- Department of Cardiology, The Affiliated Taizhou People’s Hospital of Nanjing Medical University, Taizhou School of Clinical Medicine, Nanjing Medical University, Taizhou, China
Epidermal Growth Factor Receptor-Tyrosine Kinase Inhibitors (EGFR-TKIs) are a class of oral targeted anticancer drugs that have been demonstrated to significantly inhibit tumor progression and improve clinical prognosis in patients diagnosed with EGFR-mutated tumors, particularly in those with non-small cell lung cancer. However, the sustained usage of EGFR-TKIs may cause potential cardiotoxicity, thus limiting their applicability. The primary objective of this review is to systematically analyze the evolving landscape of research pertaining to EGFR-TKI-induced cardiotoxicity and elucidate its underlying mechanisms, such as PI3K signaling pathway inhibition, ion channel blockade, oxidative stress, inflammatory responses, and apoptosis. Additionally, the review includes an exploration of risk assessment for cardiotoxicity induced by EGFR-TKIs, along with management and response strategies. Prospective research directions are outlined, emphasizing the need for more accurate predictors of cardiotoxicity and the development of innovative intervention strategies. In summation, this review consolidates recent research advances, illuminates the risks associated with EGFR-TKI-induced cardiac toxicity and presents crucial insights for refining clinical dosage protocols, optimizing patient management strategies, and unraveling the intricate mechanisms governing EGFR-TKI-induced cardiotoxicity.
1 Introduction
Tyrosine kinases constitute a family of proteins that, upon activation, catalyze the phosphorylation of tyrosine residues on other intracellular proteins (Gocek et al., 2014). Epidermal Growth Factor Receptor-Tyrosine Kinase Inhibitors (EGFR-TKIs) function as competitive inhibitors of EGFR-TK activation, displaying high affinity for the adenosine triphosphate (ATP) binding site of EGFR. Consequently, they competitively bind to the intracellular tyrosine kinase domain of EGFR, inhibiting ATP from binding to its site. This disruption of ATP binding leads to the inhibition of receptor autophosphorylation, thereby obstructing downstream signaling. Ultimately, EGFR-TKIs impede tumor growth, proliferation, and differentiation, inducing apoptosis in tumor cells (Chaar et al., 2018; Harrison et al., 2020).
EGFR-TKIs is indicated for patients with a diverse array of tumors carrying EGFR mutations, encompassing non-small cell lung cancer (NSCLC), pancreatic cancer, glioblastoma, and head and neck squamous cell carcinoma (Mukasa et al., 2010; Wang et al., 2024; Zhang et al., 2021). Its most prevalent application is in the management of NSCLC which accounts for approximately 85% of all lung cancer cases (Alanazi et al., 2021). The survival rate of NSCLC varies depending on the stage of the disease at diagnosis (Siegel et al., 2012). If detected early, when the tumor is still confined to the lungs, the 5-year survival rate can be as high as 56%. However, most patients are diagnosed at later stages (Majeed et al., 2021), such as when the tumor has spread to other organs, at which point the 5-year survival rate may drop to as low as 5% (Gettinger and Lynch, 2011). Additionally, the progression rate of NSCLC can range from relatively slow to extremely rapid, depending on the biological characteristics of the tumor and its molecular markers (Yuan et al., 2019). In the Chinese NSCLC population, approximately 30% of patients exhibit EGFR mutations, showcasing a significant impact of EGFR-TKIs on this subgroup (Shi et al., 2014). Currently, three generations of EGFR-TKIs are in clinical use: the first generation includes Gefitinib, Erlotinib, and Icotinib; the second generation comprises Afatinib, Dacomitinib, and Lapatinib; the third generation encompasses Osimertinib, Almonertinib (Aumolertinib), Alflutinib (Furmonertinib), and Lazertinib (Table 1). The fourth-generation EGFR-TKIs such as EAI045, TQB3804, and JNJ-61186372 (JNJ-372) are either in development or undergoing clinical trials. Notably, the third-generation EGFR-TKIs have successfully overcome resistance observed with first- and second-generation counterparts. They act as irreversible EGFR inhibitors, effectively managing cancer progression induced by EGFR-TKI-sensitive and T790M-resistant mutations, with an added advantage of controlling brain metastases (Cross et al., 2014; Remon and Planchard, 2015; Anand et al., 2019). While monotherapy with EGFR-TKIs has demonstrated superior efficacy and tolerability compared to standard chemotherapy, a notable drawback is their association with cardiac toxicity (Chang et al., 2023). The concurrent manifestation of cardiotoxicity frequently necessitates the temporary or premature discontinuation of anticancer therapy in patients undergoing targeted treatment (Ikebe et al., 2020). Striking a balance between the benefits of targeted cancer therapy and the cardiovascular risks has becoming a pressing and intricate concern for clinicians (Schiefer et al., 2018).
2 Mechanisms of cardiotoxicity caused by EGFR-TKIs
While the precise mechanism underlying cardiotoxicity induced by EGFR-TKIs remains incompletely understood, existing studies offer a basis for exploring potential mechanisms from various perspectives.
2.1 Inhibition of the PI3K signaling pathway and effects on ion channels
The fast-activating delayed rectifier K+ current (IKr), mediated by the K+ channel encoded by the human ether-a-go-go-related gene (hERG), constitutes the principal component of cardiac repolarization during the third phase of the cardiac action potential, particularly in large animals, including humans. Pharmacological blockade of the cardiac potassium channel of hERG leads to a decrease in IKr, which is a crucial molecular mechanism contributing to the prolongation of QT intervals and, consequently, the initiation of cardiac arrhythmias (Yang and Nerbonne, 2016; Chiamvimonvat et al., 2017; Helliwell et al., 2023). Intriguingly, some drugs that induced long QT syndrome by directly blocking IKr also exhibit inhibitory effects on the PI3K signaling pathway, a phenomenon that may contribute to their arrhythmogenic potential (Yang et al., 2014; Ballou et al., 2015).
Many TKIs, such as nilotinib, vandetanib, erlotinib, gefitinib, and imatinib, have been demonstrated to inhibit hERG currents by directly blocking hERG channels and/or modulating acute effects on channel gating (Kopljar et al., 2018; Jie et al., 2021; Cui et al., 2022; Li et al., 2023). Additionally, certain TKIs, including erlotinib, can hinder the phosphatidylinositide 3-kinases-Serum and glucocorticoid-regulated kinase 1 (PI3K-SGK1) signaling pathway, disrupting the post-transcriptional process of hERG potassium channel functional protein. This interference promotes the internalization of mature protein on the membrane, leading to reduced membrane hERG channel protein expression, further inhibiting hERG current, and resulting in QT interval prolongation (Cui et al., 2022).
Experimental studies involving canine cardiomyocytes treated with either a TKI or a PI3K inhibitor demonstrated an increase in action potential duration, while the intracellular infusion of phosphatidylinositol 3,4,5-triphosphate, a downstream effector of the PI3K pathway, reversed this effect. This suggests that the inhibition of PI3K-SGK1 signaling contributes to the prolongation of action potential duration and QT interval (Lu et al., 2012).
Insulin receptor tyrosine kinase activates PI3Kα to produce phosphatidylinositol 3,4,5-trisphosphate, recruiting protein kinase B (Akt) and phosphatidylinositol 3-phosphate-dependent protein kinase 1 (PDK1) to the plasma membrane, leading to Akt activation. Receptor tyrosine kinase also activates atypical protein kinase C and SGK via PDK1. Akt, PDK1, atypical protein kinase C, SGK, and other downstream effectors of PI3K may regulate ion channels conducting potassium, sodium, and calcium current (Ballou et al., 2015). Zhang et al. proposed that the regulation of PI3K/Akt pathway inhibition and its downstream molecules, resulted in an increase in persistent sodium current and a decrease in L-type calcium current, potassium current (IKr and IKs), ultimately leading to QT interval prolongation (Zhang et al., 2023).
In a 2023 study, Peiwen Li’s team found that osimertinib prolonged QT interval, PR interval, QRS complex, and left atrial, left ventricular, and atrioventricular conduction time in isolated guinea pig hearts. Osimertinib also concentration-dependently blocked hERG, Nav1.5, and L-type Ca2+ channels. Therefore, they suggested that the cardiotoxic effects of osimertinib, such as QT interval prolongation and the reduction of Left Ventricular Ejection Fraction (LVEF), were attributed to its effects on hERG, Nav1.5, and L-type Ca2+ channel (Li et al., 2023). Different EGFR-TKIs may block various ion channels, but the primary ion channels they affect seem to be hERG potassium ion channels. For example, lapatinib predominantly blocks hERG channels, mildly affecting IKs current, while exhibiting no blocking effect on sodium current, inward rectifier potassium current, and calcium current (Zhang et al., 2023). In conclusion, compared to direct channel blocking, the final QT prolongation may be caused by inhibition of the PI3K/Akt signaling pathway and consequent changes in ion current (Figure 1).
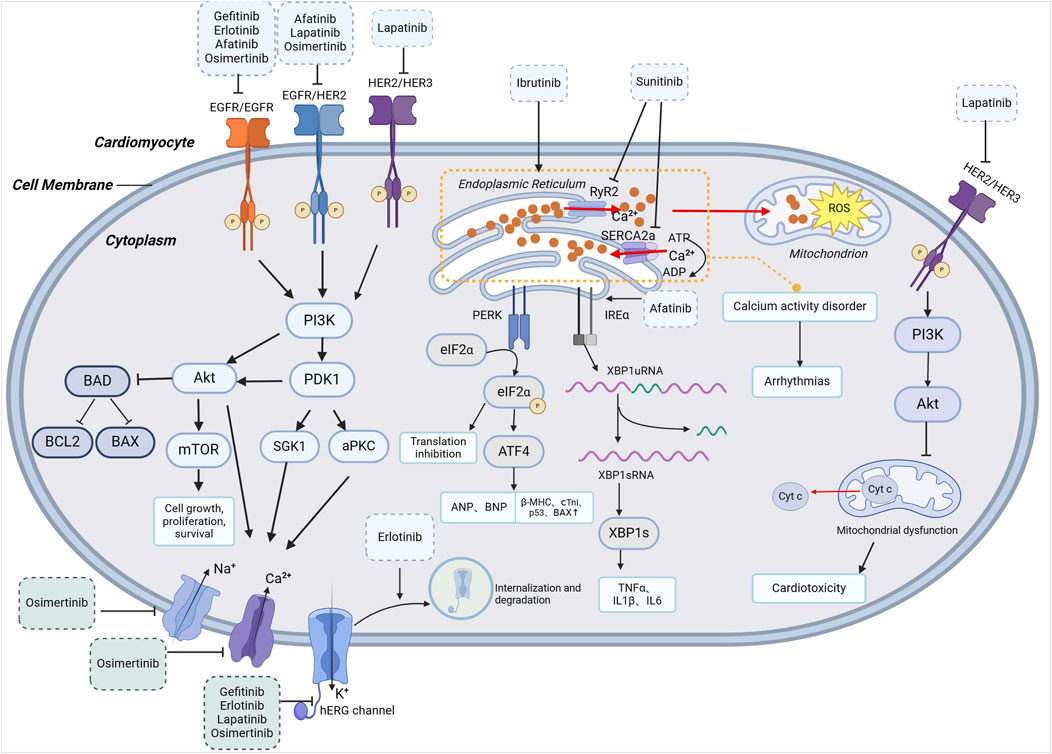
Figure 1. Mechanisms related to cardiotoxicity of EGFR-TKIs. PI3K, phosphatidylinositide 3-kinases; Akt, recruiting protein kinase B; PDK1, phosphatidylinositol 3-phosphate-dependent protein kinase 1; mTOR, mammalian target of rapamycin; BAD, Bcl-xL/Bcl-2asociated death promoter; BCL2, B-cell lymphoma-2; BAX, Bcl-2-associated X protein; PERK, Protein kinase R (PKR)-like endoplasmic reticulum kinase; IREα, Inositol requiring enzyme α; eIFα, eukaryotic initiation factor α; ATF4, activating transcription factor 4; XBP1, X-Box Binding Protein 1.
2.2 Inhibition of HER2
The well-established EGF family comprises four members, discovered in sequential order: EGFR, also known as human epidermal growth factor receptor 1 (HER1, ERBB1), human epidermal growth factor receptor 2 (HER2, ERBB2), human epidermal growth factor receptor 3 (HER3, ERBB3), and human epidermal growth factor receptor 4 (HER4, ERBB4) (Kwapiszewski et al., 2016). HER2 is expressed across multiple organ systems, including the cell membrane of cardiomyocytes, playing a crucial role in cardiomyocyte survival, growth, and stress response (Lenneman and Sawyer, 2016). Its related pathway, the ErbB/neuregulin 1 (NRG1) pathway, stands as a significant mechanism facilitating cardiac adaptation to stress (Odiete et al., 2012; Belmonte et al., 2015; Grego-Bessa et al., 2023). NRG1 activates ErbB2 phosphorylation and intracellular signaling through PI3K/Akt and ERK1/2 pathways. Its downstream signaling involves the upregulation of glutathione reductase and endothelial nitric oxide synthase mRNA, leading to decreased production of reactive oxygen species (ROS). This suggests that ErbB2/NGR1 signaling pathway plays a certain role in the antioxidant defense of cardiomyocytes (Timolati et al., 2006; Eldridge et al., 2014; Leemasawat et al., 2020). The existing research indicates that TKIs are involved in this pathological pathway. Lapatinib exhibits cardiotoxicity by diminishing ErbB2 phosphorylation. Furthermore, research has identified that osimertinib and afatinib can impair HER2 function while inhibiting EGFR, thereby inducing cardiac dysfunction (Figure 1) (Li et al., 2008; Cross et al., 2014; Herrmann, 2020).
2.3 Oxidative stress, ER stress, and inflammatory responses
Huan Wang et al. performed a comprehensive analysis of eight TKIs exhibiting varying degrees of cardiotoxicity, assessing the phenotypic and transcriptomic responses in human cardiomyocytes subjected to different doses and durations of TKIs. Among them, afatinib, sorafenib, and ponatinib showed the ability to activate the protein kinase R-like sarcoplasmic reticulum kinase or inositol requiring enzyme 1α axis of the endoplasmic reticulum stress (ERS) pathway, leading to ERS induction and cardiac fetal gene expression, ultimately causing cardiotoxicity (Wang et al., 2023). TKI-induced ERS upregulated inflammation in cardiomyocytes, involving the inflammasome-IL1β pathway and the nuclear factor kappa-B pathway. This presents a potential therapeutic target for attenuating sorafenib- and ponatinib-induced cardiotoxicity (Figure 1). However, in the case of afatinib, ERS activation is essential for drug-induced apoptosis in tumor cells. Inhibiting ERS might adversely affect afatinib’s therapeutic efficacy, making it an unsuitable candidate for treating TKI-related cardiotoxicity. The study also revealed that afatinib induced varying degrees of lipid peroxidation and an increase in ROS in rat cardiomyocytes (Wang et al., 2023). Schreier et al. discovered that targeted deletion of EGFR in vascular smooth muscle isolated from a mouse aorta led to spontaneous cell death, implicating oxidative stress in this process (Schreier et al., 2011). Furthermore, EGFR-TKIs with concurrent HER2 inhibition were associated with a concentration-dependent increase in ROS production (Fox et al., 2020).
2.4 Autophagy and apoptosis in cardiomyocytes
Gefitinib treatment of rat cardiomyocytes demonstrated a time- and concentration-dependent upregulation of hypertrophic gene markers at both mRNA and protein levels, including BNP and β-myostroponinn heavy chain. Concurrently, anti-hypertrophic gene markers were downregulated, resulting in an increased proportion of hypertrophic cardiomyocytes. Additionally, there was a proportional and concentration-dependent elevation in the mRNA and protein levels of apoptotic markers, such as troponins I, p53, and Bcl-2-associated X protein, strongly indicating that gefitinib initiates events leading to apoptotic death in cardiomyocytes (Figure 1) (Korashy et al., 2016; AlAsmari et al., 2020). Lapatinib treatment increased the ratio of pro-apoptotic BCL-Xs to anti-apoptotic BCL-Xl protein, potentially causing ATP depletion and inducing cardiomyocyte apoptosis (Hervent and De Keulenaer, 2012). In 2020, Ali Alhoshani et al. observed an increase in the level of LC3II protein, a marker of autophagy, in mouse cardiomyocytes following gefitinib treatment (Alhoshani et al., 2020). Monika E. Grabowska’s team, in 2021, found that sorafenib, sunitinib, ponatinib, trastuzumab, and gefitinib induced high levels of apoptosis, while nilotinib and erlotinib induced lower levels of apoptosis based on a computational model of cardiac apoptosis that they constructed and validated. The reduced contractility of neonatal rat 3D engineered heart tissue after the administration of sunitinib, imatinib, sorafenib, and lapatinib was associated with impaired autophagy function and the presence of autophagic lysosomes in ultrastructural evaluation (Grabowska et al., 2021). Zhang Z et al. discovered that osimertinib could induce autophagy by inhibiting the PI3K/Akt signaling pathway (Zhang et al., 2019). It has also been suggested that the inhibition of targeted ErbB2 can alter Bcl-x splicing and induce endogenous apoptotic signals, leading to apoptosis and triggering cardiotoxicity (Rohrbach et al., 2005; Yeh and Bickford, 2009).
2.5 Calcium activity disorder
In cardiomyocytes, intracellular calcium transients integrate electrochemical signals from the action potential with molecular signaling pathways that regulate contraction, and disruptions in intracellular Ca2+ transients are associated with many arrhythmias (Bers, 2002; Altamirano and Bers, 2007; Lu et al., 2015; Kopljar et al., 2018; Du et al., 2020; Zhu et al., 2022). Ca2+ also plays a crucial role in maintaining sarcoplasmic reticulum homeostasis and facilitating cardiomyocyte contraction, while Ca2+ overload is associated with ERS and systolic/diastolic defects (Frisk et al., 2021; Wang et al., 2023). According to Jong J. Kim, drug-induced ventricular arrhythmia in patients with type 2 long QT are underlain by synchronized systolic subcellular Ca2+ elevation (Kim et al., 2015). Beyond long QT syndrome, abnormalities in intracellular free calcium have been implicated in various pathologies (Bers, 2014; Dridi et al., 2020; Hutchings et al., 2022). In human and animal models of heart failure (HF), the reduced amplitude and slower attenuation of intracellular free Ca2+ transients, stemming from diminished expression/function of sarcoplasmic reticulum Ca2+-ATPase, are often accompanied by increased Na+/Ca2+ exchanger activity and decreased inward rectifier potassium current amplitude. In these scenarios, residual adrenergic activity might induce sarcoplasmic reticulum Ca2+ overload, triggering spontaneous Ca2+ release events and initiating arrhythmias (Pogwizd et al., 2001; London et al., 2003). Spontaneous Ca2+ release through the cardiac ryanodine receptor (RyR2), termed “storage overload-induced Ca2+ release”, is a common mechanism of arrhythmia. Arrhythmias induced by silmitasertib (a casein kinase 2 inhibitor) and sunitinib have been reported to result from their effects on RyR2 and increased storage overload-induced Ca2+ release (Figure 1) (Chakraborty et al., 2019). Although EGFR-TKIs such as osimertinib may have a similar effect, further studies are necessary to confirm this hypothesis.
2.6 Mitochondrial dysfunction
Lei Sun et al. conducted experiments using a combination of lapatinib and doxorubicin to treat cardiomyocytes. The results showed that lapatinib enhanced oxidative stress and ferroptosis in cardiomyocytes by inhibiting the PI3K/Akt pathway, which ultimately resulted in mitochondrial dysfunction. This exacerbation of doxorubicin-induced cardiotoxicity was linked to lapatinib’s impact on cellular processes (Sun et al., 2022). Numerous studies have demonstrated that HER2 inhibition can induce mitochondrial dysfunction and disrupt adequate energy supply (Grazette et al., 2004; Gordon et al., 2009). While lapatinib did not exhibit a significant effect on nitric oxide synthase expression and basal mitochondrial respiration, it did impair the reserve oxygen consumption rate (Figure 1) (Rana et al., 2012; Hsu et al., 2018). These findings suggested that lapatinib’s interference with mitochondrial function might contribute to the exacerbation of doxorubicin-induced cardiotoxicity, potentially through the disruption of cellular energy homeostasis.
2.7 Other possible mechanisms of cardiotoxicity
The study conducted by Huan Wang et al. also revealed that afatinib induced the deletion of TNNT2 protein, known as cardiac troponin T2, a vital component of the myocardial sarcomere and a marker of myocardial injury. The diminished expression of this protein may have adverse effects on cardiomyocyte contraction, suggesting a potential mechanism for afatinib-induced cardiotoxicity (Wang et al., 2023). Gefitinib was found to significantly enhance platelets’ ability to produce thromboxane A2, thereby elevating their prothrombotic capacity (Kanazawa et al., 2006; Lynch et al., 2011; Zaborowska-Szmit et al., 2016). Additionally, it has been proposed that gefitinib, through its inhibition of epidermal growth factor, might render the surface of atherosclerotic plaques more susceptible to damage, potentially contributing to cardiotoxicity (Miyagawa et al., 1995; Kawakami et al., 2003; Zaborowska-Szmit et al., 2020). Chronic erlotinib treatment, as reported by Mak et al., led to hypomagnesemia, triggering substance P receptor-mediated oxidative stress and resulting in cardiac dysfunction (Mak et al., 2015). Takahisa Noma’s findings suggested that β-inhibitor-mediated trans-activation of the β1-adrenergic receptor EGFR confers cardioprotection. In a mouse model of catecholamine overdose, EGFR signaling itself exhibited a protective effect (Noma et al., 2007). Pharmacologic inhibition of EGFR by erlotinib resulted in dilated cardiomyopathy after chronic catecholamine infusion in mice. Consequently, in the presence of catecholamine overproduction, erlotinib may impede myocardial protective signaling by inhibiting EGFR, ultimately leading to HF (Noma et al., 2007; Nagashio et al., 2021).
3 Clinical manifestations of EGFR-TKIs-related cardiotoxicity
Since the advent of EGFR-TKI drugs, their associated cardiovascular adverse events have been extensively documented, including prolonged QT intervals, HF, atrial fibrillation (AF), myocardial infarction (MI), pericardial effusion, hypertension, and cardiac valvular lesions (Figure 2) (Lenneman and Sawyer, 2016; Kenigsberg et al., 2017; Chitturi et al., 2022).
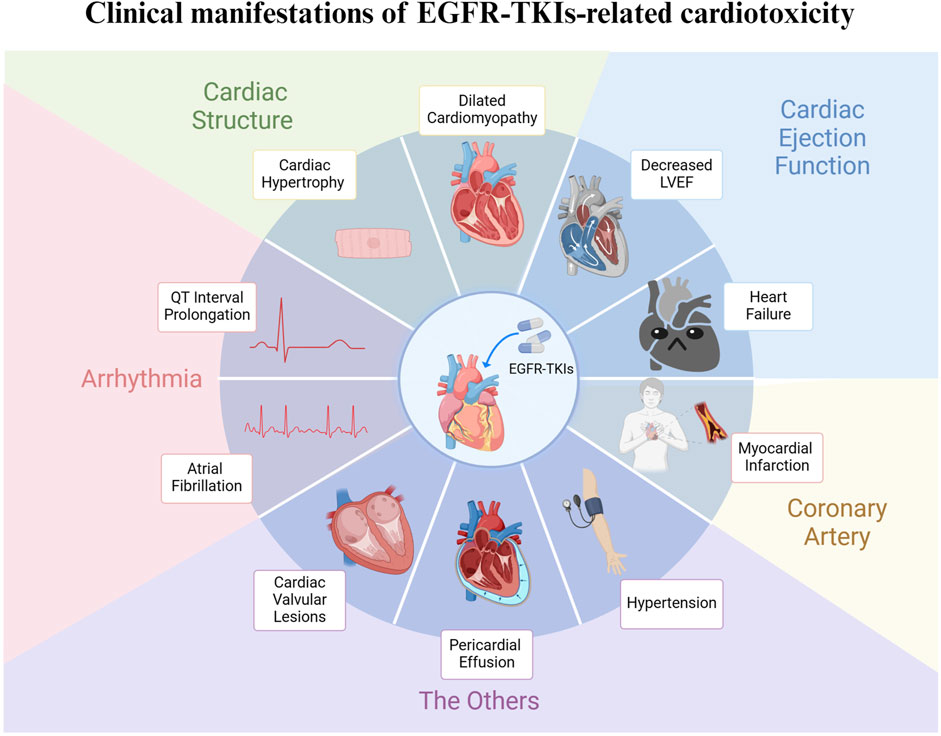
Figure 2. Clinical manifestations of EGFR-TKIs-related cardiotoxicity. The toxic effects of EGFR-TKIs on the heart have different clinical manifestations, which can be roughly divided into the following aspects. Effects on cardiac structure: myocardial hypertrophy, dilated cardiomyopathy; The effects on cardiac ejection function: decreased left ventricular ejection fraction (LVEF), heart failure; The effects on cardiac rhythm: QT interval prolongation, atrial fibrillation, etc.; The effects on coronary artery: myocardial infarction; Others: pericardial effusion, cardiac valvular lesions, hypertension, etc.
3.1 QT interval prolongation
Extended QT intervals pose an elevated risk for severe arrhythmias, including torsade de pointes (TdP), a potentially fatal condition precipitating sudden cardiac death (Davies et al., 2023). Sarah Waliany assessed the cardiovascular adverse event risk associated with EGFR-TKIs utilizing the WHO pharmacovigilance database VigiBase. The investigation, spanning different categories of TKIs deployed in NSCLC-targeted therapies, revealed that EGFR-TKIs escalated the odds of QT interval prolongation relative to the complete database (ROR = 1.39, 99% CI: 1.08–1.80, IC025 = 0.18). Intriguingly, when contrasted with other targeted therapies, EGFR-TKIs demonstrated a decreased likelihood of QT interval prolongation (ROR = 0.26, 99% CI: 0.19–0.35).
Within the realm of EGFR-TKIs, the third-generation variant, osimertinib, exhibited substantially higher rates of QT interval prolongation compared to other EGFR-TKIs (ROR = 49.19, 99% CI: 25.88–93.47), other targeted NSCLC therapies (ROR = 6.13, 99% CI: 4.43–8.48), and the overall VigiBase database (ROR = 14.34, 99% CI: 10.79–19.04, IC025 = 3.38). Importantly, osimertinib manifested significantly increased odds of QT interval prolongation, with a median onset of 29.3 days, suggesting a robust correlation between osimertinib use and QT interval prolongation, with a 49-fold higher incidence compared to other EGFR-TKIs (Waliany et al., 2021).
Given that osimertinib is the recommended first-line medication for patients with EGFR-mutated NSCLC according to international guidelines (Piper-Vallillo and Sequist, 2019), vigilance against cardiac QT interval prolongation during treatment is crucial, especially for patients with a history of QT prolongation or concurrent use of other QT interval-prolonging medications (Bian et al., 2020; Maimaitituersun et al., 2023). Encouragingly, the predominant cardiac adverse effect of osimertinib has garnered attention from both oncologists and cardiologists. Numerous clinicians have reported cases of QT prolongation induced by EGFR-TKIs treatment, contributing to the evolving understanding of this phenomenon (Oyakawa et al., 2017; Schiefer et al., 2018; Matsuura et al., 2021; Guo et al., 2023).
3.2 HF and pericardial effusion
Sarah Waliany et al. selected four EGFR-TKIs in their study, namely gefitinib, erlotinib, afatinib, and osimertinib. Osimertinib exhibited a pronounced impact compared to other EGFR-TKIs, presenting a ROR of 6.75 (99% CI: 5.29–8.62) relative to other NSCLC targeted therapies (ROR = 3.64, 99% CI: 2.94–4.50) and the full database (ROR = 5.37, 99% CI: 4.42–6.54, IC025 = 2.14). Notably, osimertinib has been demonstrated to increase the risk of HF, second only to its risk of QT interval prolongation, with a median onset of 85 days (Waliany et al., 2021).
In 2015, Michael S. Ewer conducted a clinical study on afatinib, indicating no association with HF or decreased LVEF (Ewer et al., 2015). However, in 2019, Giacomo Nuvola et al. reported a case of pericardial effusion following afatinib treatment (Nuvola et al., 2019). Subsequently, in 2020, Saori Ikebe et al. documented a case of concurrent HF and QT prolongation during osimertinib treatment (Ikebe et al., 2020).
In a Phase I study of lapatinib plus trametinib in patients with KRAS-mutant colorectal, non-small cell lung, and pancreatic cancer, a total of 34 patients were enrolled in the study at six different dose levels, including 15 patients with NSCLC. The investigators found that 5 of the 34 patients had decreased LVEF. One patient permanently discontinued study therapy because LVEF dropped from 70% at baseline to 24% after nine cycles and did not improve to more than 50% within 4 weeks of treatment interruption. There was also one patient with a 21% decrease in LVEF who had multiple treatment interruptions (Huijberts et al., 2020).
3.3 AF
Wei-Ting Chang et al. conducted a comprehensive analysis of data spanning from 2001 to 2014, focusing on NSCLC patients. Utilizing a Cox proportional risk model, they discerned a significantly elevated risk of ventricular arrhythmia in patients treated with EGFR-TKIs compared to those treated with platinum analogues. Conversely, the risk of AF did not exhibit a significant change relative to patients treated with platinum analogues (Chang et al., 2023).
In a separate investigation, Kartik Anand compared the cardiotoxicity risk of osimertinib with other drugs and various EGFR-TKIs (erlotinib, afatinib, and gefitinib) using the FDA Adverse Event Reporting System (FAERS). The Risk Odds Ratio (ROR) (95% CI) of AF induced by osimertinib was 4.0 (2.8–5.8) compared to all other drugs in FAERS and 2.1 (1.3–3.5) compared to other EGFR-TKIs. These findings suggeste that osimertinib has a higher risk of AF compared to all other drugs in FAERS and other EGFR-TKIs (Anand et al., 2019).
3.4 Hypertension
A meticulous systematic review, assessing and contrasting the efficacy and safety of erlotinib combined with bevacizumab versus erlotinib monotherapy for advanced NSCLC patients, unveiled noteworthy findings: the amalgamation of erlotinib and bevacizumab showed an increased risk of hypertension (RR = 5.15; 95% CI: 3.59, 7.39; p < 0.00001) (Sakharkar and Kurup, 2023). It is well-established that hypertension stands as a prevalent adverse reaction associated with targeted vascular endothelial growth factor receptor inhibitors, comprising both monoclonal antibodies and small molecule kinase inhibitors (Brinda et al., 2016; Yang and Bu, 2016; Chaar et al., 2018; Pantazi and Tselepis, 2022). While the direct causative link between EGFR-TKI drugs in isolation and hypertension remains uncertain, existing evidence suggests the involvement of EGFR/ErbB1 in vascular remodeling (Xiong et al., 2023). Schreier et al. substantiated this by revealing targeted EGFR deficiency in vascular smooth muscle isolated from mouse aortas precipitated spontaneous cell death (Schreier et al., 2011).
3.5 MI
In 2005, K. Yamaguchi et al. documented a case of acute MI subsequent to gefitinib treatment, proposing that gefitinib might induce such events by augmenting platelet aggregation (Yamaguchi et al., 2005). In 2015, Tulay Ku et al. reported two instances of acute cardiovascular events associated with erlotinib treatment (Kus et al., 2015). In a retrospective single-center cohort study conducted in 2020 by Kei Kunimasa, the focus was on evaluating osimertinib-related cardiac adverse events within a real-world setting. This study concluded that the incidence of cardiac adverse events in patients undergoing osimertinib treatment was 4.9%, offering a detailed account of a MI case following osimertinib administration (Kunimasa et al., 2020).
3.6 Cardiac hypertrophy and cardiomyopathy
In 2016, François Pinquié et al. reported a 71-year-old female patient with metastatic NSCLC who developed dilated cardiomyopathy after 26 months of erlotinib treatment. Despite symptomatic management, the patient’s LVEF dropped to 25% (Pinquié et al., 2016). Concurrently, Hesham M. Korashy employed an in vitro rat model to explore the impact of gefitinib on the heart. The study revealed that 21 days of gefitinib treatment (20 and 30 mg/kg) in Wistar albino rats led to an increase in mRNA and protein of B-type natriuretic peptide (BNP) and beta-myosin heavy chain, indicating an increase in the expresssion of hypertrophy genes. Simultaneously, anti-hypertrophic genes were inhibited, accompanied by an increase in the percentage of mast cells (Korashy et al., 2016). In 2020, Abdullah F. AlAsmari corroborated that gefitinib can induce cardiac hypertrophy by using an adult male Wistar rat model. Interestingly, AlAsmari found that the GLP-1 receptor agonist liraglutide demonstrated potential cardioprotective effects against this hypertrophic process (AlAsmari et al., 2020).
In 2022, German E. Ramos et al. reported a clinical case of afatinib inducing Takotsubo cardiomyopathy in an NSCLC patient (Ramos et al., 2022). Subsequently, in 2023, Karishma Patel et al. presented a case of osimertinib-induced biventricular cardiomyopathy, accompanied by cardiac magnetic resonance imaging abnormalities. Following the initiation of goal-directed drug therapy for HF and the retention of osimertinib, the patient’s biventricular function returned to normal. Notably, upon subsequent administration of afatinib, a second-generation EGFR-TKI, there was no recurrence of cardiomyopathy (Patel et al., 2023).
3.7 Cardiac valvular lesions
Kei Kunimasa conducted a comprehensive statistical analysis encompassing 123 patients diagnosed with advanced NSCLC and EGFR mutations at the International Cancer Institute, Osaka, spanning the period from 2014 to 2019. Their study showed that 6 patients (4.9%) receiving osimertinib encountered serious cardiac adverse events classified as Common Terminology Criteria for Adverse Events grade 3 or higher. Notably, two of these cases were identified as valvular heart disease (Kunimasa et al., 2020).
4 Potential pathways or drugs that protect against the cardiotoxicity of EGFR-TKIs
According to Xiaoyan Cui et al., the activation of SGK1 demonstrated a counteracting effect on arrhythmias induced by TKIs such as imatinib and erlotinib (Figure 3) (Cui et al., 2022). AMPK (Adenosine 5′-monophosphate (AMP)-activated protein kinase), an AMP-dependent protein kinase, serves as a key regulator of bioenergetic metabolism expressed in various metabolism-associated organs. It can be activated by multiple stimuli, including cellular stress, exercise, and various hormones affecting cellular metabolism (Toyama et al., 2016). The activation of the AMPK pathway by lapatinib may contribute to its comparatively lower cardiotoxicity compared to other TKIs (Shell et al., 2008). Other AMPK activators, such as metformin, have been demonstrated to have protective effects against several cardiac conditions (Figure 3) (Bromage and Yellon, 2015).
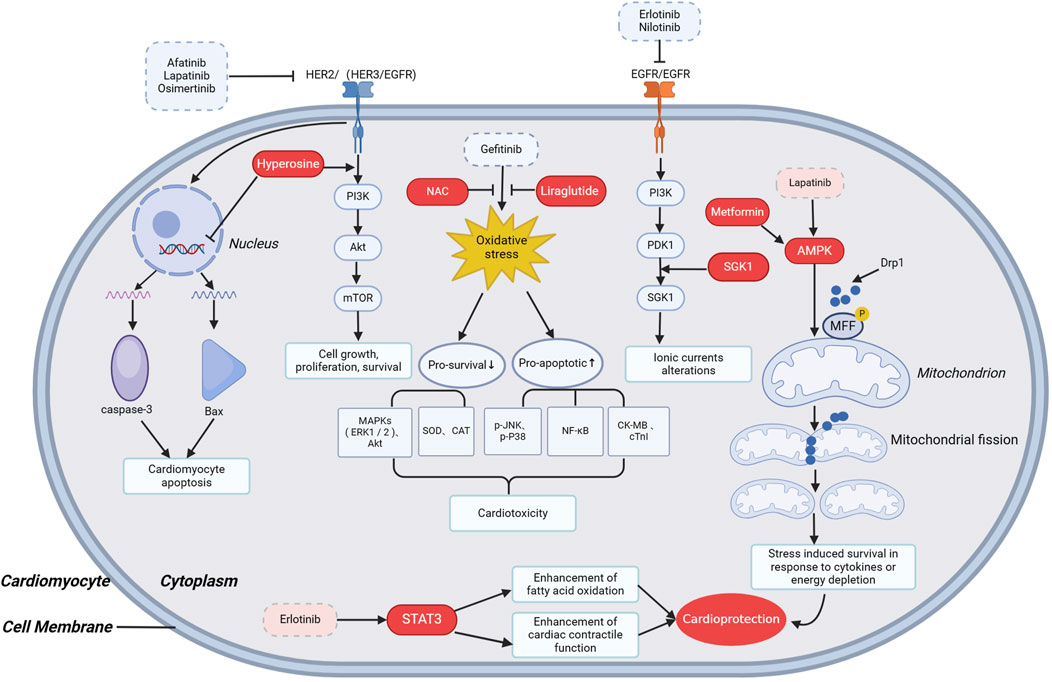
Figure 3. Potential pathways or drugs that protect against the cardiotoxicity of EGFR-TKIs. NAC, N-acetylcysteine; AMPK, Adenosine 5′-monophosphate (AMP)-activated protein kinase; MFF, Mitochondrial fission factor; STAT3, Signal transducer and activator of transcription 3; MAPK, mitogen-activated protein kinase; ERK1/2, extracellular regulated protein kinases1/2; SOD, Superoxide dismutase; CAT, Catalase; p-JNK, p-Jun N-terminal kinase; NF-ĸB, nuclear factor kappa-B; CK-MB, Creatine kinase isoenzymes-MB; cTnI, cardiac troponin I.
Erlotinib, a highly selective EGFR inhibitor with low cardiotoxicity risk, is associated with upregulation of the JAK/STAT pathway in mouse models, potentially providing cardioprotection (Figure 3) (Stuhlmiller et al., 2017). In vivo and cellular studies indicated that erlotinib treatment led to STAT3 activation, a phenomenon not observed with sorafenib and sunitinib treatment. Co-administration of erlotinib with STAT3 inhibitors resulted in a decrease in systolic function (Stuhlmiller et al., 2017).
In an experimental rat model, both metoprolol and diltiazem significantly prevented the QT-prolonging effect of pazopanib (Akman et al., 2014). Similarly, Chia-Hao Chang conducted a retrospective cohort study and found that prior β-blocker use was associated with a better prognosis in treatment-naive advanced lung adenocarcinoma patients treated with first-line EGFR-TKIs (Chang et al., 2020).
Based on a self-developed and validated computational model of cardiomyocyte apoptosis, Grabowska’s team performed network simulations of the combination treatment of sorafenib and the antioxidant N-acetylcysteine (NAC), showing that NAC protected cardiomyocytes from sorafenib-induced apoptosis. According to this, they hypothesized that ROS inhibition by NAC or other antioxidants would reduce cell apoptosis induced by TKIs such as sunitinib, ponatinib, trastuzumab, gefitinib, nilotinib, and erlotinib (Figure 3) (Grabowska et al., 2021).
According to the research conducted by Abdullah F. AlAsmari et al., gefitinib treatment resulted in an increase in oxidative stress genes, a decrease in antioxidant proteins, and a decrease in pro-survival kinases, whereas pretreatment with the GLP-receptor agonist liraglutide attenuated these effects and was cardioprotective through the activation of pro-survival kinases (Figure 3) (AlAsmari et al., 2020).
As we know, trastuzumab is a representative drug targeting HER2. Shanshan Wei et al. recently demonstrated that trastuzumab downregulated the PI3K/Akt signaling pathway in mice and H3c9 cells. Combination therapy with hypericin can effectively increase the p-Akt/Akt ratio, thereby inhibiting trastuzumab-induced cardiotoxicity (Figure 3) (Wei et al., 2023). These findings encouraged further prospective clinical studies to validate the potential of the above pathways or drugs as adjuvant anticancer therapy to attenuate cardiotoxicity.
5 Risk assessment
Cancer and cardiovascular disease share many common risk factors, with cancer itself increasing the risk for cardiovascular events (Anderson and Sawyer, 2008; Lyon et al., 2020). A comprehensive cardiovascular assessment is therefore crucial before initiating cardiotoxic EGFR-TKIs, ideally at cancer diagnosis and before starting treatment. This allows oncology teams to integrate cardiovascular risk into treatment decisions, undertake personalized monitoring and follow-up, establish appropriate treatment protocols, and refer high-risk patients to cardiac oncology services. It is crucial to emphasize that continuous cardiovascular monitoring during treatment is also vital to prevent serious adverse events (Lyon et al., 2022).
Generally, the baseline cardiovascular risk assessment for tumor patients may include the following components: clinical history (including past medical history), physical examination, serum cardiac markers, electrocardiogram (ECG), cardiac imaging, cardiovascular risk factor screening, and cardiopulmonary fitness assessment (Curigliano et al., 2016; Lyon et al., 2022). The medical history should be thorough, as previous cardiovascular conditions, chronic kidney disease, and genetic factors can elevate the risk of cardiovascular disease during treatment (Lyon et al., 2020). Physical examinations should record vital signs and identify potential signs of undiagnosed cardiovascular disease such as HF, pericardial disease, and arrhythmias. Common cardiac markers include troponin, high-sensitive cardiac troponin, BNP, and NT-proBNP. Research indicates that in patients treated with antitumor drugs, troponin can detect cardiotoxicity at a preclinical stage, well before any decrease in LVEF occurs (Cardinale et al., 2010). A baseline 12-lead ECG, an accessible and routine test, provides vital insights into potential cardiovascular diseases. The Fridericia correction (QTcF) is recommended to measure the QT interval (Porta-Sánchez et al., 2017). In the general population, the upper normal limit for QTc values is 450 ms for men and 460 ms for women, with QTc≥500 ms associated with a two-to three-fold increased risk of TdP (115). Although the incidence of QTc≥500 ms and TdP in cancer treatment is low, extending the QTc to ≥480 ms necessitates closer clinical monitoring (Zamorano et al., 2016; Herrmann, 2020; Salem et al., 2021). Echocardiography, particularly three-dimensional (3D), is the preferred method for assessing LVEF and cardiac function. LVEF below 50% is considered a risk factor for future cardiovascular diseases in most cardiotoxic cancer therapies and should warrant physician attention. When 3D ultrasound is unavailable, two-dimensional ultrasound may be considered (Santoro et al., 2017; Zhang et al., 2018; Čelutkienė et al., 2020). If ultrasound image quality is inadequate, cardiac MRI is recommended if available (Wu et al., 2009; Plana et al., 2018; Dobbin et al., 2020).
Key cardiovascular risk factors include hypertension (blood pressure >140/90 mmHg), diabetes (fasting blood glucose >126 mg/dL or HbA1c >6.5%), dyslipidemia (total cholesterol >240 mg/dL, LDL cholesterol >160 mg/dL, HDL cholesterol <40 mg/dL), obesity, smoking, sedentary lifestyle, advanced age, and others (Sharifi-Rad et al., 2020; Cheng et al., 2021). The 2022 ESC Guidelines recommend a three-monthly echocardiograph for patients taking osimertinib and advise lipid monitoring at least twice a year (Lyon et al., 2022). Additionally, factors such as timing and method of administration, potential drug interactions, individual susceptibility (including genetic abnormalities), female gender, bradycardia, electrolyte imbalances (hyper/hypokalemia, hypomagnesemia, hypocalcemia), hypothermia, hypothyroidism, and pre-existing medical conditions all contribute to the prolonged QT interval and TdP risk of drug response (Roden, 2016; Zamorano et al., 2016; Curigliano et al., 2020; Kaira et al., 2020). These factors should be thoroughly considered in the cardiac risk assessment before and during treatment with EGFR-TKIs.
6 Management and countermeasures of cardiotoxicity induced by EGFR-TKIs
Currently, there is no global consensus on the specific issue of management and countermeasures of cardiotoxicity caused by EGFR-TKIs. However, existing research and clinical experience suggest that comprehensive preventive measures, monitoring, treatment adjustments, and multidisciplinary collaboration are crucial in managing cardiotoxicity induced by EGFR-TKIs.
Educating patients to recognize and report cardiac symptoms like palpitations, chest pain, and dyspnea is crucial (Lyon et al., 2022). Medical professionals must act swiftly and appropriately when monitoring indicators show abnormalities during treatment. While cardiotoxic effects induced by EGFR-TKIs are often reversible and non-fatal after discontinuing or adjusting the medication, immediate attention is needed for life-threatening complications such as prolonged QT interval and ventricular arrhythmia. Treatment should be paused for patients with a QTc interval over 500 milliseconds on consecutive ECGs until the QTc interval falls below 481 milliseconds or returns to baseline (if baseline is ≥ 481 milliseconds), after which medication can be cautiously resumed at a reduced dose. Permanent discontinuation is necessary for patients with prolonged QTc who exhibit symptoms or signs of torsades de pointes, polymorphic ventricular tachycardia, or severe arrhythmias (Virani et al., 2016; Coppola et al., 2018; Guo et al., 2023). Despite a lack of formal consensus, clinical experience supports the efficacy of interventions like ventricular pacing and supplementation with potassium and magnesium in shortening the QT interval and terminating TdP (Fradley et al., 2021; Zeppenfeld et al., 2022; Zhang et al., 2022).
Regular echocardiography monitoring is essential, with LVEF being a crucial indicator that should ideally be above 55% for healthy individuals. Should LVEF decrease by more than 10% from baseline or fall below 50%, it is recommended to reduce, switch, or temporarily halt EGFR-TKI treatment until cardiac indicators improve (Virani et al., 2016; Lyon et al., 2020; Lyon et al., 2022). Hypertension, a prevalent cardiovascular risk factor and a possible side effect of EGFR-TKIs, demands serious attention from clinicians. For patients with cardiovascular risk factors, antihypertensive treatment should commence if blood pressure (BP) > 130/80 mmHg; for others, intervention should start at BP > 140/90 mmHg, with ACE inhibitors or ARBs as the first-line treatment. When BP > 180/110 mmHg, consideration should be given to stopping or changing cancer-targeted therapy until BP is controlled<140/90 mmHg (Lyon et al., 2022). Cancer treatment can resume, potentially at a reduced dosage, once BP stabilizes. Additionally, factors like stress, pain, excessive alcohol intake, renal impairment, untreated sleep apnea, obesity, and reduced physical activity, which can influence blood pressure, should be addressed (Jaspan et al., 2024).
Beyond these strategies, cardiac rehabilitation, which includes aerobic exercise, strength training, and educational activities, is vital for enhancing cardiac health and overall patient wellness (Viamonte et al., 2024). The emotional and psychological support provided by mental health professionals is also crucial. Finally, the effective management and prevention of cardiotoxicity associated with EGFR-TKIs rely on the collaboration among cardiologists, oncologists, pharmacists, and rehabilitation specialists. This multidisciplinary approach ensures the safety and effectiveness of the treatment and maximizes the quality of life and treatment outcomes for patients.
7 Future prospects
Future perspectives on EGFR-TKI-induced cardiotoxicity encompass more in-depth investigations and refined monitoring methods to comprehensively understand and manage this potential risk. As medical science advances, we anticipate more precise molecular biology and genetics studies that hold the promise of identifying individual characteristics predisposing patients to cardiotoxicity from EGFR-TKIs. Adopting an individualized medicine approach could empower physicians to better foresee a patient’s cardiac response and implement suitable measures, such as adjusting drug dosages or selecting alternative treatment options.
Furthermore, upcoming studies may delve into novel drug combinations or interventions aimed at mitigating the risk of cardiotoxicity associated with EGFR-TKIs, thus enhancing patient outcomes and overall survival. However, the realization of these advancements will need continuous clinical trials and rigorous monitoring to ascertain the safety and efficacy of these innovative strategies. In conclusion, the future outlook for EGFR-TKI-induced cardiotoxicity involves personalized treatment approaches, improved risk prediction, and more effective therapeutic strategies, ultimately enhancing the quality of life and survival prospects for individuals with EGFR-mutated cancers.
8 Conclusions
EGFR-TKIs currently stand out as the most effective and comparatively well-tolerated drugs for clinically treating patients with EGFR mutations. However, their use is accompanied by a significantly elevated risk of cardiotoxicity compared to traditional chemotherapeutic agents like platinum. This heightened risk often necessitates the reduction or discontinuation of EGFR-TKI treatment during targeted therapy, ultimately leading to tumor progression. Presently, the mechanisms underlying cardiotoxicity induced by EGFR-TKIs remain incompletely understood, and the distinctions in cardiotoxicity mechanisms among different EGFR-TKIs remain unclear.
Consequently, extensive research is imperative to unravel these mechanisms in the future. This endeavor is crucial not only for enhancing our understanding of the intricacies of EGFR-TKI-induced cardiotoxicity but also for providing valuable insights into the development of new drugs. Moreover, such insights can contribute to the formulation of preventive or therapeutic strategies for managing cardiac damage in patients undergoing clinical treatment with EGFR-TKIs. The pursuit of these research avenues holds the potential to advance both the safety profile of existing therapies and the development of novel interventions in the clinical landscape.
Author contributions
XL: Writing–original draft, Writing–review and editing. YL: Writing–review and editing. SL: Writing–review and editing. JH: Writing–review and editing. ZR: Writing–review and editing.
Funding
The author(s) declare that financial support was received for the research, authorship, and/or publication of this article. The author(s) declare financial support was received for the research, authorship, and/or publication of this article. The work was financially supported by Jiangsu Province Natural Science Foundation youth project (No. 20230299).
Conflict of interest
The authors declare that the research was conducted in the absence of any commercial or financial relationships that could be construed as a potential conflict of interest.
Publisher’s note
All claims expressed in this article are solely those of the authors and do not necessarily represent those of their affiliated organizations, or those of the publisher, the editors and the reviewers. Any product that may be evaluated in this article, or claim that may be made by its manufacturer, is not guaranteed or endorsed by the publisher.
References
Akman, T., Erbas, O., Akman, L., and Yilmaz, A. U. (2014). Prevention of pazopanib-induced prolonged cardiac repolarization and proarrhythmic effects. Arq. Bras. Cardiol. 103 (5), 403–409. doi:10.5935/abc.20140138
Alanazi, A., Yunusa, I., Elenizi, K., and Alzarea, A. I. (2021). Efficacy and safety of tyrosine kinase inhibitors in advanced non-small-cell lung cancer harboring epidermal growth factor receptor mutation: a network meta-analysis. Lung cancer Manag. 10 (1), Lmt43. doi:10.2217/lmt-2020-0011
AlAsmari, A. F., Ali, N., AlAsmari, F., AlAnazi, W. A., AlShammari, M. A., Al-Harbi, N. O., et al. (2020). Liraglutide attenuates gefitinib-induced cardiotoxicity and promotes cardioprotection through the regulation of MAPK/NF-κB signaling pathways. Saudi Pharm. J. 28 (4), 509–518. doi:10.1016/j.jsps.2020.03.002
Alhoshani, A., Alanazi, F. E., Alotaibi, M. R., Attwa, M. W., Kadi, A. A., Aldhfyan, A., et al. (2020). EGFR inhibitor gefitinib induces cardiotoxicity through the modulation of cardiac PTEN/Akt/FoxO3a pathway and reactive metabolites formation: in vivo and in vitro rat studies. Chem. Res. Toxicol. 33 (7), 1719–1728. doi:10.1021/acs.chemrestox.0c00005
Altamirano, J., and Bers, D. M. (2007). Voltage dependence of cardiac excitation-contraction coupling: unitary Ca2+ current amplitude and open channel probability. Circulation Res. 101 (6), 590–597. doi:10.1161/CIRCRESAHA.107.152322
Anand, K., Ensor, J., Trachtenberg, B., and Bernicker, E. H. (2019). Osimertinib-induced cardiotoxicity: a retrospective review of the FDA adverse events reporting system (FAERS). JACC CardioOncology 1 (2), 172–178. doi:10.1016/j.jaccao.2019.10.006
Anderson, B., and Sawyer, D. B. (2008). Predicting and preventing the cardiotoxicity of cancer therapy. Expert Rev. Cardiovasc. Ther. 6 (7), 1023–1033. doi:10.1586/14779072.6.7.1023
Ando, K., Wada, T., and Cao, X. (2020). Precise safety pharmacology studies of lapatinib for onco-cardiology assessed using in vivo canine models. Sci. Rep. 10 (1), 738. doi:10.1038/s41598-020-57601-x
Ballou, L. M., Lin, R. Z., and Cohen, I. S. (2015). Control of cardiac repolarization by phosphoinositide 3-kinase signaling to ion channels. Circulation Res. 116 (1), 127–137. doi:10.1161/CIRCRESAHA.116.303975
Belmonte, F., Das, S., Sysa-Shah, P., Sivakumaran, V., Stanley, B., Guo, X., et al. (2015). ErbB2 overexpression upregulates antioxidant enzymes, reduces basal levels of reactive oxygen species, and protects against doxorubicin cardiotoxicity. Am. J. physiology Heart circulatory physiology 309 (8), H1271–H1280. doi:10.1152/ajpheart.00517.2014
Bers, D. M. (2002). Cardiac excitation-contraction coupling. Nature 415 (6868), 198–205. doi:10.1038/415198a
Bers, D. M. (2014). Cardiac sarcoplasmic reticulum calcium leak: basis and roles in cardiac dysfunction. Annu. Rev. physiology 76, 107–127. doi:10.1146/annurev-physiol-020911-153308
Bian, S., Tang, X., and Lei, W. (2020). A case of torsades de pointes induced by the third-generation EGFR-TKI, osimertinib combined with moxifloxacin. BMC Pulm. Med. 20 (1), 181. doi:10.1186/s12890-020-01217-4
Brinda, B. J., Viganego, F., Vo, T., Dolan, D., and Fradley, M. G. (2016). Anti-VEGF-induced hypertension: a review of pathophysiology and treatment options. Curr. Treat. options Cardiovasc. Med. 18 (5), 33. doi:10.1007/s11936-016-0452-z
Bromage, D. I., and Yellon, D. M. (2015). The pleiotropic effects of metformin: time for prospective studies. Cardiovasc. Diabetol. 14, 109. doi:10.1186/s12933-015-0273-5
Cardinale, D., Colombo, A., Torrisi, R., Sandri, M. T., Civelli, M., Salvatici, M., et al. (2010). Trastuzumab-induced cardiotoxicity: clinical and prognostic implications of troponin I evaluation. J. Clin. Oncol. official J. Am. Soc. Clin. Oncol. 28 (25), 3910–3916. doi:10.1200/JCO.2009.27.3615
Čelutkienė, J., Pudil, R., López-Fernández, T., Grapsa, J., Nihoyannopoulos, P., Bergler-Klein, J., et al. (2020). Role of cardiovascular imaging in cancer patients receiving cardiotoxic therapies: a position statement on behalf of the heart failure association (HFA), the European association of cardiovascular imaging (EACVI) and the cardio-oncology council of the European society of cardiology (ESC). Eur. J. heart Fail. 22 (9), 1504–1524. doi:10.1002/ejhf.1957
Chaar, M., Kamta, J., and Ait-Oudhia, S. (2018). Mechanisms, monitoring, and management of tyrosine kinase inhibitors-associated cardiovascular toxicities. OncoTargets Ther. 11, 6227–6237. doi:10.2147/OTT.S170138
Chakraborty, A. D., Gonano, L. A., Munro, M. L., Smith, L. J., Thekkedam, C., Staudacher, V., et al. (2019). Activation of RyR2 by class I kinase inhibitors. Br. J. Pharmacol. 176 (6), 773–786. doi:10.1111/bph.14562
Chang, C. H., Lee, C. H., Ko, J. C., Chang, L. Y., Lee, M. C., Zhang, J. F., et al. (2020). Effect of β-blocker in treatment-naïve patients with advanced lung adenocarcinoma receiving first-generation EGFR-TKIs. Front. Oncol. 10, 583529. doi:10.3389/fonc.2020.583529
Chang, W.-T., Lin, H.-W., Chang, T.-C., Lin, S.-H., and Li, Y.-H. (2023). The association between tyrosine kinase inhibitors and fatal arrhythmia in patients with non-small cell lung cancer in Taiwan. Front. Oncol. 13, 1172036. doi:10.3389/fonc.2023.1172036
Cheng, M., Yang, F., Liu, J., Yang, D., Zhang, S., Yu, Y., et al. (2021). Tyrosine kinase inhibitors-induced arrhythmias: from molecular mechanisms, pharmacokinetics to therapeutic strategies. Front. Cardiovasc. Med. 8, 758010. doi:10.3389/fcvm.2021.758010
Chiamvimonvat, N., Chen-Izu, Y., Clancy, C. E., Deschenes, I., Dobrev, D., Heijman, J., et al. (2017). Potassium currents in the heart: functional roles in repolarization, arrhythmia and therapeutics. J. physiology 595 (7), 2229–2252. doi:10.1113/JP272883
Chitturi, K. R., Burns, E. A., Muhsen, I. N., Anand, K., and Trachtenberg, B. H. (2022). Cardiovascular risks with epidermal growth factor receptor (EGFR) tyrosine kinase inhibitors and monoclonal antibody therapy. Curr. Oncol. Rep. 24 (4), 475–491. doi:10.1007/s11912-022-01215-1
Coppola, C., Rienzo, A., Piscopo, G., Barbieri, A., Arra, C., and Maurea, N. (2018). Management of QT prolongation induced by anti-cancer drugs: target therapy and old agents. Different algorithms for different drugs. Cancer Treat. Rev. 63, 135–143. doi:10.1016/j.ctrv.2017.11.009
Cross, D. A. E., Ashton, S. E., Ghiorghiu, S., Eberlein, C., Nebhan, C. A., Spitzler, P. J., et al. (2014). AZD9291, an irreversible EGFR TKI, overcomes T790M-mediated resistance to EGFR inhibitors in lung cancer. Cancer Discov. 4 (9), 1046–1061. doi:10.1158/2159-8290.CD-14-0337
Cui, X., Sun, J., Li, C., Qiu, S., Shi, C., Ma, J., et al. (2022). Downregulation of hERG channel expression by tyrosine kinase inhibitors nilotinib and vandetanib predominantly contributes to arrhythmogenesis. Toxicol. Lett. 365, 11–23. doi:10.1016/j.toxlet.2022.06.001
Curigliano, G., Cardinale, D., Dent, S., Criscitiello, C., Aseyev, O., Lenihan, D., et al. (2016). Cardiotoxicity of anticancer treatments: epidemiology, detection, and management. CA a cancer J. Clin. 66 (4), 309–325. doi:10.3322/caac.21341
Curigliano, G., Lenihan, D., Fradley, M., Ganatra, S., Barac, A., Blaes, A., et al. (2020). Management of cardiac disease in cancer patients throughout oncological treatment: ESMO consensus recommendations. Ann. Oncol. official J. Eur. Soc. Med. Oncol. 31 (2), 171–190. doi:10.1016/j.annonc.2019.10.023
Davies, R. A., Ladouceur, V. B., Green, M. S., Joza, J., Juurlink, D. N., Krahn, A. D., et al. (2023). The 2023 Canadian cardiovascular society clinical practice update on management of the patient with a prolonged QT interval. Can. J. Cardiol. 39 (10), 1285–1301. doi:10.1016/j.cjca.2023.06.011
Dobbin, S. J. H., Mangion, K., Berry, C., Roditi, G., Basak, S., Sourbron, S., et al. (2020). Cardiotoxicity and myocardial hypoperfusion associated with anti-vascular endothelial growth factor therapies: prospective cardiac magnetic resonance imaging in patients with cancer. Eur. J. heart Fail. 22 (7), 1276–1277. doi:10.1002/ejhf.1847
Dridi, H., Kushnir, A., Zalk, R., Yuan, Q., Melville, Z., and Marks, A. R. (2020). Intracellular calcium leak in heart failure and atrial fibrillation: a unifying mechanism and therapeutic target. Nat. Rev. Cardiol. 17 (11), 732–747. doi:10.1038/s41569-020-0394-8
Du, B., Chakraborty, P., Azam, M. A., Massé, S., Lai, P. F. H., Niri, A., et al. (2020). Acute effects of ibrutinib on ventricular arrhythmia in spontaneously hypertensive rats. JACC CardioOncology 2 (4), 614–629. doi:10.1016/j.jaccao.2020.08.012
Eldridge, S., Guo, L., Mussio, J., Furniss, M., Hamre, J., and Davis, M. (2014). Examining the protective role of ErbB2 modulation in human-induced pluripotent stem cell-derived cardiomyocytes. Toxicol. Sci. official J. Soc. Toxicol. 141 (2), 547–559. doi:10.1093/toxsci/kfu150
Ewer, M. S., Patel, K., O'Brien, D., and Lorence, R. M. (2015). Cardiac safety of afatinib: a review of data from clinical trials. Cardio-oncology Lond. Engl. 1 (1), 3. doi:10.1186/s40959-015-0006-7
Fox, D. B., Garcia, N. M. G., McKinney, B. J., Lupo, R., Noteware, L. C., Newcomb, R., et al. (2020). NRF2 activation promotes the recurrence of dormant tumour cells through regulation of redox and nucleotide metabolism. Nat. Metab. 2 (4), 318–334. doi:10.1038/s42255-020-0191-z
Fradley, M. G., Beckie, T. M., Brown, S. A., Cheng, R. K., Dent, S. F., Nohria, A., et al. (2021). Recognition, prevention, and management of arrhythmias and autonomic disorders in cardio-oncology: a scientific statement from the American heart association. Circulation 144 (3), e41–e55. doi:10.1161/CIR.0000000000000986
Frisk, M., Le, C., Shen, X., Røe, Å. T., Hou, Y., Manfra, O., et al. (2021). Etiology-dependent impairment of diastolic cardiomyocyte calcium homeostasis in heart failure with preserved ejection fraction. J. Am. Coll. Cardiol. 77 (4), 405–419. doi:10.1016/j.jacc.2020.11.044
Gettinger, S., and Lynch, T. (2011). A decade of advances in treatment for advanced non-small cell lung cancer. Clin. chest Med. 32 (4), 839–851. doi:10.1016/j.ccm.2011.08.017
Gocek, E., Moulas, A. N., and Studzinski, G. P. (2014). Non-receptor protein tyrosine kinases signaling pathways in normal and cancer cells. Crit. Rev. Clin. laboratory Sci. 51 (3), 125–137. doi:10.3109/10408363.2013.874403
Gordon, L. I., Burke, M. A., Singh, A. T., Prachand, S., Lieberman, E. D., Sun, L., et al. (2009). Blockade of the erbB2 receptor induces cardiomyocyte death through mitochondrial and reactive oxygen species-dependent pathways. J. Biol. Chem. 284 (4), 2080–2087. doi:10.1074/jbc.M804570200
Grabowska, M. E., Chun, B., Moya, R., and Saucerman, J. J. (2021). Computational model of cardiomyocyte apoptosis identifies mechanisms of tyrosine kinase inhibitor-induced cardiotoxicity. J. Mol. cellular Cardiol. 155, 66–77. doi:10.1016/j.yjmcc.2021.02.014
Grazette, L. P., Boecker, W., Matsui, T., Semigran, M., Force, T. L., Hajjar, R. J., et al. (2004). Inhibition of ErbB2 causes mitochondrial dysfunction in cardiomyocytes: implications for herceptin-induced cardiomyopathy. J. Am. Coll. Cardiol. 44 (11), 2231–2238. doi:10.1016/j.jacc.2004.08.066
Grego-Bessa, J., Gómez-Apiñaniz, P., Prados, B., Gómez, M. J., MacGrogan, D., and de la Pompa, J. L. (2023). Nrg1 regulates cardiomyocyte migration and cell cycle in ventricular development. Circulation Res. 133 (11), 927–943. doi:10.1161/CIRCRESAHA.123.323321
Guo, G. G., Luo, X., Zhu, K., Li, L. L., and Ou, Y. F. (2023). Fatal ventricular arrhythmias after osimertinib treatment for lung adenocarcinoma: a case report. J. geriatric Cardiol. JGC 20 (3), 242–246. doi:10.26599/1671-5411.2023.03.009
Harrison, P. T., Vyse, S., and Huang, P. H. (2020). Rare epidermal growth factor receptor (EGFR) mutations in non-small cell lung cancer. Seminars cancer Biol. 61, 167–179. doi:10.1016/j.semcancer.2019.09.015
Helliwell, M. V., Zhang, Y., El Harchi, A., Dempsey, C. E., and Hancox, J. C. (2023). Inhibition of the hERG potassium channel by a methanesulphonate-free E-4031 analogue. Pharm. (Basel, Switz.) 16 (9), 1204. doi:10.3390/ph16091204
Herrmann, J. (2020). Adverse cardiac effects of cancer therapies: cardiotoxicity and arrhythmia. Nat. Rev. Cardiol. 17 (8), 474–502. doi:10.1038/s41569-020-0348-1
Hervent, A. S., and De Keulenaer, G. W. (2012). Molecular mechanisms of cardiotoxicity induced by ErbB receptor inhibitor cancer therapeutics. Int. J. Mol. Sci. 13 (10), 12268–12286. doi:10.3390/ijms131012268
Hsu, W. T., Huang, C. Y., Yen, C. Y. T., Cheng, A. L., and Hsieh, P. C. H. (2018). The HER2 inhibitor lapatinib potentiates doxorubicin-induced cardiotoxicity through iNOS signaling. Theranostics 8 (12), 3176–3188. doi:10.7150/thno.23207
Huijberts, S., van Geel, R., van Brummelen, E. M. J., Opdam, F. L., Marchetti, S., Steeghs, N., et al. (2020). Phase I study of lapatinib plus trametinib in patients with KRAS-mutant colorectal, non-small cell lung, and pancreatic cancer. Cancer Chemother. Pharmacol. 85 (5), 917–930. doi:10.1007/s00280-020-04066-4
Hutchings, D. C., Madders, G. W. P., Niort, B. C., Bode, E. F., Waddell, C. A., Woods, L. S., et al. (2022). Interaction of background Ca(2+) influx, sarcoplasmic reticulum threshold and heart failure in determining propensity for Ca(2+) waves in sheep heart. J. physiology 600 (11), 2637–2650. doi:10.1113/JP282168
Ikebe, S., Amiya, R., Minami, S., Ihara, S., Higuchi, Y., and Komuta, K. (2020). Osimertinib-induced cardiac failure with QT prolongation and torsade de pointes in a patient with advanced pulmonary adenocarcinoma. Int. cancer Conf. J. 10 (1), 68–71. doi:10.1007/s13691-020-00450-2
Jaspan, V. N., Greenberg, G. S., Parihar, S., Park, C. M., Somers, V. K., Shapiro, M. D., et al. (2024). The role of sleep in cardiovascular disease. Curr. Atheroscler. Rep. 26, 249–262. doi:10.1007/s11883-024-01207-5
Jie, L. J., Li, Y. D., Zhang, H. Q., Mao, L., Xie, H. B., Zhou, F. G., et al. (2021). Mechanisms of gefitinib-induced QT prolongation. Eur. J. Pharmacol. 910, 174441. doi:10.1016/j.ejphar.2021.174441
Kaira, K., Ogiwara, Y., and Naruse, I. (2020). Occurrence of ventricular fibrillation in a patient with lung cancer receiving osimertinib. J. Thorac. Oncol. official Publ. Int. Assoc. Study Lung Cancer 15 (4), e54–e55. doi:10.1016/j.jtho.2019.11.029
Kanazawa, S., Yamaguchi, K., Kinoshita, Y., Muramatsu, M., Komiyama, Y., and Nomura, S. (2006). Aspirin reduces adverse effects of gefitinib. Anti-cancer drugs 17 (4), 423–427. doi:10.1097/01.cad.0000203385.45163.76
Kawakami, A., Tanaka, A., Chiba, T., Nakajima, K., Shimokado, K., and Yoshida, M. (2003). Remnant lipoprotein-induced smooth muscle cell proliferation involves epidermal growth factor receptor transactivation. Circulation 108 (21), 2679–2688. doi:10.1161/01.CIR.0000093278.75565.87
Kenigsberg, B., Jain, V., and Barac, A. (2017). Cardio-oncology related to heart failure: epidermal growth factor receptor target-based therapy. Heart Fail. Clin. 13 (2), 297–309. doi:10.1016/j.hfc.2016.12.002
Kim, J. J., Němec, J., Li, Q., and Salama, G. (2015). Synchronous systolic subcellular Ca2+-elevations underlie ventricular arrhythmia in drug-induced long QT type 2. Circulation Arrhythmia Electrophysiol. 8 (3), 703–712. doi:10.1161/CIRCEP.114.002214
Kopljar, I., Lu, H. R., Van Ammel, K., Otava, M., Tekle, F., Teisman, A., et al. (2018). Development of a human iPSC cardiomyocyte-based scoring system for cardiac hazard identification in early drug safety de-risking. Stem cell Rep. 11 (6), 1365–1377. doi:10.1016/j.stemcr.2018.11.007
Korashy, H. M., Attafi, I. M., Ansari, M. A., Assiri, M. A., Belali, O. M., Ahmad, S. F., et al. (2016). Molecular mechanisms of cardiotoxicity of gefitinib in vivo and in vitro rat cardiomyocyte: role of apoptosis and oxidative stress. Toxicol. Lett. 252, 50–61. doi:10.1016/j.toxlet.2016.04.011
Kunimasa, K., Kamada, R., Oka, T., Oboshi, M., Kimura, M., Inoue, T., et al. (2020). Cardiac adverse events in EGFR-mutated non-small cell lung cancer treated with osimertinib. JACC CardioOncology 2 (1), 1–10. doi:10.1016/j.jaccao.2020.02.003
Kus, T., Aktas, G., Sevinc, A., Kalender, M. E., and Camci, C. (2015). Could erlotinib treatment lead to acute cardiovascular events in patients with lung adenocarcinoma after chemotherapy failure? OncoTargets Ther. 8, 1341–1343. doi:10.2147/OTT.S84480
Kwapiszewski, R., Pawlak, S. D., and Adamkiewicz, K. (2016). Anti-EGFR agents: current status, forecasts and future directions. Target. Oncol. 11 (6), 739–752. doi:10.1007/s11523-016-0456-3
Leemasawat, K., Phrommintikul, A., Chattipakorn, S. C., and Chattipakorn, N. (2020). Mechanisms and potential interventions associated with the cardiotoxicity of ErbB2-targeted drugs: insights from in vitro, in vivo, and clinical studies in breast cancer patients. Cell. Mol. life Sci. CMLS 77 (8), 1571–1589. doi:10.1007/s00018-019-03340-w
Lenneman, C. G., and Sawyer, D. B. (2016). Cardio-oncology: an update on cardiotoxicity of cancer-related treatment. Circulation Res. 118 (6), 1008–1020. doi:10.1161/CIRCRESAHA.115.303633
Li, D., Ambrogio, L., Shimamura, T., Kubo, S., Takahashi, M., Chirieac, L. R., et al. (2008). BIBW2992, an irreversible EGFR/HER2 inhibitor highly effective in preclinical lung cancer models. Oncogene 27 (34), 4702–4711. doi:10.1038/onc.2008.109
Li, P., Tian, X., Wang, G., Jiang, E., Li, Y., and Hao, G. (2023). Acute osimertinib exposure induces electrocardiac changes by synchronously inhibiting the currents of cardiac ion channels. Front. Pharmacol. 14, 1177003. doi:10.3389/fphar.2023.1177003
London, B., Baker, L. C., Lee, J. S., Shusterman, V., Choi, B. R., Kubota, T., et al. (2003). Calcium-dependent arrhythmias in transgenic mice with heart failure. Am. J. physiology Heart circulatory physiology 284 (2), H431–H441. doi:10.1152/ajpheart.00431.2002
Lu, H. R., Whittaker, R., Price, J. H., Vega, R., Pfeiffer, E. R., Cerignoli, F., et al. (2015). High throughput measurement of Ca++ dynamics in human stem cell-derived cardiomyocytes by kinetic image cytometery: a cardiac risk assessment characterization using a large panel of cardioactive and inactive compounds. Toxicol. Sci. official J. Soc. Toxicol. 148 (2), 503–516. doi:10.1093/toxsci/kfv201
Lu, Z., Wu, C. Y., Jiang, Y. P., Ballou, L. M., Clausen, C., Cohen, I. S., et al. (2012). Suppression of phosphoinositide 3-kinase signaling and alteration of multiple ion currents in drug-induced long QT syndrome. Sci. Transl. Med. 4 (131), 131ra50. doi:10.1126/scitranslmed.3003623
Lynch, D. R., Kickler, T. S., and Rade, J. J. (2011). Recurrent myocardial infarction associated with gefitinib therapy. J. thrombosis thrombolysis 32 (1), 120–124. doi:10.1007/s11239-010-0539-4
Lyon, A. R., Dent, S., Stanway, S., Earl, H., Brezden-Masley, C., Cohen-Solal, A., et al. (2020). Baseline cardiovascular risk assessment in cancer patients scheduled to receive cardiotoxic cancer therapies: a position statement and new risk assessment tools from the Cardio-Oncology Study Group of the Heart Failure Association of the European Society of Cardiology in collaboration with the International Cardio-Oncology Society. Eur. J. heart Fail. 22 (11), 1945–1960. doi:10.1002/ejhf.1920
Lyon, A. R., López-Fernández, T., Couch, L. S., Asteggiano, R., Aznar, M. C., Bergler-Klein, J., et al. (2022). 2022 ESC guidelines on cardio-oncology developed in collaboration with the European hematology association (EHA), the European society for therapeutic radiology and oncology (ESTRO) and the international cardio-oncology society (IC-OS). Eur. heart J. 43 (41), 4229–4361. doi:10.1093/eurheartj/ehac244
Maimaitituersun, G., Abulimiti, B., Jin, M., Dong, X., and Fu, Z. (2023). Gefitinib increases the incidence of QT prolongation in patients with non-small cell lung cancer. Int. Heart J. 64 (3), 365–373. doi:10.1536/ihj.22-583
Majeed, U., Manochakian, R., Zhao, Y., and Lou, Y. (2021). Targeted therapy in advanced non-small cell lung cancer: current advances and future trends. J. Hematol. and Oncol. 14 (1), 108. doi:10.1186/s13045-021-01121-2
Mak, I. T., Kramer, J. H., Chmielinska, J. J., Spurney, C. F., and Weglicki, W. B. (2015). EGFR-TKI, erlotinib, causes hypomagnesemia, oxidative stress, and cardiac dysfunction: attenuation by NK-1 receptor blockade. J. Cardiovasc. Pharmacol. 65 (1), 54–61. doi:10.1097/FJC.0000000000000163
Matsuura, C., Kato, T., and Koyama, K. (2021). Successful management of refractory torsades de pointes due to drug-induced long QT syndrome guided by point-of-care monitoring of ionized magnesium. Cureus 13 (3), e13939. doi:10.7759/cureus.13939
Miyagawa, J., Higashiyama, S., Kawata, S., Inui, Y., Tamura, S., Yamamoto, K., et al. (1995). Localization of heparin-binding EGF-like growth factor in the smooth muscle cells and macrophages of human atherosclerotic plaques. J. Clin. investigation 95 (1), 404–411. doi:10.1172/JCI117669
Mukasa, A., Wykosky, J., Ligon, K. L., Chin, L., Cavenee, W. K., and Furnari, F. (2010). Mutant EGFR is required for maintenance of glioma growth in vivo, and its ablation leads to escape from receptor dependence. Proc. Natl. Acad. Sci. United States of America 107 (6), 2616–2621. doi:10.1073/pnas.0914356107
Nagashio, K., Tajiri, K., Sato, K., and Ieda, M. (2021). Erlotinib-induced cardiomyopathy in a patient with metastatic non-small cell lung cancer. Int. Heart J. 62 (5), 1171–1175. doi:10.1536/ihj.21-130
Noma, T., Lemaire, A., Naga Prasad, S. V., Barki-Harrington, L., Tilley, D. G., Chen, J., et al. (2007). Beta-arrestin-mediated beta1-adrenergic receptor transactivation of the EGFR confers cardioprotection. J. Clin. investigation 117 (9), 2445–2458. doi:10.1172/JCI31901
Nuvola, G., Dall'Olio, F. G., Melotti, B., Sperandi, F., and Ardizzoni, A. (2019). Cardiac toxicity from afatinib in EGFR-mutated NSCLC: a rare but possible side effect. J. Thorac. Oncol. official Publ. Int. Assoc. Study Lung Cancer 14 (7), e145–e6. doi:10.1016/j.jtho.2019.02.027
Odiete, O., Hill, M. F., and Sawyer, D. B. (2012). Neuregulin in cardiovascular development and disease. Circulation Res. 111 (10), 1376–1385. doi:10.1161/CIRCRESAHA.112.267286
Oyakawa, T., Nakashima, K., and Naito, T. (2017). Cardiac dysfunction caused by osimertinib. J. Thorac. Oncol. official Publ. Int. Assoc. Study Lung Cancer 12 (10), e159–e160. doi:10.1016/j.jtho.2017.05.016
Pantazi, D., and Tselepis, A. D. (2022). Cardiovascular toxic effects of antitumor agents: pathogenetic mechanisms. Thrombosis Res. 213, S95–S102. doi:10.1016/j.thromres.2021.12.017
Patel, K., Hsu, K. Y., Lou, K., Soni, K., Lee, Y. J., Mulvey, C. K., et al. (2023). Osimertinib-induced biventricular cardiomyopathy with abnormal cardiac MRI findings: a case report. Cardio-oncology Lond. Engl. 9 (1), 38. doi:10.1186/s40959-023-00190-1
Pinquié, F., de Chabot, G., Urban, T., and Hureaux, J. (2016). Maintenance treatment by erlotinib and toxic cardiomyopathy: a case report. Oncology 90 (3), 176–177. doi:10.1159/000444186
Piper-Vallillo, A. J., and Sequist, L. V. (2019). Cardiac risk-informed treatment of EGFR-mutant lung cancer with osimertinib. JACC CardioOncology 1 (2), 179–181. doi:10.1016/j.jaccao.2019.11.011
Plana, J. C., Thavendiranathan, P., Bucciarelli-Ducci, C., and Lancellotti, P. (2018). Multi-modality imaging in the assessment of cardiovascular toxicity in the cancer patient. JACC Cardiovasc. imaging 11 (8), 1173–1186. doi:10.1016/j.jcmg.2018.06.003
Pogwizd, S. M., Schlotthauer, K., Li, L., Yuan, W., and Bers, D. M. (2001). Arrhythmogenesis and contractile dysfunction in heart failure: roles of sodium-calcium exchange, inward rectifier potassium current, and residual beta-adrenergic responsiveness. Circulation Res. 88 (11), 1159–1167. doi:10.1161/hh1101.091193
Porta-Sánchez, A., Gilbert, C., Spears, D., Amir, E., Chan, J., Nanthakumar, K., et al. (2017). Incidence, diagnosis, and management of QT prolongation induced by cancer therapies: a systematic review. J. Am. Heart Assoc. 6 (12), e007724. doi:10.1161/JAHA.117.007724
Ramos, G. E., Caglevic, C., Bulnes, J. F., Panay, S. E., Zapata, M. I., Daniele, A. J., et al. (2022). Takotsubo cardiomyopathy Afatinib-related in a non-small cell lung cancer patient: case report. Front. Cardiovasc. Med. 9, 1060813. doi:10.3389/fcvm.2022.1060813
Rana, P., Anson, B., Engle, S., and Will, Y. (2012). Characterization of human-induced pluripotent stem cell-derived cardiomyocytes: bioenergetics and utilization in safety screening. Toxicol. Sci. official J. Soc. Toxicol. 130 (1), 117–131. doi:10.1093/toxsci/kfs233
Remon, J., and Planchard, D. (2015). AZD9291 in EGFR-mutant advanced non-small-cell lung cancer patients. Future Oncol. 11 (22), 3069–3081. doi:10.2217/fon.15.250
Roden, D. M. (2016). Predicting drug-induced QT prolongation and torsades de pointes. J. physiology 594 (9), 2459–2468. doi:10.1113/JP270526
Rohrbach, S., Muller-Werdan, U., Werdan, K., Koch, S., Gellerich, N. F., and Holtz, J. (2005). Apoptosis-modulating interaction of the neuregulin/erbB pathway with anthracyclines in regulating Bcl-xS and Bcl-xL in cardiomyocytes. J. Mol. cellular Cardiol. 38 (3), 485–493. doi:10.1016/j.yjmcc.2004.12.013
Sakharkar, P., and Kurup, S. (2023). Comparing efficacy of erlotinib and bevacizumab combination with erlotinib monotherapy in patients with advanced non-small cell lung cancer (NSCLC): a systematic review and meta-analysis. Dis. (Basel, Switz.) 11 (4), 146. doi:10.3390/diseases11040146
Salem, J. E., Nguyen, L. S., Moslehi, J. J., Ederhy, S., Lebrun-Vignes, B., Roden, D. M., et al. (2021). Anticancer drug-induced life-threatening ventricular arrhythmias: a World Health Organization pharmacovigilance study. Eur. heart J. 42 (38), 3915–3928. doi:10.1093/eurheartj/ehab362
Santoro, C., Arpino, G., Esposito, R., Lembo, M., Paciolla, I., Cardalesi, C., et al. (2017). 2D and 3D strain for detection of subclinical anthracycline cardiotoxicity in breast cancer patients: a balance with feasibility. Eur. heart J. Cardiovasc. Imaging 18 (8), 930–936. doi:10.1093/ehjci/jex033
Schiefer, M., Hendriks, L. E. L., Dinh, T., Lalji, U., and Dingemans, A.-M. C. (2018). Current perspective: osimertinib-induced QT prolongation: new drugs with new side-effects need careful patient monitoring. Eur. J. Cancer 91, 92–98. doi:10.1016/j.ejca.2017.12.011
Schreier, B., Döhler, M., Rabe, S., Schneider, B., Schwerdt, G., Ruhs, S., et al. (2011). Consequences of epidermal growth factor receptor (ErbB1) loss for vascular smooth muscle cells from mice with targeted deletion of ErbB1. Arteriosclerosis, thrombosis, Vasc. Biol. 31 (7), 1643–1652. doi:10.1161/ATVBAHA.111.223537
Sharifi-Rad, J., Rodrigues, C. F., Sharopov, F., Docea, A. O., Can Karaca, A., Sharifi-Rad, M., et al. (2020). Diet, lifestyle and cardiovascular diseases: linking pathophysiology to cardioprotective effects of natural bioactive compounds. Int. J. Environ. Res. public health 17 (7), 2326. doi:10.3390/ijerph17072326
Shell, S. A., Lyass, L., Trusk, P. B., Pry, K. J., Wappel, R. L., and Bacus, S. S. (2008). Activation of AMPK is necessary for killing cancer cells and sparing cardiac cells. Cell cycle (Georgetown, Tex) 7 (12), 1769–1775. doi:10.4161/cc.7.12.6016
Shi, Y., Au, J. S.-K., Thongprasert, S., Srinivasan, S., Tsai, C.-M., Khoa, M. T., et al. (2014). A prospective, molecular epidemiology study of EGFR mutations in asian patients with advanced non–small-cell lung cancer of adenocarcinoma histology (PIONEER). J. Thorac. Oncol. 9 (2), 154–162. doi:10.1097/JTO.0000000000000033
Siegel, R., DeSantis, C., Virgo, K., Stein, K., Mariotto, A., Smith, T., et al. (2012). Cancer treatment and survivorship statistics, 2012. CA a cancer J. Clin. 62 (4), 220–241. doi:10.3322/caac.21149
Stuhlmiller, T. J., Zawistowski, J. S., Chen, X., Sciaky, N., Angus, S. P., Hicks, S. T., et al. (2017). Kinome and transcriptome profiling reveal broad and distinct activities of erlotinib, sunitinib, and sorafenib in the mouse heart and suggest cardiotoxicity from combined signal transducer and activator of transcription and epidermal growth factor receptor inhibition. J. Am. Heart Assoc. 6 (10), e006635. doi:10.1161/JAHA.117.006635
Sun, L., Wang, H., Xu, D., Yu, S., Zhang, L., and Li, X. (2022). Lapatinib induces mitochondrial dysfunction to enhance oxidative stress and ferroptosis in doxorubicin-induced cardiomyocytes via inhibition of PI3K/AKT signaling pathway. Bioengineered 13 (1), 48–60. doi:10.1080/21655979.2021.2004980
Timolati, F., Ott, D., Pentassuglia, L., Giraud, M. N., Perriard, J. C., Suter, T. M., et al. (2006). Neuregulin-1 beta attenuates doxorubicin-induced alterations of excitation-contraction coupling and reduces oxidative stress in adult rat cardiomyocytes. J. Mol. cellular Cardiol. 41 (5), 845–854. doi:10.1016/j.yjmcc.2006.08.002
Toyama, E. Q., Herzig, S., Courchet, J., Lewis, T. L., Losón, O. C., Hellberg, K., et al. (2016). Metabolism. AMP-activated protein kinase mediates mitochondrial fission in response to energy stress. Sci. (New York, NY) 351 (6270), 275–281. doi:10.1126/science.aab4138
Viamonte, S. G., Tavares, A., Alves, A. J., Joaquim, A., Vilela, E., Capela, A., et al. (2024). Cost-effectiveness analysis of a cardio-oncology rehabilitation framework compared to an exercise intervention for cancer survivors with high cardiovascular risk. Eur. J. Prev. Cardiol., zwae181. doi:10.1093/eurjpc/zwae181
Virani, S. A., Dent, S., Brezden-Masley, C., Clarke, B., Davis, M. K., Jassal, D. S., et al. (2016). Canadian cardiovascular society guidelines for evaluation and management of cardiovascular complications of cancer therapy. Can. J. Cardiol. 32 (7), 831–841. doi:10.1016/j.cjca.2016.02.078
Waliany, S., Zhu, H., Wakelee, H., Padda, S. K., Das, M., Ramchandran, K., et al. (2021). Pharmacovigilance analysis of cardiac toxicities associated with targeted therapies for metastatic NSCLC. J. Thorac. Oncol. official Publ. Int. Assoc. Study Lung Cancer 16 (12), 2029–2039. doi:10.1016/j.jtho.2021.07.030
Wang, H., Wang, Y., Li, J., He, Z., Boswell, S. A., Chung, M., et al. (2023). Three tyrosine kinase inhibitors cause cardiotoxicity by inducing endoplasmic reticulum stress and inflammation in cardiomyocytes. BMC Med. 21 (1), 147. doi:10.1186/s12916-023-02838-2
Wang, Y., Zhou, Q., Liu, C., Zhang, R., Xing, B., Du, J., et al. (2024). Targeting IL-6/STAT3 signaling abrogates EGFR-TKI resistance through inhibiting Beclin-1 dependent autophagy in HNSCC. Cancer Lett. 586, 216612. doi:10.1016/j.canlet.2024.216612
Wei, S., Ma, W., Xie, S., Liu, S., Xie, N., Li, W., et al. (2023). Hyperoside protects trastuzumab-induced cardiotoxicity via activating the PI3K/Akt signaling pathway. Cardiovasc. drugs Ther. doi:10.1007/s10557-023-07522-4
Wu, C. F., Chuang, W. P., Li, A. H., and Hsiao, C. H. (2009). Cardiac magnetic resonance imaging in sunitinib malate-related cardiomyopathy: no late gadolinium enhancement. J. Chin. Med. Assoc. JCMA 72 (6), 323–327. doi:10.1016/S1726-4901(09)70379-X
Xiong, Y., Wang, Y., Yang, T., Luo, Y., Xu, S., and Li, L. (2023). Receptor tyrosine kinase: still an interesting target to inhibit the proliferation of vascular smooth muscle cells. Am. J. Cardiovasc. drugs drugs, devices, other interventions 23 (5), 497–518. doi:10.1007/s40256-023-00596-3
Yamaguchi, K., Kanazawa, S., Kinoshita, Y., Muramatsu, M., and Nomura, S. (2005). Acute myocardial infarction with lung cancer during treatment with gefitinib: the possibility of gefitinib-induced thrombosis. Pathophysiol. haemostasis thrombosis 34 (1), 48–50. doi:10.1159/000088548
Yang, K. C., and Nerbonne, J. M. (2016). Mechanisms contributing to myocardial potassium channel diversity, regulation and remodeling. Trends Cardiovasc. Med. 26 (3), 209–218. doi:10.1016/j.tcm.2015.07.002
Yang, T., Chun, Y. W., Stroud, D. M., Mosley, J. D., Knollmann, B. C., Hong, C., et al. (2014). Screening for acute IKr block is insufficient to detect torsades de pointes liability: role of late sodium current. Circulation 130 (3), 224–234. doi:10.1161/CIRCULATIONAHA.113.007765
Yang, Y., and Bu, P. (2016). Progress on the cardiotoxicity of sunitinib: prognostic significance, mechanism and protective therapies. Chemico-biological Interact. 257, 125–131. doi:10.1016/j.cbi.2016.08.006
Yeh, E. T., and Bickford, C. L. (2009). Cardiovascular complications of cancer therapy: incidence, pathogenesis, diagnosis, and management. J. Am. Coll. Cardiol. 53 (24), 2231–2247. doi:10.1016/j.jacc.2009.02.050
Yuan, M., Huang, L. L., Chen, J. H., Wu, J., and Xu, Q. (2019). The emerging treatment landscape of targeted therapy in non-small-cell lung cancer. Signal Transduct. Target. Ther. 4, 61. doi:10.1038/s41392-019-0099-9
Zaborowska-Szmit, M., Kowalski, D. M., Piórek, A., Krzakowski, M., and Szmit, S. (2016). A decrease in D-dimer concentration and an occurrence of skin rash as iatrogenic events and complementary predictors of survival in lung cancer patients treated with EGFR tyrosine kinase inhibitors. Pharmacol. Rep. PR. 68 (6), 1140–1148. doi:10.1016/j.pharep.2016.07.003
Zaborowska-Szmit, M., Krzakowski, M., Kowalski, D. M., and Szmit, S. (2020). Cardiovascular complications of systemic therapy in non-small-cell lung cancer. J. Clin. Med. 9 (5), 1268. doi:10.3390/jcm9051268
Zamorano, J. L., Lancellotti, P., Rodriguez Muñoz, D., Aboyans, V., Asteggiano, R., Galderisi, M., et al. (2016). 2016 ESC Position Paper on cancer treatments and cardiovascular toxicity developed under the auspices of the ESC Committee for Practice Guidelines: the Task Force for cancer treatments and cardiovascular toxicity of the European Society of Cardiology (ESC). Eur. heart J. 37 (36), 2768–2801. doi:10.1093/eurheartj/ehw211
Zeppenfeld, K., Tfelt-Hansen, J., de Riva, M., Winkel, B. G., Behr, E. R., Blom, N. A., et al. (2022). 2022 ESC Guidelines for the management of patients with ventricular arrhythmias and the prevention of sudden cardiac death. Eur. heart J. 43 (40), 3997–4126. doi:10.1093/eurheartj/ehac262
Zhang, K. W., Finkelman, B. S., Gulati, G., Narayan, H. K., Upshaw, J., Narayan, V., et al. (2018). Abnormalities in 3-dimensional left ventricular mechanics with anthracycline chemotherapy are associated with systolic and diastolic dysfunction. JACC Cardiovasc. imaging 11 (8), 1059–1068. doi:10.1016/j.jcmg.2018.01.015
Zhang, X., Gao, Y., Yang, B., Ma, S., Zuo, W., and Wei, J. (2023). The mechanism and treatment of targeted anti-tumour drugs induced cardiotoxicity. Int. Immunopharmacol. 117, 109895. doi:10.1016/j.intimp.2023.109895
Zhang, Y., Wang, X., Pan, Y., Du, B., Nanthakumar, K., and Yang, P. (2022). Overdrive pacing in the acute management of osimertinib-induced ventricular arrhythmias: a case report and literature review. Front. Cardiovasc. Med. 9, 934214. doi:10.3389/fcvm.2022.934214
Zhang, Z., Guo, Y., Chen, M., Chen, F., Liu, B., and Shen, C. (2021). Kaempferol potentiates the sensitivity of pancreatic cancer cells to erlotinib via inhibition of the PI3K/AKT signaling pathway and epidermal growth factor receptor. Inflammopharmacology 29 (5), 1587–1601. doi:10.1007/s10787-021-00848-1
Zhang, Z., Zhang, M., Liu, H., and Yin, W. (2019). AZD9291 promotes autophagy and inhibits PI3K/Akt pathway in NSCLC cancer cells. J. cellular Biochem. 120 (1), 756–767. doi:10.1002/jcb.27434
Zhu, Y., Zheng, B., Cai, C., Lin, Z., Qin, H., Liu, H., et al. (2022). Febuxostat increases ventricular arrhythmogenesis through calcium handling dysregulation in human-induced pluripotent stem cell-derived cardiomyocytes. Toxicol. Sci. official J. Soc. Toxicol. 189 (2), 216–224. doi:10.1093/toxsci/kfac073
Keywords: cardiotoxicity, epidermal growth factor receptor-tyrosine kinase inhibitors, cardio-oncology, non-small cell lung cancer, cardiovascular toxicity
Citation: Li X, Lin Y, Lin S, Huang J and Ruan Z (2024) Advancements in understanding cardiotoxicity of EGFR- TKIs in non-small cell lung cancer treatment and beyond. Front. Pharmacol. 15:1404692. doi: 10.3389/fphar.2024.1404692
Received: 21 March 2024; Accepted: 05 August 2024;
Published: 15 August 2024.
Edited by:
Liang Guo, Bristol Myers Squibb, United StatesReviewed by:
Martin Hohenegger, Medical University of Vienna, AustriaVera Marisa Costa, University of Porto, Portugal
Copyright © 2024 Li, Lin, Lin, Huang and Ruan. This is an open-access article distributed under the terms of the Creative Commons Attribution License (CC BY). The use, distribution or reproduction in other forums is permitted, provided the original author(s) and the copyright owner(s) are credited and that the original publication in this journal is cited, in accordance with accepted academic practice. No use, distribution or reproduction is permitted which does not comply with these terms.
*Correspondence: Zhongbao Ruan, ruanzhongbao@njmu.edu.cn