- 1Metro College of Health Sciences and Research, Greater Noida, India
- 2School of Pharmacy, Sharda University, Greater Noida, India
- 3Department of Biology, College of Science, Imam Mohammad Ibn Saud Islamic University (IMSIU), Riyadh, Saudi Arabia
- 4Amity Institute of Pharmacy, Amity University, Noida, India
- 5Department of Biotechnology, GLA University, Mathura, India
- 6Department of Computer Engineering, Parul University, Vadodara, India
- 7Department of Biochemistry, College of Medicine, Imam Mohammad Ibn Saud Islamic University (IMSIU), Riyadh, Saudi Arabia
- 8Department of Biology, College of Science, Imam Mohammad Ibn Saud Islamic University, Riyadh, Saudi Arabia
- 9Department of Biochemistry, Faculty of Science, Ain Shams University, Cairo, Egypt
- 10Department of Basic Medical Sciences, College of Medicine, Prince Sattam bin Abdulaziz University, Al-Kharj, Saudi Arabia
- 11School of Pharmacy, KPJ Healthcare University, Nilai, Malaysia
- 12Department of Biotechnology, Graphic Era (Deemed to be University), Dehradun, Uttarakhand, India
- 13Lloyd School of Pharmacy, Greater Noida, India
- 14Biological and Bio-computational Laboratory, Department of Life Science, Sharda School of Basic Sciences and Research, Sharda University, Greater Noida, India
- 15DST-FIST Laboratory, Department of Life Sciences, School of Basic Sciences and Research, Sharda University, Greater Noida, India
Cancer prevention is currently envisioned as a molecular-based approach to prevent carcinogenesis in pre-cancerous stages, i.e., dysplasia and carcinoma in situ. Cancer is the second-leading cause of mortality worldwide, and a more than 61% increase is expected by 2040. A detailed exploration of cancer progression pathways, including the NF-kβ signaling pathway, Wnt-B catenin signaling pathway, JAK-STAT pathway, TNF-α-mediated pathway, MAPK/mTOR pathway, and apoptotic and angiogenic pathways and effector molecules involved in cancer development, has been discussed in the manuscript. Critical evaluation of these effector molecules through molecular approaches using phytomolecules can intersect cancer formation and its metastasis. Manipulation of effector molecules like NF-kβ, SOCS, β-catenin, BAX, BAK, VEGF, STAT, Bcl2, p53, caspases, and CDKs has played an important role in inhibiting tumor growth and its spread. Plant-derived secondary metabolites obtained from natural sources have been extensively studied for their cancer-preventing potential in the last few decades. Eugenol, anethole, capsaicin, sanguinarine, EGCG, 6-gingerol, and resveratrol are some examples of such interesting lead molecules and are mentioned in the manuscript. This work is an attempt to put forward a comprehensive approach to understanding cancer progression pathways and their management using effector herbal molecules. The role of different plant metabolites and their chronic toxicity profiling in modulating cancer development pathways has also been highlighted.
Highlights
• Molecular investigation of pre-cancerous lesion development in cancer progression pathways is identified as a potential target in cancer prevention.
• Modulation in the expression of effector molecules like SOCS, p53, VEGF, STAT, BAX, Bcl2, NF-κB, CDKs, and β-catenin may provide an opportunity to hamper the carcinogenic continuum at different stages of progression.
• Phytochemicals like 6-gingerol, silymarin, resveratrol, apigenin, EGCG, and β-carotene impair signaling pathways and inhibit the development of cancer in situ, thereby preventing the progression to invasive cancer.
1 Introduction
Cancer is one of the leading causes of death around the globe. Continuous exposure to risk factors, including physiological, behavioral, environmental, and professional factors, is attributed to the increase in cancer burden worldwide (Eloranta et al., 2021; Ferlay et al., 2021). Approximately 90% of cancer cases are an outcome of environmental factors and somatic mutations, and the rest are due to mutations in the germline (Sung et al., 2021).
According to the World Health Organization (WHO) and the Global Cancer Observatory (GCO), more than 19 million people are currently affected by cancer, and the number of active cases may rise to more than 28 million people by 2040 in a calendar year. Cancer mortality data have been analyzed by the WHO and GCO from 1930 to 2020 (Mathur et al., 2020; Siegel et al., 2020). Lung and bronchus cancer have a higher mortality rate, followed by breast, uterus, and stomach cancer (Mathur et al., 2020; Siegel et al., 2020). Figure 1 depicts the number of cancer-afflicted patients and the mortality reported in recent databases and projections up to 2040. Prostate cancer has been reported to be the most common cancer among males (29%), and breast cancer (31%) is most common among females (Figure 1C). Cancer death rates have been declining in males and females since the early 1990s; from 1991 to 2019, the combined death rate dropped by 32%. Breast cancer death rates changed little between 1930 and 1989 but decreased by 42% from 1989 to 2019 due to earlier detection and improved treatment. It is indicated that there has been a significant drop in the mortality rates for a few specific cancers, like stomach and colorectal cancer, in recent years. (Figure 1D). However, the predicted new cancer cases per year in Asia and Africa are expected to rise to 59% and 89% of the current prevalence, respectively, by 2040 (Jones and Baylin, 2007).
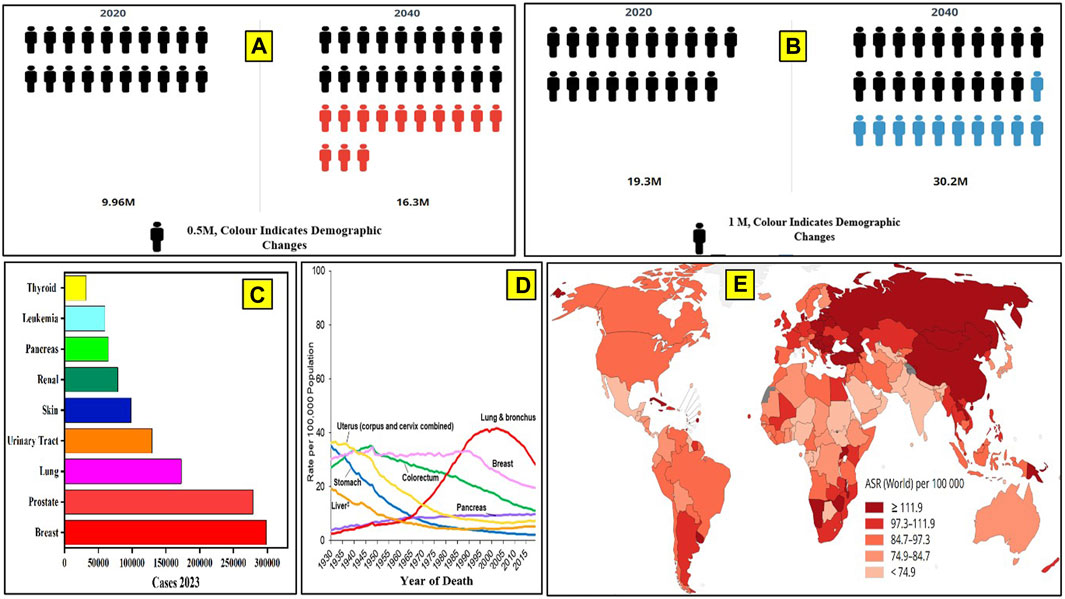
Figure 1. Global cancer data. (A) Number of cancer cases in 2020 and the cases projected by 2040, as per WHO. (B) Number of deaths due to cancer reported until 2020 and the number of deaths projected up to the year 2040. (C) Number of cancer cases reported in the United States in 2023. (D) Cancer mortality data from 1930 to 2020. (E) Number of cancer-related deaths in different continents in a calendar year (2020).
Cancer progression is a complex biological process with a diversified understanding (Jones and Baylin, 2007). However, different pathways have been extensively studied in the last few decades due to advancements in imagining and analysis technology (Arruebo et al., 2011). The general landscape of oncogenesis has been depicted in Figure 2 which represents several risk factors that convert normal healthy cells into abnormal cancerous cells through molecular manifestations (Wang et al., 2017). Carcinogens, biological agents, and inflammation may trigger deregulated metabolism, which leads to genetic mutation and may result in the development of cancer (Gandhi et al., 2014). These manifestations at the cellular level may start interfering at cell cycle checkpoints (Liu C. G. et al., 2020). Figure 2 depicts that the altered cell cycle affects cell signaling and also starts avoiding immune cells (Zheng and Ma, 2017). A series of biological events are the reason for the transformation of a healthy cell into a cancerous cell, including carcinogens, genetic alterations, inflammation, and dysregulated metabolism. At the molecular level, such factors of cancer development disbalance the levels of tumor suppressor genes and oncogenes, resulting in cell cycle deregulation. Furthermore, replicative immortality and altered cell signaling mechanisms are causing the progression of cancer and have been reported to avoid immune cells, growth suppressors, and angiogenesis, which yields the metastasis of cancerous cells. The role of cell death resistance and uninterrupted angiogenesis has also been linked to cell proliferation and cancer development (Figure 2).
Nowadays, chemotherapy, targeted therapy, radiotherapy, and surgical techniques are commonly employed in cancer treatment interventions. However, each of the conventional treatment options possesses its inherent challenges that increase the chances of low quality of life for cancer patients, including side effects and the peril of relapse (Han et al., 2022). Chemotherapies like platinum-based drugs (carboplatin and cisplatin) have been reported to cause nephrotoxicity, cardiovascular diseases, and low-grade gliomas in up to 47% of children. Lavniti M. et al. have reported that oxaliplatin and etoposides have also induced neuropathy, which may cause permanent gastrointestinal dysfunctions with CNS depression in cancer-afflicted and treated patients (Eiermann et al., 2001; Shipitsin and Polyak, 2008). Tachycardia, decreased immunity, and bone marrow depression are major drawbacks of antibody-based treatment (rituximab) and targeted therapies. Surgery and radiation therapy for cancer management induce anorexia, lymphedema, neutropenia, sepsis, hair-loss, and immunological disorders (Spychalski et al., 2007). Many of the adverse effects of cancer treatments are managed by some phytoconstituents. However, treatments are always associated with various types of adversities. Hence, scientists and clinicians around the globe advocate the use of dietary phytoconstituents to prevent cancer progression. Interestingly, the amount of phytoconstituents present in diets is not clinically significant enough to block the cancer progression if initiated (Liu et al., 2019). Plant-derived phytomolecules (capsaicin, resveratrol, fisetin, 6-gingerol, etc.) have been utilized in the treatment of cancer and have provided evidence for their intervention in different cancer progression pathways, as discussed below. Recently, the focus has shifted toward cancer prevention with phytochemicals obtained from natural sources. These phytochemicals can target effector molecules in cancer-developing pathways and are the major focus of this manuscript.
2 Signaling pathways and molecules as targets for the prevention of cancer
Signaling pathways are chains of chemical processes where several molecules in a cell interact to regulate a cell function, such as cell division or cell death. Targeted medicine in cancer treatment utilizes molecular modifications in cancer genes and signaling pathways to influence the development of new therapies. Different pathways of cancer progression, like JAK-STAT, Wnt-β-catenin, NF-κB, MAPK pathways, and different signaling molecules, have the tendency to crosstalk with other signaling pathways. These signaling pathways that serve as potential targets in cancer prevention have been discussed in the subsequent sub-sections.
2.1 JAK-STAT pathway
About 50% of breast cancer and leukemia and 90% of cervical cancer are caused by dysregulations in the Janus kinase (JAK)/signal transducer and activator of transcription (STAT) transduction pathway (JAK/STAT pathway) (Hu et al., 2013). This pathway serves as a crucial mediator in many cellular processes, including cell death, differentiation, and apoptosis (Shao et al., 2020). When cytokine-mediated glycoproteins like interferons, interleukins, and growth factors interact with cytokine receptors (CRs) on the surface of the target cell, it activates JAK molecules through transphosphorylation in endothelial and vascular smooth muscles (Loscocco and Vannucchi, 2022). Activated JAK initiates the phosphorylation of tyrosine residues located in the cytoplasmic region of CRs, which creates the binding positions for STATs (Hu et al., 2021). STATs are phosphorylated on tyrosine residues by tyrosine kinase, leading to the formation of STAT homo/hetero dimers in the cytosol. These dimers further translocate to the nucleus, bind with deoxyribose nucleic acid (DNA), and regulate the transcription of myc, p21, and Bcl-xl (Figure 3) (Bose et al., 2020). Dimers of unphosphorylated STATs can also localize to the nucleus and bind to heterochromatin protein 1. This further promotes heterochromatin formation, resulting in transcriptional upregulation. Typically, STATs are supposed to initiate the transcription of genes, but their unphosphorylated form can also downregulate the expression of several genes by interacting with HP1α and acting as a tumor suppressor (Brooks and Putoczki, 2020).
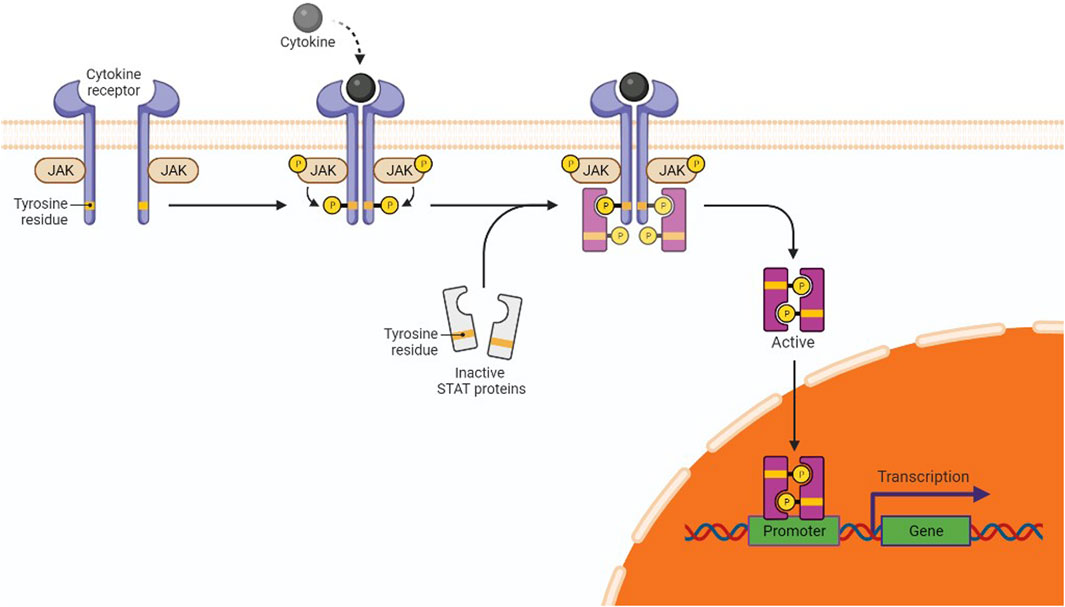
Figure 3. JAK/STAT in gene expression and cancer pathway. The interaction of cytokines or growth factors with their receptors (cytoplasmic tails of membrane cytokine receptors) induces dimerization/oligomerization of these receptors by inducing a conformational change in the cytoplasmic domain. JAKs or other families of tyrosine kinases are auto-phosphorylated or trans-phosphorylated as a result of this interaction. The phosphorylated JAKs form the sites for the binding of other signaling molecules with an SH2 domain (like STAT proteins) by phosphorylating the cytoplasmic tails of the receptor on tyrosine residues. Cytoplasmic STATs bind to phosphorylated receptors. STATs can assemble into homodimers or heterodimers that can translocate to the nucleus and activate gene transcription.
Escalated expression of negative regulators of the JAK/STAT pathway, viz., the suppressors of cytokine signaling (SOCS), can reduce pathway activation and tumorigenesis as well. Expression of SOCS/SOCS3 reduces the constitutive phosphorylation of STAT molecules at the tyrosine residues of CRs. Brantley et al. have reported the cessation of oncogenesis and transcription of genes due to SOCS expression in different tumor samples as it downregulates the secretion of cytokines; hence, the JAK-STAT pathway is not activated (He et al., 2003).
2.2 Wnt β-catenin pathway
Wnt-β signaling has been found to be active in over 50% of breast cancer and 25% of melanoma. Dysregulation of the Wnt β-catenin pathway has been reported in various types of cancer, including breast, lung, pancreatic, ovarian, colorectal, and gastric cancer (Zhan et al., 2017). Low-density lipoprotein receptor-related proteins (LRP) and frizzled receptors form a complex as a result of the interaction with a wingless-related integration site (Wnt) (Deitrick and Pruitt, 2016). The frizzled receptor recruits disheveled protein (DVI), which leads to the phosphorylation of the LRP receptors. It causes the inactivation of the destruction complex composed of Axin, adenomatosis polyposis coli (APC), casein kinase (CK), and glycogen synthase kinase (GSK). It disrupts the Axin-mediated degradation of β-catenin, as shown in Figure 4. The translocation and accumulation of β-catenin in the nucleus causes the replacement of Groucho in the nucleus and leads to the activation of the Wnt-responsive genes (Krishnamurthy and Kurzrock, 2018; Shen et al., 2022). Erythroblast transformation-specific (ETS)-related genes (ERGs) have also been reported to control the Wnt-signaling pathway by stabilizing β-catenin. In mouse models, ERG has been shown to regulate the secretion of vascular endothelial growth factor (VEGF) and vascular stability through Wnt signaling (Daisy Precilla et al., 2022). It is possible because ERG has been reported to have the potential for the transcription of frizzled class 4 receptors and simultaneously provide stability of β-catenin in endothelial cells (ECs). A reduced level of β-catenin has been associated with reduced tumor formation (Patel et al., 2019; Liu et al., 2021).
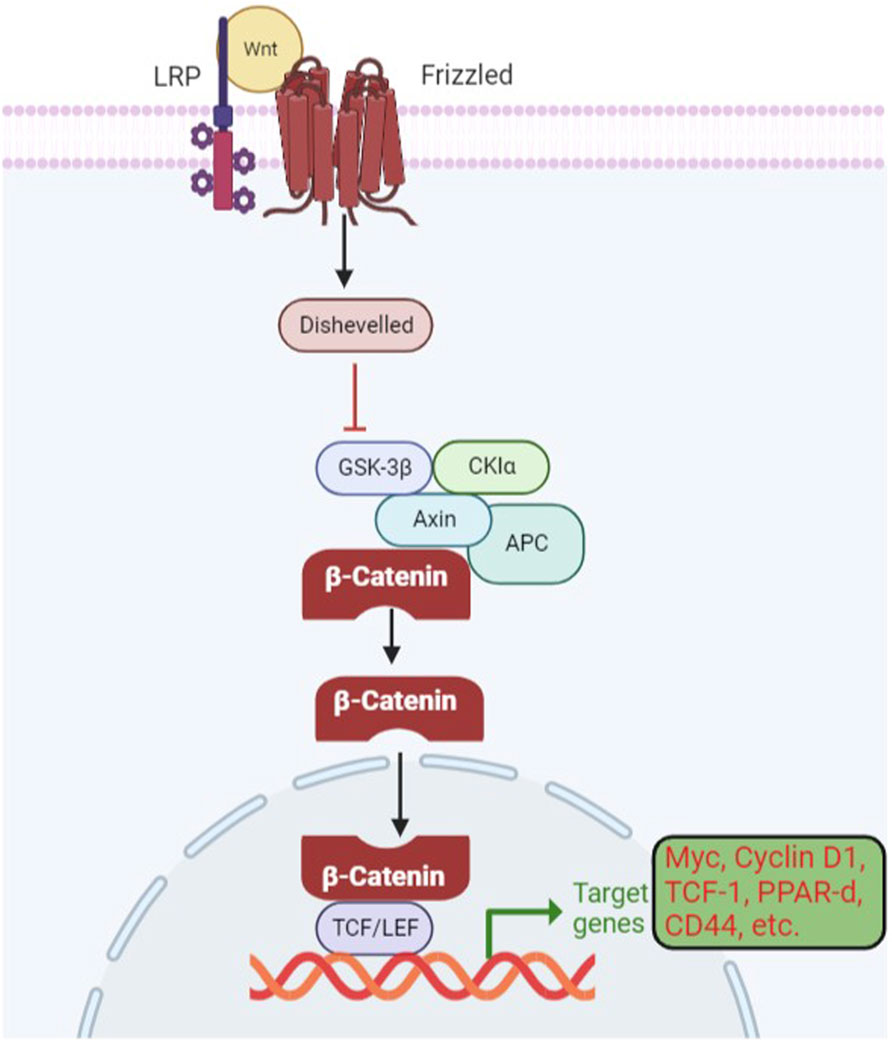
Figure 4. Wnt β-catenin signaling pathway in gene expression and cancer development. When Wnts are attached to their LRP5/6 and frizzled protein receptors, the cytoplasmic protein DVL is activated, which results in the inhibition of GSK3 in the destruction complex. The target gene is then transcriptionally regulated as a result of stabilized catenin binding to the TCF/LEF transcription factors in the nucleus.
2.3 p53 gene: association with cancer progression
The p53 protein plays a pivotal role in the transcription of several genes and also acts as a tumor suppressor. It is often referred to as the “guardian of the genome” because of its ability to detect DNA damage and promote either DNA repair or programmed cell death in damaged cells. Over 50% of human cancer involves the deactivation of p53 or mutations in p53 (Li, 2021). Whenever oxidative stress or genetic alterations initiate DNA damage, an automated correction mechanism gets activated via the protein sensor of DNA damage, i.e., ATM/CHK2. This, in turn, phosphorylates p53, which may check the damage through either of the transducer mechanisms, as shown in Figure 5 (Nakano and Vousden, 2001). p53 has also been reported to trigger PUMA and BAX molecules to accelerate the apoptotic pathways. p53 is involved in the inhibition of the cyclin-dependent kinases (CDK) complex, causing Rb dephosphorylation, resulting in cell cycle arrest (Akhter et al., 2014). Qiu L et al. reported that p53/p21 disbalance affects many biological processes, including cell proliferation by arresting the cell cycle at the G2/M phase and apoptosis. p53 has been reported to inhibit epithelial–mesenchymal transition (EMT) (Borsos et al., 2022) by reducing the levels of zinc finger E-box-binding homeobox 1 and 2 (ZEB1 and ZEB2) expressions (Banin et al., 1998). To sum up, p53 may either activate DNA repair enzymes (PARPs and polymerases), cause temporary cell cycle arrest at the G1 phase to remove the damaged portions (quiescence/senescence), or accelerate the secretion of PUMA/BAX to induce apoptosis in cancerous cells, as depicted in Figure 5. So, the activation of p53 through small molecules might be a key to preventing damaged cells’ conversion into cancerous cells (Yu et al., 2001).
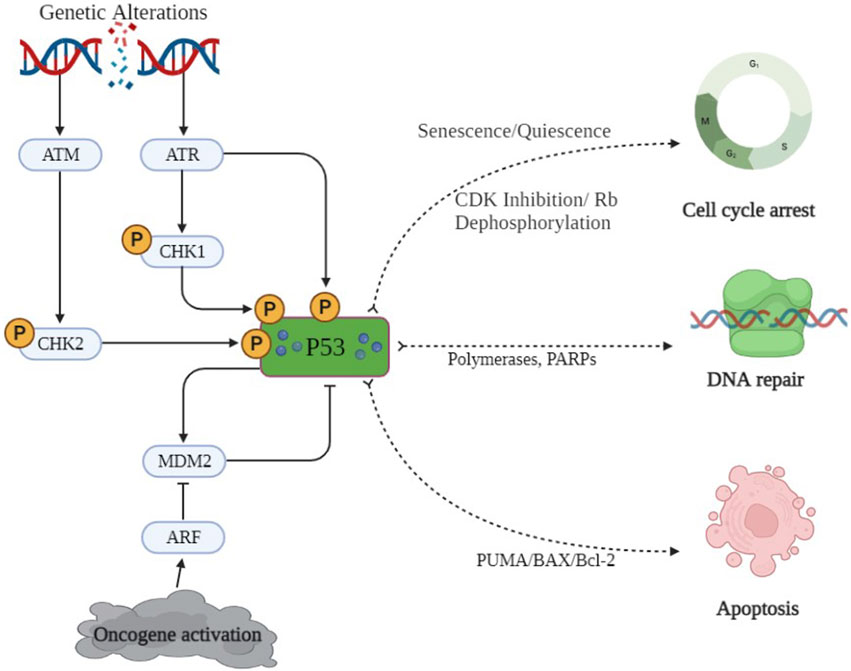
Figure 5. p53 signaling pathway. DNA damage and oncogene activation is only a few of the cellular stressors that activate the sensor proteins ATM and CHK2. Murine double-minute 2 (MDM2), which is a target of p53 and also controls p53 stability, creates a negative feedback loop. Activated p53 is involved in numerous pathways, including apoptosis pathways, cell proliferation, and DNA repair and cell cycle arrest.
2.4 Cyclin D and CDK complex
Cyclin D is a member of the cyclin protein family and is involved in the regulation of the cell cycle and its functions. The cyclin D/cyclin dependent kinase-4 (CDK-4) complex is a multi-protein structure made up of cyclin D and CDK4, a serine–threonine kinase (Bury et al., 2021). The synthesis of cyclin D starts with the action of growth factors. Cyclin D1 protein converts the inactivated form of CDK4/6 to its activated form and mediates the G1/S phase transition (Zhang et al., 2021; Liu et al., 2022). This further triggers the phosphorylation of the Rb protein and leads to uncontrolled cell proliferation. Overexpression of cyclin D1 has been observed in a wide range of cancers, including breast cancer, lung cancer, and prostate cancer (Li et al., 2022). It is associated with poor prognosis and resistance to cancer treatment. Cancer preventive agents have been reported to inhibit the cyclin D1 protein and its complex, which further decreases cell proliferation by dysregulating cyc-D expression and results in cell cycle arrest. CDK/cyc-D inhibitors have been developed to control cancer progression and are currently under clinical trials, like abemaciclib and ribociclib (Heptinstall et al., 2018).
2.5 TNF-α/NF-κB signaling pathway
Tumor necrosis factor-α (TNF-α) is a cytokine that plays a complex role (pro-inflammatory and anti-tumor) in cancer patients. In the early stages of cancer development, TNF-α can stimulate the immune system, attack cancer cells, and lead to apoptosis of tumor cells. However, in the later stages of cancer development, chronic inflammation can occur, which can promote the growth and spread of cancer cells. TNF-α can also stimulate the production of angiogenic factors, which can act as fuel for the growth of tumors. At the molecular level, when TNF-α binds to its receptor present at the cell surface of a normal healthy cell, it gets inactivated due to the presence of the silencer of the death domain (SODD) (Alharbi et al., 2021). The binding of TNF-α with SODD restores the activities of the tumor necrosis factor receptor (TNFR), and SODD is liberated/released. This complex is attacked by TNF-R1-associated death domain protein (TRADD) and turns into the TNFR-TRADD complex (Aggarwal et al., 2006). Further transduction depends on the type of protein molecule that gets attached to the complex. If FAS-associated death domain (FADD) molecules bind with this complex, it causes the release of pro-caspase 8, which further causes apoptosis of cancerous cells using caspase-cascade. On the other hand, if TNF receptor-associated factor (TRAF-2) interacts with the TNFR-TRADD complex, it causes the activation of the inhibitor of apoptotic proteins (IAPs) and, hence, nuclear factor- κB (NF-κB) is activated (Figure 6) (Liu W. et al., 2020). NF-κB further promotes cell survival by increasing the expression of the cellular inhibitor of apoptosis protein-1 (cIAPs) and inducible nitric oxide synthase (iNOS). Whenever there is a reduction in the activity of cytoplasmic IAPs and receptor-interacting protein (RIP1) kinase, receptor internalization occurs. This further results in the activation of caspase 8 and, then, causes the apoptosis of the particular cell (Aggarwal et al., 2002). Whenever there is an increase in cytoplasmic IAPs, it retards the activity of the electron transport chain in the mitochondria. This further activates reactive oxygen species (ROS), then RIP-1 is recruited, and the process of NF-κB activation starts again (Storci et al., 2010). In cancerous cells, after the formation of the TRADD-SODD complex, it has a higher affinity for TRAF-2 than the FADD molecule. This causes increased NF-κB production inside cancerous cells and leads to cell survival by preventing apoptosis. The manipulation of TNF-α and its downstream pathways may offer newer therapeutic opportunities for cancer prevention.
2.6 Regulation of apoptotic molecules
Programmed cell death or apoptosis of any cell relies on three steps, i.e., initiation, execution, and phagocytosis. It is an essential biological process that plays a critical role in various physiological conditions, including cancer. In the intrinsic pathway of apoptosis, DNA lesions release ataxia-telangiectasia mutated (ATM) serine and threonine kinase (Plati et al., 2011). These activate p53, which further upregulates the p53-upregulated modulator of apoptosis (PUMA) (Hassan et al., 2014; Goldar et al., 2015). B-cell lymphoma-2 (Bcl-2)-associated X protein (BAX) is activated in the cytosol of the cell by the upregulation of PUMA, and it opens the voltage-dependent anion-selective channel (VDAC) on the surface of the mitochondria. It results in the release of cytochrome C out of the cytosol (Duan et al., 2023). It binds with pro-apoptotic apoptosis protease-activating factor 1 (APAF-1), and the apoptosome is activated (Verbrugge et al., 2010; International, 2020), which further activates caspase 9 and caspase 3 (Figure 7). Furthermore, the activation of nuclease and protease causes DNA fragmentation, which leads to cell death. In the extrinsic pathway, the signaling is attributed to TNF-α and FS-7-associated surface antigen (FAS) molecules. These molecules activate TNF or FAS receptors. The death-inducing signaling complex (DISC) complex is formed (Ouyang et al., 2012). The interaction of FAS with the FAS receptor causes the activation of FADD, which further releases pro-caspase 8 and caspase 3. This caspase 3 activates caspase-dependent DNAase and proteolytic enzymes, which in turn degrade DNA and proteins, culminating in cell death (Clarke and Tyler, 2009). B-cell lymphoma-2 (Bcl-2) is the negative regulator of this pathway, which has antagonistic actions for BAX. In the extrinsic pathway, the conversion of the BH3 interacting domain (Bid) to truncated (t-Bid) due to the activation of the caspase-cascade complex releases BAX in the cytosol, which might then start the further process of the intrinsic pathway (Xu Y. et al., 2020; Yang et al., 2020). In cancerous cells, due to the dysregulation of p53, PUMA has not been activated. This further leads to decreased BAX production; hence, as a result of this, the caspase-cascade system does not get activated, and ultimately, the cells survive.
2.7 Association of VEGF with metastasis
Angiogenesis is the process by which new blood vessels are formed from pre-existing vessels. Tumor microenvironment features include cell recruitment, release of soluble factors, and hypoxia, all of which contribute to angiogenesis. Angiogenesis is also characterized by resistance to anti-angiogenic therapy. The development of cancerous cells requires a high amount of oxygen and nutrients and releases HIF1α in comparison to healthy cells. Furthermore, it releases the VEGF. The interaction of the VEGF with its receptor (VEGF-R) secretes proteases that make the blood vessels leaky and lead to the generation of new blood vessels toward growing cancerous cells. When the platelets of normal or cancerous cells are drawn to hypoxic areas, they become activated and release the stimulatory substances they have stored there into the tumor microenvironment. The bone marrow’s endothelial progenitor cells (EPCs) and myeloid cells relocate to the tumor microenvironment, where they locally release more soluble factors (Nishida et al., 2006; Lv et al., 2023). The tumor cells become more invasive in this environment and are able to metastasize to distant organs by intravasation into the lymphatics or vasculature (Figure 8) (Rajabi and Mousa, 2017). Valid regimens for cancer prevention must consider the targets of multiple cell types that are targetable for angiogenic factors, such as platelet-derived growth factor (PDGF), PDGF receptor (PDGFR), angiopoietin 1/2 (Ang1/2), ECs, and EPCs (Rajabi and Mousa, 2017).
3 Role of plant-derived secondary metabolites in cancer prevention
Phytochemicals, including paclitaxel, docetaxel, and resveratrol, have shown anticancer activity and completed clinical trials (Choudhari et al., 2020). Phyto-analogs have shown desirable effects on p53, BAX, VEGF, β-catenin, BAK, Bcl-2, CDKs, and interleukins. Some secondary metabolites work either by preventing the formation of cancer cells or by inducing the death of existing cancer cells. For example, flavonoids, a class of secondary metabolites found in many fruits and vegetables, have been shown to inhibit the growth and proliferation of cancer cells in vitro and in vivo. Polyphenols, another class of secondary metabolites found in many plant-based foods, have been shown to have similar anticancer properties (Hosseini and Ghorbani, 2015). Overall, secondary metabolites play an important role in cancer prevention by providing a natural source of anticancer compounds that can be incorporated into a healthy diet. Although more research is needed to fully understand the mechanisms underlying their anticancer properties, consuming a variety of fruits and vegetables rich in secondary metabolites is likely to have important health benefits, including reducing the risk of cancer. Here, in this section, we have divided selected herbal plants into their classes as per their metabolic nature, and the activity of these drugs has been summarized in Table 1.
3.1 Role of flavonoids
Plant species contain a group of secondary polyphenolic compounds known as flavonoids, which are generally included in human diets. Chemically, flavonoids have a 15-carbon skeleton that is made up of two phenyl rings and a heterocyclic ring. The abbreviation for this carbon structure is C6–C3–C6. Flavonoids are known for diverse pharmacological activities (Nagoor Meeran et al., 2021; Singh et al., 2023).
Apigenin is classified as a flavone among the different classes of flavonoids. It is abundantly present in common fruits such as grapefruit, plant-derived beverages, and vegetables such as parsley, onions, oranges, tea, chamomile, wheat, sprouts, and some seasonings (Li Y. et al., 2020). The chemo-preventive role of this flavonoid (apigenin) has been tested on wild-type and specially modified mice (female Swiss albino mice) (Ganjare et al., 2011; Hang et al., 2022). It has shown a reduction in angiogenesis by reducing the VEGF level in breast cancer (Ai et al., 2017; Meng et al., 2017). The effect of apigenin was recorded in UVB-induced cancers in wild-type (WT) and modified (TKO) mice. Apigenin has shown significant cancer prevention and recovery by reducing tumor volume and VEGF compared to the negative control. Apigenin has also shown a reduction in blood capillary formation in the apigenin-treated mice group compared to that in other groups (Ai et al., 2017; Meng et al., 2017).
One such secondary metabolite, or flavonoid, is curcumin. Curcumin is a non-toxic diarylheptanoid belonging to the group of curcuminoids. It is obtained from the dried rhizome of Curcuma longa Linn. belonging to the family Zingiberaceae (Oliveira et al., 2021). Curcumin has been reported for its cancer-preventive effects on different breast cancer cell lines (MCF-7 and MDA-MB-231 cell lines). It has been reported to boost autophagy in cancerous cells. It has shown stimulatory effects on apoptotic enzymes (BAK/BAX). It has also been reported to boost p53 levels in MCF-7 cell lines (Wang et al., 2016). Curcumin has also shown synergistic actions with berberine and potentially alters the levels of STATs, Β-catenin, Bcl2, BAX, p53, NF-κB, and CDKs. In WIPO PCT, a patent has been filed for the cancer-preventive effects of curcumin and turmeric oil with the application number WO2014068597A2, in which the drug has decreased the levels of NF-κB and reduced the proliferation of cancerous cells.
Another flavonoid involved in cancer-preventing activities is silymarin. Silymarin is a standardized milk thistle seed extract containing a mixture of flavonolignans (Silybum marianum) belonging to the group of flavonoids (Abouzid et al., 2016). Silymarin has been reported to inhibit organic anion transporters (OAT) and ATP-binding cassettes (ABC) transporters, showing cancer-preventive effects. It has also been reported to boost the activity of apoptotic molecules and BAX, which activates the caspase system of apoptosis in 6 -week-old SINCAR mice with squamous cell carcinoma (Malekpour-Dehkordi et al., 2022). It has also reduced tumor volume and prevented tumorigenicity by reducing TNF-a levels (Wang et al., 2023).
Ginestin is a phytoestrogen belonging to the flavonoid group. It is found in soybeans and soybean-enriched products (Cools et al., 2014). At the molecular level, ginestin is active against CDKs, cell proliferation, angiogenesis, invasion, metastasis, and apoptotic processes. Ginestin has shown effects on Akt, NF-κB, matrix metalloproteinases (MMPs), and BAX/Bcl-2 signaling pathways for breast cancer prevention in C57Bl/6 mice (Sarkar and Li, 2002). It has also been reported to show synergistic effects with resveratrol via boosting the expression of p53 and diminishing the NF-kB pathway of cancer development (Spagnuolo et al., 2015).
One more flavonoid reported to have cancer-preventive potential is fisetin. Fisetin is a flavanol from the flavonoid group of polyphenols. It is obtained from fruits and vegetables such as strawberries, apples, persimmon, grapes, onions, and cucumber (Gleńsk et al., 2021). Fisetin is also a good antioxidant and is involved in the inhibition of the Wnt signaling pathway. It has been reported to inhibit YB-1 protein and proliferation in nasopharyngeal carcinoma. This study was performed on CNE11 and CNE1-LMP1 cell lines of nasopharyngeal carcinoma (Ravula et al., 2021).
One more glycosyloxyflavone flavonoid with cancer-preventing potential is baicalin. Scutellaria baicalensis (SB) extract contains baicalin, which is a natural antioxidant, belonging to the family Lamiaceae (Li et al., 2004). Baicalin has been reported to prevent tumor formation by increasing the levels of p53 and BAX against breast cancer induced in Swiss albino mice (Zhao et al., 2016; Huang et al., 2022). Baicalin has also been reported to reduce the levels of ILs, Bcl2, and VEGF, showing cancer-preventive effects (Shehatta et al., 2022). Baicalin has shown its synergistic effects along with 5-fluorouracil (5-FU).
3.2 Role of terpenoids
Isoprene is utilized to make a group of chemical compounds known as terpenoids, commonly known as isoprenoids. Terpenes and the 55-carbon molecule isoprene are two examples of organic compounds that are found in nature. These are multi-cyclic structures with oxygen-containing functional groups. Examples include lycopene, dihydrotanshinone, and carotene. Lycopene, a naturally occurring carotenoid, is extracted from tomatoes, belonging to the Solanaceae family (Adetunji et al., 2021). Lycopene has shown a chemo-preventive effect by inhibiting the NF-κB pathway and Bcl2, which further stops cancer proliferation in HCT116 and SW480 cell lines of colorectal carcinoma (Adetunji et al., 2021). It has also reduced lung and liver inflammation by activating SIRTUINS and has been shown to alter the levels of oncogenes like NP73 and upregulate p53 to prevent proliferation, as evaluated against colorectal cancer cell lines (Lim and Wang, 2020). Lycopene has also shown synergistic effects in combination with β-cryptoxanthin. According to the IPO, no patent has been filed for its chemo-preventive efficacy; however, its efficacy has been reported for protecting the skin from UV radiation.
Dihydrotanshinone is another example in this class. Dihydrotanshinone belongs to a class of lipophilic diterpenoids and is extracted from Salvia miltiorrhiza Bunge (Wang et al., 2019). Dihydrotanshinone has shown cancer-preventive effects against liver cancer in different cell lines (HepG2 and HT-29 cell lines). It potentially reduces the levels of VEGF and Bcl2 while boosting the activity of caspases. These effects are involved in inhibiting angiogenesis and stimulating apoptosis by interfering with the intrinsic and extrinsic pathways of cell death (Su Y. S. et al., 2021).
β-carotene is a type of carotenoid obtained from different pigmented sources, like carrots. Carrot is a root vegetable called Daucus carota in scientific terms and belongs to the family Apiaceae (Metibemu et al., 2021). β-carotene has increased the number of apoptotic cells with an increase in the activity of caspases in colon cancer cells. It has also been reported to reduce the levels of NF-κB, Bcl2, and SOD proteins against colon cancer cell lines (HCT116 and SW480 cell lines) (Jain et al., 2018).
Artemisinin, derived from the shrubs of Artemisia annua, exhibits promising anticancer properties, particularly in breast cancer. At a dose of 300 mg/kg, it has demonstrated the remarkable ability to induce membranous translocation of beta-catenin while inhibiting the uncontrolled activation of the Wnt/b-catenin pathway, which is a critical signaling cascade implicated in cancer progression (Li et al., 2007). This dual mechanism effectively prevents cancer development and progression. The efficacy of artemisinin was evaluated in a preclinical study using Balb/c female nude mice as a model for breast cancer (Augustin et al., 2020). The results underscore its potential as a therapeutic agent in combating breast cancer, offering a glimpse into its mechanism of action and providing valuable insights for further clinical exploration (Ma et al., 2021).
Eugenol, a naturally occurring phenylpropanoid, is found in a variety of plants, including clove buds, tulsi leaves, turmeric, pepper, and ginger (Wang et al., 2020) Eugenol has been reported to reduce the activities of caspases and Bcl2, as shown on the MCF-7 cell line of breast cancer (do Nascimento Silva et al., 2016). Eugenol has reduced the tumor volume and proliferation of cancerous cells, both in combination with gemcitabine and alone (Li Z. et al., 2020). A US patent has also been published for the cancer-preventing profile of eugenol with the application number US20020103174A1. It has reduced tumor growth and size by more than 36% in the LNCaP and DU145 cell lines of human prostate cancer. Furthermore, in vivo activity has been performed using LNCaP human prostate tumor xenografts and concluded with a decreased tumor size and weight with increased apoptotic cells (Hussain et al., 2011).
Fennel seeds are obtained from the plant Foeniculum vulgare belonging to the family Umbelliferae. Since ancient times, the dried seeds have been used in food and medicine. Anethole has reduced the activity of NF-κB and STATs in female Sprague Dawley rats, leading to a decrease in tumor growth and proliferation in a breast cancer model (Lubet et al., 1997). Anethole has affected the TNF-α and JAK-STAT pathways of cancer development and showed cancer-preventive activity. Anethole has also been reported for its synergistic effects with curcumin (Contant et al., 2021).
3.3 Role of alkaloids
Alkaloids are any of a class of naturally occurring organic nitrogen-containing bases. Alkaloids have diverse and important physiological effects on humans and other animals. Well-known alkaloids include capsaicin, strychnine, sanguinarine, ephedrine, and nicotine. Alkaloids are significant chemical substances that provide a wealth of potential therapeutic targets and cancer-preventive potential as well. An important alkaloid, capsaicin is also known as 8-methyl-N-vanillyl-6-non amid and is obtained from Capsicum annuum (chili peppers) and Capsicum frutescens (includes bird’s eye chili) belonging to the family Solanaceae (Hayman and Kam, 2008). Capsaicin and its derivatives have been reported to overexpress SOCS to decrease STAT actions in gastric cancer (AGS cells) (Abdelrahman Mohamed and Abdullah AlQarni, 2019). It has also been reported to reduce NF-κB, resulting in cancer proliferation (Lubet et al., 1997). Another important alkaloid included in cancer prevention is sanguinarine, which is a polycyclic quaternary alkaloid extracted from bloodroot or Sanguinaria canadensis (Akhtar et al., 2019). In cancer prevention, sanguinarine has been found to reduce proliferation by reducing NF-κB levels (Zhang et al., 2019; Khan et al., 2021). It has also increased the level of BAX/BAK, which is responsible for the increase in apoptosis and necrosis in A431 and NHEK cells of epidermoid carcinoma (Achkar et al., 2017; Almeida et al., 2017; Kuttikrishnan et al., 2019). It has also shown synergistic effects with curcumin and quercetin (Almeida et al., 2017).
3.4 Role of phenolics
The wide presence of phenolic compounds in plants and herbal species (such as fruits, vegetables, cereals, olives, legumes, and cocoa) and beverages (such as tea, coffee, beer, and wine) contributes to the overall organoleptic characteristics of plant foods. Phenolics can boost the body’s immune system’s ability to identify and eradicate cancer cells and block angiogenesis, the formation of new blood vessels required for tumor development. EGCG, gingerol, fisetin, resveratrol, etc., are few examples in this class. Resveratrol is a type of polyphenolic compound isolated from grapes and their extract. It consists of the ripe fruits of Vitis vinifera belonging to the group of grapes (Huminiecki and Horbańczuk, 2018). Resveratrol decreases proliferation by targeting the NF-κB pathway (Mizerska-Kowalska et al., 2022). It has potentially activated apoptosis (reduced Bcl2 and Stat3) and activated the overexpression of SOCS, hence targeting the JAK-STAT pathway in the SCCHN cells of squamous cell carcinoma (Baek et al., 2016). It has also shown synergistic effects with 5-fluorouracil (a cytotoxic drug). An Indian patent office search clarifies that a patent has not been applied for the cancer-preventive action of the same drug, although the extract of Vitis vinifera has been granted a patent for a similar activity.
Another phenolic compound with cancer-prevention activity is EGCG. EGCG, an ester of epigallocatechin and gallic acid, is obtained from the plant Camellia sinensis belonging to the family Theaceae (Sojoodi et al., 2020). In terms of cancer-preventive activities, EGCG has been reported to reduce NF-ΚB overexpression and STAT actions (Rady et al., 2018; Ferrari et al., 2022). It has been reported to decrease VEGF, BAX, NF-κB, mitogen-activated extracellular signal-regulated kinase (MEK), and CDKs in HSC-3 cell lines of oral carcinoma (Aggarwal et al., 2022). EGCG has also shown synergistic effects with curcumin and resveratrol (Rady et al., 2018; Ferrari et al., 2022).
Gingerol is a polyphenolic compound with diverse activities. Gingerol has been extracted from Zingiber officinale belonging to the family Zingiberaceae. Gingerol has been reported to increase the expression of APC, p53, and TUNEL-positive nuclei and, subsequently, decrease the expression of TNF-α, IL-1β, inducible nitric oxide synthase (iNOS), COX-2, and cyclin D1 in male BALB/c mice with colorectal cancer (Farombi et al., 2020). There is an increase in the activity of gingerol when administered with corn oil with synergism. Gingerol has been reported to reduce tumor growth to a significant extent by targeting VEGF-A and WNT-3A. Gingerol has been shown to reduce p53 and NF-κB expression.
Another phenolic compound in this class is CAPE. Caffeic acid phenethyl ester (CAPE) is a natural phenolic chemical compound and an ester of caffeic acid and phenethyl alcohol (Kabała-Dzik et al., 2018). CAPE has also shown a cancer-preventive role by targeting the NF-κB activation pathway and STAT expressions in male Wistar rats with liver cancer (Carrasco-Legleu et al., 2004). It has been reported to reduce gene expression, resulting in the reduced proliferation of liver cancer cells.
Chemical compounds known as organometallic compounds (OMCs) have at least one bond between a metallic element and an organic molecule’s carbon atom. They are promising therapeutic candidates for the treatment of cancer because of their structural variety, ligand exchange, redox, and catalytic characteristics. OMCs include, for example, allicin and broccoli. Allicin is a diallylthiosulfinate or a type of organosulfur compound obtained from bulbs of Allium sativum (garlic) belonging to the family Amaryllidaceae (Chen et al., 2018). These organosulfur compounds have been found to be active against VEGF and proliferation by reducing the NF-κB activity in A/J mice for treating colorectal cancer (Saraswati and Agrawal, 2013). Garlic has also been reported to reduce the levels of iNOS expression in colorectal cancerous cells (Mansingh et al., 2018).
Broccoli comes under the group of cabbages belonging to the family Brassicaceae. Broccoli has been used to control cholesterol and fibromyalgia and in the treatment of several types of cancers (Tokuşoğlu and Parvizi, 2018). Metallic components of broccoli are active against cell proliferation, decrease NF-κB, and reduce the tumor size in SUM149 and SUM159 cell lines of breast cancer. It has been reported to inhibit CDKs in breast cancer cell lines (Burnett et al., 2017). It has also shown synergistic effects with curcumin, caffeic acid, and sorafenib. A patent office search clarifies that a patent has been filed for broccoli and its constituents for the treatment of cancer, but there is no filed patent for its cancer-preventive action.
3.5 Others
Vitamin D is a type of fat-soluble steroid that is essential for the body for its various pharmacological applications. The kinetic form of vitamin D is calcitriol (Rinninella et al., 2022). This sub-type of vitamin D has been reported to activate the expression of cleaved caspase-3, Bax, and p-AMPK and inhibit the expressions of p-Bcl-2, c-Myc, p-IGF-IR, p-mTOR, p-P70S6K, and p-S6, which further leads to apoptosis of the cancerous cells in Hs695T for amelanotic melanoma (Aslam et al., 2021). It has also shown synergistic effects with vitamin A and metformin. A patent office search clarifies that a patent has been filed for the chemo-preventive potential of vitamin D3 in WIPO PCT with the application number WO2006039281A2. It has been reported to induce cell cycle arrest at the G1 phase, causing decreased cell proliferation.
Syzygium samarangense (SS) is a type of flowering plant belonging to the family Myrtaceae. This extract of Syzygium samarangense has been reported to activate apoptosis and arrest the cell cycle in HEP G2-C8 cell lines of liver cancer (Su Z. Y. et al., 2021). This drug has elevated the levels of Bcl-2 and p53 for inducing apoptotic activity (Scuto et al., 2021). p53 also causes the secretion of PUMA and increases the rate of the intrinsic pathway of apoptosis.
3.5.1 Toxicological impact of the chemo-preventive agents
Herbal cancer-preventive agents require their administration for a longer duration in minimum doses. So, it is important to consider their toxicological impact on the human body because these drugs interact with the signaling pathways of cells at the molecular level. Some herbal cancer-preventive agents may have toxicological effects on the body, particularly if taken in high doses or for prolonged periods of time. For example, some herbal agents, such as comfrey, may contain pyrrolizidine alkaloids that can cause liver damage and even cancer with long-term use. Their short mechanism of action in chemoprevention and reported toxicities are summarized in Table 2.
4 Conclusion
Cancer-prevention pathways involve a complex interplay of genetic, environmental, and lifestyle factors that can impact the development and progression of cancer. Although there is no sure-fire way to prevent cancer, there are many strategies that can be adopted to reduce the risk of developing the disease. Some of the most effective cancer-prevention strategies include maintaining a healthy diet and weight, getting regular exercise, avoiding tobacco and excessive alcohol consumption, and protecting oneself from harmful environmental exposures. The majority of the cancer-preventive phyto-analogs are included in a human’s daily diet in crude form, but there is a challenge in ensuring the minimum amount of required API to be taken for cancer-preventive actions. Additionally, regular cancer screening can help detect the disease at an early stage, when it is more treatable. Research has also identified several biological pathways and mechanisms that can impact cancer development and progression. These include oxidative stress, inflammation, DNA damage and repair, immune system function, and signaling pathways. Targeting these pathways with dietary products provides extraordinary evidence for preventing and targeting cancer in the future, as depicted in Figure 9. Numerous novel targets have been identified, including SOCS, STAT3, Nrf2, NF-B, cell cycle regulators cyclin D1, D2, and D3, INPs, and Wnt, which are expressed improperly in pre-cancer lesions. Worldwide, the scientific fraternity is involved in researching such newer drug candidates consistently for targeting these proteins at the molecular level to diminish the cancer progression and development further.
Author contributions
VS: writing–original draft, conceptualization, and writing–review and editing. AC: conceptualization and writing–review and editing. SB: writing–review and editing and formal analysis. SG: writing–review and editing. RM: writing–review and editing and writing–original draft. SK: writing–review and editing and formal analysis. MA: writing–review and editing and formal analysis. MS: writing–review and editing and formal analysis. SS: formal analysis and writing–review and editing. DV: formal analysis and writing–review and editing. AG: writing–review and editing. SjK: supervision, validation, and writing–original draft. SdK: data curation, supervision, and writing–original draft.
Funding
The author(s) declare that financial support was received for the research, authorship, and/or publication of this article. This work was supported and funded by the Deanship of Scientific Research at Imam Mohammad Ibn Saud Islamic University (IMSIU) (grant number IMSIU-RG23059).
Acknowledgments
Authors want to thanks Department of Sciences and Technology (Government of India) for providing us the DST FIST sponsored lab facilities (TPN 69942).
Conflict of interest
The authors declare that the research was conducted in the absence of any commercial or financial relationships that could be construed as a potential conflict of interest.
Publisher’s note
All claims expressed in this article are solely those of the authors and do not necessarily represent those of their affiliated organizations, or those of the publisher, the editors, and the reviewers. Any product that may be evaluated in this article, or claim that may be made by its manufacturer, is not guaranteed or endorsed by the publisher.
References
Abdelrahman Mohamed, M., and Abdullah AlQarni, E. (2019). Chemopreventive effect of capsaicin in experimentally induced hamster buccal pouch carcinogenesis (Immunohistochemical study Bcl-2). Egypt Dent. J. 65, 1237–1243. doi:10.21608/EDJ.2019.72201
Abouzid, S. F., Chen, S. N., McAlpine, J. B., Friesen, J. B., and Pauli, G. F. (2016). Silybum marianum pericarp yields enhanced silymarin products. Fitoterapia 112, 136–143. doi:10.1016/j.fitote.2016.05.012
Achkar, I. W., Mraiche, F., Mohammad, R. M., and Uddin, S. (2017). Anticancer potential of sanguinarine for various human malignancies. Future Med. Chem. 9, 933–950. doi:10.4155/FMC-2017-0041
Adetunji, C. O., Akram, M., Mtewa, A. G., Jeevanandam, J., Egbuna, C., Ogodo, A. C., et al. (2021). “Biochemical and pharmacotherapeutic potentials of lycopene in drug discovery,” in Preparation of phytopharmaceuticals for the management of disorders: the development of nutraceuticals and traditional medicine (Elsevier), 307–360. doi:10.1016/B978-0-12-820284-5.00015-0
Aggarwal, B. B., Shishodia, S., Ashikawa, K., and Bharti, A. C. (2002). The role of TNF and its family members in inflammation and cancer: lessons from gene deletion. Curr. drug. targets. Inflamm. allergy 1, 327–341. doi:10.2174/1568010023344571
Aggarwal, B. B., Shishodia, S., Takada, Y., Jackson-Bernitsas, D., Ahn, K. S., Sethi, G., et al. (2006). TNF blockade: an inflammatory issue. Res. Found. Workshop. 56, 161–186. doi:10.1007/3-540-37673-9_10
Aggarwal, V., Tuli, H. S., Tania, M., Srivastava, S., Ritzer, E. E., Pandey, A., et al. (2022). Molecular mechanisms of action of epigallocatechin gallate in cancer: recent trends and advancement. Semin. Cancer Biol. 80, 256–275. doi:10.1016/J.SEMCANCER.2020.05.011
Ahmed, S., Ullah, N., Parveen, S., Javed, I., Asyilla, N., Jalil, C., et al. (2022). Effect of silymarin as an adjunct therapy in combination with sofosbuvir and ribavirin in hepatitis C patients: a miniature clinical trial. Hindawi Oxidative Med. Cell. Longev. 2022, 9199190. doi:10.1155/2022/9199190
Ai, X. Y., Qin, Y., Liu, H. J., Cui, Z. H., Li, M., Yang, J. H., et al. (2017). Apigenin inhibits colonic inflammation and tumorigenesis by suppressing STAT3-NF-κB signaling. Oncotarget 8, 100216–100226. doi:10.18632/ONCOTARGET.22145
Akhtar, S., Achkar, I. W., Siveen, K. S., Kuttikrishnan, S., Prabhu, K. S., Khan, A. Q., et al. (2019). Sanguinarine induces apoptosis pathway in multiple myeloma cell lines via inhibition of the JAK2/STAT3 signaling. Front. Oncol. 9, 285. doi:10.3389/fonc.2019.00285
Akhter, R., Sanphui, P., and Biswas, S. C. (2014). The essential role of p53-up-regulated modulator of apoptosis (Puma) and its regulation by FoxO3a transcription factor in β-amyloid-induced neuron death. J. Biol. Chem. 289, 10812–10822. doi:10.1074/jbc.m113.519355
Alharbi, K. S., Fuloria, N. K., Fuloria, S., Rahman, S. B., Al-Malki, W. H., Javed Shaikh, M. A., et al. (2021). Nuclear factor-kappa B and its role in inflammatory lung disease. Chem. Biol. Interact. 345, 109568. doi:10.1016/J.CBI.2021.109568
Almeida, I. V., Fernandes, L. M., Biazi, B. I., and Vicentini, V. E. P. (2017). Evaluation of the anticancer activities of the plant alkaloids sanguinarine and chelerythrine in human breast adenocarcinoma cells. Anticancer Agents Med. Chem. 17, 1586–1592. doi:10.2174/1871520617666170213115132
Arruebo, M., Vilaboa, N., Sáez-Gutierrez, B., Lambea, J., Tres, A., Valladares, M., et al. (2011). Assessment of the evolution of cancer treatment therapies. Cancers 3, 3279–3330. doi:10.3390/CANCERS3033279
Aslam, A., Ahmad, J., Baghdadi, M. A., Idris, S., Almaimani, R., Alsaegh, A., et al. (2021). Chemopreventive effects of vitamin D3 and its analogue, paricalcitol, in combination with 5-fluorouracil against colorectal cancer: the role of calcium signalling molecules. Biochimica Biophysica Acta (BBA) - Mol. Basis Dis. 1867, 166040. doi:10.1016/J.BBADIS.2020.166040
Augustin, Y., Staines, H. M., and Krishna, S. (2020). Artemisinins as a novel anti-cancer therapy: targeting a global cancer pandemic through drug repurposing. Pharmacol. Ther. 216, 107706. doi:10.1016/j.pharmthera.2020.107706
Baek, S. H., Ko, J. H., Lee, H., Jung, J., Kong, M., Lee, J. W., et al. (2016). Resveratrol inhibits STAT3 signaling pathway through the induction of SOCS-1: role in apoptosis induction and radiosensitization in head and neck tumor cells. Phytomedicine 23, 566–577. doi:10.1016/J.PHYMED.2016.02.011
Bakhtiari-Dovvombaygi, H., Izadi, S., Zare, M., Asgari Hassanlouei, E., Dinpanah, H., Mohammad Ahmadi-Soleimani, S., et al. (2021). Vitamin D3 administration prevents memory deficit and alteration of biochemical parameters induced by unpredictable chronic mild stress in rats. Sci. Rep. 11, 16271. doi:10.1038/s41598-021-95850-6
Banin, S., Moyal, L., Shieh, S. Y., Taya, Y., Anderson, C. W., Chessa, L., et al. (1998). Enhanced phosphorylation of p53 by ATM in response to DNA damage. Science 281, 1674–1677. doi:10.1126/science.281.5383.1674
Borsos, B. N., Pantazi, V., Páhi, Z. G., Majoros, H., Ujfaludi, Z., Berzsenyi, I., et al. (2022). The role of p53 in the DNA damage-related ubiquitylation of S2P RNAPII. PLoS One 17, e0267615. doi:10.1371/JOURNAL.PONE.0267615
Bose, S., Banerjee, S., Mondal, A., Chakraborty, U., Pumarol, J., Croley, C. R., et al. (2020). Targeting the JAK/STAT signaling pathway using phytocompounds for cancer prevention and therapy. Cells 9, 1451. doi:10.3390/CELLS9061451
Bozic, D., Baralić, K., Živančević, K., Miljaković, E. A., Ćurčić, M., Antonijević, B., et al. (2022). Predicting sulforaphane-induced adverse effects in colon cancer patients via in silico investigation. Biomed. Pharmacother. 146, 112598. doi:10.1016/J.BIOPHA.2021.112598
Brooks, A. J., and Putoczki, T. (2020). JAK-STAT signalling pathway in cancer. Cancers 12, 1971–2012. doi:10.3390/CANCERS12071971
Burnett, J. P., Lim, G., Li, Y., Shah, R. B., Lim, R., Paholak, H. J., et al. (2017). Sulforaphane enhances the anticancer activity of taxanes against triple negative breast cancer by killing cancer stem cells. Cancer Lett. 394, 52–64. doi:10.1016/J.CANLET.2017.02.023
Bury, M., le Calvé, B., Ferbeyre, G., Blank, V., and Lessard, F. (2021). New insights into CDK regulators: novel opportunities for cancer therapy. Trends Cell Biol. 31, 331–344. doi:10.1016/J.TCB.2021.01.010
Cai, Y., Yu, S. S., He, Y., Bi, X. Y., Gao, S., Yan, T. D., et al. (2021). EGCG inhibits pressure overload-induced cardiac hypertrophy via the PSMB5/Nmnat2/SIRT6-dependent signalling pathways. Acta Physiol. 231, e13602. doi:10.1111/APHA.13602
Carrasco-Legleu, C. E., Márquez-Rosado, L., Fattel-Fazenda, S., Arce-Popoca, E., Pérez-Carreón, J. I., and Villa-Treviño, S. (2004). Chemoprotective effect of caffeic acid phenethyl ester on promotion in a medium-term rat hepatocarcinogenesis assay. Int. J. Cancer 108, 488–492. doi:10.1002/IJC.11595
Chen, H., Zhu, B., Zhao, L., Liu, Y., Zhao, F., Feng, J., et al. (2018). Allicin inhibits proliferation and invasion in vitro and in vivo via SHP-1-Mediated STAT3 signaling in cholangiocarcinoma. Cell. Physiology Biochem. 47, 641–653. doi:10.1159/000490019
Choudhari, A. S., Mandave, P. C., Deshpande, M., Ranjekar, P., and Prakash, O. (2020). Phytochemicals in cancer treatment: from preclinical studies to clinical practice. Front. Pharmacol. 10, 497776. doi:10.3389/fphar.2019.01614
Clarke, P., and Tyler, K. L. (2009). Apoptosis in animal models of virus-induced disease. Nat. Rev. Microbiol. 7, 144–155. doi:10.1038/NRMICRO2071
Contant, C., Rouabhia, M., Loubaki, L., Chandad, F., and Semlali, A. (2021). Anethole induces anti-oral cancer activity by triggering apoptosis, autophagy and oxidative stress and by modulation of multiple signaling pathways. Sci. Rep. 11, 13087. doi:10.1038/S41598-021-92456-W
Cools, S., van den Broeck, W., Vanhaecke, L., Heyerick, A., Bossaert, P., Hostens, M., et al. (2014). Feeding soybean meal increases the blood level of isoflavones and reduces the steroidogenic capacity in bovine corpora lutea, without affecting peripheral progesterone concentrations. Anim. Reprod. Sci. 144, 79–89. doi:10.1016/J.ANIREPROSCI.2013.12.008
Daisy Precilla, S., Biswas, I., Kuduvalli, S. S., and Anitha, T. S. (2022). Crosstalk between PI3K/AKT/mTOR and WNT/β-Catenin signaling in GBM - could combination therapy checkmate the collusion? Cell Signal 95, 110350. doi:10.1016/j.cellsig.2022.110350
Deitrick, J., and Pruitt, W. M. (2016). Wnt/β catenin-mediated signaling commonly altered in colorectal cancer. Prog. Mol. Biol. Transl. Sci. 144, 49–68. doi:10.1016/bs.pmbts.2016.09.010
do Nascimento Silva, M. K., de Alencar Carvalho, V. R., and Matias, E. F. F. (2016). Chemical profile of essential oil of ocimum gratissimum L. And evaluation of antibacterial and drug resistance-modifying activity by gaseous contact method. Pharmacogn. J. 8, 4–9. doi:10.5530/pj.2016.1.2
Duan, C., Yu, M., Xu, J., Li, B. Y., Zhao, Y., and Kankala, R. K. (2023). Overcoming cancer multi-drug resistance (MDR): reasons, mechanisms, nanotherapeutic solutions, and challenges. Biomed. Pharmacother. 162, 114643. doi:10.1016/J.BIOPHA.2023.114643
Dutta, A., Dahiya, A., Prakash, A., and Agrawala, P. K. (2021). Acute toxicity of diallyl sulfide derived from Allium sativum (garlic) in mice and its possible mechanisms. Phytomedicine Plus 1, 100084. doi:10.1016/J.PHYPLU.2021.100084
Eiermann, W., Paepke, S., Appfelstaedt, J., Llombart-Cussac, A., Eremin, J., Vinholes, J., et al. (2001). Preoperative treatment of postmenopausal breast cancer patients with letrozole: a randomized double-blind multicenter study. Ann. Oncol. 12, 1527–1532. doi:10.1023/A:1013128213451
Elkashty, O. (2020) Effectiveness of sulforaphane in increasing drug mediated cytotoxicity toward cancer stem cells in head and neck squamous cell carcinoma. McGill University.
Eloranta, S., Smedby, K. E., Dickman, P. W., and Andersson, T. M. (2021). Cancer survival statistics for patients and healthcare professionals – a tutorial of real-world data analysis. J. Intern Med. 289, 12–28. doi:10.1111/JOIM.13139
Farombi, E. O., Ajayi, B. O., and Adedara, I. A. (2020). 6-Gingerol delays tumorigenesis in benzo[a]pyrene and dextran sulphate sodium-induced colorectal cancer in mice. Food Chem. Toxicol. 142, 111483. doi:10.1016/J.FCT.2020.111483
Ferlay, J., Colombet, M., Soerjomataram, I., Parkin, D. M., Piñeros, M., Znaor, A., et al. (2021). Cancer statistics for the year 2020: an overview. Int. J. Cancer 149, 778–789. doi:10.1002/IJC.33588
Ferrari, E., Bettuzzi, S., and Naponelli, V. (2022). The potential of epigallocatechin gallate (EGCG) in targeting autophagy for cancer treatment: a narrative review. Int. J. Mol. Sci. 23, 6075. doi:10.3390/IJMS23116075
Fuentes, C., Verdú, S., Fuentes, A., Ruiz, M. J., and Barat, J. M. (2022). In vivo toxicity assessment of eugenol and vanillin-functionalised silica particles using Caenorhabditis elegans. Ecotoxicol. Environ. Saf. 238, 113601. doi:10.1016/J.ECOENV.2022.113601
Fukai, T., and Ushio-Fukai, M. (2011). Superoxide dismutases: role in redox signaling, vascular function, and diseases. Antioxid. Redox Signal 15, 1583–1606. doi:10.1089/ARS.2011.3999
Gandhi, N. S., Tekade, R. K., and Chougule, M. B. (2014). Nanocarrier mediated delivery of siRNA/miRNA in combination with chemotherapeutic agents for cancer therapy: current progress and advances. J. Control. Release 194, 238–256. doi:10.1016/J.JCONREL.2014.09.001
Ganjare, A. B., Nirmal, S. A., and Patil, A. N. (2011). Use of apigenin from Cordia dichotoma in the treatment of colitis. Fitoterapia 82, 1052–1056. doi:10.1016/J.FITOTE.2011.06.008
Gilardini Montani, M. S., D’Eliseo, D., Cirone, M., Di Renzo, L., Faggioni, A., Santoni, A., et al. (2015). Capsaicin-mediated apoptosis of human bladder cancer cells activates dendritic cells via CD91. Nutrition 31, 578–581. doi:10.1016/j.nut.2014.05.005
Gleńsk, M., Dudek, M. K., Rybacka, A., Włodarczyk, M., and Fecka, I. (2021). Triterpenoids from strawberry fragaria × ananassa duch. Cultivar senga sengana leaves. Ind. Crops Prod. 169, 113668. doi:10.1016/J.INDCROP.2021.113668
Goldar, S., Khaniani, M. S., Derakhshan, S. M., and Baradaran, B. (2015). Molecular mechanisms of apoptosis and roles in cancer development and treatment. Asian Pac. J. Cancer Prev. 16, 2129–2144. doi:10.7314/APJCP.2015.16.6.2129
Han, Y. H., Liu, C. G., Chen, B. Q., Fu, C. P., Kankala, R. K., Wang, S. B., et al. (2022). Orchestrated tumor apoptosis (Cu2+) and bone tissue calcification (Ca2+) by hierarchical Copper/Calcium-ensembled bioactive silica for osteosarcoma therapy. Chem. Eng. J. 435, 134820. doi:10.1016/J.CEJ.2022.134820
Hang, N. T., Thi Tu Uyen, T., and Van Phuong, N. (2022). Green extraction of apigenin and luteolin from celery seed using deep eutectic solvent. J. Pharm. Biomed. Anal. 207, 114406. doi:10.1016/J.JPBA.2021.114406
Hao, R., Jiang, Y., Li, F., Sun-Waterhouse, D., and Li, D. (2021). MiR-182–5p/TLR4/NF-κB axis contributes to the protective effect of caffeic acid phenethyl ester against cadmium-induced spleen toxicity and associated damage in mice. Food Chem. Toxicol. 158, 112654. doi:10.1016/J.FCT.2021.112654
Hao, W., Zhu, X., Liu, Z., Song, Y., Wu, S., Lu, X., et al. (2022). Resveratrol alleviates aluminum-induced intestinal barrier dysfunction in mice. Environ. Toxicol. 37, 1373–1381. doi:10.1002/TOX.23490
Hassan, M., Watari, H., Abualmaaty, A., Ohba, Y., and Sakuragi, N. (2014). Apoptosis and molecular targeting therapy in cancer. Biomed. Res. Int. 2014, 150845. doi:10.1155/2014/150845
Hayman, M., and Kam, P. C. A. (2008). Capsaicin: a review of its pharmacology and clinical applications. Curr. Anaesth. Crit. Care 19, 338–343. doi:10.1016/J.CACC.2008.07.003
He, B., You, L., Uematsu, K., Zang, K., Xu, Z., Lee, A. Y., et al. (2003). SOCS-3 is frequently silenced by hypermethylation and suppresses cell growth in human lung cancer. Proc. Natl. Acad. Sci. U. S. A. 100, 14133–14138. doi:10.1073/pnas.2232790100
Heptinstall, A. B., Adiyasa, I., Cano, C., and Hardcastle, I. R. (2018). Recent advances in CDK inhibitors for cancer therapy. Future Med. Chem. 10, 1369–1388. doi:10.4155/FMC-2017-0246
Hosseini, A., and Ghorbani, A. (2015). Cancer therapy with phytochemicals: evidence from clinical studies. Avicenna J. Phytomed 5, 84–97.
Hu, X., Dutta, P., Tsurumi, A., Li, J., Wang, J., Land, H., et al. (2013). Unphosphorylated STAT5A stabilizes heterochromatin and suppresses tumor growth. Proc. Natl. Acad. Sci. U. S. A. 110, 10213–10218. doi:10.1073/pnas.1221243110
Hu, X., li, J., Fu, M., Zhao, X., and Wang, W. (2021). The JAK/STAT signaling pathway: from bench to clinic. Signal Transduct. Target. Ther. 6 (1), 402–433. doi:10.1038/s41392-021-00791-1
Huang, Y.-T., Liang, Q.-Q., Zhang, H.-R., Chen, S.-Y., Xu, L.-H., Zeng, B., et al. (2022). Baicalin inhibits necroptosis by decreasing oligomerization of phosphorylated MLKL and mitigates caerulein-induced acute pancreatitis in mice. Int. Immunopharmacol. 108, 108885. doi:10.1016/J.INTIMP.2022.108885
Huminiecki, L., and Horbańczuk, J. (2018). The functional genomic studies of resveratrol in respect to its anti-cancer effects. Biotechnol. Adv. 36, 1699–1708. doi:10.1016/J.BIOTECHADV.2018.02.011
Hussain, A., Brahmbhatt, K., Priyani, A., Ahmed, M., Rizvi, T. A., and Sharma, C. (2011). Eugenol enhances the chemotherapeutic potential of gemcitabine and induces anticarcinogenic and anti-inflammatory activity in human cervical cancer cells. Cancer Biother Radiopharm. 26, 519–527. doi:10.1089/CBR.2010.0925
International, B. M. R. (2020). Retracted: apoptosis and molecular targeting therapy in cancer. Biomed. Res. Int. 2020, 2451249. doi:10.1155/2020/2451249
Jain, A., Sharma, G., Kushwah, V., Ghoshal, G., Jain, A., Singh, B., et al. (2018). Beta carotene-loaded zein nanoparticles to improve the biopharmaceutical attributes and to abolish the toxicity of methotrexate: a preclinical study for breast cancer. Artif. Cells Nanomed Biotechnol. 46, 402–412. doi:10.1080/21691401.2018.1428811
Jeffery, J., Sinha, D., Srihari, S., Kalimutho, M., and Khanna, K. K. (2015). Beyond cytokinesis: the emerging roles of CEP55 in tumorigenesis. Oncogene 35 (6), 683–690. doi:10.1038/onc.2015.128
Jiang, L., Zeng, H., Ni, L., Qi, L., Xu, Y., Xia, L., et al. (2019). HIF-1α preconditioning potentiates antioxidant activity in ischemic injury: the role of sequential administration of dihydrotanshinone I and protocatechuic aldehyde in cardioprotection. Antioxid. Redox Signal 31, 227–242. doi:10.1089/ARS.2018.7624
Jones, P. A., and Baylin, S. B. (2007). The epigenomics of cancer. Cell 128, 683–692. doi:10.1016/J.CELL.2007.01.029
Kabała-Dzik, A., Rzepecka-Stojko, A., Kubina, R., Wojtyczka, R. D., Buszman, E., and Stojko, J. (2018). Caffeic acid versus caffeic acid phenethyl ester in the treatment of breast cancer MCF-7 cells: migration rate inhibition. Integr. Cancer Ther. 17, 1247–1259. doi:10.1177/1534735418801521
Kavalappa, Y. P., Udayawara Rudresh, D., Gopal, S. S., Haranahalli Shivarudrappa, A., Stephen, N. M., Rangiah, K., et al. (2019). β-carotene isolated from the marine red alga, Gracillaria sp. potently attenuates the growth of human hepatocellular carcinoma (HepG2) cells by modulating multiple molecular pathways. J. Funct. Foods 52, 165–176. doi:10.1016/J.JFF.2018.11.015
Khan, A. Q., Rashid, K., AlAmodi, A. A., Agha, M. V., Akhtar, S., Hakeem, I., et al. (2021). Reactive oxygen species (ROS) in cancer pathogenesis and therapy: an update on the role of ROS in anticancer action of benzophenanthridine alkaloids. Biomed. Pharmacother. 143, 112142. doi:10.1016/j.biopha.2021.112142
Krishnamurthy, N., and Kurzrock, R. (2018). Targeting the Wnt/beta-catenin pathway in cancer: update on effectors and inhibitors. Cancer Treat. Rev. 62, 50–60. doi:10.1016/J.CTRV.2017.11.002
Krishnan, K., Steptoe, A. L., Martin, H. C., Wani, S., Nones, K., Waddell, N., et al. (2013). MicroRNA-182-5p targets a network of genes involved in DNA repair. RNA 19, 230–242. doi:10.1261/RNA.034926.112
Kuttikrishnan, S., Siveen, K. S., Prabhu, K. S., Khan, A. Q., Akhtar, S., Mateo, J. M., et al. (2019). Sanguinarine suppresses growth and induces apoptosis in childhood acute lymphoblastic leukemia. Leuk. Lymphoma 60, 782–794. doi:10.1080/10428194.2018.1494270
Li, H. B., Jiang, Y., and Chen, F. (2004). Separation methods used for Scutellaria baicalensis active components. J. Chromatogr. B 812, 277–290. doi:10.1016/J.JCHROMB.2004.06.045
Li, L. N., Zhang, H. D., Yuan, S. J., Tian, Z. Y., Wang, L., and Sun, Z. X. (2007). Artesunate attenuates the growth of human colorectal carcinoma and inhibits hyperactive Wnt/beta-catenin pathway. Int. J. Cancer 121, 1360–1365. doi:10.1002/IJC.22804
Li, M. (2021). The role of P53 up-regulated modulator of apoptosis (PUMA) in ovarian development, cardiovascular and neurodegenerative diseases. Apoptosis 26 (5 26), 235–247. doi:10.1007/S10495-021-01667-Z
Li, T., Li, J., Chen, Z., Zhang, S., Li, S., Wageh, S., et al. (2022). Glioma diagnosis and therapy: current challenges and nanomaterial-based solutions. J. Control. Release 352, 338–370. doi:10.1016/J.JCONREL.2022.09.065
Li, X., Ao, M., Zhang, C., Fan, S., Chen, Z., and Yu, L. (2021). Zingiberis rhizoma recens: a review of its traditional uses, phytochemistry, pharmacology, and toxicology. Evidence-based Complementary Altern. Med. 2021, 6668990. doi:10.1155/2021/6668990
Li, Y., Cheng, X., Chen, C., Huijuan, W., Zhao, H., Liu, W., et al. (2020a). Apigenin, a flavonoid constituent derived from P. villosa, inhibits hepatocellular carcinoma cell growth by CyclinD1/CDK4 regulation via p38 MAPK-p21 signaling. Pathol. Res. Pract. 216, 152701. doi:10.1016/J.PRP.2019.152701
Li, Z., Veeraraghavan, V. P., Mohan, S. K., Bolla, S. R., Lakshmanan, H., Kumaran, S., et al. (2020b). Apoptotic induction and anti-metastatic activity of eugenol encapsulated chitosan nanopolymer on rat glioma C6 cells via alleviating the MMP signaling pathway. J. Photochem Photobiol. B 203, 111773. doi:10.1016/J.JPHOTOBIOL.2019.111773
Lim, J. Y., and Wang, X. D. (2020). Mechanistic understanding of β-cryptoxanthin and lycopene in cancer prevention in animal models. Biochimica Biophysica Acta (BBA) - Mol. Cell Biol. Lipids 1865, 158652. doi:10.1016/J.BBALIP.2020.158652
Liu, C. G., Han, Y. H., Kankala, R. K., Wang, S. B., and Chen, A. Z. (2020a). Subcellular performance of nanoparticles in cancer therapy. Int. J. Nanomedicine 15, 675–704. doi:10.2147/IJN.S226186
Liu, C. G., Han, Y. H., Zhang, J. T., Kankala, R. K., Wang, S. B., and Chen, A. Z. (2019). Rerouting engineered metal-dependent shapes of mesoporous silica nanocontainers to biodegradable Janus-type (sphero-ellipsoid) nanoreactors for chemodynamic therapy. Chem. Eng. J. 370, 1188–1199. doi:10.1016/J.CEJ.2019.03.272
Liu, J., Peng, Y., and Wei, W. (2022). Cell cycle on the crossroad of tumorigenesis and cancer therapy. Trends Cell Biol. 32, 30–44. doi:10.1016/j.tcb.2021.07.001
Liu, W., Lu, X., Shi, P., Yang, G., Zhou, Z., Li, W., et al. (2020b). TNF-α increases breast cancer stem-like cells through up-regulating TAZ expression via the non-canonical NF-κB pathway. Sci. Rep. 10 (1), 1804–1811. doi:10.1038/s41598-020-58642-y
Liu, X., Su, K., Sun, X., Jiang, Y., Wang, L., Hu, C., et al. (2021). Sec62 promotes stemness and chemoresistance of human colorectal cancer through activating Wnt/β-catenin pathway. J. Exp. Clin. Cancer Res. 40, 132–217. doi:10.1186/s13046-021-01934-6
Loscocco, G. G., and Vannucchi, A. M. (2022). Role of JAK inhibitors in myeloproliferative neoplasms: current point of view and perspectives. Int. J. Hematol. 115, 626–644. doi:10.1007/S12185-022-03335-7
Lubet, R. A., Steele, V. E., Eto, I., Juliana, M. M., Kelloff, G. J., and Grubbs, C. J. (1997). Chemopreventive efficacy of anethole trithione, N-Acetyl-L-Cysteine, miconazole and phenethylisothiocyanate in the dmba-induced rat mammary cancer model. J. Cancer 72, 95–101. doi:10.1002/(sici)1097-0215(19970703)72:1<95::aid-ijc14>3.0.co;2-9
Lv, S., Liu, Y., Xie, C., Xue, C., Du, S., and Yao, J. (2023). Emerging role of interactions between tumor angiogenesis and cancer stem cells. J. Control. Release 360, 468–481. doi:10.1016/J.JCONREL.2023.06.036
Ma, Z., Woon, C. Y. N., Liu, C. G., Cheng, J. T., You, M., Sethi, G., et al. (2021). Repurposing artemisinin and its derivatives as anticancer drugs: a chance or challenge? Front. Pharmacol. 12, 828856. doi:10.3389/fphar.2021.828856
Malekpour-Dehkordi, Z., Nourbakhsh, M., Shahidi, M., Sarraf, N., and Sharifi, R. (2022). Silymarin diminishes oleic acid-induced lipid accumulation in HepG2 cells by modulating the expression of endoplasmic reticulum stress markers. J. Herb. Med. 33, 100565. doi:10.1016/j.hermed.2022.100565
Mansingh, D., Dalpati, N., Sali, V., and Rachel Vasanthi, A. (2018). Alliin the precursor of allicin in garlic extract mitigates proliferation of gastric adenocarcinoma cells by modulating apoptosis. Pharmacogn. Mag. 14, 84. doi:10.4103/PM.PM_342_17
Mathur, P., Sathishkumar, K., Chaturvedi, M., Das, P., Sudarshan, K. L., Santhappan, S., et al. (2020). Cancer statistics, 2020: report from national cancer registry programme, India. JCO Glob. Oncol. 6, 1063–1075. doi:10.1200/go.20.00122
Meng, S., Zhu, Y., Li, J. F., Wang, X., Liang, Z., Li, S. Q., et al. (2017). Apigenin inhibits renal cell carcinoma cell proliferation. Oncotarget 8, 19834–19842. doi:10.18632/ONCOTARGET.15771
Metibemu, D. S., Akinloye, O. A., Omotuyi, I. O., Okoye, J. O., Popoola, M. A., and Akamo, A. J. (2021). Carotenoid-enriched fractions from spondias mombin demonstrate HER2 ATP kinase domain inhibition: computational and in vivo animal model of breast carcinoma studies. Front. Oncol. 11, 687190. doi:10.3389/fonc.2021.687190
Mizerska-Kowalska, M., Płazí, W., Sowa, S., Paduch, R., Moreno, Q. G., Herrera, R. A., et al. (2022). Proapoptotic effect and molecular docking analysis of curcumin–resveratrol hybrids in colorectal cancer chemoprevention. Molecules 27, 3486. doi:10.3390/MOLECULES27113486
Nagoor Meeran, M. F., Javed, H., Sharma, C., Goyal, S. N., Kumar, S., Jha, N. K., et al. (2021). Can Echinacea be a potential candidate to target immunity, inflammation, and infection - the trinity of coronavirus disease 2019. Heliyon 7, e05990. doi:10.1016/J.HELIYON.2021.E05990
Nakano, K., and Vousden, K. H. (2001). PUMA, a novel proapoptotic gene, is induced by p53. Mol. Cell 3, 683–694. doi:10.1016/s1097-2765(01)00214-3
Nayak, S., and Sashidhar, R. B. (2010). Metabolic intervention of aflatoxin B1 toxicity by curcumin. J. Ethnopharmacol. 127, 641–644. doi:10.1016/J.JEP.2009.12.010
Nica-Badea, D., and Udristioiu, A. (2021). The relevance of supplemental vitamin D in malignancies. Anticancer Agents Med. Chem. 21, 1942–1949. doi:10.2174/1871520621666210112115846
Nishida, N., Yano, H., Nishida, T., Kamura, T., and Kojiro, M. (2006). Angiogenesis in cancer. Vasc. Health Risk Manag. 2, 213–219. doi:10.2147/VHRM.2006.2.3.213
Oliveira, G., Marques, C., de Oliveira, A., de Almeida dos Santos, A., do Amaral, W., Ineu, R. P., et al. (2021). Extraction of bioactive compounds from Curcuma longa L. using deep eutectic solvents: in vitro and in vivo biological activities. Innovative Food Sci. Emerg. Technol. 70, 102697. doi:10.1016/j.ifset.2021.102697
Ouyang, L., Shi, Z., Zhao, S., Wang, F. T., Zhou, T. T., Liu, B., et al. (2012). Programmed cell death pathways in cancer: a review of apoptosis, autophagy and programmed necrosis. Cell Prolif. 45, 487–498. doi:10.1111/J.1365-2184.2012.00845.X
Patel, S., Alam, A., Pant, R., and Chattopadhyay, S. (2019). Wnt signaling and its significance within the tumor microenvironment: novel therapeutic insights. Front. Immunol. 10, 2872. doi:10.3389/fimmu.2019.02872
Plati, J., Bucur, O., and Khosravi-Far, R. (2011). Apoptotic cell signaling in cancer progression and therapy. Integr. Biol. 3, 279–296. doi:10.1039/c0ib00144a
Prakash Maurya, R., Prajapat, M. K., Singh, V. P., Roy, M., Todi, R., Bosak, S., et al. (2021). Serum malondialdehyde as a biomarker of oxidative stress in patients with primary ocular carcinoma: impact on response to chemotherapy. Impact Response Chemother. 15, 871–879. doi:10.2147/OPTH.S287747
Rady, I., Mohamed, H., Rady, M., Siddiqui, I. A., and Mukhtar, H. (2018). Cancer preventive and therapeutic effects of EGCG, the major polyphenol in green tea. Egypt. J. Basic Appl. Sci. 5, 1–23. doi:10.1016/J.EJBAS.2017.12.001
Rajabi, M., and Mousa, S. A. (2017). The role of angiogenesis in cancer treatment. Biomedicines 5, 34–35. doi:10.3390/BIOMEDICINES5020034
Ravula, A. R., Teegala, S. B., Kalakotla, S., Pasangulapati, J. P., Perumal, V., and Boyina, H. K. (2021). Fisetin, potential flavonoid with multifarious targets for treating neurological disorders: an updated review. Eur. J. Pharmacol. 910, 174492. doi:10.1016/J.EJPHAR.2021.174492
Rinninella, E., Mele, M. C., Raoul, P., Cintoni, M., and Gasbarrini, A. (2022). Vitamin D and colorectal cancer: chemopreventive perspectives through the gut microbiota and the immune system. BioFactors 48, 285–293. doi:10.1002/BIOF.1786
Şahin, A. G., and Karatepe, M. (2022). Vitamins A, E, C, β -carotene contents and MDA level of freshwater mussel, (Unio elongatulus eucirrus Bourguignat 1860) in the Karakaya Dam Lake. Ege J. Fish. Aquatic Sci. 39, 120–124. doi:10.12714/EGEJFAS.39.2.05
Saraswati, S., and Agrawal, S. S. (2013). Brucine, an indole alkaloid from Strychnos nux-vomica attenuates VEGF-induced angiogenesis via inhibiting VEGFR2 signaling pathway in vitro and in vivo. Cancer Lett. 332, 83–93. doi:10.1016/J.CANLET.2013.01.012
Sarkar, A., Das, S., Rahaman, A., Das Talukdar, A., Bhattacharjee, S., and Mandal, D. P. (2020). Eugenol and capsaicin exhibit anti-metastatic activity: via modulating TGF-β signaling in gastric carcinoma. Food Funct. 11, 9020–9034. doi:10.1039/D0FO00887G
Sarkar, F. H., and Li, Y. (2002). Mechanisms of cancer chemoprevention by soy isoflavone genistein. Cancer Metastasis Rev. 21, 265–280. doi:10.1023/a:1021210910821
Scuto, M., Trovato Salinaro, A., Caligiuri, I., Ontario, M. L., Greco, V., Sciuto, N., et al. (2021). Redox modulation of vitagenes via plant polyphenols and vitamin D: novel insights for chemoprevention and therapeutic interventions based on organoid technology. Mech. Ageing Dev. 199, 111551. doi:10.1016/J.MAD.2021.111551
Shao, F., Pang, X., and Baeg, G. H. (2020). Targeting the JAK/STAT signaling pathway for breast cancer. Curr. Med. Chem. 28, 5137–5151. doi:10.2174/0929867328666201207202012
Shehatta, N. H., Okda, T. M., Omran, G. A., and Abd-Alhaseeb, M. M. (2022). Baicalin; a promising chemopreventive agent, enhances the antitumor effect of 5-FU against breast cancer and inhibits tumor growth and angiogenesis in Ehrlich solid tumor. Biomed. Pharmacother. 146, 112599. doi:10.1016/J.BIOPHA.2021.112599
Shen, L., Pan, L., Ju, C., and Wu, X. (2022). The role of Wnt/β-catenin pathway for skin-derived precursors differentiating into corneal endothelial cell-like cells. Exp. Eye Res. 218, 109008. doi:10.1016/j.exer.2022.109008
Shipitsin, M., and Polyak, K. (2008). The cancer stem cell hypothesis: in search of definitions, markers, and relevance. Lab. Investig. 88 (5), 459–463. doi:10.1038/labinvest.2008.14
Siegel, R. L., Miller, K. D., and Jemal, A. (2020). Cancer statistics, 2020. CA Cancer J. Clin. 70, 7–30. doi:10.3322/CAAC.21590
Singh, M., Lo, S. H., Dubey, R., Kumar, S., Chaubey, K. K., and Kumar, S. (2023). Plant-derived natural compounds as an emerging antiviral in combating COVID-19. Indian J. Microbiol. 63, 429–446. doi:10.1007/s12088-023-01121-5
Sojoodi, M., Wei, L., Erstad, D. J., Yamada, S., Fujii, T., Hirschfield, H., et al. (2020). Epigallocatechin gallate induces hepatic stellate cell senescence and attenuates development of hepatocellular carcinoma. Cancer Prev. Res. 13, 497–508. doi:10.1158/1940-6207.CAPR-19-0383
Spagnuolo, C., Russo, G. L., Orhan, I. E., Habtemariam, S., Daglia, M., Sureda, A., et al. (2015). Genistein and cancer: current status, challenges, and future directions. Adv. Nutr. 6, 408–419. doi:10.3945/AN.114.008052
Spychalski, M., Dziki, L., and Dziki, A. (2007). Chemoprevention of colorectal cancer - a new target needed? Colorectal Dis. 9, 397–401. doi:10.1111/J.1463-1318.2006.01166.X
Storci, G., Sansone, P., Mari, S., D’Uva, G., Tavolari, S., Guarnieri, T., et al. (2010). TNFalpha up-regulates SLUG via the NF-kappaB/HIF1alpha axis, which imparts breast cancer cells with a stem cell-like phenotype. J. Cell. Physiol. 225, 682–691. doi:10.1002/jcp.22264
Su, S. J., Yeh, T. M., Chuang, W. J., Ho, C. L., Chang, K. L., Cheng, H. L., et al. (2005). The novel targets for anti-angiogenesis of genistein on human cancer cells. Biochem. Pharmacol. 69, 307–318. doi:10.1016/J.BCP.2004.09.025
Su, Y. S., Kuo, M. Z., Kuo, Y. T., Huang, S. W., Lee, C. J., Su, Z. Y., et al. (2021a). Diterpenoid anthraquinones as chemopreventive agents altered microRNA and transcriptome expressions in cancer cells. Biomed. Pharmacother. 136, 111260. doi:10.1016/J.BIOPHA.2021.111260
Su, Z. Y., Chan, Y. F., Tung, Y. C., Sung, P. H., Chen, P. C., Lin, Z. H., et al. (2021b). Evaluating skin cancer chemopreventive potential of water extract of Syzygium samarangense leaves through activation of the Nrf2-mediated cellular defense system. South Afr. J. Bot. 137, 303–310. doi:10.1016/J.SAJB.2020.10.029
Suh, Y., Afaq, F., Johnson, J. J., and Mukhtar, H. (2009). A plant flavonoid fisetin induces apoptosis in colon cancer cells by inhibition of COX2 and Wnt/EGFR/NF-kappaB-signaling pathways. Carcinogenesis 30, 300–307. doi:10.1093/carcin/bgn269
Sung, H., Ferlay, J., Siegel, R. L., Laversanne, M., Soerjomataram, I., Jemal, A., et al. (2021). Global cancer statistics 2020: GLOBOCAN estimates of incidence and mortality worldwide for 36 cancers in 185 countries. CA Cancer J. Clin. 71, 209–249. doi:10.3322/CAAC.21660
Tokuşoğlu, Ö., and Parvizi, M. (2018). “Nutraceutical powders as sources of anticarcinogenic vitamins and carotenoids biochemistry and medical genomics approach,” in Food by-product based functional food powders, 203–248. doi:10.1201/9781315373607-10
Tsai, C. F., Chen, J. H., Chang, C. N., Lu, D. Y., Chang, P. C., Wang, S. L., et al. (2018). Fisetin inhibits cell migration via inducing HO-1 and reducing MMPs expression in breast cancer cell lines. Food Chem. Toxicol. 120, 528–535. doi:10.1016/J.FCT.2018.07.059
Verbrugge, I., Johnstone, R. W., and Smyth, M. J. (2010). SnapShot: extrinsic apoptosis pathways. Cell 143, e1–e2. doi:10.1016/j.cell.2010.12.004
Wang, J., Man, G. C. W., Chan, T. H., Kwong, J., and Wang, C. C. (2018). A prodrug of green tea polyphenol (–)-epigallocatechin-3-gallate (Pro-EGCG) serves as a novel angiogenesis inhibitor in endometrial cancer. Cancer Lett. 412, 10–20. doi:10.1016/j.canlet.2017.09.054
Wang, K., Zhang, C., Bao, J., Jia, X., Liang, Y., Wang, X., et al. (2016). Synergistic chemopreventive effects of curcumin and berberine on human breast cancer cells through induction of apoptosis and autophagic cell death. Sci. Rep. 6, 26064. doi:10.1038/SREP26064
Wang, L., Cheng, L., Zhang, B., Wang, N., and Wang, F. (2019). Tanshinone prevents alveolar bone loss in ovariectomized osteoporosis rats by up-regulating phosphoglycerate dehydrogenase. Toxicol. Appl. Pharmacol. 376, 9–16. doi:10.1016/j.taap.2019.05.014
Wang, M., Wang, J., Li, B., Meng, L., and Tian, Z. (2017). Recent advances in mechanism-based chemotherapy drug-siRNA pairs in co-delivery systems for cancer: a review. Colloids Surf. B Biointerfaces 157, 297–308. doi:10.1016/j.colsurfb.2017.06.002
Wang, N., Xiao, Y. F., Qian, X. Y., and Han, Y. Q. (2020). Preparation and characterization of eugenol/starch inclusion compound freeze-dried powder. Mod. Food Sci. Technol. 36, 180–187. doi:10.13982/J.MFST.1673-9078.2020.12.0586
Wang, X., Yang, X., Wang, J., Li, L., Zhang, Y., Jin, M., et al. (2022). Cardiotoxicity of sanguinarine via regulating apoptosis and MAPK pathways in zebrafish and HL1 cardiomyocytes. Comp. Biochem. Physiology Part C Toxicol. Pharmacol. 252, 109228. doi:10.1016/J.CBPC.2021.109228
Wang, Y., Yuan, A. J., Wu, Y. J., Wu, L. M., and Zhang, L. (2023). Silymarin in cancer therapy: mechanisms of action, protective roles in chemotherapy-induced toxicity, and nanoformulations. J. Funct. Foods 100, 105384. doi:10.1016/J.JFF.2022.105384
Wang, Z., Xie, Y., Sabier, M., Zhang, T., Deng, J., Song, X., et al. (2021). Trans-anethole is a potent toxic fumigant that partially inhibits rusty grain beetle (Cryptolestes ferrugineus) acetylcholinesterase activity. Ind. Crops Prod. 161, 113207. doi:10.1016/J.INDCROP.2020.113207
Wei, H., Ge, Q., Zhang, L. Y., Xie, J., Gan, R. H., Lu, Y. G., et al. (2022). EGCG inhibits growth of tumoral lesions on lip and tongue of K-Ras transgenic mice through the Notch pathway. J. Nutr. Biochem. 99, 108843. doi:10.1016/J.JNUTBIO.2021.108843
Xiang, Q., Guo, W., Tang, X., Cui, S., Zhang, F., Liu, X., et al. (2021). Capsaicin—the spicy ingredient of chili peppers: a review of the gastrointestinal effects and mechanisms. Trends Food Sci. Technol. 116, 755–765. doi:10.1016/J.TIFS.2021.08.034
Xu, M., Jiang, L. F., Wu, T., Chu, J. H., Wei, Y. D., Aa, J. Y., et al. (2018). Inhibitory effects of danshen components on CYP2C8 and CYP2J2. Chem. Biol. Interact. 289, 15–22. doi:10.1016/J.CBI.2018.04.011
Xu, X. D., Teng, Y., Zou, J. Y., Ye, Y., Song, H., and Wang, Z. Y. (2020a). Effects of lycopene on vascular remodeling through the LXR–PI3K–AKT signaling pathway in APP/PS1 mice: lycopene in vascular remodeling via the LXR–PI3K–AKT pathway. Biochem. Biophys. Res. Commun. 526, 699–705. doi:10.1016/j.bbrc.2020.02.063
Xu, Y., Zhou, Q., Feng, X., Dai, Y., Jiang, Y., Jiang, W., et al. (2020b). Disulfiram/copper markedly induced myeloma cell apoptosis through activation of JNK and intrinsic and extrinsic apoptosis pathways. Biomed. Pharmacother. 126, 110048. doi:10.1016/J.BIOPHA.2020.110048
Yang, L., Shi, P., Zhao, G., Xu, J., Peng, W., Zhang, J., et al. (2020). Targeting cancer stem cell pathways for cancer therapy. Signal Transduct. Target. Ther. 5 (1), 8–35. doi:10.1038/s41392-020-0110-5
Yu, J., Zhang, L., Hwang, P. M., Kinzler, K. W., and Vogelstein, B. (2001). PUMA induces the rapid apoptosis of colorectal cancer cells. Mol. Cell 3, 673–682. doi:10.1016/s1097-2765(01)00213-1
Zhan, T., Rindtorff, N., and Boutros, M. (2017). Wnt signaling in cancer. Oncogene 36, 1461–1473. doi:10.1038/ONC.2016.304
Zhang, B., Wang, X., Deng, J., Zheng, H., Liu, W., Chen, S., et al. (2019). p53-dependent upregulation of miR-16-2 by sanguinarine induces cell cycle arrest and apoptosis in hepatocellular carcinoma. Cancer Lett. 459, 50–58. doi:10.1016/J.CANLET.2019.05.042
Zhang, M., Zhang, L., Hei, R., Li, X., Cai, H., Wu, X., et al. (2021). CDK inhibitors in cancer therapy, an overview of recent development. Am. J. Cancer Res. 11, 1913–1935.
Zhao, Q., Chen, X. Y., and Martin, C. (2016). Scutellaria baicalensis, the golden herb from the garden of Chinese medicinal plants. Sci. Bull. (Beijing) 61, 1391–1398. doi:10.1007/S11434-016-1136-5
Zheng, M., and Ma, J. (2017). Advantages and disadvantages of minimally invasive surgery in colorectal cancer surgery. Zhonghua Wei Chang. Wai Ke Za Zhi 20, 601–605.
Glossary
Keywords: cancer prevention, molecular pathways, effector molecules, herbal metabolites, toxicology
Citation: Sharma V, Chaudhary AA, Bawari S, Gupta S, Mishra R, Khan S-U-D, Ali MAM, Shahid M, Srivastava S, Verma D, Gupta A, Kumar S and Kumar S (2024) Unraveling cancer progression pathways and phytochemical therapeutic strategies for its management. Front. Pharmacol. 15:1414790. doi: 10.3389/fphar.2024.1414790
Received: 09 April 2024; Accepted: 09 May 2024;
Published: 23 August 2024.
Edited by:
Basel A. Abdel-Wahab, Najran University, Saudi ArabiaReviewed by:
Ruo Wang, Shanghai Jiao Tong University, ChinaRanjith Kumar Kankala, Huaqiao University, China
Copyright © 2024 Sharma, Chaudhary, Bawari, Gupta, Mishra, Khan, Ali, Shahid, Srivastava, Verma, Gupta, Kumar and Kumar. This is an open-access article distributed under the terms of the Creative Commons Attribution License (CC BY). The use, distribution or reproduction in other forums is permitted, provided the original author(s) and the copyright owner(s) are credited and that the original publication in this journal is cited, in accordance with accepted academic practice. No use, distribution or reproduction is permitted which does not comply with these terms.
*Correspondence: Sandeep Kumar, cGguc2FuZGVlcEBnbWFpbC5jb20=; Sanjay Kumar, U2FuamF5Lmt1bWFyN0BzaGFyZGEuYWMuaW4=
†These authors contributed equally and shared first authorship