- Department of Pharmacy, Affiliated Hospital of Jining Medical University, Jining, China
Introduction: Traditional Chinese medicine (TCM) is gaining worldwide popularity as a complementary and alternative medicine. The isolation and characterization of active ingredients from TCM has become optional strategies for drug development. In order to overcome the inherent limitations of these natural products such as poor water solubility and low bioavailability, the combination of nanotechnology with TCM has been explored. Taking advantage of the benefits offered by the nanoscale, various drug delivery systems have been designed to enhance the efficacy of TCM in the treatment and prevention of diseases.
Methods: The manuscript aims to present years of research dedicated to the application of nanotechnology in the field of TCM.
Results: The manuscript discusses the formulation, characteristics and therapeutic effects of nano-TCM. Additionally, the formation of carrier-free nanomedicines through self-assembly between active ingredients of TCM is summarized. Finally, the paper discusses the safety behind the application of nano-TCM and proposes potential research directions.
Discussion: Despite some achievements, the safety of nano-TCM still need special attention. Furthermore, exploring the substance basis of TCM formulas from the perspective of nanotechnology may provide direction for elucidating the scientific intension of TCM formulas.
1 Introduction
Since ancient times, plants have been widely used as medicinal agents for various diseases. TCM, which consists of plants, animals, and minerals, has been used in China for thousands of years (Wang et al., 2018). Among the various species used in TCM, plants account for 90% (Zuo et al., 2021). Plants are rich in bioactive metabolites, which offers the potential to treat a wide range of diseases. Based on the inherent advantages of natural products, active ingredients of TCM are currently being screened for the treatment of diseases such as cancer, diabetes, cardiovascular diseases and inflammation. For example, it has been found that flavonoids and non-flavonoid polyphenolic compounds exhibit favorable anti-inflammatory effects both in vivo and in vitro (Peng et al., 2023). The alkaloid berberine (BBR) extracted from Coptis chinensis Franch. has anti-inflammatory effect. Camptothecin (CPT) is extracted from Camptotheca acuminata Decne., which has a good anti-tumor effect (Swamy et al., 2021; Lan et al., 2022).
However, the compositional complexity and toxicity-related issues associated with herbal ingredients pose greater challenges to their use as medicines and therefore make it difficult to transition from clinical trial to the use of nanotechnology (Patra et al., 2018; Peng et al., 2023). Using drug delivery systems to deliver these ingredients may be an option to address these issues. Nanotechnology has been introduced into the research of TCM, leading to the concept of nano-TCM. By incorporating natural products in nanocarriers, their properties, such as bioavailability, targeting ability and controlled release, can be effectively improved. In nano-based delivery of herbal ingredients, organic, inorganic, and polymeric nanostructures, including nanoparticles (NPs), micelles, liposomes, and dendrimers, are often considered (Patra et al., 2018).
Nanotechnology has been widely applied in various aspects of TCM (Zheng et al., 2022). Herein, this review focused on the integration of nanotechnology with natural products derived from Chinese herbs. Different drug delivery systems based on TCM have been carefully designed and can be classified into two platforms: nanocarriers and carrier-free nanomedicines. Liposomes, micelles, NPs, and dendrimers are commonly used nanocarriers. Carrier-free nanomedicines include self-assembled nanomedicines, pharmaceutical cocrystals, and Pickering emulsions. Despite these achievements, further understanding of the safety of nano-TCM is necessary to accelerate future clinical translation. Moreover, exploring how TCM theories, such as personalized diagnosis and prescription, can be better incorporated into modern research requires further investigation. Overall, the remarkable progress in nano-TCM highlights the significant role of nanotechnology in advancing the modernization of TCM research.
2 Active ingredients of TCM
TCM is a large repository of bioactive metabolites including terpenes, flavonoids, alkaloids, glycosides, saponins and so on. For example, ginkgolides are diterpenes, ginsenosides are triterpenes, baicalin is a flavonoid, and BBR is a benzylisoquinoline alkaloid. According to statistics, 25% of new molecular entities approved by the U.S. Food and Drug Administration (FDA) from natural sources are derived from plant natural products (Li and Weng, 2017). As shown in Figure 1, medicinal plants produce structurally and functionally diverse secondary metabolites. These compounds serve as the material basis for the therapeutic effects of TCM and are also sources for innovative drugs.
3 Nano-TCM
Based on the clinical validation of several active ingredients, such as artemisinin (ART), paclitaxel (PTX), and BBR, TCM is being increasing accepted as a potential source of clinical drugs. However, due to strong hydrophobicity, poor in vivo stability, low bioavailability, and potential systemic toxicity within therapeutic doses, some ingredients fail to meet the requirements for clinical application (Watkins et al., 2015). Nano-TCM offers several advantages over herbal medicines. It improves bioavailability, enables sustained release, and enhances the solubility and permeability of poorly soluble drugs, allowing them to overcome biological barriers (Watkins et al., 2015). The application strategies for nano-TCM can be broadly categorized into two types. The first type is nanocarriers, which focus on the “efficacy” of TCM’s ingredients. It involves the development of novel carriers, such as liposomes, NPs, dendrimers, and micelles to encapsulate and deliver these active ingredients. The second type is carrier-free nanomedicine, which emphasizes the “functionality” of certain herbal ingredients. In this approach, these ingredients act as solubilizers, stabilizers, or targeting ligands, exerting therapeutic effects while also serving as carriers themselves.
3.1 Advantages of nano-TCM
3.1.1 Improved bioavailability
Many identified ingredients of TCM, such as curcumin (CUR), resveratrol (RES), and ART, are lipophilic in nature. Due to their poor solubility in the bloodstream, these compounds often require high doses for therapeutic efficacy, which can lead to toxicity or poor patient compliance (Muqbil et al., 2011). On the other hand, alkaloids such as ephedrine (EPH) and matrine (MT) are hydrophilic and encounter obstacles in crossing biological membranes (Bonifácio et al., 2014). Encapsulating these active ingredients in nanocarriers can enhance their bioavailability and reduce the required dosage to achieve therapeutic effects. CUR is a polyphenolic compound derived from the rhizome of Curcuma longa Linn., and it possesses anti-inflammatory, antioxidant, and anticancer properties (Kadota et al., 2020). Takahashi et al. (2009) encapsulated CUR in lipid-based nanocarriers (LECs). The area under the curve (AUC) analysis revealed that the AUC value in rats after oral administration of LECs was 4.96 times greater than that of free CUR. In another study, the relative bioavailability of CUR-loaded lipid polymeric NPs was increased 18.2-fold compared to free CUR (Liu Y. et al., 2019).
3.1.2 Targeted delivery
The second advantage of nano-TCM is its ability to target specific tissues or organs. Targeted delivery can increase the proportion of drugs reaching specific tissues to improve bioavailability and reduce drug side effects. Targeted delivery strategies can be divided into two categories: active targeting, which involves attaching targeting ligands to the surface of the carrier, and passive targeting, which relies on inherent properties such as size, shape, and surface charge to reach the target area without specific chemical interactions.
Active targeting is achieved by attaching different types of ligands, such as peptides, antibodies, proteins, and nucleic acids, to the surface of the carrier to improve the target to non-target ratio. Nanocarriers conjugated with folic acid (FA) have shown promise in cancer treatment. Due to the overexpression of folic receptors, FA-grafted nanocarriers can target cancer cells. Hong et al. (2021) synthesized β-cyclodextrin-polycaprolactone block copolymers and conjugated them with FA to construct CUR-loaded nanoparticles (FA-CUR-NPs) using the emulsion evaporation method. Under tumor microenvironment conditions (pH 6.4), the release rate of CUR from FA-CUR-NPs was three times greater than that under systemic circulation conditions (pH 7.4). Compared to free CUR and CUR-NPs, oral administration of FA-CUR-NPs reduced the tumor volume by three times and two times, respectively, after 30 days in mice. These indicates that FA-CUR-NPs demonstrate significantly improved therapeutic efficacy in vivo and that FA can be successfully used as a tumor-targeting ligand to enhance cellular internalization.
Lactoferrin (LF) is a glycoprotein of the transferrin (TF) family that can bind to TF receptors (TFRs) and LF membrane internalization receptors (LFRs) that are highly expressed on the surface of cancer cells and blood-brain barrier (BBB), thereby promoting entry into the cell nucleus (Agwa and Sabra, 2021). This characteristic can be used to develop active targeted drug delivery systems. A novel mesoporous magnetic nanocarrier was formed by grafting LF onto mesoporous oxide nanoparticles (MIONs) through an EDC coupling reaction. The nanocarrier can continuously release perfluorohexane (PFH) and PTX to achieve deep penetration of drugs in tumors. Prior to exposure to high-frequency magnetic fields (MF), LF-MIONs loaded with PTX and PFH exhibited slow in vitro release. A local increase in the temperature of the MIONs triggered the vaporization of PFH, leading to severe damage to the tumor spheroids. Additionally, it promoted deep penetration and increased accumulation of the nanocarrier within the tumor, thus increasing the killing potential. After a single exposure to a magnetic field for 16 days, significant inhibition of tumor growth was observed (Su et al., 2015).
Passive targeting is often an effective and cost-effective choice. Many tumors exhibit enhanced permeability and retention (EPR) effects due to vascular leakage, which is the main driving force for passive targeting (Maeda et al., 2013). NPs utilize the EPR effect to deliver drug molecules to the tumor site in a controlled and targeted manner, demonstrating significant advantages in cancer treatment. Chen et al. (2023) prepared pH-responsive and biodegradable calcium orthophosphate@liposomes (CaP@Lip) NPs for loading hydrophobic PTX and hydrophilic doxorubicin (DOX) hydrochloride. Under physiological conditions, the obtained NPs carry a negative charge but they convert to a positive charge when exposed to a weakly acidic environment, thereby promoting drug internalization. Additionally, CaP@Lip NPs undergo degradation under acidic conditions (pH 5.5), facilitating drug release and rapid metabolism of the NPs in the body. At pH 5.5, nearly 63.33% of PTX was released within 48 h, while at pH 7.4, the 40.47% of the PTX was released. The slow release of PTX in NPs and the pH-responsive drug release minimize the adverse effects on healthy cells, making it beneficial for tumor treatment (Chen et al., 2023).
3.1.3 Controlled release
The third advantage of nano-TCM is the ability to control drug release. The amount and rate of drug release from nanocarriers depend on various factors, such as the carrier material, formulation size, drug molecules, and microenvironment (Watkins et al., 2015). The choice of carrier material significantly influences drug release characteristics, and the type of polymer can be adjusted to affect the release profile. By polymerizing folic acid-conjugated nanocellulose (FA-NC) with glycidyl methacrylate (GMA) and 2-hydroxyethyl methacrylate (HEMA), Anirudhan et al. prepared an innovative drug delivery system. The hydrogen bonding interaction between the polymer carrier and CUR enhanced the loading efficiency of CUR. Approximately 91.0% of the drug was released within 48 h under acidic conditions, demonstrating controlled release without premature leakage (Anirudhan et al., 2021). In another study, Hu et al. (2023) used a zinc-based nanoscale metal-organic framework (NMOF) as carriers to prepare BR@Zn-BTB NPs loaded with BBR. It was further encapsulated using a hydrogel with ROS scavenging ability (due to the introduction of quaternary ammonium and phenyl borate functionalities) to obtain a BR@Zn-BTB gel (BZ-Gel). As the pH increased, the drug release rate from the BZ-Gel also increased. At pH 8.0, the 36-h release rate of BR was close to 80%, while at pH 7.4, it was approximately 60%, and at pH 7.0 and 6.5, it was close to 40%. In chronic and infectious wounds, the pH of the skin surface becomes alkaline (between 7.5 and 8.9) (Zhu et al., 2020). Therefore, this pH-responsive drug release BR-Gel is suitable for treating malignant diabetic foot wounds. In addition to pH responsiveness, controllable drug release nanocarriers also include temperature-sensitive (Qi X.-J. et al., 2020), redox-responsive (Guo et al., 2017), ion-sensitive (Li et al., 2015) and other types. Taking into account the target location, properties of natural compounds, and preferred carrier materials, optimizing nanocarriers will yield significant benefits.
3.2 Nanocarriers
Developing single herbal ingredient into nanomedicines is considered an innovative strategy in the development of new drugs. The strategy of nanocrystallization of single active ingredients is similar to existing mature chemical drug preparation methods, making it technically feasible. This approach allows the active ingredients to fully exert their efficacy while avoiding the challenges of the complex material basis and quality control of TCM. In recent years, researchers have extensively explored various nanocarriers, including NPs, liposomes, micelles, dendrimers, and so on (Figure 2). These new formulations help overcome the low water solubility and bioavailability issues of herbal ingredients, enabling targeted delivery to specific sites or prolonging circulation in the body.
3.2.1 Liposomes
Liposomes, a commonly used delivery systems for natural products, were first discovered by Bangham et al. in the 1960s, with the first publication in 1964 (Bangham and Horne, 1964). Liposomes are spherical structures composed of lipid molecules with both hydrophilic and hydrophobic properties (Sebaaly et al., 2016). Driven by hydrophobic interactions and other molecular interactions, amphiphilic lipid molecules spontaneously assemble into liposomes in an aqueous environment. The liposome membrane can consist of one or multiple lipid bilayers. With an aqueous core inside, the polar head groups face the inner and outer aqueous phases (Manna et al., 2019). This structure endows liposomes with the ability to encapsulate molecules with different solubilities. Lipophilic drugs can be encapsulated within phospholipid bilayers or adsorbed onto the surface of liposomes, while hydrophilic drugs can be encapsulated by the internal aqueous core. Additionally, liposomes exhibit excellent biocompatibility and biodegradability due to their phospholipid bilayer structure, facilitating favorable interactions with cell membranes and promoting effective cellular uptake (He et al., 2019).
3.2.1.1 Preparation of liposomes
The preparation techniques for liposomes can be divided into traditional methods and novel methods, and different techniques can have an impact on the final characteristics of liposomes, such as size and encapsulation efficiency (EE). Traditional methods include film hydration, reverse-phase evaporation, solvent injection, and detergent removal. These methods generally involve four steps: 1) dissolution of lipids (usually using organic solvents), 2) removal of organic solvents, 3) purification and separation of liposomes, and 4) analysis of the final liposomes (Akbarzadeh et al., 2013).
The film hydration method, also known as the Bangham method, was the earliest reported technique for liposome preparation (Bangham et al., 1967). In this method, lipids are generally dissolved in organic solvents such as ether, chloroform, or methanol, and then a lipid film is formed by evaporating and drying the organic solvent. The lipid film is then hydrated using an aqueous solvent to form liposomes (Nkanga et al., 2019). The main disadvantages of this method are the production of large and uneven liposomes, low EE, and difficulty in completely removing organic solvents.
The initial steps of the reverse-phase evaporation method are similar to those of the film hydration method. First, phospholipids are dissolved in an organic solvent to form a thin film, after which the solvent is evaporated to remove them. Next, the film is redissolved in an organic solvent, and water is added to form a water-in-oil emulsion (Pattni et al., 2015). The emulsion was then subjected to ultrasound treatment to make it more uniform. Finally, the organic solvent is evaporated under reduced pressure to form a liposome suspension (Akbarzadeh et al., 2013). The advantage of this method is that it achieves high EE in liposomes, but the disadvantage is that the encapsulated compounds are exposed to ultrasound conditions and organic solvents (Monteiro et al., 2014).
The solvent injection method involves rapidly injecting a lipid solution dissolved in an organic solvent into an aqueous medium to form liposomes (William et al., 2020). This method is commonly used for liposome preparation due to its simplicity, strong reproducibility, fast speed, and minimal lipid degradation or oxidation. However, this method still has several limitations, such as poor solubility of certain compounds in ethanol, low EE for hydrophilic compounds, and incomplete removal of ethanol (Maherani et al., 2011).
In the detergent removal method, phospholipids are dissolved by a detergent at the critical micelle concentration (Nkanga et al., 2019). After removing the detergent by column chromatography or dialysis, the phospholipid molecules self-assemble in an aqueous medium to form liposomes (Akbarzadeh et al., 2013). The size and uniformity of the liposomes produced by this method can be influenced by the initial ratio of phospholipids to detergents and the efficiency of detergent removal (Maherani et al., 2011). The disadvantage of this method is that impurities may be present in liposomes, and interactions between the detergent and the compounds can also occur.
Currently, research on new liposome preparation methods has focuses mainly on expanding the industrial production scale and making them suitable for various phospholipids and drugs (Pattni et al., 2015). Some of these new methods are improvements on traditional methods, such as direct hydration of lipid components after ultrasound treatment to avoid dissipation (Manca et al., 2013). Additionally, the application of supercritical fluid (SCF) technology in liposome production has also been explored. This method utilizes a supercritical fluid, such as CO2, maintained under supercritical conditions. The SCF method offers several advantages, including low solvent cost, environmental friendliness, controllable particle size, in-situ sterilization, and suitability for large-scale production (William et al., 2020).
3.2.1.2 Application of liposomes
Triptolide (TP) is an epoxy diterpenoid compound isolated from Tripterygium wilfordii Hook F. that has demonstrated anti-inflammatory, anti-tumor, and anti-infective properties (Chen et al., 2018). However, the narrow therapeutic window, poor water solubility, and rapid metabolism of TP limit its clinical application. To reduce adverse reactions and improve treatment efficacy, Yu et al. (2021) designed a light-activated liposome (TP/Ce6-lp). By combining the photosensitizer Ce6 with TP, this liposome can synergistically treat liver cancer through the controlled release of TP and photodynamic therapy. Studies on its anti-tumor activity have shown that TP/Ce6-lp induces cell apoptosis by upregulating Caspase-3/PARP protein expression, resulting in good therapeutic effects on patient-derived hepatocellular carcinoma xenografts (PDXHCC) after irradiation (Figure 3).
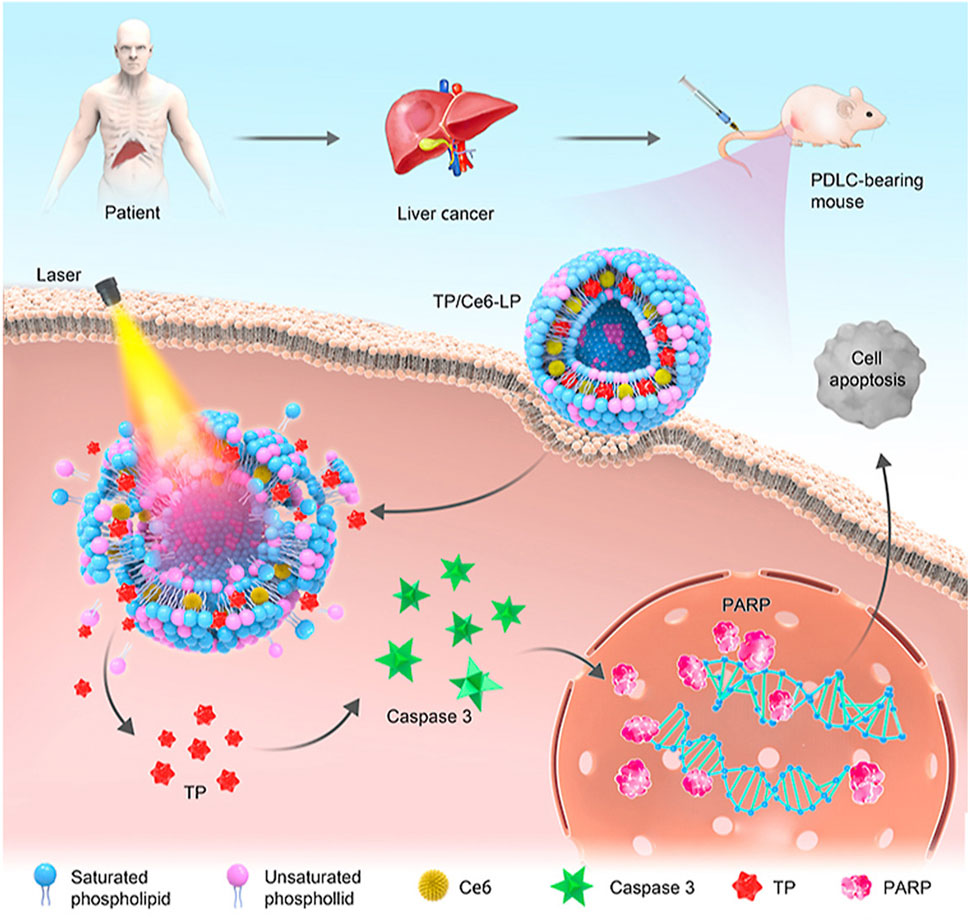
Figure 3. Schematic illustration of preparation, targeting mechanism, synchronous intracellular drug release, and visualization of photosensitive liposome@TP (TP/Ce6-LP). Reprinted from Acta Pharmaceutica Sinica B, L. Yu, Z. Wang, Z. Mo, B. Zou, Y. Yang, R. Sun, W. Ma, M. Yu, S. Zhang, Z. Yu, Synergetic delivery of triptolide and Ce6 with light-activatable liposomes for efficient hepatocellular carcinoma therapy, 2004-2015, Copyright (2021), with permission from Elsevier [OR APPLICABLE SOCIETY COPYRIGHT OWNER].
In the field of TCM, TP is commonly used to treat rheumatoid arthritis. To improve the transdermal delivery of TP in collagen-induced arthritis (CIA) rats, Chen et al., 2015) prepared a TP-loaded liposome hydrogel (TP-LHP) in the form of a microneedle patch and evaluated its pharmacokinetics and pharmacodynamics. The results showed that, after 1 week of treatment, TP-LHP had the effect on reducing joint swelling in all treatment dose groups, with the high-dose group showing the greatest efficacy. TP-LHP demonstrated sustained and stable release of TP, and significant efficacy was observed after 4 weeks of continuous treatment, indicating that the combination of TP-LHP and the microneedle delivery strategy is effective for the treatment of rheumatoid arthritis.
The active ingredients of TCM can also participate in the construction of liposomes, which can exert dual effects. In recent years, numerous published reports have shown that combination therapy with TCM can effectively improve tumor conditions and have synergistic effects with reduced toxicity (Chen et al., 2021; Lu et al., 2021). To overcome the potential toxic effects of traditional liposome formulations in the body (Moein Moghimi et al., 2006), Guo et al. (2022) incorporated glycyrrhizic acid (GA) into liposomes constructed with a mixture of saponins and phospholipids, using platycodin and ginsenoside as substitutes for cholesterol to construct saponin liposomes (RP-lipo). PR-lipo@GA exhibited similar morphological characteristics and drug release behavior to conventional liposomes but demonstrated stronger lung cancer cell targeting and anti-tumor capabilities in vitro, possibly attributed to the pharmacological properties of saponins themselves (Lu et al., 2018). This novel formulation of liposomal drug delivery system not only challenges the status of cholesterol as a component of liposomes but also provides an innovative system for the clinical application of combination therapy.
3.2.2 Polymeric micelles
3.2.2.1 Preparation of polymeric micelles
Polymeric micelles are core-shell aggregates formed by self-assembly of amphiphilic block copolymers at the critical micelle concentration. Depending on the hydrophobic and hydrophilic conditions as well as the solvent, micelles can adopt various shapes, including spherical, cylindrical, inverse micellar, and bottle-brush structures. The preparation methods for micelles include dilution (Liu et al., 2006), freeze-drying (Teagarden and Baker, 2002), solvent evaporation (Hibino et al., 2021), and dialysis (Minatti et al., 2003). During the preparation process, the physicochemical properties of the block copolymers, the sequence of addition, concentration, and water/organic solvent ratio can impact the size, polydispersity index, and stability of the micelles (Kotta et al., 2022). Micelles are capable of loading hydrophobic drugs into their core through physical encapsulation, chemical conjugation, and electrostatic interactions, exhibiting excellent stability and drug solubility in aqueous environments. Table 1 summarizes the materials and preparation methods of polymeric micelles used for loading components of Chinese herbs.
3.2.2.2 Application of polymeric micelles
Polymeric micelles, as drug carriers, have nanoscale sizes and narrow size distributions. The core-shell structure of these materials helps shield drugs from oxidation, enhancing drug stability. PTX, a type of diterpenoid alkaloid compound, has been found to induce cell cycle arrest and apoptosis in tumor cells by polymerizing tubulin dimers to stabilize microtubules (Bian et al., 2015). However, the anti-tumor potential of PTX is hindered by its poor water solubility, short biological half-life, and toxicity to normal tissues. Encapsulating PTX in biocompatible carriers is an alternative approach for targeted drug delivery. Wang Y. et al. (2020) synthesized a biotin-functionalized block copolymer, which called poly (N-2-hydroxypropylmethacrylamide)-block-poly (N-2-benzoyloxypropyl methacrylamide). This copolymer can self-assemble into polymer micelles in water, and its size is positively correlated with the length of hydrophobic segments. Due to the presence of biotin receptors on the surface of target cells, biotin-modified micelles achieve more effective internalization and exert stronger cytotoxicity. In another study, Huang et al. (2018) prepared a pH-responsive prodrug of PTX, which consisted of amphiphilic polyethylene glycol (PEG) and PTX. In the acidic environment of tumor tissues, the aldehyde linker is cleaved, resulting in the rapid release of PTX loaded in the micelles, followed by the release of conjugated PTX, thereby achieving programmable drug release.
Studies have shown that CUR inhibits tumor generation, proliferation, and metastasis by downregulating cyclin B1, activating the caspase-9/3 cascade, inhibiting the PI3K/Akt/mTOR signaling pathway, and suppressing matrix metalloproteinase-2 (MMP-2) (Yang et al., 2014). To overcome the poor water solubility of CUR, Sun et al. (2021) synthesized galactosamine-modified polyethylene glycol-polylactic acid (Gal-PEG-PLA) polymers, and prepared CUR-loaded Gal-PEG-PLA/D-α-tocopherol polyethylene glycol 1,000 succinate micelles (CUR-loaded Gal-PEG-PLA/TPGS). The size of these polymeric micelles is approximately 100 nm, with a drug loading capacity of 14.6%. The biodistribution results showed significant absorption of these micelles in the jejunum and ileum. Moreover, CUR-loaded micelles can reduce damage to liver and intestinal tissues, making them valuable for the oral administration of hydrophobic drugs.
3.2.3 NPs
NPs are a novel drug delivery system that are actually defined as “solid colloidal particles”. The particle size generally ranges from 10–50 nm, with an upper limit of about 1,000 nm (Mora-Huertas et al., 2010; Petros and DeSimone, 2010). NPs can load a wide range of drugs, including proteins, hydrophobic drugs, hydrophilic drugs, vaccines, and biomacromolecules. Through formulation design, NPs can achieve targeted drug delivery to organs such as the lymphatic system, spleen, lungs, brain, and liver, and can prolong the circulation time of drugs in the body (Anwar et al., 2021). NPs provide an ideal choice for the controlled and targeted administration of natural products and have attracted great interest from researchers.
3.2.3.1 Polymeric NPs
Polymeric NPs are colloidal systems composed of natural, synthetic, or semi-synthetic polymers (Van Vlerken et al., 2007). Compared to inorganic NPs, polymeric NPs typically exhibit good biocompatibility, stability, processability, and responsiveness to external stimuli (Sarcan et al., 2018). The polymer serves as the backbone of polymeric NPs and is considered the foundation of their composition. Therefore, researchers must understand the characteristics of polymers, such as biocompatibility, biodegradability, stability, permeability, and the interaction between drugs and polymers, in order to select appropriate formulations. Additionally, the properties of the formulation system can be modulated through chemical modifications, the addition of targeting molecules, the incorporation of lipids, and other methods to achieve the desired objectives of the researchers (Kumari et al., 2010).
Polymers can be classified into natural polymers and synthetic polymers based on their source. Numerous studies have reported the use of synthetic polymers for the preparation of polymeric NPs. Commonly used synthetic polymers include polylactic acid (PLA), poly (lactic-co-glycolic acid) (PLGA), and poly-ε-caprolactone (PCL). For example, Rathinavel et al. (2021) prepared polymeric NPs loaded with CUR using PCL to enhance the antibacterial effects against both gram-positive and gram-negative strains. Thuy et al. (Kang et al., 2022) used PAMAM to prepare co-loaded NPs of PTX and CUR, achieving improved bioavailability and enhanced anticancer activity against skin cancer. Kumar et al. (2014) prepared methacrylate-based NPs for CUR delivery and observed that the drug delivery system enhanced the antitumor activity and significantly reduced G0/G1 cell cycle arrest in tumor cells.
Compared to synthetic polymers, natural polymers have gained widepraed attention due to their high biocompatibility, biodegradability, stability, and cost-effectiveness (Sabra et al., 2019). Commonly used natural polymers include chitosan, alginate, and gelatin. Chitosan is a cationic alkaline polysaccharide that exhibits good biocompatibility and biodegradability and contains a large number of functional groups suitable for chemical modification. Methods for preparing chitosan NPs include ion gelation (Kalpana et al., 2010), microemulsion (Wang et al., 2008), and emulsion solvent diffusion (El-Shabouri, 2002). Rahmati et al. (2021) studied the preparation of BBR-loaded alginate/chitosan gel and evaluated its therapeutic efficacy in a rat sciatic nerve crush injury model. MTT assays confirmed the cell compatibility of the gel and demonstrated the dose-dependent effect of BBR on cell proliferation. In vivo experiments showed that the hydrogel containing 1% BBR had a positive effect on rat sciatic nerve regeneration. Dogan investigated the potential cytotoxic effects of quercetin (QUE) and QUE-loaded chitosan NPs on SH-SY5Y cells. After treatment with different concentrations of QUE (0.5, 1, 2, 4, 8 μg/mL) for 24 h, cell viability was determined using the XTT assay. The results showed that QUE-loaded chitosan NPs induced significant cytotoxicity in SH-SY5Y cells through the generation of oxidative stress and DNA damage (Dogan, 2022).
Alginate is an anionic water-soluble natural polymer with biodegradable, biocompatible, and adhesive properties. Its adhesiveness is mainly attributed to strong hydrogen bonding formed through hydroxyl and carboxyl groups interacting with adhesive glycoproteins (Nair and Laurencin, 2007). Methods for preparing alginate NPs include ion gelation, covalent cross-linking, emulsion solvent displacement, and emulsion-solvent evaporation. Ion gelation is a widely used technique for preparing alginate NPs(Damelin et al., 2015), as alginate has an affinity for multivalent cations such as Ca2+ and Zn2+(Draget et al., 1997). Ahmady et al. (2023) developed a drug delivery system based on alginate. First, alginate NPs loaded with capsaicin were prepared using cationic surfactants and nano-emulsions. The particle size of these NPs was 19.42 ± 11.8 nm, with an encapsulation efficiency of 98.7% ± 0.6%. Subsequently, poly (ε-caprolactone)-chitosan co-blended nanofibers with different mixing ratios were fabricated using electrospinning. The nanofibers with the most favorable characterization results were chosen to encapsulate the alginate NPs, resulting in a drug delivery system consisting of nanoparticle-nanofiber composites. In vitro analysis demonstrated the effective inhibition of MCF-7 human breast cancer cell proliferation by the designed nanoplatform, while it exhibited no toxicity toward human dermal fibroblasts (HDF).
Gelatin is a protein obtained by partially hydrolyzing collagen to convert it into a non-oriented protein. Based on the pH at which collagen is hydrolyzed, gelatin can be divided into two types: gelatin A (isoelectric point of 9), where collagen is hydrolyzed under acidic conditions, and gelatin B (isoelectric point of 5), where collagen is hydrolyzed under alkaline conditions. Since gelatin is water-soluble, cross-linking of gelatin may be required in the development of NPs(Lin et al., 2009; Elmowafy et al., 2023). Tumor-responsive nanocarriers are highly valuable and in demand for smart anticancer drug delivery. For this reason, Zhou et al. (2020) designed redox- and MMP-2-sensitive NPs for the delivery of PTX. Bovine serum albumin was used as the targeting ligand, and the disulfide-containing prodrug (PTX-SS-COOH) was grafted onto sulfhydryl-modified gelatin as the hydrophilic carrier. The sulfhydryl groups on gelatin can self-cross-link in air to form disulfide bonds, thus giving the NPs a stable structure. Because of their sensitivty to changes in MMP-2 concentration and redox potential, the NPs achieved multi-responsive drug delivery to the tumor microenvironment and showed excellent anti-cancer efficacy in further in vitro and in vivo experiments.
3.2.3.2 Inorganic NPs
The nanoscale synthesis of inorganic materials has led to significant changes in biology and medicine. With their nanosize and abundance of atoms on their surfaces, inorganic NPs can exhibit properties such as magnetism, conductivity, radioactivity, and light (heat) responsiveness (Auffan et al., 2009). Based on these properties, inorganic NPs are increasingly used in the field of biomedicine for applications such as therapy, diagnosis, biosensors, and material component modules (Ni et al., 2017; Hess et al., 2019; Luther et al., 2020; Mitchell et al., 2021). The most commonly used inorganic materials include pure metals (e.g., gold and silver), metal oxides (e.g., mesoporous silica and γ-Fe2O3/Fe3O4), semiconductor materials, and calcium phosphate.
Compared to other inorganic NPs, noble metal (e.g., Au, Ag, Pt, Hg, and Cu) NPs are gaining increasing attention from researchers (Ramalingam et al., 2014). Among them, gold NPs are known to be the most stable NPs. They also possess tunable optical properties, which are determined by the surface plasmon resonance effect, involving the oscillation and interaction of electrons between surface negative and positive charges (Ramalingam, 2019). As shown in Figure 4, gold NPs can be synthesized using top-down and bottom-up approaches. However, these methods often face challenges such as the use of chemically toxic reagents with safety risks, complex preparation processes, and the need for improved functionality and biocompatibility (da Silva et al., 2020). For example, common surfactants like cetyltrimethylammonium bromide and reducing agents such as hydrazine hydrate and sodium borohydride, due to their explicit toxicity, must be removed or encapsulated with biocompatible shells during the preparation process (LunáCheung et al., 2012; Kumar et al., 2019). To avoid toxicity risks and explore diverse biomedical applications, the green synthesis of gold NPs based on specific natural compounds is considered an ideal alternative method for improving the preparation process and enhancing the functionality of the final materials. These natural bioactive components also possess inherent pharmacological properties. Some active ingredients, such as CUR (Matur et al., 2020), resveratrol (Wang et al., 2017), and epigallocatechin gallate (EGCG) (Wu et al., 2018) have received increasing attention due to their unique structures and physicochemical characteristics that can confer special functions to materials. For instance, Yao et al. (2022) selected the tetracyclic triterpenoid compound ginsenoside Rh2 from Panax ginseng C.A. Meyer as a reducing agent and stabilizer to react with HAuCl4, resulting in the synthesis of Au@ginsenoside Rh2 NPs. These NPs inherited the excellent anticancer properties of ginsenoside Rh2 and improved its poor water solubility.
3.2.3.3 Bio-NPs
Currently, nanocarriers can be broadly classified into two categories: artificial nanocarriers and natural nanocarriers. Artificial nanocarriers, represented by inorganic NPs, have limitations in drug loading capacity and inevitable systemic toxicity, which to restrict their application as delivery platforms (Chen et al., 2022). The safety concerns associated with using artificially synthesized materials for drug delivery have accelerated the research and application of cell-derived nanovesicles (CDNs). CDNs include naturally secreted extracellular vesicles (e.g., exosomes and microvesicles), stimulus-induced nanovesicles, and lipid-based nanovesicles (Liu et al., 2021). CDNs are typically isolated and purified from culture media and various biological fluids, or they can be produced or modified from various cells, bacteria, fungi, or even whole plants. Currently, there are three main methods for preparing CDNs. The first method involves the separation and purification of naturally secreted CDNs using techniques such as differential ultracentrifugation (DUC), density gradient ultracentrifugation (DGUC), and ultrafiltration (UF) (Li et al., 2017). The second method involves the application of exogenous stimuli to host cells to enhance the biogenesis of CDNs, which is a feasible approach for increasing CDN production while maintaining the major characteristics of the cell membrane (Zou et al., 2019). The last method involves the extraction of bio-lipids from cells and the reconstruction of CDNs in vitro (Yang et al., 2018).
As particles that exist naturally in the environment, CDNs possess almost all the advantages of artificial nanocarriers, and they also exhibit biocompatibility and biosafety (Johnsen et al., 2018). Through optimized separation, detailed characterization, and appropriate functionalization, CDNs have been successfully prepared for delivering plant chemicals. In the field of TCM, CDNs have been studied for delivering active ingredients such as CUR, RES, QUE, triptolide, and BBR. Table 2 summarizes the reports on the delivery of herbal ingredients using CDNs.
The application of CDNs for delivering active ingredients also faces some challenges. The primary issue is the limited efficiency in obtaining CDNs, especially in regard to large-scale and highly selective separation and preparation of CDNs from complex media, which requires further research. Additionally, the functional modifications carried out on CDNs to achieve therapeutic goals may compromise their structural integrity, reduce drug loading capacity, and alter the in vivo distribution of the drugs (Chen et al., 2022). Therefore, the selection and optimization of CDN-based drug delivery systems require further consideration and improvement.
3.2.4 Dendrimers
Dendrimers are large molecules with a dendritic structure, that consist of oligomers that are repeatedly and linearly linked by branching units. As the number of polymerization generations increases, the degree of branching of the molecules continues to expand, eventually leading to the formation of a closed three-dimensional spherical structure (Abbasi et al., 2014). The number of branching points (also known as focal points) from the central core to the surface is referred to as “generation” (Tomalia, 2005). For instance, dendrimers with 5 branching points are called the “fifth generation” and are denoted as “G5-dendrimer”. Therefore, the fifth generation PAMAM dendrimer is referred to as G5-PAMAM.
Dendrimers possess controllable physicochemical properties, enriched active functional groups, and internal cavity structures, making them promising drug delivery carriers (Madaan et al., 2014). There are two approaches for dendrimer-based drug delivery. One is through non-covalent interactions, where the drug molecules are encapsulated within the internal cavities of dendrimers, providing protection against metabolic processes and enhancing the bioavailability of the drugs. The other approach involves covalent interactions, where the drug molecules are covalently linked to the dendrimers using cleavable functional groups such as esters and amines, enabling effective drug release and controlled release of the drugs (Chis et al., 2020).
Compared to more mature technologies such as liposomes, NPs, and micelles, dendrimers have a relatively late start in the field of drug delivery. Currently, one of the most successful companies utilizing dendrimers as drug delivery platforms is Starpharma from Australia. Their DEP® platform has advanced multiple drugs to clinical stages (Kelly et al., 2020). In the field of TCM, there have been reports on the use of dendrimers for the delivery of active ingredients such as MT (Alibolandi et al., 2017), PTX (Bhatt et al., 2019), BBR(Yadav et al., 2023), QUE (Madaan et al., 2016), ellagic acid (Priyadarshi et al., 2021), RES (Pentek et al., 2017), and CUR (Gamage et al., 2016).
3.3 Carrier-free nanomedicines
Currently, the development of nanocrystallization of single active ingredients has to some extent overlooked the compatibility of TCM formulas. This is because clinically used TCM contain various components with different physicochemical properties. Therefore, it is necessary to explore multi-component nanomedicines. The theory of TCM compatibility refers to the combination of various herbs, reflecting the synergistic effects of different components. Utilizing multiple active ingredients through nanotechnology is an effective strategy. Some structurally ideal active ingredients can be utilized as carriers, while some active ingredients can act simultaneously as carriers and drugs, fully exploiting their physicochemical properties and pharmacological actions. Various nanostructures formed by self-assembly of active ingredients of TCM are referred to as carrier-free nanomedicines (Li L. et al., 2020).
3.3.1 Self-assembled nanomedicines
Carrier-free self-assembled nanomedicines of TCM refer to the formation of stable and specific structures through non-covalent interactions such as hydrogen bonding, van der Waals forces, π-π stacking, electrostatic interactions, and coordination bonds between active ingredients of TCM(Tian et al., 2020). Studies have found that the structural diversity of herbal ingredients enables their self-assembly capabilities, allowing them to assemble with other molecules through non-electrostatic interactions. The preparation of self-assembled nanomedicines is simple and allows for high drug loading, while achieving highly stable drug delivery without the use of carriers (Figure 5) (Zhi et al., 2020). Currently, common ingredients of TCM with self-assembly properties include terpenoids, glycosides (Mao et al., 2022), and quinones (Wu et al., 2022). These natural molecules can self-assemble at the interface of different solvents to form carrier-free nanomedicines.
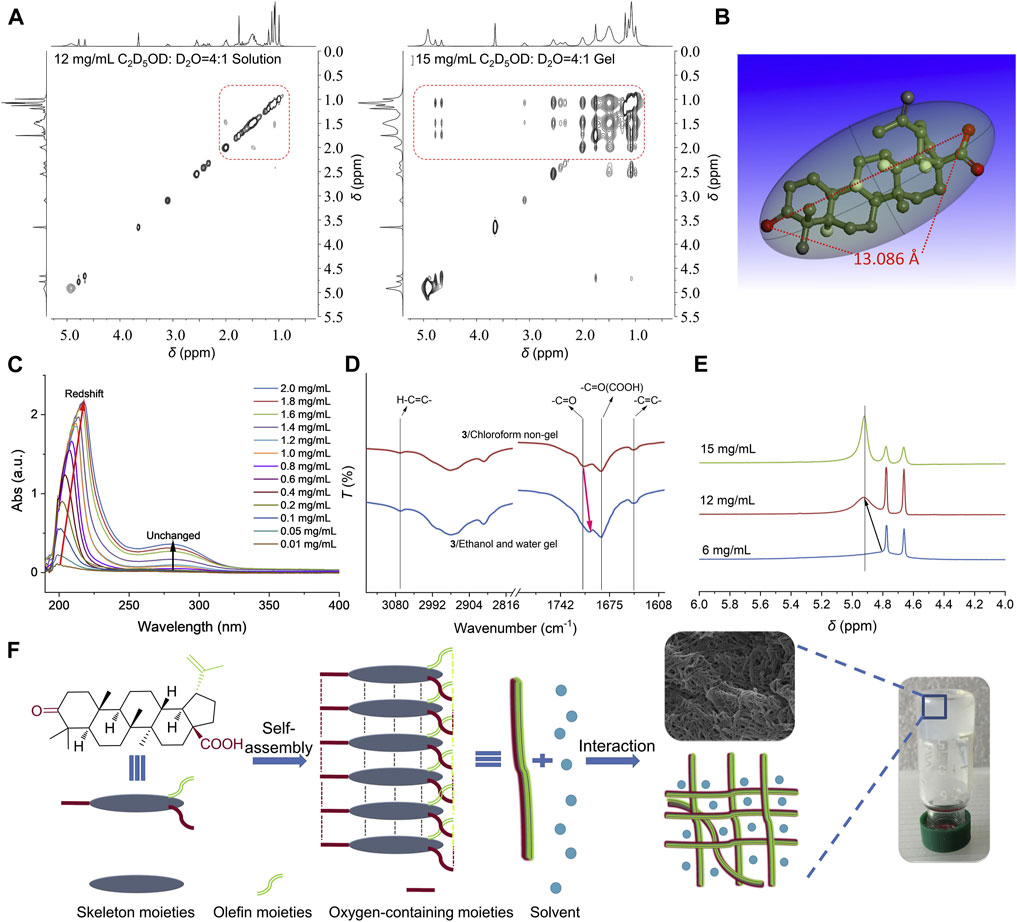
Figure 5. Formation of injectable NPG scaffold. (A) 2D NOESY spectra of compound 3 in mixed solvent of deuterated ethanol and deuterated water (4:1) at different concentrations. (B) Molecular length of compound 3. (C) UV spectra of compound 3 in ethanol/water mixed solvent (1:1) at different concentrations. (D) IR spectra of compound 3 obtained from non-gel and gel. (E) 1H NMR spectra of compound 3 in mixed solvent of deuterated ethanol and deuterated water (4:1) at different concentrations. (F) A possible self-assembly formation process of NPG. Reprinted from Acta Pharmaceutica Sinica B, K. Zhi, J. Wang, H. Zhao, X. Yang, Self-assembled small molecule natural product gel for drug delivery: a breakthrough in new application of small molecule natural products, 913-927, Copyright (2020), with permission from Elsevier [OR APPLICABLE SOCIETY COPYRIGHT OWNER].
Ginsenosides, the main active ingredients of P. ginseng C.A., belong to triterpenoid saponins. In order to avoid potential issues associated with poor biocompatibility, low drug loading capacity, and unpredictable side effects associated with drug carriers, Tan et al. (2022) utilized the intermolecular recognition of different ginsenoside monomers to achieve self-assembled carrier-free ginsenosides nano-micelles (GSN). These self-assembled micelles exhibited a lamellar structure with a uniform particle size distribution. The molecular interactions between ginsenosides were preliminarily studied using Discovery Studio 4.0 (DS 4.0). The results demonstrated that the formation of GSN was driven by alkyl-alkyl interactions and hydrogen bonding. Additionally, GSN effectively inhibited tumor cell adhesion activity and the expression of intercellular adhesion molecule-1 (ICAM-1). Importantly, in an in vivo H22 mouse artificial lung metastasis model, the self-assembled system significantly inhibited tumor metastasis. These results suggest that this carrier-free nanomedicine has potential for the treatment of tumor metastasis.
Rhein is an anthraquinone derivative and is present in Rheum palmatum L. In the absence of carrier materials, Wu et al. (2021) utilized hydrogen bonding and π-π stacking interactions as driving forces to self-assemble rhein and DOX into a mitochondria-targeting nanogel. This nanogel achieved 100% drug loading and not only enabled sustained controlled release but also overcame the drawbacks associated with the use of free DOX and free rhein, such as high toxicity, poor target specificity, low solubility, and low bioavailability. In the tumor environment, the rhein-DOX nanogel was taken up by HepG2 cells and delivered to the mitochondria. Subsequently, rhein and DOX were released from the fibrous structure. The rhein-DOX nanogel significantly increased intracellular reactive oxygen species (ROS) levels, decreased mitochondrial membrane potential (MMP), and further induced cell apoptosis. These results demonstrated the synergistic effect of rhein and DOX in the treatment of liver cancer.
Ursolic acid is a pentacyclic triterpenoid compound. Fan et al. (2018) designed a carrier-free nanomedicine based on the self-assembly of ursolic acid molecules. This process relies on hydrogen bonding and hydrophobic interactions between ursolic acid molecules, resulting in stable NPs with a particle size of 100–200 nm and a high drug loading capacity of up to 60%. Compared to free ursolic acid, these nanomaterials significantly inhibit cancer cell proliferation and induce apoptosis. In vivo studies, the nanomaterial significantly inhibited tumor growth and protected the liver in A549 xenograft mouse models. This carrier-free nanomedicine platform represents a strategy to enhance the anticancer effects of poorly soluble drugs.
3.3.2 Pharmaceutical cocrystals
Pharmaceutical cocrystals refer to multicomponent molecular crystals formed by two or more drug molecules through hydrogen bonding or other non-covalent interactions, with at least one molecule being an active pharmaceutical ingredient (API) and the other being a co-former (Kuminek et al., 2016; Gu et al., 2022). Traditional co-formers are often composed of safe pharmaceutical excipients, but there are now drug cocrystals composed solely of active drug substances that have been used in clinical applications. These drug cocrystals retain the advantages of individual components while exhibiting synergistic effects in terms of pharmacological activity. For example, the sacubitril valsartan sodium cocrystal has been used in the clinical treatment of heart failure (McCormack, 2016).
In the field of TCM, non-dissociating and weakly dissociating active ingredients, such as flavonoids, alkaloids, terpenoids, and polyphenols, can form cocrystal structures through intermolecular interactions like hydrogen bonding (Heng et al., 2022). In particular, due to the competitive hydrogen bonding sites within the molecular framework of flavonoids, they readily form cocrystals with co-formers that also contain hydrogen bond acceptors and donors. Currently, a series of cocrystals formed between flavonoid compounds and excipients, such as caffeine, isoniazid, nicotinamide, acetamide, betaine, and theophylline have emerged. These cocrystals exhibit good solubility, dissolution, and oral bioavailability. For instance, Luo et al. (2019) synthesized a cocrystal of luteolin with isoniazid and caffeine using liquid-assisted grinding. The solubility of the luteolin-isoniazid cocrystal was 112.3 μg/mL, approximately three times greater than that of free luteolin. According to the pharmacokinetic analysis, compared with those of free luteolin, the AUC0-∞ of the luteolin-isoniazid cocrystal and luteolin-caffeine cocrystal were 2.7-fold and 1.4-fold greater, respectively.
3.3.3 Pickering emulsion
Pickering emulsion is a type of emulsion in which solid particles act as stabilizers and adsorb onto the surface of liquid droplets. This structural uniqueness endows the material with excellent stability, biocompatibility and environmental friendliness (Ni et al., 2022). The use of Pickering emulsion can significantly improve the oral bioavailability of poorly soluble drugs such as CUR, silybin, puerarin, and rutin (Tai et al., 2020). In terms of drug delivery systems, lipophilic components can be loaded into the oil phase, hydrophilic drugs can be loaded into the aqueous phase, and amphiphilic drugs can be loaded at the oil-water interface. Additionally, Pickering emulsion can be used as precursors for preparing other dosage forms or carriers, such as nano-composite materials, magnetic solid microspheres, and hollow microcapsules (Nypelö et al., 2014).
3.3.4 Nanosized aggregates in decoction
Decoction is the main form of clinical application of TCM(Weng et al., 2019). The decoction with water as the solvent contains complex active ingredients, and it is speculated that hydrophobic compounds may be modified to increase their solubility (Kim and Park, 2017). Due to the encapsulation of hydrophobic components by amphiphilic polysaccharides or proteins, as well as the interaction between acidic and alkaline compounds, new composites may be formed during the decoction process of TCM(Zhou et al., 2019).
Liu et al. successfully isolated and characterized nanosized aggregates from Bai-Hu Tang, which exhibit excellent performance in antipyretic properties (Lü et al., 2018). Zhuang et al. found that the nanosized aggregates of Xue-Fu-Zhu-Yu Tang during boiling are closely related to its protective effect on cardiovascular system (Zhuang et al., 2008). In addition, Zhou et al. extracted colloidal NPs from Ma-Xing-Shi-Gan Tang, which were formed by hydrophobic or ionic interactions between amphiphilic molecules such as ephedrine and pseudoephedrine (Zhou et al., 2014). In summary, substances such as nanosized aggregates have played a crucial role in TCM decoctions, which has been proven through nanotechnology. This discovery not only provides ideas for understanding the mechanism of TCM, but also provides valuable reference for the development of innovative dosage forms (Zheng et al., 2022).
4 The safety of nano-TCM
Nano-based drug delivery systems hold promise for traversing biological barriers, including cell membranes and even the BBB(Cox et al., 2018; Tosi et al., 2020). However, concerns regarding their potential toxicity are also increasing. The toxicity mechanism of nano-TCM is relatively complex, which is not only related to the toxic components contained in the drug, but also to factors such as drug metabolism and elimination in the body. The advantages of improved solubility and enhanced targeting provided by nanomedicines can reduce or eliminate the toxic effects of active ingredients of TCM. TP has remarkable efficacy in anti-tumor and anti-autoimmune effects, but it is accompanied by serious adverse effects, such as toxic effects on multiple organs (liver, kidney, heart and reproductive system) (Ma et al., 2015; Wang et al., 2019). In addition, clinical applications are limited due to its poor water solubility. To overcome these problems, researchers developed a transferrin-modified TP liposome (TF-TP@LIP). This modification significantly enhances the liposome’s ability to target tumors and reduces the accumulation of the drug in non-target tissues and organs, thereby reducing drug toxicity and adverse effects (Zhao et al., 2023).
In addition, the interaction between active ingredients of TCM can also produce a detoxification effect. Licorice, the dried root and rhizome of Glycyrrhiza uralensis Fisch., Glycyrrhiza inflata Bat., or Glycyrrhiza glabra L., is an “essential herbal medicine” in TCM. It can reduce toxicity and improve efficacy in the combination application of certain herbs. Jiang et al. conducted a study on 124 bibliographies published from 1976 to 2019 and found that the interaction between licorice and toxic compounds, as well as the influence of licorice on the metabolism of toxic compounds, are the main mechanisms by which licorice plays a role in TCM formulas (Jiang et al., 2020). Euodiae Fructus (EF) is a commonly used herb with mild toxicity in clinic. Zhang et al. found that licorice processing can significantly reduce the hepatotoxicity of EF. The detoxification mechanism may be related to the antagonistic effect of licorice on toxic components (Zhang et al., 2021).
5 Challenges and opportunities
The discovery of famous drugs such as ART and BBR usually follows the concepts and strategies of Western medicine, which involve developing new drugs using isolated single natural products (You et al., 2022). This is one way in which TCM has contributed to the development of global medicine. However, there are obvious limitations to this research approach as it lacks the guidance of TCM theory. TCM involves the individual regulation of multiple components and targets, allowing the body to transition from an abnormal state to a normal state. This characteristic makes it difficult to replicate and conduct large-scale clinical trials, thus making it challenging to obtain statistically significant results (You et al., 2022). Through genomics, transcriptomics, proteomics, metabolomics and combined omics analysis, we are able to gain a more comprehensive understanding of the interactions between TCM and biological system. Due to the complexity and multi-component nature of TCM prescriptions, the application of such research techniques can not only provide a deeper understanding of drug mechanisms but also enhance the knowledge of TCM principles, so that the embedded ancient wisdom can be reinterpreted and utilized through the lens of modern science.
6 Conclusion
Nanotechnology, as a field with tremendous potential, has brought new momentum and confidence to the modernization of TCM. On the one hand, relying on advantages such as improving bioavailability, achieving controllable release, and enhancing targeted effects, developing single active ingredients into nanomedicines is considered an innovative strategy for the development of new drugs. On the other hand, interpreting the mechanism of compound prescriptions of TCM is crucial for the modernization of TCM. Exploring the substance basis of TCM formulas from the perspective of nanotechnology can provide strong support for elucidating the scientific intension of TCM formulas. In addition, exploring the physiological and biochemical responses of nano-TCM in human body with the help of omics technology is of great value in elucidating the pharmacological mechanism and targets of herbal medicines. It is worth emphasizing that the continuous integration of TCM with modern scientific principles and technologies will continue to serve the promotion of human health.
Author contributions
Y-BZ: Conceptualization, Investigation, Methodology, Resources, Software, Writing–original draft. J-FW: Conceptualization, Investigation, Methodology, Software, Writing–review and editing. M-XW: Conceptualization, Data curation, Investigation, Methodology, Supervision, Visualization, Writing–review and editing. JP: Conceptualization, Investigation, Software, Writing–review and editing. X-DK: Data curation, Formal Analysis, Methodology, Writing–review and editing. JT: Conceptualization, Funding acquisition, Investigation, Writing–review and editing.
Funding
The author(s) declare that financial support was received for the research, authorship, and/or publication of this article. This work was supported by the key research and development program of Jining (2023YXNS236).
Conflict of interest
The authors declare that the research was conducted in the absence of any commercial or financial relationships that could be construed as a potential conflict of interest.
Publisher’s note
All claims expressed in this article are solely those of the authors and do not necessarily represent those of their affiliated organizations, or those of the publisher, the editors and the reviewers. Any product that may be evaluated in this article, or claim that may be made by its manufacturer, is not guaranteed or endorsed by the publisher.
References
Abbasi, E., Aval, S. F., Akbarzadeh, A., Milani, M., Nasrabadi, H. T., Joo, S. W., et al. (2014). Dendrimers: synthesis, applications, and properties. Nanoscale Res. Lett. 9 (1), 247. doi:10.1186/1556-276X-9-247
Agwa, M. M., and Sabra, S. (2021). Lactoferrin coated or conjugated nanomaterials as an active targeting approach in nanomedicine. Int. J. Biol. Macromol. 167, 1527–1543. doi:10.1016/j.ijbiomac.2020.11.107
Ahmady, A. R., Solouk, A., Saber-Samandari, S., Akbari, S., Ghanbari, H., and Brycki, B. E. (2023). Capsaicin-loaded alginate nanoparticles embedded polycaprolactone-chitosan nanofibers as a controlled drug delivery nanoplatform for anticancer activity. J. Colloid Interface Sci. 638, 616–628. doi:10.1016/j.jcis.2023.01.139
Akbarzadeh, A., Rezaei-Sadabady, R., Davaran, S., Joo, S. W., Zarghami, N., Hanifehpour, Y., et al. (2013). Liposome: classification, preparation, and applications. Nanoscale Res. Lett. 8 (1), 102. doi:10.1186/1556-276X-8-102
Alibolandi, M., Taghdisi, S. M., Ramezani, P., Shamili, F. H., Farzad, S. A., Abnous, K., et al. (2017). Smart AS1411-aptamer conjugated pegylated PAMAM dendrimer for the superior delivery of camptothecin to colon adenocarcinoma in vitro and in vivo. Int. J. Pharm. 519 (1-2), 352–364. doi:10.1016/j.ijpharm.2017.01.044
Anirudhan, T. S., Manjusha, V., and Chithra Sekhar, V. (2021). A new biodegradable nano cellulose-based drug delivery system for pH-controlled delivery of curcumin. Int. J. Biol. Macromol. 183, 2044–2054. doi:10.1016/j.ijbiomac.2021.06.010
Anwar, M., Muhammad, F., and Akhtar, B. (2021). Biodegradable nanoparticles as drug delivery devices. J. Drug Deliv. Sci. Technol. 64, 102638. doi:10.1016/j.jddst.2021.102638
Aqil, F., Kausar, H., Agrawal, A. K., Jeyabalan, J., Kyakulaga, A. H., Munagala, R., et al. (2016). Exosomal formulation enhances therapeutic response of celastrol against lung cancer. Exp. Mol. Pathol. 101 (1), 12–21. doi:10.1016/j.yexmp.2016.05.013
Aqil, F., Munagala, R., Jeyabalan, J., Agrawal, A. K., and Gupta, R. (2017). Exosomes for the enhanced tissue bioavailability and efficacy of curcumin. AAPS J. 19 (6), 1691–1702. doi:10.1208/s12248-017-0154-9
Auffan, M., Rose, J., Bottero, J.-Y., Lowry, G. V., Jolivet, J.-P., and Wiesner, M. R. (2009). Towards a definition of inorganic nanoparticles from an environmental, health and safety perspective. Nat. Nanotechnol. 4 (10), 634–641. doi:10.1038/nnano.2009.242
Bangham, A., De Gier, J., and Greville, G. (1967). Osmotic properties and water permeability of phospholipid liquid crystals. Chem. Phys. lipids 1 (3), 225–246. doi:10.1016/0009-3084(67)90030-8
Bangham, A. D., and Horne, R. (1964). Negative staining of phospholipids and their structural modification by surface-active agents as observed in the electron microscope. J. Liposome Res. 8 (5), 660–668. doi:10.1016/s0022-2836(64)80115-7
Bhatt, H., Kiran Rompicharla, S. V., Ghosh, B., Torchilin, V., and Biswas, S. (2019). Transferrin/α-tocopherol modified poly (amidoamine) dendrimers for improved tumor targeting and anticancer activity of paclitaxel. Nanomedicine 14 (24), 3159–3176. doi:10.2217/nnm-2019-0128
Bian, Z., Yu, Y., Quan, C., Guan, R., Jin, Y., Wu, J., et al. (2015). RPL13A as a reference gene for normalizing mRNA transcription of ovarian cancer cells with paclitaxel and 10-hydroxycamptothecin treatments. Mol. Med. Rep. 11 (4), 3188–3194. doi:10.3892/mmr.2014.3108
Bonifácio, B. V., Silva, P. B., Ramos, M. A., Negri, K. M., Bauab, T. M., and Chorilli, M. (2014). Nanotechnology-based drug delivery systems and herbal medicines: a review. Int. J. Nanomed. 9, 1–15. doi:10.2147/ijn.S52634
Chen, C., Wang, J., Sun, M., Li, J., and Wang, H.-M. D. (2022). Toward the next-generation phyto-nanomedicines: cell-derived nanovesicles (CDNs) for natural product delivery. Biomed. Pharmacother. 145, 112416. doi:10.1016/j.biopha.2021.112416
Chen, G., Hao, B., Ju, D., Liu, M., Zhao, H., Du, Z., et al. (2015). Pharmacokinetic and pharmacodynamic study of triptolide-loaded liposome hydrogel patch under microneedles on rats with collagen-induced arthritis. Acta Pharm. Sin. B 5 (6), 569–576. doi:10.1016/j.apsb.2015.09.006
Chen, S.-R., Dai, Y., Zhao, J., Lin, L., Wang, Y., and Wang, Y. (2018). A mechanistic overview of triptolide and celastrol, natural products from Tripterygium wilfordii Hook F. Front. Pharmacol. 9, 104. doi:10.3389/fphar.2018.00104
Chen, X., He, H., Guo, X., Hou, M., Zhang, X., Li, S., et al. (2023). Calcium orthophosphate in liposomes for Co-delivery of doxorubicin hydrochloride/paclitaxel in breast cancer. Mol. Pharm. 20 (8), 3914–3924. doi:10.1021/acs.molpharmaceut.3c00015
Chen, Y., Sun, Y., Zhao, Q., Liu, C., and Wang, C. (2021). Shenmai injection enhances cisplatin-induced apoptosis through regulation of Mfn2-dependent mitochondrial dynamics in lung adenocarcinoma A549/DDP cells. Cancer Drug Resist. 4 (4), 1047–1060. doi:10.20517/cdr.2021.94
Chis, A. A., Dobrea, C., Morgovan, C., Arseniu, A. M., Rus, L. L., Butuca, A., et al. (2020). Applications and limitations of dendrimers in biomedicine. Molecules 25 (17), 3982. doi:10.3390/molecules25173982
Cox, A., Andreozzi, P., Dal Magro, R., Fiordaliso, F., Corbelli, A., Talamini, L., et al. (2018). Evolution of nanoparticle protein corona across the blood–brain barrier. ACS Nano 12 (7), 7292–7300. doi:10.1021/acsnano.8b03500
Damelin, L. H., Fernandes, M. A., and Tiemessen, C. T. (2015). Alginate microbead-encapsulated silver complexes for selective delivery of broad-spectrum silver-based microbicides. Int. J. Antimicrob. Agents. 46 (4), 394–400. doi:10.1016/j.ijantimicag.2015.05.016
da Silva, A. B., Rufato, K. B., de Oliveira, A. C., Souza, P. R., da Silva, E. P., Muniz, E. C., et al. (2020). Composite materials based on chitosan/gold nanoparticles: from synthesis to biomedical applications. Int. J. Biol. Macromol. 161, 977–998. doi:10.1016/j.ijbiomac.2020.06.113
Dogan, M. (2022). Assessment of mechanism involved in the apoptotic and anti-cancer activity of Quercetin and Quercetin-loaded chitosan nanoparticles. Med. Oncol. 39 (11), 176. doi:10.1007/s12032-022-01820-x
Dong, M., Wu, S., Xu, H., Yu, X., Wang, L., Bai, H., et al. (2021). Fbs-derived exosomes as a natural nano-scale carrier for icariin promote osteoblast proliferation. Front. Bioeng. Biotech. 9, 615920. doi:10.3389/fbioe.2021.615920
Draget, K. I., Skjåk-Bræk, G., and Smidsrød, O. (1997). Alginate based new materials. Int. J. Biol. Macromol. 21 (1-2), 47–55. doi:10.1016/s0141-8130(97)00040-8
Elmowafy, M., Shalaby, K., Elkomy, M. H., Alsaidan, O. A., Gomaa, H. A., Abdelgawad, M. A., et al. (2023). Polymeric nanoparticles for delivery of natural bioactive agents: recent advances and challenges. Polymers 15 (5), 1123. doi:10.3390/polym15051123
El-Shabouri, M. H. (2002). Positively charged nanoparticles for improving the oral bioavailability of cyclosporin-A. Int. J. Pharm. 249 (1-2), 101–108. doi:10.1016/s0378-5173(02)00461-1
Fan, L., Zhang, B., Xu, A., Shen, Z., Guo, Y., Zhao, R., et al. (2018). Carrier-free, pure nanodrug formed by the self-assembly of an anticancer drug for cancer immune therapy. Mol. Pharm. 15 (6), 2466–2478. doi:10.1021/acs.molpharmaceut.8b00444
Gamage, N., Jing, L., Worsham, M., and Ali, M. M. (2016). Targeted theranostic approach for glioma using dendrimer-based curcumin nanoparticle. J. Nanomed. Nanotechnol. 7 (4), 393. doi:10.4172/2157-7439.1000393
Gao, Z. S., Zhang, C. J., Xia, N., Tian, H., Li, D. Y., Lin, J. Q., et al. (2021). Berberine-loaded M2 macrophage-derived exosomes for spinal cord injury therapy. Acta Biomater. 126, 211–223. doi:10.1016/j.actbio.2021.03.018
Gu, W., Liu, D., and Sun, J. (2022). Co-crystallization of curcumin for improved photodynamic inactivation of Vibrio parahaemolyticus and its application for the preservation of cooked clams. Int. J. Food Microbiol. 378, 109816. doi:10.1016/j.ijfoodmicro.2022.109816
Guo, C., Su, Y., Wang, H., Cao, M., Diao, N., Liu, Z., et al. (2022). A novel saponin liposomes based on the couplet medicines of Platycodon grandiflorum–Glycyrrhiza uralensis for targeting lung cancer. Drug Deliv. 29 (1), 2743–2750. doi:10.1080/10717544.2022.2112997
Guo, Z., Li, S., Lv, M., Liu, Z., and Xue, W. (2017). Redox-responsive biodegradable polycation poly (amido amine) used as intranasal vaccine delivery systems. ACS Biomater. Sci. Eng. 3 (10), 2420–2430. doi:10.1021/acsbiomaterials.7b00538
Gupta, A., Costa, A. P., Xu, X., Lee, S.-L., Cruz, C. N., Bao, Q., et al. (2020). Formulation and characterization of curcumin loaded polymeric micelles produced via continuous processing. Int. J. Pharm. 583, 119340. doi:10.1016/j.ijpharm.2020.119340
Han, Y., Chu, X., Cui, L., Fu, S., Gao, C., Li, Y., et al. (2020). Neuronal mitochondria-targeted therapy for Alzheimer’s disease by systemic delivery of resveratrol using dual-modified novel biomimetic nanosystems. Drug Deliv. 27 (1), 502–518. doi:10.1080/10717544.2020.1745328
He, D., Xu, X., Li, L., Chen, C., Gong, K., Guo, Q., et al. (2021). Functional exosome-mediated delivery of triptolide endowed with targeting properties as chemotherapy carriers for ovarian carcinoma. J. Biomed. Nanotechnol. 17 (3), 426–438. doi:10.1166/jbn.2021.3041
He, H., Lu, Y., Qi, J., Zhu, Q., Chen, Z., and Wu, W. (2019). Adapting liposomes for oral drug delivery. Acta Pharm. Sin. B 9 (1), 36–48. doi:10.1016/j.apsb.2018.06.005
Heng, W., He, X., Song, Y., Han, J., Pang, Z., Qian, S., et al. (2022). Insights into cocrystallization and coamorphization engineering techniques in the delivery of traditional Chinese medicine: formation mechanism, solid-state characterization, and improved pharmaceutical properties. Cryst. Growth Des. 22 (8), 5110–5134. doi:10.1021/acs.cgd.1c01352
Hess, K. L., Medintz, I. L., and Jewell, C. M. (2019). Designing inorganic nanomaterials for vaccines and immunotherapies. Nano Today 27, 73–98. doi:10.1016/j.nantod.2019.04.005
Hibino, M., Tanaka, K., Ouchi, M., and Terashima, T. (2021). Amphiphilic random-block copolymer micelles in water: precise and dynamic self-assembly controlled by random copolymer association. Macromolecules 55 (1), 178–189. doi:10.1021/acs.macromol.1c02186
Hong, W., Guo, F., Yu, N., Ying, S., Lou, B., Wu, J., et al. (2021). A novel folic acid receptor-targeted drug delivery system based on curcumin-loaded β-cyclodextrin nanoparticles for cancer treatment. Drug Des. Dev. Ther. 15, 2843–2855. doi:10.2147/dddt.S320119
Hu, J.-J., Yu, X.-Z., Zhang, S.-Q., Zhang, Y.-X., Chen, X.-L., Long, Z.-J., et al. (2023). Hydrogel with ROS scavenging effect encapsulates BR@Zn-BTB nanoparticles for accelerating diabetic mice wound healing via multimodal therapy. iScience 26 (6), 106775. doi:10.1016/j.isci.2023.106775
Huang, D., Zhuang, Y., Shen, H., Yang, F., Wang, X., and Wu, D. (2018). Acetal-linked PEGylated paclitaxel prodrugs forming free-paclitaxel-loaded pH-responsive micelles with high drug loading capacity and improved drug delivery. Biomater. Adv. 82, 60–68. doi:10.1016/j.msec.2017.08.063
Jia, G., Han, Y., An, Y., Ding, Y., He, C., Wang, X., et al. (2018). NRP-1 targeted and cargo-loaded exosomes facilitate simultaneous imaging and therapy of glioma in vitro and in vivo. Biomaterials 178, 302–316. doi:10.1016/j.biomaterials.2018.06.029
Jiang, M., Zhao, S., Yang, S., Lin, X., He, X., Wei, X., et al. (2020). An “essential herbal medicine”—licorice: a review of phytochemicals and its effects in combination preparations. J. Ethnopharmacol. 249, 112439. doi:10.1016/j.jep.2019.112439
Johnsen, K. B., Gudbergsson, J. M., Duroux, M., Moos, T., Andresen, T. L., and Simonsen, J. B. (2018). On the use of liposome controls in studies investigating the clinical potential of extracellular vesicle-based drug delivery systems–A commentary. J. Control. Release. 269, 10–14. doi:10.1016/j.jconrel.2017.11.002
Kadota, K., Nogami, S., Uchiyama, H., and Tozuka, Y. (2020). Controlled release behavior of curcumin from kappa-carrageenan gels with flexible texture by the addition of metal chlorides. Food Hydrocoll. 101, 105564. doi:10.1016/j.foodhyd.2019.105564
Kalani, A., Kamat, P. K., Chaturvedi, P., Tyagi, S. C., and Tyagi, N. (2014). Curcumin-primed exosomes mitigate endothelial cell dysfunction during hyperhomocysteinemia. Life Sci. 107 (1-2), 1–7. doi:10.1016/j.lfs.2014.04.018
Kalpana, N., Kumar, S. S., and Nath, M. D. (2010). Chitosan nanoparticles: a promising system in novel drug delivery. Chem. Pharm. Bull. 58 (11), 1423–1430. doi:10.1248/cpb.58.1423
Kang, N., Choi, M., Lee, M., and Choi, J. S. (2022). Dendrimeric micelles composed of polyamidoamine dendrimer-peptide-cholesterol conjugates as drug carriers for the treatment of melanoma and bacterial infection. J. Ind. Eng. Chem. 114, 361–376. doi:10.1016/j.jiec.2022.07.026
Kelly, B. D., McLeod, V., Walker, R., Schreuders, J., Jackson, S., Giannis, M., et al. (2020). Abstract 1716: anticancer activity of the taxane nanoparticles, DEP® docetaxel and DEP® cabazitaxel. Cancer Res. 80 (16_Suppl. ment), 1716. doi:10.1158/1538-7445.am2020-1716
Kim, J.-E., and Park, Y.-J. (2017). High paclitaxel-loaded and tumor cell-targeting hyaluronan-coated nanoemulsions. Colloids Surf. B Biointerfaces. 150, 362–372. doi:10.1016/j.colsurfb.2016.10.050
Kim, M. S., Haney, M. J., Zhao, Y., Mahajan, V., Deygen, I., Klyachko, N. L., et al. (2016). Development of exosome-encapsulated paclitaxel to overcome MDR in cancer cells. Nanomed. Nanotech. Biol. Med. 12 (3), 655–664. doi:10.1016/j.nano.2015.10.012
Kotta, S., Aldawsari, H. M., Badr-Eldin, S. M., Nair, A. B., and Yt, K. (2022). Progress in polymeric micelles for drug delivery applications. Pharmaceutics 14 (8), 1636. doi:10.3390/pharmaceutics14081636
Kumar, A., Das, N., Satija, N. K., Mandrah, K., Roy, S. K., and Rayavarapu, R. G. (2019). A novel approach towards synthesis and characterization of non-cytotoxic gold nanoparticles using taurine as capping agent. Nanomaterials 10 (1), 45. doi:10.3390/nano10010045
Kumar, S. S. D., Surianarayanan, M., Vijayaraghavan, R., Mandal, A. B., and MacFarlane, D. R. (2014). Curcumin loaded poly(2-hydroxyethyl methacrylate) nanoparticles from gelled ionic liquid – in vitro cytotoxicity and anti-cancer activity in SKOV-3 cells. Eur. J. Pharm. Sci. 51, 34–44. doi:10.1016/j.ejps.2013.08.036
Kumari, A., Yadav, S. K., and Yadav, S. C. (2010). Biodegradable polymeric nanoparticles based drug delivery systems. Colloids Surf. B Biointerfaces. 75 (1), 1–18. doi:10.1016/j.colsurfb.2009.09.001
Kuminek, G., Cao, F., Bahia de Oliveira da Rocha, A., Gonçalves Cardoso, S., and Rodríguez-Hornedo, N. (2016). Cocrystals to facilitate delivery of poorly soluble compounds beyond-rule-of-5. Adv. Drug Deliv. Rev. 101, 143–166. doi:10.1016/j.addr.2016.04.022
Lan, Y., Wang, H., Wu, J., and Meng, X. (2022). Cytokine storm-calming property of the isoquinoline alkaloids in Coptis chinensis Franch. Front. Pharmacol. 13, 973587. doi:10.3389/fphar.2022.973587
Li, F.-S., and Weng, J.-K. (2017). Demystifying traditional herbal medicine with modern approach. Nat. Plants 3 (8), 17109. doi:10.1038/nplants.2017.109
Li, J., Yao, Z., Xiong, H., Cui, H., Wang, X., Zheng, W., et al. (2020a). Extracellular vesicles from hydroxycamptothecin primed umbilical cord stem cells enhance anti-adhesion potential for treatment of tendon injury. Stem Cell. Res. Ther. 11 (1), 500. doi:10.1186/s13287-020-02016-8
Li, L., Cui, H., Li, T., Qi, J., Chen, H., Gao, F., et al. (2020b). Synergistic effect of berberine-based Chinese medicine assembled nanostructures on diarrhea-predominant irritable bowel syndrome in vivo. Front. Pharmacol. 11, 1210. doi:10.3389/fphar.2020.01210
Li, P., Kaslan, M., Lee, S. H., Yao, J., and Gao, Z. (2017). Progress in exosome isolation techniques. Theranostics 7 (3), 789–804. doi:10.7150/thno.18133
Li, X., Du, L., Chen, X., Ge, P., Wang, Y., Fu, Y., et al. (2015). Nasal delivery of analgesic ketorolac tromethamine thermo-and ion-sensitive in situ hydrogels. Int. J. Pharm. 489 (1-2), 252–260. doi:10.1016/j.ijpharm.2015.05.009
Lin, M., Meng, S., Zhong, W., Cai, R., Du, Q., and Tomasik, P. (2009). Novel drug-loaded gelatin films and their sustained-release performance. J. Biomed. Mat. Res. Part B Appl. Biomater. 90 (2), 939–944. doi:10.1002/jbm.b.31366
Liu, H., Shen, M., Zhao, D., Ru, D., Duan, Y., Ding, C., et al. (2019a). The effect of triptolide-loaded exosomes on the proliferation and apoptosis of human ovarian cancer SKOV3 cells. Biomed. Res. Int. 2019, 2595801. doi:10.1155/2019/2595801
Liu, J., Lee, H., and Allen, C. (2006). Formulation of drugs in block copolymer micelles: drug loading and release. Curr. Pharm. Des. 12 (36), 4685–4701. doi:10.2174/138161206779026263
Liu, J., Ren, L., Li, S., Li, W., Zheng, X., Yang, Y., et al. (2021). The biology, function, and applications of exosomes in cancer. Acta Pharm. Sin. B 11 (9), 2783–2797. doi:10.1016/j.apsb.2021.01.001
Liu, Y., Jiang, Z., Hou, X., Xie, X., Shi, J., Shen, J., et al. (2019b). Functional lipid polymeric nanoparticles for oral drug delivery: rapid mucus penetration and improved cell entry and cellular transport. Nanomed. Nanotech. Biol. Med. 21, 102075. doi:10.1016/j.nano.2019.102075
Lu, L., Ding, Y., Zhang, Y., Ho, R. J., Zhao, Y., Zhang, T., et al. (2018). Antibody-modified liposomes for tumor-targeting delivery of timosaponin AIII. Int. J. Nanomed. 13, 1927–1944. doi:10.2147/IJN.S153107
Lu, L., Dong, J., and Gong, W. (2021). Advances in basic research of traditional Chinese medicine herbal formulae in the treatment of non-small cell lung cancer. Traditional Med. Mod. Med. 4 (01n04), 9–19. doi:10.1142/s2575900021300010
Lü, S., Su, H., Sun, S., Guo, Y., Liu, T., Ping, Y., et al. (2018). Isolation and characterization of nanometre aggregates from a Bai-Hu-Tang decoction and their antipyretic effect. Sci. Rep. 8 (1), 12209. doi:10.1038/s41598-018-30690-5
LunáCheung, K., PuiáHo, H., KwokáWong, C., KaiáKong, S., et al. (2012). CTAB-coated gold nanorods elicit allergic response through degranulation and cell death in human basophils. Nanoscale 4 (15), 4447–4449. doi:10.1039/c2nr30435j
Luo, Y., Chen, S., Zhou, J., Chen, J., Tian, L., Gao, W., et al. (2019). Luteolin cocrystals: characterization, evaluation of solubility, oral bioavailability and theoretical calculation. J. Drug Deliv. Sci. Technol. 50, 248–254. doi:10.1016/j.jddst.2019.02.004
Luther, D. C., Huang, R., Jeon, T., Zhang, X., Lee, Y.-W., Nagaraj, H., et al. (2020). Delivery of drugs, proteins, and nucleic acids using inorganic nanoparticles. Adv. Drug Deliv. Rev. 156, 188–213. doi:10.1016/j.addr.2020.06.020
Ma, B., Qi, H., Li, J., Xu, H., Chi, B., Zhu, J., et al. (2015). Triptolide disrupts fatty acids and peroxisome proliferator-activated receptor (PPAR) levels in male mice testes followed by testicular injury: a GC-MS based metabolomics study. Toxicology 336, 84–95. doi:10.1016/j.tox.2015.07.008
Madaan, K., Kumar, S., Poonia, N., Lather, V., and Pandita, D. (2014). Dendrimers in drug delivery and targeting: drug-dendrimer interactions and toxicity issues. J. Pharm. Bioallied. Sci. 6 (3), 139–150. doi:10.4103/0975-7406.130965
Madaan, K., Lather, V., and Pandita, D. (2016). Evaluation of polyamidoamine dendrimers as potential carriers for quercetin, a versatile flavonoid. Drug Deliv. 23 (1), 254–262. doi:10.3109/10717544.2014.910564
Maeda, H., Nakamura, H., and Fang, J. (2013). The EPR effect for macromolecular drug delivery to solid tumors: improvement of tumor uptake, lowering of systemic toxicity, and distinct tumor imaging in vivo. Adv. Drug Deliv. Rev. 65 (1), 71–79. doi:10.1016/j.addr.2012.10.002
Maherani, B., Arab-Tehrany, E., R Mozafari, M., Gaiani, C., and Linder, M. (2011). Liposomes: a review of manufacturing techniques and targeting strategies. Curr. Nanosci. 7 (3), 436–452. doi:10.2174/157341311795542453
Manca, M. L., Manconi, M., Falchi, A. M., Castangia, I., Valenti, D., Lampis, S., et al. (2013). Close-packed vesicles for diclofenac skin delivery and fibroblast targeting. Colloids Surf. B Biointerfaces. 111, 609–617. doi:10.1016/j.colsurfb.2013.07.014
Manna, S., Wu, Y., Wang, Y., Koo, B., Chen, L., Petrochenko, P., et al. (2019). Probing the mechanism of bupivacaine drug release from multivesicular liposomes. J. Control. Release. 294, 279–287. doi:10.1016/j.jconrel.2018.12.029
Mao, Q., Min, J., Zeng, R., Liu, H., Li, H., Zhang, C., et al. (2022). Self-assembled traditional Chinese nanomedicine modulating tumor immunosuppressive microenvironment for colorectal cancer immunotherapy. Theranostics 12 (14), 6088–6105. doi:10.7150/thno.72509
Matur, M., Madhyastha, H., Shruthi, T., Madhyastha, R., Srinivas, S., Navya, P., et al. (2020). Engineering bioactive surfaces on nanoparticles and their biological interactions. Sci. Rep. 10 (1), 19713. doi:10.1038/s41598-020-75465-z
McCormack, P. L. (2016). Sacubitril/valsartan: a review in chronic heart failure with reduced ejection fraction. Drugs 76 (3), 387–396. doi:10.1007/s40265-016-0544-9
Mehnath, S., Chitra, K., Karthikeyan, K., and Jeyaraj, M. (2020). Localized delivery of active targeting micelles from nanofibers patch for effective breast cancer therapy. Int. J. Pharm. 584, 119412. doi:10.1016/j.ijpharm.2020.119412
Minatti, E., Viville, P., Borsali, R., Schappacher, M., Deffieux, A., and Lazzaroni, R. (2003). Micellar morphological changes promoted by cyclization of PS-b-PI copolymer: DLS and AFM experiments. Macromolecules 36 (11), 4125–4133. doi:10.1021/ma020927e
Mitchell, M. J., Billingsley, M. M., Haley, R. M., Wechsler, M. E., Peppas, N. A., and Langer, R. (2021). Engineering precision nanoparticles for drug delivery. Nat. Rev. Drug. Disc. 20 (2), 101–124. doi:10.1038/s41573-020-0090-8
Moein Moghimi, S., Hamad, I., Bünger, R., Andresen, T. L., Jørgensen, K., Christy Hunter, A., et al. (2006). Activation of the human complement system by cholesterol-rich and pegylated liposomes—modulation of cholesterol-rich liposome-mediated complement activation by elevated serum ldl and hdl levels. J. Liposome Res. 16 (3), 167–174. doi:10.1080/08982100600848801
Monteiro, N., Martins, A., Reis, R. L., and Neves, N. M. (2014). Liposomes in tissue engineering and regenerative medicine. J. R. Soc. Interface. 11 (101), 20140459. doi:10.1098/rsif.2014.0459
Mora-Huertas, C. E., Fessi, H., and Elaissari, A. (2010). Polymer-based nanocapsules for drug delivery. Int. J. Pharm. 385 (1-2), 113–142. doi:10.1016/j.ijpharm.2009.10.018
Muqbil, I., Masood, A., Sarkar, F. H., Mohammad, R. M., and Azmi, A. S. (2011). Progress in nanotechnology based approaches to enhance the potential of chemopreventive agents. Cancers (Basel) 3 (1), 428–445. doi:10.3390/cancers3010428
Nair, L. S., and Laurencin, C. T. (2007). Biodegradable polymers as biomaterials. Prog. Polym. Sci. 32 (8-9), 762–798. doi:10.1016/j.progpolymsci.2007.05.017
Negi, P., Sharma, G., Verma, C., Garg, P., Rathore, C., Kulshrestha, S., et al. (2020). Novel thymoquinone loaded chitosan-lecithin micelles for effective wound healing: development, characterization, and preclinical evaluation. Carbohydr. Polym. 230, 115659. doi:10.1016/j.carbpol.2019.115659
Ni, D., Bu, W., Ehlerding, E. B., Cai, W., and Shi, J. (2017). Engineering of inorganic nanoparticles as magnetic resonance imaging contrast agents. Chem. Soc. Rev. 46 (23), 7438–7468. doi:10.1039/c7cs00316a
Ni, L., Yu, C., Wei, Q., Liu, D., and Qiu, J. (2022). Pickering emulsion catalysis: interfacial chemistry, catalyst design, challenges, and perspectives. Angew. Chem. Int. Ed. 61 (30), e202115885. doi:10.1002/anie.202115885
Nkanga, C. I., Bapolisi, A. M., Okafor, N. I., and Krause, R. W. M. (2019). General perception of liposomes: formation, manufacturing and applications. Liposomes-advances Perspect. doi:10.5772/intechopen.84255
Nypelö, T., Rodriguez-Abreu, C., Kolen’ko, Y. V., Rivas, J., and Rojas, O. J. (2014). Microbeads and hollow microcapsules obtained by self-assembly of pickering magneto-responsive cellulose nanocrystals. ACS Appl. Mat. Interfaces. 6 (19), 16851–16858. doi:10.1021/am504260u
Oda, C. M. R., Malfatti-Gasperini, A. A., Malachias, A., Pound-Lana, G., Mosqueira, V. C. F., Fernandes, R. S., et al. (2020). Physical and biological effects of paclitaxel encapsulation on disteraroylphosphatidylethanolamine-polyethyleneglycol polymeric micelles. Colloids Surf. B Biointerfaces. 188, 110760. doi:10.1016/j.colsurfb.2019.110760
Patra, J. K., Das, G., Fraceto, L. F., Campos, E. V. R., Rodriguez-Torres, M. d.P., Acosta-Torres, L. S., et al. (2018). Nano based drug delivery systems: recent developments and future prospects. J. Nanobiotechnol. 16 (1), 71. doi:10.1186/s12951-018-0392-8
Pattni, B. S., Chupin, V. V., and Torchilin, V. P. (2015). New developments in liposomal drug delivery. Chem. Rev. 115 (19), 10938–10966. doi:10.1021/acs.chemrev.5b00046
Peng, S., Wang, Y., Sun, Z., Zhao, L., Huang, Y., Fu, X., et al. (2023). Nanoparticles loaded with pharmacologically active plant-derived natural products: biomedical applications and toxicity. Colloids Surf. B Biointerfaces. 225, 113214. doi:10.1016/j.colsurfb.2023.113214
Pentek, T., Newenhouse, E., O’Brien, B., and Chauhan, A. S. (2017). Development of a topical resveratrol formulation for commercial applications using dendrimer nanotechnology. Molecules 22 (1), 137. doi:10.3390/molecules22010137
Petros, R. A., and DeSimone, J. M. (2010). Strategies in the design of nanoparticles for therapeutic applications. Nat. Rev. Drug. Disc. 9 (8), 615–627. doi:10.1038/nrd2591
Priyadarshi, K., Shirsath, K., Waghela, N. B., Sharma, A., Kumar, A., and Pathak, C. (2021). Surface modified PAMAM dendrimers with gallic acid inhibit, cell proliferation, cell migration and inflammatory response to augment apoptotic cell death in human colon carcinoma cells. J. Biomol. Struct. Dyn. 39 (18), 6853–6869. doi:10.1080/07391102.2020.1802344
Qi, X.-J., Liu, X.-Y., Tang, L.-M.-Y., Li, P.-F., Qiu, F., and Yang, A.-H. (2020a). Anti-depressant effect of curcumin-loaded guanidine-chitosan thermo-sensitive hydrogel by nasal delivery. Pharm. Dev. Technol. 25 (3), 316–325. doi:10.1080/10837450.2019.1686524
Qi, Y., Guo, L., Jiang, Y., Shi, Y., Sui, H., and Zhao, L. (2020b). Brain delivery of quercetin-loaded exosomes improved cognitive function in AD mice by inhibiting phosphorylated tau-mediated neurofibrillary tangles. Drug Deliv. 27 (1), 745–755. doi:10.1080/10717544.2020.1762262
Rahmati, M., Ehterami, A., Saberani, R., Abbaszadeh-Goudarzi, G., Rezaei Kolarijani, N., Khastar, H., et al. (2021). Improving sciatic nerve regeneration by using alginate/chitosan hydrogel containing berberine. Drug Deliv. Transl. Res. 11, 1983–1993. doi:10.1007/s13346-020-00860-y
Ramalingam, V. (2019). Multifunctionality of gold nanoparticles: plausible and convincing properties. Adv. Colloid Interface Sci. 271, 101989. doi:10.1016/j.cis.2019.101989
Ramalingam, V., Rajaram, R., PremKumar, C., Santhanam, P., Dhinesh, P., Vinothkumar, S., et al. (2014). Biosynthesis of silver nanoparticles from deep sea bacterium Pseudomonas aeruginosa JQ989348 for antimicrobial, antibiofilm, and cytotoxic activity. J. Basic Microbiol. 54 (9), 928–936. doi:10.1002/jobm.201300514
Rathinavel, S., Korrapati, P. S., Kalaiselvi, P., and Dharmalingam, S. (2021). Mesoporous silica incorporated PCL/Curcumin nanofiber for wound healing application. Eur. J. Pharm. Sci. 167, 106021. doi:10.1016/j.ejps.2021.106021
Sabra, R., Roberts, C. J., and Billa, N. (2019). Courier properties of modified citrus pectinate-chitosan nanoparticles in colon delivery of curcumin. Colloid Interface Sci. Commun. 32, 100192. doi:10.1016/j.colcom.2019.100192
Sarcan, E. T., Silindir-Gunay, M., and Ozer, A. Y. (2018). Theranostic polymeric nanoparticles for NIR imaging and photodynamic therapy. Int. J. Pharm. 551 (1-2), 329–338. doi:10.1016/j.ijpharm.2018.09.019
Sebaaly, C., Greige-Gerges, H., Stainmesse, S., Fessi, H., and Charcosset, C. (2016). Effect of composition, hydrogenation of phospholipids and lyophilization on the characteristics of eugenol-loaded liposomes prepared by ethanol injection method. Food Biosci. 15, 1–10. doi:10.1016/j.fbio.2016.04.005
Starigazdová, J., Nešporová, K., Čepa, M., Šínová, R., Šmejkalová, D., Huerta-Angeles, G., et al. (2020). In vitro investigation of hyaluronan-based polymeric micelles for drug delivery into the skin: the internalization pathway. Eur. J. Pharm. Sci. 143, 105168. doi:10.1016/j.ejps.2019.105168
Su, Y. L., Fang, J. H., Liao, C. Y., Lin, C. T., Li, Y. T., and Hu, S. H. (2015). Targeted mesoporous iron oxide nanoparticles-encapsulated perfluorohexane and a hydrophobic drug for deep tumor penetration and therapy. Theranostics 5 (11), 1233–1248. doi:10.7150/thno.12843
Sun, S., Du, X., Fu, M., Khan, A. R., Ji, J., Liu, W., et al. (2021). Galactosamine-modified PEG-PLA/TPGS micelles for the oral delivery of curcumin. Int. J. Pharm. 595, 120227. doi:10.1016/j.ijpharm.2021.120227
Swamy, M. K., Nath, S., Paul, S., Jha, N. K., Purushotham, B., Rohit, K. C., et al. (2021). Biotechnology of camptothecin production in Nothapodytes nimmoniana, Ophiorrhiza sp. and Camptotheca acuminata. Appl. Microbiol. Biotechnol. 105 (24), 9089–9102. doi:10.1007/s00253-021-11700-5
Tai, Z., Huang, Y., Zhu, Q., Wu, W., Yi, T., Chen, Z., et al. (2020). Utility of Pickering emulsions in improved oral drug delivery. (AV) drug Discov. A Promis. alliance. Drug. Discov. Today 25 (11), 2038–2045. doi:10.1016/j.drudis.2020.09.012
Takahashi, M., Uechi, S., Takara, K., Asikin, Y., and Wada, K. (2009). Evaluation of an oral carrier system in rats: bioavailability and antioxidant properties of liposome-encapsulated curcumin. J. Agric. Food Chem. 57 (19), 9141–9146. doi:10.1021/jf9013923
Tan, X.-R., Chao, L., Feng, K.-K., Le, J.-Q., Shen, J.-W., and Shao, J.-W. (2022). Self-assembled micelles of the natural medicine ginsenosides for cancer metastasis therapy. J. Ind. Eng. Chem. 116, 303–309. doi:10.1016/j.jiec.2022.09.020
Teagarden, D. L., and Baker, D. S. (2002). Practical aspects of lyophilization using non-aqueous co-solvent systems. Eur. J. Pharm. Sci. 15 (2), 115–133. doi:10.1016/s0928-0987(01)00221-4
Tian, X., Wang, P., Li, T., Huang, X., Guo, W., Yang, Y., et al. (2020). Self-assembled natural phytochemicals for synergistically antibacterial application from the enlightenment of traditional Chinese medicine combination. Acta Pharm. Sin. B 10 (9), 1784–1795. doi:10.1016/j.apsb.2019.12.014
Tomalia, D. A. (2005). Birth of a new macromolecular architecture: dendrimers as quantized building blocks for nanoscale synthetic polymer chemistry. Prog. Polym. Sci. 30 (3-4), 294–324. doi:10.1016/j.progpolymsci.2005.01.007
Tosi, G., Duskey, J. T., and Kreuter, J. (2020). Nanoparticles as carriers for drug delivery of macromolecules across the blood-brain barrier. Expert Opin. Drug Deliv. 17 (1), 23–32. doi:10.1080/17425247.2020.1698544
Tu, L., Wang, G., Qi, N., Wu, W., Zhang, W., Feng, J., et al. (2020). Multi-functional chitosan polymeric micelles as oral paclitaxel delivery systems for enhanced bioavailability and anti-tumor efficacy. Int. J. Pharm. 578, 119105. doi:10.1016/j.ijpharm.2020.119105
Van Vlerken, L. E., Vyas, T. K., and Amiji, M. M. (2007). Poly (ethylene glycol)-modified nanocarriers for tumor-targeted and intracellular delivery. Pharm. Res. 24, 1405–1414. doi:10.1007/s11095-007-9284-6
Wan, Y., Wang, L., Zhu, C., Zheng, Q., Wang, G., Tong, J., et al. (2018). Aptamer-conjugated extracellular nanovesicles for targeted drug delivery. Cancer Res. 78 (3), 798–808. doi:10.1158/0008-5472.Can-17-2880
Wang, J., Wong, Y.-K., and Liao, F. (2018). What has traditional Chinese medicine delivered for modern medicine? Expert Rev. Mol. Med. 20, e4. doi:10.1017/erm.2018.3
Wang, S.-R., Chen, X., Ling, S., Ni, R.-z., Guo, H., and Xu, J.-W. (2019). MicroRNA expression, targeting, release dynamics and early-warning biomarkers in acute cardiotoxicity induced by triptolide in rats. Biomed. Pharmacother. 111, 1467–1477. doi:10.1016/j.biopha.2018.12.109
Wang, W., Tang, Q., Yu, T., Li, X., Gao, Y., Li, J., et al. (2017). Surfactant-free preparation of Au@ Resveratrol hollow nanoparticles with photothermal performance and antioxidant activity. ACS Appl. Mat. Interfaces . 9 (4), 3376–3387. doi:10.1021/acsami.6b13911
Wang, X., Qiu, L., Wang, X., Ouyang, H., Li, T., Han, L., et al. (2020a). Evaluation of intestinal permeation enhancement with carboxymethyl chitosan-rhein polymeric micelles for oral delivery of paclitaxel. Int. J. Pharm. 573, 118840. doi:10.1016/j.ijpharm.2019.118840
Wang, Y., van Steenbergen, M. J., Beztsinna, N., Shi, Y., Lammers, T., van Nostrum, C. F., et al. (2020b). Biotin-decorated all-HPMA polymeric micelles for paclitaxel delivery. J. Control. Release. 328, 970–984. doi:10.1016/j.jconrel.2020.09.013
Wang, Y., Wang, X., Luo, G., and Dai, Y. (2008). Adsorption of bovin serum albumin (BSA) onto the magnetic chitosan nanoparticles prepared by a microemulsion system. Bioresour. Technol. 99 (9), 3881–3884. doi:10.1016/j.biortech.2007.08.017
Watkins, R., Wu, L., Zhang, C., Davis, R. M., and Xu, B. (2015). Natural product-based nanomedicine: recent advances and issues. Int. J. Nanomedicine. 10, 6055–6074. doi:10.2147/ijn.S92162
Weng, Q., Cai, X., Zhang, F., and Wang, S. (2019). Fabrication of self-assembled Radix Pseudostellariae protein nanoparticles and the entrapment of curcumin. Food Chem. 274, 796–802. doi:10.1016/j.foodchem.2018.09.059
William, B., Noemie, P., Brigitte, E., and Geraldine, P. (2020). Supercritical fluid methods: an alternative to conventional methods to prepare liposomes. Chem. Eng. J. 383, 123106. doi:10.1016/j.cej.2019.123106
Wu, L., Shi, Y., Ni, Z., Yu, T., and Chen, Z. (2021). Preparation of a self-assembled rhein–doxorubicin nanogel targeting mitochondria and investigation on its antihepatoma activity. Mol. Pharm. 19 (1), 35–50. doi:10.1021/acs.molpharmaceut.1c00565
Wu, L., Shi, Y., Ni, Z., Yu, T., and Chen, Z. (2022). Preparation of a self-assembled rhein–doxorubicin nanogel targeting mitochondria and investigation on its antihepatoma activity. Mol. Pharm. 19 (1), 35–50. doi:10.1021/acs.molpharmaceut.1c00565
Wu, S., Yang, X., Luo, F., Wu, T., Xu, P., Zou, M., et al. (2018). Biosynthesis of flower-shaped Au nanoclusters with EGCG and their application for drug delivery. J. Nanobiotechnol. 16, 90–14. doi:10.1186/s12951-018-0417-3
Yadav, D., Semwal, B. C., and Dewangan, H. K. (2023). Grafting, characterization and enhancement of therapeutic activity of berberine loaded PEGylated PAMAM dendrimer for cancerous cell. J. Biomater. Sci. Polym. Ed. 34 (8), 1053–1066. doi:10.1080/09205063.2022.2155782
Yang, C., Chen, H., Zhao, J., Pang, X., Xi, Y., and Zhai, G. (2014). Development of a folate-modified curcumin loaded micelle delivery system for cancer targeting. Colloids Surf. B Biointerfaces. 121, 206–213. doi:10.1016/j.colsurfb.2014.05.005
Yang, C., Zhang, M., and Merlin, D. (2018). Advances in plant-derived edible nanoparticle-based lipid nano-drug delivery systems as therapeutic nanomedicines. J. Mat. Chem. B 6 (9), 1312–1321. doi:10.1039/c7tb03207b
Yao, H., Mu, X., Wei, Z., Li, X., Wu, L., Jin, Y., et al. (2022). Facile approach for surfactant-free synthesis of Au@ ginsenoside Rh2 nanoparticles and researches on anticancer activity. Cancer Nanotechnol. 13 (1), 35. doi:10.1186/s12645-022-00142-x
You, L., Liang, K., An, R., and Wang, X. (2022). The path towards FDA approval: a challenging journey for Traditional Chinese Medicine. Pharmacol. Res. 182, 106314. doi:10.1016/j.phrs.2022.106314
Yu, L., Wang, Z., Mo, Z., Zou, B., Yang, Y., Sun, R., et al. (2021). Synergetic delivery of triptolide and Ce6 with light-activatable liposomes for efficient hepatocellular carcinoma therapy. Acta Pharm. Sin. B 11 (7), 2004–2015. doi:10.1016/j.apsb.2021.02.001
Zhang, M., Gao, M., Wu, S., Zhou, L., Cao, L., Qiao, R., et al. (2021). Hepatotoxicity comparison of crude and licorice-processed euodiae fructus in rats with stomach excess-cold syndrome. Front. Pharmacol. 12, 756276. doi:10.3389/fphar.2021.756276
Zhao, X., Yang, Y., Su, X., Xie, Y., Liang, Y., Zhou, T., et al. (2023). Transferrin-modified triptolide liposome targeting enhances anti-hepatocellular carcinoma effects. Biomedicines 11 (10), 2869. doi:10.3390/biomedicines11102869
Zheng, Y., Wang, Y., Xia, M., Gao, Y., Zhang, L., Song, Y., et al. (2022). The combination of nanotechnology and traditional Chinese medicine (TCM) inspires the modernization of TCM: review on nanotechnology in TCM-based drug delivery systems. Drug Deliv. Transl. Res. 12 (6), 1306–1325. doi:10.1007/s13346-021-01029-x
Zhi, K., Wang, J., Zhao, H., and Yang, X. (2020). Self-assembled small molecule natural product gel for drug delivery: a breakthrough in new application of small molecule natural products. Acta Pharm. Sin. B 10 (5), 913–927. doi:10.1016/j.apsb.2019.09.009
Zhou, J., Gao, G., Chu, Q., Wang, H., Rao, P., and Ke, L. (2014). Chromatographic isolation of nanoparticles from Ma-Xing-Shi-Gan-Tang decoction and their characterization. J. Ethnopharmacol. 151 (3), 1116–1123. doi:10.1016/j.jep.2013.12.029
Zhou, J., Zhang, J., Gao, G., Wang, H., He, X., Chen, T., et al. (2019). Boiling licorice produces self-assembled protein nanoparticles: a novel source of bioactive nanomaterials. J. Agric. Food Chem. 67 (33), 9354–9361. doi:10.1021/acs.jafc.9b03208
Zhou, K., Zhu, Y., Chen, X., Li, L., and Xu, W. (2020). Redox- and MMP-2-sensitive drug delivery nanoparticles based on gelatin and albumin for tumor targeted delivery of paclitaxel. Biomater. Adv. 114, 111006. doi:10.1016/j.msec.2020.111006
Zhu, Y., Zhang, J., Song, J., Yang, J., Du, Z., Zhao, W., et al. (2020). A multifunctional pro-healing zwitterionic hydrogel for simultaneous optical monitoring of pH and glucose in diabetic wound treatment. Adv. Funct. Mat. 30 (6), 1905493. doi:10.1002/adfm.201905493
Zhuang, Y., Yan, J., Zhu, W., Chen, L., Liang, D., and Xu, X. (2008). Can the aggregation be a new approach for understanding the mechanism of Traditional Chinese Medicine? J. Ethnopharmacol. 117 (2), 378–384. doi:10.1016/j.jep.2008.02.017
Zou, H., Zhu, J., and Huang, D. S. (2019). Cell membrane capsule: a novel natural tool for antitumour drug delivery. Expert Opin. Drug Deliv. 16 (3), 251–269. doi:10.1080/17425247.2019.1581762
Zuo, J., Park, C., Kung, J. Y. C., Bou-Chacra, N. A., Doschak, M., and Löbenberg, R. (2021). Traditional Chinese medicine "pill", an ancient dosage form with surprising modern pharmaceutical characteristics. Pharm. Res. 38 (2), 199–211. doi:10.1007/s11095-021-03007-x
Glossary
Keywords: TCM, active ingredients, nanotechnology, nano-TCM, drug delivery
Citation: Zhang Y-B, Wang J-F, Wang M-X, Peng J, Kong X-D and Tian J (2024) Nano-based drug delivery systems for active ingredients from traditional Chinese medicine: Harnessing the power of nanotechnology. Front. Pharmacol. 15:1405252. doi: 10.3389/fphar.2024.1405252
Received: 22 March 2024; Accepted: 20 May 2024;
Published: 07 June 2024.
Edited by:
Karim Hosni, Institut National de Recherche et d’Analyse Physico-Chimique (INRAP), TunisiaReviewed by:
Dong Kai Wang, Shenyang Pharmaceutical University, ChinaHao Wang, Chinese Academy of Sciences (CAS), China
Copyright © 2024 Zhang, Wang, Wang, Peng, Kong and Tian. This is an open-access article distributed under the terms of the Creative Commons Attribution License (CC BY). The use, distribution or reproduction in other forums is permitted, provided the original author(s) and the copyright owner(s) are credited and that the original publication in this journal is cited, in accordance with accepted academic practice. No use, distribution or reproduction is permitted which does not comply with these terms.
*Correspondence: Jie Tian, cnp0aWFuamllNjc2QDEyNi5jb20=
†These authors have contributed equally to this work