- 1College of Chinese Materia Medica, Tianjin University of Traditional Chinese Medicine, Tianjin, China
- 2College of Acupuncture-Moxibustion and Tuina, Tianjin University of Traditional Chinese Medicine, Tianjin, China
- 3Experimental Teaching and Practical Training Center, Heilongjiang University of Chinese Medicine, Harbin, China
- 4College of Integrated Chinese and Western Medicine, Tianjin University of Traditional Chinese Medicine, Tianjin, China
Breast cancer, the most prevalent malignant tumor among women globally, is significantly influenced by the Wnt/β-catenin signaling pathway, which plays a crucial role in its initiation and progression. While conventional chemotherapy, the standard clinical treatment, suffers from significant drawbacks like severe side effects, high toxicity, and limited prognostic efficacy, Traditional Chinese Medicine (TCM) provides a promising alternative. TCM employs a multi-targeted therapeutic approach, which results in fewer side effects and offers a high potential for effective treatment. This paper presents a detailed analysis of the therapeutic impacts of TCM on various subtypes of breast cancer, focusing on its interaction with the Wnt/β-catenin signaling pathway. Additionally, it explores the effectiveness of both monomeric and compound forms of TCM in the management of breast cancer. We also discuss the potential of establishing biomarkers for breast cancer treatment based on key proteins within the Wnt/β-catenin signaling pathway. Our aim is to offer new insights into the prevention and treatment of breast cancer and to contribute to the standardization of TCM.
Highlights
• Elucidated the complex role of TCM in treating breast cancer through the Wnt/β-catenin signaling pathway, emphasizing its dual capacity to modulate molecular mechanisms and enhance therapeutic potential when integrated with conventional therapies.
• Advanced a framework for TCM standardization focused on rigorous quality control, the use of biomarkers for clinical efficacy validation, and the synergy of combining TCM with established therapeutic modalities to reduce adverse effects.
1 Introduction
Breast cancer, stemming from the epithelial tissue of the mammary gland, has witnessed a steady surge in its incidence in recent years (Ni et al., 2022; Guo et al., 2023; Patel et al., 2023), solidifying its position as the foremost cancer afflicting women (Hashemi et al., 2023). As we delve deeper into the intricacies of this disease, it becomes evident that the Wnt signaling pathway stands at the crossroads of our understanding. Over the past decades, extensive research has illuminated the central role this pathway plays in breast cancer, influencing processes such as proliferation (Wend et al., 2013), metastasis (Luga et al., 2012; Harper et al., 2016), stem cell maintenance (Wang et al., 2016), drug resistance (Piva et al., 2014), and phenotyping (Piva et al., 2014; Jiang et al., 2019). It has been found that the Wnt/β-catenin axis is the core component of the Wnt signaling pathway, making the design of targeted therapy for it a growing research focus (Hernandez et al., 2012; Nishiya, 2017). However, despite the evident anti-cancer potential of inhibitors targeting this axis (Kahn, 2014), their translation from research labs to clinical application has encountered numerous obstacles. Challenges such as off-target effects (Ayadi et al., 2015), potential toxicity (Zhong et al., 2016; Wan et al., 2021), and the intricate nature of the Wnt/β-catenin signaling (Zimmerli et al., 2017) have been formidable barriers. This complexity is further underscored by the fact that no inhibitors specifically targeting the Wnt/β-catenin axis have been approved for breast cancer treatment (Zimmerli et al., 2017; Xu et al., 2020). As a result, conventional methods like surgical intervention, radiotherapy, and chemotherapy remain the primary recourse, despite their often suboptimal outcomes (Li et al., 2023).
Amidst this backdrop, TCM emerges as a beacon of hope. Its therapeutic potential is intricately linked with the modulation of the Wnt/β-catenin signaling pathway. Clinical studies have consistently emphasized the significant role TCM plays in breast cancer management, attributed to its low toxicity and comprehensive protective effects (Guo et al., 2020; Wang S. Y. et al., 2023). The diverse active metabolites within TCM not only interact with various cellular pathways but are especially adept at modulating the Wnt/β-catenin axis (Alharbi et al., 2022). This synergy between TCM, breast cancer, and the Wnt/β-catenin pathway has ignited a surge in research endeavors, examining TCM’s efficacy both as an independent therapeutic approach and in tandem with chemotherapy agents, as depicted in Figure 1A.
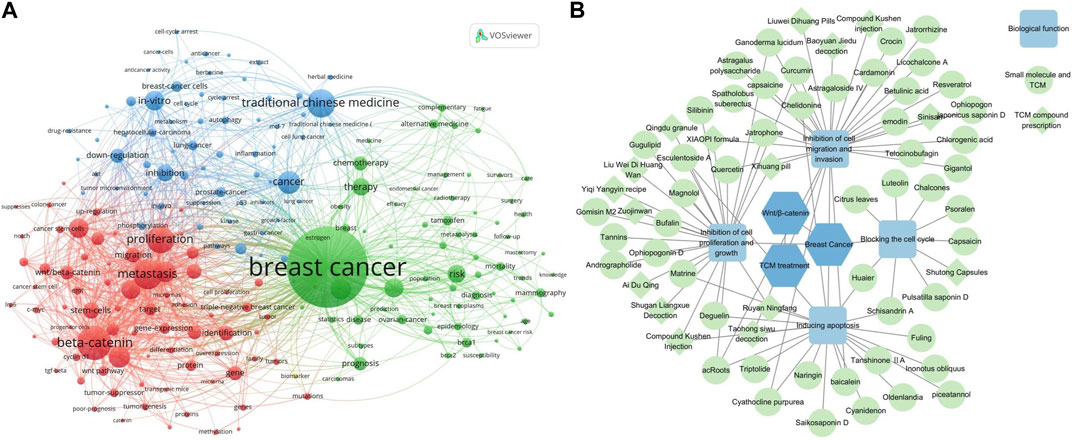
Figure 1. (A) Analysis using VOSviewer of key terms in breast cancer research, highlighting the prominence of TCM and the Wnt/β-catenin signaling pathway as current focal areas of investigation. (B) Network diagram crafted with Cytoscape software, illustrating the intricate interplay between various TCMs and their roles in breast cancer treatment. In this visualization, individual small molecule and TCM are denoted by circles, compound medicines by diamonds, and their corresponding biological functions by light blue squares. This representation not only elucidates the potential mechanisms of action of TCM, such as cell cycle blockade, inhibition of cell proliferation and growth, suppression of cell migration and invasion, and induction of apoptosis but also underscores the profound connection between TCM, breast cancer, and the pivotal Wnt/β-catenin signaling pathway.
This paper endeavors to provide a comprehensive overview of the unique attributes of the Wnt/β-catenin signaling pathway in breast cancer. Additionally, we delve into the potential of Chinese medicine monomers and compounds as therapeutic agents, specifically targeting the Wnt/β-catenin signaling pathway components (Figure 1B). The insights garnered from this study serve as a pivotal reference for future breast cancer research, paving the way for the evolution of novel therapeutic strategies tailored to different disease subtypes.
2 Traditional understanding and treatment of breast cancer in TCM
In clinical practice for early-stage breast cancer (stages I and II), treatments often involve modified radical mastectomy or breast-conserving surgery. However, these surgical procedures can cause considerable physical trauma and potentially impair limb function in patients. For more advanced stages (III and IV), chemotherapy is the typical course of action. This treatment regimen includes a combination of various drugs, such as anthracyclines, antimetabolites, alkylating agents, and platinum compounds (Marsh and Liu, 2009). However, most of these chemotherapy drugs are associated with severe side effects, including gastrointestinal disturbances, bone marrow suppression, ovarian and thyroid toxicity, and the development of drug resistance (Mortezaee et al., 2019; Zhou et al., 2022). In contrast, TCM adopts a holistic approach, focusing on syndrome differentiation and tailoring special prescriptions for specific diseases. Traditional chinese medicine aims to regulate the balance of yin and yang, harmonize qi (vital energy), and foster positive health trends. It has shown potential in treating breast cancer and its complications, mitigating adverse reactions to chemotherapy, enhancing postoperative recovery, and reducing the risk of recurrence and metastasis. Given its integration with modern science and technology and its synergistic use with Western medicine, TCM is increasingly recognized as a vital component in the comprehensive treatment of breast cancer.
Although there is no specific term for “breast cancer” in TCM, ancient Chinese medical literature often refers to it as “Ruyan” or “Mammary Mastitis.” It is traditionally linked to internal pathogenic factors such as qi stagnation, blood stasis, and phlegm turbidity. As articulated in the “Required reading for medical practitioners (医宗必读),” it is mentioned that “when the healthy qi is deficient, the pathogenic qi accumulates (正气不足而后邪气聚之)” and “where pathogenic factors converge, qi is invariably weak (邪之所凑, 其气必虚)” as cited in “Su Wen Comment on Fever (素问·评热病论篇).” This underscores the Chinese medicinal philosophy that while external factors might instigate the onset of breast cancer, it is the internal causes that play a pivotal role. Both these factors, in tandem, lead to the disease’s manifestation. Consequently, TCM’s approach to breast cancer treatment is holistic, emphasizing both local and systemic treatments, underpinned by dialectical reasoning. The primary therapeutic strategies encompass bolstering body resistance, purging pathogenic factors, detoxifying to eliminate carbuncles, and promoting blood circulation to remove stasis.
Several TCM compounds, such as ShuTong Capsule (Du et al., 2014), XiaoPi formula (Zheng et al., 2020), BaoYuanJieDu Tang (Tian et al., 2020), QingDu granule (Zhao et al., 2018), and Compound KuShen Injection (Nourmohammadi et al., 2019), which have their roots in ancient Chinese medical scriptures, have shown promising clinical results in breast cancer treatment. Concurrently, the main TCM in these prescriptions, including Ganoderma lucidum (Leyss.ex Fr.) Karst (Ganoderma lucidum) (Zhang, 2017), Astragalus mongholicus Bunge (Fabaceae; Astragali radix) (Jiang et al., 2017), Andrographis paniculata (Burm.f.) Wall. ex Nees (Acanthaceae; Andrographis herba) (Xu et al., 2022), Scleromitrion diffusum (Willd.) R. J. Wang (Rubiaceae; Hedyotis diffusa willd) (Li et al., 2017), Bupleurum chinense DC. (Apiaceae; Bupleuri radix) (Wang J. X. et al., 2018), Salvia miltiorrhiza Bunge (Lamiaceae; Salviae miltiorrhizae radix et rhizoma) (Zhao H. et al., 2022), Angelica sinensis (Oliv.) Diels (Apiaceae; Angelicae sinensis radix) (Zhu et al., 2021), Sophora flavescens Aiton (Fabaceae; Sophorae fiavescentis radix) (Li et al., 2020), and Glycyrrhiza uralensis Fisch. ex DC. (Fabaceae; Glycyrrhizae radix et rhizoma) (Bortolotto et al., 2017), have been the subject of rigorous research. These TCM have consistently demonstrated potent anti-breast cancer properties. Further studies have pinpointed that the therapeutic efficacy of these individual Chinese medicine components against breast cancer is largely attributed to their active metabolites, such as Astragalus polysaccharide (Yang S. et al., 2020), Astragaloside IV (Jiang et al., 2017), Saikosaponin D (Wang J. X. et al., 2018), Tanshinone ⅡA (Li and Lai, 2017), Matrine (Xiao et al., 2018), Capsaicin (Wu D. et al., 2020), Betulinic acid (Zheng Y. F. et al., 2019) and more.
Through numerous in vitro and in vivo experiments, it has been discovered that Chinese medicine compounds, monomers, and metabolites can inhibit the growth, migration, invasion, apoptosis, and recurrence of breast cancer by regulating Wnt/β-catenin, NF-κB, MAPK, and PI3K/AKT pathways. Through the study of network pharmacology, we found that these signaling pathways are closely related to the Wnt/β-catenin pathway. In breast cancer cells, NF-κB activation can inhibit β-catenin/TCF activity, thus negatively regulating the Wnt/β-catenin pathway (Ma and Hottiger, 2016). At the same time, the key activators of the NF-κB pathway, IKKα and IKKβ, can interact with β-catenin and phosphorylate it to positively regulate the Wnt/β-catenin pathway (Lamberti et al., 2001). Key members of the MAPK signaling pathway, such as ERK1/2, p38MAPK, and JNK, are involved in the phosphorylation of low-density lipoprotein receptor-related protein 6 (LRP6), which stimulates the expression of β-catenin, thereby influencing the Wnt/β-catenin signaling pathway (Zhang et al., 2014). There are many common connecting elements between the PI3K/AKT and Wnt/β-catenin pathways, such as GSK3β, FZD, DVL, Deptor, and eIF4E, which can interfere with the key process of regulating β-catenin degradation and β-catenin nuclear translocation (Gingras et al., 1999; Prossomariti et al., 2020). Based on these studies, we found that the mechanism of TCM in treating breast cancer is related to Wnt/β-catenin. Therefore, Wnt/β-catenin plays a crucial role in the interaction between TCM and breast cancer.
3 Wnt/β-catenin signaling and its interplay with TCM
The Wnt protein family, a collection of secreted lipid-modified glycoproteins (Oh et al., 2023), is instrumental in orchestrating a range of biological activities, from cell adhesion (Schambony et al., 2004; Astudillo and Larrain, 2014) and migration (Tuttle et al., 2014; Chai et al., 2019) to proliferation (Tang et al., 2019; Bertozzi et al., 2022), differentiation (Matsumoto et al., 2023), and survival (Jia et al., 2019). The Wnt signaling cascade bifurcates into two main branches: the canonical or classical Wnt pathway (Figure 2), commonly referred to as the Wnt/β-catenin pathway, and the non-canonical Wnt pathway, which further branches into the Wnt/planar cell polarity (PCP) pathway and the Wnt/Ca2+ pathway (Koni et al., 2020; Azbazdar et al., 2021; Patel et al., 2023) (Figures 2A, B). While these pathways have varying dependencies on β-catenin, together they form an intricate regulatory network.
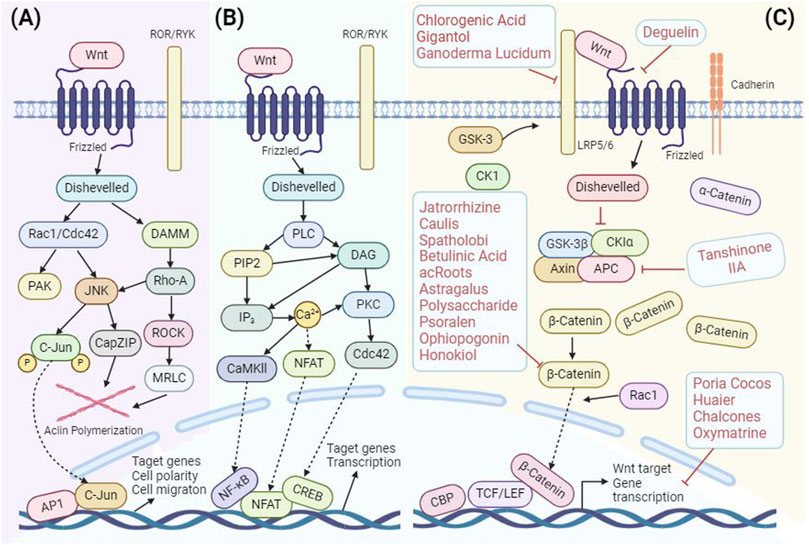
Figure 2. Diagrammatic representation of Wnt Signaling Pathways in Mammals: (A) Wnt–PCP pathway, (B) Wnt–Ca2+ pathway, and (C) Canonical Wnt pathway, with highlighted target positions modulated by TCM.
The Wnt/β-catenin signaling pathway, omnipresent in cells, is pivotal in modulating inflammatory reactions (Yang et al., 2022), lessening fibrosis (Mo et al., 2015), and counteracting osteoporosis (Zhang et al., 2017). Its significance is further underscored by its role in the etiology of various diseases. Traditional Chinese Medicine has shown potential in modulating this pathway. For example, HuangLianJieDu Decoction has been observed to attenuate the expression of LRP5/6, Wnt1, and β-catenin, thereby intervening at different junctures of the Wnt/β-catenin signaling pathway. This modulation has proven beneficial in treating conditions like psoriasis (Yang et al., 2022). Similarly, the HuangGan recipe acts by dampening the expression of Wnt1, β-catenin, and transcription factor 4, offering therapeutic advantages in conditions like glomerulosclerosis and tubulointerstitial fibrosis (Mo et al., 2015). Another notable mention is the BuShenJianPiHuoXue Decoction, which, in the context of diabetic osteoporosis, activates the Wnt/β-catenin signaling pathway and concurrently inhibits the NF-кB signaling pathway (Zhang et al., 2017). In essence, the intricate dance between TCM and the Wnt/β-catenin signaling pathway offers promising avenues for therapeutic interventions in a myriad of diseases, emphasizing the profound connection between TCM and the Wnt/β-catenin pathway.
Building on the intricate relationship between TCM and the Wnt/β-catenin signaling pathway, it is imperative to delve deeper into the pathway’s role in breast cancer. The Wnt/β-catenin pathway is intrinsically linked to the onset and progression of breast cancer.
Compared with traditional chemotherapy and radiotherapy, small molecular inhibitors are considered to be the most promising therapeutic strategies targeting the Wnt/β-catenin signaling pathway. These inhibitors are mainly divided into Wnt ligands inhibitors [e.g., WNT974 (Solzak et al., 2017), Wnt-C59 (Proffitt et al., 2013), and RXC004 (Shah et al., 2021)], Wnt receptor inhibitors [e.g., Mesd (Ma et al., 2017), Niclosamide (Lu et al., 2011), and Dickkopf1 (Bu et al., 2008)] β-catenin destruction complex inhibitors [e.g., E7449 (McGonigle et al., 2015; Asano et al., 2018; Plummer et al., 2020), XAV939 (Fang et al., 2021), and Pyrvinium (Rodgers et al., 2023)] and anti-angiogenic factors [e.g., Endostatin (Wu T. et al., 2020)]. In addition, as we all know, the combination of β-catenin and TCF4 has a low KD value and a large interaction surface. It is challenging to use a single low molecular weight inhibitor to inhibit this interaction. Hence, there is no small molecule capable of directly inhibiting Wnt signal transduction through the β-catenin target (Cui et al., 2018).
However, research has illuminated the potential of various Chinese medicines to target and modulate this pathway, offering therapeutic benefits at different stages of breast cancer. For instance, such as Ganoderma lucidum (Leyss.ex Fr.) Karst (Ganoderma lucidum) (Zhang, 2017) and metabolites of TCM [Deguelin (Murillo et al., 2009), Gigantol (Yu et al., 2018), and Chlorogenic acid (Xue et al., 2023)] have been identified to influence the upstream targets of the Wnt/β-catenin pathway, including Frizzled 7 and LRP6. By curbing the production of β-catenin, these medicines effectively stifle the Wnt/β-catenin signaling pathway. This modulation results in the suppression of breast cancer cell proliferation, invasion, and migration, while also amplifying their drug sensitivity.
In addition, the APC protein is also a key member upstream of the Wnt/β-catenin signaling pathway. APC acts as a negative regulator of β-catenin (Zhang et al., 2018) by serving as a carrier to connect glycogen synthase kinase-3β (GSK-3β) with β-catenin. This connection promotes the phosphorylation of β-catenin by GSK-3β, leading to the degradation of β-catenin and thereby maintaining β-catenin (MacDonald et al., 2009). When APC is mutated or deleted, it can still bind to β-catenin but is unable to degrade it. This results in the excessive accumulation of β-catenin in the nucleus, leading to abnormal cell proliferation, tumor formation, and drug resistance in tumor cells (Jeong et al., 2018). This mechanism explains why APC gene changes and abnormal activation of the Wnt/β-catenin signaling pathway are common in many types of cancer. It also underscores the potential of this pathway as a target for cancer treatment. It has been found that Tanshinone IIA can enhance the chemosensitivity of breast cancer cells to adriamycin by regulating the APC/β-catenin signaling pathway. It can be utilized as a potential chemosensitizer in combination with adriamycin for the treatment of breast cancer (Kanwar et al., 2010; Zhang et al., 2013; Tian et al., 2014).
Moreover, various TCM metabolites have been identified to directly target the β-catenin protein, effectively inhibiting the proliferation and metastasis of breast cancer cells and inducing apoptosis. Notable examples include Astragalus polysaccharide (Yang S. et al., 2020), Capsaicin (Wu D. et al., 2020), and Jatrophizine (Zhao and Wang, 2023), all of which are known to reduce β-catenin expression levels. Additionally, the root extract of Actinidia chinensis Planch. Gan et al. (2021) has been found to inhibit the phosphorylation of β-catenin. Psoralen (Wang et al., 2018b), the metabolite of Cullen corylifolium (L.) Medik. (Fabaceae; Psoraleae fructus), is effective in suppressing the transcriptional activity of β-catenin and Wnt target genes in breast cancer cells. These findings highlight the potential of TCM metabolites in offering targeted therapeutic actions against key pathways in breast cancer.
Lastly, TCM monomers such as Wolfiporia cocos (F.A. Wolf) Ryvarden and Gilb (Poria cocos) (Jiang and Fan, 2020) and Auriculariaauricula (L.cxHook.) Underw (Trametes robiniophila murr) (Zhang et al., 2010) as well as TCM metabolites such as Chalcone (Bortolotto et al., 2017) and Matrine (Xiao et al., 2018) have been found to target the downstream genes of the Wnt/β-catenin signaling pathway, like Cyclin D1, c-myc, and Bcl-2. Their action effectively disrupts the cell cycle progression in breast cancer cells, halting their proliferation and triggering apoptosis. This comprehensive understanding of the interplay between TCM and the Wnt/β-catenin pathway underscores the profound potential of TCM in breast cancer therapeutics (Figure 2C).
4 Heterogeneity and unique characteristics of Wnt/β-catenin activated breast cancer
Breast cancer stands out as the most prevalent malignant tumor among females. A closer look at breast cancer cells reveals a complex landscape: mutations differ from one patient to another, and within a single patient’s breast cancer tissue, a myriad of lesions can be identified. This complexity is further underscored by the varied expression levels of pivotal proteins within the same tissue, such as the Estrogen Receptor (ER), Progesterone Receptor (PR), and Human Epidermal Receptor 2 (HER-2) (Visvader, 2011; Turashvili and Brogi, 2017). Such variations underscore the pronounced heterogeneity inherent to breast cancer. This heterogeneity manifests in the form of multiple breast cancer subtypes. Clinically, two predominant subtypes emerge based on receptor status: the ER-positive, PR-positive, HER-2 amplification type, and the Triple-Negative Breast Cancer (TNBC) (Xu et al., 2022). Delving deeper into molecular markers, breast cancer can be categorized into Luminal A type, Luminal B type, HER-2 overexpression type, and Triple-Negative type, determined by the expression levels of ER, PR, HER-2, and Ki-67 (Gangrade et al., 2018; Fumagalli and Barberis, 2021; Liu H. Y. et al., 2022). It is crucial to note that the prognosis varies across different clinical stages, molecular types, and pathological types of breast cancer. Even among patients with identical clinical stages, outcomes can diverge significantly based on molecular and pathological distinctions (Fumagalli and Barberis, 2021). Given this complexity, there’s a pressing need for individualized treatment strategies tailored to specific breast cancer subtypes.
In clinical practice, the treatment approach for breast cancer varies based on the cancer’s receptor status. Patients with ER-positive and PR-positive breast cancer often opt for selective ER modulators (Maselli et al., 2019) and aromatase inhibitors (Ratre et al., 2020) as part of their treatment regimen. For those with HER2-positive breast cancer, the treatment typically includes monoclonal antibodies (Bighin et al., 2013), tyrosine kinase inhibitors (Deeks, 2017; Dhillon, 2019), and antibody-drug conjugates (Chung et al., 2020). In contrast, triple-negative breast cancer is frequently treated with chemotherapeutic agents like Doxorubicin, Cyclophosphamide, and Vinorelbine Tartrate. However, these widely used chemotherapy drugs in clinical settings are often associated with a range of side effects, including gastrointestinal disturbances (Zhou et al., 2013; Sartaj et al., 2021a; Farghadani and Naidu, 2022), osteoporosis (Essex et al., 2019; Shapiro, 2021; Meyer et al., 2024), bone marrow suppression (Zhou et al., 2013; Koliou et al., 2018; Han et al., 2021), dyslipidemia (Duman et al., 2012), and drug resistance (Sartaj et al., 2021b; Yu S. W. et al., 2023). On the other hand, TCM has demonstrated broad applicability in various types and clinical stages of breast cancer. It offers the advantages of multi-component and multi-target comprehensive treatment, with lower toxicity compared to chemical drugs. This highlights TCM’s potential as a complementary or alternative therapeutic approach in the multifaceted treatment of breast cancer (Qi et al., 2015; Zhang et al., 2020). In light of this, TCM offers a promising avenue. Traditional Chinese Medicine’s holistic approach, with its diverse array of botanical drugs, is uniquely positioned to address the multifaceted nature of breast cancer. By targeting specific pathways and mechanisms, such as the Wnt/β-catenin signaling, TCM provides a nuanced approach that aligns with the heterogeneity of breast cancer, holding promise for more effective and tailored therapeutic outcomes (Raut et al., 2022) (Figure 3).
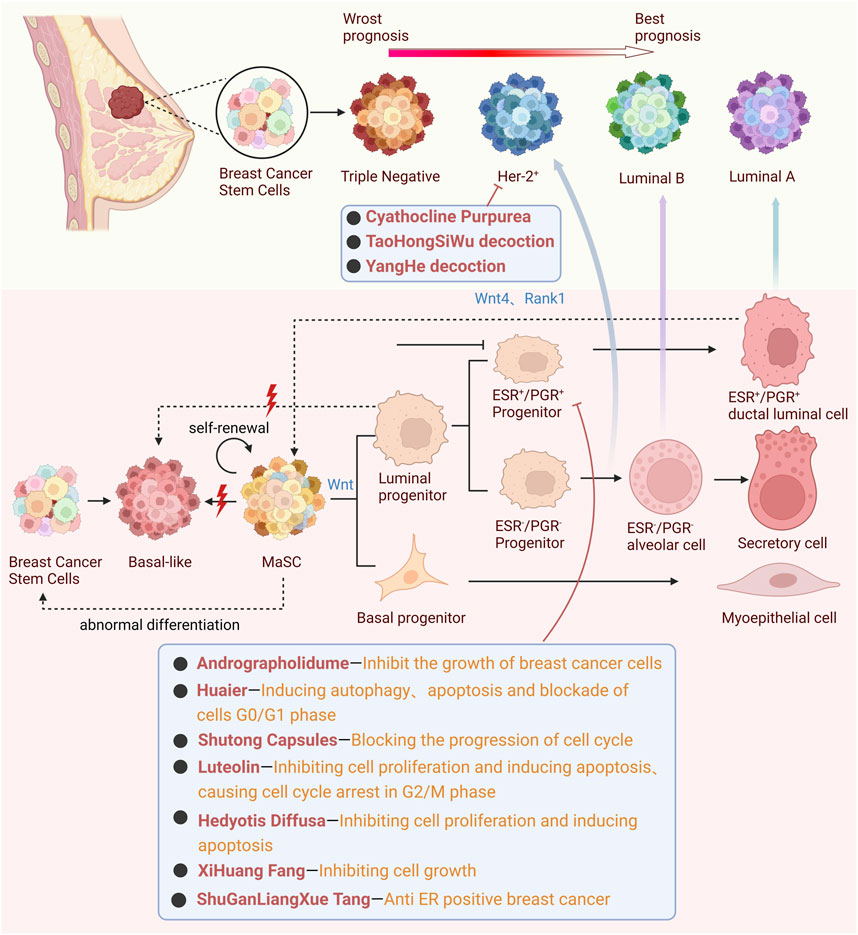
Figure 3. Mechanistic interplay of the Wnt signaling pathway in breast cancer heterogeneity: TCM’s targeting approach for HER-2+ and ER+ Subtypes.
4.1 ER-positive breast cancer
ER-positive breast cancers make up roughly 70% of all breast cancer cases (Zhu et al., 2021). The presence of ERα stands as a pivotal prognostic marker and plays a decisive role in determining clinical outcomes for ER-positive breast cancer patients (Li et al., 2014). This makes it a promising therapeutic target. Consequently, the discovery of selective ER modulators and selective estrogen downregulation factors is of paramount importance for treating this subtype of breast cancer (Park and Jordan, 2002). Commonly prescribed endocrine drugs in clinical settings include tamoxifen, anastrozole, and fulvestron (Bross et al., 2003). While these drugs have shown potential in improving progression-free survival rates (Robertson et al., 2016; Maass et al., 2019), they come with their set of challenges, including notable side effects and the potential to induce drug resistance (Qi et al., 2016). Furthermore, their effectiveness in enhancing overall survival rates and progression-free survival for advanced ER-positive patients remains somewhat constrained (Slamon et al., 2020; Albanell et al., 2022).
In this context, TCM offers a beacon of hope. With its minimal side effects, TCM presents a promising avenue for breast cancer management (Zhao M. H. et al., 2022). For instance, The ShuTong Capsule plays a role in ERα positive breast cancer cell lines MCF7 and T47D by down-regulating Cyclin D1 and ERα, the downstream target genes of the Wnt/β-catenin signaling pathway, thereby inhibiting breast cancer cell cycle progression (Du et al., 2014). Meanwhile, Scleromitrion diffusum (Willd.) R.J.Wang [Rubiaceae; Herba hedyoti diffusae] (Li et al., 2017), XiHuang Formula (Hao et al., 2018), and ShuGanLiangXue Decoction (Zhou et al., 2014) have been shown to have anti-tumor effects in ER-positive breast cancer. Furthermore, certain metabolites derived from TCM have been shown to inhibit the proliferation of ER-positive breast cancer cells and induce apoptosis simultaneously, such as andrographolide, the primary active metabolite of Andrographis paniculata (Burm.f.) Wall. ex Nees (Acanthaceae; Andrographis herba), in nude mice bearing breast cancer xenografts with MCF-7 cells (ip, 150 mg/kg/day, 16 days), can hinders Estrogen Receptor 1 transcription by targeting the ROS-FOXM1 axis and indirectly curtails the Wnt/β-catenin signaling pathway, thus stalling breast cancer cell proliferation (Xu et al., 2022). Research by Qi et al. (2016) highlighted that extracts derived from Auriculariaauricula (L.cxHook.)Underw (Trametes robiniophila murr), in female mice bearing breast cancer xenografts with MCF-7 cells (ig, 100 μL solution containing 50 mg, 40 days), inhibit the Wnt/β-catenin signaling pathway by targeting the AKT/mTOR pathway, leading to cell cycle blockade at the G0/G1 phase, and triggering autophagy-induced apoptosis. Luteolin, which is found in various vegetables, in ER-positive breast cancer cell lines MCF7, indirectly suppresses the Wnt/β-catenin signaling pathway by inhibiting the PI3K/AKT/mTOR signaling pathway, resulting in cell cycle arrest at the G2/M phase, reduced cell proliferation, and the initiation of apoptosis (Wu H. T. et al., 2020).
4.2 PR-positive breast cancer
PR, functioning as a regulatory gene for estrogen and ER (Li et al., 2022a), stands as a pivotal prognostic biomarker, influencing both the overall survival rate and disease-free survival rate in breast cancer patients (Mohammed et al., 2015). Intriguingly, Rank1 and Wnt4, direct targets of PR, play an indispensable role in paracrine actions, particularly in the induction of mitogenic signals by PR (Fu N. Y. et al., 2020). One of the remarkable capabilities of PR lumen cells is their ability to produce Wnt4. This, in turn, promotes the self-renewal of mammary stem cells (MaSCs) via the Wnt/β-catenin signaling pathway. This observation finds support in the research by Cai et al. (2014), where they identified the Wnt receptor agonist R-sport 1 as a potential novel conduit for transmitting ovarian hormone signals directly to MaSCs.
In a clinical setting, PR-positive breast cancer patients typically lean towards a combined treatment strategy, integrating endocrine therapy with adjuvant therapy, aiming to preemptively counteract cancer’s progression (Li Z. et al., 2022). However, the interplay between TCM and PR-positive breast cancer remains an area yet to be fully explored, highlighting the need for more in-depth research in this domain.
4.3 HER-2 positive breast cancer
HER-2 positive breast cancer is categorized into two specific subtypes: Luminal B and HER-2 overexpression (Zeng and Yang, 2017). Currently, the primary pharmacological interventions for HER-2 positive breast cancer include anthracyclines, paclitaxel (Schneeweiss et al., 2015), aromatase inhibitors (Ellis et al., 2001), and other related medications. However, a significant concern arises from the pronounced adverse reactions and potential drug resistance associated with most chemotherapy drugs. These side effects can range from gastrointestinal issues and immune system disruptions to cardiotoxicity and other complications (Gonzalez-Angulo et al., 2007; Zeng and Yang, 2017). Given these challenges, the standalone therapeutic efficacy of chemotherapy drugs for HER-2 positive breast cancer remains less than optimal (Javir et al., 2020).
In response to these challenges, the medical community has broadened its approach to breast cancer management. Complementary and Alternative Medicine (CAM) has gained traction as a viable strategy for both prevention and treatment (Zeng and Yang, 2017). Within the CAM spectrum, Chinese medicine stands out, offering a rich history and a plethora of therapeutic options for breast cancer management. For instance, cyathocline purpurea (Buch.-Ham. ex D. Don) Kuntze (Asteraceae; Cyathoclines purpureae herba), in HER-2 positive MDA-MB-453 cell line, showcases its therapeutic potential by reducing the size of MDA-MB-231 cells, inhibiting their proliferation and movement, and initiating their apoptosis. This is achieved through its influence on the epithelial-mesenchymal transition (EMT), tumor necrosis factor-alpha (TNF-α), and the Wnt/β-catenin signaling pathways (Javir et al., 2020). Another notable traditional remedy, TaoHongSiWu decoction, has demonstrated its capability to inhibit the Wnt/β-catenin signaling pathway by targeting the PI3K/AKT pathway, effectively curtailing the proliferation and metastasis of breast cancer cells (Jiang et al., 2021). Furthermore, research spearheaded by Zeng and his colleagues has highlighted the therapeutic potential of YangHe decoction specifically for HER-2 positive breast cancer (Zeng and Yang, 2017).
4.4 Triple-negative breast cancer
Triple-negative breast cancer, characterized by the absence of ER, PR, and HER-2 expressions (Buyuk et al., 2022), constitutes 15%–20% of all breast cancer cases (Yao et al., 2017). Although the incidence rate is low, it has a very high mortality rate (Solzak et al., 2017). The lack of hormone receptors and the non-overexpression of HER-2 protein render molecular targeting and endocrine therapies particularly challenging for TNBC (Yang C. Q. et al., 2020; Sun et al., 2020; Li Y. P. et al., 2022; Lu et al., 2023). Compounding the challenge, TNBC’s aggressive nature results in a higher risk of distant metastasis, leading to a more dire prognosis compared to other breast cancer subtypes (Saranya et al., 2020; Yang et al., 2021). In the clinical realm, the primary therapeutic interventions for advanced TNBC remain anthracycline chemotherapy drugs (Coates et al., 2015; Yu et al., 2020; Zhao et al., 2021). While these can extend patient survival, their pronounced toxic effects often prove intolerable for many patients (Goto et al., 2018). Given this backdrop, the quest for efficacious treatments for TNBC with minimal side effects has intensified.
Recent years have witnessed a surge in research exploring the potential anti-tumor properties of TCM, particularly its ability to modulate the body’s signaling pathways. These studies hint at the promise of TCM as a novel therapeutic avenue for TNBC (Yang et al., 2021). Whether as a sustained supplementary treatment or a potential alternative, TCM is emerging as a beacon of hope in the battle against TNBC. For instance, tannins, the primary active metabolite of Syzygium guineense (Willd.) DC. [Myrtaceae; Syzygium guineense] reduces the stability and transcriptional activity of β-catenin by inhibiting Wnt3a, thereby preventing the proliferation of BT-20 cells (Koval et al., 2018). Jatrophone, the primary active metabolite of Jatropha gossypiifolia L. [Euphorbiaceae; Jatropha gossypiifolia], can decrease the steady-state and non-phosphorylated (activated) β-catenin levels. It directly inhibits the migration of triple-negative breast cancer cells by targeting the cancer-causing Wnt10b/β-catenin signaling pathway. This botanical drug is anticipated to emerge as a potent new chemotherapeutic agent for treating triple-negative breast cancer, which is known for its high resistance to chemotherapy (Fatima et al., 2017). (Table 1).
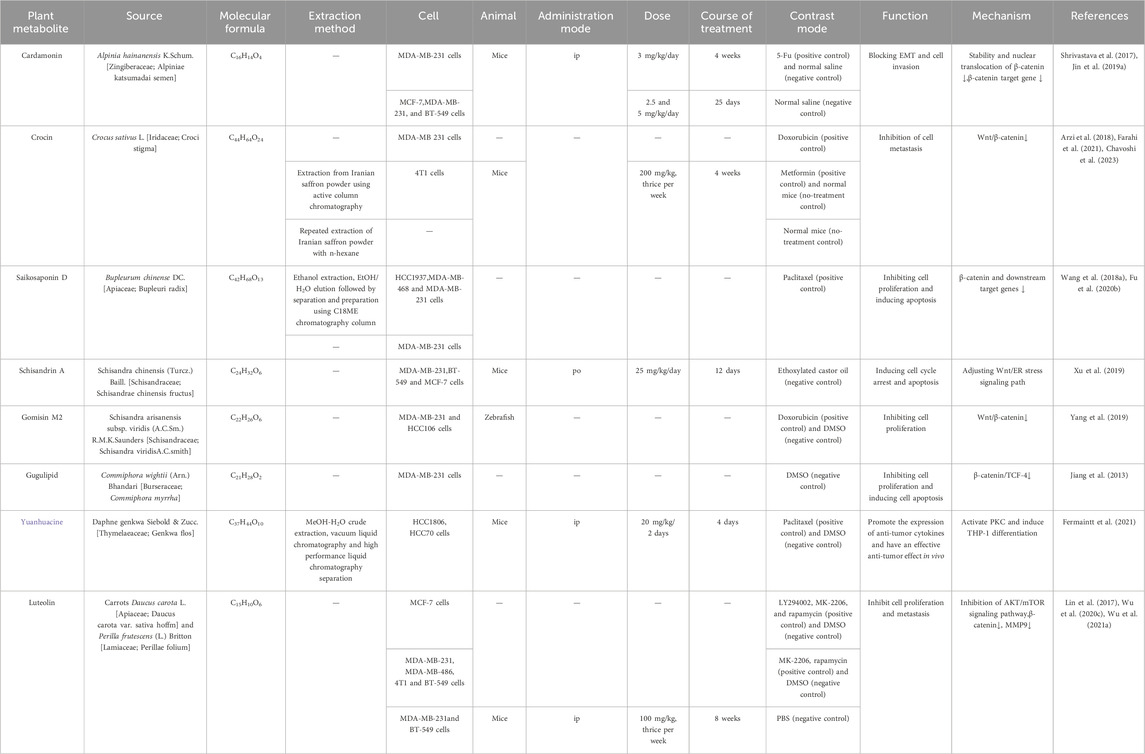
Table 1. Active phytoconstituents of TCM approaches targeting the Wnt/β-catenin pathway in TNBC treatment.
4.5 TCM interventions targeting breast cancer stem cells
Currently, the cancer stem cell model is primarily used to explain the heterogeneity of breast cancer as described above. This theory posits that cell diversity and tumor grade are generated by breast cancer stem cells (BCSCs) that can form transplantable tumors and rebuild tumor heterogeneity (Rafii and Lyden, 2003; Vermeulen et al., 2008). These cells play a crucial role in influencing early metastasis (Bozorgi et al., 2015; O’Conor et al., 2018), drug resistance (Moreira et al., 2018; Park et al., 2019), and the overall prognosis of the disease. At the same time, some molecular markers, such as CD44+/CD24-/low (Al-Hajj et al., 2003; Sheridan et al., 2006), ALDH1+ (Ginestier et al., 2007; Morimoto et al., 2009), CD133 (Kim et al., 2015), CD61 (Vaillant et al., 2008), CD49f (Ye et al., 2017), and CXCR4 (Ablett et al., 2014; Trautmann et al., 2014), can be used as biomarkers to identify BCSCs, and may serve as therapeutic targets for small molecular inhibitors. Therefore, many researchers realize that targeting BCSCs is crucial to achieve long-lasting remission of breast cancer (Gwynne et al., 2021). In experimental research, the common and unique characteristics of normal stem cells and cancer stem cells have been utilized to develop robust stem cell models. The commonly used model for BCSCs is 3D multicellular stem-like spheroids. Compared with 2D monolayer culture, 3D culture exhibits remarkable tumorigenicity, better simulating in vivo behavior, facilitating mechanism research, and aiding in the development of targeted drugs (Antoni et al., 2015).
In addition, regulatory signaling pathways targeting BCSCs have been developed for the treatment of breast cancer, especially in cases of therapeutic resistance (Zhou et al., 2019). The Wnt/β-catenin signaling pathway, as the primary driving force behind the onset and progression of breast cancer (Qayoom et al., 2021; Mao X. D. et al., 2022), plays a crucial role in sustaining the activity of BCSCs, facilitating tumor metastasis, and triggering the expression of drug-resistant genes (Lin et al., 2018; Ke et al., 2022). Based on these insights, inhibiting the activity of BCSCs by regulating the Wnt/β-catenin signaling pathway has gradually become the main focus of research (Feng et al., 2023). In recent years, an increasing number of studies have found that many metabolites of traditional Chinese medicine and compounds from traditional Chinese medicine align with this treatment concept, as detailed in (Table 2).
For example, naringenin, an active metabolite found in tomatoes, grapefruits, and oranges, can inhibit the proliferation and migration, and induce apoptosis of BCSCs by regulating the transforming growth factor-β (TGF-β) and Wnt/β-catenin pathways in MCF-7 cells. It is expected to become a targeted drug for BCSCs (Hermawan et al., 2021). Quercetin, a metabolite found in various fruits, vegetables, nuts, and seeds, has been shown to inhibit the proliferation, self-renewal, and invasiveness of BCSCs in a mouse model carrying MCF-7 cells. This inhibition is achieved by suppressing the PI3K/Akt/mTOR signaling pathway, which is related to the Wnt/β-catenin signaling pathway, and by reducing the expression levels of proteins associated with cancer progression (Li X. L. et al., 2018; Wang R. et al., 2018). At the same time, it has been found that quercetin can reverse the multidrug resistance of breast cancer cells by down-regulating the expression of P-gp and eliminating cancer stem cells through YB-1 nuclear translocation (Li S. Z. et al., 2018; Wang S. Y. et al., 2023).
5 TCM compounds and their role in modulating the Wnt/β-catenin pathway in breast cancer treatment
Traditional Chinese Medicine, deeply rooted in China’s rich cultural heritage, stands out for its unique diagnostic and therapeutic approaches, coupled with its proven clinical efficacy. Beyond its intrinsic value, TCM offers a complementary approach to conventional cancer treatments. It can amplify the anti-tumor effects of standard therapies while simultaneously mitigating their side effects. When combined with chemotherapy and radiotherapy, TCM can alleviate tumor-induced symptoms, such as cancer pain, and has been observed to prolong the survival of patients, especially those in advanced stages post-surgery (Li et al., 2012).
A growing body of research underscores the potential of TCM monomers in breast cancer treatment. These compounds have demonstrated their ability to disrupt the cell cycle, inhibit cell proliferation and growth, curtail cell migration and invasion, and trigger apoptosis. Intriguingly, many of these effects are mediated through the modulation of the Wnt/β-catenin signaling pathway, positioning TCM as a promising avenue in the therapeutic landscape of breast cancer.
5.1 Cell cycle progression modulation
Traditional Chinese Medicine monomer and its metaboliteshave shown significant potential in modulating the cell cycle progression of breast cancer cells, primarily through their interaction with the Wnt/β-catenin signaling pathway. Auriculariaauricula (L.cxHook.)Underw (Trametes robiniophila murr) (Zhang et al., 2010; Ding et al., 2016; Yang L. et al., 2017; Gao et al., 2017) and Nobiletin (Wu Y. et al., 2021), the latter being a major metabolite of Citrus folium, in MCF-7 and MDA-MB-231 cells, exhibit the capability to induce G0/G1 cell cycle arrest in breast cancer cells. This is attributed to their inhibitory effect on Cyclin D1 expression, a key downstream target of the Wnt/β-catenin pathway. Similarly, Chalcones, derived from Glycyrrhiza uralensis Fisch. ex DC. (Fabaceae; Glycyrrhizae radix et rhizoma), can halt the MCF-7 breast cancer cell cycle at the G1 phase by downregulating the expression of Bcl-2 and Cyclin D1, both of which are integral to the Wnt/β-catenin pathway (Bortolotto et al., 2017).
Psoralen, the metabolite of Cullen corylifolium (L.) Medik. (Fabaceae; Psoraleae fructus), on the other hand, impacts the Wnt/β-catenin signaling pathway by affecting the cytoplasmic accumulation and nuclear translocation of β-catenin. This results in diminished overall β-catenin levels. Moreover, Psoralen can arrest the G0/G1 phase in MCF-7 breast cancer cells and the G2/M phase in MDA-MB-231 cells (Wang et al., 2018d). Capsaicin, the primary spicy metabolite in Capsicum annuum L. (Solanaceae; Capsici fructus), has also shown promise. A study by Wu et al. (2020) revealed its potential to induce G2/M cell cycle arrest in MDA-MB-231 cells. This is achieved through a multifaceted mechanism involving reduced CDK8 expression, diminished phosphorylation of PIK3 and AKT, and the downregulation of Wnt β-catenin expression (Wu D. et al., 2020).
5.2 Inhibition of cell proliferation and growth
Through their interactions with the Wnt/β-catenin signaling pathway, TCM monomer and its metabolites have demonstrated remarkable efficacy in regulating the growth and proliferation of breast cancer cells. Ganoderma lucidum (Leyss.ex Fr.) Karst (Ganoderma lucidum) has been observed to inhibit the proliferation of human MDA-MB-231 and mouse 4T1 breast cancer cells. This is achieved by reducing LRP6 phosphorylation and inhibiting the expression of Axing, a Wnt target gene activated by Wnt3. Furthermore, Ganoderma lucidum (Leyss.ex Fr.) Karst (Ganoderma lucidum) has been shown to effectively suppress the Wnt/β-catenin signaling pathway. The concentration of Ganoderma lucidum (Leyss.ex Fr.) Karst (Ganoderma lucidum) required to achieve these effects is consistent with the concentration needed to inhibit breast cancer cell proliferation and migration (Zhang, 2017).
Deguelin, on the other hand, in MDA-MB-231 cells can reduces the expression of β-catenin and its downstream target gene, c-myc, by downregulating frizzled 7 expression. This action has been linked to the inhibition of TMBC cell proliferation (Murillo et al., 2009; Rodenberg and Brown, 2009). Actinidia chinensis Planch. root extract (AcRoots) impacts the proliferation of breast cancer MDA-MB-231 and MDA-MB-453 cells by decreasing levels of phosphorylated AKT, phosphorylated GSK-3β, and β-catenin (Gan et al., 2021). Ophiopogonin D, a steroidal glycoside derived from the TCM Ophiopogon japonicus (Thunb.) Ker Gawl. (Asparagaceae; Ophiopogonis radix), has been observed to dose-dependently inhibit the upregulation of the Wnt/β-catenin signaling pathway in MDA-MB-231 cells (Zhu et al., 2020). Honokiol is a bioactive bisphenol botanical drug found in the leaves and extracts of Magnolia officinalis Rehder and E.H.Wilson (Magnoliaceae; Magnoliae officinalis cortex) (Banik et al., 2019), which inhibits the growth of breast cancer cells and the proliferation of SK-BR-3 cells in diet-induced obese mouse models (ip, 3 mg/day, three times per week, 4 weeks) by inhibiting Wnt/β-catenin signaling pathway (Avtanski et al., 2015; Shi et al., 2020).
5.3 Modulation of cell migration and invasion
Betulinic acid, a metabolite extracted from Betula pendula subsp. mandshurica (Regel) Ashburner and McAll. (Betulaceae; Betula platyphylla suk), has been identified to elevate the expression of GRP78, curtail β-catenin-driven aerobic glycolysis in cell cultures, and inhibit lung metastasis of breast cancer cells in nude mice carrying MDA-MB-231 cells (ip, 125 and 250 mg/kg/day, 4 weeks) by regulating GRP78/β-catenin/c-Myc signal cascade. (Zheng Y. F. et al., 2019). Astragaloside IV, a primary triterpenoid from Astragalus mongholicus Bunge (Fabaceae; Astragali radix), operates by reducing Rac1 levels via Vav3 downregulation. This action subsequently affects β-catenin nuclear expression, thereby curbing the invasive tendencies of MDA-MB-231 breast cancer cells (Jiang et al., 2017). Lastly, Telocinobufagin, a metabolite from Bufonis venenum, indirectly interacts with the Wnt/β-catenin signaling pathway by regulating the PI3K/AKT/ERK/snail signaling cascade. This regulation results in a notable decrease in the mobility and invasiveness of breast cancer cells in nude mice carrying 4T1 cells (ip, 20 µg, three times a week, for 2 weeks) (Gao et al., 2018).
The LRP6 receptor, a type I transmembrane protein, is part of the low density lipoprotein receptor gene family and is highly conserved. Its phosphorylation is often seen as an indication of Wnt/β-catenin signaling pathway activation (Pinson et al., 2000; Tamai et al., 2000; Wehrli et al., 2000; Jeong and Jho, 2021). Gigantol, a hydroxybenzene metabolite sourced from several medicinal orchids, has been identified to suppress the Wnt/β-catenin signaling pathway by reducing the levels of phosphorylated LRP6 and cytoplasmic β-catenin. Consequently, it significantly curtails the survival and migratory capabilities of breast cancer HEK293, MDA-MB-231 and MDA-MB-468 cells (Yu et al., 2018). Similarly, Ganoderma lucidum (Leyss.ex Fr.) Karst (Ganoderma lucidum) has been observed to inhibit the migration of breast cancer cells, specifically MDA-MB-231 and 4T1 cellls (Zhang, 2017). Chlorogenic Acid, a notable bioactive metabolite present in various TCMs like Lonicera japonica Thunb. (Caprifoliaceae; Lonicerae japonicae flos) and Eucommia ulmoides Oliv. (Eucommiaceae; Eucommiae cortex), directly interacts with the Wnt/β-catenin signaling co-receptor LRP6. This interaction leads to a reduction in the expression of LRP6, p-LRP6, and β-catenin in MCF-7 breast cancer cells, subsequently inhibiting the invasion of these cells (Xue et al., 2023).
Furthermore, Jatrorrhizine, the metabolite of Coptis chinensis Franch. (Ranunculaceae; Coptidis rhizoma) has been found to induce apoptosis of breast cancer cells in nude mice bearing 4T1 cells (ip, 2.5 and 5 mg/kg, 4 weeks) and impede their metastasis in a dose-dependent manner through the modulation of the Wnt/β-catenin signaling pathway (Sun et al., 2019; Zhao and Wang, 2023). Astragalus polysaccharide has been found to exhibit a significant inhibitory effect on the migration and invasion of MDA-MB-231 cell transplanted tumor model in nude mice (ig, 100 mg/kg/day, 15 days). This effect is dose-dependent and is achieved through the downregulation of the Wnt/β-catenin signaling pathway, as well as the downregulation of c-Myc and Cyclin D1 levels, and the inhibition of EMT (Jiao et al., 2016; Ma et al., 2016; Yang S. et al., 2020). Spatholobus suberectus Dunn [Fabaceae; Spatholobi caulis] (Chen et al., 2016) and some metabolites of TCM, such as baicalein (Ma et al., 2016), emodin (Liu et al., 2020), acRoots (Gan et al., 2021), ophiopogonin D (Zhu et al., 2020), licochalcone A (Huang et al., 2019), and capsaicin (Wu D. et al., 2020) have demonstrated promising potential in the inhibition of invasive and migratory behaviors of breast cancer cells through their interaction with the Wnt/β-catenin signaling pathway.
5.4 Promotion of apoptosis in breast cancer cells
Wolfiporia cocos (F.A. Wolf) Ryvarden and Gilb (Poria cocos), a well-known botanical drug, is highly regarded for its dual functionality, encompassing both its anti-cancer properties and its ability to enhance the immune system. The aforementioned approach has played a pivotal role in the management of breast cancer and the provision of quality care to patients. Pachymic acid has the ability to regulate the cell cycle of breast cancer cells in nude mice transplanted tumor model of MDA-MB-231 cells (ig, 700 mg/kg/day, 25 days). This modulation can be attributed to the downregulation of cyclinD1, cyclinE, cyclin-dependent kinase 2, and cyclin dependent kinase 4, along with the upregulation of p53 and p21 proteins. The series of events described ultimately leads to the activation of programmed cell death, known as apoptosis, in breast cancer cells (Jiang and Fan, 2020).
Matrine has been identified to inhibit the growth of breast cancer cells, specifically 4T1 and MCF-7 cells. It also prompts apoptosis in these cells in a dose- and time-responsive manner. This is achieved by suppressing the expression of vascular endothelial factors and down-regulating the Wnt/β-catenin signaling pathway (Xiao et al., 2018).
Saikosaponin D, which is a major metabolite of Bupleurum chinense DC. (Apiaceae; Bupleuri radix), has demonstrated the ability to inhibit the Wnt/β-catenin signaling pathway. This inhibition has been observed to induce apoptosis and decrease proliferation in HCC1937 cells, a specific subtype of TNBC (Wang et al., 2018a). Actinidia chinensis Planch. root extract metabolites have the ability to attenuate the phosphorylation of GSK-3β, leading to a subsequent decrease in the phosphorylation of β-catenin at specific residues. This phenomenon leads to the degradation of β-catenin, which subsequently inhibits the Wnt/β-catenin signaling pathway, thereby facilitating apoptosis in MDA-MB-231 and MDA-MB-453 breast cancer cells (MacDonald et al., 2009; Gan et al., 2021). Furthermore, some metabolites of TCM, such as triptolide (Shao et al., 2014), naringin (Li et al., 2013), and baicalein (Yu P. et al., 2023), have been discovered to induce apoptosis in breast cancer cells through the inhibition of β-catenin.
6 Potential of TCM formulas in breast cancer treatment
Traditional Chinese Medicine compounds are renowned for their multifaceted approach to treating malignant tumors. They target a plethora of sites, traverse various pathways, and manifest diverse therapeutic effects. One of the standout features of TCM is its minimal toxicity and side effects. Moreover, it bolsters the body’s immune defenses and presents a reduced risk of drug resistance, making it an increasingly attractive avenue for research in recent times. Empirical studies have underscored the efficacy of TCM in inhibiting cell proliferation and growth, curtailing cell migration and invasion, promoting cell apoptosis, and amplifying anti-inflammatory responses, primarily through the modulation of the Wnt/β-catenin signaling pathway (Table 3).
6.1 Inhibition of cell proliferation and growth
Many TCM prescriptions, as part of CAM, have been utilized in the treatment of breast cancer by clearing away heat and toxins, promoting blood circulation, and eliminating blood stasis, leading to positive outcomes. Research has found that the main mechanism of action of TCM formulas is to regulate the Wnt/β-catenin signaling pathway, which inhibits the growth and proliferation of breast cancer cells. For example, the XiaoPi formula demonstrates a notable capacity to suppress the activity of tumor-associated macrophages when co-cultured with breast cancer cell lines MDA-MB-231 and 4T1. This suppression results in a marked decrease in the proliferation and self-renewal capabilities of these cancer cells. Moreover, the XiaoPi formula can counteract the secretion of CXCL1 and β-catenin, further diminishing the self-renewal and chemotherapy resistance of the cancer cells (Zheng et al., 2020). TaoHongSiWu decoction’s serum appears to modulate the Wnt signaling pathway by regulating the RNA and protein expression of pivotal targets such as HRAS, MAPK1, AKT1, GRB2, and MAPK14. This interaction with the β-catenin signaling pathway curtails cell proliferation in breast cancer cell lines MCF-7 and MDA-MB-231, with the degree of inhibition being both time and concentration-dependent (Huang et al., 2021).
6.2 Suppression of cellular migration and invasion
Most breast cancer is invasive and often metastasizes to lymph nodes and then to distant organs, including the bone, lungs, liver, and brain. At the same time, tumor metastasis is also the primary cause of death among breast cancer patients. Many studies have found that TCM prescriptions can regulate the Wnt/β-catenin signaling pathway, improve the microenvironment before breast cancer metastasis, and inhibit the metastasis ability of breast cancer cells. BaoYuanJieDu decoction offers another avenue of therapeutic potential. It acts by inhibiting the TGF-β/CCL9 signaling pathway, subsequently disrupting the Wnt/β-catenin signaling. This disruption is particularly significant as it hinders the recruitment of myelogenous suppressor cells to the lungs, a common site for breast cancer metastasis. By improving the pre-metastatic microenvironment, BaoYuanJieDu decoction not only hinders metastasis but also prolongs the survival of mice bearing 4T1 tumors (ig, 1 mL/10 g/day, 2 weeks) (Tian et al., 2020).
In a study conducted by Zhang et al. (2019), it was observed that the administration of the LiuWeiDiHuang pill resulted in the upregulation of axin-2, while simultaneously downregulating TCF-1, β-catenin, cyclin D1, and VEGF in TNBC bearing-mice (po, 2.3 g/kg/day). This modulation hinders the activation of the β-catenin/TCF-1 pathway, consequently diminishing the metastatic capacity of TNBC cells, specifically towards organs such as the lungs and liver (Zheng L. X. et al., 2019). Furthermore, there is clinical evidence indicating that women diagnosed with type 2 diabetes have a higher susceptibility to developing breast cancer compared to women without diabetes. The study revealed a correlation between elevated blood sugar levels and the development of breast cancer in breast cells (Michels et al., 2003). Additionally, it was found that the administration of the LiuWeiDiHuang pill, a TCM, can mitigate the risk of breast cancer by effectively managing diabetes (Wu C. T. et al., 2018).
RuYiPing is a frequently employed compound medication for the clinical management of metastatic breast cancer. Ye et al. (2019) discovered that the combination of RuYiPing and Platycodon grandiflorus (Jacq.) A.DC. (Campanulaceae; Platycodonis radix) has the potential to decrease the expression of IL-1β, IL-6, CXCL2, and CXCL5, which in turn helps to preserve vascular integrity in 4T1 tumor-bearing mice (5.67 and 22.68 g/kg/day, 14 days). This mechanism ultimately inhibits the pre-metastatic microenvironment in the lungs for breast cancer (Ye et al., 2019).
6.3 Further insights
Traditional Chinese medicine prescriptions follow the principle of “Jun Chen Zuo Shi” and typically consist of a variety of TCM components, each capable of exerting diverse therapeutic effects on specific targets. Research has found that TCM prescriptions can not only have therapeutic effects on breast cancer but also can treat some postoperative complications of the disease. For example, Winardi et al. (2023) discovered that the administration of SanHuangXieXin Decoction resulted in a notable decrease in patient mortality. Furthermore, patients with breast cancer who received the compound medicine exhibited lower mortality rates compared to those who solely received single ingredient treatments (Winardi et al., 2023). Simultaneously, San Huang Tang has demonstrated its efficacy in reducing inflammatory markers, including TNF-α, IL-6, IL-8, and c-reactive protein. By modulating these markers, it indirectly influences the Wnt/β-catenin signaling pathway. This modulation has been linked to a notable decrease in postoperative exudation and an enhancement in post-surgical inflammation management for breast cancer patients (Chen et al., 2021).
Another promising combination involves TaoHongSiWu Decoction paired with neoadjuvant chemotherapy, specifically the CAF/CEF or TAG/TEC regimens. This combination has been observed to effectively curb both tumor lymphangiogenesis and angiogenesis in breast cancer patients. The underlying mechanism is believed to involve the suppression of VEGF-C and VEGF-A expression, along with alterations in lymphatic vessel density and microvessel density. Moreover, the combined influence of TaoHongSiWu Decoction and chemotherapy drugs leads to a downregulation in the Wnt/β-catenin pathway, specifically targeting the downstream gene bcl-2. This results in an upregulation of the bax protein, a decrease in the bcl-2/bax ratio, and the induction of tumor cell apoptosis. At the same time, TaoHongSiWu Decoction is effective in treating upper limb swelling after breast cancer surgery, and the cure rate can reach 87.9%, showcasing its potential in treating invasive breast cancer (Jiang et al., 2021).
7 Biomarkers for breast cancer treatment using TCM based on key proteins in the Wnt pathway
Traditional Chinese Medicine has shown significant potential in breast cancer treatment, yet only a handful of TCM formulations are widely used in clinical practice. To gain broader acceptance and application for TCM in breast cancer therapy, rigorous scientific research and validation are essential.
A key challenge in TCM is the variability of ingredients in herbal formulations, making standardization and quality control paramount. Unlike the quality control methods for foreign herbal medicines, which primarily focus on chemical qualitative identification and indicator components, TCM’s complexity demands a more nuanced approach. TCM’s efficacy cannot be fully captured by chemical analysis alone due to its multi-component, multi-target nature, and the intricacies of its effects and compatibility (Wu X. et al., 2018). Research has found that biomarkers derived from metabolomics offer advantages in terms of completeness, systematicity, and quantification. They also exhibit strong discriminative power in assessing the quality of TCM. The quality evaluation of TCM can be directly and simultaneously examined from both chemical and biological aspects. The quality evaluation of TCM reflects the effectiveness and safety of this practice. Moreover, biomarkers can simplify the evaluation of the quality of TCM by offering direct indicators of biological impact (Wu X. et al., 2018; Gao et al., 2020). Thus, biomarkers have emerged as vital indicators of biological activity, offering a novel approach to assessing the safety and efficacy of TCM (Giridhar and Liu, 2019; Wu and Chu, 2021). These biomarkers, with their high specificity and sensitivity, are instrumental not only in evaluating TCM quality but also in monitoring its quality fluctuations. For example, research has shown that endogenous biomarkers such as LysoPC (22:5), valine, and shikimic acid can be utilized to assess the toxic components of Aconitum carmichaelii Debeaux (Ranunculaceae; Aconiti radix) (Zhou et al., 2016). Schisandrol A, schisandrin A, schisandrin C, and gomisin N are considered biomarkers for evaluating the quality standard of Schisandra chinensis (Turcz.) Baill. (Schisandraceae; Schisandrae chinensis fructus) (Zhang Y. et al., 2019). In the Chinese Pharmacopoeia, evodiamine is designated as a biomarker for evaluating the quality standards of Tetradium ruticarpum (A.Juss.) T.G.Hartley (Rutaceae; Evodiae fructus) and Chinese medicines containing this plant (Yang W. et al., 2017). The effectiveness of the Lianhua Qingwen Capsule can be assessed and managed using potential biomarkers such as L-ornithine, prostaglandin F2α, and arachidonic acid (Gao et al., 2020).
In addition, biomarkers can also serve as quality standards for evaluating the effectiveness of TCM in treating various diseases. For example, LysoPC (16:0), leucine, glutamine, 5-hydroxytryptamine, and other potential biomarkers are used to assess the quality of Wuda granule as a therapeutic agent for promoting recovery after surgical resection of colorectal cancer (Wang T. et al., 2020). Potential biomarkers such as glucose, lactic acid, and triglycerides can be used to evaluate the effectiveness of Salvia miltiorrhiza Bunge (Lamiaceae; Salviae miltiorrhizae radix et rhizoma) in treating colorectal cancer. Meanwhile, biomarkers such as glutathione, glyoxylate, and inosine are effective tools for assessing the potential anti-fatigue effects of Salvia miltiorrhiza Bunge (Lamiaceae; Salviae miltiorrhizae radix et rhizoma) (Wang Y. et al., 2021). Biomarkers such as D-galactose, inositol, and glycolol can be used to assess the effectiveness of Aconitum carmichaelii Debeaux (Ranunculaceae; Aconiti radix) in combination with Ampelopsis japonica (Thunb.) Makino (Vitaceae; Ampelopsis radix) for treating rheumatoid arthritis (Jin H. et al., 2019). Glycocholic acid, taurocholic acid, and indole acetate are considered potential biomarkers for evaluating the therapeutic effect of DaHuangXiaoShi decoction on cholestasis (Zhu and Feng, 2019). Biomarkers such as 2-ketobutyric acid, 3-hexenedioic acid, and argininic acid can be utilized as indicators to assess the therapeutic efficacy of Gout Party in the management of acute gouty arthritis (Wang et al., 2019).
For breast cancer treatment, TCM increasingly relies on biomarkers for standardization. Tumor biomarkers, produced by interactions between tumor tissues or the host and the tumor, are key in indicating tumor presence and progression (von Voithenberg et al., 2019). An ideal tumor biomarker should satisfy three key criteria: analytical validity, clinical validity, and clinical utility. Analytical validity necessitates that biomarkers exhibit accuracy, sensitiv patity, specificity, and stability. Clinical validity involves the biomarker’s capability to detect the disease’s status and project outcomes. Clinical utility implies that using the biomarker should improve patient outcomes compared to scenarios where it is not used (Merker et al., 2018).
Potential biomarkers of breast cancer, such as L-Arginine, arachidonic (Mao C. et al., 2022), urea (Nam et al., 2009), and palmitic acid (Tan et al., 2020), have been proven to be highly correlated with the Wnt/β-catenin signalinghway. The key protein components of this pathway, including β-catenin (Abu El Abbass et al., 2020), APC (Wang X. C. et al., 2021), c-Myc (Liu et al., 2021; Gao et al., 2023), Bcl-2 (Kolecková et al., 2017), and cyclin D1 (Liu N. Q. et al., 2022), are related to the occurrence, progression, and metastasis of breast cancer. They meet the criteria of tumor biomarkers and are expected to become potential prognostic and predictive biomarkers of breast cancer. Utilizing biomarkers to assess TCM quality is a critical step towards enhancing its existing quality control standards and achieving standardization. Therefore, the relationship between TCM and the Wnt/β-catenin signaling pathway is integral to developing a standardized and robust quality evaluation system for TCM, particularly in the context of breast cancer treatment.
8 Conclusion and outlook
Recently, there has been a notable surge in the integration of TCM into the comprehensive care of cancer patients, particularly in the postoperative and adjuvant stages of those undergoing radiotherapy and chemotherapy. Beyond its conventional uses, TCM extends its therapeutic reach by addressing the adverse effects that often follow breast cancer surgery, radiotherapy, and chemotherapy, utilizing methods such as dietary therapy and acupoint application. This integrative approach has yielded promising therapeutic outcomes. Contrasting with the often harsh nature of conventional radiotherapy and chemotherapy, TCM is characterized by its gentler approach, typically resulting in fewer side effects and reduced toxicity. This makes it an appealing option for enhancing patient comfort, alleviating pain, and potentially improving life expectancy. An abundance of research highlights the diverse mechanisms by which TCM can effectively combat tumors, offering a wider range of therapeutic options for managing breast cancer. This expanded repertoire of treatments underscores the growing significance of TCM in the holistic care of breast cancer patients.
This review delves into the influence of Chinese medicine monomers and compounds on various aspects of breast cancer cell dynamics, from cell cycle regulation and proliferation to migration, invasion, and apoptosis, all through the lens of the Wnt/β-catenin signaling pathway. The insights gleaned from this exploration aim to lay the groundwork for the future design of innovative drugs tailored to specific breast cancer subtypes or particular phases of the Wnt/β-catenin signaling cascade.
Author contributions
HL: Conceptualization, Writing–original draft, Writing–review and editing. JL: Data curation, Writing–review and editing. YZ: Visualization, Writing–original draft and editing. CZ: Investigation, Writing–original draft. JG: Visualization, Writing–original draft and Writing–review. YS: Formal Analysis, Writing–review and editing. HF: Methodology, Supervision, Writing–review and editing. YL: Methodology, Supervision, Writing–original draft, Writing–review and editing.
Funding
The authors declare that financial support was received for the research, authorship, and/or publication of this article. This research was funded by the National Natural Science Foundation of China, grant numbers 82074030, 82104568, and 82374050; College Students’ Innovation and Entrepreneurship Training Program Project of China, grant number 202310063009; Tianjin Science and Technology Plan Project, grant number 21JCQNJC01740.
Conflict of interest
The authors declare that the research was conducted in the absence of any commercial or financial relationships that could be construed as a potential conflict of interest.
Publisher’s note
All claims expressed in this article are solely those of the authors and do not necessarily represent those of their affiliated organizations, or those of the publisher, the editors and the reviewers. Any product that may be evaluated in this article, or claim that may be made by its manufacturer, is not guaranteed or endorsed by the publisher.
Abbreviations
AcRoots, Actinidia chinensis Planch. Root; BCSCs, Breast Cancer Stem Cells; CAM, Complementary and Alternative Medicine; CXCL, Chemokine (C-X-C) Ligand; EMT, Epithelial-Mesenchymal Transition; ER, Estrogen Receptor; GSK-3β, Glycogen Synthase Kinase-3β; HER-2, Human Epidermal Receptor 2; LRP6, Low Density Lipoprotein Receptor-Related Protein 6; MaSCs, Mammary Stem Cells; PR, Progesterone Receptor; TCM, Traditional Chinese Medicine; TGF-β, Transforming Growth Factor-β; TNBC, Triple-Negative Breast Cancer; TNF-α, Tumor Necrosis Factor-alpha; VEGF, Vascular Endothelial Growth Factor.
References
Abdollahi, P., Ebrahimi, M., Motamed, N., and Samani, F. S. (2015). Silibinin affects tumor cell growth because of reduction of stemness properties and induction of apoptosis in 2D and 3D models of MDA-MB-468. Anti-Cancer Drugs 26, 487–497. doi:10.1097/cad.0000000000000205
Ablett, M. P., O'Brien, C. S., Sims, A. H., Farnie, G., and Clarke, R. B. (2014). A differential role for CXCR4 in the regulation of normal versus malignant breast stem cell activity. Oncotarget 5, 599–612. doi:10.18632/oncotarget.1169
Abu El Abbass, K., Abdellateif, M. S., Gawish, A. M., Zekri, A. R. N., Malash, I., and Bahnassy, A. A. (2020). The role of breast cancer stem cells and some related molecular biomarkers in metastatic and nonmetastatic breast cancer. Clin. Breast Cancer 20, E373–E384. doi:10.1016/j.clbc.2019.11.008
Albanell, J., Martinez, M. T., Ramos, M., O'Connor, M., de la Cruz-Merino, L., Santaballa, A., et al. (2022). Randomized phase II study of fulvestrant plus palbociclib or placebo in endocrine-sensitive, hormone receptor-positive/HER2-advanced breast cancer: GEICAM/2014-12 (FLIPPER). Eur. J. Cancer 161, 26–37. doi:10.1016/j.ejca.2021.11.010
Al-Hajj, M., Wicha, M. S., Benito-Hernandez, A., Morrison, S. J., and Clarke, M. F. (2003). Prospective identification of tumorigenic breast cancer cells. Proc. Natl. Acad. Sci. U. S. A. 100, 3983–3988. doi:10.1073/pnas.0530291100
Alharbi, K. S., Almalki, W. H., Makeen, H. A., Albratty, M., Meraya, A. M., Nagraik, R., et al. (2022). Role of Medicinal plant-derived Nutraceuticals as a potential target for the treatment of breast cancer. J. Food Biochem. 46, e14387. doi:10.1111/jfbc.14387
Antoni, D., Burckel, H., Josset, E., and Noel, G. (2015). Three-dimensional cell culture: a breakthrough in vivo. Int. J. Mol. Sci. 16, 5517–5527. doi:10.3390/ijms16035517
Arzi, L., Farahi, A., Jafarzadeh, N., Riazi, G., Sadeghizadeh, M., and Hoshyar, R. (2018). Inhibitory effect of crocin on metastasis of triple-negative breast cancer by interfering with wnt/β-catenin pathway in murine model. DNA Cell Biol. 37, 1068–1075. doi:10.1089/dna.2018.4351
Asano, M., Matsui, J., Towle, M. J., Wu, J. Y., McGonigle, S., De Boisferon, M. H., et al. (2018). Broad-spectrum preclinical antitumor activity of eribulin (Halaven®): combination with anticancer agents of differing mechanisms. Anticancer Res. 38, 3375–3385. doi:10.21873/anticanres.12604
Astudillo, P., and Larrain, J. (2014). Wnt signaling and cell-matrix adhesion. Curr. Mol. Med. 14, 209–220. doi:10.2174/1566524014666140128105352
Avtanski, D. B., Nagalingam, A., Kuppusamy, P., Bonner, M. Y., Arbiser, J. L., Saxena, N. K., et al. (2015). Honokiol abrogates leptin-induced tumor progression by inhibiting Wnt1-MTA1-β-catenin signaling axis in a microRNA-34a dependent manner. Oncotarget 6, 16396–16410. doi:10.18632/oncotarget.3844
Ayadi, M., Bouygues, A., Ouaret, D., Ferrand, N., Chouaib, S., Thiery, J. P., et al. (2015). Chronic chemotherapeutic stress promotes evolution of stemness and WNT/beta-catenin signaling in colorectal cancer cells: implications for clinical use of WNT-signaling inhibitors. Oncotarget 6, 18518–18533. doi:10.18632/oncotarget.3934
Azbazdar, Y., Karabicici, M., Erdal, E., and Ozhan, G. (2021). Regulation of wnt signaling pathways at the plasma membrane and their misregulation in cancer. Front. Cell. Dev. Biol. 9, 631623. doi:10.3389/fcell.2021.631623
Banik, K., Ranaware, A. M., Deshpande, V., Nalawade, S. P., Padmavathi, G., Bordoloi, D., et al. (2019). Honokiol for cancer therapeutics: a traditional medicine that can modulate multiple oncogenic targets. Pharmacol. Res. 144, 192–209. doi:10.1016/j.phrs.2019.04.004
Bertozzi, A., Wu, C. C., Hans, S., Brand, M., and Weidinger, G. (2022). Wnt/β-catenin signaling acts cell-autonomously to promote cardiomyocyte regeneration in the zebrafish heart. Dev. Biol. 481, 226–237. doi:10.1016/j.ydbio.2021.11.001
Bighin, C., Pronzato, P., and Del Mastro, L. (2013). Trastuzumab emtansine in the treatment of HER-2-positive metastatic breast cancer patients. Future Oncol. 9, 955–957. doi:10.2217/fon.13.74
Bortolotto, L. F. B., Barbosa, F. R., Silva, G., Bitencourt, T. A., Beleboni, R. O., Baek, S. J., et al. (2017). Cytotoxicity of trans-chalcone and licochalcone A against breast cancer cells is due to apoptosis induction and cell cycle arrest. Biomed. Pharmacother. 85, 425–433. doi:10.1016/j.biopha.2016.11.047
Bozorgi, A., Khazaei, M., and Khazaei, M. R. (2015). New findings on breast cancer stem cells: a review. J. Breast Canc 18, 303–312. doi:10.4048/jbc.2015.18.4.303
Bross, P. F., Baird, A., Chen, G., Jee, J. M., Lostritto, R. T., Morse, D. E., et al. (2003). Fulvestrant in postmenopausal women with advanced breast cancer. Clin. Cancer Res. 9, 4309–4317.
Bu, G. J., Lu, W. Y., Liu, C. C., Selander, K., Yoneda, T., Hall, C., et al. (2008). Breast cancer-derived Dickkopf1 inhibits osteoblast differentiation and osteoprotegerin expression: implication for breast cancer osteolytic bone metastases. Int. J. Cancer 123, 1034–1042. doi:10.1002/ijc.23625
Buyuk, B., Jin, S., and Ye, K. M. (2022). Epithelial-to-Mesenchymal transition signaling pathways responsible for breast cancer metastasis. Cell. Mol. Bioeng. 15, 1–13. doi:10.1007/s12195-021-00694-9
Cai, C. G., Yu, Q. C., Jiang, W. M., Liu, W., Song, W. Q., Yu, H., et al. (2014). R-spondin1 is a novel hormone mediator for mammary stem cell self-renewal. Genes Dev. 28, 2205–2218. doi:10.1101/gad.245142.114
Chai, W. X., Sun, L. G., Dai, F. H., Shao, H. S., Zheng, N. G., and Cai, H. Y. (2019). Inhibition of PRRX2 suppressed colon cancer liver metastasis via inactivation of Wnt/beta-catenin signaling pathway. Pathol. Res. Pract., 8. doi:10.1016/j.prp.2019.152593
Chavoshi, H., Taheri, M., Yim, M. L., and Sabzichi, M. (2023). Crocin-loaded liposomes sensitize MDA-MB 231 breast cancer cells to doxorubicin by inducing apoptosis. Process Biochem. 130, 272–280. doi:10.1016/j.procbio.2023.04.012
Chen, C., Yuan, S. M., Chen, X. H., Xie, J. P., and Wei, Z. (2023). Xihuang pill induces pyroptosis and inhibits progression of breast cancer cells via activating the cAMP/PKA signalling pathway. Am. J. Cancer Res. 13, 1347–1362.
Chen, F., Zhu, L., Hu, J. Y., Jiang, S. J., Liu, H., Zheng, J., et al. (2020). Bufalin attenuates triple-negative breast cancer cell stemness by inhibiting the expression of SOX2/OCT4. Oncol. Lett. 9, 171. doi:10.3892/ol.2020.12028
Chen, F. Q., Li, J. Q., Wang, H., and Ba, Q. (2021). Anti-tumor effects of Chinese medicine compounds by regulating immune cells in microenvironment. Front. Oncol. 11, 746917. doi:10.3389/fonc.2021.746917
Chen, X., Li, Q., Kan, X. X., Wang, Y. J., Li, Y. J., Yang, Q., et al. (2016). Extract of Caulis Spatholobi, a novel blocker targeting tumor cell-induced platelet aggregation, inhibits breast cancer metastasis. Oncol. Rep. 36, 3215–3224. doi:10.3892/or.2016.5184
Chung, Y. C., Chiu, H. H., Wei, W. C., Chang, K. J., and Chao, W. T. (2020). Application of trastuzumab emtansine in HER-2-positive and KRAS/BRAF-mutated colon cancer cells. Eur. J. Clin. Invest. 50, e13255. doi:10.1111/eci.13255
Coates, A. S., Winer, E. P., Goldhirsch, A., Gelber, R. D., Gnant, M., Piccart-Gebhart, M., et al. (2015). Tailoring therapies-improving the management of early breast cancer: st gallen international expert consensus on the primary therapy of early breast cancer 2015. Ann. Oncol. 26, 1533–1546. doi:10.1093/annonc/mdv221
Cui, C., Zhou, X. L., Zhang, W. D., Qu, Y., and Ke, X. S. (2018). Is β-catenin a druggable target for cancer therapy? Trends biochem. Sci. 43, 623–634. doi:10.1016/j.tibs.2018.06.003
Deeks, E. D. (2017). Neratinib: first global approval. Drugs 77, 1695–1704. doi:10.1007/s40265-017-0811-4
Dhillon, S. (2019). Neratinib in early-stage breast cancer: a profile of its use in the eu. Clin. Drug Invest. 39, 221–229. doi:10.1007/s40261-018-0741-2
Ding, X., Yang, Q. F., Kong, X. N., Haffty, B. G., Gao, S. M., and Moran, M. S. (2016). Radiosensitization effect of Huaier on breast cancer cells. Oncol. Rep. 35, 2843–2850. doi:10.3892/or.2016.4630
Du, C. Z., Li, Z., Wang, S., Zhou, Z. M., Wang, J. K., Dong, J., et al. (2014). Tongshu Capsule down-regulates the expression of estrogen receptor alpha and suppresses human breast cancer cell proliferation. Plos One, 7. doi:10.1371/journal.pone.0104261
Du, J., Sun, Y., Wang, X. F., Lu, Y. Y., Zhou, Q. M., and Su, S. B. (2013). Establishment of an experimental breast cancer ZHENG model and curative effect evaluation of zuo-jin wan. Evid.-based Complement. Altern. Med. 2013, 324732. doi:10.1155/2013/324732
Duan, X. C., Pan, L. Y., Bao, Q. Y., and Peng, D. Y. (2020). UPLC-Q-TOF-MS study of the mechanism of THSWD for breast cancer treatment. Front. Pharmacol. 10, 1625. doi:10.3389/fphar.2019.01625
Duman, B. B., Paydas, S., Tetiker, T., Gunaldi, M., Afsar, C. U., Erçolak, V., et al. (2012). Capecitabine-induced hypertriglyceridemia and hyperglycemia: two cases. Pharmacology 90, 212–215. doi:10.1159/000342382
Ellis, M. J., Coop, A., Singh, B., Mauriac, L., Llombert-Cussac, A., Janicke, F., et al. (2001). Letrozole is more effective neoadjuvant endocrine therapy than tamoxifen for ErbB-1- and/or ErbB-2-positive, estrogen receptor-positive primary breast cancer: evidence from a phase III randomized trial. J. Clin. Oncol. 19, 3808–3816. doi:10.1200/jco.2001.19.18.3808
Essex, A. L., Pin, F., Huot, J. R., Bonewald, L. F., Plotkin, L. I., and Bonetto, A. (2019). Bisphosphonate treatment ameliorates chemotherapy-induced bone and muscle abnormalities in young mice. Front. Endocrinol. 10, 809. doi:10.3389/fendo.2019.00809
Fang, P., Peng, L., Wang, H. Z., and Zhang, X. (2021). The role of miR-200c decorated liposomal nanoparticles as a carrier target for wnt/β-catenin signaling to regulate triple-negative breast cancer cell line. Sci. Adv. Mater. 13, 1622–1628. doi:10.1166/sam.2021.4076
Farahi, A., Abedini, M. R., Javdani, H., Arzi, L., Chamani, E., Farhoudi, R., et al. (2021). Crocin and Metformin suppress metastatic breast cancer progression via VEGF and MMP9 downregulations: in vitro and in vivo studies. Mol. Cell. Biochem. 476, 3341–3351. doi:10.1007/s11010-020-04043-8
Farghadani, R., and Naidu, R. (2022). Curcumin as an enhancer of therapeutic efficiency of chemotherapy drugs in breast cancer. Int. J. Mol. Sci. 19, 2144. doi:10.3390/ijms23042144
Fatima, I., El-Ayachi, I., Taotao, L., Lillo, M. A., Krutilina, R., Seagroves, T. N., et al. (2017). The natural compound Jatrophone interferes with Wnt/beta-catenin signaling and inhibits proliferation and EMT in human triple-negative breast cancer. Plos One, 18. doi:10.1371/journal.pone.0189864
Feng, X. C., Ge, J., Fu, H., Miao, L., Zhao, F., Wang, J. Y., et al. (2023). Discovery of small molecule 13-catenin suppressors that enhance immunotherapy. Bioorg. Chem., 13. doi:10.1016/j.bioorg.2023.106754
Fermaintt, C. S., Peramuna, T., Cai, S. X., Takahashi-Ruiz, L., Essif, J. N., Grant, C. V., et al. (2021). Yuanhuacine is a potent and selective inhibitor of the basal-like 2 subtype of triple negative breast cancer with immunogenic potential. Cancers 13, 2834. doi:10.3390/cancers13112834
Firouzi, J., Sotoodehnejadnematalahi, F., Shokouhifar, A., Rahimi, M., Sodeifi, N., Sahranavardfar, P., et al. (2022). Silibinin exhibits anti-tumor effects in a breast cancer stem cell model by targeting stemness and induction of differentiation and apoptosis. BioImpacts 12, 415–429. doi:10.34172/bi.2022.23336
Fu, N. Y., Nolan, E., Lindeman, G. J., and Visvader, J. E. (2020a). Stem cells and the differentiation hierarchy in mammary gland development. Physiol. Rev. 100, 489–523. doi:10.1152/physrev.00040.2018
Fu, R. Q., Zhang, L., Li, Y. Y., Li, B., Ming, Y., Li, Z. W., et al. (2020b). Saikosaponin D inhibits autophagosome-lysosome fusion and induces autophagy-independent apoptosis in MDA-MB-231 breast cancer cells. Mol. Med. Rep. 22, 1026–1034. doi:10.3892/mmr.2020.11155
Fu, Y. J., Chang, H., Peng, X. L., Bai, Q., Yi, L., Zhou, Y., et al. (2014). Resveratrol inhibits breast cancer stem-like cells and induces autophagy via suppressing wnt/beta-catenin signaling pathway. Plos One, 8. doi:10.1371/journal.pone.0102535
Fumagalli, C., and Barberis, M. (2021). Breast cancer heterogeneity. Diagnostics 11, 1555. doi:10.3390/diagnostics11091555
Gan, C. C., Jin, Z., Wei, X. P., and Jin, M. N. (2021). Actinidia chinensis Planch. root extract inhibits the proliferation, migration and invasion of breast cancer cells via the AKT/GSK-3β signaling pathway. Folia histochem. Cytobiol. 59, 226–235. doi:10.5603/FHC.a2021.0023
Gangrade, A., Pathak, V., Augelli-Szafran, C. E., Wei, H. X., Oliver, P., Suto, M., et al. (2018). Preferential inhibition of wnt/-catenin signaling by novel benzimidazole compounds in triple-negative breast cancer. Int. J. Mol. Sci. 17. doi:10.3390/ijms19051524
Gao, D., Niu, M., Wei, S. Z., Zhang, C. E., Zhou, Y. F., Yang, Z. W., et al. (2020). Identification of a pharmacological biomarker for the bioassay-based quality control of a thirteen-component TCM formula (Lianhua qingwen) used in treating influenza A virus (H1N1) infection. Front. Pharmacol. 13, 746. doi:10.3389/fphar.2020.00746
Gao, F. Y., Li, X. T., Xu, K., Wang, R. T., and Guan, X. X. (2023). c-MYC mediates the crosstalk between breast cancer cells and tumor microenvironment. Cell Commun. Signal. 21, 28. doi:10.1186/s12964-023-01043-1
Gao, S. M., Li, X. Y., Ding, X., Jiang, L. Y., and Yang, Q. F. (2017). Huaier extract restrains the proliferative potential of endocrine-resistant breast cancer cells through increased ATM by suppressing miR-203. Sci. Rep. 7, 7313. doi:10.1038/s41598-017-07550-9
Gao, Y. X., Shi, L. H., Cao, Z., Zhu, X. T., Li, F., Wang, R. Y., et al. (2018). Telocinobufagin inhibits the epithelial-mesenchymal transition of breast cancer cells through the phosphoinositide 3-kinase/protein kinase B/extracellular signal-regulated kinase/Snail signaling pathway. Oncol. Lett. 15, 7837–7845. doi:10.3892/ol.2018.8349
Ginestier, C., Hur, M. H., Charafe-Jauffret, E., Monville, F., Dutcher, J., Brown, M., et al. (2007). ALDH1 is a marker of normal and malignant human mammary stem cells and a predictor of poor clinical outcome. Cell Stem Cell 1, 555–567. doi:10.1016/j.stem.2007.08.014
Gingras, A. C., Raught, B., and Sonenberg, N. (1999). eIF4 initiation factors: effectors of mRNA recruitment to ribosomes and regulators of translation. Annu. Rev. Biochem. 68, 913–963. doi:10.1146/annurev.biochem.68.1.913
Giridhar, K. V., and Liu, M. C. (2019). Available and emerging molecular markers in the clinical management of breast cancer. Expert Rev. Mol. diagn. 19, 919–928. doi:10.1080/14737159.2019.1664901
Gonzalez-Angulo, A. M., Morales-Vasquez, F., and Hortobagyi, G. N. (2007). “Overview of resistance to systemic therapy in patients with breast cancer,” in Breast cancer chemosensitivity. Editors D. Yu, and M. C. Hung (Berlin: Springer-Verlag Berlin), 1–22.
Goto, W., Kashiwagi, S., Takada, K., Asano, Y., Takahashi, K., Fujita, H., et al. (2018). Significance of intrinsic breast cancer subtypes on the long-term prognosis after neoadjuvant chemotherapy. J. Transl. Med. 10, 307. doi:10.1186/s12967-018-1679-0
Gui, Y., Dai, Y. F., Wang, Y. M., Li, S. R., Xiang, L., Tang, Y. Q., et al. (2022). Taohong Siwu Decoction exerts anticancer effects on breast cancer via regulating MYC, BIRC5, EGF and PIK3R1 revealed by HTS2 technology. Comp. Struct. Biotechnol. J. 20, 3461–3472. doi:10.1016/j.csbj.2022.06.044
Guo, L. T., Kong, D. G., Liu, J. H., Zhan, L., Luo, L., Zheng, W. J., et al. (2023). Breast cancer heterogeneity and its implication in personalized precision therapy. Exp. Hematol. Oncol. 12, 3. doi:10.1186/s40164-022-00363-1
Guo, Y. B., Chen, B. B., Pei, X. H., and Zhang, D. W. (2020). Radix stephaniae tetrandrine: an emerging role for management of breast cancer. Curr. Pharm. Des. 26, 25–36. doi:10.2174/1381612826666200110143706
Gwynne, W. D., Shakeel, M. S., Girgis-Gabardo, A., and Hassell, J. A. (2021). The role of serotonin in breast cancer stem cells. Molecules 26, 3171. doi:10.3390/molecules26113171
Hagiwara, K., Kosaka, N., Yoshioka, Y., Takahashi, R., Takeshita, F., and Ochiya, T. (2012). Stilbene derivatives promote Ago2-dependent tumour-suppressive microRNA activity. Sci. Rep. 2, 314. doi:10.1038/srep00314
Han, W. Z., Meng, F. W., Gan, H., Guo, F., Ke, J. F., and Wang, L. P. (2021). Targeting self-assembled F127-peptide polymer with pH sensitivity for release of anticancer drugs. RSC Adv. 11, 1461–1471. doi:10.1039/d0ra09898a
Hao, J., Jin, Z. Q., Zhu, H. X., Liu, X. H., Mao, Y., Yang, X., et al. (2018). Antiestrogenic activity of the xi-huang formula for breast cancer by targeting the estrogen receptor α. Cell. Physiol. biochem. 47, 2199–2215. doi:10.1159/000491533
Harper, K. L., Sosa, M. S., Entenberg, D., Hosseini, H., Cheung, J. F., Nobre, R., et al. (2016). Mechanism of early dissemination and metastasis in Her2(+) mammary cancer. Nature 540, 588–592. doi:10.1038/nature20609
Hashemi, M., Hasani, S., Hajimazdarany, S., Ghadyani, F., Olyaee, Y., Khodadadi, M., et al. (2023). Biological functions and molecular interactions of Wnt/13-catenin in breast cancer: revisiting signaling networks. Int. J. Biol. Macromol. 18. doi:10.1016/j.ijbiomac.2023.123377
Hermawan, A., Ikawati, M., Jenie, R. I., Khumaira, A., Putri, H., Nurhayati, I. P., et al. (2021). Identification of potential therapeutic target of naringenin in breast cancer stem cells inhibition by bioinformatics and in vitro studies. Saudi Pharm. J. 29, 12–26. doi:10.1016/j.jsps.2020.12.002
Hernandez, A. R., Klein, A. M., and Kirschner, M. W. (2012). Kinetic responses of β-catenin specify the sites of Wnt control. Science 338, 1337–1340. doi:10.1126/science.1228734
Huang, S., Chen, Y., Pan, L. Y., Fei, C. Y., Wang, N., Chu, F. R., et al. (2021). Exploration of the potential mechanism of tao hong Si Wu decoction for the treatment of breast cancer based on network pharmacology and in vitro experimental verification. Front. Oncol. 11, 731522. doi:10.3389/fonc.2021.731522
Huang, W. C., Su, H. H., Fang, L. W., Wu, S. J., and Liou, C. J. (2019). Licochalcone A inhibits cellular motility by suppressing E-cadherin and MAPK signaling in breast cancer. Cells 8, 218. doi:10.3390/cells8030218
Javir, G., Joshi, K., Khedkar, V., and Rojatkar, S. (2020). 6 alpha-Hydroxy-4 14, 10 15 -guainadien-8 beta, 12-olide induced cell cycle arrest via modulation of EMT and Wnt/beta-catenin pathway in HER-2 positive breast cancer cells. J. Steroid Biochem. Mol. Biol., 16. doi:10.1016/j.jsbmb.2019.105514
Jeong, W., and Jho, E. H. (2021). Regulation of the low-density lipoprotein receptor-related protein LRP6 and its association with disease: wnt/beta-catenin signaling and beyond. Front. Cell. Dev. Biol. 14. doi:10.3389/fcell.2021.714330
Jeong, W. J., Ro, E. J., and Choi, K. Y. (2018). Interaction between Wnt/β-catenin and RAS-ERK pathways and an anti-cancer strategy via degradations of β-catenin and RAS by targeting the Wnt/β-catenin pathway. npj Precis. Oncol. 2, 5. doi:10.1038/s41698-018-0049-y
Jia, L., Pina-Crespo, J., and Li, Y. H. (2019). Restoring Wnt/beta-catenin signaling is a promising therapeutic strategy for Alzheimer's disease. Mol. Brain, 11. doi:10.1186/s13041-019-0525-5
Jiang, G., Xiao, X., Zeng, Y., Nagabhushanam, K., Majeed, M., and Xiao, D. (2013). Targeting beta-Catenin signaling to induce apoptosis in human breast cancer cells by z-Guggulsterone and Gugulipid extract of Ayurvedic medicine plant Commiphora mukul. BMC Complement. Altern. Med. 13, 203. doi:10.1186/1472-6882-13-203
Jiang, H. J., Li, M. M., Du, K. Q., Ma, C., Cheng, Y. F., Wang, S. J., et al. (2021). Traditional Chinese medicine for adjuvant treatment of breast cancer: taohong siwu decoction. Chin. Med. 16, 129. doi:10.1186/s13020-021-00539-7
Jiang, K., Lu, Q., Li, Q., Ji, Y. J., Chen, W. L., and Xue, X. H. (2017). Astragaloside IV inhibits breast cancer cell invasion by suppressing Vav3 mediated Rac1/MAPK signaling. Int. Immunopharmacol. 42, 195–202. doi:10.1016/j.intimp.2016.10.001
Jiang, S. J., Zhang, M. F., Zhang, Y. H., Zhou, W. P., Zhu, T., Ruan, Q., et al. (2019). WNT5B governs the phenotype of basal-like breast cancer by activating WNT signaling. Cell Commun. Signal. 17, 109. doi:10.1186/s12964-019-0419-2
Jiang, Y., and Fan, L. P. (2020). Evaluation of anticancer activities of Poria cocos ethanol extract in breast cancer: in vivo and in vitro, identification and mechanism. J. Ethnopharmacol. 257, 112851. doi:10.1016/j.jep.2020.112851
Jiao, R., Liu, Y. X., Gao, H., Xiao, J., and So, K. F. (2016). The anti-oxidant and antitumor properties of plant polysaccharides. Am. J. Chin. Med. 44, 463–488. doi:10.1142/s0192415x16500269
Jin, H., Ma, N., Li, X., Kang, M., Guo, M., and Song, L. (2019b). Application of GC/MS-Based metabonomic profiling in studying the therapeutic effects of Aconitum carmichaeli with Ampelopsis japonica extract on collagen-induced arthritis in rats. Molecules 24, 1934. doi:10.3390/molecules24101934
Jin, J. M., Qiu, S. P., Wang, P., Liang, X. H., Huang, F., Wu, H., et al. (2019a). Cardamonin inhibits breast cancer growth by repressing HIF-1 alpha-dependent metabolic reprogramming. J. Exp. Clin. Cancer Res., 16. doi:10.1186/s13046-019-1351-4
Kahn, M. (2014). Can we safely target the WNT pathway? Nat. Rev. Drug Discov. 13, 513–532. doi:10.1038/nrd4233
Kanwar, S. S., Yu, Y. J., Nautiyal, J., Patel, B. B., and Majumdar, A. P. N. (2010). The Wnt/β-catenin pathway regulates growth and maintenance of colonospheres. Mol. Cancer 9, 212. doi:10.1186/1476-4598-9-212
Ke, D. Y. J., El-Sahli, S., and Wang, L. S. (2022). The potential of natural products in the treatment of triple-negative breast cancer. Curr. Cancer Drug Targets 22, 388–403. doi:10.2174/1568009622666211231140623
Kim, S. J., Kim, Y. S., Jang, E. D., Seo, K. J., and Kim, J. S. (2015). Prognostic impact and clinicopathological correlation of CD133 and ALDH1 expression in invasive breast cancer. J. Breast Canc 18, 347–355. doi:10.4048/jbc.2015.18.4.347
Kim, S. L., Choi, H. S., Kim, J. H., Jeong, D. K., Kim, K. S., and Lee, D. S. (2019). Dihydrotanshinone-induced NOX5 activation inhibits breast cancer stem cell through the ROS/Stat3 signaling pathway. Oxidative Med. Cell. Longev. 2019, 9296439. doi:10.1155/2019/9296439
Kolecková, M., Kolár, Z., Ehrmann, J., Korínková, G., and Trojanec, R. (2017). Age-associated prognostic and predictive biomarkers in patients with breast cancer. Oncol. Lett. 13, 4201–4207. doi:10.3892/ol.2017.6000
Koliou, P., Karavasilis, V., Theochari, M., Pollack, S. M., Jones, R. L., and Thway, K. (2018). Advances in the treatment of soft tissue sarcoma: focus on eribulin. Cancer Manag. Res. 10, 207–216. doi:10.2147/cmar.S143019
Koni, M., Pinnaro, V., and Brizzi, M. F. (2020). The wnt signalling pathway: a tailored target in cancer. Int. J. Mol. Sci. 26, 7697. doi:10.3390/ijms21207697
Koval, A., Pieme, C. A., Queiroz, E. F., Ragusa, S., Ahmed, K., Blagodatski, A., et al. (2018). Tannins from Syzygium guineense suppress Wnt signaling and proliferation of Wnt-dependent tumors through a direct effect on secreted Wnts. Cancer Lett. 435, 110–120. doi:10.1016/j.canlet.2018.08.003
Lamberti, C., Lin, K. M., Yamamoto, Y., Verma, U., Verma, I. M., Byers, S., et al. (2001). Regulation of beta-catenin function by the IkappaB kinases. J. Biol. Chem. 276, 42276–42286. doi:10.1074/jbc.M104227200
Li, H. Z., Yang, B., Huang, J., Xiang, T. X., Yin, X. D., Wan, J. Y., et al. (2013). Naringin inhibits growth potential of human triple-negative breast cancer cells by targeting β-catenin signaling pathway. Toxicol. Lett. 220, 219–228. doi:10.1016/j.toxlet.2013.05.006
Li, J., Li, L., Liu, R., and Lin, H. S. (2012). Establishing Chinese medicine characteristic tumor response evaluation system is the key to promote internationalization of Chinese medicine oncology. Chin. J. Integr. Med. 18, 730–736. doi:10.1007/s11655-012-1254-0
Li, K., and Lai, H. (2017). TanshinoneIIA enhances the chemosensitivity of breast cancer cells to doxorubicin through down-regulating the expression of MDR-related ABC transporters. Biomed. Pharmacother. 96, 371–377. doi:10.1016/j.biopha.2017.10.016
Li, S. Z., Zhao, Q., Wang, B., Yuan, S., Wang, X. Y., and Li, K. (2018b). Quercetin reversed MDR in breast cancer cells through down-regulating P-gp expression and eliminating cancer stem cells mediated by YB-1 nuclear translocation. Phytother. Res. 32, 1530–1536. doi:10.1002/ptr.6081
Li, X., Liang, T., Chen, S. S., Wang, M., Wang, R., Li, K., et al. (2020). Matrine suppression of self-renewal was dependent on regulation of LIN28A/Let-7 pathway in breast cancer stem cells. J. Cell. Biochem. 121, 2139–2149. doi:10.1002/jcb.29396
Li, X., Sun, R., Chen, W. P., Lu, B. M., Li, X. Y., Wang, Z. J., et al. (2014). A systematic in silico mining of the mechanistic implications and therapeutic potentials of estrogen receptor (ER)-alpha in breast cancer. Plos One, 11. doi:10.1371/journal.pone.0091894
Li, X. L., Zhou, N., Wang, J., Liu, Z. J., Wang, X. H., Zhang, Q., et al. (2018a). Quercetin suppresses breast cancer stem cells (CD44(+)/CD24(-)) by inhibiting the PI3K/Akt/mTOR-signaling pathway. Life Sci. 196, 56–62. doi:10.1016/j.lfs.2018.01.014
Li, X. T., Wang, X. Q., Xie, C. F., Zhu, J. Y., Meng, Y., Chen, Y., et al. (2018c). Sonic hedgehog and Wnt/β-catenin pathways mediate curcumin inhibition of breast cancer stem cells. Anti-Cancer Drugs 29, 208–215. doi:10.1097/cad.0000000000000584
Li, Y., Wang, J. H., Lin, F., Yang, Y. F., and Chen, S. S. (2017). A methodology for cancer therapeutics by systems pharmacology-based analysis: a case study on breast cancer-related traditional Chinese medicines. Plos One 12, e0169363. doi:10.1371/journal.pone.0169363
Li, Y. P., Chu, X. H., Yin, Y., Li, H. K., Fu, H., Feng, X. C., et al. (2023). Design, synthesis, and evaluation of 4-(3-(3,5-dimethylisoxazol-4-yl)benzyl)phthalazin-1(2H)-one derivatives: potent BRD4 inhibitors with anti-breast cancer activity. Front. Pharmacol. 14, 1289003. doi:10.3389/fphar.2023.1289003
Li, Y. P., Liu, L., Shang, H. T., Feng, X. C., Fan, N., Wang, J. Y., et al. (2022b). Self-assembling anchorage of hyaluronic acid on the nanoparticle surface confers superiority of triple negative breast cancer treatment. Pharmaceutics 14, 2461. doi:10.3390/pharmaceutics14112461
Li, Z., Wei, H. R., Li, S. Y., Wu, P., and Mao, X. Y. (2022a). The role of Progesterone receptors in breast cancer. Drug Des. Dev. Ther. 16, 305–314. doi:10.2147/dddt.S336643
Liao, M. M., Wang, C. W., Yang, B. W., Huang, D. P., Zheng, Y. F., Wang, S. Q., et al. (2021). Autophagy blockade by ai du qing formula promotes chemosensitivity of breast cancer stem cells via GRP78/β-catenin/ABCG2 Axis. Front. Pharmacol. 12, 19. doi:10.3389/fphar.2021.659297
Lin, C. C., Lo, M. C., Moody, R., Jiang, H., Harouaka, R., Stevers, N., et al. (2018). Targeting LRP8 inhibits breast cancer stem cells in triple-negative breast cancer. Cancer Lett. 438, 165–173. doi:10.1016/j.canlet.2018.09.022
Lin, C. Y., Wang, L., Wang, H., Yang, L. Q., Guo, H. J., and Wang, X. J. (2013). Tanshinone IIA inhibits breast cancer stem cells growth in vitro and in vivo through attenuation of IL-6/STAT3/NF-kB signaling pathways. J. Cell. Biochem. 114, 2061–2070. doi:10.1002/jcb.24553
Lin, D., Kuang, G., Wan, J. Y., Zhang, X., Li, H. Z., Gong, X., et al. (2017). Luteolin suppresses the metastasis of triple-negative breast cancer by reversing epithelial-to-mesenchymal transition via downregulation of β-catenin expression. Oncol. Rep. 37, 895–902. doi:10.3892/or.2016.5311
Liu, C. L., Dong, L. H., Sun, Z., Wang, L., Wang, Q. P., Li, H. Y., et al. (2018). Esculentoside A suppresses breast cancer stem cell growth through stemness attenuation and apoptosis induction by blocking IL-6/STAT3 signaling pathway. Phytother. Res. 32, 2299–2311. doi:10.1002/ptr.6172
Liu, H. Y., Li, X., Li, H. Y., Feng, L., Sun, G. S., Sun, G. Q., et al. (2022a). Potential molecular mechanisms and clinical progress in liver metastasis of breast cancer. Biomed. Pharmacother. 149, 112824. doi:10.1016/j.biopha.2022.112824
Liu, N. Q., Cao, W. H., Wang, X., Chen, J. Y., and Nie, J. Y. (2022b). Cyclin genes as potential novel prognostic biomarkers and therapeutic targets in breast cancer. Oncol. Lett. 24, 374. doi:10.3892/ol.2022.13494
Liu, Q., Hodge, J., Wang, J. F., Wang, Y. Z., Wang, L. M., Singh, U., et al. (2020). Emodin reduces breast cancer lung metastasis by suppressing macrophage-induced breast cancer cell epithelial-mesenchymal transition and cancer stem cell formation. Theranostics 10, 8365–8381. doi:10.7150/thno.45395
Liu, Y. Q., Zhu, C. J., Tang, L., Chen, Q., Guan, N., Xu, K., et al. (2021). MYC dysfunction modulates stemness and tumorigenesis in breast cancer. Int. J. Biol. Sci. 17, 178–187. doi:10.7150/ijbs.51458
Lu, B. Y., Natarajan, E., Raghavendran, H. R. B., and Markandan, U. D. (2023). Molecular classification, treatment, and genetic biomarkers in triple-negative breast cancer: a review. Technol. Cancer Res. Treat., 10. doi:10.1177/15330338221145246
Lu, W. Y., Lin, C. H., Roberts, M. J., Waud, W. R., Piazza, G. A., and Li, Y. H. (2011). Niclosamide suppresses cancer cell growth by inducing Wnt co-receptor LRP6 degradation and inhibiting the Wnt/β-catenin pathway. Plos One 6, e29290. doi:10.1371/journal.pone.0029290
Luga, V., Zhang, L., Viloria-Petit, A. M., Ogunjimi, A. A., Inanlou, M. R., Chiu, E., et al. (2012). Exosomes mediate stromal mobilization of autocrine wnt-PCP signaling in breast cancer cell migration. Cell 151, 1542–1556. doi:10.1016/j.cell.2012.11.024
Ma, B., and Hottiger, M. O. (2016). Crosstalk between wnt/β-catenin and NF-κB signaling pathway during inflammation. Front. Immunol. 7, 378. doi:10.3389/fimmu.2016.00378
Ma, J. L., Lu, W. Y., Chen, D. Q., Xu, B., and Li, Y. H. (2017). Role of wnt Co-receptor LRP6 in triple negative breast cancer cell migration and invasion. J. Cell. Biochem. 118, 2968–2976. doi:10.1002/jcb.25956
Ma, K., Chen, H., Wang, K., Han, X., Zhang, Y., Wang, H., et al. (2022). Pterostilbene inhibits the metastasis of TNBC via suppression of ? catenin-mediated epithelial to mesenchymal transition and stemness. J. Funct. Food., 13. doi:10.1016/j.jff.2022.105219
Ma, X. C., Yan, W. J., Dai, Z. J., Gao, X. Y., Ma, Y. A., Xu, Q. T., et al. (2016). Baicalein suppresses metastasis of breast cancer cells by inhibiting EMT via downregulation of SATB1 and Wnt/β-catenin pathway. Drug Des. Dev. Ther. 10, 1419–1441. doi:10.2147/dddt.S102541
Maass, N., Ostermann, H., Possinger, K., Klein, P., Tesch, H., Muhlenhoff, L., et al. (2019). ACT-FASTER, a prospective cohort study exploring treatment patterns with fulvestrant and exemestane in postmenopausal patients with advanced hormone receptor-positive breast cancer under real-life conditions in Germany. Breast Care 14, 401–408. doi:10.1159/000493882
MacDonald, B. T., Tamai, K., and He, X. (2009). Wnt/beta-catenin signaling: components, mechanisms, and diseases. Dev. Cell 17, 9–26. doi:10.1016/j.devcel.2009.06.016
Mao, C., Wang, M., Li, L., and Tang, J.-H. (2022b). Circulating metabolites serve as diagnostic biomarkers for HER2-positive breast cancer and have predictive value for trastuzumab therapy outcomes. J. Clin. Laboratory Analysis 36, e24212. doi:10.1002/jcla.24212
Mao, X. D., Wei, X., Xu, T., Li, T. P., and Liu, K. S. (2022a). Research progress in breast cancer stem cells: characterization and future perspectives. Am. J. Cancer Res. 12, 3208–3222.
Marsh, S., and Liu, G. (2009). Pharmacokinetics and pharmacogenomics in breast cancer chemotherapy. Adv. Drug Deliv. Rev. 61, 381–387. doi:10.1016/j.addr.2008.10.003
Maselli, A., Parlato, S., Puglisi, R., Raggi, C., Spada, M., Macchia, D., et al. (2019). Autoantibodies specific to ERα are involved in tamoxifen resistance in hormone receptor positive breast cancer. Cells 8, 750. doi:10.3390/cells8070750
Matsumoto, S., Harada, A., Seta, M., Akita, M., Gon, H., Fukumoto, T., et al. (2023). Wnt signaling stimulates cooperation between GREB1 and HNF4a to promote proliferation in hepatocellular carcinoma. Cancer Res. 83, 2312–2327. doi:10.1158/0008-5472.Can-22-3518
McGonigle, S., Chen, Z. H., Wu, J. Y., Chang, P., Kolber-Simonds, D., Ackermann, K., et al. (2015). E7449: a dual inhibitor of PARP1/2 and tankyrase1/2 inhibits growth of DNA repair deficient tumors and antagonizes Wnt signaling. Oncotarget 6, 41307–41323. doi:10.18632/oncotarget.5846
Merker, J. D., Oxnard, G. R., Compton, C., Diehn, M., Hurley, P., Lazar, A. J., et al. (2018). Circulating tumor DNA analysis in patients with cancer American society of clinical oncology and College of American pathologists joint review. Arch. Pathol. Lab. Med. 142, 1242–1253. doi:10.5858/arpa.2018-0901-SA
Meyer, C., Brockmueller, A., Buhrmann, C., and Shakibaei, M. (2024). Prevention and Co-management of breast cancer-related osteoporosis using resveratrol. Nutrients 16, 708. doi:10.3390/nu16050708
Michels, K. B., Solomon, C. G., Hu, F. B., Rosner, B. A., Hankinson, S. E., Colditz, G. A., et al. (2003). Type 2 diabetes and subsequent incidence of breast cancer in the Nurses' Health Study. Diabetes Care 26, 1752–1758. doi:10.2337/diacare.26.6.1752
Mo, L. Q., Xiao, X. Y., Song, S. L., Miao, H., Liu, S. T., Guo, D., et al. (2015). Protective effect of Huang Gan formula in 5/6 nephrectomized rats by depressing the Wnt/β-catenin signaling pathway. Drug Des. Dev. Ther. 9, 2867–2881. doi:10.2147/dddt.S81157
Mohammed, H., Russell, I. A., Stark, R., Rueda, O. M., Hickey, T. E., Tarulli, G. A., et al. (2015). Progesterone receptor modulates ERα action in breast cancer. Nature 523, 313–317. doi:10.1038/nature14583
Moreira, M. P., Braga, L. D., Cassali, G. D., and Silva, L. M. (2018). STAT3 as a promising chemoresistance biomarker associated with the CD44(+/high)/CD24(-/low)/ALDH(+) BCSCs-like subset of the triple-negative breast cancer (TNBC) cell line. Exp. Cell Res. 363, 283–290. doi:10.1016/j.yexcr.2018.01.018
Morimoto, K., Kim, S. J., Tanei, T., Shimazu, K., Tanji, Y., Taguchi, T., et al. (2009). Stem cell marker aldehyde dehydrogenase 1-positive breast cancers are characterized by negative estrogen receptor, positive human epidermal growth factor receptor type 2, and high Ki67 expression. Cancer Sci. 100, 1062–1068. doi:10.1111/j.1349-7006.2009.01151.x
Mortezaee, K., Ahmadi, A., Haghi-Aminjan, H., Khanlarkhani, N., Salehi, E., Nashtaei, M. S., et al. (2019). Thyroid function following breast cancer chemotherapy: a systematic review. J. Cell. Biochem. 120, 12101–12107. doi:10.1002/jcb.28771
Mukherjee, S., Mazumdar, M., Chakraborty, S., Manna, A., Saha, S., Khan, P., et al. (2014). Curcumin inhibits breast cancer stem cell migration by amplifying the E-cadherin/beta-catenin negative feedback loop. Stem Cell Res. Ther. 19. doi:10.1186/scrt506
Murillo, G., Peng, X. J., Torres, K. E. O., and Mehta, R. G. (2009). Deguelin inhibits growth of breast cancer cells by modulating the expression of key members of the wnt signaling pathway. Cancer Prev. Res. 2, 942–950. doi:10.1158/1940-6207.Capr-08-0232
Nam, H., Chung, B. C., Kim, Y., Lee, K., and Lee, D. (2009). Combining tissue transcriptomics and urine metabolomics for breast cancer biomarker identification. Bioinformatics 25, 3151–3157. doi:10.1093/bioinformatics/btp558
Ni, F., Fu, H., Feng, X. C., Chen, Y. T., Wang, J. Y., Wu, Y. Q., et al. (2022). Long non-coding RNAs play an important regulatory role in tumorigenesis and tumor progression through aerobic glycolysis. Front. Mol. Biosci. 9, 19. doi:10.3389/fmolb.2022.941653
Nishiya, N. (2017). Screening for chemical suppressors of the wnt/β-catenin signaling pathway. Yakugaku Zasshi-J. Pharm. Soc. Jpn. 137, 133–136. doi:10.1248/yakushi.16-00229-2
Nourmohammadi, S., Aung, T. N., Cui, J., Pei, J. X. V., De Ieso, M. L., Harata-Lee, Y., et al. (2019). Effect of compound kushen injection, a natural compound mixture, and its identified chemical components on migration and invasion of colon, brain, and breast cancer cell lines. Front. Oncol. 14, 314. doi:10.3389/fonc.2019.00314
O’Conor, C. J., Chen, T., Gonzalez, I., Cao, D. F., and Peng, Y. (2018). Cancer stem cells in triple-negative breast cancer: a potential target and prognostic marker. Biomark. Med. 12, 813–820. doi:10.2217/bmm-2017-0398
Oh, W. T., Yang, Y. S., Xie, J., Ma, H., Kim, J. M., Park, K. H., et al. (2023). WNT-modulating gene silencers as a gene therapy for osteoporosis, bone fracture, and critical-sized bone defects. Mol. Ther. 31, 435–453. doi:10.1016/j.ymthe.2022.09.018
Park, S. Y., Choi, J. H., and Nam, J. S. (2019). Targeting cancer stem cells in triple-negative breast cancer. Cancers 11, 965. doi:10.3390/cancers11070965
Park, W. C., and Jordan, V. C. (2002). Selective estrogen receptor modulators (SERMS) and their roles in breast cancer prevention. Trends Mol. Med. 8, 82–88. doi:10.1016/s1471-4914(02)02282-7
Patel, D. K., Kesharwani, R., Verma, A., Al-Abbasi, F. A., Anwar, F., and Kumar, V. (2023). Scope of Wnt signaling in the precise diagnosis and treatment of breast cancer. Drug Discov. Today 28, 103597. doi:10.1016/j.drudis.2023.103597
Pinson, K. I., Brennan, J., Monkley, S., Avery, B. J., and Skarnes, W. C. (2000). An LDL-receptor-related protein mediates Wnt signalling in mice. Nature 407, 535–538. doi:10.1038/35035124
Piva, M., Domenici, G., Iriondo, O., Rabano, M., Simoes, B. M., Comaills, V., et al. (2014). Sox2 promotes tamoxifen resistance in breast cancer cells. EMBO Mol. Med. 6, 66–79. doi:10.1002/emmm.201303411
Plummer, R., Dua, D., Cresti, N., Drew, Y., Stephens, P., Foegh, M., et al. (2020). First-in-human study of the PARP/tankyrase inhibitor E7449 in patients with advanced solid tumours and evaluation of a novel drug-response predictor. Br. J. Cancer 123, 525–533. doi:10.1038/s41416-020-0916-5
Proffitt, K. D., Madan, B., Ke, Z. Y., Pendharkar, V., Ding, L. J., Lee, M. A., et al. (2013). Pharmacological inhibition of the wnt acyltransferase PORCN prevents growth of WNT-driven mammary cancer. Cancer Res. 73, 502–507. doi:10.1158/0008-5472.Can-12-2258
Prossomariti, A., Piazzi, G., Alquati, C., and Ricciardiello, L. (2020). Are wnt/β-catenin and PI3K/AKT/mTORC1 distinct pathways in colorectal cancer? Cell. Mol. Gastroenterol. Hepatol. 10, 491–506. doi:10.1016/j.jcmgh.2020.04.007
Qayoom, H., Wani, N. A., Alshehri, B., and Mir, M. A. (2021). An insight into the cancer stem cell survival pathways involved in chemoresistance in triple-negative breast cancer. Future Oncol. 17, 4185–4206. doi:10.2217/fon-2021-0172
Qi, F. H., Zhao, L., Zhou, A. Y., Zhang, B., Li, A. Y., Wang, Z. X., et al. (2015). The advantages of using traditional Chinese medicine as an adjunctive therapy in the whole course of cancer treatment instead of only terminal stage of cancer. Biosci. Trends 9, 16–34. doi:10.5582/bst.2015.01019
Qi, W. W., Sun, M. J., Kong, X. N., Li, Y. M., Wang, X. L., Lv, S. G., et al. (2016). Huaier extract synergizes with tamoxifen to induce autophagy and apoptosis in ER-positive breast cancer cells. Oncotarget 7, 26003–26015. doi:10.18632/oncotarget.8303
Rafii, S., and Lyden, D. (2003). Therapeutic stem and progenitor cell transplantation for organ vascularization and regeneration. Nat. Med. 9, 702–712. doi:10.1038/nm0603-702
Ratre, P., Mishra, K., Dubey, A., Vyas, A., Jain, A., and Thareja, S. (2020). Aromatase inhibitors for the treatment of breast cancer: a journey from the scratch. Anti-Cancer Agents Med. Chem. 20, 1994–2004. doi:10.2174/1871520620666200627204105
Raut, D., Vora, A., and Bhatt, L. K. (2022). The Wnt/β-catenin pathway in breast cancer therapy: a pre-clinical perspective of its targeting for clinical translation. Expert Rev. Anticancer Ther. 22, 97–114. doi:10.1080/14737140.2022.2016398
Robertson, J. F. R., Bondarenko, I. M., Trishkina, E., Dvorkin, M., Panasci, L., Manikhas, A., et al. (2016). Fulvestrant 500 mg versus anastrozole 1 mg for hormone receptor-positive advanced breast cancer (FALCON): an international, randomised, double-blind, phase 3 trial. Lancet 388, 2997–3005. doi:10.1016/s0140-6736(16)32389-3
Rodenberg, J. M., and Brown, P. H. (2009). A novel look into estrogen receptor-negative breast cancer prevention with the natural, multifunctional signal transduction inhibitor Deguelin. Cancer Prev. Res. 2, 915–918. doi:10.1158/1940-6207.Capr-09-0208
Rodgers, S. J., Ooms, L. M., and Mitchell, C. A. (2023). The FDA-approved drug Pyrvinium selectively targets ER+ breast cancer cells with high INPP4B expression. Cancers 15, 135. doi:10.3390/cancers15010135
Saranya, T., Kavithaa, K., Paulpandi, M., Ramya, S., Preethi, S., Balachandar, V., et al. (2020). Enhanced apoptogenesis and oncogene regulatory mechanism of troxerutin in triple negative breast cancer cells. Toxicol. Res. 9, 230–238. doi:10.1093/toxres/tfaa029
Sartaj, A., Baboota, S., and Ali, J. (2021a). Exploring the therapeutic potential of nanostructured lipid carrier approaches to tackling the inherent lacuna of chemotherapeutics and herbal drugs against breast cancer. J. Drug Deliv. Sci. Technol. 63, 102451. doi:10.1016/j.jddst.2021.102451
Sartaj, A., Qamar, Z., Qizilbash, F. F., Md, S., Alhakamy, N. A., Baboota, S., et al. (2021b). Polymeric nanoparticles: exploring the current drug development and therapeutic insight of breast cancer treatment and recommendations. Polymers 13, 4400. doi:10.3390/polym13244400
Schambony, A., Kunz, M., and Gradl, D. (2004). Cross-regulation of Wnt signaling and cell adhesion. Differentiation 72, 307–318. doi:10.1111/j.1432-0436.2004.07207002.x
Schneeweiss, A., Ruckhaberle, E., and Huober, J. (2015). Chemotherapy for metastatic breast cancer - an anachronism in the era of personalised and targeted oncological therapy? Geburtshilfe Frauenheilkd 75, 574–583. doi:10.1055/s-0035-1546150
Shah, K., Panchal, S., and Patel, B. (2021). Porcupine inhibitors: novel and emerging anti-cancer therapeutics targeting the Wnt signaling pathway. Pharmacol. Res. 167, 105532. doi:10.1016/j.phrs.2021.105532
Shao, H. M., Ma, J. H., Guo, T. H., and Hu, R. R. (2014). Triptolide induces apoptosis of breast cancer cells via a mechanism associated with the Wnt/β-catenin signaling pathway. Exp. Ther. Med. 8, 505–508. doi:10.3892/etm.2014.1729
Shapiro, C. L. (2021). Bone-modifying agents (BMAs) in breast cancer. Clin. Breast Cancer 21, E618–E630. doi:10.1016/j.clbc.2021.04.009
Sheridan, C., Kishimoto, H., Fuchs, R. K., Mehrotra, S., Bhat-Nakshatri, P., Turner, C. H., et al. (2006). CD44+/CD24- breast cancer cells exhibit enhanced invasive properties:: an early step necessary for metastasis. Breast Cancer Res. 8, R59. doi:10.1186/bcr1610
Shi, H., Wang, Y. G., Yao, M. L., Zhang, D., Fang, W. L., Zhou, T., et al. (2020). Honokiol inhibits the growth of SKBR3 cells. Transl. Cancer Res. 9, 7596–7604. doi:10.21037/tcr-20-3110
Shrivastava, S., Jeengar, M. K., Thummuri, D., Koval, A., Katanaev, V. L., Marepally, S., et al. (2017). Cardamonin, a chalcone, inhibits human triple negative breast cancer cell invasiveness by downregulation of Wnt/β-catenin signaling cascades and reversal of epithelial-mesenchymal transition. Biofactors 43, 152–169. doi:10.1002/biof.1315
Slamon, D. J., Neven, P., Chia, S., Fasching, P. A., De Laurentiis, M., Im, S. A., et al. (2020). Overall survival with ribociclib plus fulvestrant in advanced breast cancer. N. Engl. J. Med. 382, 514–524. doi:10.1056/NEJMoa1911149
Solzak, J. P., Atale, R. V., Hancock, B. A., Sinn, A. L., Pollok, K. E., Jones, D. R., et al. (2017). Dual PI3K and Wnt pathway inhibition is a synergistic combination against triple negative breast cancer. npj Breast Cancer 8, 17. doi:10.1038/s41523-017-0016-8
Su, L., Jiang, Y. M., Xu, Y., Li, X. Y., Gao, W. B., Xu, C. W., et al. (2018). Xihuang pill promotes apoptosis of Treg cells in the tumor microenvironment in 4T1 mouse breast cancer by upregulating MEKK1/SEK1/JNK1/AP-1 pathway. Biomed. Pharmacother. 102, 1111–1119. doi:10.1016/j.biopha.2018.03.063
Sun, X. Y., Wang, M. Z., Wang, M. S., Yu, X. T., Guo, J. Y., Sun, T., et al. (2020). Metabolic reprogramming in triple-negative breast cancer. Front. Oncol. 10, 428. doi:10.3389/fonc.2020.00428
Sun, Y. F., Gao, X. Y., Wu, P. P., Wink, M., Li, J. H., Dian, L. L., et al. (2019). Jatrorrhizine inhibits mammary carcinoma cells by targeting TNIK mediated Wnt/beta-catenin signalling and epithelial-mesenchymal transition (EMT). Phytomedicine, 13. doi:10.1016/j.phymed.2019.153015
Tamai, K., Semenov, M., Kato, Y., Spokony, R., Liu, C. M., Katsuyama, Y., et al. (2000). LDL-receptor-related proteins in Wnt signal transduction. Nature 407, 530–535. doi:10.1038/35035117
Tan, B., Zhang, Y., Zhang, T., He, J., Luo, X., Bian, X., et al. (2020). Identifying potential serum biomarkers of breast cancer through targeted free fatty acid profiles screening based on a GC-MS platform. Biomed. Chromatogr. 34, e4922. doi:10.1002/bmc.4922
Tang, D. M., He, Y. Z., Li, W. Y., and Li, H. W. (2019). Wnt/beta-catenin interacts with the FGF pathway to promote proliferation and regenerative cell proliferation in the zebrafish lateral line neuromast. Exp. Mol. Med., 16. doi:10.1038/s12276-019-0247-x
Tian, J., He, H. B., and Lei, G. H. (2014). Wnt/β-catenin pathway in bone cancers. Tumor Biol. 35, 9439–9445. doi:10.1007/s13277-014-2433-8
Tian, S., Song, X. T., Wang, Y., Wang, X. Y., Mou, Y., Chen, Q., et al. (2020). Chinese herbal medicine Baoyuan Jiedu decoction inhibits the accumulation of myeloid derived suppressor cells in pre-metastatic niche of lung via TGF-beta/CCL9 pathway. Biomed. Pharmacother., 9. doi:10.1016/j.biopha.2020.110380
Trautmann, F., Cojoc, M., Kurth, I., Melin, N., Bouchez, L. C., Dubrovska, A., et al. (2014). CXCR4 as biomarker for radioresistant cancer stem cells. Int. J. Radiat. Biol. 90, 687–699. doi:10.3109/09553002.2014.906766
Turashvili, G., and Brogi, E. (2017). Tumor heterogeneity in breast cancer. Front. Med. 4, 227. doi:10.3389/fmed.2017.00227
Tuttle, A. M., Hoffman, T. L., and Schilling, T. F. (2014). Rabconnectin-3a regulates vesicle endocytosis and canonical wnt signaling in zebrafish neural crest migration. PLoS. Biol. 12, e1001852. doi:10.1371/journal.pbio.1001852
Vaillant, F., Asselin-Labat, M. L., Shackleton, M., Forrest, N. C., Lindeman, G. J., and Visvader, J. E. (2008). The mammary progenitor marker CD61/beta3 integrin identifies cancer stem cells in mouse models of mammary tumorigenesis. Cancer Res. 68, 7711–7717. doi:10.1158/0008-5472.Can-08-1949
Vermeulen, L., Sprick, M. R., Kemper, K., Stassi, G., and Medema, J. P. (2008). Cancer stem cells - old concepts, new insights. Cell Death Differ. 15, 947–958. doi:10.1038/cdd.2008.20
von Voithenberg, L. V., Crocetti, E., Martos, C., Dimitrova, N., Giusti, F., Randi, G., et al. (2019). Cancer registries-guardians of breast cancer biomarker information: a systematic review. Int. J. Biol. Markers 34, 194–199. doi:10.1177/1724600819836097
Wan, M. Q., Huang, L., Liu, J. P., Liu, F. S., Chen, G. L., Ni, H. W., et al. (2021). Cyclosporine A induces cardiac developmental toxicity in zebrafish by up-regulation of wnt signaling and oxidative stress. Front. Pharmacol. 12, 747991. doi:10.3389/fphar.2021.747991
Wang, J. X., Qi, H., Zhang, X. L., Si, W., Xu, F. F., Hou, T., et al. (2018a). Saikosaponin D from Radix Bupleuri suppresses triple-negative breast cancer cell growth by targeting β-catenin signaling. Biomed. Pharmacother. 108, 724–733. doi:10.1016/j.biopha.2018.09.038
Wang, N., Zheng, Y. F., Gu, J. Y., Cai, Y. L., Wang, S. Q., Zhang, F. X., et al. (2017). Network-pharmacology-based validation of TAMS/CXCL-1 as key mediator of XIAOPI formula preventing breast cancer development and metastasis. Sci. Rep. 7, 14513. doi:10.1038/s41598-017-15030-3
Wang, R., Yang, L. X., Li, S., Ye, D. M., Yang, L. H., Liu, Q. Y., et al. (2018c). Quercetin inhibits breast cancer stem cells via downregulation of aldehyde dehydrogenase 1A1 (ALDH1A1), chemokine receptor type 4 (CXCR4), mucin 1 (MUC1), and epithelial cell adhesion molecule (EpCAM). Med. Sci. Monit. 24, 412–420. doi:10.12659/msm.908022
Wang, S. Q., Wang, N., Huang, X. W., Yang, B. W., Zheng, Y. F., Zhang, J. P., et al. (2020a). Baohuoside i suppresses breast cancer metastasis by downregulating the tumor-associated macrophages/C-X-C motif chemokine ligand 1 pathway. Phytomedicine 78, 153331. doi:10.1016/j.phymed.2020.153331
Wang, S. Y., Yang, S. Y., Yang, X. J., Deng, D., Li, J., and Dong, M. Q. (2023a). Research progress of traditional Chinese medicine monomers in reversing multidrug resistance of breast cancer. Am. J. Chin. Med. 51, 575–594. doi:10.1142/s0192415x23500283
Wang, T., Xu, Y., Chen, Q., Zheng, W., Wang, J., Zeng, H., et al. (2020b). Metabolomics analysis of laparoscopic surgery combined with Wuda granule to promote rapid recovery of patients with colorectal cancer using UPLC/Q-TOF-MS/MS. Evid.-based Complement. Altern. Med. 2020, 5068268. doi:10.1155/2020/5068268
Wang, X., Jung, Y. S., Jun, S., Lee, S., Wang, W. Q., Schneider, A., et al. (2016). PAF-Wnt signaling-induced cell plasticity is required for maintenance of breast cancer cell stemness. Nat. Commun. 13, 10633. doi:10.1038/ncomms10633
Wang, X. C., Jia, J., Gu, X. H., Zhao, W. W., Chen, C. P., Wu, W. X., et al. (2021b). Screening of breast cancer methylation biomarkers based on the TCGA database. Int. J. Gen. Med. 14, 9833–9839. doi:10.2147/ijgm.S322857
Wang, X. H., Xu, C. F., Hua, Y. T., Cheng, K., Zhang, Y. Z., Liu, J., et al. (2018b). Psoralen induced cell cycle arrest by modulating Wnt/β-catenin pathway in breast cancer cells. Sci. Rep. 8, 14001. doi:10.1038/s41598-018-32438-7
Wang, X. H., Xu, C. F., Hua, Y. T., Cheng, K., Zhang, Y. Z., Liu, J., et al. (2018d). Psoralen induced cell cycle arrest by modulating Wnt/beta-catenin pathway in breast cancer cells. Sci. Rep., 7. doi:10.1038/s41598-018-32438-7
Wang, X. Y., Tang, G. H., Guo, H., Ma, J. J., Liu, D. M., Wang, Y. W., et al. (2023b). Research progress on the anti-tumor mechanism and reversal of multidrug resistance of zuojin pill and its main components, evodiamine and berberine. Nat. Prod. Commun. 18, 1934578X2311614. doi:10.1177/1934578x231161414
Wang, Y., Bi, C., Pang, W., Liu, Y., Yuan, Y., Zhao, H., et al. (2019). Plasma metabolic profiling analysis of Gout party on acute Gout arthritis rats based on UHPLC-Q-TOF/MS combined with multivariate statistical analysis. Int. J. Mol. Sci. 20, 5753. doi:10.3390/ijms20225753
Wang, Y., Zhou, W., Lyu, C., Li, Q., Kou, F., Jiang, M., et al. (2021a). Metabolomics study on the intervention effect of Radix Salviae Miltiorrhizae extract in exercise-induced exhaustion rat using gas chromatography coupled to mass spectrometry. J. Chromatogr. B-Analytical Technol. Biomed. Life Sci. 1178, 122805. doi:10.1016/j.jchromb.2021.122805
Wehrli, M., Dougan, S. T., Caldwell, K., O'Keefe, L., Schwartz, S., Vaizel-Ohayon, D., et al. (2000). Arrow encodes an LDL-receptor-related protein essential for Wingless signalling. Nature 407, 527–530. doi:10.1038/35035110
Wend, P., Runke, S., Wend, K., Anchondo, B., Yesayan, M., Jardon, M., et al. (2013). WNT10B/β-catenin signalling induces HMGA2 and proliferation in metastatic triple-negative breast cancer. EMBO Mol. Med. 5, 264–279. doi:10.1002/emmm.201201320
Winardi, D., Wu, C. H., Chiang, J. H., Chen, Y. H., Hsieh, C. L., Yang, J. C., et al. (2023). The use of san-huang-xie-xin-tang reduces the mortality rate among breast cancer patients. Cancers 15, 1213. doi:10.3390/cancers15041213
Wu, C. H., Hong, B. H., Ho, C. T., and Yen, G. C. (2015). Targeting cancer stem cells in breast cancer: potential anticancer properties of 6-shogaol and pterostilbene. J. Agric. Food Chem. 63, 2432–2441. doi:10.1021/acs.jafc.5b00002
Wu, C. T., Tsai, Y. T., Lin, J. G., Fu, S. L., and Lai, J. N. (2018a). Chinese herbal products and the reduction of risk of breast cancer among females with type 2 diabetes in Taiwan a case-control study. Med. Baltim. 97, e11600. doi:10.1097/md.0000000000011600
Wu, D., Jia, H. Y., Zhang, Z. R., and Li, S. J. (2020a). Capsaicin suppresses breast cancer cell viability by regulating the CDK8/PI3K/Akt/Wnt/β‑catenin signaling pathway. Mol. Med. Rep. 22, 4868–4876. doi:10.3892/mmr.2020.11585
Wu, H. J., and Chu, P. Y. (2021). Recent discoveries of macromolecule- and cell-based biomarkers and therapeutic implications in breast cancer. Int. J. Mol. Sci. 47, 636. doi:10.3390/ijms22020636
Wu, H. T., Lin, J., Liu, Y. E., Chen, H. F., Hsu, K. W., Lin, S. H., et al. (2021a). Luteolin suppresses androgen receptor-positive triple-negative breast cancer cell proliferation and metastasis by epigenetic regulation of MMP9 expression via the AKT/mTOR signaling pathway. Phytomedicine 81, 153437. doi:10.1016/j.phymed.2020.153437
Wu, H. T., Liu, Y. E., Hsu, K. W., Wang, Y. F., Chan, Y. C., Chen, Y., et al. (2020c). MLL3 induced by luteolin causes apoptosis in tamoxifen-resistant breast cancer cells through H3K4 monomethylation and suppression of the PI3K/AKT/mTOR pathway. Am. J. Chin. Med. 48, 1221–1241. doi:10.1142/s0192415x20500603
Wu, T., Duan, X., Hu, T. H., Mu, X. X., Jiang, G., and Cui, S. (2020b). Effect of endostatin on Wnt pathway of stem-like cells in bladder cancer in tumor microenvironment. Mol. Biol. Rep. 47, 3937–3948. doi:10.1007/s11033-020-05487-3
Wu, X., Zhang, H., Fan, S., Zhang, Y., Yang, Z., Fan, S., et al. (2018b). Quality markers based on biological activity: a new strategy for the quality control of traditional Chinese medicine. Phytomedicine 44, 103–108. doi:10.1016/j.phymed.2018.01.016
Wu, Y., Cheng, C. S., Li, Q., Chen, J. X., Lv, L. L., Xu, J. Y., et al. (2021b). The application of Citrus folium in breast cancer and the mechanism of its main component Nobiletin: a systematic review. Evid.-based Complement. Altern. Med. 2021, 2847466. doi:10.1155/2021/2847466
Xiao, X., Ao, M., Xu, F., Li, X., Hu, J. L., Wang, Y., et al. (2018). Effect of matrine against breast cancer by downregulating the vascular endothelial growth factor via the Wnt/β-catenin pathway. Oncol. Lett. 15, 1691–1697. doi:10.3892/ol.2017.7519
Xu, T., Jiang, Y. Y., Yuan, S. Y., Zhang, L., Chen, X. H., Zhao, W. L., et al. (2022). Andrographolide inhibits ER-positive breast cancer growth and enhances fulvestrant efficacy via ROS-FOXM1-ER-a Axis. Front. Oncol. 12, 899402. doi:10.3389/fonc.2022.899402
Xu, W., Lin, H., Zhang, Y., Chen, X., Hua, B., Hou, W., et al. (2011). Compound Kushen Injection suppresses human breast cancer stem-like cells by down-regulating the canonical Wnt/β-catenin pathway. J. Exp. Clin. Cancer Res. 30, 103. doi:10.1186/1756-9966-30-103
Xu, X. F., Zhang, M. F., Xu, F. Y., and Jiang, S. J. (2020). Wnt signaling in breast cancer: biological mechanisms, challenges and opportunities. Mol. Cancer 19, 165. doi:10.1186/s12943-020-01276-5
Xu, X. H., Rajamanicham, V., Xu, S. J., Liu, Z. D., Yan, T., Liang, G., et al. (2019). Schisandrin A inhibits triple negative breast cancer cells by regulating Wnt/ER stress signaling pathway. Biomed. Pharmacother. 115, 108922. doi:10.1016/j.biopha.2019.108922
Xue, W., Hao, J., Zhang, Q. P., Jin, R. H., Luo, Z., Yang, X., et al. (2023). Chlorogenic acid inhibits epithelial-mesenchymal transition and invasion of breast cancer by down-regulating LRP6. J. Pharmacol. Exp. Ther. 384, 254–264. doi:10.1124/jpet.122.001189
Yang, C. Q., Liu, J., Zhao, S. Q., Zhu, K., Gong, Z. Q., Xu, R., et al. (2020b). Recent treatment progress of triple negative breast cancer. Prog. Biophys. Mol. Biol. 151, 40–53. doi:10.1016/j.pbiomolbio.2019.11.007
Yang, L., Song, Z. C., Wang, X. L., Yang, W., Wang, M. Q., and Liu, H. (2017a). Huaier extract enhances the treatment efficacy of paclitaxel in breast cancer cells via the NF-κB/IκBα pathway. Oncol. Rep. 38, 3455–3464. doi:10.3892/or.2017.6024
Yang, S., Sun, S. Q., Xu, W. Q., Yu, B. X., Wang, G. M., and Wang, H. B. (2020a). Astragalus polysaccharide inhibits breast cancer cell migration and invasion by regulating epithelial‑mesenchymal transition via the Wnt/β‑catenin signaling pathway. Mol. Med. Rep. 21, 1819–1832. doi:10.3892/mmr.2020.10983
Yang, W., Ma, L., Li, S., Cui, K., Lei, L., and Ye, Z. (2017b). Evaluation of the cardiotoxicity of evodiamine in vitro and in vivo. Molecules 22, 943. doi:10.3390/molecules22060943
Yang, X. S., Luo, G. Y., Fu, L., Huang, H., Wang, L. F., Yin, L. H., et al. (2022). Intervention mechanism of hunag-lian jie-du decoction on canonical wnt/beta-catenin signaling pathway in psoriasis mouse model. Evid.-based Complement. Altern. Med., 11. doi:10.1155/2022/3193572
Yang, Y. G., Hao, E. W., Pan, X. L., Tan, D. C., Du, Z. C., Xie, J. L., et al. (2019). Gomisin M2 from Baizuan suppresses breast cancer stem cell proliferation in a zebrafish xenograft model. Aging-US 11, 8347–8361. doi:10.18632/aging.102323
Yang, Z. M., Zhang, Q. H., Yu, L. H., Zhu, J. Y., Cao, Y., and Gao, X. F. (2021). The signaling pathways and targets of traditional Chinese medicine and natural medicine in triple-negative breast cancer. J. Ethnopharmacol. 264, 113249. doi:10.1016/j.jep.2020.113249
Yao, H., He, G. C., Yan, S. C., Chen, C., Song, L. J., Rosol, T. J., et al. (2017). Triple-negative breast cancer: is there a treatment on the horizon? Oncotarget 8, 1913–1924. doi:10.18632/oncotarget.12284
Ye, F., Zhon, X. R., Qiu, Y., Yang, L. B., Wei, B., Zhang, Z., et al. (2017). CD49f can act as a biomarker for local or distant recurrence in breast cancer. J. Breast Canc 20, 142–149. doi:10.4048/jbc.2017.20.2.142
Ye, Y., Zhang, B., Liang, Q. Y., Wang, D. D., Bai, F. C., Li, Y. H., et al. (2022). Exploring the pharmacological mechanism of compound kushen injection in the treatment of breast cancer using in vitro experiments: coupling network pharmacology with GEO database. Front. Oncol. 12, 946758. doi:10.3389/fonc.2022.946758
Ye, Y. Y., Pei, L. X., Wu, C. Y., and Liu, S. (2019). Protective effect of traditional Chinese medicine formula RP on lung microenvironment in pre-metastasis stage of breast cancer. Integr. Cancer Ther. 18, 1534735419876341. doi:10.1177/1534735419876341
Yu, K. D., Ye, F. G., He, M., Fan, L., Ma, D., Mo, M., et al. (2020). Effect of adjuvant paclitaxel and carboplatin on survival in women with triple-negative breast cancer A phase 3 randomized clinical trial. JAMA Oncol. 6, 1390–1396. doi:10.1001/jamaoncol.2020.2965
Yu, P., Li, J. Y., Luo, Y. Q., Sun, J. Y., Hu, Y. F., Lin, B., et al. (2023b). Mechanistic role of scutellaria baicalensis georgi in breast cancer therapy. Am. J. Chin. Med. 51, 279–308. doi:10.1142/s0192415x23500155
Yu, S. B., Wang, Z. Y., Su, Z. J., Song, J. X., Zhou, L., Sun, Q., et al. (2018). Gigantol inhibits Wnt/beta-catenin signaling and exhibits anticancer activity in breast cancer cells. BMC Complement. Altern. Med., 8. doi:10.1186/s12906-018-2108-x
Yu, S. W., Zheng, J. L., Zhang, Y., Meng, D. D., Wang, Y. J., Xu, X. Y., et al. (2023a). The mechanisms of multidrug resistance of breast cancer and research progress on related reversal agents. Bioorg. Med. Chem. 95, 117486. doi:10.1016/j.bmc.2023.117486
Zeng, L. T., and Yang, K. L. (2017). Exploring the pharmacological mechanism of Yanghe Decoction on HER2-positive breast cancer by a network pharmacology approach. J. Ethnopharmacol. 199, 68–85. doi:10.1016/j.jep.2017.01.045
Zhang, N., Kong, X. L., Yan, S., Yuan, C. Z., and Yang, Q. F. (2010). Huaier aqueous extract inhibits proliferation of breast cancer cells by inducing apoptosis. Cancer Sci. 101, 2375–2383. doi:10.1111/j.1349-7006.2010.01680.x
Zhang, X. P., Li, M. Q., Zuo, K. Q., Li, D., Ye, M., Ding, L. B., et al. (2013). Upregulated miR-155 in papillary thyroid carcinoma promotes tumor growth by targeting APC and activating Wnt/β-catenin signaling. J. Clin. Endocrinol. Metab. 98, E1305–E1313. doi:10.1210/jc.2012-3602
Zhang, X. Q., Yao, C., Bian, W. H., Chen, X., Xue, J. X., Zhu, Z. Y., et al. (2019a). Effects of Astragaloside IV on treatment of breast cancer cells execute possibly through regulation of Nrf2 via PI3K/AKT/mTOR signaling pathway. Food Sci. Nutr. 7, 3403–3413. doi:10.1002/fsn3.1154
Zhang, Y. (2017). Ganoderma lucidum (Reishi) suppresses proliferation and migration of breast cancer cells via inhibiting Wnt/β-catenin signaling. Biochem. Biophys. Res. Commun. 488, 679–684. doi:10.1016/j.bbrc.2017.04.086
Zhang, Y., Guo, L., Li, Y. H., Feng, G. H., Teng, F., Li, W., et al. (2018). MicroRNA-494 promotes cancer progression and targets adenomatous polyposis coli in colorectal cancer. Mol. Cancer 17, 11. doi:10.1186/s12943-017-0753-1
Zhang, Y., Lv, X., Liu, R., Zhang, M., Liu, H., Gao, H., et al. (2019b). An integrated strategy for ascertaining quality marker of Schisandra chinensis (Turcz.) Baill based on correlation analysis between depression-related monoaminergic metabolites and chemical components profiling. J. Chromatogr. A 1598, 122–131. doi:10.1016/j.chroma.2019.03.056
Zhang, Y., Pizzute, T., and Pei, M. (2014). A review of crosstalk between MAPK and Wnt signals and its impact on cartilage regeneration. Cell Tissue Res. 358, 633–649. doi:10.1007/s00441-014-2010-x
Zhang, Y. B., Li, H. J., Zhang, J., Zhao, C. R., Lu, S., Qiao, J. P., et al. (2020). The combinatory effects of natural products and chemotherapy drugs and their mechanisms in breast cancer treatment. Phytochem. Rev. 19, 1179–1197. doi:10.1007/s11101-019-09628-w
Zhang, Y. Q., Liu, M. M., Li, H. S., Chen, Z. T., Liang, N., Xu, J. G., et al. (2017). Traditional Chinese medicine Bushen-Jianpi-Huoxue decoction prevents diabetic osteoporosis in rats via Wnt and nuclear factor-kappa B signaling pathways. Int. J. Rheum. Dis. 20, 941–948. doi:10.1111/1756-185x.13050
Zhao, H., Han, B., Li, X., Sun, C. T., Zhai, Y. F., Li, M., et al. (2022a). Salvia miltiorrhiza in breast cancer treatment: a review of its phytochemistry, derivatives, nanoparticles, and potential mechanisms. Front. Pharmacol. 16, 872085. doi:10.3389/fphar.2022.872085
Zhao, M. H., Pan, B. T., He, Y. J., Niu, B., and Gao, X. A. (2022b). Elucidating the pharmacological mechanism by which Si-Wu-Tang induces cellular senescence in breast cancer via multilevel data integration. Aging-US 14, 5812–5837. doi:10.18632/aging.204185
Zhao, S., and Wang, Y. (2023). Effects of jatrorrhizine on proliferation, apoptosis and invasion of breast cancer cells by regulating wnt/beta-catenin signaling pathway. Indian J. Pharm. Sci. 85, 31–36. doi:10.36468/pharmaceutical-sciences.1065
Zhao, W. J., Liu, J. H., Li, Y. Q., Chen, Z. C., Qi, D. M., and Zhang, Z. (2021). Immune effect of active components of traditional Chinese medicine on triple-negative breast cancer. Front. Pharmacol. 9, 731741. doi:10.3389/fphar.2021.731741
Zhao, X., Liu, J. Y., Feng, L. L., Ge, S. S., Yang, S. J., Chen, C., et al. (2018). Anti-angiogenic effects of Qingdu granule on breast cancer through inhibiting NFAT signaling pathway. J. Ethnopharmacol. 222, 261–269. doi:10.1016/j.jep.2018.01.013
Zheng, L. X., Zheng, Q., Yu, Z. P., Wang, J., Ren, X. Y., Gong, Y., et al. (2019b). Liuwei Dihuang pill suppresses metastasis by regulating the wnt pathway and disrupting beta-catenin/T cell factor interactions in a murine model of triple-negative breast cancer. J. Tradit. Chin. Med. 39, 826–832.
Zheng, Y. F., Liu, P. X., Wang, N., Wang, S. Q., Yang, B. W., Li, M., et al. (2019a). Betulinic acid suppresses breast cancer metastasis by targeting GRP78-mediated glycolysis and ER stress apoptotic pathway. Oxidative Med. Cell. Longev. 2019, 8781690. doi:10.1155/2019/8781690
Zheng, Y. F., Wang, N., Wang, S. Q., Yang, B. W., Situ, H. L., Zhong, L. D., et al. (2020). XIAOPI formula inhibits the pre-metastatic niche formation in breast cancer via suppressing TAMs/CXCL1 signaling. Cell Commun. Signal. 18, 48. doi:10.1186/s12964-020-0520-6
Zheng, Y. F., Zhang, J. P., Huang, W. Q., Zhong, L. L. D., Wang, N., Wang, S. Q., et al. (2021). Sini san inhibits chronic psychological stress-induced breast cancer stemness by suppressing cortisol-mediated GRP78 activation. Front. Pharmacol. 12, 714163. doi:10.3389/fphar.2021.714163
Zhong, Y., Katavolos, P., Nguyen, T., Lau, T., Boggs, J., Sambrone, A., et al. (2016). Tankyrase inhibition causes reversible intestinal toxicity in mice with a therapeutic index < 1. Toxicol. Pathol. 44, 267–278. doi:10.1177/0192623315621192
Zhou, B., Kwan, B., Desai, M. J., Nalawade, V., Ruddy, K. J., Nathan, P. C., et al. (2022). Long-term antimüllerian hormone patterns differ by cancer treatment exposures in young breast cancer survivors. Fertil. Steril. 117, 1047–1056. doi:10.1016/j.fertnstert.2022.01.016
Zhou, H., Zhang, P., Hou, Z., Xie, J., Wang, Y., Yang, B., et al. (2016). Research on the relationships between endogenous biomarkers and exogenous toxic substances of acute toxicity in radix Aconiti. Molecules 21, 1623. doi:10.3390/molecules21121623
Zhou, J., Zhang, X. M., Li, M., Wu, W. Q., Sun, X., Zhang, L., et al. (2013). Novel lipid hybrid albumin nanoparticle greatly lowered toxicity of pirarubicin. Mol. Pharm. 10, 3832–3841. doi:10.1021/mp400303w
Zhou, J. J., Chen, Q. S., Zou, Y. H., Chen, H. H., Qi, L. N., and Chen, Y. D. (2019). Stem cells and cellular origins of breast cancer: updates in the rationale, controversies, and therapeutic implications. Front. Oncol. 9, 820. doi:10.3389/fonc.2019.00820
Zhou, N., Han, S. Y., Chen, Y. Z., Zhou, F., Zheng, W. X., and Li, P. P. (2018). Shugan liangxue decoction down-regulates estrogen receptor α expression in breast cancer cells. Chin. J. Integr. Med. 24, 518–524. doi:10.1007/s11655-015-2123-4
Zhou, N., Han, S. Y., Zhou, F., and Li, P. P. (2014). Anti-tumor effect of Shu-gan-Liang-Xue decoction in breast cancer is related to the inhibition of aromatase and steroid sulfatase expression. J. Ethnopharmacol. 154, 687–695. doi:10.1016/j.jep.2014.04.045
Zhou, Q. M., Sun, Y., Lu, Y. Y., Zhang, H., Chen, Q. L., and Su, S. B. (2017). Curcumin reduces mitomycin C resistance in breast cancer stem cells by regulating Bcl-2 family-mediated apoptosis. Cancer Cell Int. 17, 84. doi:10.1186/s12935-017-0453-3
Zhu, G., and Feng, F. (2019). UPLC-MS-based metabonomic analysis of intervention effects of Da-Huang-Xiao-Shi decoction on ANIT-induced cholestasis. J. Ethnopharmacol. 238, 111860. doi:10.1016/j.jep.2019.111860
Zhu, H. N., You, J., Wen, Y., Jia, L., Gao, F., Ganesan, K., et al. (2021). Tumorigenic risk of Angelica sinensis on ER-positive breast cancer growth through ER-induced stemness in vitro and in vivo. J. Ethnopharmacol. 280, 114415. doi:10.1016/j.jep.2021.114415
Zhu, X., Wang, K., and Chen, Y. (2020). Ophiopogonin D suppresses TGF-beta 1-mediated metastatic behavior of MDA-MB-231 breast carcinoma cells via regulating ITGB1/FAK/Src/AKT/beta-catenin/MMP-9 signaling axis. Toxicol. Vitro, 9. doi:10.1016/j.tiv.2020.104973
Keywords: breast cancer, Wnt/β-catenin signaling pathway, traditional Chinese medicine, chemotherapy drugs, therapeutic approach
Citation: Li H, Li J, Zhang Y, Zhao C, Ge J, Sun Y, Fu H and Li Y (2024) The therapeutic effect of traditional Chinese medicine on breast cancer through modulation of the Wnt/β-catenin signaling pathway. Front. Pharmacol. 15:1401979. doi: 10.3389/fphar.2024.1401979
Received: 16 March 2024; Accepted: 19 April 2024;
Published: 09 May 2024.
Edited by:
Ruiwen Zhang, University of Houston, United StatesReviewed by:
Nitin Telang, Consultant, Montvale, NJ, United StatesRun-Lan Wan, The Affiliated Hospital of Southwest Medical University, China
Maoyuan Zhao, Chengdu University of Traditional Chinese Medicine, China
Copyright © 2024 Li, Li, Zhang, Zhao, Ge, Sun, Fu and Li. This is an open-access article distributed under the terms of the Creative Commons Attribution License (CC BY). The use, distribution or reproduction in other forums is permitted, provided the original author(s) and the copyright owner(s) are credited and that the original publication in this journal is cited, in accordance with accepted academic practice. No use, distribution or reproduction is permitted which does not comply with these terms.
*Correspondence: Hui Fu, ZnVoMDA1MEB0anV0Y20uZWR1LmNu; Yingpeng Li, bGl5aW5ncGVuZ0B0anV0Y20uZWR1LmNu
†These authors have contributed equally to this work