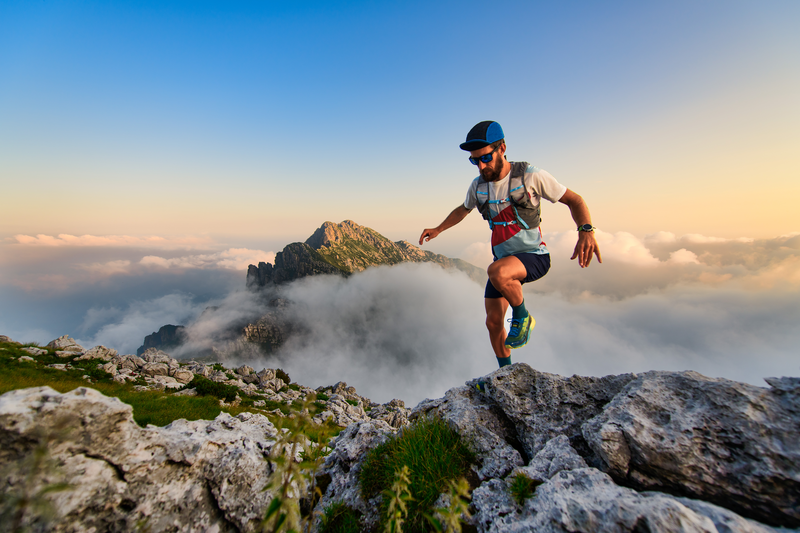
95% of researchers rate our articles as excellent or good
Learn more about the work of our research integrity team to safeguard the quality of each article we publish.
Find out more
EDITORIAL article
Front. Pharmacol. , 07 May 2024
Sec. Pharmacology of Infectious Diseases
Volume 15 - 2024 | https://doi.org/10.3389/fphar.2024.1406043
This article is part of the Research Topic Combinational therapy and nanotechnologies in combating pathogenic microbes and antibiotic resistance View all 5 articles
Editorial on the Research Topic
Combinational therapy and nanotechnologies in combating pathogenic microbes and antibiotic resistance
While antibiotics have significantly reduced bacterial infections and deaths, their indiscriminate use and environmental factors have resulted in multidrug resistance (MDR), which limits the effectiveness of current therapies. MDR is estimated to cause 700,000 deaths annually and could rise to 10 million by 2050 without immediate intervention (O’Neill, 2016). Developing new antibiotics is a potential solution, but the development pipeline is limited, and MDR evolves rapidly. To overcome these limitations, a comprehensive understanding of the limitations of current antibiotic therapy and new combinatorial strategies with multiple antibiotics and antimicrobials (Tyers and Wright, 2019) are needed.
The current topic explores the comparative pharmacokinetics (PK) of polymyxin B (PMB), a last-resort antibiotic for MDR Gram-negative bacterial infections, and a meta-analysis of high-dose isoniazid therapy for MDR or XDR Mycobacterium tuberculosis (MTB). Additionally, it introduces new combination therapies involving glutathione (GSH) and nanocomposites.
Carbapenem-resistant Klebsiella pneumoniae (CRKP) is a major contributor to nosocomial infections in humans, particularly those leading to hospital-acquired urinary, pneumonia, and bloodstream infections (Pendleton et al., 2013) and has been regarded as one of the critical-priority bacteria by the WHO (Tacconelli et al., 2018; Zhen et al., 2021). This drug-resistant bacterium is challenging to treat due to its resistance to multiple antibiotics like β-lactams, fluoroquinolones, and aminoglycosides. However, exogenous GSH, with its antibacterial properties and ability to clear biofilms, could be a potential solution (Das et al., 2019). GSH supports antibiotics like quinolones and aminoglycosides and enhances bacterial killing and impacts antibiotic effectiveness (Goswami et al., 2006; Goswami and Jawali, 2007). The study by Yi et al. (2023) demonstrated that GSH increases the potency of meropenem, a commonly used antibiotic for severe MDR Gram-negative pathogens, including CRKP (Truong et al., 2022). The authors determined the minimum inhibitory concentration (MIC) and minimum bactericidal concentration (MBC) of GSH against 30 CRKP isolates and found that 9 mg/mL of GSH effectively eradicated 99.9% of CRKP. Additionally, the synergy between GSH and meropenem was evaluated by determining the fractional inhibitory concentration index (FICI), and it was found that 86.7% of the isolates showed significant antagonism of bacterial growth after 24 h of exposure to the synergistic combination. The GSH-induced potency of meropenem was found to be concentration-dependent, and the underlying mechanisms were assessed by analyzing common antimicrobial mechanisms, including ROS generation and metabolite analysis. The study found that the increase in membrane permeability due to alterations in glycerophospholipids is the plausible mechanism of the synergy, which could potentially provide a new route for CRKP treatment.
Polymyxins are typically considered last-resort antibiotics against extensively drug-resistant (XDR) Gram-negative bacteria, with PMB being associated with a high rate of nephrotoxicity, believed to originate from its accumulation in the renal proximal tubule (Manchandani et al., 2015; Yun et al., 2015; Liu et al., 2023). While international guidelines recommend calculating the dose of PMB based on the patient’s weight, regardless of age (Tsuji et al., 2019), the relationship between age, illness status, and polymyxin-related nephrotoxicity remains controversial. To investigate PMB exposure in elderly and young critically ill patients and determine the covariates of PK for PMB in critically ill patients, Zeng et al. (2024) measured plasma PMB concentrations over a 24-hour period at steady state. Their results showed that total body weight, rather than age, was the primary factor affecting PMB clearance, consistent with prior studies. However, this study also revealed that elderly patients exhibited delayed PMB clearance and metabolism compared to young critically ill patients. This research is noteworthy as it is the first to compare PMB exposure and individual PK parameters in critically ill patients of different ages, given standard PMB dosing, and contributes to optimizing PMB use in clinical practice for critically ill patients. A limitation of this study, however, is its small sample size of critically ill patients with varying renal functions, ages, and body weights.
Mycobacterium tuberculosis continues to be a significant challenge as it is the leading cause of mortality globally (WHO, 2023). Despite its simplicity, the action mechanism of isoniazid (INH), the most efficient prophylactic drug against MTB infections since 1952 (Fernandes et al., 2017), is complicated (Unissa et al., 2016). Its continuous use can result in the emergence of MDR and XDR MTB due to acquired genetic mutations (Dominguez et al., 2023). Although the WHO previously recommended high-dose INH as an MDR and XDR MTB regimen (WHO, 2019), it was removed from standard treatment in recent WHO guidelines (WHO, 2020) due to insufficient efficacy data. However, high-dose INH is still indicated for children, patients without sufficient alternatives, and special mutants caused by low-level INH resistance (Dominguez et al., 2023). Some studies have reported the clinical efficacy of the regimen (Cambau et al., 2015; Lempens et al., 2018), but no recent systematic reviews and meta-analyses have been published on the clinical efficacy and safety outcomes of high-dose INH therapy. A study by Zhou et al. (2024) found that high-dose INH administration for MDR-MTB treatment is associated with excellent efficacy and a favorable outcome, with an acceptable adverse-event profile. However, more research is needed to investigate the impact of high-dose INH on long-term outcomes and its role in specific subpopulations.
Nanocomposites, made up of matrix materials and nanofillers, are a promising alternative to conventional antibiotics, as per a comprehensive review by Saravanan et al. (2023). These nanocomposites, including metal/metal oxides, chitosan-metals, titanium-based nanoparticles, graphene-based materials, and multi-walled carbon nanotubes with or without polymers, can serve as effective antibacterial agents when tailored to enhance treatment efficacy and reduce the risk of MDR. Furthermore, combining these nanocomposites with existing antibiotics can create novel antimicrobials that increase the efficacy of current antibiotics or enable the use of abandoned antibiotics due to resistance. However, challenges such as toxicity, safety, scalability, selectivity, and bioavailability need to be addressed before clinical application.
The studies discussed in this Research Topic will offer insights into current drug usage against MDR or XDR bacterial infections, materials for targeted bacterial killing, and the development of biocompatible and modality-specific antimicrobials.
K-sK: Conceptualization, Funding acquisition, Writing–original draft, Writing–review and editing.
The author(s) declare that financial support was received for the research, authorship, and/or publication of this article. This work was supported by a two-year research grant of Pusan National University (K-sK).
The author declares that the research was conducted in the absence of any commercial or financial relationships that could be construed as a potential conflict of interest.
All claims expressed in this article are solely those of the authors and do not necessarily represent those of their affiliated organizations, or those of the publisher, the editors and the reviewers. Any product that may be evaluated in this article, or claim that may be made by its manufacturer, is not guaranteed or endorsed by the publisher.
Cambau, E., Viveiros, M., Machado, D., Raskine, L., Ritter, C., Tortoli, E., et al. (2015). Revisiting susceptibility testing in MDR-TB by a standardized quantitative phenotypic assessment in a European multicentre study. J. Antimicrob. Chemother. 70 (3), 686–696. doi:10.1093/jac/dku438
Das, T., Paino, D., Manoharan, A., Farrell, J., Whiteley, G., Kriel, F. H., et al. (2019). Conditions under which glutathione disrupts the biofilms and improves antibiotic efficacy of both ESKAPE and non-ESKAPE species. Front. Microbiol. 10, 2000. doi:10.3389/fmicb.2019.02000
Domínguez, J., Boeree, M. J., Cambau, E., Chesov, D., Conradie, F., Cox, V., et al. (2023). Clinical implications of molecular drug resistance testing for Mycobacterium tuberculosis: a 2023 TBnet/RESIST-TB consensus statement. Lancet Infect. Dis. 23 (4), e122–e137. doi:10.1016/S1473-3099(22)00875-1
Fernandes, G. F. D. S., Salgado, H. R. N., and Santos, J. L. D. (2017). Isoniazid: a review of characteristics, properties and analytical methods. Crit. Rev. Anal. Chem. 47 (4), 298–308. doi:10.1080/10408347.2017.1281098
Goswami, M., and Jawali, N. (2007). Glutathione-mediated augmentation of beta-lactam antibacterial activity against Escherichia coli. J. Antimicrob. Chemother. 60 (1), 184–185. doi:10.1093/jac/dkm121
Goswami, M., Mangoli, S. H., and Jawali, N. (2006). Involvement of reactive oxygen species in the action of ciprofloxacin against Escherichia coli. Antimicrob. Agents Chemother. 50 (3), 949–954. doi:10.1128/AAC.50.3.949-954.2006
Lempens, P., Meehan, C. J., Vandelannoote, K., Fissette, K., de Rijk, P., Van Deun, A., et al. (2018). Isoniazid resistance levels of Mycobacterium tuberculosis can largely be predicted by high-confidence resistance-conferring mutations. Sci. Rep. 8 (1), 3246. doi:10.1038/s41598-018-21378-x
Liu, S., Wu, Y., Qi, S., Shao, H., Feng, M., Xing, L., et al. (2023). Polymyxin B therapy based on therapeutic drug monitoring in carbapenem-resistant organisms sepsis: the PMB-CROS randomized clinical trial. Crit. Care. 27 (1), 232. doi:10.1186/s13054-023-04522-6
Manchandani, P., Zhou, J., Ledesma, K. R., Truong, L. D., Chow, D. S., Eriksen, J. L., et al. (2015). Characterization of polymyxin B biodistribution and disposition in an animal model. Antimicrob. Agents Chemother. 60 (2), 1029–1034. doi:10.1128/AAC.02445-15
Pendleton, J. N., Gorman, S. P., and Gilmore, B. F. (2013). Clinical relevance of the ESKAPE pathogens. Expert Rev. Anti Infect. Ther. 11 (3), 297–308. doi:10.1586/eri.13.12
Tacconelli, E., Carrara, E., Savoldi, A., Harbarth, S., Mendelson, M., Monnet, D. L., et al. (2018). Discovery, research, and development of new antibiotics: the WHO priority list of antibiotic-resistant bacteria and tuberculosis. Lancet Infect. Dis. 18 (3), 318–327. doi:10.1016/S1473-3099(17)30753-3
Truong, A. Q., Dao, X. C., Vu, D. H., Nguyen, H. A., Do, T. H. G., Tran, N. T., et al. (2022). Optimizing meropenem in highly resistant Klebsiella pneumoniae environments: population pharmacokinetics and dosing simulations in critically ill patients. Antimicrob. Agents Chemother. 66 (11), e0032122. doi:10.1128/aac.00321-22
Tsuji, B. T., Pogue, J. M., Zavascki, A. P., Paul, M., Daikos, G. L., Forrest, A., et al. (2019). International consensus guidelines for the optimal use of the polymyxins: endorsed by the American college of clinical pharmacy (ACCP), European society of clinical microbiology and infectious diseases (ESCMID), infectious diseases society of America (IDSA), international society for anti-infective Pharmacology (ISAP), society of critical care medicine (SCCM), and society of infectious diseases pharmacists (SIDP). Pharmacotherapy 39 (1), 10–39. doi:10.1002/phar.2209
Tyers, M., and Wright, G. D. (2019). Drug combinations: a strategy to extend the life of antibiotics in the 21st century. Nat. Rev. Microbiol. 17, 141–155. doi:10.1038/s41579-018-0141-x
Unissa, A. N., Subbian, S., Hanna, L. E., and Selvakumar, N. (2016). Overview on mechanisms of isoniazid action and resistance in Mycobacterium tuberculosis. Infect. Genet. Evol. 45, 474–492. doi:10.1016/j.meegid.2016.09.004
WHO (2019) WHO consolidated guidelines on drug-resistant tuberculosis treatmen. Dosing of medicines used in second-line MDR-TB regimens by weight band in patients under 15 years. Geneva: World Health Organization. Available at: https://www.ncbi.nlm.nih.gov/books/NBK539514/table/annex2.tab2/.
WHO (2020) WHO consolidated guidelines on tuberculosis. Module 4: treatment-drug-resistant tuberculosis treatment. Geneva: World Health Organization.
WHO (2023) Global tuberculosis report. Geneva: World health organization 2023. Available at: https://www.who.int/teams/global-tuberculosis-programme/tb-reports/global-tuberculosis-report-2023.
Yun, B., Azad, M. A., Wang, J., Nation, R. L., Thompson, P. E., Roberts, K. D., et al. (2015). Imaging the distribution of polymyxins in the kidney. J. Antimicrob. Chemother. 70 (3), 827–829. doi:10.1093/jac/dku441
Keywords: multidrug-resistant pathogens, combinational therapy, nanocomposites, infectious diseases, tuberculosis
Citation: Kim K-s (2024) Editorial: Combinational therapy and nanotechnologies in combating pathogenic microbes and antibiotic resistance. Front. Pharmacol. 15:1406043. doi: 10.3389/fphar.2024.1406043
Received: 24 March 2024; Accepted: 23 April 2024;
Published: 07 May 2024.
Edited and reviewed by:
Hendrik W. Van Veen, University of Cambridge, United KingdomCopyright © 2024 Kim. This is an open-access article distributed under the terms of the Creative Commons Attribution License (CC BY). The use, distribution or reproduction in other forums is permitted, provided the original author(s) and the copyright owner(s) are credited and that the original publication in this journal is cited, in accordance with accepted academic practice. No use, distribution or reproduction is permitted which does not comply with these terms.
*Correspondence: Kwang-sun Kim, a3dhbmdzdW4ua2ltQHB1c2FuLmFjLmty
Disclaimer: All claims expressed in this article are solely those of the authors and do not necessarily represent those of their affiliated organizations, or those of the publisher, the editors and the reviewers. Any product that may be evaluated in this article or claim that may be made by its manufacturer is not guaranteed or endorsed by the publisher.
Research integrity at Frontiers
Learn more about the work of our research integrity team to safeguard the quality of each article we publish.