- 1Department of Biomedical Sciences, City University of Hong Kong, Kowloon, Hong Kong SAR, China
- 2Department of Neurosciences, City University of Hong Kong, Kowloon, Hong Kong SAR, China
- 3Centre for Regenerative Medicine and Health (CRMH), Hong Kong, Hong Kong SAR, China
- 4Department of Botany, Bacha Khan University Charsadda, Charsadda, Pakistan
- 5Department of Medical Laboratories, College of Applied Medical Sciences, Qassim University, Buraydah, Saudi Arabia
Epilepsy is one of the most common, severe, chronic, potentially life-shortening neurological disorders, characterized by a persisting predisposition to generate seizures. It affects more than 60 million individuals globally, which is one of the major burdens in seizure-related mortality, comorbidities, disabilities, and cost. Different treatment options have been used for the management of epilepsy. More than 30 drugs have been approved by the US FDA against epilepsy. However, one-quarter of epileptic individuals still show resistance to the current medications. About 90% of individuals in low and middle-income countries do not have access to the current medication. In these countries, plant extracts have been used to treat various diseases, including epilepsy. These medicinal plants have high therapeutic value and contain valuable phytochemicals with diverse biomedical applications. Epilepsy is a multifactorial disease, and therefore, multitarget approaches such as plant extracts or extracted phytochemicals are needed, which can target multiple pathways. Numerous plant extracts and phytochemicals have been shown to treat epilepsy in various animal models by targeting various receptors, enzymes, and metabolic pathways. These extracts and phytochemicals could be used for the treatment of epilepsy in humans in the future; however, further research is needed to study the exact mechanism of action, toxicity, and dosage to reduce their side effects. In this narrative review, we comprehensively summarized the extracts of various plant species and purified phytochemicals isolated from plants, their targets and mechanism of action, and dosage used in various animal models against epilepsy.
1 Introduction
Epilepsy is one of the most common chronic and heterogeneous neurological disorders, affecting more than 60 million individuals worldwide (Moshé et al., 2015). Epilepsy is characterized by seizures, which are abnormal electrical activities in the brain resulting in motor, sensory, or psychomotor experiences (Potnis et al., 2020). These activities in the brain occur due to the imbalance of excitatory and inhibitory neuronal pathways, and the tendency to develop seizures again and again leads to epilepsy. According to the latest report of the International League Against Epilepsy (ILAE), epilepsy is defined as” two or more unprovoked seizures greater than 24 h apart, or single unprovoked seizure in an individual with 60% chances of having another seizure in the next 10 years, or an epilepsy syndrome (clinical, EEG, clinical, genetics and age-dependent features) (Falco-Walter, 2020; Specchio et al., 2022; Wirrell et al., 2022). The signs and symptoms of epilepsy are shown in Figure 1A. The global prevalence of epilepsy is 1%, and among them, 80% of inhabitants live in low-income and lower-middle-income countries. According to the World Health Organization (WHO), about 75% of the affected individuals do not get proper treatment for the management of epilepsy, and this percentage has also reached 90% in some low-income countries, as these anti-epileptic drugs (AEDs) are inaccessible, too expensive, or have unwanted side effects (O’Donohoe et al., 2020; Pironi et al., 2022). Currently, more than thirty pharmacological drugs have been approved by the United States Food and Drug Administration (USFDA) and are available in the market (Perucca, 2021), as shown in Figure 1B. Almost 70% of the affected people become seizure-free after proper medication with the available AEDs. Still, 25%–35% of people show resistance to the current medications and are termed refractory, intractable, or drug-resistant epilepsy, and almost 50% of the sudden deaths from epilepsy belong to the drug-resistant epilepsy group (Mula, 2021; Borowicz-Reutt et al., 2023; Rocha et al., 2023). Currently, different treatment options are available, as shown in Figure 1C, including pharmacological treatment, but every treatment strategy has its own limiting factors. Due to the different limitations of the treatment options against epilepsy, phytotherapeutic treatment or herbal medicines got huge attention worldwide due to its safety, low toxicity, easy availability, cost-effectiveness, and multitargeting ability to focus on the various medicinal plants and their phytochemicals for the treatment of epilepsy.
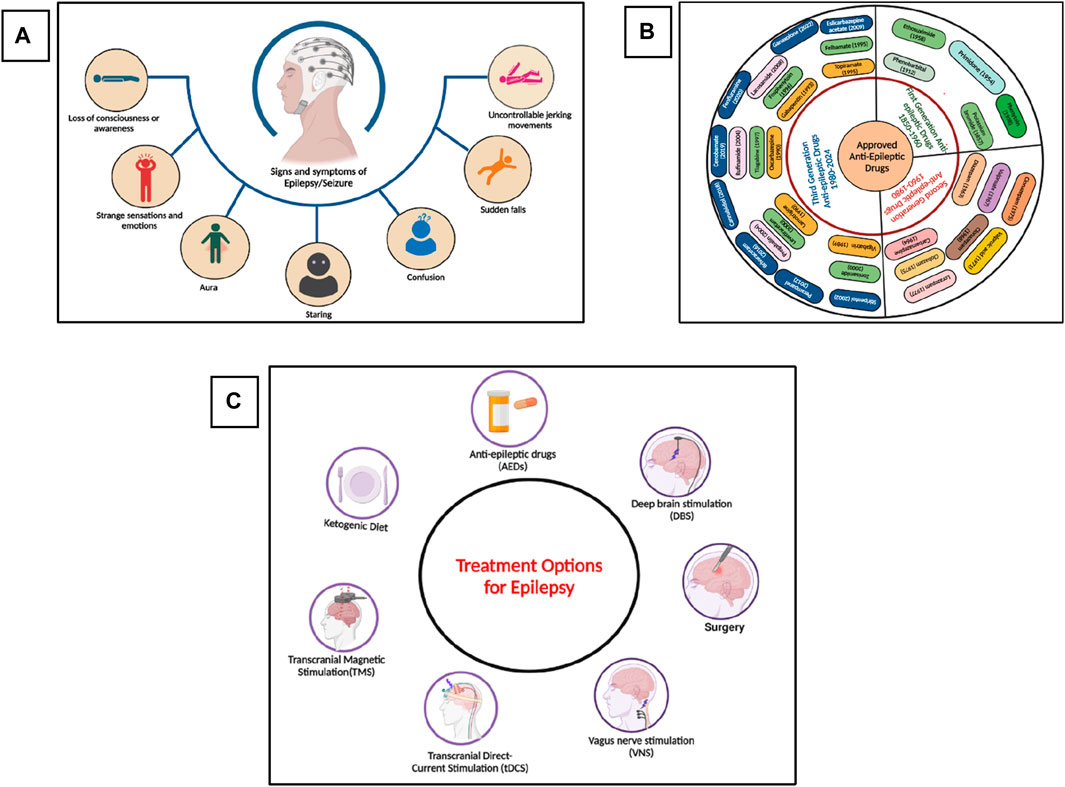
Figure 1. (A) Signs and symptoms of seizure and epilepsy, (B) Approved and Marketed AEDs by USFDA, (C) Different treatment options for the treatment of epilepsy. (Figures were generated using Biorender online version).
Medicinal plants are the main sources of pharmaceuticals in early discovery. Even in the 21st century, 80% of the population in lower-income countries depends on extracts of various plants for the preventive and curative treatment of various diseases, including neurological disorders, malaria, mycetoma, etc. Plant species such as Bacopa monnieri, Centella Asiatica, Curcuma longa, Cyperus rotundus, Morinda citrifolia, and Withania somnifera are among the most common plants used for treating neurological disorders (Berdigaliyev and Aljofan, 2020; Bhat and Sharma, 2020; Fitzgerald et al., 2020; Porras et al., 2020). Medicinal plants generate different types of substances that have promising therapeutic potential, which are termed phytochemicals or bioactive compounds. These phytochemicals have the potential to target specific receptors in various diseases and perform therapeutic activities. The WHO also recommends the use of plant-derived medicines with better safety and therapeutic efficacy in various countries (Palhares et al., 2015). In the last few years, scientists have focused on natural products, especially medicinal plants, to find cost-effective, safer, and potent agents for preventive, prophylactic, and other medicinal purposes. Many studies have been carried out to evaluate the therapeutic potential of various types of phytochemicals against epilepsy at a pre-clinal stage. (Kaur et al., 2021; He et al., 2023). It has been reported that numerous phytochemicals isolated from plants have promising potential to reach various drug targets in epileptic animal models (Chipiti et al., 2021; Subedi and Gaire, 2021; Zadali et al., 2022; Carreño-González et al., 2023). These phytochemicals can interact with different types of receptors in the central nervous system (CNS), such as N-methyl-D-aspartate receptor (NMDAR), Gamma-aminobutyric acid receptor (GABAAR), and α-amino-3-hydroxy-5-methyl-4-isoxazolepropionic acid receptor (AMPAR), channels like voltage gated sodium, potassium, and calcium channels, and other enzymes and pathways that play a significant role in the initiation and propagation of epileptic seizures. In this narrative review, we summarized the up-to-date information on various plants/medicinal plant extracts, specific phytochemicals isolated from various plants, their mechanism of action, and how these interact with various targets and help in the control of seizures and treatment of epilepsy.
2 Approved drugs and pharmacological targets
2.1 Current treatment options
Epilepsy affects people of all ages, with a high prevalence in people aged more than 65. Overall, 25%–35% of people still show resistance to the available AEDs. (Campos-Bedolla et al., 2022; Asadi-Pooya et al., 2023). To overcome this issue, different treatment options have been developed, such as surgery (if the seizure is focal, i.e., originated from a specific region of a brain such as in the case of temporal lobe epilepsy in which the seizure originated from the hippocampus), ketogenic diet, deep brain stimulation (DBS), vagus nerve stimulation (VNS), transcranial magnetic stimulation (TMS), and transcranial direct current stimulation (tDCR) (Harris and Angus-Leppan, 2020; Löscher et al., 2020; Asadi-Pooya et al., 2023). Each of these approaches has its own limitations, such as availability, cost, success rate, etc (Thijs et al., 2019). Currently, the most common way to treat epilepsy is pharmacological drugs, as it is easily available and used, have a high success rate, and are cost-effective as compared to other treatment approaches. In the 19th century, potassium bromide and some medicinal plants were used to treat seizures and epilepsy. Then, at the start of the 20th century, phenobarbital, which is a GABAA receptor agonist, was officially approved for the treatment of epilepsy in 1912. From 1850 to 1960, mostly five drugs were commonly used to treat seizures and epilepsy (Perucca, 2021; Tomson et al., 2023), as shown in Figure 1B. Then, until 1980, eight more drugs were approved by the USFDA for the treatment of epilepsy. Later in the 21st century, the advancement in the field of neurosciences, especially in the mechanism of disease and diagnostic approaches, the development of drugs against various neurological disorders, especially epilepsy, got much attention, and within the last 3 decades, more than twenty drugs have been approved by the USFDA for the treatment of epilepsy (Perucca, 2021). AEDs that have been approved by the US FDA from 1950 till 2024 are summarized in Figure 1B. Researchers are now focusing on the drugs’ cost-effectiveness and high success rate, and several drugs and other treatment approaches are currently in various phases of clinical trials (ClinicalTrials.gov 2024).
2.2 Pharmacological targets of the approved AEDs
Epilepsy develops due to the imbalance of action or resting membrane potential (Scharfman, 2007). Various receptors, membrane channels, and enzymes have been involved in the imbalance of depolarization and hyperpolarization of neurons, which then leads to a seizure (Armijo et al., 2005). Some of these are present on the presynaptic neuron, while some are on postsynaptic neurons. These protein or membrane channels include voltage-gated sodium channels responsible for the sodium ion (Na+) influx in the cell during normal functioning (Mantegazza et al., 2010). The sodium channels are normally found in three states (see Section 7); in case of seizure, these channels are in an active state for a longer time, or it takes more time to go to an inactive state due to mutation occurring in the proteins subunits of the channels or due to any other brain insult (Crill, 1996; Mantegazza et al., 2010). So when more sodium channels are active for a longer time, a high influx of Na+ will occur in presynaptic neurons, leading to the imbalance of normal signalling in the neuron and generating an imbalance in the excitatory and inhibitory system of the neurons, leading to a seizure. (Kaplan et al., 2016). Some approved drugs such as Rufinamide, Oxcarbazepine, and Phenytoin targeting Na+ channels to control the seizures and the treatment of epilepsy (Perucca, 2021).
At the end of presynaptic neurons, calcium channels are present, which allow the calcium ion (Ca2+) to go inside the cell and perform normal function, which helps in the release of the neurotransmitter (Meir et al., 1999). However, in the case of epilepsy, dysfunction of these important channels occurs due to mutations in the subunits or any other brain insult. Therefore, the concentration of Ca2+ in the cell is out of control, and hence, an influx of more Ca2+ occurs, which leads to the release of more neurotransmitters and disturbs the normal function of the cell (Carvill, 2019). Several clinically approved drugs such as Gabapentin, Pregabalin, and Ethosuximide block these channels and hence control the seizure and epilepsy (Tomson et al., 2023).
Similarly, during normal cell functioning, Na+ influx and potassium (K+) outflux occur to maintain normal cell function. However, during the seizure, the voltage-gated potassium channels (Kv) fail to efflux enough K+ ions out of the cell to maintain a normal level of ions inside the cell, which causes an imbalance of the action potential and again leads to the disturbance of excitatory and inhibitory cycle of the neurons and finally develops seizure (Gao et al., 2022). In addition, a few more channels and enzymes are involved in the initiation and propagation of seizure (Rho and Boison, 2022; Perucca et al., 2023a; Perucca et al., 2023b).
Similarly, on postsynaptic neurons, three main important receptors are present, which play a pivotal role in the cell signalling and development of epilepsy and are also the main target of the current and development of new AEDs. These receptors or channels include NMDARs and AMPARs to which glutamate, a primary excitatory neurotransmitter attached, opens the channels and allows more positive ions (Na+/Ca2+) to go inside the postsynaptic neuron and generate an action potential (Sivakumar et al., 2022; Chen et al., 2023). Some clinically approved AEDs, such as Perampanel and Valproate, target these two receptors on the postsynaptic neuron to control the generation or propagation of the action potential in epilepsy (Tomson et al., 2023). In addition, GABARs, to which GABA, an inhibitory neurotransmitter, binds, are the most important target to control seizures and treat epilepsy; they are also present on the postsynaptic neuron, which allows chlorine ions (Cl-) to go inside the cell to maintain and balance the internal environment (Fu et al., 2022; Akyuz et al., 2023; Bryson et al., 2023). Among the currently approved AEDs, more than 35% of the drug target is GABAR, which acts as an agonist to open the channels for a longer time. These includes Stiripentol, Vigabatrin, Diazepam, and Clonazepam (Perucca, 2021). Similarly, some drugs target various enzymes involved in the development of seizures and epilepsy. The details of channels, receptors, and enzymes involved in the development of seizures and epilepsy, as well as the main target of the drugs, are shown in Figure 2.
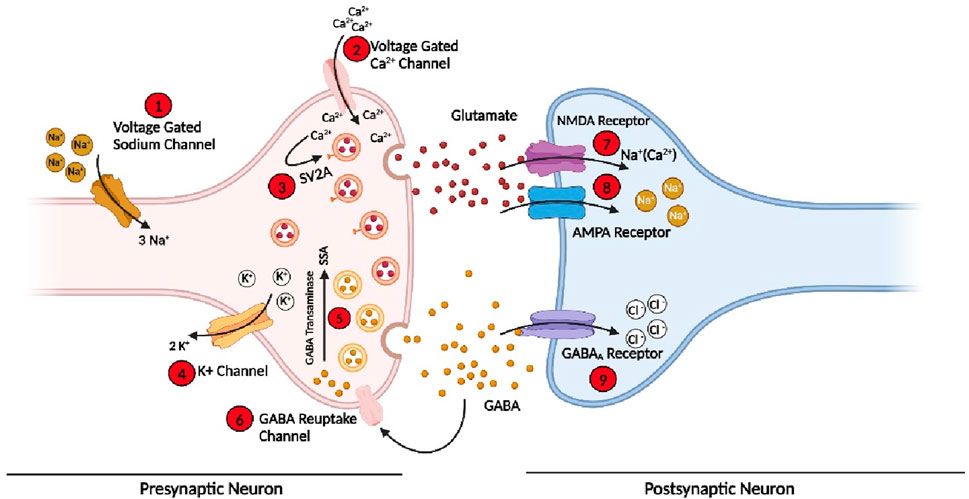
Figure 2. Various receptors, membrane protein channels, and enzymes involved in the initiation and propagation of seizure and the targets for the current AEDs and future drugs. (Bio-render online version was used for this figure; for abbreviations, please see the list of abbreviations).
3 Plants/medicinal plants and phytochemicals
Phytochemicals, also termed phytonutrients, are bioactive compounds produced by different plants in various parts such as stem, leaves, roots, and flowers as a result of primary and secondary metabolism (Nahar et al., 2020). These bioactive compounds play a significant role in the growth of plants or a host’s defense against various pathogens and competitors (Bose et al., 2020). There are countless phytochemicals, however, only a small number have been identified (Xiao and Bai, 2019). Phytochemicals include polyphenols, alkaloids, carotenoids, terpenoids, flavonoids, limonoids, coumarins, indoles, isoflavones, lignans, organosulfur, catechins, furyl compounds, phenolic acids, stilbenoids, isothiocyanates, saponins, procyanidins, phenylpropanoids, anthraquinones, ginsenosides and so on (Xiao and Bai, 2019; Welcome, 2020). These phytochemicals have been considered one of the most widely used treatment options in different countries for the treatment of various diseases such as cancer, neurological disorders, and other virological infections (Behl et al., 2021; George et al., 2021; Yadav, 2021; Lim and Park, 2023). These phytochemicals have multifunctional properties such as antioxidant, anti-inflammatory, antidiabetic, antimicrobial, anticancer, and immunomodulatory, and they act as antagonists and agonists for various types of inhibitory and excitatory receptors in the body, especially in the CNS. Blood brain barrier (BBB) is one of the main hurdles in developing drugs against various neurological disorders, as most of the compounds with high molecular weight cannot cross and attenuate BBB (Akhtar et al., 2021; Chowdhury et al., 2021; Xiong et al., 2021; Yadav, 2021; Correia et al., 2022). However, it has been reported that most phytochemicals such as allopregnanolone, asiaticoside, berberine, catalpol, curcumin, and many more can cross BBB and attenuate the inflammation and hyperpermeability in various pathological conditions (Yadav, 2021).
Several studies have been reported on the efficacy of plant extracts and phytochemicals against various diseases related to the central nervous system (Ayeni et al., 2022; Luthra and Roy, 2022). Curcumin, generally used as a spice in food, is reported to have a promising anti neurodegenerative effect in animal models and also in humans, especially against dementia. In addition, spicatoside, resveratrol, broccoli, and many more have been studied for their effect against various neurological and neuroprotective efficacy (Subedi et al., 2020). Moreover, medicinal plants (phytochemicals and metabolites) have been extensively used for the treatment of various neurological diseases, especially epilepsy in most of the low- or middle-income countries (Asiimwe et al., 2021; Birhan, 2022). These plants, as a treatment option for epilepsy, have very low cost, high availability, and fewer side effects as compared to other treatment options (Sucher and Carles, 2015; Kaur et al., 2021). These play a significant role in the treatment of epilepsy by interfering with the excitatory pathway or inhibitory pathway. Hundreds of plants have been extensively studied in vitro and in vivo (Khan et al., 2020; Kaur et al., 2021). However, only a few have reached clinical trials. Similarly, Cannabidiol is a bioactive metabolite found in marijuana, a plant species that has high antiepileptic properties (Kaur et al., 2021). Therefore, in 2018, the USFDA approved CBD-rich Epidiolex against two severe forms of epilepsy in children (Golub and Reddy, 2021). In addition, CBD-based treatment is currently in phase 4 clinical trials for the treatment of refractory epilepsy (Kaur et al., 2021). A lot of work has been done on the use of plant extract and isolated phytochemicals against epilepsy; we will discuss their targets and the mechanism for the treatment of epilepsy.
4 Modulation of the inhibitory system
The imbalance in the excitatory and inhibitory neuronal systems in the brain leads to seizures and epilepsy. Different types of neurotransmitters in the brain play an important role in the pathophysiology of epilepsy. Among them, GABA and glutamate are the key neurotransmitters directly involved in the excitatory and inhibitory pathways (Jangra and Budhwar, 2022). Among inhibitory neurons and neurotransmitters, GABA is the key inhibitory neurotransmitter released in the brain synapses, accounting for more than 40% (Treiman, 2001). Glutamic acid decarboxylase (GAD) enzyme converts glutamate to GABA, which is then released from the presynaptic GABAergic neuron and acts on the post-synaptic neuron. GABA has three receptors, i.e., GABAA, GABAB, and GABAC, on the post-synaptic neurons (Briggs and Galanopoulou, 2011), and among them, GABAA is of critical importance as this receptor is involved in controlling and balancing action potential and main target of the AEDs. When GABA is releases from the presynaptic neuron, it attaches to the GABAAR on the post-synaptic neuron, allowing the influx of Cl− ion and hence controlling the action potential by balancing the internal environment (Kullmann et al., 2005). In addition, there is a GABA reuptake channel on the presynaptic neuron, which allows the influx of GABA after performing function or extra GABA released in the synapses, as shown in Figure 2. In the case of epilepsy, dysregulation occurs in the inhibitory system, especially in GABAAR, lower production of GABA by GABAergic neurons, and/or catabolism of GABA, and hence, imbalance occurs, which leads to epilepsy (He et al., 2023). These inhibitory neurotransmitters and receptors are the main target of the drugs, and about 35% of the available pharmacological drugs target the inhibitory pathway of CNS.
It has been reported that several plant extracts and isolated phytochemicals from various plants have the ability to modulate the inhibitory system in several ways. (Kennedy and Wightman, 2011). One of the possible mechanisms reported is plant extracts, and their phytochemicals could increase the levels of GABA in the brain by activating enzymes responsible for the synthesis of GABA. Recently, Ali Mohammad and coworkers evaluated the epileptic potential of the stem bark extract of the medicinal plant Vateria indica Linn, which is commonly known as White Damamr. The authors first analyzed the phytochemical profile of the plant and found that the plant is rich in various bioactive compounds, especially flavonoids, glycosides, phenolics, tannins, saponins, polysaccharides, and steroids. They used three different mice models, the maximal electrical shock (MES) model of epilepsy, ionized (INH) induced model, and pentylenetetrazol (PTZ) model, and evaluated the effect of the stem bark extract against various parameters such as seizure onset time, duration of convulsions, as well as estimated the level of GABA in the brain using two different doses of 250 and 500 mg/kg and compared with the standard AEDs diazepam. The authors concluded that the plant extract showed strong antiepileptic activities by increasing the onset time of the seizure and decreasing the duration of convulsions. The authors documented that the extracts lead to an increase in the level of GABA in the brain, especially in INH and PTZ models, which could be the possible mechanism of the extract (Alshabi et al., 2022). The authors did not mention the mechanism of how the extract, or its phytochemicals, increases the GABA level in the brain. However, as previously reported, some phytochemicals increase the activity of L-glutamate decarboxylase (L-GAD), an enzyme responsible for the conversion of L-glutamate to GABA, and therefore, the level of GABA increases in the brain, especially in the hippocampus which leads to the activation of inhibitory pathway and help in the control of seizure (Kandeda et al., 2021a). In addition, there are some author limitations of this study, such as the death rate in the MES group, which was 50%, which is higher and could not be acceptable as no reasons were provided. Similarly, further extensive investigation could be carried out to study the proper mechanism of action of the extracts; safety, cytotoxicity, other side effects, and specific dosage are critical.
Similar results on the effect of plant extract on the alteration of GABA levels have been reported by Kandeda et al. (2021b). They used a hydroethanolic extract of roots of Pergularia daemia against a PTZ-induced temporal lobe epileptic mice model. The authors used four different concentrations of 1.6, 4, 8, and 16 mg/kg and compared the results with the clinically approved drugs sodium valproate. They reported that the concentrations 4–16 mg/kg have a promising antiepileptic potential and protected the mice against myoclonic jerk and generalized tonic-clonic jerk compared to the sodium valproate. Similarly, all the doses increased the seizure score. As altered GABA, GABA-T and L-GAD have been reported in PTZ kindling-induced models. Here in this study, the authors revealed that this plant extract increased the concentration of GABA by 240% in groups treated with 4 and 8 mg/kg, which is almost 3-fold higher than the increase in the sodium valproate group (96%). They also confirmed that PTZ leads to the reduction of GABA levels by 71%. In addition, they also documented the effect of this plant’s extract against the inflammatory biomarker, cognitive functions, hippocampal neuronal damage, and oxidative stress marker, and revealed that it leads to the improvement of all these parameters as this plant is rich in bioactive compounds such as cardenolides, phenols, alkaloids, flavonoids, triterpenes, saponins, glycosides, cardiac glycosides, saponins, and carbohydrates (Sridevi et al., 2014; Chandak and Dighe, 2019). Similarly, the roots extract of P. daemia has also been investigated against the pilocarpine (PILO)-induced (Kandeda et al., 2017) and kainic acid (KA) induced mice models (Kandeda et al., 2021a); in both studies, it showed promising potential against epilepsy. Therefore, this plant could be used as a treatment option against temporal lobe epilepsy after further investigation of its safety and toxicity. Similar studies including (Yempala et al., 2014; Kandeda et al., 2022) have also been reported on the alteration of GABA level by various isolated phytochemicals or plant extracts. Different types of plant extracts extracted phytochemicals, the model and species used, and the dosage reported against epilepsy targeting GABA have been summarized in Table 1.
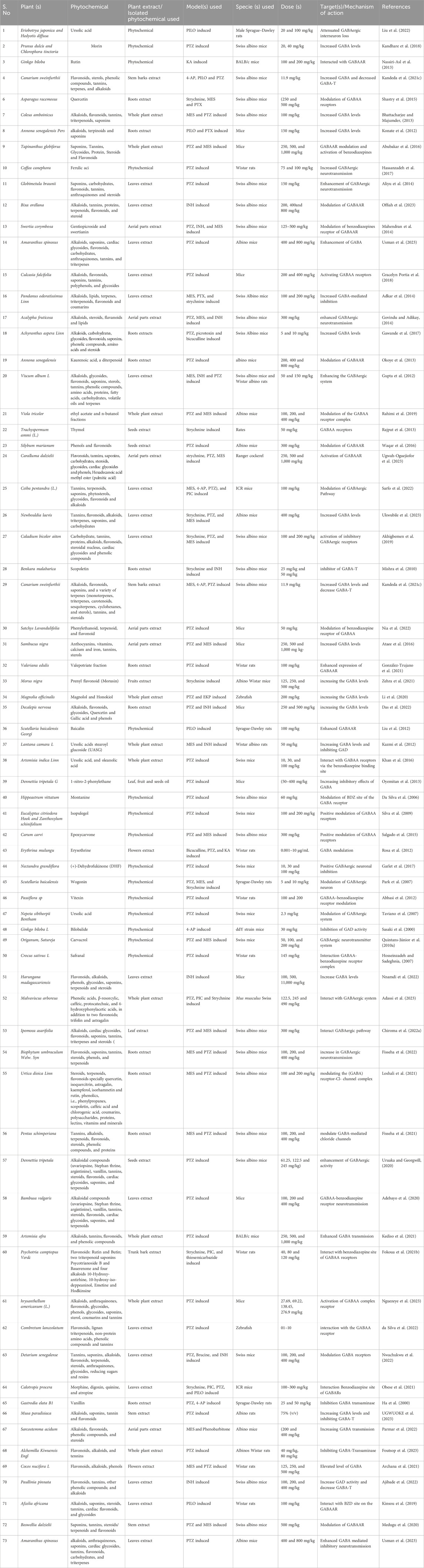
Table 1. Effect of plant extracts and isolated phytochemicals from various plants, their mechanism of action, against various models epilepsy through the modulation of inhibitory system.
Secondly, the extracts and phytochemicals can also interact with the GABAAR. GABAAR is also a ligand-gated channel, and GABA neurotransmitters act as a ligand. When GABA neurotransmitters bind with the GABAAR on postsynaptic neurons, that leads to the opening of chlorine channels and, finally, the influx of Cl− into the cell, which reduces neuronal excitability by hyperpolarization of the membrane (Ghit et al., 2021). Dysfunction or mutation in the subunit(s) of GABAAR fails to hyperpolarize the membrane (Mele et al., 2019). Several studies have reported the interaction of phytochemicals and plant extracts with GABAAR, causing hyperpolarization and reducing neuronal excitability. Arenaria kansuensis Maxim. (AKM) and Asterothamnus centrali-asiaticus Novopokr. (ACN) are considered the two important medicinal herbs worldwide, especially in Chinese medicine, Persian, ancient Greek, Central Asian, and Ayurved. The phytochemicals analysis of these plants showed that they are rich in alkaloids and flavonoids and possess various medicinal properties (Wang et al., 2016a; Wang et al., 2016b). Liu et al. investigated the structure-activity relation of flavonoids isolated from these plants with the benzodiazepine site of GABAAR and searched for anticonvulsive compounds in the PTZ-induced epilepsy mice model. They revealed that several flavonoids isolated from the whole plant extract of these plants had a strong binding affinity with GABAAR. In addition, 2′,4′,5,7-tetrahydroxy-5′, 6-dimethoxyflavone, a flavonoid compound isolated from the ACN, exhibited potent anticonvulsant activity. Finally, the authors concluded that flavones could be considered strong antiepileptic drugs that have a strong affinity for the GABAAR receptor and can be used for the treatment of seizures (Liu et al., 2018). However, further studies are required to determine the safety, further efficacy, side effects, and dosage of the extract or isolated phytochemical flavone. Similarly, the interaction of Euterpe oleracea Martius (Açai) extract with GABAAR has also been reported (Muto et al., 2022). HPLC/MS analysis of Acai revealed that Açai stone possesses high levels of various polyphenolic compounds such as caffeic acid, cinnamtannin, procyanidin, catechin, polymeric proanthocyanidins, followed by traces of another phenolic compound (Muto et al., 2021). In this study, the authors used PTZ-induced epileptic rats as an epilepsy model and used a specific dose of 300 mg/kg and compared with the marketed drug diazepam and evaluated by electroencephalographic (EEG) profiling. The authors reported that there is no significant difference between the experimental group and the treatment group with diazepam. They further conclude that Euterpe oleracea stone (EEOS) extract interacts with the benzodiazepine subunit of GABAAR and exerts anticonvulsive activity. Therefore, this extract could also be used to treat epilepsy. However, extensive investigation could be required on the safety and further efficacy. Similarly, several studies have been reported on the interaction of plant extract and phytochemicals with GABAAR, which could alleviate or treat seizures and epilepsy, summarized in Table 1.
The third possible mechanism through which the extract or phytochemicals interact with the inhibitory pathway is the targeting of GABA-T. In the pre-synaptic neurons, the GABA-T converts the GABA into succinic semialdehyde (SSA) and, therefore, makes the neuron GABA deficient and elevates the glutamate levels, which are excitatory neurotransmitters (Lu et al., 2023). It has been reported that various phytochemicals and bioactive molecules from various medicinal plants can interact with this enzyme, leading to increased GABA levels and decreased glutamate levels to balance the excitatory and inhibitory potential, hence alleviating seizure. Mishra et al. investigated the anticonvulsive effect of Benkara malabarica (Linn.) ethanolic roots extract in strychnine-induced and INH-induced acute convulsion mice model (Mishra et al., 2010). This is a medicinal plant and is commonly used in various regions of India for the treatment of various types of diseases, including epilepsy (Nadkarni, 1954). The authors used 2 mg/kg for strychnine and 300 mg/kg for the INH model and compared with the group treated with standard drugs like phenytoin and diazepam. The authors reported that the plant extract has strong antiepileptic properties and could be used as a treatment option after further investigation. The authors hypothesized that the antiepileptic activities of the plant extract are due to the interaction of phytochemicals with GABA-T. To prove this hypothesis, the authors performed a GABA-T activity assay as described by Salvador and Albers (Salvador and Albers, 1959). The authors documented that the plant extract had GABA-T inhibitory activity (IC50 = 0.721 mg/mL). In addition, the GABA-T inhibitory activity of Scopoletin, which is a major constituent of the extract, was IC50 = 10.57 μM. They further concluded that GABA-T inhibitory activity might be due to scopoletin alone or in combination with other compounds in the extract.
Similarly, another study has reported that the aqueous stem bark extract of C. schweinfurthii alleviates seizures (Kandeda et al., 2021c). They also reported that the GABA pathway is involved. The authors used various doses against different animal models of epilepsy and reported that the different doses of extract showed significant antiepileptic activity against various animal models. Further studying the mechanism of action of the extract, they concluded that the phytochemicals in the plant extract interacts with the GABA pathway as after treatment with the extract, the GABA levels increased while the GABA-T levels were reduced. They concluded that the antiepileptic activity of Canarium schweinfurthii was due to the inhibition of GABA-T by the bioactive compounds present in the extract, which could be used alone or in combination with other drugs for seizure control. Furthermore, this plant has a double mechanism of action (increased GABA and decreased GABA-T); therefore, it could be a strong AED in the near future after further investigation. Several other studies have been reported on the interaction of extracts and various phytochemicals with the GABA-T, as summarized in Table 1.
5 Modulation of excitatory pathway
Glutamate is one of the principal excitatory neurotransmitters in the CNS that plays a critical role and performs various functions such as learning, memory, synaptic plasticity, nerve degeneration, neurogenesis, and many more (Haroon et al., 2017). However, excessive glutamate in the body leads to severe pathogenic events that may then cause diseases such as Alzheimer’s disease, Parkinson’s, and epilepsy. The receptors for glutamate are broadly categorized into two classes, i.e., ionotropic glutamate and metabotropic receptors (Afshari et al., 2020). The ionotropic receptors include NMDA (Ca2+ influx), kainate, and AMPA (Na+ Influx) receptors, while the latter one includes G protein-coupled receptors that activate the intracellular cascade (Crupi et al., 2019). Among these receptors, the NMDA and AMPA play a significant role in the initiation and propagation of epileptic seizure as these both allow the high influx of Ca+ and Na+ ions, respectively, which leads to the imbalance of excitatory and inhibitory pathways and therefore, these two receptors represent key clinical research targets (Hanada, 2020). It is well known that the agonists for these two receptors lead to the development of epilepsy in animals or humans. While antagonist leads to the inhibition of seizures by blocking respective receptors, and therefore, these are potential targets for the development of drugs against epilepsy. Several studies have reported that medicinal plants and phytochemicals can also lead to the modulation and inhibition of these receptors, which may lead to the development of potent drugs against epilepsy.
Zizyphi Spinosi Semen (ZSS) is a medicinal plant widely used in Chinese medicine for the treatment of various diseases, including insomnia and some other psychiatric disorders (Zhao et al., 2016). The phytochemical analysis of this plant has reported that it contains various types of bioactive compounds that have strong medicinal values and are used for the treatment of various diseases. Several saponins have been identified from ZSS, including seven types of jujuboside (A-H) (Xiao et al., 2018; He et al., 2020). Xi Wang reported that Jujuboside A has strong antiepileptic activities by increasing the levels of GABA in the hippocampus, which are inhibitory neurotransmitters (Wang et al., 2015). Similarly, Panpan Song et al. reported that jujuboside B (jub B) also leads to an increase of the GABA as well as overexpression of GABAAR, which plays a critical role in the control of seizures. A febrile seizure is a non-epileptic seizure affecting children between 3 months and 5 years, and phytotherapeutic treatment could also help in the control of this seizure by targeting the excitatory pathway of the CNS (Song et al., 2017; Jin et al., 2023 hypothesized and then proved that jujuboside can modulate the excitatory pathway by inhibiting the glutamate receptor AMPA and could be used as a potent agent against febrile seizure (Jin et al., 2023). In this study, they used EEG recording for the monitoring of current in the mouse model of febrile seizure and to evaluate the efficacy of the Jub B as shown in Figure 3A. The mass spectroscopy was used for the identification of Jub B in the brain, and they revealed that significant amount of Jub B was present in the brain, it means that it can easily cross the BBB which is one the main barrier in the development of drugs against neurological disorders. The authors then finally concluded that Jub B can suppress the neuronal excitation in the hippocampus by inhibiting the activity of AMPAR and, therefore, cause relief from febrile seizure. JuB B showed a strong antiseizure effect against epileptic seizures; however, similar targets are also involved in epileptic seizures. Therefore, it could be used against various animal models of epilepsy and could show a strong antiepileptic activity based on the above results.
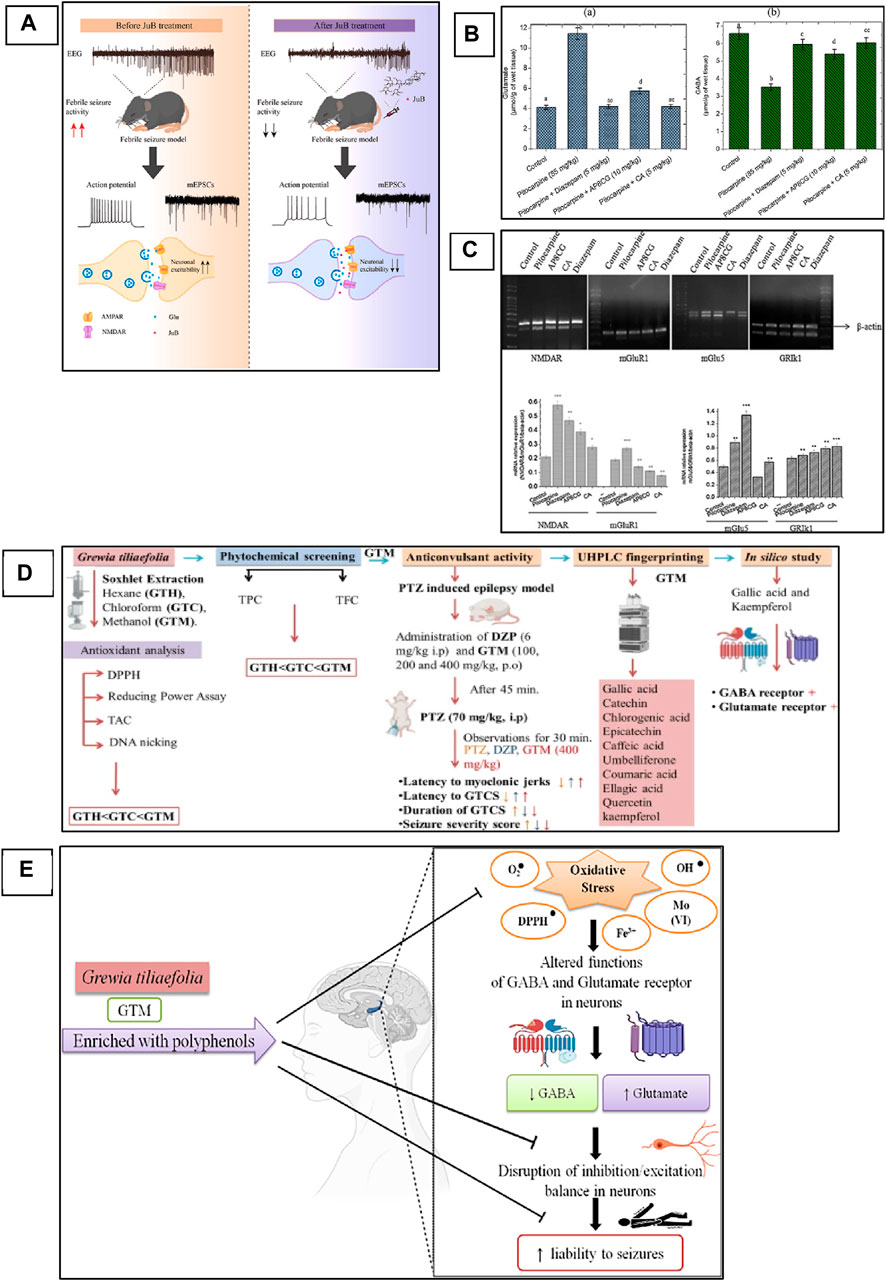
Figure 3. Phytochemicals can modulate NMDAR and AMPAR in the animal model of epilepsy. (A) Mechanistic overview of the effect of Jujuboside B from medicinal plants against febrile seizures and the mechanism of action on AMPA receptor (Jin et al., 2023). Copyright permission@ Elsevier 2023. (B) apigenin-8-C-glycoside (AP8CG) and Chlorogenic acid (CA) effects on the levels of glutamate and GABA in the hippocampus of mice and their comparison with standard drugs and control group. (C) mRNA profile of NMDAR, mGlu1, and mGLU5 in experimental groups (treated with AP8CG and CA and control groups (Aseervatham et al., 2016). Copyright permission@ Elsevier 2023. (D) A mechanistic overview of the antiepileptic activity of Grewia tiliaefolia in mice followed by in silico analysis of important phytochemical that involved in the modulation of NMDAR. (E) Possible antiepileptic mechanism of Grewia tiliaefolia (Rajput et al., 2023). Copyright permission@ Springer Nature 2023.
Similarly, apigenin-8-C-glycoside (AP8CG), commonly known as vitexin, and Chlorogenic acid (CA), are two important phytochemicals present in various plants and widely used in medicines (Liang and Kitts, 2015). The antiepileptic activities of these two compounds have been reported in PILO-induced epilepsy mice models (Aseervatham et al., 2016). The authors reported that they administered a 10 mg/kg dose of AP8CG and a 5 mg/kg dose of CA to mice, and the results were compared with the standard drug diazepam. The authors reported that both compounds showed potential antiepileptic activities. However, CA showed stronger activities on the levels of glutamate and GABA than AP8CG, as shown in Figure 3B. By explaining the mechanism of action, the authors revealed that these compounds selectively inhibit the expression of NMDAR, Metabotropic glutamate receptor 1 (mGlu1), and Metabotropic glutamate receptor 5 (mGlu5). They also evaluated the mRNA profile of NMDAR, mGlu1, and mGLU5 and compared them with the standard drugs and control groups and reported that a significant association was observed in the experimental groups and other groups, as shown in Figure 3C. Therefore, these two compounds need further investigation and could be used for the treatment of epilepsy.
Recently, Ankita Rajput and colleagues carried out a comprehensive study on the antiepileptic activity of aerial parts extract of Grewia tiliaefolia in the PTZ induced mice model of epilepsy and further screening of the plant followed by in silico analysis of the phytochemical as shown in Figure 3D (Rajput et al., 2023). The other members of the family of this plant showed neuroprotective activities, antidepressants, anti-anxiety, etc (Jebin et al., 2019). Here in this study, the authors reported that the plant extract significantly increased the latency of myoclonic jerks and generalized tonic-clonic seizures (GTCS). In addition, it also reduced the severity of seizures associated with the GTCS. Further, they analyzed the extract with HPLC for phytochemical profile and found that the extracts have several bioactive compounds that could be used for various neurological diseases. From the in silico analysis of these phytochemicals, the authors revealed that gallic acid and kaempferol compounds from the extract have shown interaction as agonists with GABA and antagonists with Glu-NMDA. Therefore, they concluded that the possible antiepileptic effect of the extract is due to the increase of GABA and the decrease of Glu-NMDA. They further revealed that this effect could be due to these two compounds, and the possible mechanism can be, as shown in Figure 3E. However, further studies could be carried out to confirm the involvement of these two compounds that can be used then as a potent antiepileptic agent in the future. A similar study has been reported on the computational investigation of W. somnifera and their in silico analysis of the phytochemicals (Kumar and Patnaik, 2016). The authors analyzed 25 different phytochemicals that showed related activities and found that Anaferine, Beta-Sitosterol, Withaferin A, Withanolide A, Withanolide B, and Withanolide D have shown the interaction with GluN2B containing NMDAR, which is one of the main targets to control the seizure. Therefore, these compounds could be used in vivo first, and after confirmation and thorough investigation of safety and efficacy, they can be used as potent antiepileptic agents in the future.
Several studies have reported that the phytotherapeutic treatment can modulate NMDAR and AMPAR, which can alleviate seizures and epilepsy. However, there are some limitations to every study. Therefore, to develop a potent antiepileptic agent, extensive investigation could be carried out not only on the reduction of seizure and severity but also on the side effects or safety of the plant or phytochemicals. We have summarized the literature on phytochemicals and plant extracts that modulate the excitatory pathway, especially NMDAR and AMPAR, as shown in Table 2.
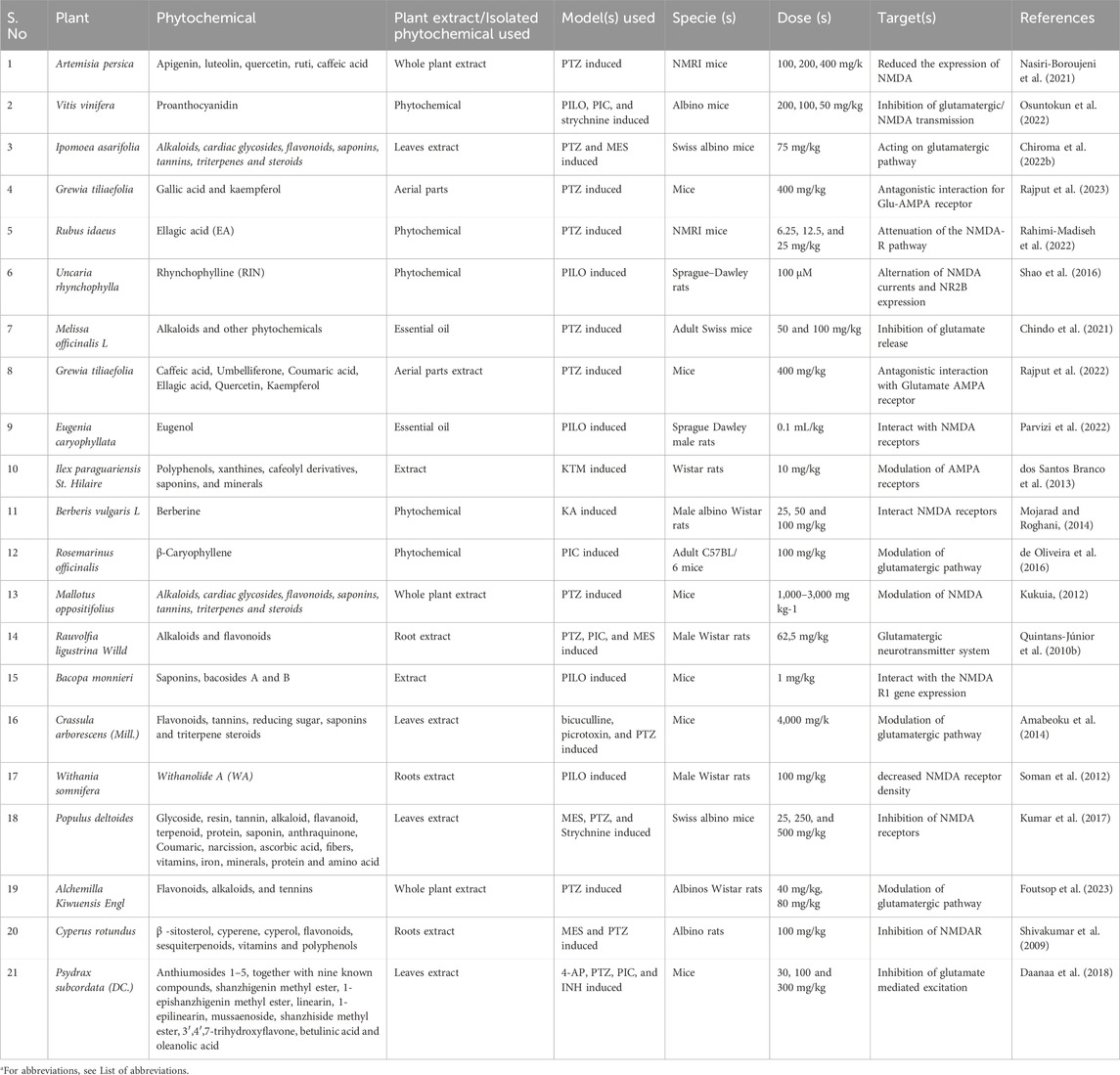
Table 2. The antiepileptic properties of plant extract and purified phytochemicals through the modulation of the glutamatergic pathway (especially NMDARs and AMPARs).
6 Interaction with Voltage Gated Calcium Channels
Voltage Gated Calcium Channels (VGCCs), also termed Voltage-Dependent Calcium Channels (VDCCs), are widely expressed in the CNS of humans and other mammalian and play a critical role in the regulation of synaptic transmission, phosphorylation/dephosphorylation of protein, gene transcription, as well as perform various other functions including the survival and death of the cell and adaptive responses to the synaptic activity (Xu and Tang, 2018). However, alteration in these channels leads to the imbalance of cellular events that causes pathological consequences (Djamshidian et al., 2002). The abnormal activation of these channels leads to the influx of Ca2+ into the cell, which plays a critical role in the imbalance of the action potential and finally helps in the triggering and propagation of seizure. Based on the electrophysiological studies, the VGCCs are classified into five different types, i.e., L-, N-, P/Q-, R-, and T-type (Catterall, 2000). The detailed explanation of VGCCs regarding structure, function, types, and role in the pathophysiology of epilepsy has been comprehensively explained by Jie and Feng, as shown in Figure 4A (Xu and Tang, 2018), and Rajakulendran and Michael. Several clinically approved drugs target these channels for the control of seizures and the treatment of epilepsy. Similarly, medicinal plants have diverse bioactive compounds that can effectively block these channels and can control seizures. Currently, various studies on the use of plant extracts and phytochemicals against epilepsy targeting VGCCs have been reported. We suggest that if extensive investigations of these phytotherapeutics are carried out to determine their safety, efficacy, and dosage, then these could be used as potential agents for the treatment of epilepsy.
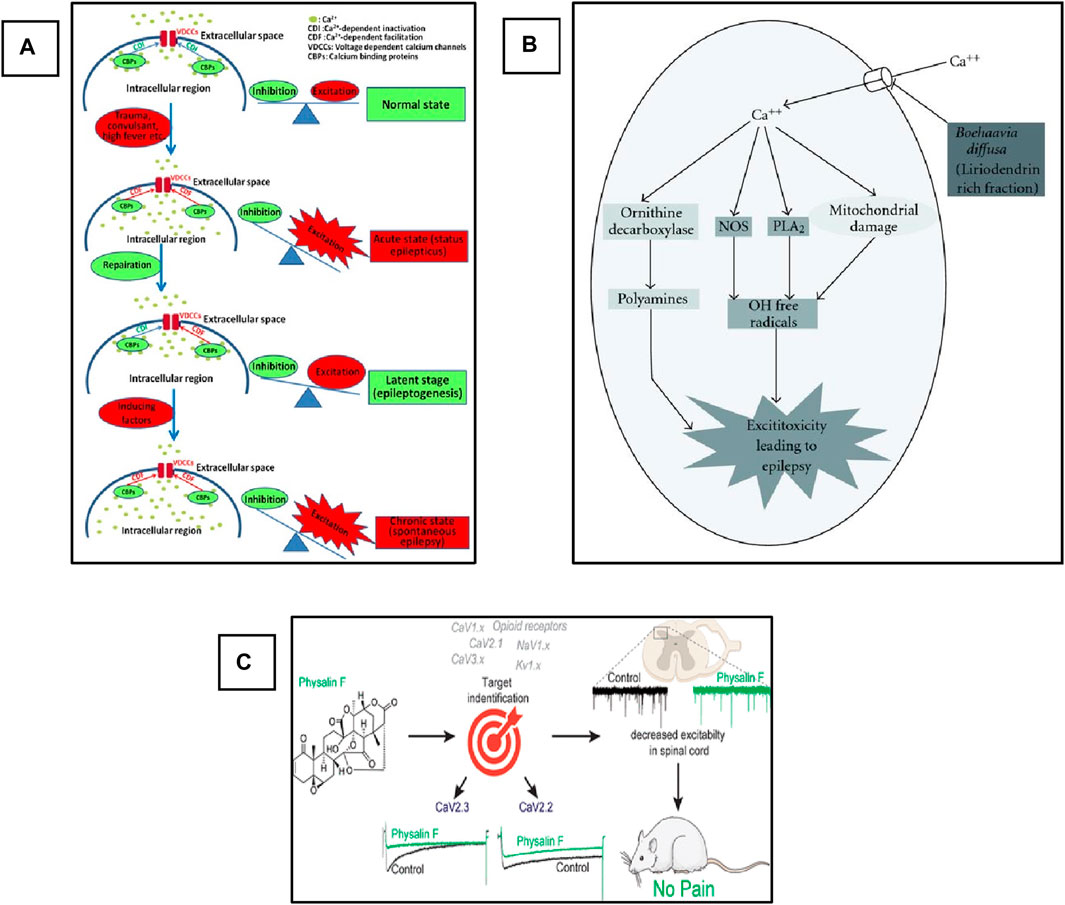
Figure 4. Schematic representation of the role of VGCCs in epilepsy and pharmacological targets for phytochemicals. (A) Role of Ca+2 channels in the development of epilepsy. Adopted from (Xu and Tang, 2018). Copyright permission@ MDPI 2018, (B) Possible anti-convulsant mechanism of (B). diffusa on the inhibition of VGCCs. Adopted from (Kaur and Goel, 2011). Copyright permission@ Hindawi 2011. (C) Schematic representation of inhibition of CaV2.3 (R-Type) and CaV2.2 (N-Type) Voltage-Gated Calcium Channels by Physalin F in pain model (Shan et al., 2019). Copyright permission@ ACS 2019.
Mandeep and Rajesh evaluated the anticonvulsive activity of dried root extract of Boerhaavia diffusa (BD) in the PTZ-induced model of Swiss albino mice (Kaur and Goel, 2009). BD contains several phytochemicals that have a potential medicinal value, including flavonoids, terpenoids, punarnavoside, Liriodendrin, liriodendrin, and many more (Sahu et al., 2008). At the end of the 20th century, it was reported that the liriodendrin isolated from the methanolic extract of BD showed antagonistic activity for calcium channels (Lami et al., 1990; Lami et al., 1991). In addition, the root extract of BD can be used for the treatment of epilepsy (Adesina, 1979). Therefore, the authors decided to investigate the anticonvulsive activity of whole root extract, phenolic compounds, and liriodendrin in various doses and compared the results with the standard drug diazepam-treated group. The authors reported that more than 80% protection and recovery were observed in the group treated with BD crude extract and liriodendrin. The authors concluded this activity could be due to the antagonistic activity of the liriodendrin, and they designed a mechanistic pathway of the possible mechanism of the plant extract against VGCCs, as shown in Figure 4B. Briefly, the authors concluded that the observed anti-convulsant activity was attributed to its VGCCs antagonistic action. This activity was retained only in the liriodendrin-rich fraction isolated from Boerhaavia diffusa. Additionally, this was confirmed by the significant anti-convulsant activity of the liriodendrin-rich fraction in BAY k-8644-induced seizures. In addition to extracts and fractions having antiepileptic activity, they act on i) other ionic channels such as sodium and potassium, ii) antioxidant activity, iii) neurotransmitter modulation, and iv) anti-inflammatory activity (Kaur and Goel, 2011).
Recently, a very interesting study carried out by Paz and colleagues used a natural product, Argentina C, derived from the native American medicinal plant species Parthenium incanum against mouse model of postoperative pain (Duran et al., 2023). The authors reported that this compound attenuates the pain by inhibiting the two most important VGSCs and VGCCs. They concluded that the compounds have very strong dual inhibiting properties and could be used for the treatment of pain. On the other hand, these two channels also play a significant role in the pathophysiology of epilepsy and are two important pharmacological targets in epilepsy. In addition, drug-resistant epilepsy is mostly treated by a combination of two drugs with different mechanisms of action. Here, this plant-derived compound targets and inhibits two different channels. Therefore, if this compound is used against various epileptic models, especially drug-resistant models, it could show strong activity against seizure and can be used as a potent antiepileptic agent in the future. Similarly, another natural compound, Physalin F, isolated from the aerial parts extract of medicinal herb, Physalis acutifolia, has been used against neuropathic pain. They also reported that this compound blocks CaV2.3 (R-type) and CaV2.2 (N-type) VGCCs and decreases excitation in the spinal card, as shown in Figure 4C (Shan et al., 2019). This compound can also be used against epilepsy in animal models, and it could give promising results as these VGCCs are also the main targets in the development of drugs against epilepsy. In addition, another study reported the use of Betulinic acid (BA) derived from lavender Hyptis emoryi and reported that it attenuates nerve injury-associated peripheral sensory neuropathy by inhibiting the N and T-type calcium channels, which are also the main target of epileptic drugs. The results indicated that the BA leads to the downregulation and inhibition of the Cav3.2 and Cav3.3 calcium channels (Bellampalli et al., 2019). BA could also be evaluated against epilepsy and seizure. As here in this study, it showed promising results in neuropathy.
Several studies have been reported on the inhibitory effect of various types of plant extracts and derived compounds in various diseases. These compounds could be investigated against epilepsy. We summarized the details of some studies that reported various medicinal plants and phytochemicals for the treatment of seizures and epilepsy, as shown in Table 3.
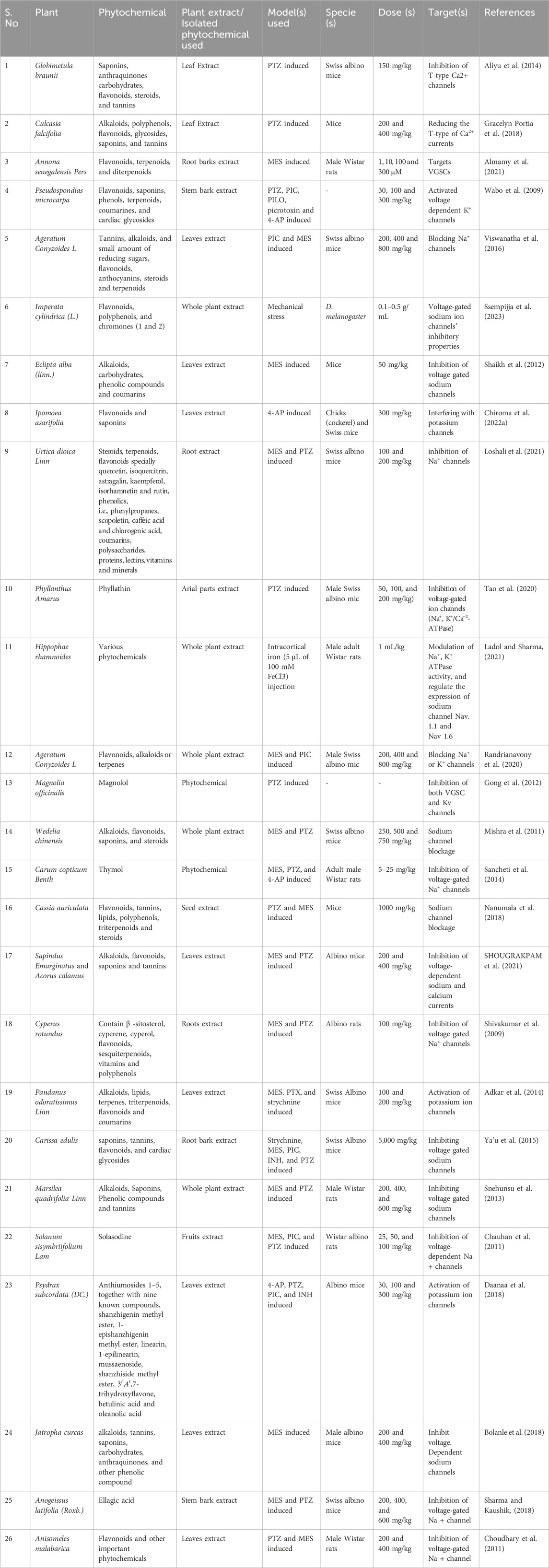
Table 3. Anticonvulsive effect of plant extract and phytochemicals isolated from various plants targeting VGCCs, VGSCs, and Kv.
7 Modulation of the voltage-gated sodium channels
Voltage-gated sodium channels (VGSCs or NaVs) are membrane proteins that selectively conduct Na+ across the membrane. These channels are the mediators of intrinsic neuronal excitability and are responsible for the generation and propagation of action potential (Kwong and Carr, 2015). However, the abnormal expression and function of these channels lead to neurological disorders such as migraine, epilepsy, neuropathy, and pain. VGSCs have three functional states, as shown in Figure 5 (Mantegazza et al., 2010). During the resting membrane stage of the neuron, these are in the closed state. It opens in a few hundred microseconds in response to membrane depolarization by the process called activation and allows the influx of Na + to the cell, which is then inactivated in a few milliseconds by the process termed fast inactivation. The time from the activated state to the inactivated state is the most important, and the activation of channels for a longer time or longer time required from the activated state to the inactivated state is the main cause of imbalance in excitatory and inhibitory pathways that leads to epilepsy and other neurological disorders. The inhibition or blocking of VGSCs can control the seizure (Agbo et al., 2023). Therefore, several clinically approved AEDs are targeting VGSCs, including the widely used phenytoin, lamotrigine, and carbamazepine. Several drugs that are currently in various phases of clinical trials are also targeting these channels. Much research has been carried out on the use of plant extract and phytochemicals to inhibit VGSCs, which are widely used in various countries for the control of seizures and treated epilepsy.
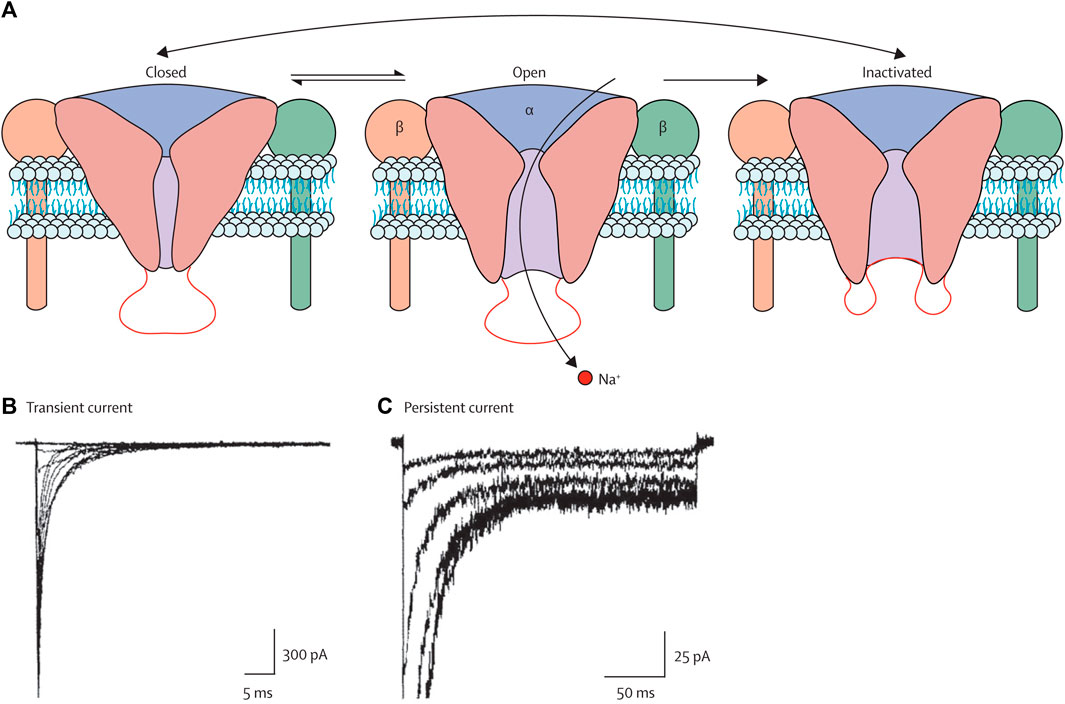
Figure 5. Three states of the VGSCs, i.e., closed, open, and inactivated states (Mantegazza et al., 2010). Copyright permission@ Elsevier 2010.
The USFDA and the European Medicines Agency (EMA) approved cannabidiol (CBD) in the purified form from the cannabis plant against Dravet syndrome and Lennox−Gastaut syndrome and are currently available under the trade name of Epidiolex (Anderson et al., 2021a). The approval and strong anticonvulsive effect of CBD attract the researcher whether other phytocannabinoids also have similar anticonvulsive properties. Therefore, Anderson et al. also evaluated the four different types of lesser studies of phytocannabinoids against different types of epilepsy and found that these compounds show stronger anticonvulsive properties (Anderson et al., 2021a; Anderson et al. 2021b; Anderson et al. 2022). However, the mechanism was unknown. Recently, they hypothesized that as the CBD has Na+ channel modulation properties, it is possible that the other phytocannabinoids could modulate the sodium channels. They investigated the mechanism of action of these phytocannabinoids and found that, among five studied compounds, two were sodium channel blockers and had an almost similar mechanism of action as CBD (Milligan et al., 2023). However, further confirmation could be carried out in animal models followed by humans. From these results, we can conclude that the cannabis family has many members of different plant species, and it could contain different types of phytochemicals and metabolites that have similar mechanisms of action and could control the seizure by action on sodium or can treat any other channels that can control the seizures. Saponins are produced by plants and lower animals, as well as bacteria (Singh and Goel, 2016). These play a central role in various diseases, such as hypertension, atherosclerosis, inflammation, cognitive impairments, allergic reactions, and cancer. Singh et al. reported the anticonvulsive effect of saponins extracted from the roots of Ficus religiosa. The authors used various epileptic mice models and found that the saponins showed strong anticonvulsive effects in various models. In addition, they concluded that they modulated the GABAergic, Na+, and Ca2+. Furthermore, they documented that they only deactivated the VGSCs and VGCCs without affecting the ligand-gated channels (Singh and Goel, 2016). These results are very interesting as they target three different channels and could be used against intractable epilepsy (When an individual seizure does not respond to at least two clinically approved drugs with different mechanisms of action, termed intractable epilepsy). Further research is needed to study this compound in drug-resistant epilepsy models and investigate the safety and efficacy of specific doses. The plant extracts, various isolated phytochemicals, and their mechanism of action on the VGSCs are summarized in Table 3.
Moreover, several studies have been reported on various types of phytochemicals, and medicinal plant extracts invitro against epilepsy by modulation of the Na + channels and show promising activities (Lai et al., 2022; Milligan et al., 2022). These plants and compounds could be investigated on the animal models to further evaluate their efficacy, toxicity, safety, and dose determination.
8 Modulation of voltage-gated potassium channels
Potassium channels (Kv channels) are the largest family, consisting of twelve sub-families. There is a total of 350 expressed ion channels have been reported in the mammalian brain, of which 145 are voltage-gated channels. Among the voltage-gated channels, 40 are Kv channels, which are further classified into 12 subfamilies (Kv1-Kv12) and represent the largest group of channels (Köhling and Wolfart, 2016). These are activated by the depolarization and deactivated by the repolarization (Köhling and Wolfart, 2016; Ranjan et al., 2019). Kv channels plays a significant role in the homeostasis of the cell’s internal environment by the efflux of potassium (K+) ions from the cell and, therefore, plays a role in the control of action potential and hyperpolarization. However, when dysregulation occurs in the normal function of these channels, such as the mutation in the subunits of Kv channels is one of the causes of inherited epilepsy (Nikitin and Vinogradova, 2021). Therefore, these are considered the main targets for the development of drugs against epilepsy. It has been reported that the extract of several plants and phytochemicals has the potential to modulate these receptors to help in the control of seizures (Khan et al., 2020).
4-hydroxybenzoic acid (4-hba) is a benzoic acid derivative and is present in various plants, including Dendrocalamus asper, commonly known as bamboo. 4-hba has a lower molecular weight of 138.12 and, therefore, can cross the BBB and cerebrospinal fluid (CSF) (Camusso et al., 2007). In addition, no cytotoxic activities have been reported. Jingli and colleagues investigated the effect of the 4hba on the Kv, and they reported that 4hba enhanced the activity of the potassium channels family Kv1.4 and contributed to the reduction of membrane excitation (Zhang et al., 2018). Therefore, from these results, we can conclude that the 4hba compound could help in controlling the depolarization and excitation of neurons in seizures and, therefore, can be a potent agent against epilepsy in the future. In addition, further investigation can be carried out for the study of other phytochemicals from the same plant and evaluation of their anticonvulsive properties.
Pseudospondias macrocarpa is widely used for the treatment of various diseases, especially for neurological disorders in Africa. It has been traditionally reported that, the plant has a sedative effect on the people who can sit or sleep under this plant (Wickens, 1986). The anticonvulsive activity in six various epilepsy animal models has been reported. The authors reported that 30, 100, and 300 mg/kg ethanolic leaves extract significantly increased the seizure onset time and reduced the frequency and duration of the seizures. In addition, the prophylactic use of the extracts significantly improved the survival rate. The authors did not investigate the mechanism of action of the extract; however, from the previous studies on the other plants and phytochemicals from the same groups, they concluded that the promising ability of the plant extract may probably be due to affecting the GABAergic, NMDA, Kv channels, and nitric oxide cGMP pathways (Adongo et al., 2017). However, further studies should be carried out to find out the exact mechanism of action for further use to treat epilepsy and other diseases. Recently, a similar study was reported by Manville et al., 2023. In this study, they used Salvia rosmarinus, commonly known as Rosemary extract, against epilepsy. In this article, the authors reported that this plant contains two important compounds, carnosic acid and phenolic diterpene, and both showed promising activities on the selective isoforms of KCNQ (Manville et al., 2023). These compounds could be used as potential antiepileptic agents alone or in combination with other drugs.
The type of drug and dosage varies from person to person based on the types and severity of epilepsy. For example, a combination of drugs will be given to control the seizure. It has also been reported that the phytochemicals in combination with modern drugs have a strong antiepileptic effect as compared to the modern drug alone. Manville and Abbott 2018 conducted a very interesting study in which they used two components, mallotoxin (MTX) and isovaleric acid (IVA), of Mallotus oppositifolius leaf extract (Manville and Abbott, 2018). First, the authors evaluated the anticonvulsive effect of these two compounds separately, and they were ineffective. Then they tested the synergistic effect of these two compounds, and interestingly, they showed promising results on the modulation and opening of Kv channels isoform KCNQ2–5, and the activation of these channels is of critical importance in the control of seizure, action potential, and repolarization. After that, they co-administered these two compounds in combination with the modern antiepileptic drug retigabine. From the results, they concluded that the two Phyto flavonoids in combination with the modern molecules were more effective than the two phytocompounds alone (Manville and Abbott, 2018). We can conclude that the medicinal plant extract and phytochemicals have antiepileptic activities alone; however, if we use them in combination with modern drugs, it could further enhance the efficacy of the drug. However, further research could be carried out to determine the effect of the current phytochemicals tested in combination with modern drugs.
Several studies have been reported on the use of plant extract or isolated phytochemicals against various types of epilepsy models. We summarized the recent literature, and Table 3 shows all those plants and phytochemicals that play a significant role in controlling seizures by affecting the Kv channels.
9 Antioxidant and anti-inflammatory activity of plant extracts and phytochemicals
We discussed how a specific receptor, channel, or enzymes play a significant role in the development of epilepsy. In addition, we also discussed that epilepsy is basically the imbalance of the excitatory and inhibitory neuronal pathways, in which when excitation occurs then, the inhibitory neurons fail to control the action potential and repolarize the membrane. However, the dysfunction of these molecular entities involved is not only due to mutations in the genes. There are several reasons which directly or indirectly contribute to the development of epilepsy. For example, different types of microorganisms, such as bacteria, viruses, and fungi, can cause epilepsy. When these microorganisms enter the body or specifically the brain, it causes inflammation due to which different types of interleukins or cytokines are released; it also can activate the autoimmune system, which releases antibodies that can attack different types of receptors and contribute to epileptogenesis and hence considered the cause of epilepsy. Vezzani et al., 2016 summarized comprehensive information on the causes and mechanism of infection and inflammation in epilepsy. In addition, some metabolites and other signalling pathways and receptors are directly or indirectly involved. Plants and phytochemicals can also act on these molecules and can help control seizures and treat epilepsy (Vezzani et al., 2016).
The excessive increase of reactive oxygen species (ROS) leads to an increase in levels of Ca+2 ions, which play a critical role in the initiation and propagation of seizure, as well as results in the entry of the neurotoxin and inflammatory cells, which are considered mediators of seizure (DeLorenzo et al., 2006). Therefore, the reduction and control of ROS help in the control of seizures. The effect of ferulic acid, a phenolic compound found in many plants, has been evaluated against the PTZ-induced model of epilepsy (Hassanzadeh et al., 2017). The authors administered 70 and 100 mg/kg doses of ferulic acid and reported that both of the doses significantly reduced seizure scores, myoclonic jerks, and cognitive decline. The authors investigated the glutathione (GSH) levels, which are the indicators of ROS, and they found that significant differences were found in the treatment group and PTZ controlled group. They finally concluded that the antiseizure effect of ferulic acid was due to the reduction of GSH content in the brain (Hassanzadeh et al., 2017). In another study, Smilin Bell Aseervatham et al., 2020 evaluated Passiflora caerulea L. fruit extract against the PILO-induced model, and it reported that the phytochemicals such as apigenin-6,8-di-C-β-D-glucopyranoside present ameliorate the seizure, cognitive deficit, and oxidative stress (Smilin Bell Aseervatham et al., 2020). Several studies have reported that the phytochemicals target the ROS and reduce the oxidative stress to control the seizure (Table 4).
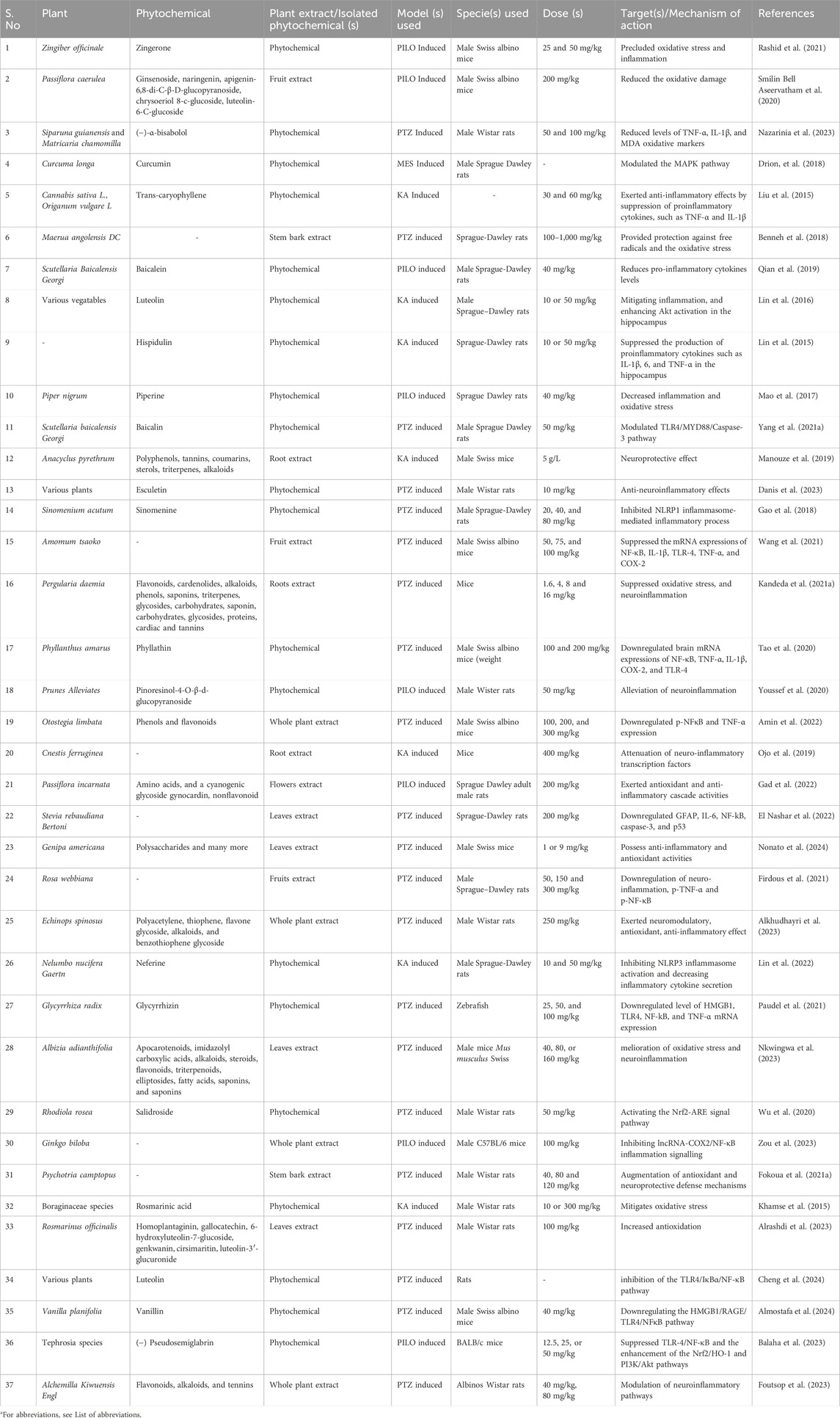
Table 4. Effect of plant extracts and isolated phytochemicals from various plants on inflammation, reactive oxygen species, oxidative stress, and other pathways for the control of seizure and treatment of epilepsy.
Tumor necrosis factor (TNF- α), interferon (IFN- γ), and interleukins such as IL-6 and IL-1β have been reported to cause the failure of the BBB. Similarly, the disparity in pro and anti-inflammatory cytokines further worsens organ damage and is involved in the pathogenesis of epilepsy (Abdallah et al., 2022). IL-6 is a pro-inflammatory cytokine and is considered one of the biomarkers found in the blood and brain of epileptic patients (Youn et al., 2013). Shaimaa and coworkers conducted a comprehensive study on the use of Moringa oleifera seeds extract in pilocarpine-induced epileptic rats with temporal lobe epilepsy (Fayez et al., 2023). The authors used various doses of the extract and reported that the extract extensively modulates the pro and anti-inflammatory cytokines. They further clarify that the extract contained various important phytochemicals that had anticonvulsive effects by suppressing the pro-inflammatory cytokines TNF-α, IL-1β, IL-6, and IFN-ɣ and increasing the levels of anti-inflammatory cytokines TGF-β and IL-10 in the hippocampal tissue of the animal model. The authors also compared the results with the standard drug diazepam treatment group and concluded that the moringa seed extracts possess strong antiepileptic properties and could be used for the treatment of seizures Figure 6 (Fayez et al., 2023). In another study, the authors used P. daemia against a PTZ-induced model of temporal lobe epilepsy. They also reported that the extract and reported that the phytochemicals in the extract lead to the suppression of the pro-inflammatory cytokines such as TNF 1β and −6 in the hippocampus and, therefore, lead to a decrease in the latency and duration of seizure and increase the score of seizure. In addition, they further reported that the extract alleviates kainite-induced impairment and finally concluded that the extract possesses strong antiepileptic properties and could be used for the treatment of epilepsy (Kandeda et al., 2021b). Emblica officinalis hydrochloric acid extracts also reported that same results in kainic acid induce model of epilepsy. Many studies have reported that plant extracts and phytochemicals could suppress the pro-inflammatory phytochemicals as well as increase the anti-inflammatory phytochemicals (Golechha et al., 2011). Therefore, these plants could be used for the treatment of epilepsy. We summarized some phytochemicals and plant sources that have anti-inflammatory properties and were investigated for the treatment of epilepsy, as shown in Table 4.
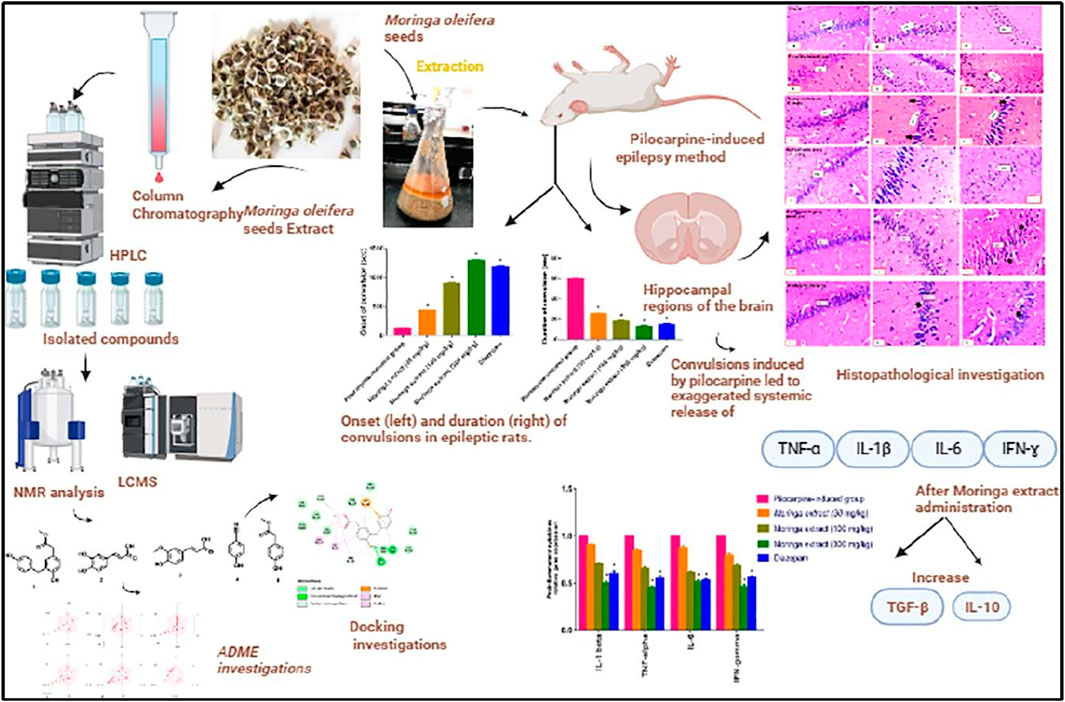
Figure 6. A comprehensive summary of moringa extracts in the rat model of temporal lobe epilepsy (Fayez et al., 2023). Copyright permission@ Elsevier 2023.
In addition, phytochemicals can also target and modulate various signalling pathways, which also play a role in the development and propagation of seizures. Several studies have been reported, including Inhibition of the NF-κB/TLR-4 Pathway (Tao et al., 2020), interaction with CREB-BDNF Pathway (Sharma et al., 2019; Zarneshan et al., 2022), AMPK/PPAR-α (Yang Y. et al., 2021) and AKT/CREB/BDNF (Yu et al., 2019) signalling pathways, and GluR2/ERK I/II pathway inhibition.
10 Conclusion and future prospects
Epilepsy is a complex and multifactorial neurological disease in which various receptors, membrane protein channels, enzymes, and pathways are involved in the epileptogenesis and worsening of a disease condition. Although a plethora of drugs are available in the market, but still about 30% of individuals show resistance to the current medication. In addition, these drugs also have some limitations, such as side effects, high price, and availability, especially in low-income countries. Therefore, there is a need for multitargeted approaches that are pocket-friendly, readily available and have no or fewer side effects.
Since immemorial, medicinal plants have been widely used for the treatment of various types of ailments, including neurological disorders, especially epilepsy. There are several advantages of these phytotherapeutic options compared to the synthetic drugs and pharmacological agents as mentioned. Plant extracts and isolated phytochemicals have been widely used in various animal models and have shown promising potential for the control of seizures and the treatment of epilepsy. These plant-based materials can target all the known targets for the currently available AEDs, as shown in Figure 2. In addition, it also targets other important pathways such as inflammatory, oxidative stress, and many others, as shown in section 9, as their inherited properties, such as anti-inflammatory and antioxidants, are mainly responsible for neuroprotective effects as well. Furthermore, the multitargeted potential of these plant-based materials has been observed to be superior to that of synthetic drugs. These can target various types of targets at the same time. For example, the whole plant extract of Alchemilla kiwuensis was used against the PTZ-induced epileptic rats model. The authors document that the extract has a significant antiepileptic effect, decreasing GABA-T enzymes, which leads to an increase in the GABA levels in the brain. In addition, it modulates the glutamatergic pathway and possesses anti-neurotic and antioxidant properties. This means that a single plant extract can target four pathways. In the drug resistant epilepsy, which is one of the main focus of the current antiepileptic research in which, two or more drugs with different mechanism of action or targets are used for the treatment. From the current literature, we can conclude that the plant extracts and isolated phytochemicals possess strong antiepileptic properties and could be used for the treatment of epilepsy, especially in lower income countries and patients with drug-resistant epilepsy. WHO also recommends the use of medicinal plants in various healthcare benefits programs in various countries. However, before the use, safety, dosage, exact mechanism of action, and therapeutic efficacy should be properly evaluated.
Until this time, hundreds of plants and their phytochemicals have been evaluated in vitro and in vivo against epilepsy and showed a potential effect. Unfortunately, only a few phytochemicals enter human clinical trials. Most phytochemicals are used in countries where people have lower purchasing power. Perhaps, in addition to this fact, the lack of investment in basic research, applied research, and folk medicine to understand certain plants also occurs. Similarly, other reasons could be ignorance of side effects, safety, specific dose, cytotoxicity, exact mechanism of action, complete knowledge and structure of the phytochemicals, and many more. Therefore, precise, rigorous, and extensive research could be carried out to investigate all these before entering into clinical trials. In addition, traditional methods for the identification of species are also adequate, and therefore, DNA barcoding could be used for the identification and screening of plant species. In conclusion, medicinal plants could be one of the main treatment options against epilepsy in the near future if we ensure the safety, efficacy, and effective dosage. This treatment strategy will be cost-effective, easily available worldwide, lower toxicity on health cells, and could eliminate the burden of epilepsy.
Author contributions
AW: Formal Analysis, Supervision, Writing–original draft, Writing–review and editing. AU: Conceptualization, Methodology, Writing–review and editing. RU: Formal Analysis, Writing–review and editing. MRR: Formal Analysis, Writing–review and editing. MA: Visualization, Writing–review and editing. STB: Conceptualization, Methodology, Writing–review and editing. FA: Funding acquisition, Project administration, Writing–review and editing.
Funding
The author(s) declare that financial support was received for the research, authorship, and/or publication of this article. This work is supported by the Deanship of Graduate Studies and Scientific Research (QU-APC-2024-9/1), Qassim University, Saudi Arabia..
Acknowledgments
The Researchers would like to thank the Deanship of Graduate Studies and Scientific Research at Qassim University for financial support (QU-APC-2024-9/1)..
Conflict of interest
The authors declare that the research was conducted in the absence of any commercial or financial relationships that could be construed as a potential conflict of interest.
Publisher’s note
All claims expressed in this article are solely those of the authors and do not necessarily represent those of their affiliated organizations, or those of the publisher, the editors and the reviewers. Any product that may be evaluated in this article, or claim that may be made by its manufacturer, is not guaranteed or endorsed by the publisher.
References
Abbasi, E., Nassiri-Asl, M., Shafeei, M., and Sheikhi, M. (2012). Neuroprotective effects of vitexin, a flavonoid, on pentylenetetrazole-induced seizure in rats. Chem. Biol. drug Des. 80 (2), 274–278. doi:10.1111/j.1747-0285.2012.01400.x
Abdallah, S. H., Mostafa, N. M., Mohamed, M. A. E. H., Nada, A. S., and Singab, A. N. B. (2022). UPLC-ESI-MS/MS profiling and hepatoprotective activities of Stevia leaves extract, butanol fraction and stevioside against radiation-induced toxicity in rats. Nat. Prod. Res. 36 (21), 5619–5625. doi:10.1080/14786419.2021.2015594
Abubakar, K., Adebisi, I., Ugwah-Oguejiofor, J., Idris, G., Idris, B., and Mshelia, H. (2016). Phytochemical screening and anticonvulsant activity of the residual aqueous fraction of Tapinanthus globiferus growing on Ficus glums. Herb. Med. 2 (2), 1–6. doi:10.21767/2472-0151.100013
Adassi, M. B., Ngoupaye, G. T., Yassi, F. B., Foutsop, A. F., Kom, T. D., and Bum, E. N. (2023). Revealing the most effective anticonvulsant part of Malvaviscus arboreus Dill. Ex Cav. and its acute and sub-acute toxicity. J. Ethnopharmacol. 303, 115995. doi:10.1016/j.jep.2022.115995
Adebayo, M., Akinpelu, L., Okwuofu, E., Ibia, D., Lawson-Jack, A., and Igbe, I. (2020). Anticonvulsant, antiamnesic and anxiolytic activities of methanol leaf extract of Bambusa vulgaris (Poaceae) in mice. J. Afr. Assoc. Physiological Sci. 8 (2), 149–157.
Adesina, S. K. (1979). Anticonvulsant properties of the roots of Boerhaavia diffusa Linnaeus. Q. J. crude drug Res. 17 (2), 84–86. doi:10.3109/13880207909067455
Adkar, P. P., Jadhav, P. P., Ambavade, S. D., Shelke, T. T., and Bhaskar, V. H. (2014). Protective effect of leaf extract of Pandanus odoratissimus Linn on experimental model of epilepsy. Int. J. Nutr. Pharmacol. Neurological Dis. 4 (2), 81–87. doi:10.4103/2231-0738.129590
Adongo, D. W., Mante, P. K., Kukuia, K. K., Biney, R. P., Boakye-Gyasi, E., Benneh, C. K., et al. (2017). Anticonvulsant activity of Pseudospondias microcarpa (A. Rich) Engl. hydroethanolic leaf extract in mice: the role of excitatory/inhibitory neurotransmission and nitric oxide pathway. J. Ethnopharmacol. 206, 78–91. doi:10.1016/j.jep.2017.05.017
Afshari, A. R., Fanoudi, S., Rajabian, A., Sadeghnia, H. R., Mollazadeh, H., and Hosseini, A. (2020). Potential protective roles of phytochemicals on glutamate-induced neurotoxicity: a review. Iran. J. basic Med. Sci. 23 (9), 1113–1123. doi:10.22038/ijbms.2020.43687.10259
Agbo, J., Ibrahim, Z. G., Magaji, S. Y., Mutalub, Y. B., Mshelia, P. P., and Mhyha, D. H. (2023). Therapeutic efficacy of voltage-gated sodium channel inhibitors in epilepsy. Acta Epileptol. 5 (1), 16. doi:10.1186/s42494-023-00127-2
Ajibade, M. A., Akhigbemen, A. M., Okolie, N. P., and Ozolua, R. I. (2022). Methanol leaf extract of Paullinia pinnata exerts sleep-enhancing and anticonvulsant effects via a mechanism involving the GABAergic pathway. Epilepsy Res. 183, 106943. doi:10.1016/j.eplepsyres.2022.106943
Akhigbemen, A. M., Ozolua, R. I., Bafor, E. E., and Okwuofu, E. O. (2019). Evaluation of some neuropharmacological effects of Caladium bicolor aiton (araceae) leaf extracts in mice. Metab. Brain Dis. 34, 537–544. doi:10.1007/s11011-019-0390-z
Akhtar, A., Andleeb, A., Waris, T. S., Bazzar, M., Moradi, A.-R., Awan, N. R., et al. (2021). Neurodegenerative diseases and effective drug delivery: a review of challenges and novel therapeutics. J. Control. Release 330, 1152–1167. doi:10.1016/j.jconrel.2020.11.021
Akyuz, E., Lazarowski, A., De Cabo, C., Godlevsky, L. S., Shaikh, M. F., and Doganyigit, Z. (2023). Editorial: ion-channels in epilepsy. Front. Neurology 14, 1263539. doi:10.3389/fneur.2023.1263539
Aliyu, M. M., Musa, A. I. i., Kamal, M. J. a., and Mohammed, M. G. (2014). Phytochemical screening and anticonvulsant studies of ethyl acetate fraction of Globimetula braunii on laboratory animals. Asian Pac. J. Trop. Biomed. 4 (4), 285–289. doi:10.12980/APJTB.4.2014C925
Alkhudhayri, A., Abdel Moneim, A. E., Rizk, S., Bauomy, A. A., and Dkhil, M. A. (2023). The neuroprotective effect associated with echinops spinosus in an acute seizure model induced by pentylenetetrazole. Neurochem. Res. 48 (1), 273–283. doi:10.1007/s11064-022-03738-2
Almamy, K., Franck, D., Richard, W. S., Lotfi, F., Norah, B., Valrie, M., et al. (2021). Therapeutic potential of Annona senegalensis Pers (Annonaceae), a plant used in traditional medicine for the treatment of epilepsy in Burkina Faso. Afr. J. Pharm. Pharmacol. 15 (1), 1–9. doi:10.5897/ajpp2020.5203
Almostafa, M. M., Mohamed, M. E., and Younis, N. S. (2024). Ameliorative effects of vanillin against pentylenetetrazole-induced epilepsy and associated memory loss in mice: the role of Nrf2/HO-1/NQO1 and HMGB1/RAGE/TLR4/NFκB pathways. Int. Immunopharmacol. 129, 111657. doi:10.1016/j.intimp.2024.111657
Alrashdi, J., Albasher, G., Alanazi, M. M., Al-Qahtani, W. S., Alanezi, A. A., and Alasmari, F. (2023). Effects of Rosmarinus officinalis L. extract on neurobehavioral and neurobiological changes in male rats with pentylenetetrazol-induced epilepsy. Toxics 11 (10), 826. doi:10.3390/toxics11100826
Alshabi, A. M., Shaikh, I. A., and Asdaq, S. M. B. (2022). The antiepileptic potential of Vateria indica Linn in experimental animal models: effect on brain GABA levels and molecular mechanisms. Saudi J. Biol. Sci. 29 (5), 3600–3609. doi:10.1016/j.sjbs.2022.02.059
Amabeoku, G. J., Mbamalu, O. N., Davids, T., Fakude, S., Gqwaka, A., Mbai, F., et al. (2014). Evaluation of the anticonvulsant activity of the leaf methanol extract of Crassula arborescens (Mill.) Willd.(Crassulaceae) in mice. J. Pharm. Pharmacol. 2, 393–403.
Amin, F., Tabassum, S., Sarwar, S., Qureshi, R., Sohaib Khalid, M., Riaz, N., et al. (2022). Neuroprotective effect of otostegia limbata against PTZ-induced mice model of epilepsy by attenuated expression of p-NFκB and TNF-α. Front. Neurosci. 16, 779681. doi:10.3389/fnins.2022.779681
Anderson, L. L., Ametovski, A., Lin Luo, J., Everett-Morgan, D., McGregor, I. S., Banister, S. D., et al. (2021a). Cannabichromene, related phytocannabinoids, and 5-fluoro-cannabichromene have anticonvulsant properties in a mouse model of Dravet Syndrome. ACS Chem. Neurosci. 12 (2), 330–339. doi:10.1021/acschemneuro.0c00677
Anderson, L. L., Heblinski, M., Absalom, N. L., Hawkins, N. A., Bowen, M. T., Benson, M. J., et al. (2021b). Cannabigerolic acid, a major biosynthetic precursor molecule in cannabis, exhibits divergent effects on seizures in mouse models of epilepsy. Br. J. Pharmacol. 178 (24), 4826–4841. doi:10.1111/bph.15661
Anderson, L. L., Udoh, M., Everett-Morgan, D., Heblinski, M., McGregor, I. S., Banister, S. D., et al. (2022). Olivetolic acid, a cannabinoid precursor in Cannabis sativa, but not CBGA methyl ester exhibits a modest anticonvulsant effect in a mouse model of Dravet syndrome. J. Cannabis Res. 4 (1), 2–9. doi:10.1186/s42238-021-00113-w
Archana, B., Mudavath, R. N., Enumula, V., Ravali, N., and Kumar, P. S. (2021). Evaluation of antiepileptic activity of flowers of cocos nucifera L. Against experimentally induced convulsions in rats. J. Drug Deliv. Ther. 11 (6), 159–166. doi:10.22270/jddt.v11i6.5097
Armijo, J., Shushtarian, M., Valdizan, E., Cuadrado, A., and Adin, J. (2005). Ion channels and epilepsy. Curr. Pharm. Des. 11 (15), 1975–2003. doi:10.2174/1381612054021006
Asadi-Pooya, A. A., Brigo, F., Lattanzi, S., and Blumcke, I. (2023). Adult epilepsy. Lancet 402 (10399), 412–424. doi:10.1016/S0140-6736(23)01048-6
Aseervatham, G. S. B., Suryakala, U., Sundaram, S., Bose, P. C., and Sivasudha, T. (2016). Expression pattern of NMDA receptors reveals antiepileptic potential of apigenin 8-C-glucoside and chlorogenic acid in pilocarpine induced epileptic mice. Biomed. Pharmacother. 82, 54–64. doi:10.1016/j.biopha.2016.04.066
Asiimwe, S., Anywar, G. U., Kakudidi, E. K., and Tugume, P. (2021). Medicinal plants in Uganda as potential therapeutics against neurological disorders. Med. Herbs Fungi Neurotox. vs. Neuroprotection, 421–443. doi:10.1007/978-981-33-4141-8_17
Ataee, R., Falahati, A., Ebrahimzadeh, M., and Shokrzadeh, M. (2016). Anticonvulsant activities of Sambucus nigra. Eur. Rev. Med. Pharmacol. Sci. 20 (14), 3123–3126.
Ayeni, E. A., Gong, Y., Yuan, H., Hu, Y., Bai, X., and Liao, X. (2022). Medicinal plants for anti-neurodegenerative diseases in West Africa. J. Ethnopharmacol. 285, 114468. doi:10.1016/j.jep.2021.114468
Balaha, M. F., Alamer, A. A., Abdel-Kader, M. S., and Alharthy, K. M. (2023). Ameliorative potential of (-) pseudosemiglabrin in mice with pilocarpine-induced epilepsy: antioxidant, anti-inflammatory, anti-apoptotic, and neurotransmission modulation. Int. J. Mol. Sci. 24 (13), 10773. doi:10.3390/ijms241310773
Behl, T., Rocchetti, G., Chadha, S., Zengin, G., Bungau, S., Kumar, A., et al. (2021). Phytochemicals from plant foods as potential source of antiviral agents: an overview. Pharmaceuticals 14 (4), 381. doi:10.3390/ph14040381
Bellampalli, S. S., Ji, Y., Moutal, A., Cai, S., Wijeratne, E. K., Gandini, M. A., et al. (2019). Betulinic acid, derived from the desert lavender Hyptis emoryi, attenuates paclitaxel-HIV-and nerve injury-associated peripheral sensory neuropathy via block of N-and T-type calcium channels. Pain 160 (1), 117–135. doi:10.1097/j.pain.0000000000001385
Benneh, C. K., Biney, R. P., Tandoh, A., Ampadu, F. A., Adongo, D. W., Jato, J., et al. (2018). Maerua angolensis DC.(capparaceae) stem bark extract protects against pentylenetetrazole-induced oxidative stress and seizures in rats. Evidence-Based Complementary Altern. Med. 2018, 9684138. doi:10.1155/2018/9684138
Berdigaliyev, N., and Aljofan, M. (2020). An overview of drug discovery and development. Future Med. Chem. 12 (10), 939–947. doi:10.4155/fmc-2019-0307
Bhat, A. H., and Sharma, H. (2020). Medicinal plants: a potent source of diuretics. Adv. Pharmacol. Uses Med. Plants Nat. Prod. 67. doi:10.4018/978-1-7998-2094-9.ch004
Bhattacharjee, P., and Majumder, P. (2013). Investigation of phytochemicals and anti-convulsant activity of the plant Coleus amboinicus (lour.). Int. J. Green Pharm. (IJGP) 7 (3), 211. doi:10.4103/0973-8258.120223
Birhan, Y. S. (2022). Medicinal plants utilized in the management of epilepsy in Ethiopia: ethnobotany, pharmacology and phytochemistry. Chin. Med. 17 (1), 129–137. doi:10.1186/s13020-022-00686-5
Bolanle, O. I., Oviasogie, O. D., Owolabi, O. J., Akhigbemen, A. M., Obarisiagbon, P. A., and Osaigbovo, C. A. (2018). Evaluation of the anti-convulsant activity of aqueous leaf extract of jatropha curcas (euphorbiaceae) in mice. Trop. J. Nat. Prod. Res. (TJNPR) 2 (11), 489–493. doi:10.26538/tjnpr/v2i11.5
Borowicz-Reutt, K., Czernia, J., and Krawczyk, M. (2023). Genetic background of epilepsy and antiepileptic treatments. Int. J. Mol. Sci. 24 (22), 16280. doi:10.3390/ijms242216280
Bose, S., Malik, J., and Mandal, S. C. (2020). “Application of phytochemicals in pharmaceuticals,” in Advances in pharmaceutical biotechnology: recent progress and future applications (Singapore: Springer Nature), 55–68. doi:10.1007/978-981-15-2195-9_5
Briggs, S. W., and Galanopoulou, A. S. (2011). Altered GABA signaling in early life epilepsies. Neural plast. 2011, 527605. doi:10.1155/2011/527605
Bryson, A., Reid, C., and Petrou, S. (2023). Fundamental Neurochemistry Review: GABAA receptor neurotransmission and epilepsy: principles, disease mechanisms and pharmacotherapy. J. Neurochem. 165 (1), 6–28. doi:10.1111/jnc.15769
Campos-Bedolla, P., Feria-Romero, I., and Orozco-Suárez, S. (2022). Factors not considered in the study of drug-resistant epilepsy: drug-resistant epilepsy: assessment of neuroinflammation. Epilepsia Open 7, S68–S80. doi:10.1002/epi4.12590
Camusso, W., Sacco, S., Maffei, M., and Bertea, C. M. (2007). Effect of benzoic acid hydroxyl-and methoxy-ring substituents on cucumber (Cucumis sativus L.) root membrane potential. J. Plant Interact. 2 (3), 185–193. doi:10.1080/17429140701596349
Carreño-González, A. J., Liberato, J. L., Celani, M. V., Lopes, N. P., Lopes, J. L. C., Neto, L. G., et al. (2023) Neuroprotective activity of chlorogenic acid from Brazilian Arnica in rodents subjected to status epilepticus induced by lithium-pilocarpine.
Carvill, G. L. (2019). Calcium channel dysfunction in epilepsy: gain of CACNA1E. Epilepsy Curr. 19 (3), 199–201. doi:10.1177/1535759719845324
Catterall, W. A. (2000). Structure and regulation of voltage-gated Ca2+ channels. Annu. Rev. Cell Dev. Biol. 16 (1), 521–555. doi:10.1146/annurev.cellbio.16.1.521
Chandak, R. R., and Dighe, N. S. (2019). A review on phytochemical and pharmacological profile of Pergularia daemia linn. J. Drug Deliv. Ther. 9 (4-s), 809–814. doi:10.22270/jddt.v9i4-s.3426
Chauhan, K., Sheth, N., Ranpariya, V., and Parmar, S. (2011). Anticonvulsant activity of solasodine isolated from Solanum sisymbriifolium fruits in rodents. Pharm. Biol. 49 (2), 194–199. doi:10.3109/13880209.2010.508499
Chen, T.-S., Huang, T.-H., Lai, M.-C., and Huang, C.-W. (2023). The role of glutamate receptors in epilepsy. Biomedicines 11 (3), 783. doi:10.3390/biomedicines11030783
Cheng, Y., Zhang, Y., Huang, P., Cheng, Q., and Ding, H. (2024). Luteolin ameliorates pentetrazole-induced seizures through the inhibition of the TLR4/NF-κB signaling pathway. Epilepsy Res. 201, 107321. doi:10.1016/j.eplepsyres.2024.107321
Chindo, B. A., Howes, M.-J. R., Abuhamdah, S., Yakubu, M. I., Ayuba, G. I., Battison, A., et al. (2021). New insights into the anticonvulsant effects of essential oil from melissa officinalis L.(Lemon balm). Front. Pharmacol. 12, 760674. doi:10.3389/fphar.2021.760674
Chipiti, T., Viljoen, A. M., Cordero-Maldonado, M. L., Veale, C. G., Van Heerden, F. R., Sandasi, M., et al. (2021). Anti-seizure activity of African medicinal plants: the identification of bioactive alkaloids from the stem bark of Rauvolfia caffra using an in vivo zebrafish model. J. Ethnopharmacol. 279, 114282. doi:10.1016/j.jep.2021.114282
Chiroma, S. S., Nazifi, A. B., Jamilu, Y. u., Musa, A., Bichi, L. A., and Chiroma, S. M. (2022a). Anticonvulsant activity and mechanism of actions of fractions of Ipomoea asarifolia (Desr)(Convolvulaceae) ethanol leaf extract. Bull. Natl. Res. Centre 46 (1), 150. doi:10.1186/s42269-022-00839-4
Chiroma, S. S., Nazifi, A. B., Ya’u, J., Aliyu, M., Bichi, L. A., and Chiroma, S. M. (2022b). Anti-seizure properties of Ipomoea asarifolia (Desr.)(Convolvulaceae) ethanolic leaf extract in laboratory animals. Bull. Natl. Res. Centre 46 (1), 205–207. doi:10.1186/s42269-022-00898-7
Choudhary, N., Bijjem, K. R. V., and Kalia, A. N. (2011). Antiepileptic potential of flavonoids fraction from the leaves of Anisomeles malabarica. J. Ethnopharmacol. 135 (2), 238–242. doi:10.1016/j.jep.2011.02.019
Chowdhury, E. A., Noorani, B., Alqahtani, F., Bhalerao, A., Raut, S., Sivandzade, F., et al. (2021). Understanding the brain uptake and permeability of small molecules through the BBB: a technical overview. J. Cereb. Blood Flow Metabolism 41 (8), 1797–1820. doi:10.1177/0271678X20985946
ClinicalTrials.gov (2024). Clinical trials for epilepsy. Available at: https://www.clinicaltrials.gov/search?cond=Epilepsy (Accessed April 26, 2024).
Correia, A., Monteiro, A., Silva, R., Moreira, J., Lobo, J. S., and Silva, A. (2022). Lipid nanoparticles strategies to modify pharmacokinetics of central nervous system targeting drugs: crossing or circumventing the blood-brain barrier (BBB) to manage neurological disorders. Adv. Drug Deliv. Rev. 189, 114485. doi:10.1016/j.addr.2022.114485
Crill, W. E. (1996). Persistent sodium current in mammalian central neurons. Annu. Rev. physiology 58 (1), 349–362. doi:10.1146/annurev.ph.58.030196.002025
Crupi, R., Impellizzeri, D., and Cuzzocrea, S. (2019). Role of metabotropic glutamate receptors in neurological disorders. Front. Mol. Neurosci. 12, 20. doi:10.3389/fnmol.2019.00020
Daanaa, S., Abotsi, W. K. M., Boakye-Gyasi, E., and Woode, E. (2018). Anticonvulsant effect of the hydroethanolic leaf extract of Psydrax subcordata (DC.) Bridson in murine models. J. Ethnopharmacol. 213, 384–394. doi:10.1016/j.jep.2017.11.028
Danis, A., Kilinc, Y. B., Torun, I. E., Hanci, F., Kilinc, E., and Ankarali, H. (2023). Esculetin alleviates pentylenetetrazole-induced seizures, cognitive impairment and pro-inflammatory cytokines and suppresses penicillin-induced epileptiform activity in rats. Life Sci. 313, 121300. doi:10.1016/j.lfs.2022.121300
Das, K., Khan, M. S., Muthukumar, A., Bhattacharyya, S., Usha, M., Venkatesh, S., et al. (2022). Phytochemical screening and potential anticonvulsant activity of aqueous root extract of Decalepis nervosa Wight and Arn. Thai J. Pharm. Sci. 46 (3), 307–315. doi:10.56808/3027-7922.2575
Da Silva, A. F. S., De Andrade, J. P., Bevilaqua, L. R., De Souza, M. M., Izquierdo, I., Henriques, A. T., et al. (2006). Anxiolytic-antidepressant-and anticonvulsant-like effects of the alkaloid montanine isolated from Hippeastrum vittatum. Pharmacol. Biochem. Behav. 85 (1), 148–154. doi:10.1016/j.pbb.2006.07.027
da Silva, A. W., Ferreira, M. K. A., Pereira, L. R., Rebouças, E. L., Coutinho, M. R., Dos, J., et al. (2022). Combretum lanceolatum extract reverses anxiety and seizure behavior in adult zebrafish through GABAergic neurotransmission: an in vivo and in silico study. J. Biomol. Struct. Dyn. 40 (20), 9801–9814. doi:10.1080/07391102.2021.1935322
DeLorenzo, R. J., Sun, D. A., and Deshpande, L. S. (2006). Erratum to “Cellular mechanisms underlying acquired epilepsy: the calcium hypothesis of the induction and maintenance of epilepsy” [Pharmacol. Ther. 105(3) (2005) 229–266]. Pharmacol. Ther. 111 (1), 288–325. doi:10.1016/j.pharmthera.2004.10.015
de Oliveira, C. C., de Oliveira, C. V., Grigoletto, J., Ribeiro, L. R., Funck, V. R., Grauncke, A. C. B., et al. (2016). Anticonvulsant activity of β-caryophyllene against pentylenetetrazol-induced seizures. Epilepsy and Behav. 56, 26–31. doi:10.1016/j.yebeh.2015.12.040
Djamshidian, A., Grassl, R., Seltenhammer, M., Czech, T., Baumgartner, C., Schmidbauer, M., et al. (2002). Altered expression of voltage-dependent calcium channel alpha(1) subunits in temporal lobe epilepsy with Ammon's horn sclerosis. Neuroscience 111 (1), 57–69. doi:10.1016/s0306-4522(01)00528-0
dos Santos Branco, C., Scola, G., Rodrigues, A. D., Cesio, V., Laprovitera, M., Heinzen, H., et al. (2013). Anticonvulsant, neuroprotective and behavioral effects of organic and conventional yerba mate (Ilex paraguariensis St. Hil.) on pentylenetetrazol-induced seizures in Wistar rats. Brain Res. Bull. 92, 60–68. doi:10.1016/j.brainresbull.2012.11.008
Drion, C., Van Scheppingen, J., Arena, A., Geijtenbeek, K., Kooijman, L., Van Vliet, E., et al. (2018). Effects of rapamycin and curcumin on inflammation and oxidative stress in vitro and in vivo—in search of potential anti-epileptogenic strategies for temporal lobe epilepsy. J. neuroinflammation 15, 212–311. doi:10.1186/s12974-018-1247-9
Duran, P., Loya-López, S., Ran, D., Tang, C., Calderon-Rivera, A., Gomez, K., et al. (2023). The natural product argentatin C attenuates postoperative pain via inhibition of voltage-gated sodium and T-type voltage-gated calcium channels. Br. J. Pharmacol. 180 (9), 1267–1285. doi:10.1111/bph.15974
El Nashar, E. M., Obydah, W., Alghamdi, M. A., Saad, S., Yehia, A., Maryoud, A., et al. (2022). Effects of Stevia rebaudiana Bertoni extracts in the rat model of epilepsy induced by pentylenetetrazol: sirt-1, at the crossroads between inflammation and apoptosis. J. Integr. Neurosci. 21 (1), 21. doi:10.31083/j.jin2101021
Falco-Walter, J. (2020). Epilepsy—definition, classification, pathophysiology, and epidemiology. Seminars neurology 40, 617–623. Thieme Medical Publishers. Inc. 333 Seventh Avenue, 18th Floor, New York, NY. doi:10.1055/s-0040-1718719
Fayez, S., Shady, N. H., Fawzy, I. M., Maher, S. A., Elrehany, M., Alqahtani, A. M., et al. (2023). Moringa extract reverses pilocarpine-induced hippocampal sclerosis in rats with temporal lobe epilepsy. J. Funct. Foods 111, 105905. doi:10.1016/j.jff.2023.105905
Firdous, A., Sarwar, S., Shah, F. A., Tabasum, S., Zeb, A., Nadeem, H., et al. (2021). Contribution of attenuation of TNF-α and NF-κB in the anti-epileptic, anti-apoptotic and neuroprotective potential of Rosa webbiana fruit and its chitosan encapsulation. Molecules 26 (8), 2347. doi:10.3390/molecules26082347
Fisseha, N., Hammeso, W. W., and Nureye, D. (2022). Anticonvulsant activity of hydro alcoholic extract and solvent fractions of biophytum umbraculum welw. Syn (oxalidaceae) root in mice. J. Exp. Pharmacol. 14, 291–299. doi:10.2147/JEP.S374890
Fisseha, N., Shibeshi, W., and Bisrat, D. (2021). Evaluation of anticonvulsant activity of 80% methanolic root bark extract and solvent fractions of pentas schimperiana (A. Rich.) Vatke (Rubiaceae) in Swiss Albino Mice. Adv. Pharmacol. Pharm. Sci. 2021, 6689879–6689887. doi:10.1155/2021/6689879
Fitzgerald, M., Heinrich, M., and Booker, A. (2020). Medicinal plant analysis: a historical and regional discussion of emergent complex techniques. Front. Pharmacol. 10, 1480. doi:10.3389/fphar.2019.01480
Fokoua, A. R., Ajayi, A. M., Ben-Azu, B., Chouna, R., Folarin, O., Olopade, J., et al. (2021a). The antioxidant and neuroprotective effects of the Psychotria camptopus Verd. Hook.(Rubiaceae) stem bark methanol extract contributes to its antiepileptogenic activity against pentylenetetrazol kindling in male Wistar rats. Metab. Brain Dis. 36 (7), 2015–2027. doi:10.1007/s11011-021-00825-1
Fokoua, A. R., Ndjenda, M. K., Wuyt, A. K., Bomba, F. D. T., Dongmo, A. K., Chouna, R., et al. (2021b). Anticonvulsant effects of the aqueous and methanol extracts from the stem bark of Psychotria camptopus Verdc.(Rubiacaea) in rats. J. Ethnopharmacol. 272, 113955. doi:10.1016/j.jep.2021.113955
Foutsop, A. F., Ateufack, G., Adassi, B. M., Yassi, F. B., Kom, T. D., Noungoua, C. M., et al. (2023). The aqueous lyophilisate of Alchemilla kiwuensis engl.(rosaceae) displays antiepileptogenic and antiepileptic effects on PTZ-induced kindling in rats: evidence of modulation of glutamatergic and GABAergic pathways coupled to antioxidant properties. Neurochem. Res. 48 (10), 3228–3248. doi:10.1007/s11064-023-03982-0
Fu, X., Wang, Y.-J., Kang, J.-Q., and Mu, T.-W. (2022) GABAA receptor variants in epilepsy. Epilepsy.
Gad, R. A., Abdel-Reheim, E. S., Ebaid, H., Alhazza, I. M., and Abuelsaad, A. S. (2022). Mitigating effects of Passiflora incarnata on oxidative stress and neuroinflammation in case of pilocarpine-Induced status epilepticus model. J. King Saud University-Science 34 (3), 101886. doi:10.1016/j.jksus.2022.101886
Gao, B., Wu, Y., Yang, Y.-J., Li, W.-Z., Dong, K., Zhou, J., et al. (2018). Sinomenine exerts anticonvulsant profile and neuroprotective activity in pentylenetetrazole kindled rats: involvement of inhibition of NLRP1 inflammasome. J. Neuroinflammation 15, 152–212. doi:10.1186/s12974-018-1199-0
Gao, K., Lin, Z., Wen, S., and Jiang, Y. (2022). Potassium channels and epilepsy. Acta Neurol. Scand. 146 (6), 699–707. doi:10.1111/ane.13695
Garlet, Q. I., da Costa Pires, L., Milanesi, L. H., Marafiga, J. R., Baldisserotto, B., Mello, C. F., et al. (2017). "(+)-Dehydrofukinone modulates membrane potential and delays seizure onset by GABAa receptor-mediated mechanism in mice. Toxicol. Appl. Pharmacol. 332, 52–63. doi:10.1016/j.taap.2017.07.010
Gawande, D. Y., Druzhilovsky, D., Gupta, R. C., Poroikov, V., and Goel, R. K. (2017). Anticonvulsant activity and acute neurotoxic profile of Achyranthes aspera Linn. J. Ethnopharmacol. 202, 97–102. doi:10.1016/j.jep.2017.03.018
George, B. P., Chandran, R., and Abrahamse, H. (2021). Role of phytochemicals in cancer chemoprevention: insights. Antioxidants 10 (9), 1455. doi:10.3390/antiox10091455
Ghit, A., Assal, D., Al-Shami, A. S., and Hussein, D. E. E. (2021). GABAA receptors: structure, function, pharmacology, and related disorders. J. Genet. Eng. Biotechnol. 19 (1), 123–215. doi:10.1186/s43141-021-00224-0
Golechha, M., Bhatia, J., Ojha, S., and Arya, D. S. (2011). Hydroalcoholic extract of Emblica officinalis protects against kainic acid-induced status epilepticus in rats: evidence for an antioxidant, anti-inflammatory, and neuroprotective intervention. Pharm. Biol. 49 (11), 1128–1136. doi:10.3109/13880209.2011.571264
Golub, V., and Reddy, D. S. (2021). Cannabidiol therapy for refractory epilepsy and seizure disorders. Cannabinoids Neuropsychiatric Disord. 1264, 93–110. doi:10.1007/978-3-030-57369-0_7
Gong, C.-L., Wong, K.-L., Cheng, K.-S., Kuo, C.-S., Chao, C.-C., Tsai, M.-F., et al. (2012). Inhibitory effects of magnolol on voltage-gated Na+ and K+ channels of NG108-15 cells. Eur. J. Pharmacol. 682 (1-3), 73–78. doi:10.1016/j.ejphar.2012.02.013
González-Trujano, M. E., Contreras-Murillo, G., López-Najera, C. A., Hidalgo-Flores, F. J., Navarrete-Castro, A., Sánchez, C. G., et al. (2021). Anticonvulsant activity of Valeriana edulis roots and valepotriates on the pentylenetetrazole-induced seizures in rats. J. Ethnopharmacol. 265, 113299. doi:10.1016/j.jep.2020.113299
Govindu, S., and Adikay, S. (2014). Evaluation of antiepileptic activity of chloroform extract of Acalypha fruticosa in mice. Pharmacogn. Res. 6 (2), 108–112. doi:10.4103/0974-8490.128970
Gracelyn Portia, A., Mwaniki, D., and Ng’wena, A. (2018) Phytochemical and antiepileptic activity of the ethanol leaf extracts of culcasia falcifolia in pentylenetetrazole induced seizure in mice.
Gupta, G., Kazmi, I., Afzal, M., Rahman, M., Saleem, S., Ashraf, M. S., et al. (2012). Sedative, antiepileptic and antipsychotic effects of Viscum album L.(Loranthaceae) in mice and rats. J. Ethnopharmacol. 141 (3), 810–816. doi:10.1016/j.jep.2012.03.013
Ha, J.-H., Lee, D.-U., Lee, J.-T., Kim, J.-S., Yong, C.-S., Kim, J.-A., et al. (2000). 4-Hydroxybenzaldehyde from Gastrodia elata B1. is active in the antioxidation and GABAergic neuromodulation of the rat brain. J. Ethnopharmacol. 73 (1-2), 329–333. doi:10.1016/s0378-8741(00)00313-5
Hanada, T. (2020). Ionotropic glutamate receptors in epilepsy: a review focusing on AMPA and NMDA receptors. Biomolecules 10 (3), 464. doi:10.3390/biom10030464
Haroon, E., Miller, A. H., and Sanacora, G. (2017). Inflammation, glutamate, and glia: a trio of trouble in mood disorders. Neuropsychopharmacology 42 (1), 193–215. doi:10.1038/npp.2016.199
Harris, L., and Angus-Leppan, H. (2020). Epilepsy: diagnosis, classification and management. Medicine 48 (8), 522–528. doi:10.1016/j.mpmed.2020.05.001
Hassanzadeh, P., Arbabi, E., Atyabi, F., and Dinarvand, R. (2017). Ferulic acid exhibits antiepileptogenic effect and prevents oxidative stress and cognitive impairment in the kindling model of epilepsy. Life Sci. 179, 9–14. doi:10.1016/j.lfs.2016.08.011
He, S.-R., Zhao, C.-B., Zhang, J.-X., Wang, J., Wu, B., and Wu, C.-J. (2020). Botanical and traditional uses and phytochemical, pharmacological, pharmacokinetic, and toxicological characteristics of Ziziphi Spinosae Semen: a review. Evidence-Based Complementary Altern. Med. 2020, 5861821. doi:10.1155/2020/5861821
He, X., Chen, X., Yang, Y., Xie, Y., and Liu, Y. (2023). Medicinal plants for epileptic seizures: phytoconstituents, pharmacology and mechanisms revisited. J. Ethnopharmacol. 320, 117386. doi:10.1016/j.jep.2023.117386
Hosseinzadeh, H., and Sadeghnia, H. (2007). Protective effect of safranal on pentylenetetrazol-induced seizures in the rat: involvement of GABAergic and opioids systems. Phytomedicine 14 (4), 256–262. doi:10.1016/j.phymed.2006.03.007
Jangra, S., and Budhwar, V. (2022) Ethno medicinal plants with anticonvulsant activity through GABAergic mechanism-A review.
Jebin, R., Molla, M. I., Chowdhury, S. M., and Rafe, M. R. (2019). Antidepressant and sedative-hypnotic activities of methanolic extract of Grewia asiatica Linn. leaves in mice. Bangladesh Pharm. J. 22 (2), 185–191. doi:10.3329/bpj.v22i2.42303
Jin, B., Bai, W., Zhao, J., Qin, X., Guo, H., Li, Y., et al. (2023). Jujuboside B inhibits febrile seizure by modulating AMPA receptor activity. J. Ethnopharmacol. 304, 116048. doi:10.1016/j.jep.2022.116048
Kandeda, A. K., Mabou, S. T., and Moutchida, C. (2022). An aqueous extract of Lantana camara attenuates seizures, memory impairment, and anxiety in kainate-treated mice: evidence of GABA level, oxidative stress, immune and neuronal loss modulation. Epilepsy and Behav. 129, 108611. doi:10.1016/j.yebeh.2022.108611
Kandeda, A. K., Moto, F. C. O., Ayissi, R. E. M., Omam, J. P. O., Ojong, L., and Bum, E. N. (2021a). Pergularia daemia hydro-ethanolic extract protects against pentylenetetrazole kindling-induced seizures, oxidative stress, and neuroinflammation in mice. J. Ethnopharmacol. 279, 114338. doi:10.1016/j.jep.2021.114338
Kandeda, A. K., Moto, F. C. O., Omam, J. P. O., Ayissi, R. E. M., Ojong, L., and Bum, E. N. (2021b). Pergularia daemia alters epileptogenesis and attenuates cognitive impairment in kainate-treated mice: insight into anti-inflammatory mechanisms. Epilepsy and Behav. 115, 107707. doi:10.1016/j.yebeh.2020.107707
Kandeda, A. K., Taiwe, G. S., Ayissi, R. E. M., and Moutchida, C. (2021c). An aqueous extract of Canarium schweinfurthii attenuates seizures and potentiates sleep in mice: evidence for involvement of GABA Pathway. Biomed. Pharmacother. 142, 111973. doi:10.1016/j.biopha.2021.111973
Kandeda, A. K., Taiwe, G. S., Moto, F. C., Ngoupaye, G. T., Nkantchoua, G. C., Njapdounke, J. S., et al. (2017). Antiepileptogenic and neuroprotective effects of Pergularia daemia on pilocarpine model of epilepsy. Front. Pharmacol. 8, 440. doi:10.3389/fphar.2017.00440
Kandhare, A. D., Mukherjee, A. A., and Bodhankar, S. L. (2018). Anti-epileptic effect of morin against experimental pentylenetetrazol-induced seizures via modulating brain monoamines and oxidative stress. Asian Pac. J. Trop. Biomed. 8 (7), 352–359. doi:10.4103/2221-1691.237078
Kaplan, D. I., Isom, L. L., and Petrou, S. (2016). Role of sodium channels in epilepsy. Cold Spring Harb. Perspect. Med. 6 (6), a022814. doi:10.1101/cshperspect.a022814
Kaur, J., Famta, P., Famta, M., Mehta, M., Satija, S., Sharma, N., et al. (2021). Potential anti-epileptic phytoconstituents: an updated review. J. Ethnopharmacol. 268, 113565. doi:10.1016/j.jep.2020.113565
Kaur, M., and Goel, R. K. (2009). Anti-convulsant activity of Boerhaavia diffusa: plausible role of calcium channel antagonism. Evid. Based Complementary Altern. 2011, 310420. doi:10.1093/ecam/nep192
Kaur, M., and Goel, R. K. (2011). Anti-convulsant activity of Boerhaavia diffusa: plausible role of calcium channel antagonism. Evidence-based Complementary Altern. Med. 2011, 310420. doi:10.1093/ecam/nep192
Kazmi, I., Afzal, M., Gupta, G., and Anwar, F. (2012). Antiepileptic potential of ursolic acid stearoyl glucoside by GABA receptor stimulation. CNS Neurosci. Ther. 18 (9), 799–800. doi:10.1111/j.1755-5949.2012.00369.x
Kediso, T. E., Tolessa, T., Getachew, F., Makonnen, E., and Seifu, D. (2021). Effect of 70% ethanol extract and its solvent fractions of Artemisia afra (Jacq. Ex Willd.) against pentylenetetrazole-induced seizure in mice. Evidence-Based Complementary Altern. Med. 2021, 6690965. doi:10.1155/2021/6690965
Kennedy, D. O., and Wightman, E. L. (2011). Herbal extracts and phytochemicals: plant secondary metabolites and the enhancement of human brain function. Adv. Nutr. 2 (1), 32–50. doi:10.3945/an.110.000117
Khamse, S., Sadr, S. S., Roghani, M., Hasanzadeh, G., and Mohammadian, M. (2015). Rosmarinic acid exerts a neuroprotective effect in the kainate rat model of temporal lobe epilepsy: underlying mechanisms. Pharm. Biol. 53 (12), 1818–1825. doi:10.3109/13880209.2015.1010738
Khan, A. U., Akram, M., Daniyal, M., Akhter, N., Riaz, M., Akhtar, N., et al. (2020). Awareness and current knowledge of epilepsy. Metab. Brain Dis. 35, 45–63. doi:10.1007/s11011-019-00494-1
Khan, I., Karim, N., Ahmad, W., Abdelhalim, A., and Chebib, M. (2016). GABA-A receptor modulation and anticonvulsant, anxiolytic, and antidepressant activities of constituents from Artemisia indica Linn. Evidence-Based Complementary Altern. Med. 2016, 1215393. doi:10.1155/2016/1215393
Kinsou, L. D., Adovelande, J., Assogba, M. F., Sezan, A., and Gbénou, J. D. (2019) Evaluation of anti-convulsant activity of afzelia africana leaves aqueous extract on wistar rat.
Köhling, R., and Wolfart, J. (2016). Potassium channels in epilepsy. Cold Spring Harb. Perspect. Med. 6 (5), a022871. doi:10.1101/cshperspect.a022871
Konate, A., Sawadogo, W., Dubruc, F., Caillard, O., Ouedraogo, M., and Guissou, I. (2012). Phytochemical and anticonvulsant properties of" Annona senegalensis" Pers.(Annonaceae), plant used in Burkina folk medicine to treat epilepsy and convulsions. Br. J. Pharmacol. Toxicol. 3 (5), 245–250.
Kukuia, K. K. E. (2012). Anticonvulsant, antidepressant and anxiolytic effects of Mallotus oppositifolius (geiseler) müll (Ghana: Arg.(Euphorbiaceae), University of Cape Coast).
Kullmann, D. M., Ruiz, A., Rusakov, D. M., Scott, R., Semyanov, A., and Walker, M. C. (2005). Presynaptic, extrasynaptic and axonal GABAA receptors in the CNS: where and why? Prog. biophysics Mol. Biol. 87 (1), 33–46. doi:10.1016/j.pbiomolbio.2004.06.003
Kumar, G., and Patnaik, R. (2016). Exploring neuroprotective potential of Withania somnifera phytochemicals by inhibition of GluN2B-containing NMDA receptors: an in silico study. Med. hypotheses 92, 35–43. doi:10.1016/j.mehy.2016.04.034
Kumar, K., Shankhdhar, P. K., and Chauhan, R. (2017). Evaluation of antiepileptic activity of ethanolic extract of populus deltoides leaf in mice. World J. Pharma Res. 6 (8), 923–940. doi:10.20959/wjpr20178-7853
Kwong, K., and Carr, M. J. (2015). Voltage-gated sodium channels. Curr. Opin. Pharmacol. 22, 131–139. doi:10.1016/j.coph.2015.04.007
Ladol, S., and Sharma, D. (2021). Pharmacotherapeutic effects of hippophaerhamnoides in rat model of post-traumatic epilepsy in view of oxidative stress, Na+, K+ ATPase activity and sodium ion channel expression. Acta Sci. Neurol. 4, 02–11. doi:10.31080/asne.2021.04.0359
Lai, M.-C., Wu, S.-N., and Huang, C.-W. (2022). Zingerone modulates neuronal voltage-gated Na+ and L-type Ca2+ currents. Int. J. Mol. Sci. 23 (6), 3123. doi:10.3390/ijms23063123
Lami, N., Kadota, S., Kikuchi, T., and MoMosE, Y. (1991). Constituents of the roots of Boerhaavia diffusa L. III. Identification of Ca2+ channel antagonistic compound from the methanol extract. Chem. Pharm. Bull. 39 (6), 1551–1555. doi:10.1248/cpb.39.1551
Lami, N., Kadota, S., Tezuka, Y., and Kikuchi, T. (1990). Constituents of the roots of Boerhaavia diffusa L. II.: structure and stereochemistry of a new rotenoid, Boeravinone C. Chem. Pharm. Bull. 38 (6), 1558–1562. doi:10.1248/cpb.38.1558
Li, J., Copmans, D. l., Partoens, M., Hunyadi, B. l., Luyten, W., and de Witte, P. (2020). Zebrafish-based screening of antiseizure plants used in traditional Chinese medicine: magnolia officinalis extract and its constituents magnolol and honokiol exhibit potent anticonvulsant activity in a therapy-resistant epilepsy model. ACS Chem. Neurosci. 11 (5), 730–742. doi:10.1021/acschemneuro.9b00610
Liang, N., and Kitts, D. D. (2015). Role of chlorogenic acids in controlling oxidative and inflammatory stress conditions. Nutrients 8 (1), 16. doi:10.3390/nu8010016
Lim, H. M., and Park, S.-H. (2023). Regulation of reactive oxygen species by phytochemicals for the management of cancer and diabetes. Crit. Rev. Food Sci. Nutr. 63 (22), 5911–5936. doi:10.1080/10408398.2022.2025574
Lin, T.-Y., Hung, C.-Y., Chiu, K.-M., Lee, M.-Y., Lu, C.-W., and Wang, S.-J. (2022). Neferine, an alkaloid from lotus seed embryos, exerts antiseizure and neuroprotective effects in a kainic acid-induced seizure model in rats. Int. J. Mol. Sci. 23 (8), 4130. doi:10.3390/ijms23084130
Lin, T. Y., Lu, C. W., and Wang, S. J. (2016). Luteolin protects the hippocampus against neuron impairments induced by kainic acid in rats. Neurotoxicology 55, 48–57. doi:10.1016/j.neuro.2016.05.008
Lin, T. Y., Lu, C. W., Wang, S. J., and Huang, S. K. (2015). Protective effect of hispidulin on kainic acid-induced seizures and neurotoxicity in rats. Eur. J. Pharmacol. 755, 6–15. doi:10.1016/j.ejphar.2015.02.041
Liu, H., Song, Z., Liao, D., Zhang, T., Liu, F., Zhuang, K., et al. (2015). Neuroprotective effects of trans-caryophyllene against kainic acid induced seizure activity and oxidative stress in mice. Neurochem. Res. 40, 118–123. doi:10.1007/s11064-014-1474-0
Liu, K.-m., Huang, Y., Wan, P.-p., Lu, Y.-h., Zhou, N., Li, J.-j., et al. (2022). Ursolic acid protects neurons in temporal lobe epilepsy and cognitive impairment by repressing inflammation and oxidation. Front. Pharmacol. 13, 877898. doi:10.3389/fphar.2022.877898
Liu, Y.-F., Gao, F., Li, X.-W., Jia, R.-H., Meng, X.-D., Zhao, R., et al. (2012). The anticonvulsant and neuroprotective effects of baicalin on pilocarpine-induced epileptic model in rats. Neurochem. Res. 37, 1670–1680. doi:10.1007/s11064-012-0771-8
Liu, Z., Lindemeyer, A. K., Liang, J., Wallner, M., Shao, X. M., Shao, Y., et al. (2018). Flavonoids isolated from Tibetan medicines, binding to GABAA receptor and the anticonvulsant activity. Phytomedicine 50, 1–7. doi:10.1016/j.phymed.2018.09.198
Löscher, W., Potschka, H., Sisodiya, S. M., and Vezzani, A. (2020). Drug resistance in epilepsy: clinical impact, potential mechanisms, and new innovative treatment options. Pharmacol. Rev. 72 (3), 606–638. doi:10.1124/pr.120.019539
Loshali, A., Joshi, B. C., Sundriyal, A., and Uniyal, S. (2021). Antiepileptic effects of antioxidant potent extract from Urtica dioica Linn. root on pentylenetetrazole and maximal electroshock induced seizure models. Heliyon 7 (2), e06195. doi:10.1016/j.heliyon.2021.e06195
Lu, X., Dai, P., Ma, H., and Lyu, D. (2023). Regulatory effect of exogenous γ-aminobutyric acid on respiratory rate through the γ-aminobutyric acid shunt in malus baccata (L.) borkh. Roots under suboptimal low root-zone temperature. Horticulturae 9 (2), 268. doi:10.3390/horticulturae9020268
Luthra, R., and Roy, A. (2022). Role of medicinal plants against neurodegenerative diseases. Curr. Pharm. Biotechnol. 23 (1), 123–139. doi:10.2174/1389201022666210211123539
Mahendran, G., Thamotharan, G., Sengottuvelu, S., and Bai, V. N. (2014). Evaluation of anticonvulsant, sedative, anxiolytic, and phytochemical profile of the methanol extract from the aerial parts of Swertia corymbosa (Griseb.) wight ex CB Clarke. BioMed Res. Int. 2014, 542385. doi:10.1155/2014/542385
Manouze, H., Bouchatta, O., Bennis, M., Sokar, Z., and Ba-M’hamed, S. (2019). Anticonvulsive and neuroprotective effects of aqueous and methanolic extracts of Anacyclus pyrethrum root in kainic acid-induced-status epilepticus in mice. Epilepsy Res. 158, 106225. doi:10.1016/j.eplepsyres.2019.106225
Mantegazza, M., Curia, G., Biagini, G., Ragsdale, D. S., and Avoli, M. (2010). Voltage-gated sodium channels as therapeutic targets in epilepsy and other neurological disorders. Lancet Neurology 9 (4), 413–424. doi:10.1016/S1474-4422(10)70059-4
Manville, R. W., and Abbott, G. W. (2018). Ancient and modern anticonvulsants act synergistically in a KCNQ potassium channel binding pocket. Nat. Commun. 9 (1), 3845. doi:10.1038/s41467-018-06339-2
Manville, R. W., Hogenkamp, D., and Abbott, G. W. (2023). Ancient medicinal plant rosemary contains a highly efficacious and isoform-selective KCNQ potassium channel opener. Commun. Biol. 6 (1), 644–717. doi:10.1038/s42003-023-05021-8
Mao, K., Lei, D., Zhang, H., and You, C. (2017). Anticonvulsant effect of piperine ameliorates memory impairment, inflammation and oxidative stress in a rat model of pilocarpine-induced epilepsy. Exp. Ther. Med. 13 (2), 695–700. doi:10.3892/etm.2016.4001
Medugu, A. N., Yakubu, J., Medugu, U. N., Marte, H. I., Tata, F. Y., and Balami, V. M. (2020). Phytochemical and anti-epileptic studies of ethanol extract of boswellia dalzielii (frankincense tree) stem bark. Eur. J. Med. Plants 31 (8), 94–100. doi:10.9734/ejmp/2020/v31i830262
Meir, A., Ginsburg, S., Butkevich, A., Kachalsky, S. G., Kaiserman, I., Ahdut, R., et al. (1999). Ion channels in presynaptic nerve terminals and control of transmitter release. Physiol. Rev. 79 (3), 1019–1088. doi:10.1152/physrev.1999.79.3.1019
Mele, M., Costa, R. O., and Duarte, C. B. (2019). Alterations in GABAA-receptor trafficking and synaptic dysfunction in brain disorders. Front. Cell. Neurosci. 13, 77. doi:10.3389/fncel.2019.00077
Milligan, C. J., Anderson, L. L., Bowen, M. T., Banister, S. D., McGregor, I. S., Arnold, J. C., et al. (2022). A nutraceutical product, extracted from Cannabis sativa, modulates voltage-gated sodium channel function. J. Cannabis Res. 4 (1), 30. doi:10.1186/s42238-022-00136-x
Milligan, C. J., Anderson, L. L., McGregor, I. S., Arnold, J. C., and Petrou, S. (2023). Beyond CBD: inhibitory effects of lesser studied phytocannabinoids on human voltage-gated sodium channels. Front. Physiology 14, 1081186. doi:10.3389/fphys.2023.1081186
Mishra, G., Singh, P., Garg, V., Parvez, N., Yadav, S., Hwisa, N., et al. (2011). Phytochemical screening and anticonvulsant activity of Wedelia chinensis. therapy 1, 4. doi:10.13040/IJPSR.0975-8232.2(1).25-29
Mishra, N., Oraon, A., Dev, A., Jayaprakash, V., Basu, A., Pattnaik, A. K., et al. (2010). Anticonvulsant activity of Benkara malabarica (Linn.) root extract: in vitro and in vivo investigation. J. Ethnopharmacol. 128 (2), 533–536. doi:10.1016/j.jep.2010.01.042
Mojarad, T. B., and Roghani, M. (2014). The anticonvulsant and antioxidant effects of berberine in kainate-induced temporal lobe epilepsy in rats. Basic Clin. Neurosci. 5 (2), 124–130.
Moshé, S. L., Perucca, E., Ryvlin, P., and Tomson, T. (2015). Epilepsy: new advances. Lancet 385 (9971), 884–898. doi:10.1016/S0140-6736(14)60456-6
Mula, M. (2021). Pharmacological treatment of focal epilepsy in adults: an evidence based approach. Expert Opin. Pharmacother. 22 (3), 317–323. doi:10.1080/14656566.2020.1829594
Muto, N. A., Hamoy, M., da Silva Ferreira, C. B., Hamoy, A. O., Lucas, D. C. R., de Mello, V. J., et al. (2022). Extract of Euterpe oleracea Martius stone presents anticonvulsive activity via the GABAA receptor. Front. Cell. Neurosci. 16, 872743. doi:10.3389/fncel.2022.872743
Muto, N. A., Hamoy, M., Lucas, D. C. R., Teixeira, B. B., Almeida, A. F. S., de Castro Navegantes, T., et al. (2021). Myorelaxation, respiratory depression and electrocardiographic changes caused by the administration of extract of açai (Euterpe oleracea Mart.) stone in rats. Toxicol. Rep. 8, 829–838. doi:10.1016/j.toxrep.2021.03.024
Nahar, L., Xiao, J., and Sarker, S. D. (2020). “Introduction of phytonutrients,” in Handbook of dietary phytochemicals, 1–17.
Nanumala, S. K., Haripriya, T., Singh, S. S., Shalini, S., Divya, N., and Priyanka, B. V. (2018). Anti convulsant effect of Cassia auriculata seed extract in mice. Eur. J. Biomed. 5 (5), 583–586.
Nasiri-Boroujeni, S., Rahimi-Madiseh, M., Lorigooini, Z., Piroti, K., Rafieian-Koupaei, M., and Amini-Khoei, H. (2021). NMDA receptor mediates the anticonvulsant effect of hydroalcoholic extract of Artemisia persica in PTZ-Induced Seizure in Mice. Evidence-Based Complementary Altern. Med. 2021, 6422451. doi:10.1155/2021/6422451
Nassiri-Asl, M., Farivar, T. N., Abbasi, E., Sadeghnia, H. R., Sheikhi, M., Lotfizadeh, M., et al. (2013). Effects of rutin on oxidative stress in mice with kainic acid-induced seizure. J. Integr. Med. 11 (5), 337–342. doi:10.3736/jintegrmed2013042
Nazarinia, D., Moslehi, A., and Hashemi, P. (2023). (-)-α-bisabolol exerts neuroprotective effects against pentylenetetrazole-induced seizures in rats by targeting inflammation and oxidative stress. Physiology Behav. 272, 114351. doi:10.1016/j.physbeh.2023.114351
Nguezeye, Y., Yadang, F. S. A., Pale, S., Jugha, V. T., Mambou, H. M. A. Y., Bila, R. B., et al. (2023). Anticonvulsant effects of <i&gt;Chrysanthellum americanum&lt;/i&gt; L. (Vatke) aqueous extract in mice pilocarpine model of epilepsy and associated memory impairment: role of antioxidant defense system and cholinergic transmission. J. Biosci. Med. 11 (6), 81–102. doi:10.4236/jbm.2023.116006
Nia, H. B., Naseri, A., Emamhadi, M., Ghadarjani, S., Aghaei, I., Dehpour, A. R., et al. (2022). Palmitoylethanolamide attenuates PTZ-induced seizures through CB1 and CB2 receptors. Epilepsy Res. 117, 23–28. doi:10.1016/j.eplepsyres.2015.08.010
Nikitin, E., and Vinogradova, L. (2021). Potassium channels as prominent targets and tools for the treatment of epilepsy. Expert Opin. Ther. Targets 25 (3), 223–235. doi:10.1080/14728222.2021.1908263
Nkwingwa, B. K., Wado, E. K., Foyet, H. S., Bouvourne, P., Jugha, V. T., Mambou, A. H. M. Y., et al. (2023). Ameliorative effects of Albizia adianthifolia aqueous extract against pentylenetetrazole-induced epilepsy and associated memory loss in mice: role of GABAergic, antioxidant defense and anti-inflammatory systems. Biomed. Pharmacother. 165, 115093. doi:10.1016/j.biopha.2023.115093
Nnamdi, N. S., Maduabuchi, O. R., Asogwa, F. K., Jude, A. I., and Celestine, U. O. (2022). Anticonvulsant activity of methanol extract of harungana madagascariensis leaf on mice model of isoniazid-induced siezure. J. Adv. Med. Pharm. Sci. 24 (8), 34–41. doi:10.9734/jamps/2022/v24i8572
Nonato, D. T. T., Aragão, G. F., Craveiro, R. M. C. B., Pereira, M. G., Vasconcelos, S. M. M., Wong, D. V. T., et al. (2024). Polysaccharide-rich extract of Genipa americana leaves protects seizures and oxidative stress in the mice model of pentylenetetrazole-induced epilepsy. Biomed. Pharmacother. 172, 116212. doi:10.1016/j.biopha.2024.116212
Nwachukwu, E. O., Akuodor, G. C., Oyindamola, J. O., Chilaka, K. C., Ilo, C. E., Obi, E., et al. (2022) Evaluation of the anticonvulsant properties of the ethanol extract of Detarium senegalense leaves in mice.
Obese, E., Biney, R. P., Henneh, I. T., Adakudugu, E. A., Anokwah, D., Agyemang, L. S., et al. (2021). The anticonvulsant effect of hydroethanolic leaf extract of calotropis procera (ait) R. Br.(Apocynaceae). Neural Plast. 2021, 5566890. doi:10.1155/2021/5566890
O’Donohoe, T., Choudhury, A., and Callander, E. (2020). Global macroeconomic burden of epilepsy and the role for neurosurgery: a modelling study based upon the 2016 Global Burden of Disease data. Eur. J. neurology 27 (2), 360–368. doi:10.1111/ene.14085
Offiah, R. O., Okolo, K. O., and Ifeoma, A. N. (2023). Preliminary phytochemical, GC-MS analysis and antiepileptic studies on the methanol leaf extract of Bixa orellana (bixaceae). Igbinedion Univ. Okada J. Pharm. Sci. 2 (1), 13–26.
Ojo, E. S., Ishola, I. O., Ben-Azu, B., Afolayan, O. O., James, A. B., Ajayi, A. M., et al. (2019). Ameliorative influence of Cnestis ferruginea vahl ex DC (Connaraceae) root extract on kainic acid-induced temporal lobe epilepsy in mice: role of oxidative stress and neuroinflammation. J. Ethnopharmacol. 243, 112117. doi:10.1016/j.jep.2019.112117
Okoye, T. C., Akah, P. A., Omeje, E. O., Okoye, F. B., and Nworu, C. S. (2013). Anticonvulsant effect of kaurenoic acid isolated from the root bark of Annona senegalensis. Pharmacol. Biochem. Behav. 109, 38–43. doi:10.1016/j.pbb.2013.05.001
Osuntokun, O. S., Oyedokun, S. O., Olayiwola, G., Adekomi, D. A., Oladokun, O. O., Adedokun, K. I., et al. (2022). Proanthocyanidin-rich-fraction of Vitis vinifera seed abrogates convulsion indices: glutamatergic/NMDA inhibition, enhancement of anti-neu N, and NRF2 expression. Trop. J. Nat. Prod. Res. 6 (6). doi:10.26538/tjnpr/v1i4.5
Oyemitan, I. A., Elusiyan, C. A., Akanmu, M. A., and Olugbade, T. A. (2013). Hypnotic, anticonvulsant and anxiolytic effects of 1-nitro-2-phenylethane isolated from the essential oil of Dennettia tripetala in mice. Phytomedicine 20 (14), 1315–1322. doi:10.1016/j.phymed.2013.07.005
Palhares, R. M., Gonçalves Drummond, M., dos Santos Alves Figueiredo Brasil, B., Pereira Cosenza, G., das Graças Lins Brandão, M., and Oliveira, G. (2015). Medicinal plants recommended by the world health organization: DNA barcode identification associated with chemical analyses guarantees their quality. PloS one 10 (5), e0127866. doi:10.1371/journal.pone.0127866
Park, H. G., Yoon, S. Y., Choi, J. Y., Lee, G. S., Choi, J. H., Shin, C. Y., et al. (2007). Anticonvulsant effect of wogonin isolated from Scutellaria baicalensis. Eur. J. Pharmacol. 574 (2-3), 112–119. doi:10.1016/j.ejphar.2007.07.011
Parmar, H., Gupta, S. K., and Shrivastava, S. K. (2022). Anti-convulsant activity of aqueous extract of aerial parts of sarcostemma acidum W. and A. NeuroQuantology 20 (20), 1730–1733. doi:10.48047/NQ.2022.20.20.NQ109178
Parvizi, F., Mehrabi, S., Naghizadeh, A., Kamalinejad, M., Goudarzi, S., and Farahmandfar, M. (2022). Comparison of intranasal and intraperitoneal administration of Eugenia caryophyllata (clove) essential oil on spatial memory, anxiety-like behavior and locomotor activity in a pilocarpine-induced status epilepticus rat model. BMC Complementary Med. Ther. 22 (1), 231. doi:10.1186/s12906-022-03711-0
Paudel, Y. N., Khan, S. U., Othman, I., and Shaikh, M. F. (2021). Naturally occurring HMGB1 inhibitor, glycyrrhizin, modulates chronic seizures-induced memory dysfunction in zebrafish model. ACS Chem. Neurosci. 12 (18), 3288–3302. doi:10.1021/acschemneuro.0c00825
Perucca, E. (2021). The pharmacological treatment of epilepsy: recent advances and future perspectives. Acta Epileptol. 3 (1), 22. doi:10.1186/s42494-021-00055-z
Perucca, E., Bialer, M., and White, H. S. (2023a). New GABA-targeting therapies for the treatment of seizures and epilepsy: I. Role of GABA as a modulator of seizure activity and recently approved medications acting on the GABA system. CNS drugs 37 (9), 755–779. doi:10.1007/s40263-023-01027-2
Perucca, E., White, H. S., and Bialer, M. (2023b). New GABA-targeting therapies for the treatment of seizures and epilepsy: II. Treatments in clinical development. CNS drugs 37 (9), 781–795. doi:10.1007/s40263-023-01025-4
Pironi, V., Ciccone, O., Beghi, E., Paragua-Zuellig, H., Patel, A. A., Giussani, G., et al. (2022). Survey on the worldwide availability and affordability of antiseizure medications: report of the ILAE task force on access to treatment. Epilepsia 63 (2), 335–351. doi:10.1111/epi.17155
Porras, G., Chassagne, F., Lyles, J. T., Marquez, L., Dettweiler, M., Salam, A. M., et al. (2020). Ethnobotany and the role of plant natural products in antibiotic drug discovery. Chem. Rev. 121 (6), 3495–3560. doi:10.1021/acs.chemrev.0c00922
Potnis, V., Albhar, K. G., Nanaware, P. A., and Pote, V. S. (2020). A review on epilepsy and its management. J. Drug Deliv. Ther. 10 (3), 273–279. doi:10.22270/jddt.v10i3.4090
Qian, X., Wang, Z.-R., Zheng, J.-J., Ding, J.-Q., Zhong, J.-G., Zhang, T.-Y., et al. (2019). Baicalein improves cognitive deficits and hippocampus impairments in temporal lobe epilepsy rats. Brain Res. 1714, 111–118. doi:10.1016/j.brainres.2019.02.028
Quintans-Júnior, L. J., Guimarães, A. G., Araújo, B. E., Oliveira, G. F., Santana, M. T., Moreira, F. V., et al. (2010a). Carvacrol,(-)-borneol and citral reduce convulsant activity in rodents. Afr. J. Biotechnol. 9 (39), 6566–6572.
Quintans-Júnior, L. J., Siqueira, J. S., Melo, M. S., Silva, D. A., Morais, L. C., Souza, M. d. F. V., et al. (2010b). Anticonvulsant evaluation of rauvolfia ligustrina willd. Ex roem. and schult., apocynaceae, in rodents. Rev. Bras. Farmacogn. 20, 54–59. doi:10.1590/s0102-695x2010000100012
Rahimi, V. B., Askari, V. R., Hosseini, M., Yousefsani, B. S., and Sadeghnia, H. R. (2019). Anticonvulsant activity of Viola tricolor against seizures induced by pentylenetetrazol and maximal electroshock in mice. Iran. J. Med. Sci. 44 (3), 220–226.
Rahimi-Madiseh, M., Lorigooini, Z., Boroujeni, S. N., Taji, M., and Amini-Khoei, H. (2022). The role of the NMDA receptor in the anticonvulsant effect of ellagic acid in pentylenetetrazole-induced seizures in male Mice. Behav. Neurol. 2022, 9015842. doi:10.1155/2022/9015842
Rajput, A., Sharma, P., Kumar, N., Singh, H., Singh, T., Singh, S., et al. (2022) Revealing Anticonvulsant Potential of Grewia tiliaefolia in Pentylenetetrazole induced Epilepsy in mice: in vitro, in vivo and, in silico analysis.
Rajput, A., Sharma, P., Kumar, N., Singh, H., Singh, T., Singh, S., et al. (2023). Anticonvulsant potential of Grewia tiliaefolia in pentylenetetrazole induced epilepsy: insights from in vivo and in silico studies. Metab. Brain Dis. 38 (7), 2355–2367. doi:10.1007/s11011-023-01252-0
Rajput, M. A., Khan, R. A., and Feroz, Z. (2013). Evaluation of antiepileptic activity of the methanol extract of Trachyspermum ammi (L.). Archives Biol. Sci. 65 (3), 815–819. doi:10.2298/abs1303815r
Randrianavony, P., Quansah, N., Djoudi, R., Quansah, N. A., and Randimbivololona, F. (2020). Anticonvulsant activity of hydroalcoholic extract of ageratum conyzoides L.(Asteraceae) in mice. Eur. Sci. J. ESJ 16. doi:10.19044/esj.2020.v16n18p114
Ranjan, R., Logette, E., Marani, M., Herzog, M., Tâche, V., Scantamburlo, E., et al. (2019). A kinetic map of the homomeric voltage-gated potassium channel (Kv) family. Front. Cell. Neurosci. 13, 358. doi:10.3389/fncel.2019.00358
Rashid, S., Wali, A. F., Rashid, S. M., Alsaffar, R. M., Ahmad, A., Jan, B. L., et al. (2021). Zingerone targets status epilepticus by blocking hippocampal neurodegeneration via regulation of redox imbalance, inflammation and apoptosis. Pharmaceuticals 14 (2), 146. doi:10.3390/ph14020146
Rho, J. M., and Boison, D. (2022). The metabolic basis of epilepsy. Nat. Rev. Neurol. 18 (6), 333–347. doi:10.1038/s41582-022-00651-8
Rocha, L. L., Lazarowski, A., and Cavalheiro, E. A. (2023) Pharmacoresistance in epilepsy: from genes and molecules to promising therapies. Springer Nature.
Rosa, D. S., Faggion, S. A., Gavin, A. S., de Souza, M. A., Fachim, H. A., dos Santos, W. F., et al. (2012). Erysothrine, an alkaloid extracted from flowers of Erythrina mulungu Mart. ex Benth: evaluating its anticonvulsant and anxiolytic potential. Epilepsy and Behav. 23 (3), 205–212. doi:10.1016/j.yebeh.2012.01.003
Sahu, A., Damiki, L., Nilanjan, G., and Dubey, S. (2008). Phytopharmacological review of Boerhaavia diffusa linn.(punarnava). Pharmacogn. Rev. 2 (4), 14.
Salgado, P. R. R., Fonsêca, D. V. d., Braga, R. M., Melo, C. G. F. d., Andrade, L. N., Almeida, R. N. d., et al. (2015). Comparative anticonvulsant study of epoxycarvone stereoisomers. Molecules 20 (11), 19660–19673. doi:10.3390/molecules201119649
Salvador, R., and Albers, R. W. (1959). The distribution of glutamic-γ-aminobutyric transaminase in the nervous system of the rhesus monkey. J. Biol. Chem. 234 (4), 922–925. doi:10.1016/s0021-9258(18)70203-2
Sancheti, J., Shaikh, M. F., Chaudhari, R., Somani, G., Patil, S., Jain, P., et al. (2014). Characterization of anticonvulsant and antiepileptogenic potential of thymol in various experimental models. Naunyn-Schmiedeberg's archives Pharmacol. 387, 59–66. doi:10.1007/s00210-013-0917-5
Sarfo, A., Abotsi, W. K. M., Ekuadzi, E., and Woode, E. (2022). Ceiba pentandra (L.) Gaertn hydroethanolic leaf extract exhibits anticonvulsant properties in mouse models. Phytomedicine Plus 2 (2), 100263. doi:10.1016/j.phyplu.2022.100263
Sasaki, K., Hatta, S., Wada, K., Ohshika, H., and Haga, M. (2000). Bilobalide prevents reduction of γ-aminobutyric acid levels and glutamic acid decarboxylase activity induced by 4-O-methylpyridoxine in mouse hippocampus. Life Sci. 67 (6), 709–715. doi:10.1016/s0024-3205(00)00657-3
Scharfman, H. E. (2007). The neurobiology of epilepsy. Curr. neurology Neurosci. Rep. 7 (4), 348–354. doi:10.1007/s11910-007-0053-z
Shaikh, M. F., Sancheti, J., and Sathaye, S. (2012). Phytochemical and pharmacological investigations of Eclipta alba (Linn.) Hassak leaves for antiepileptic activity. Int. J. Pharm. Pharm. Sci. 4 (4), 319–323.
Shan, Z., Cai, S., Yu, J., Zhang, Z., Vallecillo, T. G. M., Serafini, M. J., et al. (2019). Reversal of peripheral neuropathic pain by the small-molecule natural product Physalin F via block of CaV2. 3 (R-type) and CaV2. 2 (N-type) voltage-gated calcium channels. ACS Chem. Neurosci. 10 (6), 2939–2955. doi:10.1021/acschemneuro.9b00166
Shao, H., Yang, Y., Mi, Z., Zhu, G.-x., Qi, A.-p., Ji, W.-G., et al. (2016). Anticonvulsant effect of Rhynchophylline involved in the inhibition of persistent sodium current and NMDA receptor current in the pilocarpine rat model of temporal lobe epilepsy. Neuroscience 337, 355–369. doi:10.1016/j.neuroscience.2016.09.029
Sharma, P., Kumar, A., and Singh, D. (2019). Dietary flavonoids interaction with CREB-BDNF pathway: an unconventional approach for comprehensive management of epilepsy. Curr. Neuropharmacol. 17 (12), 1158–1175. doi:10.2174/1570159X17666190809165549
Sharma, V. C., and Kaushik, A. (2018). Evaluation of anticonvulsant effects of stem bark of Anogeissus latifolia (Roxb.) in mice. J. Appl. Pharm. Sci. 8 (11), 069–074. doi:10.7324/JAPS.2018.81110
Shastry, R. A., Madagundi, S. D., Habbu, P. V., Patil, B. S., Joshi, S. D., and Kulkarni, V. H. (2015). Phytochemical investigation and antiepileptic activity of Asparagus racemosus (Wild) root extracts in rodents. RGUHS J. Pharm. Sci. 5 (3), 97–103. doi:10.5530/rjps.2015.3.3
Shivakumar, S., Suresh, H., Hallikeri, C., Hatapakki, B., Handiganur, J., Sankh, K., et al. (2009). Anticonvulsant effect of Cyperus rotundus Linn rhizomes in rats. J. Nat. Remedies 192–196. doi:10.18311/jnr/2009/239
Shougrakpam, P., Bhattacharjee, A., Gunindro, N., and Rita, S. (2021) Comparative study of anticonvulsant effect of the leaves of sapindus emarginatus and acorus Calamus in experimentally induced animal models of epilepsy.
Silva, M. I. G., Silva, M. A. G., de Aquino Neto, M. R., Moura, B. A., de Sousa, H. L., de Lavor, E. P. H., et al. (2009). Effects of isopulegol on pentylenetetrazol-induced convulsions in mice: possible involvement of GABAergic system and antioxidant activity. Fitoterapia 80 (8), 506–513. doi:10.1016/j.fitote.2009.06.011
Singh, D., and Goel, R. K. (2016). Anticonvulsant mechanism of saponins fraction from adventitious roots of Ficus religiosa: possible modulation of GABAergic, calcium and sodium channel functions. Rev. Bras. Farmacogn. 26, 579–585. doi:10.1016/j.bjp.2015.10.007
Sivakumar, S., Ghasemi, M., and Schachter, S. C. (2022). Targeting NMDA receptor complex in management of epilepsy. Pharmaceuticals 15 (10), 1297. doi:10.3390/ph15101297
Smilin Bell Aseervatham, G., Abbirami, E., Sivasudha, T., and Ruckmani, K. (2020). Passiflora caerulea L. fruit extract and its metabolites ameliorate epileptic seizure, cognitive deficit and oxidative stress in pilocarpine-induced epileptic mice. Metab. Brain Dis. 35, 159–173. doi:10.1007/s11011-019-00501-5
Snehunsu, A., Mukunda, N., Satish Kumar, M., Sadhana, N., Naduvil Narayanan, S., Vijay Kapgal, K., et al. (2013). Evaluation of anti-epileptic property of Marsilea quadrifolia Linn. in maximal electroshock and pentylenetetrazole-induced rat models of epilepsy. Brain Inj. 27 (13-14), 1707–1714. doi:10.3109/02699052.2013.831121
Soman, S., Korah, P., Jayanarayanan, S., Mathew, J., and Paulose, C. (2012). Oxidative stress induced NMDA receptor alteration leads to spatial memory deficits in temporal lobe epilepsy: ameliorative effects of Withania somnifera and Withanolide A. Neurochem. Res. 37, 1915–1927. doi:10.1007/s11064-012-0810-5
Song, P., Zhang, Y., Ma, G., Zhang, Y., Zhou, A., and Xie, J. (2017). Gastrointestinal absorption and metabolic dynamics of jujuboside A, a saponin derived from the seed of Ziziphus jujuba. J. Agric. food Chem. 65 (38), 8331–8339. doi:10.1021/acs.jafc.7b02748
Specchio, N., Wirrell, E. C., Scheffer, I. E., Nabbout, R., Riney, K., Samia, P., et al. (2022). International League against epilepsy classification and definition of epilepsy syndromes with onset in childhood: position paper by the ILAE task force on nosology and definitions. Epilepsia 63 (6), 1398–1442. doi:10.1111/epi.17241
Sridevi, G., Prema, S., Sekar, S., and Sembulingam, K. (2014). Phytochemical analysis of Pergularia daemia for its bioactive components through gas chromatographic mass spectrometry (GCMS). IOSR J. Pharm. 4 (5), 41–46. doi:10.9790/3013-0405041046
Ssempijja, F., Dare, S. S., Bukenya, E. E., Kasozi, K. I., Kenganzi, R., Fernandez, E. M., et al. (2023). Attenuation of seizures, cognitive deficits, and brain histopathology by phytochemicals of imperata cylindrica (L.) P. Beauv (poaceae) in acute and chronic mutant Drosophila melanogaster epilepsy models. J. Evidence-Based Integr. Med. 28, 2515690X231160191. doi:10.1177/2515690X231160191
Subedi, L., and Gaire, B. P. (2021). Tanshinone IIA: a phytochemical as a promising drug candidate for neurodegenerative diseases. Pharmacol. Res. 169, 105661. doi:10.1016/j.phrs.2021.105661
Subedi, L., Lee, S. E., Madiha, S., Gaire, B. P., Jin, M., Yumnam, S., et al. (2020). Phytochemicals against TNF α-mediated neuroinflammatory diseases. Int. J. Mol. Sci. 21 (3), 764. doi:10.3390/ijms21030764
Sucher, N. J., and Carles, M. C. (2015). A pharmacological basis of herbal medicines for epilepsy. Epilepsy and Behav. 52, 308–318. doi:10.1016/j.yebeh.2015.05.012
Tao, Z., Chun-Yan, H., Hua, P., Bin-Bin, Y., and Xiaoping, T. (2020). Phyllathin from phyllanthus amarus ameliorates epileptic convulsion and kindling associated post-Ictal depression in mice via inhibition of NF-κB/TLR-4 pathway. Dose-Response 18 (3), 1559325820946914. doi:10.1177/1559325820946914
Taviano, M., Miceli, N., Monforte, M., Tzakou, O., and Galati, E. (2007). Ursolic acid plays a role in Nepeta sibthorpii Bentham CNS depressing effects. Phytotherapy Res. 21 (4), 382–385. doi:10.1002/ptr.2076
Thijs, R. D., Surges, R., O'Brien, T. J., and Sander, J. W. (2019). Epilepsy in adults. Lancet 393 (10172), 689–701. doi:10.1016/S0140-6736(18)32596-0
Tomson, T., Zelano, J., Dang, Y. L., and Perucca, P. (2023). The pharmacological treatment of epilepsy in adults. Epileptic Disord. 25 (5), 649–669. doi:10.1002/epd2.20093
Treiman, D. M. (2001). GABAergic mechanisms in epilepsy. Epilepsia 42, 8–12. doi:10.1046/j.1528-1157.2001.042suppl.3008.x
Ugwah-Oguejiofor, C. J., Amuda, M. B., Abubakar, K., Ugwah, O. M., Ofokansi, M. N., and Mshelia, H. E. (2023). An experimental evaluation of anticonvulsant activity of aqueous extract of Caralluma dalzielii NE Brown. Phytomedicine Plus 3 (1), 100401. doi:10.1016/j.phyplu.2022.100401
Ugwuoke, S. C., Nwanelo, V. O., Obinna, M., and Anosike, C. A. (2023). Antiepileptic effect of musa paradisiaca stem juice on pentylenetetrazole (PTZ)-induced seizures in albino rats. J. Res. Pharm. 27 (3), 1056–1065. doi:10.29228/jrp.398
Ukwubile, C. A., Ikpefan, E. O., Dibal, M. Y., Umeano, V. A., Menkiti, D. N., Kaosi, C. C., et al. (2023). Pharmacognostic profiles, evaluation of analgesic, anti-inflammatory and anticonvulsant activities of Newbouldia laevis (P. Beauv.) Seem. ex Bureau leaf and root extracts in Wistar rats. J. Ethnopharmacol. 314, 116632. doi:10.1016/j.jep.2023.116632
Uruaka, C., and Georgwill, O. (2020). Evaluation of the anticonvulsant, hypnotic and anxiolytic-like effects of methanol seed extract of Dennettia tripetala in mice. J. Afr. Assoc. physiological Sci. 8 (1), 41–49.
Usman, S., Ahmad, M. M., Tahir, A., and Umar, A. K. (2023). Exploring the antiepileptic potential of Amaranthus spinosus: an experimental study in albino mice. Sci. Pharm. 2 (3), 106–114. doi:10.58920/sciphar02030106
Vezzani, A., Fujinami, R. S., White, H. S., Preux, P.-M., Blümcke, I., Sander, J. W., et al. (2016). Infections, inflammation and epilepsy. Acta neuropathol. 131, 211–234. doi:10.1007/s00401-015-1481-5
Viswanatha, G. L., Venkataranganna, M. V., Prasad, N. B. L., and Ashok, G. (2016). Evaluation of anti-epileptic activity of leaf extracts of Punica granatum on experimental models of epilepsy in mice. J. Intercult. Ethnopharmacol. 5 (4), 415–421. doi:10.5455/jice.20160904102857
Wabo, P., Fossi, O. T., Kuiate, J.-R., Mpoame, B. M., and Dschang, P. (2009) In vitro antioxidant potential and phytochemical constituents of three cameroonian medicinal plants used to manage parasitic diseases.
Wang, K., Liu, Y., Shi, Y., Yan, M., Rengarajan, T., and Feng, X. (2021). Amomum tsaoko fruit extract exerts anticonvulsant effects through suppression of oxidative stress and neuroinflammation in a pentylenetetrazol kindling model of epilepsy in mice. Saudi J. Biol. Sci. 28 (8), 4247–4254. doi:10.1016/j.sjbs.2021.06.007
Wang, X.-x., Ma, G.-i., Xie, J.-b., and Pang, G.-c. (2015). Influence of JuA in evoking communication changes between the small intestines and brain tissues of rats and the GABAA and GABAB receptor transcription levels of hippocampal neurons. J. Ethnopharmacol. 159, 215–223. doi:10.1016/j.jep.2014.11.012
Wang, Y.-M., Zhao, J.-Q., Yang, C.-Y., Tao, Y.-D., Mei, L.-J., and Shi, Y.-P. (2016a). Anti-oxidant components from the aerial parts of Asterothamnus centrali-asiaticus. Phytochem. Lett. 17, 71–74. doi:10.1016/j.phytol.2016.07.021
Wang, Y.-M., Zhao, J.-Q., Yang, J.-L., Tao, Y.-D., Mei, L.-J., and Shi, Y.-P. (2016b). Isolation and identification of saponins from the natural pasturage Asterothamnus centrali-asiaticus employing preparative two-dimensional reversed-phase liquid chromatography/hydrophilic interaction chromatography. J. Agric. food Chem. 64 (24), 4950–4957. doi:10.1021/acs.jafc.6b02096
Waqar, H., Khan, H. M., and Anjum, A. A. (2016). Antiepileptic potential of Silybum marianum seeds in pentylenetetrazol-induced kindled mice. Bangladesh J. Pharmacol. 11 (3), 603–609. doi:10.3329/bjp.v11i3.26181
Welcome, M. O. (2020). Blood brain barrier inflammation and potential therapeutic role of phytochemicals. PharmaNutrition 11, 100177. doi:10.1016/j.phanu.2020.100177
Wirrell, E. C., Nabbout, R., Scheffer, I. E., Alsaadi, T., Bogacz, A., French, J. A., et al. (2022). Methodology for classification and definition of epilepsy syndromes with list of syndromes: report of the ILAE Task Force on Nosology and Definitions. Epilepsia 63 (6), 1333–1348. doi:10.1111/epi.17237
Wu, Y., Wang, Y., Wu, Y., Li, T., and Wang, W. (2020). Salidroside shows anticonvulsant and neuroprotective effects by activating the Nrf2-ARE pathway in a pentylenetetrazol-kindling epileptic model. Brain Res. Bull. 164, 14–20. doi:10.1016/j.brainresbull.2020.08.009
Xiao, H.-B., Wang, Y.-S., Luo, Z.-F., and Lu, X.-Y. (2018). SZSJ protects against insomnia by a decrease in ADMA level and an improvement in DDAH production in sleep-deprived rats. Life Sci. 209, 97–102. doi:10.1016/j.lfs.2018.07.044
Xiao, J., and Bai, W. (2019). Bioactive phytochemicals. Crit. Rev. Food Sci. Nutr. 59 (6), 827–829. doi:10.1080/10408398.2019.1601848
Xiong, B., Wang, Y., Chen, Y., Xing, S., Liao, Q., Chen, Y., et al. (2021). Strategies for structural modification of small molecules to improve blood–brain barrier penetration: a recent perspective. J. Med. Chem. 64 (18), 13152–13173. doi:10.1021/acs.jmedchem.1c00910
Xu, J.-H., and Tang, F.-R. (2018). Voltage-dependent calcium channels, calcium binding proteins, and their interaction in the pathological process of epilepsy. Int. J. Mol. Sci. 19 (9), 2735. doi:10.3390/ijms19092735
Yadav, D. K. (2021). Potential therapeutic strategies of phytochemicals in neurodegenerative disorders. Curr. Top. Med. Chem. 21 (31), 2814–2838. doi:10.2174/1568026621666211201150217
Yang, J., Jia, Z., Xiao, Z., Zhao, J., Lu, Y., Chu, L., et al. (2021a). Baicalin rescues cognitive dysfunction, mitigates neurodegeneration, and exerts anti-epileptic effects through activating TLR4/MYD88/caspase-3 pathway in rats. Drug Des. Dev. Ther. 15, 3163–3180. doi:10.2147/DDDT.S314076
Yang, Y., Li, Y., Han, J., and Wang, Y. (2021b). Gastrodin attenuates lithium-pilocarpine-induced epilepsy by activating AMPK-mediated PPARα in a juvenile rat model. Biosci. Biotechnol. Biochem. 85 (4), 798–804. doi:10.1093/bbb/zbab013
Ya’u, J., Yaro, A., Malami, S., Musa, M., Abubakar, A., Yahaya, S., et al. (2015). Anticonvulsant activity of aqueous fraction of Carissa edulis root bark. Pharm. Biol. 53 (9), 1329–1338. doi:10.3109/13880209.2014.981280
Yempala, T., Sridevi, J. P., Yogeeswari, P., Sriram, D., and Kantevari, S. (2014). Rational design and synthesis of novel dibenzo [b, d] furan-1, 2, 3-triazole conjugates as potent inhibitors of Mycobacterium tuberculosis. Eur. J. Med. Chem. 71, 160–167. doi:10.1016/j.ejmech.2013.10.082
Youn, Y., Sung, I. K., and Lee, I. G. (2013). The role of cytokines in seizures: interleukin (IL)-1β, IL-1Ra, IL-8, and IL-10. Korean J. Pediatr. 56 (7), 271–274. doi:10.3345/kjp.2013.56.7.271
Youssef, F. S., Menze, E. T., and Ashour, M. L. (2020). A potent lignan from Prunes alleviates inflammation and oxidative stress in lithium/pilocarpine-induced epileptic seizures in rats. Antioxidants 9 (7), 575. doi:10.3390/antiox9070575
Yu, X., Guan, Q., Wang, Y., Shen, H., Zhai, L., Lu, X., et al. (2019). Anticonvulsant and anti-apoptosis effects of salvianolic acid B on pentylenetetrazole-kindled rats via AKT/CREB/BDNF signaling. Epilepsy Res. 154, 90–96. doi:10.1016/j.eplepsyres.2019.05.007
Zadali, R., Baghery, M., Abbasi, M., Yavari, N., Miran, M., and Ebrahimi, S. N. (2022). Anticonvulsant activity of Iranian medicinal plants and molecular docking studies of isolated phytochemicals. South Afr. J. Bot. 149, 646–657. doi:10.1016/j.sajb.2022.06.044
Zarneshan, S. N., Fakhri, S., and Khan, H. (2022). Targeting Akt/CREB/BDNF signaling pathway by ginsenosides in neurodegenerative diseases: a mechanistic approach. Pharmacol. Res. 177, 106099. doi:10.1016/j.phrs.2022.106099
Zehra, T., Sarfaraz, S., and Ikram, R. (2021). Dose dependent anticonvulsant activity of Morus nigra in strychnine induced seizures model. Pak J. Pharm. Sci. 34, 2167–2171. doi:10.36721/PJPS.2021.34.6.REG.2167-2171.1
Zhang, J., Mohamad, F. H., Wong, J. H., Mohamad, H., Ismail, A. H., Yusoff, A. A. M., et al. (2018). The effects of 4-hydroxybenzoic acid identified from bamboo (Dendrocalamus asper) shoots on Kv1. 4 channel. Malays. J. Med. Sci. MJMS 25 (1), 101–113. doi:10.21315/mjms2018.25.1.12
Zhao, Y., Zhang, X., Li, J., Bian, Y., Sheng, M., Liu, B., et al. (2016). Jujuboside B reduces vascular tension by increasing Ca2+ influx and activating endothelial nitric oxide synthase. PloS one 11 (2), e0149386. doi:10.1371/journal.pone.0149386
Zou, X., Liu, S., Zou, H., Zhou, W., Fu, H., Wei, J., et al. (2023). Inflammatory mechanisms of Ginkgo Biloba extract in improving memory functions through lncRNA-COX2/NF-κB pathway in mice with status epilepticus. CNS Neurosci. Ther. 29 (1), 471–482. doi:10.1111/cns.14019
Glossary
Keywords: epilepsy, plant extracts, phytochemicals, antiepileptic, anticonvulsive, antagonist, agonist
Citation: Waris A, Ullah A, Asim M, Ullah R, Rajdoula MR, Bello ST and Alhumaydhi FA (2024) Phytotherapeutic options for the treatment of epilepsy: pharmacology, targets, and mechanism of action. Front. Pharmacol. 15:1403232. doi: 10.3389/fphar.2024.1403232
Received: 19 March 2024; Accepted: 09 May 2024;
Published: 24 May 2024.
Edited by:
Amira Zaky, Alexandria University, EgyptReviewed by:
Germain Sotoing Taiwe, University of Buea, CameroonGwladys Temkou Ngoupaye, University of Dschang, Cameroon
Wagner Ferreira Dos Santos, University of São Paulo, Brazil
Copyright © 2024 Waris, Ullah, Asim, Ullah, Rajdoula, Bello and Alhumaydhi. This is an open-access article distributed under the terms of the Creative Commons Attribution License (CC BY). The use, distribution or reproduction in other forums is permitted, provided the original author(s) and the copyright owner(s) are credited and that the original publication in this journal is cited, in accordance with accepted academic practice. No use, distribution or reproduction is permitted which does not comply with these terms.
*Correspondence: Fahad A. Alhumaydhi, Zi5hbGh1bWF5ZGhpQHF1LmVkdS5zYQ==; Abdul Waris, YXdhcmlzQGJzLnFhdS5lZHUucGs=