, which in turn will put obese people in a state of chronic inflammation (MacDonald-Ramos et al., 2024). Insulin resistance can also lead to an increase in blood sugar, and the body secretes more insulin to maintain normal blood sugar levels, leading to hyperpancreatic islet emia and the eventual development of diabetes mellitus type 2 (MacDonald-Ramos et al., 2021). Silymarin’s excellent anti-inflammatory and antioxidant stress effects can be used in IR treatment to alleviate the adverse effects of diseases on the body and prevent the occurrence of diabetes. A number of studies have shown that insulin resistance is effectively inhibited after treatment with a certain dose of silymarin administered to an animal model of insulin resistance, which is achieved by restoring the IRS-1/P13K/Akt pathway and blocking the phosphorylation of c-Jun N-terminal kinase (JNK) and inhibitor of kappa B kinase (Zhang et al., 2013a; Li et al., 2015; Guo et al., 2016).
5.9 Treatment of diabetes
Diabetes mellitus is a prevalent metabolic disorder with multiple causes resulting from insufficient insulin secretion or defective insulin action (Stolf et al., 2017). Hyperphagia, polydipsia, polyuria, and weight loss are the common symptoms of diabetes. Failure to control blood glucose levels in a timely manner can result in more than 100 complications, including nephropathy, neuropathy, impaired healing, oxidative stress, cataracts, hepatotoxicity, and cardiomyopathy. In severe cases, it can lead to multi-organ damage and organ failure, such as diabetic end-stage renal failure (Alberti and Zimmet, 1998; Conserva et al., 2016). Silymarin has been shown to be effective in treating alloxan-induced diabetes in rats (Soto et al., 2010). It also increased the activity and expression levels of Superoxide dismutase, GSH peroxidase, and Catalase in the pancreas of diabetic rats. This mechanism of action may be related to the activation of the promoter regions of these enzymes by flavonolignans (Soto et al., 1998; Soto et al., 2003). Additionally, silymarin improved pancreatic morphology and endocrine function in diabetic rats and repaired damaged kidney tissue (Soto et al., 2004). Patients with end-stage diabetic nephropathy exhibit significant thiol deficiency, which is directly related to a decrease in T cell activity and an increase in the synthesis of TNF-α. This promotes ROS production by neutrophils. Silymarin ameliorated or reversed these symptoms (Dietzmann et al., 2002). (Figure 7) (Table 2).
6 Progress in the biosynthesis of silymarin
Coniferyl alcohol and taxifolin are precursors of silymarin biosynthesis, and these two substances have certain organ dependence in S. marianum. Studies have shown that coniferyl alcohol is distributed in the whole plant of silymarin, while taxifolin is mainly distributed in flowers, pericarp and embryos, which is also related to the accumulation of silymarin in pericarp (Lv et al., 2017).
Mechanistic studies have shown that silymarin is produced through the oxidative coupling of coniferyl alcohol and taxifolin, which is formed from the conversion of phenylalanine via the phenylalanine pathway (Martinelli et al., 2017). (Figure 8) This pathway catalyzes the dehydrogenation of phenylalanine by phenylalanine deaminase (PAL) to produce trans-cinnamic acid, which is then catalyzed by cinnamic acid-4-hydroxylase (C4H) to produce p-coumaric acid. Taxifolin is produced by the catalysis of p-coumaric acid to generate p-coumaroyl coenzyme A. Under the catalysis of chalcone synthase (CHS), one molecule of p-coumaroyl coenzyme A is condensed with three molecules of malonyl coenzyme A to generate naringenin chalcone. Naringenin chalcone undergoes successive catalytic reactions of chalcone isomerase (CHI), flavanone 3-hydroxylase (F3H), and flavanone 3′-hydroxylase (F3′H), isomerization, and hydroxylation to finally obtain taxifolin. (Hammerbacher et al., 2019).
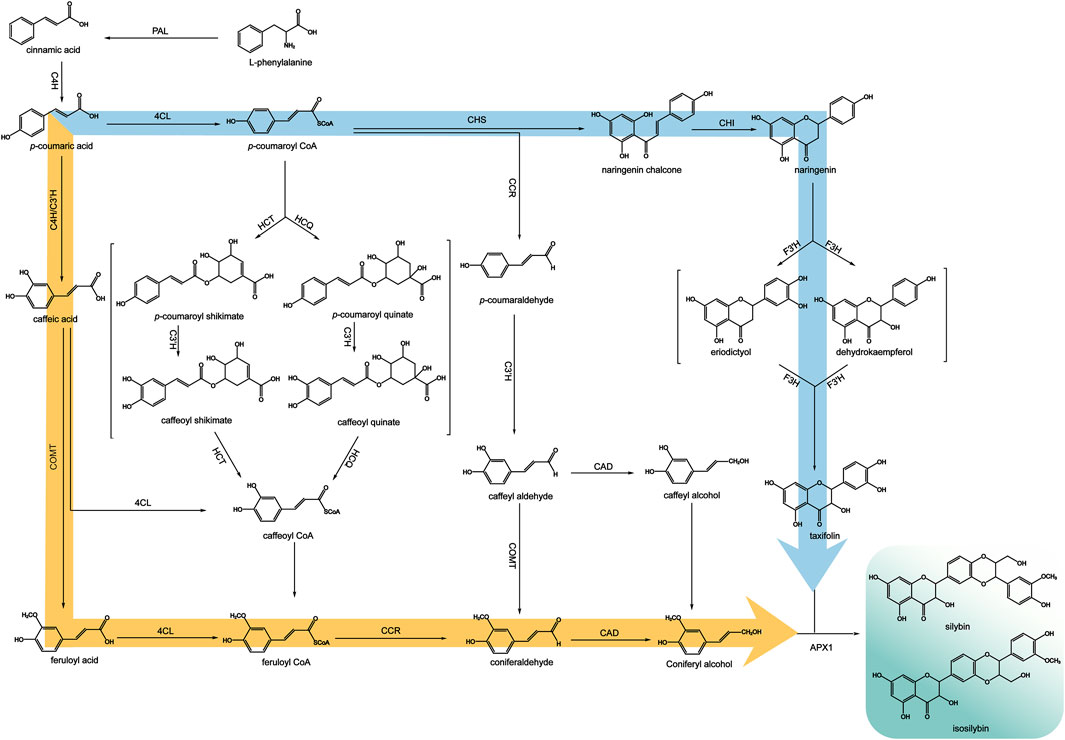
Figure 8. Biosynthetic pathways of silymarin (adapted from (Yang et al., 2020)).
Coniferyl alcohol is produced by the action of 4-coumaroyl-coenzyme A ligase (4CL), which catalyzes the conversion of coumaric acid to coumaroyl-coenzyme A. Then this compound is hydroxylated by coumaric acid-3-hydroxylase (C3H) to form caffeoyl CoA. Another way is using coumaroyl-coenzyme A as a substrate, cysteine protease (CST) or quinuclidinic acid hydroxycinnamoyltransferase (CQT) catalyze the production of p-coumaroyl shikimic acid or p-coumaroyl quinic acid. These compounds are then hydroxylated by C3H to produce the intermediates, caffeoyl shikimic acid and caffeoyl quinic acid. Finally, CST or CQT catalyze the conversion of these intermediates to caffeoyl CoA, which is methylated by caffeoyl coenzyme A-O-methyltransferase (CCoAOMT) to produce feruloyl CoA. Because the substrates of C3H, 4CL and methyltransferase are relatively broad, there are other mechanisms from p-coumaric acid to p-coumaroyl CoA, that is, p-coumaric acid is first catalyzed by C3H to generate caffeic acid, and caffeic acid is catalyzed by 4CL to generate caffeoyl CoA and then methylated. Alternatively, caffeic acid can be catalyzed by caffeic acid-O-methyltransferase (COMT) to form the methylated product ferulic acid, which is then catalyzed by 4CL to form feruloyl CoA. Finally, feruloyl CoA is catalyzed by cinnamoyl-CoA reductase (CCR) to produce coniferyl dehyde, which is then catalyzed by cinnamyl alcohol dehydrogenase (CAD) to produce coniferyl alcohol (Jin et al., 2016). Studies have shown that peroxidases, particularly APX1, can couple taxifolin and coniferyl alcohol to generate silybin and isosilybin (Shin et al., 2015; Drouet et al., 2020).
7 Comprehensive utilization
7.1 Edible oil
Achenes of S. marianum have high oil content, usually ranging from 30% to 35%. Silymarin oil is rich in various bioactive compounds including phenolic acids, tocopherols, fatty acids, and phytosterols (Chambers et al., 2017). The oil of achenes in S. marianum is also a natural source of vitamin E (Zarrouk et al., 2019). The oil extraction of achenes in S. marianum is effective in preventing oxidative stress and restoring normal levels of cholesterol, triglycerides, LDL, and liver markers associated with liver pathology. Therefore, it is often recommended as a beneficial cooking oil (Shin et al., 2015). Cold-pressed oil is produced using a simple method that does not require a high-energy input or added chemicals. This method is more economical and environmentally friendly than traditional refined oils. The oil is purified using water, sedimentation, filtration, and centrifugation. Additionally, the cold-pressed oil technique allows for greater retention of valuable substances in achenes of S. marianum (Kalinowska et al., 2022).
7.2 Forage
After extracting the active ingredients from achenes of S. marianum, a byproduct of residue is produced. Recently, increasing attention has been paid to the use of S. marianum residue as animal feed (Stastnik et al., 2020). S. marianum residue has starch and protein contents of up to 30% and 20%, respectively, without any toxic side effects. They are rich in amino acids and trace elements (Liu et al., 2012). This is not only conducive to the absorption and supply of energy and protein required for animal growth, but also conducive to the absorption and supply of trace elements. Additionally, it has health benefits, improves immunity, and indirectly reduces the use of antibiotics and other drugs. It has higher added value and can be used as a new high-quality feed for poultry, livestock, and fishery farmin (Chambers et al., 2017; Stastnik et al., 2020; Krepkova et al., 2021).
7.3 Cosmetics
In recent years, natural antioxidants have gained attention because of their harmful effects of synthetic antioxidants on the human body (Singh and Agarwal, 2009). Elastase and collagenase affect the regeneration or degradation of the extracellular matrix of the skin dermis, resulting in loss of skin tone, wrinkle formation, and loss of elasticity (Chambers et al., 2017). Studies have shown that silymarin inhibits collagenase and elastase to a lesser extent (Nichols and Katiyar, 2010; Drouet et al., 2019).
7.4 Foods
The young leaves of S. marianum are tender, juicy, crisp, and refreshed, which are excellent vegetables for consume (Liu et al., 2016). Achenes of S. marianum are protein-rich and can be used to produce protein powder, which is characterized by high protein content, low fat content, and low cholesterol levels (Liu, 2021). According to Krepkova et al., combining oil of S. marianum with baking can increase the nutritional value of food by providing extra vitamins, proteins, and linoleic acid (Krepkova et al., 2021).
7.5 Others
S. marianum can reach heights of 1.5–2.0 m and quickly form barriers that are impassable to livestock within 2 months. The flowers are large, brightly colored, and numerous, with a long bloom period, making them suitable for ornamental purposes. Additionally, the plant can be used as green manure after oil extracted from the achenes, which provides the soil with a rich source of nutrients, promotes crop growth, and improves soil quality. S. marianum is a valuable honey plant owing to its high yield and potential health benefits, such as liver and stomach protection. Therefore, it is a promising source of nectar for the development of new honey products (Pereira et al., 2015). (Figure 9).
8 Conclusion and prospects
This research analyzed the status of S. marianum researches in recent years. More than 20 types of flavonolignans constituents have been isolated from S. marianum. S. marianum has been found to have a variety of pharmacological effects, including hepatoprotective, cardioprotective, anti-inflammatory, anticancer, antioxidant, immunomodulatory, and neuroprotective effects. In addition to playing a role in the field of medicine, S. marianum is also used to produce edible oil, protein powder, forage and so on. Its excellent antioxidant effect is also very suitable for making cosmetics to protect the skin.
In recent years, researchers have been committed to the development and utilization of S. marianum, but there are still many aspects that are not perfect. First, the quality of S. marianum is a hot issue of concern. It is necessary to adjust measures to local conditions to find the most suitable areas for plant growth, to ensure the yield and quality of medicinal materials, and to promote the healthy development of medicinal plants and regional economy. The second is that the planting technology is not perfect, and the mature cultivation experience of the original producing area can be used for reference to ensure the growth and survival of S. marianum. The third is the development and utilization of chemical components. At present, there are more than 20 types of flavonolignans isolated from S. marianum (Wang et al., 2020b). The discovery of these components provides more possibilities for the functional research of S. marianum. The extraction method of the active ingredient is continuously optimized to improve the extraction rate of silymarin-related components. Silymarin is commonly extracted by degreasing the achenes of the plant and extracting them using methanol (Wianowska and Wiśniewski, 2015). Higher yields and purity of silymarin can be obtained using the chemo-enzymatic method (Biedermann et al., 2014). Secondly, except for the main active ingredient silybin, the role and mechanism of other components are not clear enough. It is of great significance to clarify the role and mechanism of these components, and more efforts are needed in the future. Finally, the difference in the content of active ingredients in the achenes of S. marianum in different regions and the difference in the content of active ingredients in different varieties in the same region are also worthy of our research, which provides a theoretical basis for the production of higher quality medicinal materials and the breeding of excellent varieties of S. marianum.
In clinical medication, the therapeutic effect of S. marianum on the liver has been repeatedly verified in the long-term of medication practice, but the improvement of bioavailability still needs continuous research. Researchers are improving the bioavailability of silymarin through nanocrystals, nanosuspensions and solid dispersions, and complexes of cyclodextrins and phospholipids. In particular, the combination of silymarin with phosphatidylcholine increased the bioavailability of silymarin 4.6-fold compared to the extract alone (Javed et al., 2011; MacDonald-Ramos et al., 2021). Chemotherapy is a conventional means of cancer treatment, but there are also obvious drawbacks. Because of its strong toxicity, poor targeting, many side effects and difficult to control, the vast majority of patients develop drug resistance, which ultimately leads to the failure of chemotherapy. Therefore, plant-based therapeutic agents with low toxic and side effects, high anticancer activity and synergistic effect with anticancer drugs are the focus of our current research. In addition, other pharmacological effects of silymarin, such as prevention and treatment of diabetes, protection of myocardial cells, anti-platelet aggregation, anti-oxidation, and gastric protection, have not been widely valued and utilized. Therefore, researchers should conduct in-depth research on this and fully tap its medicinal value in order to better serve the majority of patients.
Due to the limited genetic information about S. marianum, the biosynthesis and regulation mechanism of silymarin has been difficult to elucidate. Exploring and revealing the genetic information in S. marianum is of great significance for the development of silymarin biosynthesis. In addition to medicinal use, S. marianum is also a multi-purpose economic crop, which has many application values such as ornamental, animal husbandry, food and healthcare. The plants of S. marianum are tall, gorgeous flowers, strong resistance, can be used for urban greening. The oil of S. marianum is rich in nutrients and a variety of beneficial ingredients, which can be used to produce edible oil, lubricating oil, soap and so on. S. marianum can also be used as nectar, feed, cosmetic raw materials, green manure and so on. The comprehensive utilization of S. marianum is aimed at exploring more value of resources, protecting human health and promoting regional economic development.
In summary, S. marianum is an important resource for human health. Its chemical compositions, pharmacological mechanisms, and biosynthesis need to be further studied in order to provide a theoretical basis for the development of medicinal functions of S. marianum. This review provides a valuable background for the research of S. marianum, and provides a reference for further research and application of this medicinal plant.
Author contributions
XZ: Writing–original draft. ML: Writing–review and editing. ZW: Writing–review and editing. PW: Writing–review and editing. LK: Writing–review and editing. JW: Writing–review and editing. WW: Writing–review and editing. LM: Writing–review and editing. SJ: Writing–review and editing. WR: Writing–review and editing. LD:Writing–review and editing. WM: Writing–review and editing. XL: Writing–review and editing.
Funding
The author(s) declare that financial support was received for the research, authorship, and/or publication of this article. This work was supported by National Key Research and Development Program, Research and Demonstration of Collection, Screening and Breeding Technology of Ginseng and other Genuine Medicinal Materials, Project [Grant Number: 2021YFD1600901]; Heilongjiang Touyan Innovation Team Program [Grant Number:2019 No. 5]; Special Project to Popularize Classics of Chinese Medicine in Heilongjiang Province, The Comprehensive Benefits of Silybum marianum were Explored Based on Materia Medica Research and Cultivation Technology [Grant Number: ZYW 2023-026].
Conflict of interest
The authors declare that the research was conducted in the absence of any commercial or financial relationships that could be construed as a potential conflict of interest.
Publisher’s note
All claims expressed in this article are solely those of the authors and do not necessarily represent those of their affiliated organizations, or those of the publisher, the editors and the reviewers. Any product that may be evaluated in this article, or claim that may be made by its manufacturer, is not guaranteed or endorsed by the publisher.
References
Abenavoli, L., Capasso, R., Milic, N., and Capasso, F. (2010). Milk thistle in liver diseases: past, present, future. Phytother. Res. 24 (10), 1423–1432. doi:10.1002/ptr.3207
Abenavoli, L., Izzo, A. A., Milić, N., Cicala, C., Santini, A., and Capasso, R. (2018). Milk thistle (Silybum marianum): a concise overview on its chemistry, pharmacological, and nutraceutical uses in liver diseases. Phytother. Res. 32 (11), 2202–2213. doi:10.1002/ptr.6171
Alarcon de la Lastra, C., Martin, M. J., and Marhuenda, E. (1992). Gastric anti-ulcer activity of silymarin, a lipoxygenase inhibitor, in rats. J. Pharm. Pharmacol. 44 (11), 929–931. doi:10.1111/j.2042-7158.1992.tb03239.x
Alberti, K. G., and Zimmet, P. Z. (1998). Definition, diagnosis and classification of diabetes mellitus and its complications. Part 1: diagnosis and classification of diabetes mellitus provisional report of a WHO consultation. Diabet. Med. 15 (7), 539–553. doi:10.1002/(sici)1096-9136(199807)15:7<539::Aid-dia668>3.0.Co;2-s
Arnone, A., Merlini, L., and Zanarotti, A. (1979). Constituents of Silybum marianum. Structure of isosilybin and stereochemistry of silybin. J. Chem. Soc. Chem. Commun. (16), 696–697. doi:10.1039/C39790000696
Bachelder, R. E., Ribick, M. J., Marchetti, A., Falcioni, R., Soddu, S., Davis, K. R., et al. (1999). p53 inhibits alpha 6 beta 4 integrin survival signaling by promoting the caspase 3-dependent cleavage of AKT/PKB. J. Cell Biol. 147 (5), 1063–1072. doi:10.1083/jcb.147.5.1063
Ball, K. R., and Kowdley, K. V. (2005). A review of Silybum marianum (milk thistle) as a treatment for alcoholic liver disease. J. Clin. Gastroenterology 39 (6), 520–528. doi:10.1097/01.mcg.0000165668.79530.a0
Begum, S. A., Sahai, M., and Ray, A. B. (2010). Non-conventional lignans: coumarinolignans, flavonolignans, and stilbenolignans. Fortschritte der Chemie organischer Naturstoffe/Prog. Chem. Org. Nat. Prod. 93, 1–70. doi:10.1007/978-3-7091-0140-7_1
Bencze-Nagy, J., Strifler, P., Horváth, B., Such, N., Farkas, V., Dublecz, K., et al. (2023). Effects of dietary milk thistle (Silybum marianum) supplementation in ducks fed mycotoxin-contaminated diets. Vet. Sci. 10 (2), 100. doi:10.3390/vetsci10020100
Biedermann, D., Vavříková, E., Cvak, L., and Křen, V. (2014). Chemistry of silybin. Nat. Prod. Rep. 31 (9), 1138–1157. doi:10.1039/c3np70122k
Bittencourt, M. L. F., Rodrigues, R. P., Kitagawa, R. R., and Gonçalves, R. C. R. (2020). The gastroprotective potential of silibinin against Helicobacter pylori infection and gastric tumor cells. Life Sci. 256, 117977. doi:10.1016/j.lfs.2020.117977
Borah, A., Paul, R., Choudhury, S., Choudhury, A., Bhuyan, B., Das Talukdar, A., et al. (2013). Neuroprotective potential of silymarin against CNS disorders: insight into the pathways and molecular mechanisms of action. CNS Neurosci. Ther. 19 (11), 847–853. doi:10.1111/cns.12175
Cartee, L., Wang, Z., Decker, R. H., Chellappan, S. P., Fusaro, G., Hirsch, K. G., et al. (2001). The cyclin-dependent kinase inhibitor (CDKI) flavopiridol disrupts phorbol 12-myristate 13-acetate-induced differentiation and CDKI expression while enhancing apoptosis in human myeloid leukemia cells. Cancer Res. 61 (6), 2583–2591.
Chambers, C. S., Holeckova, V., Petraskova, L., Biedermann, D., Valentova, K., Buchta, M., et al. (2017). The silymarin composition. and why does it matter? Food Res. Int. 100 (Pt 3), 339–353. doi:10.1016/j.foodres.2017.07.017
Chen, Y., and Wang, C. (1998). Basic research on comprehensive utilization of milk thistle II fruit oil and protein. Acta Agric. Boreali-Occidentalis Sin. (01), 84–86.
Cheung, C. W., Vesey, D. A., Nicol, D. L., and Johnson, D. W. (2007). Silibinin inhibits renal cell carcinoma via mechanisms that are independent of insulin-like growth factor-binding protein 3. BJU Int. 99 (2), 454–460. doi:10.1111/j.1464-410X.2007.06571.x
Chinni, S. R., Li, Y., Upadhyay, S., Koppolu, P. K., and Sarkar, F. H. (2001). Indole-3-carbinol (I3C) induced cell growth inhibition, G1 cell cycle arrest and apoptosis in prostate cancer cells. Oncogene 20 (23), 2927–2936. doi:10.1038/sj.onc.1204365
Chittezhath, M., Deep, G., Singh, R. P., Agarwal, C., and Agarwal, R. (2008). Silibinin inhibits cytokine-induced signaling cascades and down-regulates inducible nitric oxide synthase in human lung carcinoma A549 cells. Mol. Cancer Ther. 7 (7), 1817–1826. doi:10.1158/1535-7163.Mct-08-0256
Choi, S., Ham, S., Lee, Y. I., Kim, J., Lee, W. J., and Lee, J. H. (2023). Silibinin downregulates types I and III collagen expression via suppression of the mTOR signaling pathway. Int. J. Mol. Sci. 24 (18), 14386. doi:10.3390/ijms241814386
Committee, N. P. (2020). Chinese pharmacopoeia. Beijing: China Medical Science and Technology Press.
Conserva, F., Gesualdo, L., and Papale, M. (2016). A systems biology overview on human diabetic nephropathy: from genetic susceptibility to post-transcriptional and post-translational modifications. J. Diabetes Res. 2016, 7934504. doi:10.1155/2016/7934504
Cotton, J., and Spandau, D. F. (1997). Ultraviolet B-radiation dose influences the induction of apoptosis and p53 in human keratinocytes. Radiat. Res. 147 (2), 148–155. doi:10.2307/3579415
Csupor, D., Csorba, A., and Hohmann, J. (2016). Recent advances in the analysis of flavonolignans of Silybum marianum. J. Pharm. Biomed. Analysis 130, 301–317. doi:10.1016/j.jpba.2016.05.034
Dahmani, R., Just, P. A., and Perret, C. (2011). The Wnt/β-catenin pathway as a therapeutic target in human hepatocellular carcinoma. Clin. Res. Hepatol. Gastroenterol. 35 (11), 709–713. doi:10.1016/j.clinre.2011.05.010
Dan, W., Fan, Y., Hou, T., Wei, Y., Liu, B., Que, T., et al. (2022). Silibinin inhibits the migration, invasion and epithelial-mesenchymal transition of prostate cancer by activating the autophagic degradation of YAP. J. Cancer 13 (13), 3415–3426. doi:10.7150/jca.63514
de Avelar, C. R., Nunes, B. V. C., da Silva Sassaki, B., Dos Santos Vasconcelos, M., de Oliveira, L. P. M., Lyra, A. C., et al. (2023). Efficacy of silymarin in patients with non-alcoholic fatty liver disease - the Siliver trial: a study protocol for a randomized controlled clinical trial. Trials 24 (1), 177. doi:10.1186/s13063-023-07210-6
Dhanalakshmi, S., Mallikarjuna, G. U., Singh, R. P., and Agarwal, R. (2004). Silibinin prevents ultraviolet radiation-caused skin damages in SKH-1 hairless mice via a decrease in thymine dimer positive cells and an up-regulation of p53-p21/Cip1 in epidermis. Carcinogenesis 25 (8), 1459–1465. doi:10.1093/carcin/bgh152
Dietzmann, J., Thiel, U., Ansorge, S., Neumann, K. H., and Täger, M. (2002). Thiol-inducing and immunoregulatory effects of flavonoids in peripheral blood mononuclear cells from patients with end-stage diabetic nephropathy. Free Radic. Biol. Med. 33 (10), 1347–1354. doi:10.1016/s0891-5849(02)01043-2
Drouet, S., Leclerc, E. A., Garros, L., Tungmunnithum, D., Kabra, A., Abbasi, B. H., et al. (2019). A green ultrasound-assisted extraction optimization of the natural antioxidant and anti-aging flavonolignans from milk thistle Silybum marianum (L.) Gaertn. Fruits for cosmetic applications. Antioxidants 8 (8), 304. doi:10.3390/antiox8080304
Drouet, S., Tungmunnithum, D., Lainé, É., and Hano, C. (2020). Gene expression analysis and metabolite profiling of silymarin biosynthesis during milk thistle (Silybum marianum (L.) Gaertn.) fruit ripening. Int. J. Mol. Sci. 21 (13), 4730. doi:10.3390/ijms21134730
Faixová, D., Ratvaj, M., Maruščáková, I. C., Hrčková, G., Karaffová, V., Faixová, Z., et al. (2023). Silybin showed higher cytotoxic, antiproliferative, and anti-inflammatory activities in the CaCo cancer cell line while retaining viability and proliferation in normal intestinal IPEC-1 cells. Life (Basel) 13 (2), 492. doi:10.3390/life13020492
Fallah, M., Davoodvandi, A., Nikmanzar, S., Aghili, S., Mirazimi, S. M. A., Aschner, M., et al. (2021). Silymarin (milk thistle extract) as a therapeutic agent in gastrointestinal cancer. Biomed. Pharmacother. 142, 112024. doi:10.1016/j.biopha.2021.112024
Fan, S., Li, L., Chen, S., Yu, Y., Qi, M., Tashiro, S.-I., et al. (2011). Silibinin induced-autophagic and apoptotic death is associated with an increase in reactive oxygen and nitrogen species in HeLa cells. Free Radic. Res. 45 (11-12), 1307–1324. doi:10.3109/10715762.2011.618186
Fan, Y., Hou, T., Dan, W., Liu, T., Luan, J., Liu, B., et al. (2020). Silibinin inhibits epithelial‑mesenchymal transition of renal cell carcinoma through autophagy-dependent Wnt/β-catenin signaling. Int. J. Mol. Med. 45 (5), 1341–1350. doi:10.3892/ijmm.2020.4521
Feng, R., Chen, J. H., Liu, C. H., Xia, F. B., Xiao, Z., Zhang, X., et al. (2019). A combination of Pueraria lobata and Silybum marianum protects against alcoholic liver disease in mice. Phytomedicine 58, 152824. doi:10.1016/j.phymed.2019.152824
Flora, K., Hahn, M., Rosen, H., and Benner, K. (1998). Milk thistle (Silybum marianum) for the therapy of liver disease. Am. J. Gastroenterol. 93 (2), 139–143. doi:10.1111/j.1572-0241.1998.00139.x
Fotedar, R., Bendjennat, M., and Fotedar, A. (2004). Role of p21WAF1 in the cellular response to UV. Cell Cycle 3 (2), 132–135. doi:10.4161/cc.3.2.658
Gándara, L., Sandes, E., Di Venosa, G., Prack Mc Cormick, B., Rodriguez, L., Mamone, L., et al. (2014). The natural flavonoid silybin improves the response to Photodynamic Therapy of bladder cancer cells. J. Photochem Photobiol. B 133, 55–64. doi:10.1016/j.jphotobiol.2014.03.006
Gharagozloo, M., Velardi, E., Bruscoli, S., Agostini, M., Di Sante, M., Donato, V., et al. (2010). Silymarin suppress CD4+ T cell activation and proliferation: effects on NF-kappaB activity and IL-2 production. Pharmacol. Res. 61 (5), 405–409. doi:10.1016/j.phrs.2009.12.017
Gioti, K., Papachristodoulou, A., Benaki, D., Havaki, S., Beloukas, A., Vontzalidou, A., et al. (2019). Silymarin enriched extract (Silybum marianum) additive effect on doxorubicin-mediated cytotoxicity in PC-3 prostate cancer cells. Planta Med. 85 (11-12), 997–1007. doi:10.1055/a-0954-6704
Gjörloff Wingren, A., Ziyad Faik, R., Holefors, A., Filecovic, E., and Gustafsson, A. (2023). In vitro effects of undifferentiated callus extracts from Plantago major L, Rhodiola rosea L and Silybum marianum L in normal and malignant human skin cells. Heliyon 9 (6), e16480. doi:10.1016/j.heliyon.2023.e16480
Graves, P. R., Yu, L., Schwarz, J. K., Gales, J., Sausville, E. A., O'Connor, P. M., et al. (2000). The Chk1 protein kinase and the Cdc25C regulatory pathways are targets of the anticancer agent UCN-01. J. Biol. Chem. 275 (8), 5600–5605. doi:10.1074/jbc.275.8.5600
Gu, M., Zhao, P., Huang, J., Zhao, Y., Wang, Y., Li, Y., et al. (2016). Silymarin ameliorates metabolic dysfunction associated with diet-induced obesity via activation of farnesyl X receptor. Front. Pharmacol. 7, 345. doi:10.3389/fphar.2016.00345
Guo, J., and Yang, X. (2008). Research progress on the protective effect of flavonoids on experimental liver injury in animals. Chin. Pharmacol. Bull. (01), 5–10.
Guo, Y., Wang, S., Wang, Y., and Zhu, T. (2016). Silymarin improved diet-induced liver damage and insulin resistance by decreasing inflammation in mice. Pharm. Biol. 54 (12), 2995–3000. doi:10.1080/13880209.2016.1199042
Gupta, O. P., Sing, S., Bani, S., Sharma, N., Malhotra, S., Gupta, B. D., et al. (2000). Anti-inflammatory and anti-arthritic activities of silymarin acting through inhibition of 5-lipoxygenase. Phytomedicine 7 (1), 21–24. doi:10.1016/s0944-7113(00)80017-3
Hammerbacher, A., Kandasamy, D., Ullah, C., Schmidt, A., Wright, L. P., and Gershenzon, J. (2019). Flavanone-3-Hydroxylase plays an important role in the biosynthesis of spruce phenolic defenses against bark beetles and their fungal associates. Front. Plant Sci. 10, 208. doi:10.3389/fpls.2019.00208
Han, K., Li, C., Zhang, X., and Shang, L. (2019). DUXAP10 inhibition attenuates the proliferation and metastasis of hepatocellular carcinoma cells by regulation of the Wnt/β-catenin and PI3K/Akt signaling pathways. Biosci. Rep. 39 (5). doi:10.1042/bsr20181457
Hanafy, N. A. N., and El-Kemary, M. A. (2022). Silymarin/curcumin loaded albumin nanoparticles coated by chitosan as muco-inhalable delivery system observing anti-inflammatory and anti COVID-19 characterizations in oleic acid triggered lung injury and in vitro COVID-19 experiment. Int. J. Biol. Macromol. 198, 101–110. doi:10.1016/j.ijbiomac.2021.12.073
Hermanto, U., Zong, C. S., and Wang, L. H. (2001). ErbB2-overexpressing human mammary carcinoma cells display an increased requirement for the phosphatidylinositol 3-kinase signaling pathway in anchorage-independent growth. Oncogene 20 (51), 7551–7562. doi:10.1038/sj.onc.1204964
Hii, S. I., Nicol, D. L., Gotley, D. C., Thompson, L. C., Green, M. K., and Jonsson, J. R. (1998). Captopril inhibits tumour growth in a xenograft model of human renal cell carcinoma. Br. J. Cancer 77 (6), 880–883. doi:10.1038/bjc.1998.145
Imai-Sumida, M., Chiyomaru, T., Majid, S., Saini, S., Nip, H., Dahiya, R., et al. (2017). Silibinin suppresses bladder cancer through down-regulation of actin cytoskeleton and PI3K/Akt signaling pathways. Oncotarget 8 (54), 92032–92042. doi:10.18632/oncotarget.20734
Javed, S., Kohli, K., and Ali, M. (2011). Reassessing bioavailability of silymarin. Altern. Med. Rev. 16 (3), 239–249.
Javeed, A., Ahmed, M., Sajid, A. R., Sikandar, A., Aslam, M., Hassan, T. U., et al. (2022). Comparative assessment of phytoconstituents, antioxidant activity and chemical analysis of different parts of milk thistle Silybum marianum L. Molecules 27 (9), 2641. doi:10.3390/molecules27092641
Jin, L., Si-li, Y., Ya-ting, M., Tie-jun, Z., and Guang-rong, Z. (2016). Research progress in metabolic engineering and synthetic biology for natural lignan production. Chin. Traditional Herb. Drugs. Chin. Herb. Med. 47 (14), 2556–2562.
Kalinowska, M., Płońska, A., Trusiak, M., Gołębiewska, E., and Gorlewska-Pietluszenko, A. (2022). Comparing the extraction methods, chemical composition, phenolic contents and antioxidant activity of edible oils from Cannabis sativa and Silybum marianu seeds. Sci. Rep. 12 (1), 20609. doi:10.1038/s41598-022-25030-7
Kaloga, M. (1981). Isosilychristin, ein neues Flayonolignan aus Silybum marianum L. Gaertn./Isosilychristin, a New Flavonolignan from Silybum marianum L.Gaertn./Isosilychristin, a New Flavonolignan Silybum marianum L.Gaertn. 36(2), 262–265. doi:10.1515/znb-1981-0225
Karem, H. A., Omar, F. K., Ahmad, S. A., Nizar, M. M., and Ahmed, A.A.-S. (2021). Anticancer and antimutagenic activity of Silybum marianum L. and Eucalyptus camaldulensis Dehnh. against skin cancer induced by DMBA: in vitro and in vivo models. Pak J. Pharm. Sci. 34 (3), 987–993.
Karimzadeh, M. R., Masoudi Chelegahi, A., Shahbazi, S., and Reiisi, S. (2024). Co-treatment of silymarin and cisplatin inhibited cell proliferation, induced apoptosis in ovarian cancer. Mol. Biol. Rep. 51 (1), 118. doi:10.1007/s11033-023-09026-8
Khalil, M. R., El-Demerdash, R. S., Elminshawy, H. H., Mehanna, E. T., Mesbah, N. M., and Abo-Elmatty, D. M. (2021). Therapeutic effect of bone marrow mesenchymal stem cells in a rat model of carbon tetrachloride induced liver fibrosis. Biomed. J. 44 (5), 598–610. doi:10.1016/j.bj.2020.04.011
Kim, S. H., Choo, G. S., Yoo, E. S., Woo, J. S., Han, S. H., Lee, J. H., et al. (2019). Silymarin induces inhibition of growth and apoptosis through modulation of the MAPK signaling pathway in AGS human gastric cancer cells. Oncol. Rep. 42 (5), 1904–1914. doi:10.3892/or.2019.7295
Kim, S. H., Choo, G. S., Yoo, E. S., Woo, J. S., Lee, J. H., Han, S. H., et al. (2021). Silymarin inhibits proliferation of human breast cancer cells via regulation of the MAPK signaling pathway and induction of apoptosis. Oncol. Lett. 21 (6), 492. doi:10.3892/ol.2021.12753
Krepkova, L. V., Babenko, A. N., Saybel’, O. L., Lupanova, I. A., Kuzina, O. S., Job, K. M., et al. (2021). Valuable hepatoprotective plants - how can we optimize waste free uses of such highly versatile resources? Front. Pharmacol. 12, 738504. doi:10.3389/fphar.2021.738504
Kurokawa, H., Lenferink, A. E., Simpson, J. F., Pisacane, P. I., Sliwkowski, M. X., Forbes, J. T., et al. (2000). Inhibition of HER2/neu (erbB-2) and mitogen-activated protein kinases enhances tamoxifen action against HER2-overexpressing, tamoxifen-resistant breast cancer cells. Cancer Res. 60 (20), 5887–5894.
Kwon, D. Y., Jung, Y. S., Kim, S. J., Kim, Y. S., Choi, D. W., and Kim, Y. C. (2013). Alterations in sulfur amino acid metabolism in mice treated with silymarin: a novel mechanism of its action involved in enhancement of the antioxidant defense in liver. Planta Med. 79 (12), 997–1002. doi:10.1055/s-0032-1328704
Lashgarian, H. E., Adamii, V., Ghorbanzadeh, V., Chodari, L., Kamali, F., Akbari, S., et al. (2020). Silibinin inhibit cell migration through downregulation of RAC1 gene expression in highly metastatic breast cancer cell line. Drug Res. (Stuttg) 70 (10), 478–483. doi:10.1055/a-1223-1734
Lau, E. Y., Lo, J., Cheng, B. Y., Ma, M. K., Lee, J. M., Ng, J. K., et al. (2016). Cancer-associated fibroblasts regulate tumor-initiating cell plasticity in hepatocellular carcinoma through c-met/FRA1/HEY1 signaling. Cell Rep. 15 (6), 1175–1189. doi:10.1016/j.celrep.2016.04.019
Li, B., Shimizu, Y., Kobayashi, T., Terada, N., Yoshimura, K., Kamba, T., et al. (2012). Overexpression of ETS-1 is associated with malignant biological features of prostate cancer. Asian J. Androl. 14 (6), 860–863. doi:10.1038/aja.2012.107
Li, F., Sun, Y., Jia, J., Yang, C., Tang, X., Jin, B., et al. (2018). Silibinin attenuates TGF-β1-induced migration and invasion via EMT suppression and is associated with COX-2 downregulation in bladder transitional cell carcinoma. Oncol. Rep. 40 (6), 3543–3550. doi:10.3892/or.2018.6728
Li, H. B., Yang, Y. R., Mo, Z. J., Ding, Y., and Jiang, W. J. (2015). Silibinin improves palmitate-induced insulin resistance in C2C12 myotubes by attenuating IRS-1/PI3K/Akt pathway inhibition. Braz J. Med. Biol. Res. 48 (5), 440–446. doi:10.1590/1414-431x20144238
Li, S., Mai, S., Lin, Y., Zhang, J., He, L., Zhang, X., et al. (2022a). Effect and mechanism of silybin on protoporphyrin Ⅸ in INH/RFP induced liver injury model. Anat. Res. 44 (02), 151–155. doi:10.20021/j.cnki.1671-0770.2022.009
Li, W., Qu, X., Kang, X., Zhang, H., Zhang, X., Hu, H., et al. (2022b). Silibinin eliminates mitochondrial ROS and restores autophagy through IL6ST/JAK2/STAT3 signaling pathway to protect cardiomyocytes from doxorubicin-induced injury. Eur. J. Pharmacol. 929, 175153. doi:10.1016/j.ejphar.2022.175153
Li, X., Zhou, R., Han, Y., Zeng, J., Shi, L., Mao, Y., et al. (2023). Silibinin attenuates experimental periodontitis by downregulation of inflammation and oxidative stress. Oxid. Med. Cell Longev. 2023, 5617800. doi:10.1155/2023/5617800
Liu, C. (2021). Study on extraction technology of milk thistle protein Functional evaluation and preparation of bread. M.S: Harbin University of Commerce.
Liu, T., Zhang, M., and Wang, Y. (2012). Effects of milk thistle residue compound feed on pork quality. Feed Ind. 33 (11), 18–20.
Liu, Y., Liu, Z., and Zheng, Y. (2016). Research progress of milk thistle. J. Ginseng Res. 28 (02), 55–58. doi:10.19403/j.cnki.1671-1521.2016.02.016
Lu, P., Mamiya, T., Lu, L. L., Mouri, A., Zou, L., Nagai, T., et al. (2009). Silibinin prevents amyloid beta peptide-induced memory impairment and oxidative stress in mice. Br. J. Pharmacol. 157 (7), 1270–1277. doi:10.1111/j.1476-5381.2009.00295.x
Lv, Y., Gao, S., Xu, S., Du, G., Zhou, J., and Chen, J. (2017). Spatial organization of silybin biosynthesis in milk thistle [Silybum marianum (L.) Gaertn]. Plant J. 92 (6), 995–1004. doi:10.1111/tpj.13736
Ma, X., Yu, X., Li, R., Cui, J., Yu, H., Ren, L., et al. (2024). Berberine-silybin salt achieves improved anti-nonalcoholic fatty liver disease effect through regulating lipid metabolism. J. Ethnopharmacol. 319 (Pt 2), 117238. doi:10.1016/j.jep.2023.117238
Ma, Z., Liu, W., Zeng, J., Zhou, J., Guo, P., Xie, H., et al. (2015). Silibinin induces apoptosis through inhibition of the mTOR-GLI1-BCL2 pathway in renal cell carcinoma. Oncol. Rep. 34 (5), 2461–2468. doi:10.3892/or.2015.4224
MacDonald-Ramos, K., Michan, L., Martinez-Ibarra, A., and Cerbon, M. (2021). Silymarin is an ally against insulin resistance: a review. Ann. Hepatol. 23, 100255. doi:10.1016/j.aohep.2020.08.072
MacDonald-Ramos, K., Monroy, A., Bobadilla-Bravo, M., and Cerbon, M. (2024). Silymarin reduced insulin resistance in non-diabetic women with obesity. Int. J. Mol. Sci. 25 (4), 2050. doi:10.3390/ijms25042050
Mao, J. T., Xue, B., Lu, Q. Y., Lundmark, L., Burns, W., Yang, J., et al. (2023). Combinations of grape seed procyanidin extract and milk thistle silymarin extract against lung cancer - the role of MiR-663a and FHIT. Life Sci. 318, 121492. doi:10.1016/j.lfs.2023.121492
Marengo, A., Rosso, C., and Bugianesi, E. (2016). Liver cancer: connections with obesity, fatty liver, and cirrhosis. Annu. Rev. Med. 67, 103–117. doi:10.1146/annurev-med-090514-013832
Marmouzi, I., Bouyahya, A., Ezzat, S. M., El Jemli, M., and Kharbach, M. (2021). The food plant Silybum marianum (L.) Gaertn.: phytochemistry, Ethnopharmacology and clinical evidence. J. Ethnopharmacol. 265, 113303. doi:10.1016/j.jep.2020.113303
Martinelli, T. (2019). Identification of milk thistle shatter-resistant mutant lines with altered lignocellulosic profile for the complete domestication of the species. Crop Sci. 59 (5), 2119–2127. doi:10.2135/cropsci2019.02.0103
Martinelli, T., Whittaker, A., Benedettelli, S., Carboni, A., and Andrzejewska, J. (2017). The study of flavonolignan association patterns in fruits of diverging Silybum marianum (L.) Gaertn. chemotypes provides new insights into the silymarin biosynthetic pathway. Phytochemistry 144, 9–18. doi:10.1016/j.phytochem.2017.08.013
Matsumura, Y., and Ananthaswamy, H. N. (2004). Toxic effects of ultraviolet radiation on the skin. Toxicol. Appl. Pharmacol. 195 (3), 298–308. doi:10.1016/j.taap.2003.08.019
Mi, X. J., Choi, H. S., Perumalsamy, H., Shanmugam, R., Thangavelu, L., Balusamy, S. R., et al. (2022). Biosynthesis and cytotoxic effect of silymarin-functionalized selenium nanoparticles induced autophagy mediated cellular apoptosis via downregulation of PI3K/Akt/mTOR pathway in gastric cancer. Phytomedicine 99, 154014. doi:10.1016/j.phymed.2022.154014
Miranda, L. M. O., Agostini, L. D. C., Lima, W. G., Camini, F. C., and Costa, D. C. (2020). Silymarin attenuates hepatic and pancreatic redox imbalance independent of glycemic regulation in the alloxan-induced diabetic rat model. Biomed. Environ. Sci. 33 (9), 690–700. doi:10.3967/bes2020.090
Morishima, C., Shuhart, M. C., Wang, C. C., Paschal, D. M., Apodaca, M. C., Liu, Y., et al. (2010). Silymarin inhibits in vitro T-cell proliferation and cytokine production in hepatitis C virus infection. Gastroenterology 138 (2), 671–681. doi:10.1053/j.gastro.2009.09.021
Mueller, A., Odze, R., Jenkins, T. D., Shahsesfaei, A., Nakagawa, H., Inomoto, T., et al. (1997). A transgenic mouse model with cyclin D1 overexpression results in cell cycle, epidermal growth factor receptor, and p53 abnormalities. Cancer Res. 57 (24), 5542–5549.
Mukhtar, S., Xiaoxiong, Z., Qamer, S., Saad, M., Mubarik, M. S., Mahmoud, A. H., et al. (2021). Hepatoprotective activity of silymarin encapsulation against hepatic damage in albino rats. Saudi J. Biol. Sci. 28 (1), 717–723. doi:10.1016/j.sjbs.2020.10.063
Nazir, S. U., Kumar, R., Singh, A., Khan, A., Tanwar, P., Tripathi, R., et al. (2019). Breast cancer invasion and progression by MMP-9 through Ets-1 transcription factor. Gene 711, 143952. doi:10.1016/j.gene.2019.143952
Nichols, J. A., and Katiyar, S. K. (2010). Skin photoprotection by natural polyphenols: anti-inflammatory, antioxidant and DNA repair mechanisms. Arch. Dermatol Res. 302 (2), 71–83. doi:10.1007/s00403-009-1001-3
Nusse, R., and Clevers, H. (2017). Wnt/β-Catenin signaling, disease, and emerging therapeutic modalities. Cell 169 (6), 985–999. doi:10.1016/j.cell.2017.05.016
Papadimou, S. G., and Golia, E. E. (2024). Green and sustainable practices for an energy plant cultivation on naturally contaminated versus spiked soils. The impact of ageing soil pollution in the circular economy framework. Environ. Res. 246, 118130. doi:10.1016/j.envres.2024.118130
Pelter, A., and Hänsel, R. (1968). The structure of silybin (silybum substance E6), the first flavonolignan. Tetrahedron Lett. 9 (25), 2911–2916. doi:10.1016/S0040-4039(00)89610-0
Pelter, A., Hansel, R., and Kaloga, M. (1977). The structure of silychristine. Tetrahedron Lett. 18 (51), 4547–4548. doi:10.1016/S0040-4039(01)83563-2
Peng, R., Wang, S., Wang, R., Wang, Y., Wu, Y., and Yuan, Y. (2017). Antifibrotic effects of tanshinol in experimental hepatic fibrosis by targeting PI3K/AKT/mTOR/p70S6K1 signaling pathways. Discov. Med. 23 (125), 81–94.
Pereira, C., Barreira, J. C., Calhelha, R. C., Lopes, M., Queiroz, M. J., Vilas-Boas, M., et al. (2015). Is honey able to potentiate the antioxidant and cytotoxic properties of medicinal plants consumed as infusions for hepatoprotective effects? Food Funct. 6 (5), 1435–1442. doi:10.1039/c4fo01206b
Pferschy-Wenzig, E. M., Kunert, O., Thumann, T., Moissl-Eichinger, C., and Bauer, R. (2023). Characterization of metabolites from milk thistle flavonolignans generated by human fecal microbiota. Phytochemistry 215, 113834. doi:10.1016/j.phytochem.2023.113834
Poluha, W., Poluha, D. K., Chang, B., Crosbie, N. E., Schonhoff, C. M., Kilpatrick, D. L., et al. (1996). The cyclin-dependent kinase inhibitor p21 (WAF1) is required for survival of differentiating neuroblastoma cells. Mol. Cell Biol. 16 (4), 1335–1341. doi:10.1128/mcb.16.4.1335
Polyak, K., Kato, J. Y., Solomon, M. J., Sherr, C. J., Massague, J., Roberts, J. M., et al. (1994). p27Kip1, a cyclin-Cdk inhibitor, links transforming growth factor-beta and contact inhibition to cell cycle arrest. Genes Dev. 8 (1), 9–22. doi:10.1101/gad.8.1.9
Polyak, S. J., Morishima, C., Shuhart, M. C., Wang, C. C., Liu, Y., and Lee, D. Y. (2007). Inhibition of T-cell inflammatory cytokines, hepatocyte NF-kappaB signaling, and HCV infection by standardized Silymarin. Gastroenterology 132 (5), 1925–1936. doi:10.1053/j.gastro.2007.02.038
Pradhan, S. C., and Girish, C. (2006). Hepatoprotective herbal drug, silymarin from experimental pharmacology to clinical medicine. Indian J. Med. Res. 124 (5), 491–504.
Rahnama, S., Tehrankhah, Z. M., Mohajerani, F., Mohammadi, F. S., Yeganeh, Z. Y., Najafi, F., et al. (2023). Milk thistle nano-micelle formulation promotes cell cycle arrest and apoptosis in hepatocellular carcinoma cells through modulating miR-155-3p/SOCS2/PHLDA1 signaling axis. BMC Complement. Med. Ther. 23 (1), 337. doi:10.1186/s12906-023-04168-5
Ramasamy, K., and Agarwal, R. (2008). Multitargeted therapy of cancer by silymarin. Cancer Lett. 269 (2), 352–362. doi:10.1016/j.canlet.2008.03.053
Rambaldi, A., Jacobs, B. P., and Gluud, C. (2007). Milk thistle for alcoholic and/or hepatitis B or C virus liver diseases. Cochrane Database Syst. Rev. 2007 (4), Cd003620. doi:10.1002/14651858.CD003620.pub3
Raouf, A., Li, V., Kola, I., Watson, D. K., and Seth, A. (2000). The Ets1 proto-oncogene is upregulated by retinoic acid: characterization of a functional retinoic acid response element in the Ets1 promoter. Oncogene 19 (15), 1969–1974. doi:10.1038/sj.onc.1203505
Rosário, M., and Birchmeier, W. (2003). How to make tubes: signaling by the Met receptor tyrosine kinase. Trends Cell Biol. 13 (6), 328–335. doi:10.1016/s0962-8924(03)00104-1
Saller, R., Brignoli, R., Melzer, J., and Meier, R. (2008). An updated systematic review with meta-analysis for the clinical evidence of silymarin. Forsch Komplementmed 15 (1), 9–20. doi:10.1159/000113648
Saller, R., Meier, R., and Brignoli, R. (2001). The use of silymarin in the treatment of liver diseases. Drugs 61 (14), 2035–2063. doi:10.2165/00003495-200161140-00003
Salomone, F., Barbagallo, I., Godos, J., Lembo, V., Currenti, W., Cinà, D., et al. (2017). Silibinin restores NAD⁺ levels and induces the SIRT1/AMPK pathway in non-alcoholic fatty liver. Nutrients 9 (10), 1086. doi:10.3390/nu9101086
Sayeed, M. M. (1998). Neutrophil signaling alteration: an adverse inflammatory response after burn shock. Med. (B Aires) 58 (4), 386–392.
Sayyed, A., Heuertz, R., and Ezekiel, U. R. (2022). Curcumin, but not its degradation products, in combination with silibinin is primarily responsible for the inhibition of colon cancer cell proliferation. Micropubl. Biol. 2022. doi:10.17912/micropub.biology.000617
Schwacha, M. G., and Chaudry, I. H. (2002). The cellular basis of post-burn immunosuppression: macrophages and mediators. Int. J. Mol. Med. 10 (3), 239–243.
Sener, G., Sehirli, O., Cetinel, S., Ercan, F., Yüksel, M., Gedik, N., et al. (2005a). Amelioration of sepsis-induced hepatic and ileal injury in rats by the leukotriene receptor blocker montelukast. Prostagl. Leukot. Essent. Fat. Acids 73 (6), 453–462. doi:10.1016/j.plefa.2005.07.008
Sener, G., Toklu, H., Ercan, F., and Erkanli, G. (2005b). Protective effect of beta-glucan against oxidative organ injury in a rat model of sepsis. Int. Immunopharmacol. 5 (9), 1387–1396. doi:10.1016/j.intimp.2005.03.007
Shaw, R. J., and Cantley, L. C. (2006). Ras, PI(3)K and mTOR signalling controls tumour cell growth. Nature 441 (7092), 424–430. doi:10.1038/nature04869
Sherr, C. J. (1996). Cancer cell cycles. Science 274 (5293), 1672–1677. doi:10.1126/science.274.5293.1672
Shin, S., Lee, J. A., Kim, M., Kum, H., Jung, E., and Park, D. (2015). Anti-glycation activities of phenolic constituents from Silybum marianum (Milk Thistle) flower in vitro and on human explants. Molecules 20 (3), 3549–3564. doi:10.3390/molecules20033549
Singh, R. P., and Agarwal, R. (2009). Cosmeceuticals and silibinin. Clin. Dermatol 27 (5), 479–484. doi:10.1016/j.clindermatol.2009.05.012
Singh, R. P., Deep, G., Chittezhath, M., Kaur, M., Dwyer-Nield, L. D., Malkinson, A. M., et al. (2006). Effect of silibinin on the growth and progression of primary lung tumors in mice. J. Natl. Cancer Inst. 98 (12), 846–855. doi:10.1093/jnci/djj231
Singh, R. P., Dhanalakshmi, S., and Agarwal, R. (2002). Phytochemicals as cell cycle modulators--a less toxic approach in halting human cancers. Cell Cycle 1 (3), 155–160. doi:10.4161/cc.1.3.117
Singhal, N. K., Srivastava, G., Patel, D. K., Jain, S. K., and Singh, M. P. (2011). Melatonin or silymarin reduces maneb- and paraquat-induced Parkinson's disease phenotype in the mouse. J. Pineal Res. 50 (2), 97–109. doi:10.1111/j.1600-079X.2010.00819.x
Song, X. (2023). Protective effect of silybin on hepatocyte injury induced by ethanol or acetaldehyde. Shenyang Pharmaceutical University.
Soto, C., Mena, R., Luna, J., Cerbón, M., Larrieta, E., Vital, P., et al. (2004). Silymarin induces recovery of pancreatic function after alloxan damage in rats. Life Sci. 75 (18), 2167–2180. doi:10.1016/j.lfs.2004.04.019
Soto, C., Pérez, J., García, V., Uría, E., Vadillo, M., and Raya, L. (2010). Effect of silymarin on kidneys of rats suffering from alloxan-induced diabetes mellitus. Phytomedicine 17 (14), 1090–1094. doi:10.1016/j.phymed.2010.04.011
Soto, C., Recoba, R., Barrón, H., Alvarez, C., and Favari, L. (2003). Silymarin increases antioxidant enzymes in alloxan-induced diabetes in rat pancreas. Comp. Biochem. Physiol. C Toxicol. Pharmacol. 136 (3), 205–212. doi:10.1016/s1532-0456(03)00214-x
Soto, C. P., Perez, B. L., Favari, L. P., and Reyes, J. L. (1998). Prevention of alloxan-induced diabetes mellitus in the rat by silymarin. Comp. Biochem. Physiol. C Pharmacol. Toxicol. Endocrinol. 119 (2), 125–129. doi:10.1016/s0742-8413(97)00198-9
Stastnik, O., Pavlata, L., and Mrkvicova, E. (2020). The milk thistle seed cakes and hempseed cakes are potential feed for poultry. Anim. (Basel) 10 (8), 1384. doi:10.3390/ani10081384
Stolf, A. M., Cardoso, C. C., and Acco, A. (2017). Effects of silymarin on diabetes mellitus complications: a review. Phytotherapy Res. 31 (3), 366–374. doi:10.1002/ptr.5768
Sun, R., Xu, D., Wei, Q., Zhang, B., Aa, J., Wang, G., et al. (2020). Silybin ameliorates hepatic lipid accumulation and modulates global metabolism in an NAFLD mouse model. Biomed. Pharmacother. 123, 109721. doi:10.1016/j.biopha.2019.109721
Surai, P. (2015). Silymarin as a natural antioxidant: an overview of the current evidence and perspectives. Antioxidants 4 (1), 204–247. doi:10.3390/antiox4010204
Taghiabadi, E., Imenshahidi, M., Abnous, K., Mosafa, F., Sankian, M., Memar, B., et al. (2012). Protective effect of silymarin against acrolein-induced cardiotoxicity in mice. Evidence-Based Complementary Altern. Med. 2012, 352091–352114. doi:10.1155/2012/352091
Tan, M., Jing, T., Lan, K. H., Neal, C. L., Li, P., Lee, S., et al. (2002). Phosphorylation on tyrosine-15 of p34(Cdc2) by ErbB2 inhibits p34(Cdc2) activation and is involved in resistance to taxol-induced apoptosis. Mol. Cell 9 (5), 993–1004. doi:10.1016/s1097-2765(02)00510-5
Templeton, A. J., Diez-Gonzalez, L., Ace, O., Vera-Badillo, F., Seruga, B., Jordán, J., et al. (2014). Prognostic relevance of receptor tyrosine kinase expression in breast cancer: a meta-analysis. Cancer Treat. Rev. 40 (9), 1048–1055. doi:10.1016/j.ctrv.2014.08.003
Toklu, H. Z., Tunalı-Akbay, T., Erkanlı, G., Yüksel, M., Ercan, F., and Şener, G. (2007). Silymarin, the antioxidant component of Silybum marianum, protects against burn-induced oxidative skin injury. Burns 33 (7), 908–916. doi:10.1016/j.burns.2006.10.407
Toklu, H. Z., Tunali Akbay, T., Velioglu-Ogunc, A., Ercan, F., Gedik, N., Keyer-Uysal, M., et al. (2008). Silymarin, the antioxidant component of Silybum marianum, prevents sepsis-induced acute lung and brain injury. J. Surg. Res. 145 (2), 214–222. doi:10.1016/j.jss.2007.03.072
Tyagi, A., Agarwal, C., Harrison, G., Glode, L. M., and Agarwal, R. (2004). Silibinin causes cell cycle arrest and apoptosis in human bladder transitional cell carcinoma cells by regulating CDKI-CDK-cyclin cascade, and caspase 3 and PARP cleavages. Carcinogenesis 25 (9), 1711–1720. doi:10.1093/carcin/bgh180
Tyagi, A., Singh, R. P., Ramasamy, K., Raina, K., Redente, E. F., Dwyer-Nield, L. D., et al. (2009). Growth inhibition and regression of lung tumors by silibinin: modulation of angiogenesis by macrophage-associated cytokines and nuclear factor-kappaB and signal transducers and activators of transcription 3. Cancer Prev. Res. (Phila) 2 (1), 74–83. doi:10.1158/1940-6207.Capr-08-0095
Verdura, S., Cuyàs, E., Ruiz-Torres, V., Micol, V., Joven, J., Bosch-Barrera, J., et al. (2021). Lung cancer management with silibinin: a historical and translational perspective. Pharmaceuticals 14 (6), 559. doi:10.3390/ph14060559
Wagner, H., Hörhammer, L., and Seitz, M. (1968). Chemical evaluation of a silymarin-containing flavonoid concentrate from Silybum marianum (L.) Gaertn. Arzneimittelforschung 18 (6), 696–698.
Wagner, H., Seligmann, O., Hörhammer, L., Seitz, M., and Sonnenbichler, J. (1971). Zur struktur von silychristin, einem zweiten silymarin-isomeren aus silybum marianum. Tetrahedron Lett. 12 (22), 1895–1899. doi:10.1016/S0040-4039(01)96737-1
Wagner, H., Seligmann, O., Seitz, M., Abraham, D., and Sonnenbichler, J. (1976). Notizen: Silydianin und Silychristin, zwei isomere Silymarine aus Silybum marianum L. Gaertn. (Mariendistel)/Silydianin and Silychristin, two Isomeric Silymarins from Silybum marianum L. Gaertn. (milk thistle), zwei isomere Silymarine aus Silybum marianum L. Gaertn. (Mariendistel)/Silydianin Silychristin, two Isomeric Silymarins Silybum marianum L. Gaertn. (milk thistle). 31(6), 876–884. doi:10.1515/znb-1976-0630
Wagoner, J., Negash, A., Kane, O. J., Martinez, L. E., Nahmias, Y., Bourne, N., et al. (2010). Multiple effects of silymarin on the hepatitis C virus lifecycle. Hepatology 51 (6), 1912–1921. doi:10.1002/hep.23587
Wang, J., Zhang, L., Cao, H., Shi, X., Zhang, X., Gao, Z., et al. (2022). Silibinin improves L-cell mass and function through an estrogen receptor-mediated antioxidative mechanism. Phytomedicine 99, 154022. doi:10.1016/j.phymed.2022.154022
Wang, L. G., Liu, X. M., Kreis, W., and Budman, D. R. (1997). Down-regulation of prostate-specific antigen expression by finasteride through inhibition of complex formation between androgen receptor and steroid receptor-binding consensus in the promoter of the PSA gene in LNCaP cells. Cancer Res. 57 (4), 714–719.
Wang, M. J., Lin, W. W., Chen, H. L., Chang, Y. H., Ou, H. C., Kuo, J. S., et al. (2002). Silymarin protects dopaminergic neurons against lipopolysaccharide-induced neurotoxicity by inhibiting microglia activation. Eur. J. Neurosci. 16 (11), 2103–2112. doi:10.1046/j.1460-9568.2002.02290.x
Wang, X., Zhang, Z., and Wu, S.-C. (2020a). Health benefits of Silybum marianum: phytochemistry, pharmacology, and applications. J. Agric. Food Chem. 68 (42), 11644–11664. doi:10.1021/acs.jafc.0c04791
Wang, X., Zhang, Z., and Wu, S. C. (2020b). Health benefits of Silybum marianum: phytochemistry, pharmacology, and applications. J. Agric. Food Chem. 68 (42), 11644–11664. doi:10.1021/acs.jafc.0c04791
Wang, Y. (2023). Inhibition of oxidative stress by rnai targeting cyp2e1 alleviates alcoholic liver disease a in mice. Jiangsu University.
Wei, P., Li, X., Wang, S., Dong, Y., Yin, H., Gu, Z., et al. (2022). Silibinin ameliorates formaldehyde-induced cognitive impairment by inhibiting oxidative stress. Oxid. Med. Cell Longev. 2022, 5981353. doi:10.1155/2022/5981353
Wei, Y., Zhuang, Y., Zhang, Y., Luo, L., Yu, B., and Zeng, J. (2024). Role of heat shock protein 70 in silibinin-induced apoptosis in bladder cancer. J. Cancer 15 (1), 79–89. doi:10.7150/jca.88668
Wianowska, D., and Wiśniewski, M. (2015). Simplified procedure of silymarin extraction from Silybum marianum L. Gaertner. J. Chromatogr. Sci. 53 (2), 366–372. doi:10.1093/chromsci/bmu049
Wu, J., Lou, Y. G., Yang, X. L., Wang, R., Zhang, R., Aa, J. Y., et al. (2023a). Silybin regulates P450s activity by attenuating endoplasmic reticulum stress in mouse nonalcoholic fatty liver disease. Acta Pharmacol. Sin. 44 (1), 133–144. doi:10.1038/s41401-022-00924-4
Wu, S., Chen, G., Chen, E. Y., Farshidpour, L. S., Zhang, Q., Wang, G., et al. (2023b). Core structure-activity relationship studies of 5,7,20-O-trimethylsilybins in prostate cancer cell models. Pharm. (Basel) 16 (4), 531. doi:10.3390/ph16040531
Xu, R., Qiu, S., Zhang, J., Liu, X., Zhang, L., Xing, H., et al. (2022). Silibinin schiff base derivatives counteract CCl(4)-induced acute liver injury by enhancing anti-inflammatory and antiapoptotic bioactivities. Drug Des. Devel Ther. 16, 1441–1456. doi:10.2147/dddt.S356847
Yang, J., Liang, J., Shao, L., Liu, L., Gao, K., Zhang, J.-L., et al. (2020). Green production of silybin and isosilybin by merging metabolic engineering approaches and enzymatic catalysis. Metab. Eng. 59, 44–52. doi:10.1016/j.ymben.2020.01.007
Yassin, N. Y. S., AbouZid, S. F., El-Kalaawy, A. M., Ali, T. M., Almehmadi, M. M., and Ahmed, O. M. (2022). Silybum marianum total extract, silymarin and silibinin abate hepatocarcinogenesis and hepatocellular carcinoma growth via modulation of the HGF/c-Met, Wnt/β-catenin, and PI3K/Akt/mTOR signaling pathways. Biomed. Pharmacother. 145, 112409. doi:10.1016/j.biopha.2021.112409
Yassin, N. Y. S., AbouZid, S. F., El-Kalaawy, A. M., Ali, T. M., Elesawy, B. H., and Ahmed, O. M. (2021). Tackling of renal carcinogenesis in wistar rats by Silybum marianum total extract, silymarin, and silibinin via modulation of oxidative stress, apoptosis, Nrf2, PPARγ, NF-κB, and PI3K/Akt signaling pathways. Oxid. Med. Cell Longev. 2021, 7665169. doi:10.1155/2021/7665169
Yasuda, M., Shimizu, I., Shiba, M., and Ito, S. (1999). Suppressive effects of estradiol on dimethylnitrosamine-induced fibrosis of the liver in rats. Hepatology 29 (3), 719–727. doi:10.1002/hep.510290307
Yoshida, A., Hsu, L. C., and Davé, V. (1992). Retinal oxidation activity and biological role of human cytosolic aldehyde dehydrogenase. Enzyme 46 (4-5), 239–244. doi:10.1159/000468794
You, Y., He, Q., Lu, H., Zhou, X., Chen, L., Liu, H., et al. (2020). Silibinin induces G2/M cell cycle arrest by activating drp1-dependent mitochondrial fission in cervical cancer. Front. Pharmacol. 11, 271. doi:10.3389/fphar.2020.00271
Youn, C. K., Cho, S. I., Lee, M. Y., Jeon, Y. J., and Lee, S. K. (2017). Inhibition of ERK1/2 by silymarin in mouse mesangial cells. Korean J. Physiol. Pharmacol. 21 (1), 117–124. doi:10.4196/kjpp.2017.21.1.117
Yu, H., Saif, M. S., Hasan, M., Zafar, A., Zhao, X., Waqas, M., et al. (2023). Designing a silymarin nanopercolating system using CME@ZIF-8: an approach to hepatic injuries. ACS Omega 8 (50), 48535–48548. doi:10.1021/acsomega.3c08494
Zappavigna, S., Vanacore, D., Lama, S., Potenza, N., Russo, A., Ferranti, P., et al. (2019). Silybin-induced apoptosis occurs in parallel to the increase of ceramides synthesis and miRNAs secretion in human hepatocarcinoma cells. Int. J. Mol. Sci. 20 (9), 2190. doi:10.3390/ijms20092190
Zarrouk, A., Martine, L., Grégoire, S., Nury, T., Meddeb, W., Camus, E., et al. (2019). Profile of fatty acids, tocopherols, phytosterols and polyphenols in mediterranean oils (argan oils, olive oils, milk thistle seed oils and nigella seed oil) and evaluation of their antioxidant and cytoprotective activities. Curr. Pharm. Des. 25 (15), 1791–1805. doi:10.2174/1381612825666190705192902
Zhai, S., Li, N., Chen, B., Kou, J., Xing, W., and Zhang, P. (2019). Research progress of milk thistle in the treatment of chronic hepatitis. Chin. J. Clin. Pharmacol. Ther. 24 (05), 573–579.
Zhang, C. (2011). Study on chemical constituents and antioxidant activities of milk thistle. M.S: Northwest A&F University.
Zhang, M., Weng, W., Zhang, Q., Wu, Y., Ni, S., Tan, C., et al. (2018a). The lncRNA NEAT1 activates Wnt/β-catenin signaling and promotes colorectal cancer progression via interacting with DDX5. J. Hematol. Oncol. 11 (1), 113. doi:10.1186/s13045-018-0656-7
Zhang, R., Xu, D., Zhang, Y., Wang, R., Yang, N., Lou, Y., et al. (2021). Silybin restored CYP3A expression through the sirtuin 2/nuclear factor κ-B pathway in mouse nonalcoholic fatty liver disease. Drug Metab. Dispos. 49 (9), 770–779. doi:10.1124/dmd.121.000438
Zhang, Y., Ge, Y., Chen, Y., Li, Q., Chen, J., Dong, Y., et al. (2012). Cellular and molecular mechanisms of silibinin induces cell-cycle arrest and apoptosis on HeLa cells. Cell Biochem. Funct. 30 (3), 243–248. doi:10.1002/cbf.1842
Zhang, Y., Ge, Y., Ping, X., Yu, M., Lou, D., and Shi, W. (2018b). Synergistic apoptotic effects of silibinin in enhancing paclitaxel toxicity in human gastric cancer cell lines. Mol. Med. Rep. 18 (2), 1835–1841. doi:10.3892/mmr.2018.9129
Zhang, Y., Hai, J., Cao, M., Zhang, Y., Pei, S., Wang, J., et al. (2013a). Silibinin ameliorates steatosis and insulin resistance during non-alcoholic fatty liver disease development partly through targeting IRS-1/PI3K/Akt pathway. Int. Immunopharmacol. 17 (3), 714–720. doi:10.1016/j.intimp.2013.08.019
Zhang, Y., Li, Q., Ge, Y., Chen, Y., Chen, J., Dong, Y., et al. (2013b). Silibinin triggers apoptosis and cell-cycle arrest of SGC7901 cells. Phytother. Res. 27 (3), 397–403. doi:10.1002/ptr.4733
Zhao, X., Wang, B., Wang, T., and Li, X. (2006). Inhibitory effects of silymarin on hepatic fibrosis induced by dimethylnitrosamine: experiment with rats. Chin. Med. J. 86 (36), 2563–2566.
Zheng, N., Liu, L., Liu, W.-w., Li, F., Hayashi, T., Tashiro, S.-i., et al. (2016). Crosstalk of ROS/RNS and autophagy in silibinin-induced apoptosis of MCF-7 human breast cancer cells in vitro. Acta Pharmacol. Sin. 38 (2), 277–289. doi:10.1038/aps.2016.117
Zheng, N., Liu, L., Liu, W. W., Li, F., Hayashi, T., Tashiro, S. I., et al. (2017). Crosstalk of ROS/RNS and autophagy in silibinin-induced apoptosis of MCF-7 human breast cancer cells in vitro. Acta Pharmacol. Sin. 38 (2), 277–289. doi:10.1038/aps.2016.117
Glossary
Keywords: Silybum marianum, phytochemistry, pharmacology, synthetic biology, comprehensive utilization
Citation: Zhang X, Liu M, Wang Z, Wang P, Kong L, Wu J, Wu W, Ma L, Jiang S, Ren W, Du L, Ma W and Liu X (2024) A review of the botany, phytochemistry, pharmacology, synthetic biology and comprehensive utilization of Silybum marianum. Front. Pharmacol. 15:1417655. doi: 10.3389/fphar.2024.1417655
Received: 15 April 2024; Accepted: 20 June 2024;
Published: 11 July 2024.
Edited by:
Javier Echeverria, University of Santiago, ChileReviewed by:
Alejandro Palacios, National University of La Plata, ArgentinaMarco Cerbon, National Autonomous University of Mexico, Mexico
Copyright © 2024 Zhang, Liu, Wang, Wang, Kong, Wu, Wu, Ma, Jiang, Ren, Du, Ma and Liu. This is an open-access article distributed under the terms of the Creative Commons Attribution License (CC BY). The use, distribution or reproduction in other forums is permitted, provided the original author(s) and the copyright owner(s) are credited and that the original publication in this journal is cited, in accordance with accepted academic practice. No use, distribution or reproduction is permitted which does not comply with these terms.
*Correspondence: Wei Ma, mawei@hljucm.edu.cn; Xiubo Liu, zyylxb@126.com