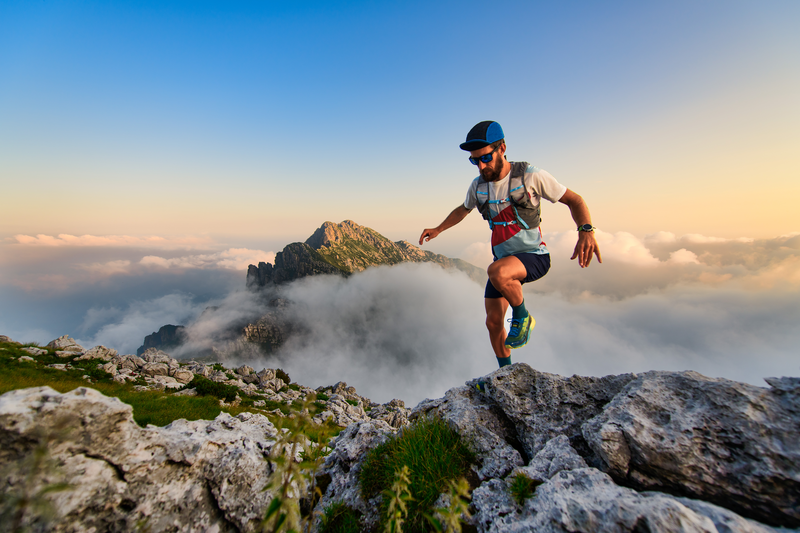
95% of researchers rate our articles as excellent or good
Learn more about the work of our research integrity team to safeguard the quality of each article we publish.
Find out more
CASE REPORT article
Front. Pharmacol. , 27 June 2024
Sec. Translational Pharmacology
Volume 15 - 2024 | https://doi.org/10.3389/fphar.2024.1403966
Background: Voriconazole is primarily metabolized by CYP2C19 and CYP3A4. Drug interactions that affect this pathway can alter its plasma exposures, resulting in untargeted voriconazole concentrations.
Case summary: In this case report, we describe the case of a 64-year-old man who was treated for non-Hodgkin’s lymphoma with continuous glucocorticoids co-administrated with voriconazole against invasive pulmonary aspergillosis. A decrease in trough concentration (Cmin) of voriconazole was observed and related with co-administration of dexamethasone in the patient carrying the CYP2C19 *1*2 genotype: voriconazole Cmin/dose ratios of 0.018 (0.1 mg L−1/5.7 mg kg−1 day−1), 0.18 (1 mg L−1/5.7 mg kg−1 day−1), and 0.23 (2 mg L−1/8.6 mg kg−1 day−1) at dexamethasone doses of 20, 12.5, and 2.5 mg, respectively. Sub-therapeutic voriconazole Cmin was associated with high- and moderate-dose dexamethasone (20 and 12.5 mg), leading to failure of antifungal treatment.
Conclusion: The extent of voriconazole–dexamethasone interaction was determined by the dose of dexamethasone and associated with the CYP2C19 *1*2 genotype. Therapeutic drug monitoring of voriconazole is necessary to avoid clinically relevant interactions for optimal antifungal therapy.
Invasive aspergillosis increases with the increase in the number of immunosuppressed patients, including those with malignant tumors, acquired immune deficiency syndrome, and who have underwent organ transplantation. Infection-related mortality remains high, particularly in severely ill patients with immunologic defects that are irreversible (Sanguinetti et al., 2019; Cadena et al., 2021). Furthermore, recent pandemics such as COVID-19 and influenza increase the morbidity and mortality caused by this invasive fungal infection (Lamoth et al., 2021). Voriconazole is the first-line therapy and widely used to prevent and treat invasive aspergillosis infection (Ullmann et al., 2018). Its therapeutic window (trough concentration, Cmin) is narrow (Wang et al., 2022). Subtherapeutic voriconazole Cmin (1–2 mg L−1) was related with treatment failures, while supratherapeutic Cmin (4.5–6 mg L−1) led to some voriconazole toxicities (Jin et al., 2016). Voriconazole Cmin exhibits great individual variability. Glucocorticoid–voriconazole interaction and CYP2C19 polymorphism were important factors that led to intra- and inter-individual variability of voriconazole Cmin (Moriyama et al., 2017). Dexamethasone, as the most prescribed glucocorticoid, is widely used in chemotherapy regimens for treatment of non-Hodgkin’s lymphoma. However, a potential interaction between dexamethasone and voriconazole can have a clinical impact in immunosuppressed patients carrying CYP2C19 *2 and *3 alleles. Furthermore, the Asian population has high frequencies of CYP2C19 mutant genes, including *2 (32%) and *3 (6%–10%) (Mizutani, 2003).
Herein, we reported an adverse dose-dependent interaction between voriconazole and dexamethasone in a non-Hodgkin’s lymphoma patient who was a CYP2C19 carrying intermediate metabolizer (IM, *1*2) and suffered from invasive pulmonary aspergillosis.
A 64-year-old Chinese man weighing 70 kg suffering for more than 1 month due to recurrent fever was transferred from an outside medical facility to the Department of Critical Care Medicine, Ruijin Hospital, Shanghai Jiao Tong University School of Medicine for further treatment on 26 May 2022. He was diagnosed with hemophagocytic lymphohistiocytosis (HLH) based on splenomegaly, hemocytopenia, elevated ferritin, hypertriglyceridemia, and abnormal coagulation function, according to the criteria of HLH-2004 (Henter et al., 2007). Then, non-Hodgkin’s lymphoma had been diagnosed through bone marrow biopsy, causing HLH. This patient received continuous glucocorticoid treatment with chemotherapeutic agents, zanubrutinib and obinutuzumab, for the treatment of HLH and non-Hodgkin’s lymphoma (Table 1). He subsequently developed hospital-acquired pneumonia. Carbapenem-resistant Klebsiella pneumoniae (CRKP), Aspergillus fumigatus, and Enterococcus faecium were identified in sputum and blood by metagenomics next-generation sequencing (mNGS) and culture, necessitating initiation of antimicrobial treatment. Antibacterial therapy included empirical piperacillin–tazobactam, polymyxin B, fosfomycin, minocycline, ceftazidime–avibactam, and vancomycin. Voriconazole and amphotericin B were administrated for aspergillosis infection.
The plasma trough concentration (Cmin) of voriconazole was 0.2 mg L−1, determined by liquid chromatography–electrospray tandem mass spectrometry (detailed in the Supplementary File S1), on 28 June 2022. Twenty-one days of intravenous (IV) voriconazole (400 mg twice on the first day and maintenance dose of 200 mg q12 h) treatment was not satisfactory (Figure 1). On assessing, Aspergillus fumigatus was found again in sputum (mNGS) on 29 June 2022. Before voriconazole initiation, IV dexamethasone 20 mg had been used for 7 days and then gradually reduced. Following dexamethasone, IV methylprednisolone 80 mg was given and then gradually reduced. Subsequently, IV dexamethasone 20 mg combined with etoposide 0.5 mg and vincristine 18 mg was intravenously administrated for chemotherapy for lymphoma from 1 July to 4 July 2022. On 4 July, voriconazole Cmin was 0.1 mg L−1 and still below the therapeutic index. His chest CT scan indicated progressive pulmonary aspergillosis with a nodule in the right upper lobe and a thick-walled cavity inside the nodule. Aspergillus fumigatus could be detected in bronchoalveolar lavage fluid (BALF) and blood by mNGS. Double maintenance dose of voriconazole (400 mg q12 h) was given on 5 July 2022, and voriconazole Cmin of 1.7 mg L−1 (July 8th) achieved the therapeutic range. In addition, this patient carrying the CYP2C19*1*2 genotype [*2: 681G>A, rs4244285; *3: 636G>A; rs4986893; *17: −806C>T; rs12248560; genotyping adopted using the Sanger DNA sequencing method with an ABI3730xl-full automatic sequencing instrument (ABI Co.)] was an intermediate metabolizer of voriconazole. The interaction between dexamethasone and voriconazole was recognized after checking the other medications of this patient. In addition, the total score of the Drug Interaction Probability Scale (DIPS) was 10 (>8: highly probable) (Horn et al., 2007). Voriconazole Cmin increased to 2.6 mg L−1 on 11 July 2022. Voriconazole dose was adjusted to 300 mg q12 h on 12 July, and voriconazole Cmin was 2.0 mg L−1 on 18 July. Aspergillus fumigatus still could be detected in BALF by mNGS. A nodule was formed in the apex of the right lung, with a small cavity inside it, as shown by CT scan (18 July 2022). The amphotericin B cholesteryl sulfate complex with dexamethasone 2.5 mg was added on 21 July 2022. Voriconazole Cmin was monitored, and its value was 2.0 mg L−1 on 27 July. The patient received the second chemotherapy regimen for lymphoma treatment consisting of dexamethasone 10 mg, etoposide 0.5 mg, and vincristine 18 mg from 1 August to 4 August. Voriconazole Cmin was 1 mg L−1 on 3 August. Voriconazole dose was adjusted to 400 mg q12 h, and voriconazole Cmin increased to 1.8 mg L−1 on 8 August. Then, voriconazole dose was reduced to 300 mg q12 h on 12 August. The chest CT scan indicated that symptoms of pulmonary aspergillosis improved on 16 August 2022. Voriconazole Cmin was 1.9 mg L−1 (August 18th) and effective against aspergillus infection.
Figure 1. Relationship between the Cmin (A) and Cmin/dose (B) ratios of voriconazole and dexamethasone dose. (a) Aspergillus fumigatus (sputum and blood mNGS). (b) Progressive pulmonary aspergillosis with a nodule in the right upper lobe and a thick-walled cavity inside the nodule (chest CT scan) and Aspergillus fumigatus (BALF and blood mNGS). (c) Pulmonary aspergillosis improved (chest CT scan). Abbreviations: C/D: Cmin/dose ratio; DXM, dexamethasone; MPS, methylprednisolone; VRZ, voriconazole.
To our knowledge, this is the first report of a dose-dependent interaction between voriconazole and dexamethasone in the patient carrying the CYP2C19*1*2 genotype (IM). The co-administration of dexamethasone increased voriconazole clearance with low exposure. Voriconazole Cmin was 0.1 mg L−1 (dose: 5.7 mg kg−1 day−1, 400 mg day−1; Cmin/dose (C/D) ratio: 0.018), when 20 mg of dexamethasone was co-administrated on 4 July 2022. Progressive pulmonary aspergillosis, indicated by CT scan and Aspergillus fumigatus in BALF, suggested unsatisfactory voriconazole therapy. Voriconazole Cmin was 2 mg L−1 (dose: 8.6 mg kg−1 day−1, 600 mg day−1; C/D ratio: 0.23) when 2.5 mg of dexamethasone was used on 27 July. Then, 12.5 mg of dexamethasone was given from 1 August to 4 August. Voriconazole Cmin was 1 mg L−1 (dose: 5.7 mg kg−1day−1, 400 mg day−1; C/D ratio: 0.18) on 3 August. Subsequent Cmin values were 1.8 mg L−1 (dose: 11.4 mg kg−1day−1, 800 mg day−1; C/D ratio: 0.16) and 1.9 mg L−1 (dose: 8.6 mg kg−1day−1, 600 mg day−1; C/D ratio: 0.22) on 8 August and 18 August, respectively (dexamethasone: 2.5 mg). This patient received effective voriconazole therapy, combined with the amphotericin B cholesteryl sulfate complex, and showed improvement in pulmonary aspergillosis.
Reduced voriconazole Cmin was due to increase in clearance, attributing to the co-administration of glucocorticoids (dexamethasone and methylprednisolone). Dexamethasone had a greater effect on voriconazole exposure compared to methylprednisolone (Dolton et al., 2012; Jia et al., 2021). Voriconazole Cmin is a good measure of drug exposure, recommended as the pharmacokinetic (PK)/pharmacodynamic parameter for regular therapeutic drug monitoring (TDM) in the clinic (Takesue et al., 2022), because it has a linear relationship with the voriconazole area under the concentration–time curve (AUC0–12h) (Hope, 2012). However, the results of this interaction in different research studies are inconsistent (Gautier-Veyret et al., 2015; Blanco-Dorado et al., 2020). It may be related with difference in the distribution of CYP2C19 genotypes and glucocorticoid dose employed among the studied population.
Voriconazole is primarily metabolized in the liver by CYP2C19 and CYP3A4 enzymes. Difference in the CYP2C19 gene between individuals can greatly affect voriconazole metabolism. The *2 and *3 alleles were loss-of-function variations. IM with one such variant had significantly lower enzyme activity than normal metabolizer (NM, *1*1) (Li et al., 2023). In the absence of functional CYP2C19, CYP3A4 became the important alternative pathway for voriconazole clearance (Murayama et al., 2007). In CYP2C19 poor metabolizer (PM) liver microsomes, inhibition of voriconazole metabolism by ketoconazole (a specific CYP3A4 inhibitor) was most potent (Hyland et al., 2003). A PK study of healthy participants (Mikus et al., 2006) also showed that co-administration of ritonavir (a potent CYP3A4 inhibitor) led to lower voriconazole clearances in CYP2C19 IM (*1*2) and PM (*2*2), compared with NM. It is inferred that high dose of dexamethasone might affect voriconazole clearance much more in IM and PM of CYP2C19.
The extent of voriconazole–dexamethasone interaction seems to depend on the dexamethasone dose. The induction of CYP450 enzymes, particularly CYP3A4, by dexamethasone is dose-dependent (Bourdin et al., 2023). Co-administration of dexamethasone with high and moderate doses (20 and 12.5 mg) seemed to impact voriconazole Cmin. Consistently, CYP3A4 induction was, respectively, >50%–<80%, >20–<50%, and <20% at the dexamethasone dose of >16 mg (high dose), >1.5–16 mg (moderate dose), and ≤1.5 mg (low dose) (Jacobs et al., 2022). Taghvaye et al. (2019) reported the significant interaction between iv voriconazole and iv dexamethasone (8 mg q12 h), leading to failure of antifungal treatment, in a 32-year-old woman with acute lymphoblastic leukemia. Wallace et al. (2016) also found the clinical relevant drug interaction between iv voriconazole and oral dexamethasone (4 mg q8 h) in an 84-year-old male patient. In our case, subtherapeutic Cmin of voriconazole (0.2 mg L−1) on 28 June and subsequent detection of Aspergillus fumigatus in sputum on 29 June indicated ineffective voriconazole therapy, associated with the treatment of both dexamethasone and methylprednisolone from 26 May to 27 June. The used methylprednisolone could enhance the CYP induction of high-dose dexamethasone (20 mg, from 1 July to 4 July), related with the 0.1 mg L−1 of voriconazole Cmin on 4 July.
A multicenter study about the PK and TDM of voriconazole (Dolton et al., 2012) showed that co-administration of dexamethasone and methylprednisolone reduced voriconazole concentrations to a greater extent than prednisone or prednisolone, associated with their potency of binding to glucocorticoid receptors (GRs). Glucocorticoids induce CYP450s, including CYP2C19 and CYP3A4, through binding to GR. In addition to that, dexamethasone strongly induces CYP3A4 via direct activation of pregnane X receptor (PXR). A recent retrospective observational study of 231 patients with 918 voriconazole Cmin (Jia et al., 2021) confirmed that the co-administration of glucocorticoids (dexamethasone, prednisone, prednisolone, and methylprednisolone) reduced the voriconazole Cmin/dose, among which dexamethasone caused the lowest median of the voriconazole Cmin/dose ratio. However, they did not analyze the effect of glucocorticoid dose on voriconazole. The study of immunocompromised patients (n = 38) (Imataki et al., 2018) found that voriconazole concentrations were significantly decreased in corticosteroid users compared to the non-user (p = .013) (the median doses of corticosteroids: 89.8 mg/d, equivalent to dexamethasone 13.47 mg). Blanco-Dorado et al. (2020) (the median doses of corticosteroids: 50 mg/d, equivalent to dexamethasone 7.5 mg) and Gautier-Veyret et al. (2015) (no data about the dose of glucocorticoids) showed no interaction between glucocorticoids and voriconazole. The inconsistent results could be explained by the heterogeneity of the type and dose of the glucocorticoids, in line with that reported in our case.
In addition, voriconazole has a long-lasting and potent inhibitory effect on CYP3A activity (Huang et al., 2021). Dexamethasone and methylprednisolone are primarily metabolized by CYP3A4 (Blanco-Dorado et al., 2020; Bourdin et al., 2023). The maximum plasma concentration (Cmax) and the AUC from 0 h to infinity (AUC0→inf) of dexamethasone could be increased by 2.44-fold and 2.60-fold, respectively, when combined with voriconazole. For methylprednisolone, Cmax and AUC0→inf were increased by 1.56-fold and 2.23-fold, respectively (Li et al., 2018).Thus, their increased exposure in combination with voriconazole may enhance the interaction. Multiple daily high doses (>16 mg) of dexamethasone increased the risk of clinical significant interactions. High-dose dexamethasone is widely administrated in the treatment of hematological malignancies (Jacobs et al., 2022). Furthermore, moderate-dose dexamethasone (>1.5–16 mg) with long treatment course (>10 days) had a considerable risk of interactions and commonly used in the treatment of palliative care and cerebral edema.
TDM of voriconazole is necessary in patients receiving >16 mg dexamethasone with multiple daily doses, particularly those with the impaired activity of CYP2C19. Further studies regarding the interaction between glucocorticoids, especially dexamethasone and voriconazole, are warranted to prevent the clinical relevant interactions for effective antifungal therapy.
The original contributions presented in the study are included in the article/Supplementary Material; further inquiries can be directed to the corresponding author.
The studies involving humans were approved by the Ethical Committee of Ruijin Hospital. The studies were conducted in accordance with the local legislation and institutional requirements. The participants provided their written informed consent to participate in this study. Written informed consent was obtained from the individual(s) for the publication of any potentially identifiable images or data included in this article.
JH: writing–original draft. YC: writing–original draft. MZ: writing–review and editing. RT: writing–review and editing.
The author(s) declare that no financial support was received for the research, authorship, and/or publication of this article.
The authors would like to thank all the staffs in Department of Critical Care Medicine, Ruijin Hospital, Shanghai Jiao Tong University School of Medicine and the patient for their cooperation in conducting this study.
The authors declare that the research was conducted in the absence of any commercial or financial relationships that could be construed as a potential conflict of interest.
All claims expressed in this article are solely those of the authors and do not necessarily represent those of their affiliated organizations, or those of the publisher, the editors, and the reviewers. Any product that may be evaluated in this article, or claim that may be made by its manufacturer, is not guaranteed or endorsed by the publisher.
The Supplementary Material for this article can be found online at: https://www.frontiersin.org/articles/10.3389/fphar.2024.1403966/full#supplementary-material
C/D, Cmin/dose ratio; DXM, dexamethasone; IM, intermediate metabolizer; NHL, non-Hodgkin’s lymphoma; VRZ, voriconazole.
Blanco-Dorado, S., Maroñas, O., Latorre-Pellicer, A., Rodríguez Jato, M. T., López-Vizcaíno, A., Gómez, M. A., et al. (2020). Impact of CYP2C19 genotype and drug interactions on voriconazole plasma concentrations: a Spain pharmacogenetic-pharmacokinetic prospective multicenter study. Pharmacotherapy 40 (1), 17–25. doi:10.1002/phar.2351
Bourdin, V., Bigot, W., Vanjak, A., Burlacu, R., Lopes, A., Champion, K., et al. (2023). Drug-drug interactions involving dexamethasone in clinical practice: myth or reality? J. Clin. Med. 12 (22), 7120. doi:10.3390/jcm12227120
Cadena, J., Thompson, G. R., and Patterson, T. F. (2021). Aspergillosis: epidemiology, diagnosis, and treatment. Infect. Dis. Clin. North Am. 35 (2), 415–434. doi:10.1016/j.idc.2021.03.008
Dolton, M. J., Ray, J. E., Chen, S. C., Ng, K., Pont, L. G., and McLachlan, A. J. (2012). Multicenter study of voriconazole pharmacokinetics and therapeutic drug monitoring. Antimicrob. Agents Chemother. 56 (9), 4793–4799. doi:10.1128/AAC.00626-12
Gautier-Veyret, E., Fonrose, X., Tonini, J., Thiebaut-Bertrand, A., Bartoli, M., Quesada, J. L., et al. (2015). Variability of voriconazole plasma concentrations after allogeneic hematopoietic stem cell transplantation: impact of cytochrome p450 polymorphisms and comedications on initial and subsequent trough levels. Antimicrob. Agents Chemother. 59 (4), 2305–2314. doi:10.1128/aac.04838-14
Henter, J. I., Horne, A., Aricó, M., Egeler, R. M., Filipovich, A. H., Imashuku, S., et al. (2007). HLH-2004: diagnostic and therapeutic guidelines for hemophagocytic lymphohistiocytosis. Pediatr. Blood Cancer 48 (2), 124–131. doi:10.1002/pbc.21039
Hope, W. W. (2012). Population pharmacokinetics of voriconazole in adults. Antimicrob. Agents Chemother. 56 (1), 526–531. doi:10.1128/AAC.00702-11
Horn, J. R., Hansten, P. D., and Chan, L. N. (2007). Proposal for a new tool to evaluate drug interaction cases. Ann. Pharmacother. 41 (4), 674–680. doi:10.1345/aph.1H423
Huang, F. R., Zhou, C., Zhang, X. Y., Shen, Y., Zhang, H. W., Wang, Y. Q., et al. (2021). Impact of CYP2C19 genotype on voriconazole exposure and effect of voriconazole on the activity of CYP3A in patients with haematological malignancies. Xenobiotica 51 (10), 1199–1206. doi:10.1080/00498254.2021.1969481
Hyland, R., Jones, B. C., and Smith, D. A. (2003). Identification of the cytochrome P450 enzymes involved in the N-oxidation of voriconazole. Drug Metab. Dispos. 31 (5), 540–547. doi:10.1124/dmd.31.5.540
Imataki, O., Yamaguchi, K., Uemura, M., and Fukuoka, N. (2018). Voriconazole concentration is inversely correlated with corticosteroid usage in immunocompromised patients. Transpl. Infect. Dis. 20 (4), e12886. doi:10.1111/tid.12886
Jacobs, T. G., Marzolini, C., Back, D. J., and Burger, D. M. (2022). Dexamethasone is a dose-dependent perpetrator of drug-drug interactions: implications for use in people living with HIV. J. Antimicrob. Chemother. 77 (3), 568–573. doi:10.1093/jac/dkab412
Jia, S. J., Gao, K. Q., Huang, P. H., Guo, R., Zuo, X. C., Xia, Q., et al. (2021). Interactive effects of glucocorticoids and cytochrome P450 polymorphisms on the plasma trough concentrations of voriconazole. Front. Pharmacol. 12, 666296. doi:10.3389/fphar.2021.666296
Jin, H., Wang, T., Falcione, B. A., Olsen, K. M., Chen, K., Tang, H., et al. (2016). Trough concentration of voriconazole and its relationship with efficacy and safety: a systematic review and meta-analysis. J. Antimicrob. Chemother. 71 (7), 1772–1785. doi:10.1093/jac/dkw045
Lamoth, F., Lewis, R. E., Walsh, T. J., and Kontoyiannis, D. P. (2021). Navigating the uncertainties of COVID-19-associated aspergillosis: a comparison with influenza-associated aspergillosis. J. Infect. Dis. 224 (10), 1631–1640. doi:10.1093/infdis/jiab163
Li, G., Li, Q., Zhang, C., Yu, Q., Li, Q., Zhou, X., et al. (2023). The impact of gene polymorphism and hepatic insufficiency on voriconazole dose adjustment in invasive fungal infection individuals. Front. Genet. 14, 1242711. doi:10.3389/fgene.2023.1242711
Li, M., Zhu, L., Chen, L., Li, N., and Qi, F. (2018). Assessment of drug-drug interactions between voriconazole and glucocorticoids. J. Chemother. 30 (5), 296–303. doi:10.1080/1120009x.2018.1506693
Mikus, G., Schöwel, V., Drzewinska, M., Rengelshausen, J., Ding, R., Riedel, K. D., et al. (2006). Potent cytochrome P450 2C19 genotype-related interaction between voriconazole and the cytochrome P450 3A4 inhibitor ritonavir. Clin. Pharmacol. Ther. 80 (2), 126–135. doi:10.1016/j.clpt.2006.04.004
Mizutani, T. (2003). PM frequencies of major CYPs in Asians and Caucasians. Drug Metab. Rev. 35 (2-3), 99–106. doi:10.1081/dmr-120023681
Moriyama, B., Obeng, A. O., Barbarino, J., Penzak, S. R., Henning, S. A., Scott, S. A., et al. (2017). Clinical pharmacogenetics implementation consortium (CPIC) guidelines for CYP2C19 and voriconazole therapy. Clin. Pharmacol. Ther. 102 (1), 45–51. doi:10.1002/cpt.583
Murayama, N., Imai, N., Nakane, T., Shimizu, M., and Yamazaki, H. (2007). Roles of CYP3A4 and CYP2C19 in methyl hydroxylated and N-oxidized metabolite formation from voriconazole, a new anti-fungal agent, in human liver microsomes. Biochem. Pharmacol. 73 (12), 2020–2026. doi:10.1016/j.bcp.2007.03.012
Sanguinetti, M., Posteraro, B., Beigelman-Aubry, C., Lamoth, F., Dunet, V., Slavin, M., et al. (2019). Diagnosis and treatment of invasive fungal infections: looking ahead. J. Antimicrob. Chemother. 74 (Suppl. 2), ii27–ii37. doi:10.1093/jac/dkz041
Taghvaye, M. H., Hadjibabaie, M., Gholami, K., Zarif-Yeganeh, M., and Ghavamzadeh, A. (2019). Significant drug interaction between voriconazole and dexamethasone: a case report. J. Oncol. Pharm. Pract. 25 (5), 1239–1242. doi:10.1177/1078155218783248
Takesue, Y., Hanai, Y., Oda, K., Hamada, Y., Ueda, T., Mayumi, T., et al. (2022). Clinical practice guideline for the therapeutic drug monitoring of voriconazole in non-asian and asian adult patients: consensus review by the Japanese society of chemotherapy and the Japanese society of therapeutic drug monitoring. Clin. Ther. 44 (12), 1604–1623. doi:10.1016/j.clinthera.2022.10.005
Ullmann, A. J., Aguado, J. M., Arikan-Akdagli, S., Denning, D. W., Groll, A. H., Lagrou, K., et al. (2018). Diagnosis and management of Aspergillus diseases: executive summary of the 2017 ESCMID-ECMM-ERS guideline. Clin. Microbiol. Infect. 24 (Suppl. 1), e1–e38. doi:10.1016/j.cmi.2018.01.002
Wallace, K. L., Filipek, R. L., La Hoz, R. M., and Williamson, J. C. (2016). Subtherapeutic voriconazole concentrations associated with concomitant dexamethasone: case report and review of the literature. J. Clin. Pharm. Ther. 41 (4), 441–443. doi:10.1111/jcpt.12401
Keywords: voriconazole trough concentration, dexamethasone dose, drug interaction, case report, therapeutic drug monitoring, aspergillosis, hematological malignancies
Citation: Huang J, Chen Y, Zhong M and Tan R (2024) Case report: dose-dependent interaction between dexamethasone and voriconazole in severely ill patients with non-Hodgkin’s lymphoma being treated for invasive pulmonary aspergillosis. Front. Pharmacol. 15:1403966. doi: 10.3389/fphar.2024.1403966
Received: 21 March 2024; Accepted: 30 May 2024;
Published: 27 June 2024.
Edited by:
Elena Ramírez, Universidad Autónoma de Madrid, SpainReviewed by:
Xin Huang, The First Affiliated Hospital of Shandong First Medical University, ChinaCopyright © 2024 Huang, Chen, Zhong and Tan. This is an open-access article distributed under the terms of the Creative Commons Attribution License (CC BY). The use, distribution or reproduction in other forums is permitted, provided the original author(s) and the copyright owner(s) are credited and that the original publication in this journal is cited, in accordance with accepted academic practice. No use, distribution or reproduction is permitted which does not comply with these terms.
*Correspondence: Ruoming Tan, c2FuZHJhdGFuMzdAaG90bWFpbC5jb20=; Ming Zhong, em0xMTcxNkByamguY29tLmNu
†These authors have contributed equally to this work
Disclaimer: All claims expressed in this article are solely those of the authors and do not necessarily represent those of their affiliated organizations, or those of the publisher, the editors and the reviewers. Any product that may be evaluated in this article or claim that may be made by its manufacturer is not guaranteed or endorsed by the publisher.
Research integrity at Frontiers
Learn more about the work of our research integrity team to safeguard the quality of each article we publish.